- Department of Preservation and Food Safety Technologies, Institute of Agrochemistry and Food Technology of the Spanish National Research Council (IATA-CSIC), Paterna, Spain
Wastewater discharge to the environment or its reuse after sanitization poses a concern for public health given the risk of transmission of human viral diseases. However, estimating the viral infectivity along the wastewater cycle presents technical challenges and still remains underexplored. Recently, human-associated crAssphage has been investigated to serve as viral pathogen indicator to monitor fecal impacted water bodies, even though its assessment as biomarker for infectious enteric viruses has not been explored yet. To this end, the occurrence of potentially infectious norovirus genogroup I (GI), norovirus GII, hepatitis A virus (HAV), rotavirus A (RV), and human astrovirus (HAstV) along with crAssphage was investigated in influent and effluent water sampled in four wastewater treatment plants (WWTPs) over 1 year by a PMAxx-based capsid integrity RT-qPCR assay. Moreover, influent and effluent samples of a selected WWTP were additionally assayed by an in situ capture RT-qPCR assay (ISC-RT-qPCR) as estimate for viral infectivity in alternative to PMAxx-RT-qPCR. Overall, our results showed lower viral occurrence and concentration assessed by ISC-RT-qPCR than PMAxx-RT-qPCR. Occurrence of potentially infectious enteric virus was estimated by PMAxx-RT-qPCR as 88–94% in influent and 46–67% in effluent wastewaters with mean titers ranging from 4.77 to 5.89, and from 3.86 to 4.97 log10 GC/L, with the exception of HAV that was sporadically detected. All samples tested positive for crAssphage at concentration ranging from 7.41 to 9.99 log10 GC/L in influent and from 4.56 to 6.96 log10 GC/L in effluent wastewater, showing higher mean concentration than targeted enteric viruses. Data obtained by PMAxx-RT-qPCR showed that crAssphage strongly correlated with norovirus GII (ρ = 0.67, p < 0.05) and weakly with HAstV and RV (ρ = 0.25–0.30, p < 0.05) in influent samples. In effluent wastewater, weak (ρ = 0.27–0.38, p < 0.05) to moderate (ρ = 0.47–0.48, p < 0.05) correlations between crAssphage and targeted viruses were observed. Overall, these results corroborate crAssphage as an indicator for fecal contamination in wastewater but a poor marker for either viral occurrence and viral integrity/infectivity. Despite the viral load reductions detected in effluent compared to influent wastewaters, the estimates of viral infectivity based on viability molecular methods might pose a concern for (re)-using of treated water.
Introduction
The microbiological analysis of raw and treated wastewater has become a hot topic in recent years due to the emerging concerns on the disease/pathogen epidemiological tracking (known as wastewater-based epidemiology, WBE) and on the safety of wastewater discarding and reusing. Monitoring of wastewater has already been implemented with success for a long time on the tracking of chemical pollutants, drug spread within communities, and antibiotic resistance genes (ARGs) (Mercan et al., 2019; de Oliveira et al., 2020). Over the last years, molecular analysis detection of viruses in wastewater samples has allowed disease surveillance as for poliovirus during the global eradication program (Asghar et al., 2014), re-emerging zoonotic pathogens such as hepatitis E virus (Miura et al., 2016; Cuevas-Ferrando et al., 2020), human enteric viruses (Hellmér et al., 2014; Prevost et al., 2015; Santiso-Bellón et al., 2020), and very recently severe acute respiratory syndrome coronavirus 2 (SARS-CoV-2) (Bivins et al., 2020b; Randazzo et al., 2020).
Human enteric viruses are the causative agents of viral gastroenteritis, hepatitis, and other diseases which predominantly transmit through the fecal–oral route (Oude Munnink and van der Hoek, 2016). Viral spread is mainly associated to person-to-person contact and ingestion of contaminated food and waters since enteric viruses are shed at huge concentrations of up to 1013 particles per gram by both symptomatic and asymptomatic individuals (Carter, 2005; Bosch et al., 2008; Okoh et al., 2010). Group A rotavirus (RV), norovirus genogroups I (GI) and II (GII), hepatitis A virus (HAV), and human astrovirus (HAstV) are the main causative agents of water−associated viral gastroenteritis and hepatitis outbreaks worldwide (Bosch et al., 2008).
Human enteric viruses show higher resistance to decontamination treatments generally applied by wastewater treatment plants (WWTPs) such as chlorination and UV radiation (Gerba et al., 2018). Consequently, reclamation in WWTP does not usually achieve total removal of viral particles from sewage (Ramírez-Castillo et al., 2015) and they are commonly found in effluent water samples analyses (Sano et al., 2016; Farkas et al., 2018).
In the context of the exacerbated water shortage, the use of reclaimed water for irrigation, recreational, or industrial applications has become a strategy to tackle this critical problem (Barcelo and Petrovic, 2011). From a public health perspective, monitoring not only the occurrence but also the infectivity of viral human pathogens may permit to estimate the adequacy of current water reclamation systems. To approach this issue, targeting specific human viral pathogens or a properly selected indicator constitutes alternative strategies to pursue.
Traditionally, methods based on cell culture have been used in clinical virology to test for viral infectivity, which show considerable limitations when applied in environmental samples because of the co-contamination of multiple virus species, the absence of permissive cell lines for certain viruses, and the cytotoxic effect of wastewater in cell culture (Gerba et al., 2018; Randazzo et al., 2018a). In recent years, there has been an enormous progress in detecting enteric viruses in water samples by real-time polymerase chain reaction (qPCR) methods (Katayama et al., 2008; Simmons and Xagoraraki, 2011; Farkas et al., 2018). Even so, molecular assays do not discriminate between viruses with infective capacity, inactivated viruses, and free genome. To overcome this limitation, viability markers and binding assays have been coupled to qPCR detection to evaluate the integrity of the viral capsid as estimate for viral infectivity also in water samples (Parshionikar et al., 2010; Kim et al., 2011; Coudray-Meunier et al., 2013; Prevost et al., 2016; Randazzo et al., 2016, 2018b,c; López-Gálvez et al., 2018; Tian et al., 2018; Leifels et al., 2019, 2020; Shirasaki et al., 2020; Canh et al., 2021a). Of note, such approach has been very recently implemented for SARS-CoV-2 detection in wastewater (Canh et al., 2021b; Cuevas-Ferrando et al., 2021).
