- Federal Research and Clinical Center of Physical-Chemical Medicine of Federal Medical Biological Agency, Moscow, Russia
G-quadruplexes (G4s) are non-canonical DNA structures that could be considered as potential therapeutic targets for antimicrobial compounds, also known as G4-stabilizing ligands. While some of these ligands are shown in vitro to have a stabilizing effect, the precise mechanism of antibacterial action has not been fully investigated. Here, we employed genome-wide RNA-sequencing to analyze the response of Mycobacterium smegmatis to inhibitory concentrations of BRACO-19 and TMPyP4 G4 ligands. The expression profile changed (FDR < 0.05, log2FC > |1|) for 822 (515↑; 307↓) genes in M. smegmatis in response to BRACO-19 and for 680 (339↑; 341↓) genes in response to TMPyP4. However, the analysis revealed no significant ligand-induced changes in the expression levels of G4-harboring genes, genes under G4-harboring promoters, or intergenic regions located on mRNA-like or template strands. Meanwhile, for the BRACO-19 ligand, we found significant changes in the replication and repair system genes, as well as in iron metabolism genes which is, undoubtedly, evidence of the induced stress. For the TMPyP4 compound, substantial changes were found in transcription factors and the arginine biosynthesis system, which may indicate multiple biological targets for this compound.
Introduction
The emergence and rapid dissemination of drug-resistant bacterial pathogens are becoming a global health challenge. In 2019, an estimated 10 million people worldwide developed tuberculosis (TB), caused by Mycobacterium tuberculosis complex bacteria. In total, nearly half a million people have developed rifampicin-resistant TB (RR-TB), 78% of whom had multidrug-resistant TB (MDR-TB; resistance to both first-line drugs rifampin and isoniazid). The global treatment success rate for MDR/RR-TB was estimated at 57%, compared to an overall success rate of 85% for all cases. Moreover, the treatment of people with resistant TB forms takes longer and requires more expensive and toxic drugs (Seung et al., 2015; Global Tuberculosis Report 2020, 2020). All of these factors encourage the development of new anti-TB agents with new mechanisms of action.
One of the recently emerged trends in the design of antimicrobial drugs suggests targeting quadruplex structures (G4s) in guanine-rich regions of the bacterial genome (Yadav et al., 2021). Such structures comprise planar arrangements of guanine tetrads held together by Hoogsteen base pairing and π–π interactions (Spiegel et al., 2020). G4s have been found in eukaryotes, viruses, and multiple bacterial species (Holder and Hartig, 2014; Ding et al., 2018; Bartas et al., 2019; Marsico et al., 2019), including M. tuberculosis (Perrone et al., 2017). Their conservation and non-random genomic distribution (Bartas et al., 2019) are likely indicative of important biological functions. In bacteria, G4s are often found in virulence-related genes (Harris and Merrick, 2015) or their promoter regions and provide a control mechanism involved in the regulation of biological pathways such as transcription and translation (Holder and Hartig, 2014; Wu et al., 2015; Shao et al., 2020). Due to the activity of specific helicases, genomic G4s do not normally persist in cells (Sauer and Paeschke, 2017; Saha et al., 2019). Some G4-resolving helicases (e.g., the PIF1 family) are conserved from bacteria to humans, highlighting the importance of efficient G4 processing (Byrd and Raney, 2017). Unresolved G4s may induce DNA breaks and damage response, recruit transcription factors, or mechanically hamper polymerase passage, affecting transcription and replication (Saranathan and Vivekanandan, 2019).
All the foregoing advocates the use of G4-stabilizing ligands to repress or fine-tune gene expression. An extensive effort is being devoted to designing new small-molecule G4 binders and elucidating the basis for antimicrobial properties of the known ones (Yadav et al., 2021). However, despite recent advances in controlling bacterial growth with exogenous ligands, the underlying mechanisms are poorly understood. Recently, it has been reported that two known G4 ligands, BRACO-19 and c-exNDI2, exhibit anti-TB activity (Perrone et al., 2017); besides, their possible genomic targets have been identified using general sequence rules and G4 prediction algorithms of relatively low stringency (Beaudoin et al., 2014). Subsequently, the stabilizing activity of TMPyP4 ligand for virulence-related G4 targets has been reported (Mishra et al., 2019). These reports encourage the future development of G4-affecting agents. However, additional studies are needed to verify the role of G4s and determine the affected metabolic pathways.
In the present study, we performed the transcriptomic analysis of the Mycobacterium smegmatis MC2155 strain in the presence of an inhibitory concentration of two G4 ligands, namely BRACO-19 and TMPyP4. Given the known ability of G4 ligands to stabilize G4s, we have identified putative G4 quadruplex sequences in M. smegmatis genome and analyzed G4-associated genes during the transcriptomic response to G4 ligands. In addition, we examined genes involved in replication and reparation, with a particular focus on helicases that may be involved in the unwinding of G4-quadruplexes.
Materials and Methods
Bacterial Strain, Growth Conditions, and Inhibition Assay
Mycobacterium smegmatis MC2155 strain was used in this study. Middlebrook 7H11 Agar (HiMedia, India) and Middlebrook 7H9 broth (HiMedia) both supplemented with 0.5% glycerol and 10% Middelbrook OADC Growth Supplement (HiMedia) were used as solid and liquid media, respectively. A frozen stock of M. smegmatis strain was cultured on a solid media for 1 day to obtain a sufficient number of cells for inoculation of broth culture.
To determine MICs for BRACO-19 (PubChem CID no. 9808666) and TMPyP4 (PubChem CID no. 135398505; Sigma-Aldrich, United States), bacterial cells were cultured overnight in Middlebrook 7H9 broth, with the subsequent dilution in the fresh 7H9 medium (1:200). The diluted culture was dispensed (196 μl) to the wells of the CELLSTAR 96 Well Cell Culture Plates (Greiner Bio-One GmbH, Germany) and 4 μl of serial 2-fold dilutions of the tested compounds in DMSO were added to the wells to final concentrations of 2.5–80 μM BRACO-19 and 0.25–16 μM for TMPyP4. The plates were incubated for 64 h at 37°C in a humid atmosphere with 5% CO2 and shaken at 250 rpm. The MICs were determined by an optical density measurement at 570 nm on xMark Microplate Absorbance Spectrophotometer (Biorad, United States) and defined as the lowest concentration of the compound at which no growth was observed. Negative control samples were treated with the same volume of DMSO; the experiments were performed in three biological replicates.
For the transcriptomic analysis, M. smegmatis cells were grown up to the mid-exponential phase (OD570 = 0.47) and transferred to the 5 ml tubes (NUOVA APTACA, Italy). Ligands were added to the tubes to a final concentration corresponding to 1 × MIC (10 μM for BRACO-19 and 4 μM for TMPyP4). The same volume of DMSO was added to the control samples (1% v/v). Bacterial cells were incubated for 4 h (cell division time; Logsdon and Aldridge, 2018) at 37°C in a humid atmosphere with 5% CO2 and shaken at 250 rpm. The experiments were carried in three biological replicates.
Total RNA Extraction and RNA-seq
Bacterial cells were harvested by centrifugation (8,000 × g, 10 min, 4°C) and subsequently washed twice with a phosphate-buffered saline (10 ml). A double volume of RNAprotect Bacteria Reagent (Qiagen, United States) was added to the pellet. The mixture was incubated at room temperature for 5 min and then centrifuged (8,000 × g, 10 min, 4°C). Samples were homogenized for 30 s using an automatic bead homogenizer (MagNAlyzer, Roche). RNA was extracted using the MagMAX mirVana Total RNA Isolation Kit (Thermo Fisher Scientific, Lithuania) on the KingFisher Flex Purification System (Thermo Fisher Scientific, United States) according to the manufacturer’s instructions. RNA was treated with DNase using Turbo DNA-Free Kit (Thermo Fisher Scientific) in the 50 μl volume, and further purified using Agencourt RNAClean XP (Beckman Coulter, United States) according to the manufacturer’s instructions. The total amount of RNA was measured with the Quant-iT Ribogreen RNA assay kit (ThermoFisher Scientific), and the quality of extracted RNA was checked by Agilent Bioanalyzer on Agilent RNA 6000 Pico Chips (Agilent Technologies, United States).