Capsid integrity is a strong indicator of virus infectivity, as virions with an accessible genome yield reduced qPCR signals, improving the molecular estimation of virions (Leifels et al., 2020). As an alternative, fecal indicator bacteria (FIB) have traditionally been used to estimate fecal contamination of environmental waters, even though surveillance data demonstrated FIB may not always correlate with human enteric viruses (Sano et al., 2016; Amarasiri et al., 2017; Sidhu et al., 2017; Gerba et al., 2018). Recently, viruses such as crAssphage (cross-assembly phage), tobacco mosaic virus (TMV), and pepper mild mottle virus (PMMoV) have been suggested as indicators for either human fecal contamination and viral human pathogen removal throughout wastewater reclamations in the WWTPs (Kitajima et al., 2014; García-Aljaro et al., 2017; Farkas et al., 2019, 2020; Symonds et al., 2019; Bivins et al., 2020a; Tandukar et al., 2020; Wu et al., 2020).
Thus, the primary goal of this study was defining the occurrence of infectious human enteric viruses in influent and effluent wastewater by rapid molecular methods and, secondly, testing the hypothesis of whether crAssphage would serve as indicator for viral infectivity.
To this end, we monitored, by PMAxx-RT-qPCR over a 1-year period, the occurrence of intact capsid potentially infectious RNA enteric viruses (i.e., norovirus GI and GII, HAV, RV, and HAstV) and crAssphage in influent and effluent water samples collected from four WWTPs in the Valencian Region (Spain). Moreover, we compared in situ capture RT-qPCR (ISC-RT-qPCR) and PMAxx-RT-qPCR assays as alternative estimates for viral infectivity using influent and effluent samples of a selected WWTP longitudinally over a year. Finally, this work contributes on the expansion of the actual data pool on spatiotemporal viral monitoring studies in raw and treated wastewater and increases the significance of qPCR results for public health, economic, and QMRA purposes.
Materials and Methods
Sampling Site and Sample Collection
Influent (n = 48) and effluent (n = 48) wastewater samples were collected regularly each month (from November 2018 to October 2019) from four WWTPs located in the Valencian region in Spain (Figure 1). Reclamation processes applied in each sampled wastewater treatment plant are described in Table 1. Samples were grabbed early in the morning (7–12 a.m.) by collecting 500–1,000 ml of water in sterile HDPE plastic containers (Labbox Labware, Spain). Collected samples were transferred on ice to the laboratory, kept refrigerated at 4°C and concentrated within 24 h as reported below.
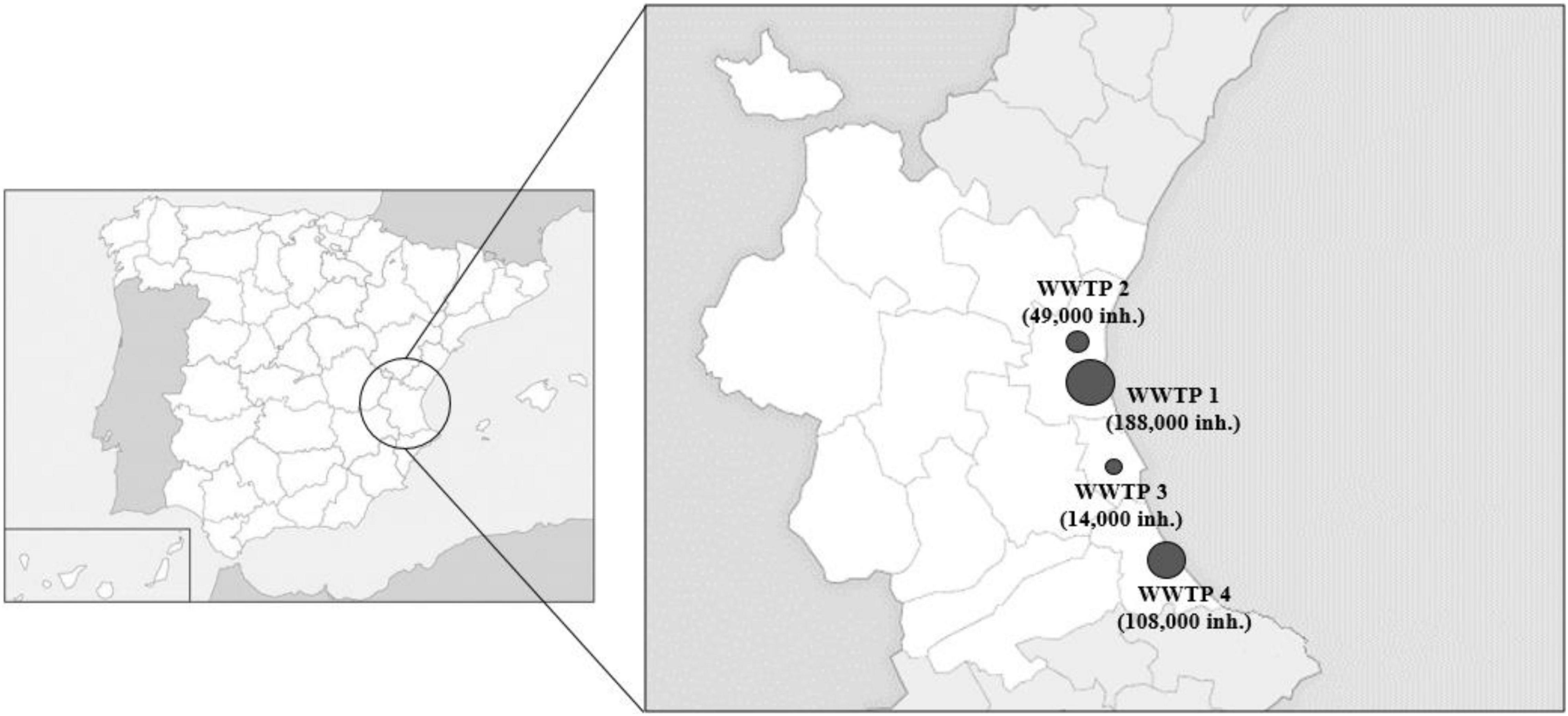
Figure 1. Geographical localization of wastewater treatment plants in Valencian region (Spain) and their population coverages included in the study. Diamonds on the figure are scaled according to population (inhabitants, inh).
Escherichia coli Counts and Physicochemical Characterization of Influent and Effluent Wastewater Samples
Influent and effluent wastewater samples were characterized by determining significant physicochemical parameters. Escherichia coli was determined as the Most Probable Number (MPN)/100 ml according to EN ISO 9308-2 (2014). The total alkalinity (referred as TA) was determined by titration by measuring the UV-Vis absorbance following the methyl orange method and expressed in mg/L CaCO3. The chemical oxygen demand (COD, mg/L O2) was determined by measuring the UV-Vis absorbance on an AP3900 laboratory robot coupled with a DR3900 spectrophotometer (Hach) following the potassium dichromate method. Total suspended solids (TSS) were determined by filtration by using glass fiber filters and results expressed in mg/L. Turbidity (Nephelometric Turbidity Unit, NTU) were determined by TU5200 Laser Turbidimeter and the oxidation-reduction potential (ORP, expressed in mV) by HQ 40D digital multi meter (Hach, United Kingdom). Physicochemical analyses and E. coli counts were performed at GAMASER laboratories (Valencia, Spain).
Virus Suspensions
Feces positive for norovirus GI, norovirus GII, and HAstV (courtesy of Dr. Buesa from Hospital Clínico Universitario, University of Valencia, Spain) were resuspended (10%, wt/vol) in phosphate-buffered saline (PBS) containing 2 M NaNO3 (Panreac, Spain), 1% beef extract (Conda, Spain), and 0.1% Triton X-100 (Thermo Fisher Scientific, Spain) (pH 7.2), vortexed and centrifuged at 1,000 × g for 5 min. The supernatants were extracted, the RNA stored at -80°C in aliquots to be used as positive amplification controls. The human RV strain Wa (ATCC VR-2018), the cytopathogenic HM-175 strain of HAV (ATCC VR-1402), and mengovirus vMC0 (CECT 100000) were propagated in MA-104, FRhK, and HeLa cell monolayers, respectively. Semipurified stocks were thereafter produced in the same cells by low-speed centrifugations of infected cell lysates (3,000 × g for 20 min). RNA extracted from infected cell lysates was used as positive amplification control and mengovirus (MgV) was used as process control as suggested in ISO 15216-2:2019 (microbiology of the food chain) for sample concentration validation (Randazzo et al., 2019).
Wastewater Concentration
Influent and effluent water samples were artificially inoculated with approximately 7 log10 PCR units (PCRU)/L of MgV, as process control. Samples were concentrated through an aluminum hydroxide adsorption-precipitation method (AAVV, 2018; Randazzo et al., 2019). Briefly, 200 ml of sample was adjusted to pH 6.0 and Al(OH)3 precipitate formed by adding 1 part 0.9 N AlCl3 solution to 100 parts of sample. Then, pH was readjusted to 6.0 and sample mixed using an orbital shaker at 150 rpm for 15 min at room temperature. Next, viruses were collected by centrifugation at 1,700 × g for 20 min. The pellet was resuspended in 10 ml of 3% beef extract pH 7.4, and samples were shaken for 10 min at 150 rpm. Finally, the concentrate was recovered by centrifugation at 1,900 × g for 30 min and the pellet was resuspended in 1 ml of PBS and stored at -80°C.
Viral Capsid Integrity Assays in Wastewater Samples
To assess the intact capsid condition of enteric viruses in influent and effluent wastewater, a main protocol based on capsid permeability to PMAxx viability dye (PMAxx-RT-qPCR) was used for all wastewater samples. Besides, an alternative method based on the specific binding ability to porcine gastric mucin (PGM) was run in parallel in samples from WWTP4 in order to evaluate its unreported capsid integrity discrimination efficiency on wastewater matrices.
For PMAxx-RT-qPCR, a previously optimized protocol was applied prior to nucleic acid extraction and RT-qPCR detection (Randazzo et al., 2016, 2018c; López-Gálvez et al., 2018). Briefly, the photoactivatable dye PMAxx™ (Biotium, United States) was added to 150 μl of each concentrated water sample at 50 μM along with 7.7 mmol/L Triton 100-X (Thermo Fisher Scientific, Spain) and incubated in the dark at room temperature for 10 min at 150 rpm. Later, samples placed in DNA LoBind 1.5 ml tubes (Eppendorf, Germany) were exposed to photoactivation using a Led-Active Blue system (GenIUL, Spain) for 15 min, and viral RNA was extracted and analyzed as described hereafter.
The in situ capture assay (ISC-RT-qPCR) was performed as previously reported (Wang and Tian, 2014; Falcó et al., 2019) in 24-well plates with some modifications. Briefly, each well was coated with 100 μl of PGM (100 μg/ml) in carbonate-bicarbonate buffer (pH 9.6) at 37°C for 1 h and then incubated overnight at 4°C. After being washed five times with 300 μl of PBS containing 0.05% Tween 20 and 0.3% BSA (PBSTB), wells were blocked with 300 μl of 3% BSA in PBS at 37°C for 2 h. Next, wells were washed five times with PBSTB, and 300 μl of concentrated water samples and controls were added to the 24-well plate and incubated at 37°C overnight. Untreated viral suspensions and those treated at 99°C for 5 min were used as positive and negative controls, respectively. Finally, after washing five times with PBSTB, 100 μl of lysis buffer from the NucleoSpin RNA virus kit (Macherey-Nagel GmbH and Co., Germany) was added to each well. Then, viral RNA was extracted and analyzed as described hereafter.
RNA Extraction and Virus Quantification
Nucleic acids from each water sample were extracted following the NucleoSpin® RNA virus kit (Macherey-Nagel GmbH and Co., Germany) manufacturer’s instructions with some modifications. In short, 150 μl of each concentrated sample was added with 25 μl Plant RNA Isolation Aid (Ambion, United Kingdom) and 600 μl of lysis buffer from the NucleoSpin® RNA virus kit and subjected to pulse-vortexing. Then, the homogenate was centrifuged for 5 min at 10,000 × g for debris removal. The supernatant was subsequently processed according to the manufacturer’s instructions. Presence of norovirus GI and GII, HAV, HAstV, RV, and MgV was detected in 96-well plates using the RNA UltraSense One-Step kit (Invitrogen SA, United States), while crAssphage occurrence was performed using the qPCR Premix Ex Taq™ kit (Takara Bio Inc.) on the LightCycler® 480 instrument (Roche Diagnostics, Switzerland). Moreover, undiluted and 10-fold diluted nucleic acid were tested in duplicate to check for inhibitors.