Total RNA (300 ng) was used for library preparation. The Ribo-Zero Plus rRNA Depletion Kit (Illumina, United States) was used to remove rRNA, and the NEBNext Ultra II Directional RNA Library Prep Kit (NEB) was used for library preparation. RNA cleanup was performed with the Agencourt RNA Clean XP kit (Beckman Coulter). Final cleanup was done using the Agencourt AMPure XP system (Beckman Coulter) after which the libraries’ size distribution and quality were assessed using a high sensitivity DNA chip (Agilent Technologies). Libraries were subsequently quantified with Quant-iT High Sensitivity dsDNA Assay Kit (Thermo Fisher Scientific). The libraries were sequenced on an Illumina HiSeq 2500 (50 bp single-end reads), with 12 pM loading concentration, and the PhiX control library (Illumina) spiked in at 5%. The RNA-seq data generated in this study have been deposited to the NCBI Sequence Read Archive under accession number PRJNA765512.1
Inferring Putative G4 Quadruplex Sequences
To infer PQSs in M. smegmatis MC2155 (GenBank accession no. CP000480.1) genome G4-iM Grinder (v1.6.1; Belmonte-Reche and Morales, 2020) package for R (v4.0.5; R Core Team, 2021) was used. The following parameters were used: Complementary = TRUE, BulgeSize = 0, RunComposition = “G,” MaxRunSize = 4, MinRunSize = 3, MaxNRuns = 0, MinNRuns = 4, MaxIL = 0, MaxPQSSize = 50, MinPQSSize = 15, MaxLoopSize = 15, MinLoopSize = 0. Size independent quadruplexes without overlapping (method 3a) with a score ≥ 40 were used for further analysis. BEDtools (v2.30.0; Quinlan and Hall, 2010) and custom Python scripts were used to assign PQSs to M. smegmatis MC2155 genes and intergenic regions (Supplementary Table S1). When PQS was located in both gene and intergenic region, it was assigned to both categories. Intergenic PQSs were assigned without regard to strand orientation. For the analysis, only PQSs located upstream (downstream for the template strand) of genes were used. To assign PQSs to promoter regions, a list of previously published M. smegmatis MC2155 transcription start sites (TSSs) was used (Li et al., 2017). Promoters were considered to be located 50 bp upstream or downstream of TSSs, depending on the strandedness of the gene.
Circular Dichroism Spectroscopy and Melting Assay
Oligodeoxyribonucleotides (ODNs) were obtained from Litekh, Russia. To verify G4 folding, circular dichroism (CD) spectra of the ODN samples in 10 mM potassium-phosphate buffer, pH 7, were registered at 15°C using Chirascan spectrophotometer (Applied Biophysics, United Kingdom). The ODN samples were annealed rapidly (heated to 90°C and then snap-cooled on ice) to facilitate intramolecular folding prior to all measurements. Moderate salt concentration was used to avoid extremely high thermal stability of the high-scoring G4s, which would hamper accurate assessment of ligands’ effects. Thermal stability (T1/2) of each G4 was calculated as an average value of the melting (Tm) and annealing (Ta) temperatures estimated based on the annealing and melting curves, respectively. The melting and annealing curves were registered using Chirascan spectrophotometer by CD monitoring at G4-specific maxima (260 nm for parallel-stranded/mixed and 295 nm for antiparallel-stranded/mixed G4s) with a temperature ramp rate of 1°C/min in the presence and in the absence of the ligands. G4 and ligand concentrations were 2.5 μM.
Differential Gene Expression Analysis
The sequenced reads were mapped to the reference M. smegmatis MC2155 genome (GenBank accession no. CP000480.1) using HISAT2 (v2.2.1; Kim et al., 2015). SAMtools (v1.11; Li et al., 2009) software was used to sort and convert SAM files to BAM, and their subsequent indexing. Mapping quality and coverage along genes were assessed with QualiMap (v2.2.2; Okonechnikov et al., 2016), individual reports were merged with MultiQC (v1.9; Ewels et al., 2016). Mapped reads were assigned to genes with featureCounts (v2.0.1; Liao et al., 2014). Differential gene expression analysis was performed using the edgeR (v3.30.3; Robinson et al., 2010) package for R. Genes with a false discovery rate (FDR) cutoff of 0.05 and with a fold change (log2FC) threshold of |1| (i.e., ≥ |2|-fold change) were considered to be differentially expressed. For intersample comparison, counts were normalized using gene length corrected trimmed mean of M-values (GeTMM; Smid et al., 2018). Further functional enrichment analysis of the GO terms and KEGG pathways for differentially expressed genes was performed using clusterProfiler (v3.18.1; Yu et al., 2012) package, categories were considered enriched with padj. ≤ 0.05. In addition, differentially expressed genes were classified into functional categories based on the PATRIC database (Wattam et al., 2014). Clusters of orthologous genes (COG) were annotated by mapping M. smegmatis MC2155 proteome to NCBI COG database (v2020)2 using DIAMOND (v2.0.9; Buchfink et al., 2014), and with eggNOG-mapper (v2; Huerta-Cepas et al., 2019). Plots were generated within R using ggplot2 (v3.3.2; Wickham, 2009), ggforce (v0.3.3; Pedersen, 2021), ggvenn (v0.1.9; Yan, 2021), ggsignif (v0.6.1; Ahlmann-Eltze and Patil, 2021), lemon (v0.4.5; Edwards, 2020), and cowplot (v1.1.0; Wilke, 2020) packages.
Statistical Analysis
All statistical analyses were performed using RStudio (v1.4.1106). Statistical evaluation was performed by the two-sided Wilcoxon rank-sum test and the Student’s t-test; value of p < 0.05 was considered to be statistically significant.
Results
Differential Gene Expression of Mycobacterium smegmatis MC2155 in the Presence of BRACO-19 and TMPyP4 G4 Ligands
To understand how G4-stabilizing compounds affect gene expression levels, the transcriptomic profiles of M. smegmatis MC2155 strain exposed to BRACO-19 and TMPyP4 for 4 h at 1 × MIC were examined (Supplementary Table S1). According to dilution-based antimicrobial susceptibility testing, BRACO-19 and TMPyP4 had in vitro minimum inhibitory concentration (MIC) values of 10 and 4 μM, respectively (Supplementary Figure S1).
Multidimensional scaling (MDS) of normalized RNA-seq data (three biological replicates and three different conditions) showed a clear separation of samples by condition (Control, BRACO-19, and TMPyP4; Figure 1A). Expression significantly changed (FDR < 0.05, log2FC > |1|) for 822 (515↑; 307↓) genes in M. smegmatis in response to BRACO-19 and for 680 (339↑; 341↓) genes in response to TMPyP4. Among the 191 differentially expressed genes (DEGs) in response to both BRACO-19 and TMPyP4, 131 genes were upregulated, and 60 genes were downregulated (Figure 1B).