Different controls were used in all assays, including a concentration control to monitor the process efficiency of each sample (spiked MgV), a negative nucleic acid extraction control, and positive and negative RT-qPCR controls. Primers, probes, and RT-qPCR conditions used in this study are listed in Supplementary Table 1.
Standard curves were determined using the Public Health England (PHE) Reference Materials for Microbiology for norovirus GI (batch number 0122-17), norovirus GII (batch number 0247-17), and HAV (batch number 0261-2017) and reported as genomic copies (GC), while standard curves for RV, MgV, and HAstV were generated by amplifying 10-fold serial dilutions of viral suspensions in quintuplicates and calculating the number of PCR units (PCRU). Standard DNA material for crAssphage standard curve generation relied on a customized gBlock gene fragment (Integrated DNA Technologies, United States) containing target sequence for CPQ_064 crAssphage primers set (Stachler et al., 2017). All (RT)-qPCR determinations followed quality control and quality assurance criteria included in EMMI Guidelines (Borchardt et al., 2021).
Statistical Analyses
Statistical data processing was performed using GraphPad Prism (GraphPad Software, La Jolla, CA, United States). The results were not normally distributed, so non-parametric Spearman’s rank correlation analyses were performed to evaluate the strength of relationship between viral titers alone, and in combination with physicochemical parameters. In all cases, values of p < 0.05 were deemed significant. Effects of wastewater treatment plant’s covered population, flow intake, and tertiary treatment (UV or chlorination) on crAssphage titers were analyzed by using the GraphPad Prism software. Correlation analyses among potentially infectious enteric viruses, crAssphage, E. coli, and physicochemical parameters were performed using viral loads, calculated as the product of viral titer per water flow for each WWTP. No log transformation was applied on data as Spearman’s rank correlation is invariant under monotone transformations like the logarithm.
Results
Occurrence of Intact Capsid Enteric Viruses and CrAssphage in Influent and Effluent Wastewater Over a 1-Year Period
Influent and effluent wastewater samples from four WWTPs located in the Valencian region (Spain) were processed by PMAxx-RT-qPCR over a 12-month period (2018–19) to determine the occurrence of potentially infectious norovirus GI, norovirus GII, HAV, RV, and HAstV, along with crAssphage (Figure 2).
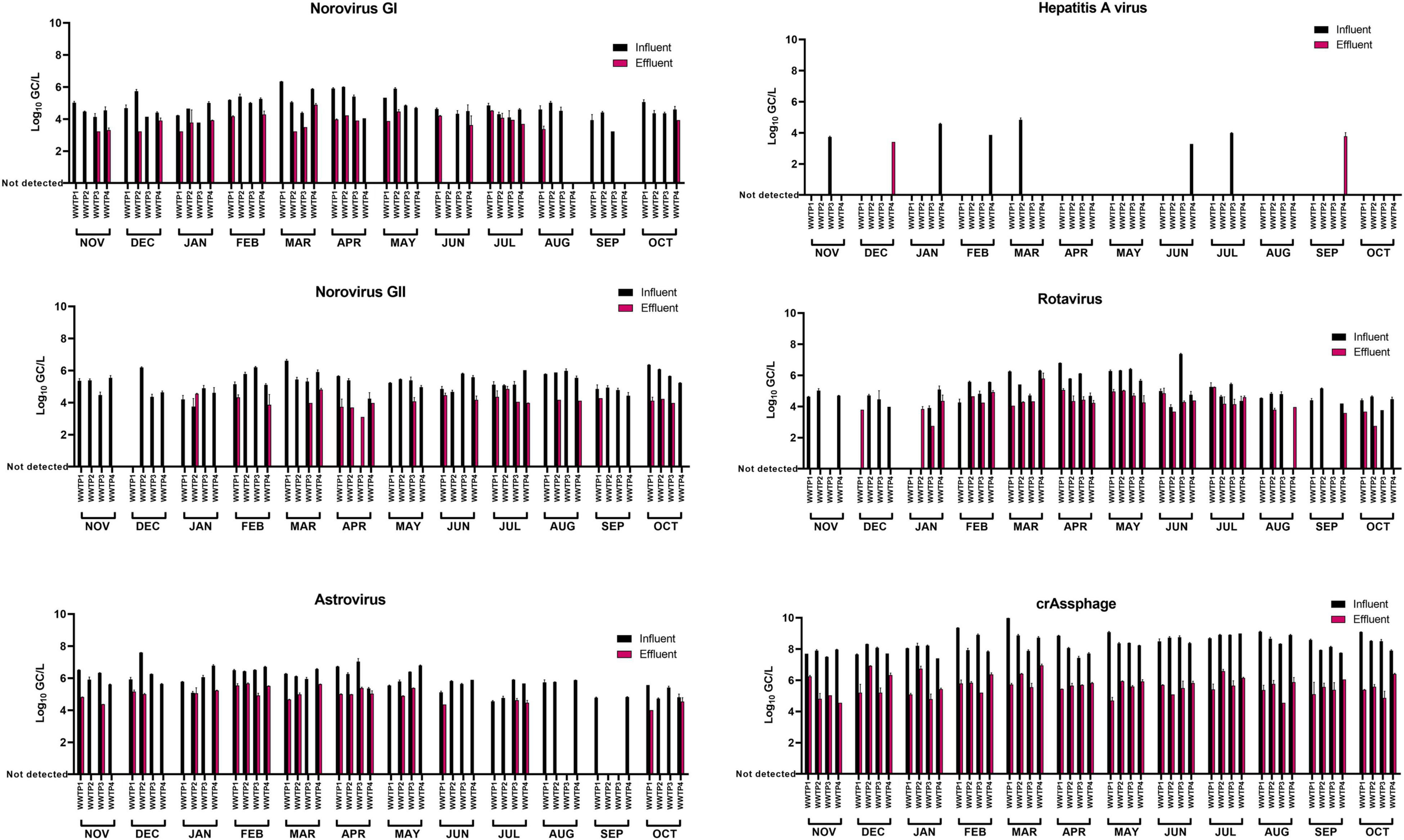
Figure 2. Occurrence of intact capsid enteric viruses and crAssphage in influent and effluent wastewater samples over a 1-year period. Capsid integrity was assessed by PMAxx-RT-qPCR. Colored bars represent mean Log10 GC/L values of two technical RT-qPCR replicates for each concentrated sample. Error bars indicate standard deviation.