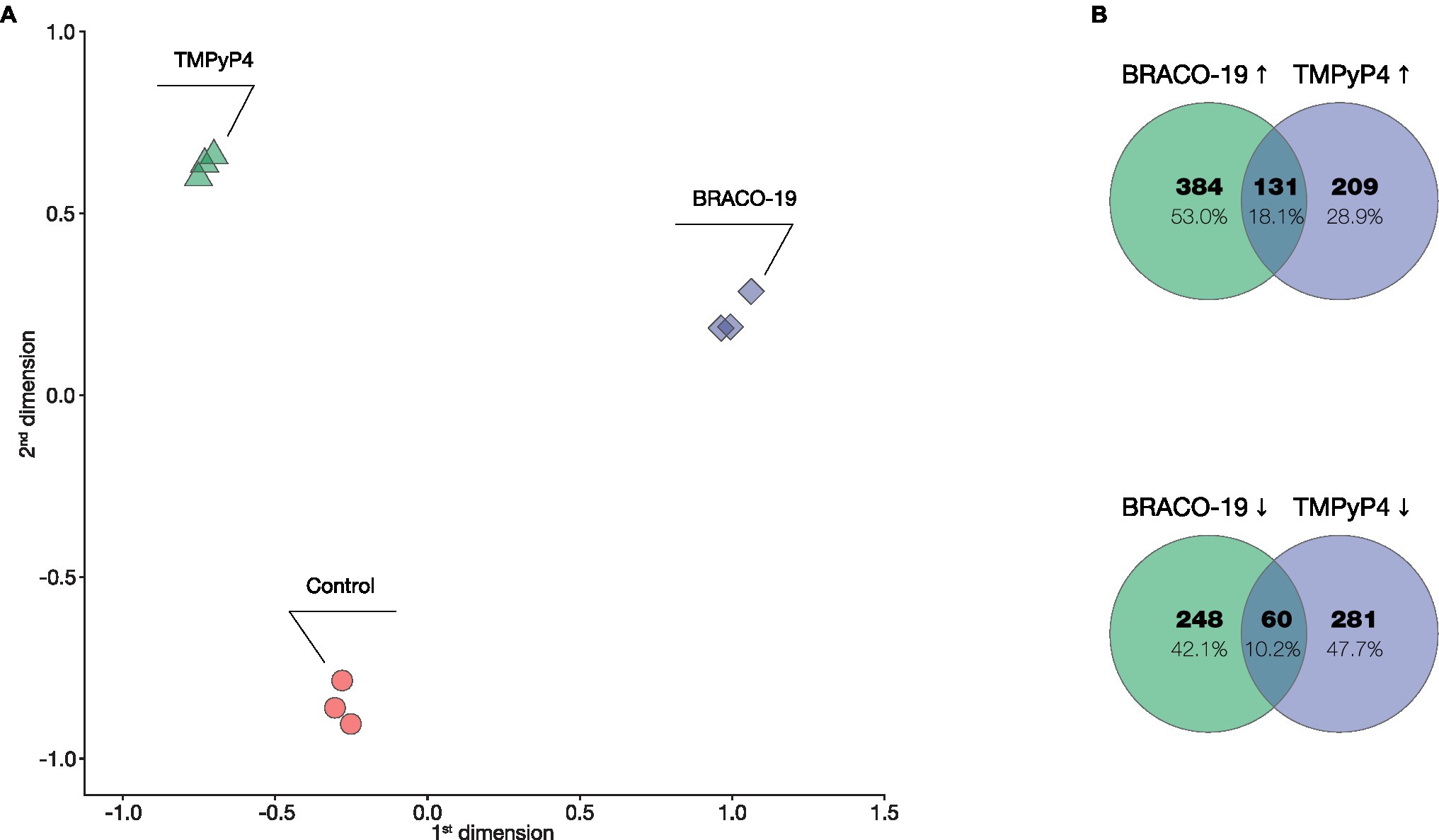
Figure 1. Transcriptional differences between BRACO-19 and TMPyP4 treated Mycobacterium smegmatis samples. (A), MDS plot of correlation of genes expression levels of three replicate samples (indicated with different colors) across three different conditions; (B), Venn diagrams showing the genes co-regulated by the presence of BRACO-19 and TMPyP4 compounds, as determined by RNA-seq.
The Effect of Ligands on the Expression of Genes Linked to G4s
Potential G-quadruplex forming sequences (PQSs) in the M. smegmatis MC2155 genome were identified using G4-iM Grinder. In total, 53 (14/53 were valid for two genes) PQSs were found in intergenic regions, 17 PQSs were located in promoter regions, 267 PQSs were situated in the mRNA-like strand of the 255 genes, and 398 PQSs were located in the template strand of the 360 genes (Supplementary Table S2).
The results of RNA-seq analysis showed that there are no significant differences in expression levels of the bulk of the genes associated with G4s (i.e., those harboring PQSs; Supplementary Figure S2). Further, analyses for PQSs located on mRNA-like strand, template strand, intergenic regions, and in promoters between the control sample and samples exposed to G4 ligands, showed no statistically significant differences (two-sided Wilcoxon test p > 0.05; Figure 2A). Besides, there was no difference in genes expression (log2FC) relative to G4 strandedness (mRNA-like or template) in intergenic regions, neither in BRACO-19 (Student’s t-test p = 0.519) nor in TMPyP4 (Student’s t-test p = 0.645) treated samples. Furthermore, no significant differences were observed in expression levels of genes co-regulated by both ligands, between genes associated with G4s (n = 14), and without G4s (n = 177; two-sided Wilcoxon test p > 0.05; Figure 2B).
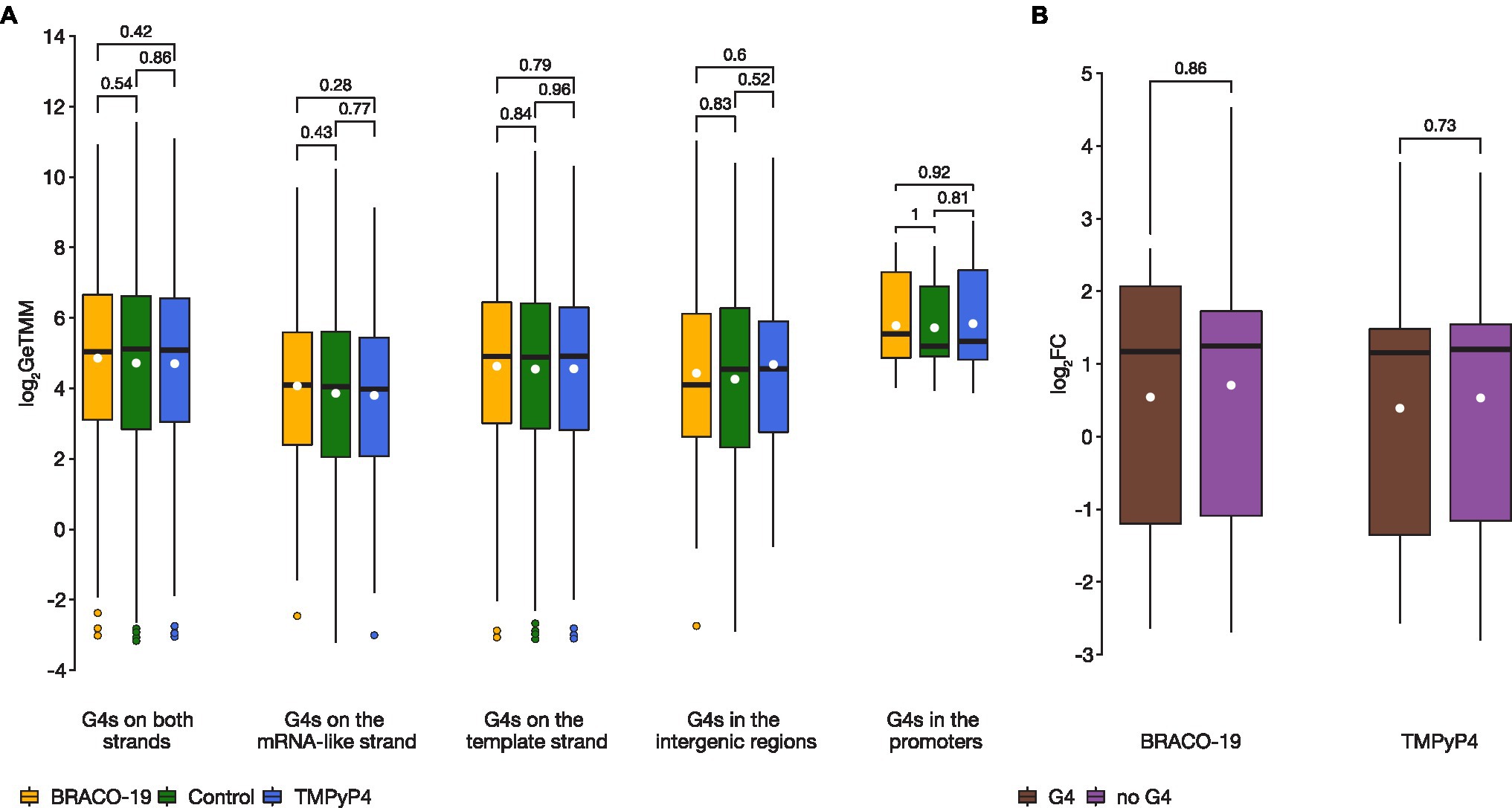
Figure 2. There were no statistically significant differences in expression levels of genes containing G4 motifs between control and treated samples. (A), A boxplot showing expression variance of G4-associated genes between control (green), on exposure to BRACO-19 (yellow), and on exposure to TMPyP4 (blue). The box shows the upper and lower quartiles, and the line within the box shows the median. The white dot indicates the mean. Points above the whiskers indicate outliers. Two-sided Wilcoxon rank-sum test with continuity correction was used to calculate values of p; (B), A boxplot showing the change in expression (log2FC) of co-regulated genes (n = 191) observed by RNA-seq of M. smegmatis MC2155 exposed to BRACO-19 and TMPyP4 ligands. The box shows the upper and lower quartiles, and the line within the box shows the median. The white dot indicates the mean. Two-sided Wilcoxon rank-sum test with continuity correction was used to calculate values of p.
To verify our RNA-seq results and G4-stabilizing effect of the ligands, we investigated 10 high-scoring PQS fragments of randomly selected genes that showed: enhanced expression levels in response to ligand treatment (n = 4), no significant changes (n = 2), or decreased expression levels (n = 4) (Supplementary Table S3). The effect of G4-ligands on PQSs stability was determined using thermal denaturation monitored by circular dichroism spectroscopy (Supplementary Figure S3). In the presence of BRACO-19 and TMPyP4, G4 stabilization was observed, nonetheless these results did not correlate with the changes in corresponding genes expression levels.
Changes in the Expression of DNA Replication and Repair Systems Mediated by G4 Ligands
Additionally, the genes of the replication and repair system were considered (Supplementary Table S4), since G4 stabilizing ligands can damage DNA and cause genome instability (Saranathan and Vivekanandan, 2019). In total, 13 and four genes exhibited more than 2-fold expression level changes under the action of BRACO-19 and TMPyP4, respectively (Table 1).
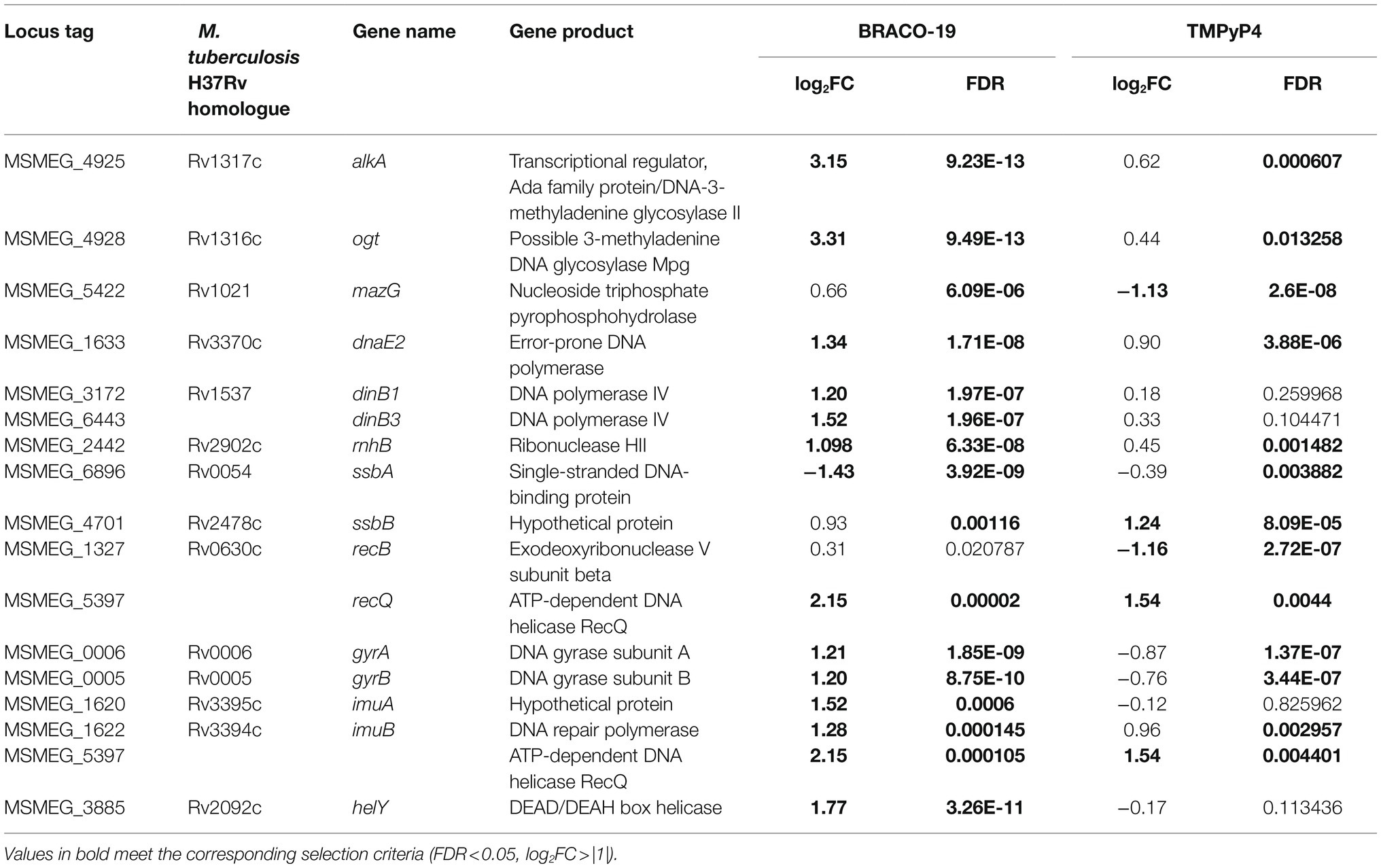
Table 1. Differentially expressed genes of the DNA replication and repair systems in M. smegmatis exposed to BRACO-19 and TMPyP4.
Genes encoding DNA polymerases DinB and DnaE2, assessor proteins ImuA and ImuB, DNA gyrases GyrA and GyrB, proteins of alkylation repair system AlkA and Ogt, as well as HelY and RecQ helicases were overexpressed on exposure to BRACO-19. Most of these genes are required for translesion DNA synthesis. Polymerase DnaE2 (C-family probable translesion polymerase) is reportedly involved in adaptive mutagenesis in response to DNA damage (Boshoff et al., 2003). Accessory proteins ImuA and ImuB facilitate DnaE2-dependent translesion synthesis43, thus contributing to bacterial SOS response. Although the role of DinB1, DinB2 [duplicated in M. smegmatis; MSMEG_2294/MSMEG_1014 (both genes had zero total read counts)], and DinB3 (specific for M. smegmatis) Y-family polymerases is still not fully understood, it has been reported that DinB1 and DinB3 are typical DNA-dependent DNA polymerases, while DinB2 misincorporates deoxyribonucleotides and ribonucleotides during templated synthesis and lesion bypass (Ordonez and Shuman, 2014). Notably, according to previous reports, neither dinB1- nor dinB2-encoded PolIV homolog is up-regulated during the mycobacterial damage response (Rand et al., 2003). The up-regulated DNA gyrases (type II DNA topoisomerases) contribute to DNA synthesis by catalyzing the introduction of negative supercoiled turns in an ATP-dependent manner, while double-stranded DNA is unwound by lengthening the RNA polymerase or helicase in front of the progressive replication fork (Aubry et al., 2006). Among genes responsible for alkylation repair, ogt and alkA had the highest expression levels. Methyltransferase Ogt and AlkA DNA-glycosylase are the major proteins of repair systems; they counteract DNA alkylation damage, thus preventing cell death (Yang et al., 2011).
In TMPyP4-treated M. smegmatis, ssbB (MSMEG_4701), which encodes the RecA accessory factor required for recombination repair during stress (Singh, 2018), was the only up-regulated repair system gene (log2FC = 1.24), and this gene was also upregulated in BRACO-19 case (log2FC = 0.93). On the contrary, ssbA (MSMEG_6896), which encodes another RecA cofactor (Singh, 2018), was downregulated for both ligands (log2FC = −1.43 for BRACO-19, and log2FC = −0.3 for TMPyP4). Such a multi-directional change in the level of expression of the aforementioned genes has been described previously for ultraviolet and hypoxia-induced stress in M. smegmatis (Singh et al., 2018).
Additional analysis of previously described helicases (DinG, UvrD1, and UvrD2; Thakur et al., 2014; Saha et al., 2019), responsible for the unwinding of the G4-quadruplexes, showed slightly increased expression levels, although only significant (FDR < 0.05) for BRACO-19 (Supplementary Table S1). Besides, DNA helicase RecQ was up-regulated under BRACO-19 and TMPyP4. RNA-activated ATPase/dATPase and 3′-5′ helicase helY gene was upregulated on exposure to BRACO-19. In the TMPyP4 case, the ATP-dependent RNA helicase MSMEG_1540 was downregulated (log2FC = −2.08).
Functions and Pathways of Mycobacterium smegmatis MC2155 Genes Responding to Treatment With G4 Ligands
Since there was no strong evidence that BRACO-19 and TMPyP4 ligands affect the expression of G4-carrying genes in M. smegmatis, further analysis of transcriptomic data was performed. Differentially expressed genes were subjected to functional classification and pathway enrichment analysis based on the Kyoto Encyclopaedia of Genes and Genomes (KEGG) and gene ontology (GO) databases (Supplementary Table S5).