It is worth to report that preliminary spiking experiments using murine norovirus (MNV, surrogate for human norovirus) and HAV assessed the effect of the wastewater concentration method on viral infectivity. According to the determination of the tissue culture infectious dose (TCID50/ml), no significant differences (p > 0.05) were observed among spiked and concentrated titers for both tested virus (data not shown).
The recoveries of MgV, spiked as viral process control, ranged between 1.18 and 37.80% (Supplementary Table 2); thus, results of targeted viruses were validated according to Haramoto et al. (2018) and the criteria included in the ISO 15216-1:2017 (recovery of control ≥1%). Viral titers of targeted viruses were not adjusted depending on the recovery of the concentration control (MgV) as back-calculation is not recommended (Haramoto et al., 2018). Norovirus GI, norovirus GII, and RV titers were 4.77 ± 0.65, 5.28 ± 0.63, and 5.08 ± 0.85 log10 GC/L in influent samples, and 3.86 ± 0.45, 4.13 ± 0.38, and 4.28 ± 0.64 log10 GC/L in effluent samples, respectively. HAstV showed the highest mean viral concentration among the five enteric RNA viruses in both influent (5.89 ± 0.68 log10 GC/L) and effluent (4.97 ± 0.43 log10 GC/L) samples. Moreover, HAstV was detected in 93.75% of influent water samples and in 50.0% of the effluent samples (Table 2). Overall, 93.8, 95.8, and 87.5% of influent samples (n = 48) and 52.1, 45.8, and 66.7% of effluent samples (n = 48) were positive for norovirus GI, norovirus GII, and RV, respectively. Finally, HAV was detected in 12 and 4.17% of the influent and effluent samples, respectively, and showed the lowest concentrations of 4.05 ± 0.56 log10 GC/L in influent samples and 3.60 ± 0.25 log10 GC/L in effluent samples. CrAssphage showed concentrations up to 3–4 log10 GC/L higher than targeted enteric RNA viruses ranging from 7.41 to 9.99 log10 GC/L in influent and from 4.56 to 6.96 log10 GC/L in effluent water samples, respectively. All samples tested positive for crAssphage and a mean decrease of 2.73 ± 0.68 log10 GC/L was observed in effluent compared to influent samples.
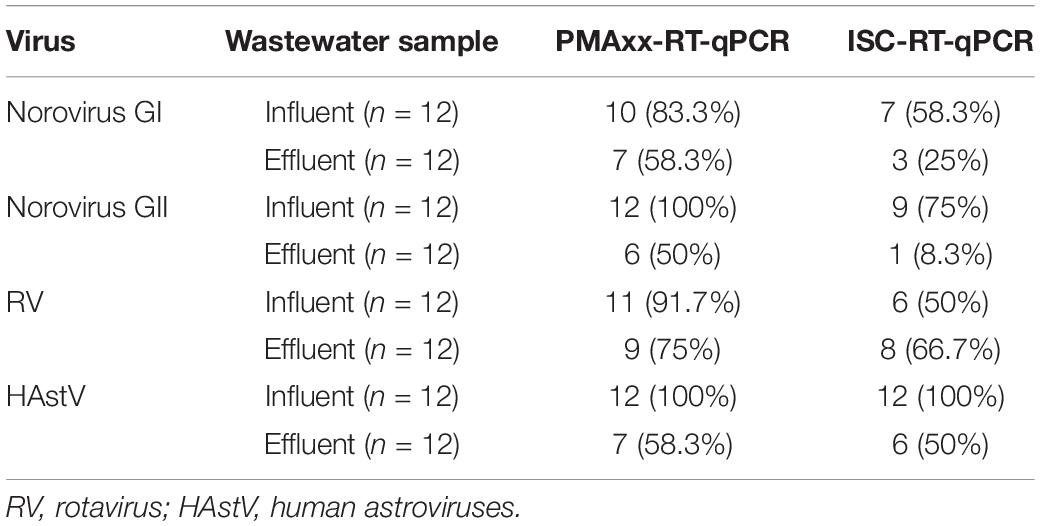
Table 2. Positive samples and percentiles of intact capsid enteric viruses assessed by PMAxx-RT-qPCR and in situ capture (ISC-)RT-qPCR assays in influent and effluent wastewater samples collected over a year from a selected wastewater treatment plant (WWTP4).
Considering enteric virus, our data showed mean log removals of 2.58, 3.70, 2.39, and 3.08 for norovirus GI, norovirus GII, RV, and HAstV, respectively (Figure 3).
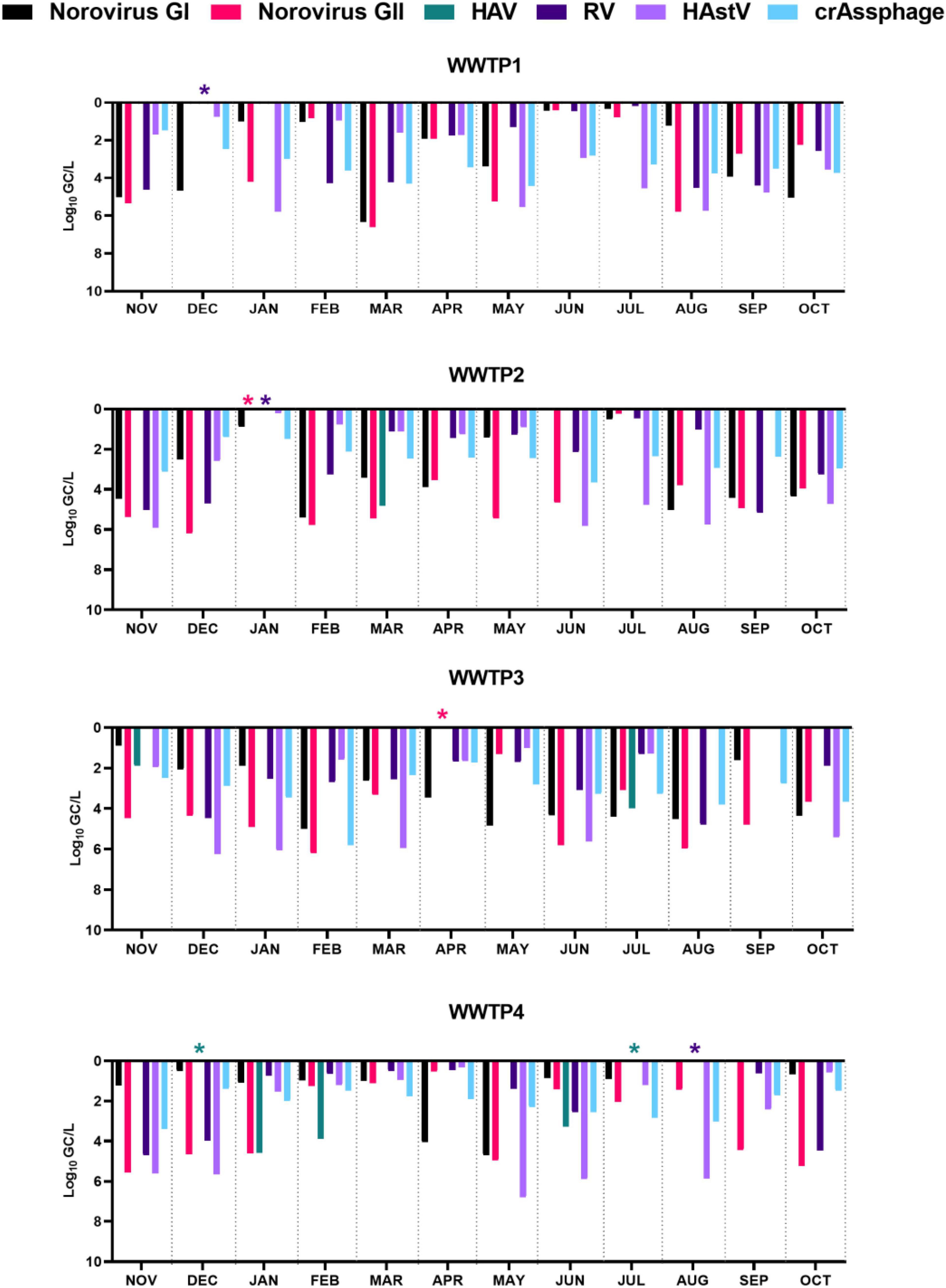
Figure 3. Enteric virus and crAssphage mean log removal (Log10 GC/L) for each wastewater treatment plant (WWTP) over a 1-year period. HAV, hepatitis A virus; RV, rotavirus; HAstV, human astroviruses; asterisks (*) indicate complete viral removal as viral load was higher in effluent than in influent wastewaters; missing bars are used for no detected virus in influent wastewaters.
Viral removal separately calculated according to tertiary treatment, indicated log decreases of 3.65, 2.37, and 3.18 for norovirus GII, RV, and HAstV in UV-treated effluent wastewater (WWTP1 and WWTP3). Chlorination treatments in WWTP2 and WWTP4 determined log removals of 2.42, 2.97, and 3.75 for norovirus GII, RV, and HAstV. Viral removal differed between UV and chlorination showing 3.00 and 3.25 mean log reductions for UV, and 2.16 and 2.31 for chlorination for norovirus GI and crAssphage, respectively (Figure 3). None of the targeted viruses showed a sharp seasonal pattern (Figure 2). Extended data on viral quantification are presented in Supplementary Table 2.
Comparing PMAxx-RT-qPCR and ISC-RT-qPCR Assays
In order to assess the efficiency of two alternative capsid integrity assays, PMAxx-RT-qPCR, and ISC-RT-qPCR were compared for detecting potential infectious norovirus GI, norovirus GII, HAstV, and RV in wastewater samples collected from a selected WWTP (n = 24). HAV was not tested by ISC-RT-qPCR because of its sporadic detection.
Overall, ISC-RT-qPCR provided lower estimates of viral occurrence than PMAxx-RT-qPCR for all tested viruses, except for HAstV, that showed 100% of positive samples in influent samples regardless of the capsid integrity assay applied (Table 2). Specifically, norovirus GI, norovirus GII, RV, and HAstV were detected by ISC-RT-qPCR in 58, 75, 50, and 100% of influent and in 25, 8, 67, and 50% of the effluent water samples. On the other hand, PMAxx-RT-qPCR estimated the occurrence of norovirus GI, norovirus GII, RV, and HAstV in 94, 96, 88, and 94% of influent and in 52, 46, 67, and 50% of effluent samples.
Regarding viral concentration, viral titers based on PMAxx-RT-qPCR assay resulted higher than those obtained by ISC-RT-qPCR in 93.75% determinations (Supplementary Figure 1).
Escherichia coli Counts and Physicochemical Parameters
The E. coli counts and physicochemical parameters of influent and effluent wastewater samples are summarized in Supplementary Table 3. E. coli ranged from 3.96 to 8.19 log10 MPN/100 ml and from below the detection limit to 5.96 log10 MPN/100 ml in influent and effluent samples, respectively. Alkalimetric titration ranged from 58.30 to 744 mg/L CaCO3 and from 44.24 to 828 mg/L CaCO3 in influent and effluent samples, respectively. COD ranged from 28.7 to 5,768 and from 11.6 to 108 mg/l O2 in influent and effluent samples, respectively. Suspended solids ranged from 69.2 to 582.3 mg/l, and from 0.9 to 63.6 mg/l in influent and effluent samples, respectively. Turbidity values ranged from 0 to 247 units in influent and from 0 to 30.02 units in effluent samples. The redox potential ranged from 1.9 to 270.4 and from 1.2 to 224 mV in influent and effluent samples, respectively.