For BRACO-19, only one KEGG pathway—msm01053 “Biosynthesis of siderophore group nonribosomal peptides” (padj. = 1.28e-05), was enriched for upregulated genes. This pathway includes mbt cluster genes (Figure 3; Supplementary Table S6), which are responsible for the synthesis of the most important iron-chelating compounds—siderophores (mycobactin and carboxymycobactin in mycobacteria; Sritharan, 2016). Analysis of the exochelin locus, which is a specific siderophore for non-virulent mycobacteria, revealed similar changes. Also, overexpression of genes involved in siderophore transport and acquisition was observed. Among these genes were the iron-dependent transporters irtAB and ESX-3 operon. The ATP binding cassette transporter IrtAB imports iron-bound siderophores across the inner membrane, while the type VII secretion system Esx-3 plays a crucial role in iron acquisition through secretion of a pair of PE–PPE family proteins. In turn, iron storage genes bfrA and bfrB, encoding a heme-containing bacterioferritin (BfrA) and a ferritin-like protein (BfrB), were slightly downregulated. It is worth noting that almost all the aforementioned genes are controlled by the IdeR (iron-dependent regulator; MSMEG_2750; Sritharan, 2016). The ideR gene was slightly upregulated (log2FC = 0.65) compared to the control, notably, there is a high-scored (x = 57) quadruplex with four G-tetrads (GGGGATGGGGTTGCCGAACGGGGAGGTGGTGGGG) located on the template strand of the gene.
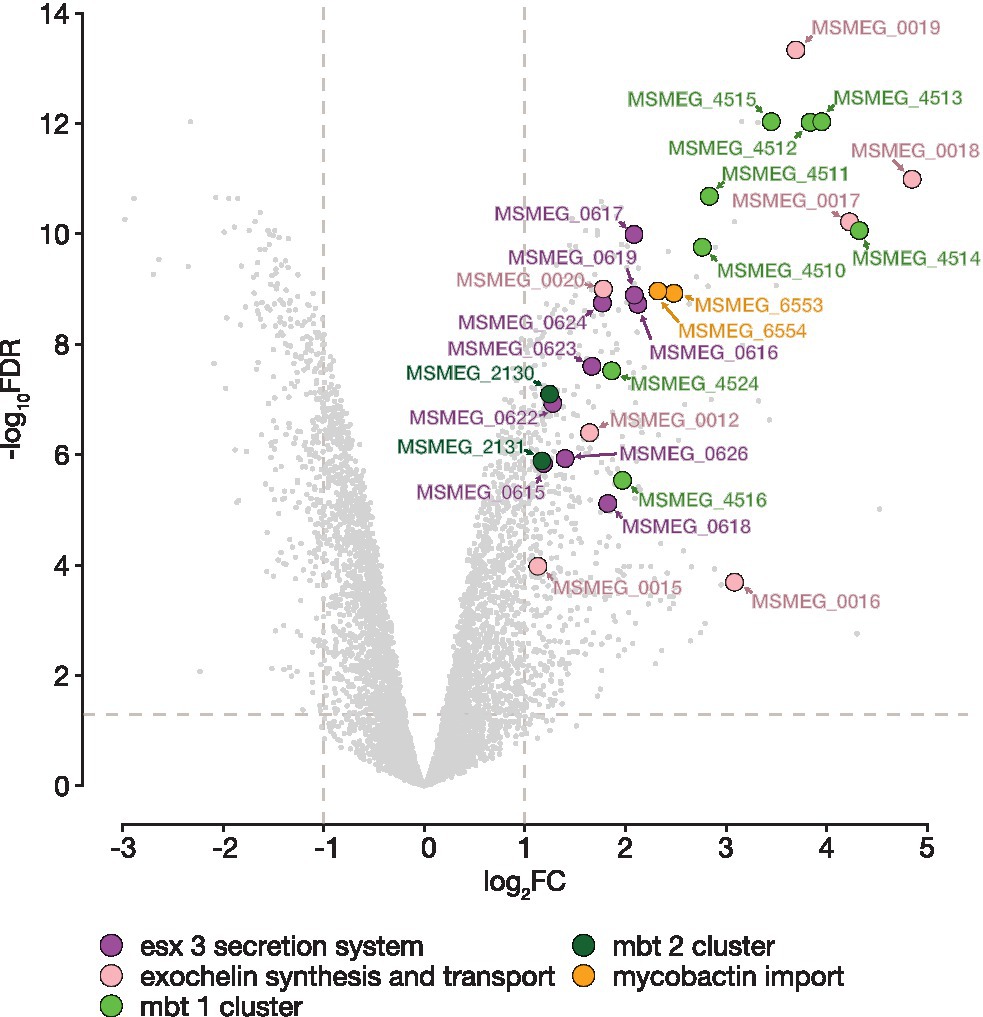
Figure 3. BRACO-19 compound affects M. smegmatis MC2155 iron metabolism. Volcano plot indicates the distribution of DEGs for the M. smegmatis MC2155 exposed to BRACO-19 ligand. Log transformed fold changes (log2FC) are plotted on the x-axis, and significance (−log10FDR) is plotted on the y-axis. Colors denote genes involved in iron metabolism with a significantly increased level of expression (FDR < 0.05, log2FC > |1|).
In the case of TMPyP4-exposed bacteria, the following biological process GO terms were enriched: GO:0006351 “transcription, DNA-templated” (padj. = 0.002) for upregulated genes, and GO:0006526 “arginine biosynthetic process” (padj. = 1.21e-05) for downregulated genes. The KEGG pathways associated with upregulated genes in the TMPyP4-treated sample, were msm00920 “Sulfur metabolism” (padj. = 0.02), and msm02010 “ABC transporters” (padj. = 0.02), and one pathway associated with down-regulated genes was msm00220 “Arginine biosynthesis” (padj. = 0.03). Primarily, GO:006351 term included potential transcription factor genes with increased expression that may reflect significant changes in metabolism in response to ligand action. KEGG pathway msm02010 included almost all the genes in msm00920 pathway (7/8) and consisted of the genes responsible for sugar transport, phosphates, and sulfur-containing amino acids. At the same time, genes involved in the synthesis of the amino acid arginine (MSMEG_3046, MSMEG_3047, MSMEG_3769-MSMEG_3776) were downregulated in TMPyP4-treated M. smegmatis. Of these, carbamoyl phosphate synthetases units A (MSMEG_3046) and B (MSMEG_3047) are involved in pyrimidine and arginine biosynthesis, catalyzing the conversion of glutamine to carbamoyl phosphate, while products of the argDBJC (MSMEG_3773-MSMEG_3776) genes are part of a five-enzyme pathway that uses glutamate to produce ornithine. Both products are used in the urea cycle to produce arginine under the action of ArgG (MSMEG_3770) in conjunction with ArgF (MSMEG_3772) and ArgH (MSMEG_3769) enzymes.
Discussion
Here, we employed RNA-seq analysis to demonstrate the impact of G4-ligands, BRACO-19 and TMPyP4, on M. smegmatis transcription and elucidate their mechanism of action. Mycobacterium smegmatis was used in this study because it is considered as a valuable model organism for mycobacterium study due to the close association with M. tuberculosis regarding biochemical properties and genetic information (Joseph Antony Sundarsingh et al., 2020), and, importantly, has a similar GC composition. Both ligands used are best known as stabilizers of human telomeric G4s, which implies cancer-specific antiproliferative activity (Rha et al., 2000; Incles et al., 2004). However, they also exhibit broad-spectrum G4 stabilizing activity and, along with other pan-quadruplex ligands, are being tested for their therapeutic potential against various bacterial species, including M. tuberculosis. In the case of M. smegmatis (this study) and M. tuberculosis (Beaudoin et al., 2014; Mishra et al., 2019), both compounds inhibited the growth at submicromolar concentrations, but TMPyP4 was slightly more active for M. smegmatis.
Both BRACO-19 and TMPyP4 have been reported to stabilize synthetic G4s from M. tuberculosis. TMPyP4 has also been shown to reduce transcription of PQS-containing genes (Beaudoin et al., 2014; Mishra et al., 2019). Based on our findings, there was no strong evidence of significant up- or down-regulation of genes associated with PQSs caused by G4-ligands. Notably, we analyzed ligands’ effects both separately and in conjunction to account for specific DEGs and the co-regulated ones, respectively. However, even for co-regulated genes, we were unable to find concrete evidence of ligand-mediated G4-stabilization. The examples of DEGs that are co-regulated by both compounds and harbor/neighbor PQSs include MSMEG_4727↓, MSMEG_1680↓, MSMEG_4689↓, MSMEG_3948↓, MSMEG_2242↑, MSMEG_3121↑, and MSMEG_2148↑ (contain PQSs on the template strand of the gene); MSMEG_1488↑, MSMEG_0868↑, MSMEG_6223↑, MSMEG_5397↑, and MSMEG_4202↑ (contain PQSs on the mRNA-like strand); MSMEG_6567↑ (contains one PQS on each strand); MSMEG_3917↑ (contains a PQS on the template strand in the intergenic region), and MSMEG_4643↓ (contains a PQS on the mRNA-like strand in the intergenic region). Moreover, additional CD assays showed stabilization of PQSs by BRACO-19 and TMPyP4, which did not correlate with the results of transcriptome analysis.
We hypothesize that the ligands act primarily during replication, when the majority of G4s are formed (as has been proven for eukaryotic cells; Rodriguez et al., 2012), and transcription changes stem partly from the disruption of replication (Lerner and Sale, 2019). It is possible that the observed transcription changes illustrate compensatory response rather than primary ligands’ effects. G4 stabilization upon replication may cause polymerase stalling and SOS-response (Maslowska et al., 2019). Enhanced recruitment of the translesion synthesis machinery or timely ligand dislodging and G4 unwinding by helicases would then be required for the tolerance of ligand-induced toxicity. In the present study, under the action of BRACO-19, we identified modest upregulation of DnaE2, DinB1, and DinB3 translesion polymerases; however, no significant changes in expression levels of SOS-regulation genes (recA and lexA) were registered. Meanwhile, treatment with BRACO-19 and TMPyP4 resulted in overexpression of M. smegmatis specific gene MSMEG_5397 encoding RecQ helicase (Table 1). The importance of RecQ DNA helicases in G4 unwinding in bacteria has been previously demonstrated in the case of Escherichia coli, although RecQ protein in M. smegmatis is not homologous to E. coli (Huber et al., 2006; Singh, 2017). In this respect, it is not possible to affirm the relationship between the overexpression of the MSMEG_5397 and the action of ligands. In addition, BRACO-19 increased the expression of the helY gene. In M. smegmatis HelY unwinds 3′-tailed RNA duplexes and RNA–DNA hybrids, but is unable to unwind a 3′-tailed duplex in which the loading strand is DNA. It is possible that HelY participates in the unwinding of RNA G4 quadruples; however, the eukaryotic homologs Ski2 and Mtr4 do not possess such properties (Uson et al., 2015).
BRACO-19-induced overexpression of iron metabolism-related genes may be an additional indication of the increased need for metalloproteins involved in replication and DNA repair (Expert et al., 2008; Puig et al., 2017), i.e., the Fe/S cluster-containing enzymes (Puig et al., 2017). Interestingly, similar expression patterns for exochelin biosynthesis, exochelin uptake, and mycobactin synthesis genes have been reported for M. smegmatis grown in iron deficiency conditions (Ojha and Hatfull, 2007) and under both oxidative and nitrosative stress (Namouchi et al., 2016).
The observed changes in iron metabolism in response to BRACO-19 treatment may, obviously, have a more straightforward explanation. They could all be driven by a single BRACO-19-sensitive (G4-harboring) master gene. We have considered such a possibility, and the iron-dependent regulator (ideR) was a primary candidate. However, ideR exhibited only minor expression changes and only in BRACO-19-treated samples. This finding goes against the straightforward explanation. Instead of primary effects, we are probably witnessing an adaptation to G4-related general replication stress.
It is reasonable to assume that the global replication stress arises from direct ligand interactions with G4 DNA. Interactions with G4 RNA have not been considered herein but should probably be the subject of future studies. Persistent mRNA G4s are expected to impair translation and tend to be depleted in prokaryotes (Guo and Bartel, 2016). Recent studies advocate the existence of transient G4s in both eukaryotic and prokaryotic RNA (Kwok et al., 2018; Shao et al., 2020). Importantly, G4 folding modulates the function of non-coding RNA (ncRNA), and stabilization of the ncRNA G4s by the ligands may eventually affect the transcription profile (Shao et al., 2020). Thus, a combination of G4-seq and rG4-seq (Marsico et al., 2019) may prove helpful to further elucidate the key targets of G4 ligands and the mechanisms behind their antibacterial activity.
Notably, nucleoid proteins, transcription factors, and probably some other factors affect G4 stability in vivo (Parekh et al., 2019), arguing that chromatin immunoprecipitation assays with G4-specific antibodies (G4-ChIP-seq) are needed to bona fide confirm G4 formation. The actual number of G4 targets may be substantially lower than that predicted by bioinformatics tools, which is the case in eukaryotes (Hänsel-Hertsch et al., 2018). Moreover, regarding the transient nature of G4 structures (Yadav et al., 2021), the effects of the ligands should ideally be monitored in dynamics. We only analyzed a single time point and emphasize that the presented results, although valid for selected conditions, are insufficient for comprehensive characterization of the G4 ligands.
In conclusion, we provided a transcriptome-wide analysis of M. smegmatis under the action of two G4 ligands. Although there have been significant changes in the transcriptional profile of the bacterium, neither BRACO-19 nor TMPyP4 treatment induced a significant change in G4 associated genes. In turn, for the BRACO-19 compound, we found significant changes in the replication and repair system genes, which is, undoubtedly, evidence of the induced stress. Changes in iron metabolism genes also support this hypothesis. For the TMPyP4 compound, we did not find changes in the repair and replication system, however, significant changes in transcription factors and the arginine biosynthesis system may indicate multiple biological targets for this compound, which does not eliminate direct interaction with DNA.
Data Availability Statement
The datasets presented in this study can be found in online repositories. The names of the repository/repositories and accession number(s) can be found in the article/Supplementary Material.
Author Contributions
ES, MZ, DB, and AV wrote the main manuscript text. DB, JB, KK, VV, and IB prepared figures and tables. MM, TV, MZ, and IB cultivated mycobacteria and performed susceptibility tests. DB, KK, and VV conducted RNA-seq analysis. ES, EI, MM, JB, and AV designed the experiment. All authors reviewed the manuscript. All authors contributed to the article and approved the submitted version.
Funding
This research was funded by the Russian Science Foundation (RSF, grant number 19-75-10109).
Conflict of Interest
The authors declare that the research was conducted in the absence of any commercial or financial relationships that could be construed as a potential conflict of interest.
Publisher’s Note
All claims expressed in this article are solely those of the authors and do not necessarily represent those of their affiliated organizations, or those of the publisher, the editors and the reviewers. Any product that may be evaluated in this article, or claim that may be made by its manufacturer, is not guaranteed or endorsed by the publisher.
Acknowledgments
The authors thank the Center for Precision Genome Editing and Genetic Technologies for Biomedicine, Federal Research and Clinical Center of Physical-Chemical Medicine of Federal Medical Biological Agency for providing the WGS platform.
Supplementary Material
The Supplementary Material for this article can be found online at: https://www.frontiersin.org/articles/10.3389/fmicb.2022.817024/full#supplementary-material
Footnotes
References
Ahlmann-Eltze, C., and Patil, I. (2021). ggsignif: Significance Brackets for “ggplot2.” Available at: https://CRAN.R-project.org/package=ggsignif (Accessed June, 2021).
Aubry, A., Mark Fisher, L., Jarlier, V., and Cambau, E. (2006). First functional characterization of a singly expressed bacterial type II topoisomerase: The enzyme from mycobacterium tuberculosis. Biochem. Biophys. Res. Commun. 348, 158–165. doi: 10.1016/J.BBRC.2006.07.017
Bartas, M., Čutová, M., Brázda, V., Kaura, P., Šťastný, J., Kolomazník, J., et al. (2019). The presence and localization of G-Quadruplex forming sequences in the domain of bacteria. Molecules 24:1711. doi: 10.3390/MOLECULES24091711
Beaudoin, J.-D., Jodoin, R., and Perreault, J.-P. (2014). New scoring system to identify RNA G-quadruplex folding. Nucleic Acids Res. 42, 1209–1223. doi: 10.1093/NAR/GKT904
Belmonte-Reche, E., and Morales, J. C. (2020). G4-iM grinder: when size and frequency matter. G-Quadruplex, i-motif and higher order structure search and analysis tool. NAR Genom. Bioinform. 2:lqz005. doi: 10.1093/NARGAB/LQZ005
Boshoff, H. I. M., Reed, M. B., Barry, C. E., and Mizrahi, V. (2003). DnaE2 polymerase contributes to In vivo survival and the emergence of drug resistance in Mycobacterium tuberculosis. Cell 113, 183–193. doi: 10.1016/S0092-8674(03)00270-8
Buchfink, B., Xie, C., and Huson, D. H. (2014). Fast and sensitive protein alignment using DIAMOND. Nat. Methods 12, 59–60. doi: 10.1038/nmeth.3176
Byrd, A. K., and Raney, K. D. (2017). Structure and function of Pif1 helicase. Biochem. Soc. Trans. 45, 1159–1171. doi: 10.1042/BST20170096
Ding, Y., Fleming, A. M., and Burrows, C. J. (2018). Case studies on potential G-quadruplex-forming sequences from the bacterial orders Deinococcales and Thermales derived from a survey of published genomes. Sci. Rep. 8, 1–11. doi: 10.1038/s41598-018-33944-4
Edwards, S. M. (2020). Lemon: Freshing Up your “ggplot2” plots. Available at: https://CRAN.R-project.org/package=lemon (Accessed June, 2021).