CrAssphage as Fecal Viral Contamination Indicator of Potentially Infectious Enteric Viruses in Wastewater Samples
To further investigate the relationship among crAssphage, potentially infectious enteric virus, and physicochemical wastewater parameters, data sets were subjected to correlation analyses (Figure 4). Specifically, Spearman’s rank correlation rho coefficients (ρ) were calculated for intact capsid viral loads (viral titer × water flow) detected by PMAxx-RT-qPCR, E. coli counts, and physicochemical parameters in both influent (n = 48) and effluent (n = 48) wastewater samples (Figure 4). Resulting ρ coefficients are described through this work as follows: weak correlation (0.2–0.39), moderate correlation (0.4–0.59), strong correlation (0.6–0.79), and very strong correlation (0.8–1). In influent waters, crAssphage showed strong correlation with intact capsid norovirus GII (ρ = 0.67), moderate correlation with intact capsid norovirus GI (ρ = 0.40), and weak correlation with HAstV, RV, and E. coli (ρ = 0.25–0.30). Among enteric viruses, a moderate correlation resulted between norovirus GI and norovirus GII (ρ = 0.56). None to poor correlations resulted among enteric viruses and physicochemical parameters. When analyzing effluent wastewater samples, crAssphage showed moderate correlation with E. coli (ρ = 0.54) and intact capsid HAstV (ρ = 0.48) and norovirus GI (ρ = 0.47). Weak correlations resulted between crAssphage and RV (ρ = 0.38) and norovirus GII (ρ = 0.34). In contrast, E. coli displayed no correlation with any of the tested enteric viruses in effluent wastewater samples (ρ = 0.01–0.15).
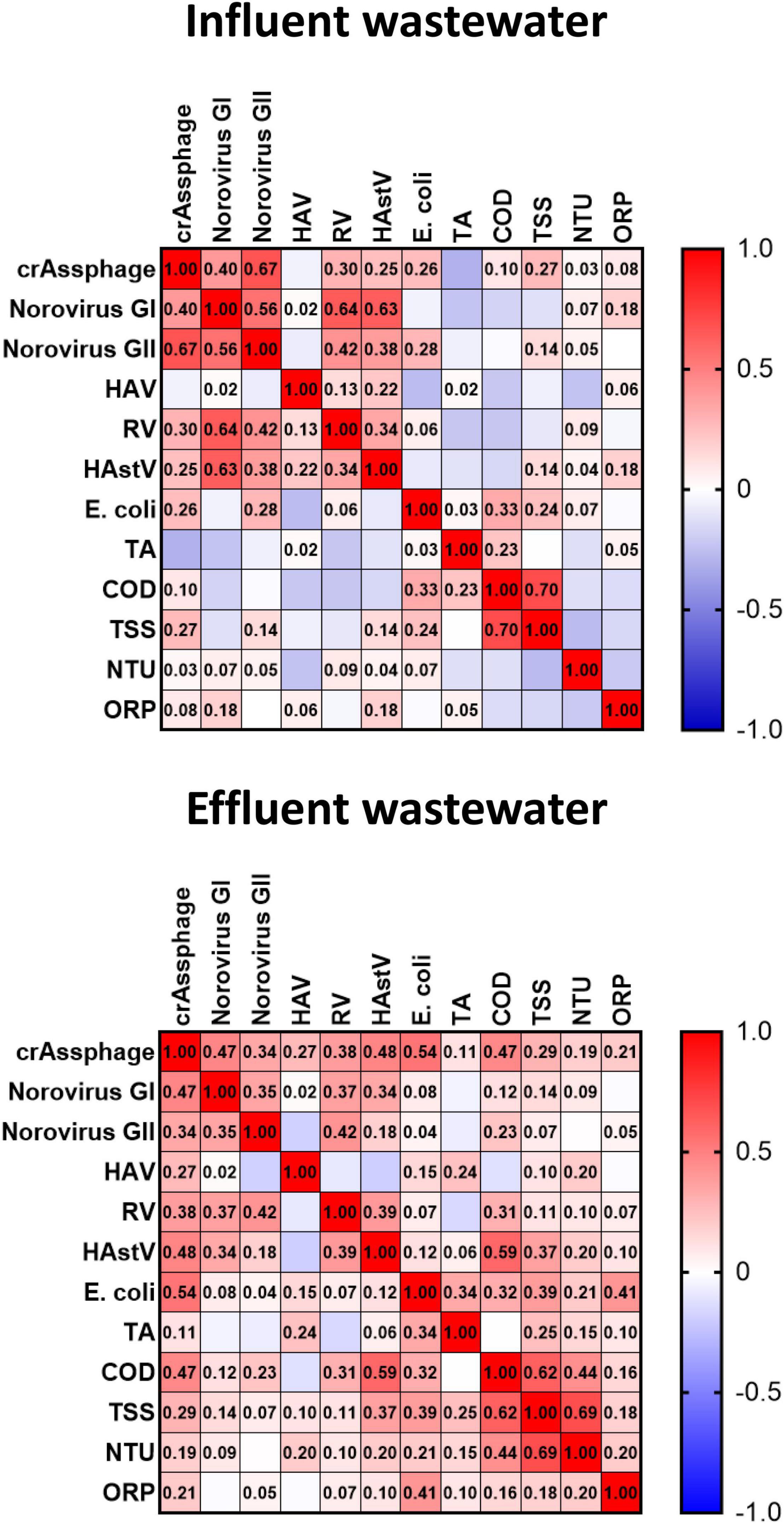
Figure 4. Spearman’s rho coefficients (ρ) of correlation analyses of intact capsid enteric viruses loads, crAssphage, and physicochemical parameters in influent and effluent wastewaters. TA, total alkalinity; COD, chemical oxygen demand; TSS, total suspended solids; NTU, turbidity; ORP, oxidation-reduction potential.
Effect of Wastewater Treatment Plant Characteristics on CrAssphage Load
The effect of key characteristics (population coverage, flow intake, and tertiary treatment) (Figure 1 and Table 1) on crAssphage load was independently evaluated for each WWTP.
CrAssphage load levels in influent (p = 0.039) and effluent (p = 0.007) wastewater statistically differed among WWTPs. Univariate results showed that variability of crAssphage load was statistically dependent on population coverage and on flow intake (p < 0.05) for influent wastewater samples. Interestingly, crAssphage concentration in effluent samples was found to significantly differ depending on the tertiary treatment (p = 0.016), suggesting that UV might be more efficient than chlorination for crAssphage removal. This was also observed for norovirus GI, but not for the remaining enteric virus (Figure 3).
Discussion
The reuse of treated wastewater and its discharge into the environment poses a challenge for public health, as reclamation treatments needs to be adequate to provide water that is suitable for its intended purpose (e.g., irrigation, recreational, or drinking water). Governments and the scientific community agree on the need for monitoring the viral population in wastewater even though there is still much uncertainty on the analytical protocols to use as well as the load reduction needed for ensuring a minimal risk from exposure to reclaimed water (Gerba et al., 2018; Fenaux et al., 2019).