Ewels, P., Magnusson, M., Lundin, S., and Käller, M. (2016). MultiQC: summarize analysis results for multiple tools and samples in a single report. Bioinformatics 32, 3047–3048. doi: 10.1093/BIOINFORMATICS/BTW354
Expert, D., Boughammoura, A., and Franza, T. (2008). Siderophore-controlled iron assimilation in THE Enterobacterium Erwinia chrysanthemi: Evidence For The Involvement Of bacterioferritin and the Suf iron-sulfur cluster assembly Machinery. J. Biol. Chem. 283, 36564–36572. doi: 10.1074/JBC.M807749200
Global Tuberculosis Report 2020 (2020). Available at: http://apps.who.int/bookorders (Accessed September 27, 2021).
Guo, J. U., and Bartel, D. P. (2016). RNA G-quadruplexes are globally unfolded in eukaryotic cells and depleted in bacteria. Science 353:aaf5371. doi: 10.1126/science.aaf5371
Hänsel-Hertsch, R., Spiegel, J., Marsico, G., Tannahill, D., and Balasubramanian, S. (2018). Genome-wide mapping of endogenous G-quadruplex DNA structures by chromatin immunoprecipitation and high-throughput sequencing. Nat. Protoc. 13, 551–564. doi: 10.1038/nprot.2017.150
Harris, L. M., and Merrick, C. J. (2015). G-Quadruplexes in pathogens: a common route to virulence control? PLoS Pathog. 11:e1004562. doi: 10.1371/JOURNAL.PPAT.1004562
Holder, I. T., and Hartig, J. S. (2014). A matter of location: influence of G-Quadruplexes on Escherichia coli gene expression. Chem. Biol. 21, 1511–1521. doi: 10.1016/J.CHEMBIOL.2014.09.014
Huber, M. D., Duquette, M. L., Shiels, J. C., and Maizels, N. (2006). A conserved G4 DNA binding domain in RecQ family helicases. J. Mol. Biol. 358, 1071–1080. doi: 10.1016/J.JMB.2006.01.077
Huerta-Cepas, J., Szklarczyk, D., Heller, D., Hernández-Plaza, A., Forslund, S. K., Cook, H., et al. (2019). eggNOG 5.0: a hierarchical, functionally and phylogenetically annotated orthology resource based on 5090 organisms and 2502 viruses. Nucleic Acids Res. 47, D309–D314. doi: 10.1093/NAR/GKY1085
Incles, C. M., Schultes, C. M., Kempski, H., Koehler, H., Kelland, L. R., and Neidle, S. (2004). A G-quadruplex telomere targeting agent produces p16-associated senescence and chromosomal fusions in human prostate cancer cells. Mol. Cancer Ther. 3, 1201–1206. doi: 10.1158/1535-7163.1201.3.10
Joseph Antony Sundarsingh, T., Ranjitha, J., Rajan, A., and Shankar, V. (2020). Features of the biochemistry of Mycobacterium smegmatis, as a possible model for Mycobacterium tuberculosis. J. Infect. Public Health 13, 1255–1264. doi: 10.1016/J.JIPH.2020.06.023
Kim, D., Langmead, B., and Salzberg, S. L. (2015). HISAT: a fast spliced aligner with low memory requirements. Nat. Methods 12, 357–360. doi: 10.1038/nmeth.3317
Kwok, C. K., Marsico, G., and Balasubramanian, S. (2018). Detecting RNA G-quadruplexes (rG4s) in the transcriptome. Cold Spring Harb. Perspect. Biol. 10:a032284. doi: 10.1101/CSHPERSPECT.A032284
Lerner, L. K., and Sale, J. E. (2019). Replication of G Quadruplex DNA. Genes 10:95. doi: 10.3390/GENES10020095
Li, H., Handsaker, B., Wysoker, A., Fennell, T., Ruan, J., Homer, N., et al. (2009). The sequence alignment/map format and SAMtools. Bioinformatics 25, 2078–2079. doi: 10.1093/BIOINFORMATICS/BTP352
Li, X., Mei, H., Chen, F., Tang, Q., Yu, Z., Cao, X., et al. (2017). Transcriptome landscape of Mycobacterium smegmatis. Front. Microbiol. 8:2505. doi: 10.3389/FMICB.2017.02505
Liao, Y., Smyth, G. K., and Shi, W. (2014). featureCounts: an efficient general purpose program for assigning sequence reads to genomic features. Bioinformatics 30, 923–930. doi: 10.1093/BIOINFORMATICS/BTT656
Logsdon, M. M., and Aldridge, B. B. (2018). Stable regulation of cell cycle events in mycobacteria: insights From inherently heterogeneous bacterial populations. Front. Microbiol. 9:514. doi: 10.3389/FMICB.2018.00514
Marsico, G., Chambers, V. S., Sahakyan, A. B., McCauley, P., and Boutell, J. M., Antonio, M. di Antonio, M. D., and Balasubramanian, S. (2019). Whole genome experimental maps of DNA G-quadruplexes in multiple species. Nucleic Acids Res. 47, 3862–3874. doi: 10.1093/NAR/GKZ179
Maslowska, K. H., Makiela-Dzbenska, K., and Fijalkowska, I. J. (2019). The SOS system: A complex and tightly regulated response to DNA damage. Environ. Mol. Mutagen. 60, 368–384. doi: 10.1002/EM.22267
Mishra, S. K., Shankar, U., Jain, N., Sikri, K., Tyagi, J. S., Sharma, T. K., et al. (2019). Characterization of G-quadruplex motifs in espB, espK, and cyp51 genes of Mycobacterium tuberculosis as potential drug targets. Mol Ther Nucleic Acids 16, 698–706. doi: 10.1016/J.OMTN.2019.04.022
Namouchi, A., Gómez-Muñoz, M., Frye, S. A., Moen, L. V., Rognes, T., Tønjum, T., et al. (2016). The Mycobacterium tuberculosis transcriptional landscape under genotoxic stress. BMC Genomics 17, 1–13. doi: 10.1186/S12864-016-3132-1
Ojha, A., and Hatfull, G. F. (2007). The role of iron in mycobacterium smegmatis biofilm formation: the exochelin siderophore is essential in limiting iron conditions for biofilm formation but not for planktonic growth. Mol. Microbiol. 66, 468–483. doi: 10.1111/J.1365-2958.2007.05935.X
Okonechnikov, K., Conesa, A., and García-Alcalde, F. (2016). Qualimap 2: advanced multi-sample quality control for high-throughput sequencing data. Bioinformatics 32, btv566–btv294. doi: 10.1093/BIOINFORMATICS/BTV566
Ordonez, H., and Shuman, S. (2014). Mycobacterium smegmatis DinB2 misincorporates deoxyribonucleotides and ribonucleotides during templated synthesis and lesion bypass. Nucleic Acids Res. 42, 12722–12734. doi: 10.1093/NAR/GKU1027
Parekh, V. J., Niccum, B. A., Shah, R., Rivera, M. A., Novak, M. J., Geinguenaud, F., et al. (2019). Role of Hfq in genome evolution: instability of G-Quadruplex sequences in E. coli. Microorganisms 8:28. doi: 10.3390/MICROORGANISMS8010028
Pedersen, T. L. (2021). ggforce: Accelerating “ggplot2.” Available at: https://CRAN.R-project.org/package=ggforce (Accessed June, 2021).
Perrone, R., Lavezzo, E., Riello, E., Manganelli, R., Palù, G., Toppo, S., et al. (2017). Mapping and characterization of G-quadruplexes in mycobacterium tuberculosis gene promoter regions. Sci. Rep. 7, 1–11. doi: 10.1038/s41598-017-05867-z
Puig, S., Ramos-Alonso, L., Romero, A. M., and Martínez-Pastor, M. T. (2017). The elemental role of iron in DNA synthesis and repair. Metallomics 9, 1483–1500. doi: 10.1039/C7MT00116A
Quinlan, A. R., and Hall, I. M. (2010). BEDTools: a flexible suite of utilities for comparing genomic features. Bioinformatics 26, 841–842. doi: 10.1093/BIOINFORMATICS/BTQ033
R Core Team (2021). R: A Language and Environment for Statistical Computing. Available at: https://www.R-project.org/ (Accessed June, 2021).
Rand, L., Hinds, J., Springer, B., Sander, P., Buxton, R. S., and Davis, E. O. (2003). The majority of inducible DNA repair genes in Mycobacterium tuberculosis are induced independently of RecA. Mol. Microbiol. 50, 1031–1042. doi: 10.1046/J.1365-2958.2003.03765.X
Rha, S. Y., Izbicka, E., Lawrence, R., Davidson, K., Sun, D., Moyer, M. P., et al. (2000). Effect of telomere and telomerase interactive agents on human tumor and normal cell lines. Clin. Cancer Res. 6, 987–993.
Robinson, M. D., McCarthy, D. J., and Smyth, G. K. (2010). edgeR: a bioconductor package for differential expression analysis of digital gene expression data. Bioinformatics 26, 139–140. doi: 10.1093/BIOINFORMATICS/BTP616
Rodriguez, R., Miller, K. M., Forment, J. V., Bradshaw, C. R., Nikan, M., Britton, S., et al. (2012). Small-molecule–induced DNA damage identifies alternative DNA structures in human genes. Nat. Chem. Biol. 8, 301–310. doi: 10.1038/nchembio.780
Saha, T., Shukla, K., Thakur, R. S., Desingu, A., and Nagaraju, G. (2019). Mycobacterium tuberculosis UvrD1 and UvrD2 helicases unwind G-quadruplex DNA. FEBS J. 286, 2062–2086. doi: 10.1111/FEBS.14798
Saranathan, N., and Vivekanandan, P. (2019). G-Quadruplexes: more Than just a kink in microbial genomes. Trends Microbiol. 27, 148–163. doi: 10.1016/J.TIM.2018.08.011
Sauer, M., and Paeschke, K. (2017). G-quadruplex unwinding helicases and their function in vivo. Biochem. Soc. Trans. 45, 1173–1182. doi: 10.1042/BST20170097
Seung, K. J., Keshavjee, S., and Rich, M. L. (2015). Multidrug-resistant tuberculosis and extensively drug-resistant tuberculosis. Cold Spring Harb. Perspect. Med. 5:a017863. doi: 10.1101/cshperspect.a017863
Shao, X., Zhang, W., Umar, M. I., Wong, H. Y., Seng, Z., Xie, Y., et al. (2020). RNA G-quadruplex structures mediate gene regulation in bacteria. mBio 11, e02926–e02919. doi: 10.1128/MBIO.02926-19
Singh, A. (2017). Guardians of the mycobacterial genome: A review on DNA repair systems in mycobacterium tuberculosis. Microbiology 163, 1740–1758. doi: 10.1099/MIC.0.000578
Singh, A. (2018). Expanding the horizon of recombination repair in mycobacteria: identification and characterization of novel proteins. FASEB J. 32(Suppl. 1):lb1-925.9. doi: 10.1096/FASEBJ.2018.32.1_SUPPLEMENT.LB1
Singh, A., Vijayan, M., and Varshney, U. (2018). Distinct properties of a hypoxia specific paralog of single stranded DNA binding (SSB) protein in mycobacteria. Tuberculosis 108, 16–25. doi: 10.1016/J.TUBE.2017.10.002
Smid, M., van den Braak, R. R. J. C., van de Werken, H. J. G., van Riet, J., van Galen, A., de Weerd, V., et al. (2018). Gene length corrected trimmed mean of M-values (GeTMM) processing of RNA-seq data performs similarly in intersample analyses while improving intrasample comparisons. BMC Bioinformatics 19:236. doi: 10.1186/S12859-018-2246-7
Spiegel, J., Adhikari, S., and Balasubramanian, S. (2020). The structure and function of DNA G-Quadruplexes. Trends Chem. 2, 123–136. doi: 10.1016/J.TRECHM.2019.07.002
Sritharan, M. (2016). Iron homeostasis in Mycobacterium tuberculosis: mechanistic insights into siderophore-mediated iron uptake. J. Bacteriol. 198, 2399–2409. doi: 10.1128/JB.00359-16
Thakur, R. S., Desingu, A., Basavaraju, S., Subramanya, S., Rao, D. N., and Nagaraju, G. (2014). Mycobacterium tuberculosis DinG is a structure-specific helicase that unwinds G4 DNA: implications for targeting G4 DNA as a novel therapeutic approach. J. Biol. Chem. 289, 25112–25136. doi: 10.1074/JBC.M114.563569
Uson, M. L., Ordonez, H., and Shuman, S. (2015). Mycobacterium smegmatis HelY is an RNA-activated ATPase/dATPase and 3′-to-5′ helicase that unwinds 3′-tailed RNA duplexes and RNA:DNA hybrids. J. Bacteriol. 197, 3057–3065. doi: 10.1128/JB.00418-15
Wattam, A. R., Abraham, D., Dalay, O., Disz, T. L., Driscoll, T., Gabbard, J. L., et al. (2014). PATRIC, the bacterial bioinformatics database and analysis resource. Nucleic Acids Res. 42, D581–D591. doi: 10.1093/NAR/GKT1099
Wilke, C. O. (2020). cowplot: Streamlined Plot Theme and Plot Annotations for “ggplot2.” Available at: https://CRAN.R-project.org/package=cowplot (Accessed June, 2021).
Wu, R., Zheng, K., Zhang, J., Hao, Y., and Tan, Z. (2015). Formation of DNA:RNA hybrid G-Quadruplex in bacterial cells and its dominance over the Intramolecular DNA G-Quadruplex in mediating transcription termination. Angew. Chem. Int. Ed. 54, 2447–2451. doi: 10.1002/ANIE.201408719
Yadav, P., Kim, N., Kumari, M., Verma, S., Sharma, T. K., Yadav, V., et al. (2021). G-quadruplex structures in bacteria: biological relevance and potential as an antimicrobial target. J. Bacteriol. 203:e0057720. doi: 10.1128/JB.00577-20
Yan, L. (2021). ggvenn: Draw Venn Diagram by “ggplot2.” Available at: https://CRAN.R-project.org/package=ggvenn (Accessed June, 2021).
Yang, M., Aamodt, R. M., Dalhus, B., Balasingham, S., Helle, I., Andersen, P., et al. (2011). The ada operon of mycobacterium tuberculosis encodes two DNA methyltransferases for inducible repair of DNA alkylation damage. DNA Repair 10, 595–602. doi: 10.1016/J.DNAREP.2011.03.007
Keywords: mycobacteria, transcriptome, RNA, G4, ligands, BRACO-19, TMPyP4
Citation: Shitikov E, Bespiatykh D, Malakhova M, Bespyatykh J, Bodoev I, Vedekhina T, Zaychikova M, Veselovsky V, Klimina K, Ilina E and Varizhuk A (2022) Genome-Wide Transcriptional Response of Mycobacterium smegmatis MC2155 to G-Quadruplex Ligands BRACO-19 and TMPyP4. Front. Microbiol. 13:817024. doi: 10.3389/fmicb.2022.817024
Edited by:
Daisuke Miyoshi, Konan University, JapanReviewed by:
Guanhui Wu, Shanghai Roche Pharmaceutical Co., Ltd., ChinaNihar Ranjan, National Institute of Pharmaceutical Education and Research, India
Copyright © 2022 Shitikov, Bespiatykh, Malakhova, Bespyatykh, Bodoev, Vedekhina, Zaychikova, Veselovsky, Klimina, Ilina and Varizhuk. This is an open-access article distributed under the terms of the Creative Commons Attribution License (CC BY). The use, distribution or reproduction in other forums is permitted, provided the original author(s) and the copyright owner(s) are credited and that the original publication in this journal is cited, in accordance with accepted academic practice. No use, distribution or reproduction is permitted which does not comply with these terms.
*Correspondence: Egor Shitikov, ZWdvcnNodGt2QGdtYWlsLmNvbQ==