Capsid integrity is a strong indicator of virus infectivity and can be a worthy tool to adjust existent workflows and qPCR procedures to indicate the capability of viruses to infect humans, thus enhancing risk assessment inferred from monitoring programs (Leifels et al., 2020). However, the present investigation did not address the question on how to use data based on capsid integrity techniques as input for quantitative risk assessment; this query needs to be specifically explored in future work. A first step into this direction could be determining the relationships of viral infectious titers estimated by capsid integrity techniques and dose response resulting from clinical trials or known outbreaks.
Presence of Potentially Infectious Enteric Viruses and Indicators in Wastewaters
This study provides additional insights on the quantitative occurrence of intact capsid enteric viruses in influent and effluent samples, and their correlation with crAssphage as a proposed viral water quality indicator.
We repetitively detected potentially infectious enteric viruses, including norovirus GI, norovirus GII, HAstV, and RV, in both influent and effluent in four different WWTPs over a year. This was further confirmed analyzing longitudinally upstream and downstream wastewater of a selected WWTP by two alternative capsid integrity assays, PMAxx-RT-qPCR and ISC-RT-qPCR, even though with different percentages (Table 2). Such release of human enteric viruses in effluent wastewater is not surprising as viral infectivity has been advised using different viability dye pretreatments (Gyawali and Hewitt, 2018; Randazzo et al., 2019; Canh et al., 2021a) and definitively demonstrated by cell culture (Simmons and Xagoraraki, 2011). However, comparing the viral titers determined by capsid integrity assays among WWTPs may not be conclusive due to different ratios of infectivity characterizing each population served by the sewerage system. This aspect could be additionally hindered for effluent wastewater samples exposed to different reclamation treatments (e.g., UV vs. chlorine) that distinctively affect viral morphology (e.g., nucleic acid vs. capsid), finally leading to diverse estimate of infectivity by capsid-integrity methods (Leifels et al., 2019).
Interestingly, a PMA-based capsid integrity assay was recently applied to assess the potential infectivity of novel HAV strains in treated wastewater in South Africa for which cell culture techniques may result to be not permissive (Rachida and Taylor, 2020).
Our results show that titers of viral particles with intact capsid in influent samples are comparable to those previously determined by RT-qPCR alone (Da Silva et al., 2007; Katayama et al., 2008; Kitajima et al., 2014; Montazeri et al., 2015; Haramoto et al., 2018), which suggests a high proportion of potentially infectious viruses, as expected.
However, capsid integrity RT-qPCR assays may not sharply discriminate infectious and inactivated viruses when subtle capsid alterations or genome damage occur because of the limited access to free RNA, the interaction with other compounds (e.g., organic acids), and the ineffective photoactivation (e.g., due to suspended solids, turbidity). These factors could differently affect capsid integrity RT-qPCR assays especially in complex matrices, such as wastewater, finally explaining the lower estimates for viral infectivity resulted from ISC-RT-qPCR compared to PMAxx-RT-qPCR. Thus, our findings further corroborate that PMAxx-RT-qPCR generally overestimate infectious viral particles (Leifels et al., 2015, 2020; López-Gálvez et al., 2018; Randazzo et al., 2018b, 2019). Nonetheless, capsid integrity RT-qPCRs better assess the potential risk of viral infection by providing more accurate information than conventional RT-qPCR alone that should be interpreted as a conservative approach.
Reduction of Potentially Infectious Enteric Viruses and Indicators During Wastewater Treatments
We observed reductions of 2–3 log10 on average between upstream and downstream wastewater, which do not comply with the most recent European legislation. Specifically, a ≥ 6 log10 decrease of rotavirus, total coliphages, or at least one of them (F-specific or somatic coliphages) is indicated to validate monitoring programs of reclaimed water used for agricultural irrigation (Regulation (EU) 2020/741, 2020). However, specific guidelines should be defined globally as pointed out by the scientific community and water operators (Sano et al., 2016; Gerba et al., 2017).
In recent years, crAssphage has emerged as viral water quality indicator because of its specificity to human fecal pollution, its high concentrations in sewage, and its global presence (Farkas et al., 2019; Bivins et al., 2020a; Honap et al., 2020). Interestingly, KWR (Netherlands) included crAssphage to normalize SARS-CoV-2 titers in influent wastewater to monitor the COVID-19 pandemic (KWR, 2020), thus its potential as biomarker is not fully explored yet.
We detected crAssphage in all influent and effluent samples at mean concentrations of 8.37 ± 0.55 and 5.64 ± 0.59 log10 GC/L, respectively. These concentrations in influent wastewaters were roughly in line with the ones reported in the United Kingdom (Farkas et al., 2019), United States (Wu et al., 2020), and in a previous study conducted also in Spain (García-Aljaro et al., 2017). Slightly lower titers were reported in Thailand (Kongprajug et al., 2019) and in Italy (Crank et al., 2020). On the contrary, higher concentration of 10.98–12.03 log10 GC/L in influent and 7.45–8.62 log10 GC/L in effluent wastewaters were reported in Japan (Malla et al., 2019). These discrepancies might be due to the population served by WWTPs, the engineering characteristics of the sewer system (e.g., retention times, treatments, etc.), and the analytical method used for viral detection (wastewater concentration procedure, the genomic target, standards used to quantify viral concentrations), among other variables. Analyzing some of those variables, we observed statistically significant differences on crAssphage titers for served population, flow intake, and among WWTPs. This finding is in accordance to a previous report by Crank et al. (2020). Additionally, crAssphage concentrations in effluent wastewater were significantly lower when wastewater was exposed to UV than to chlorination. Thus, we further corroborate existing bibliography indicating the efficient viral disinfection applying UV light irradiation (Ali, 1997; Mezzanotte et al., 2007; Shah et al., 2011; Zyara et al., 2016). The increased mean removal in UV-treated wastewaters compared to chlorinated effluents can be extended to norovirus GI, but not for the other enteric viruses tested in this study (Figure 3).
CrAssphage as Indicator for the Potential Infectivity of Enteric Viruses in Wastewater
The correlation between crAssphage and human viral pathogens has been reported in recent studies investigating wastewaters (Farkas et al., 2019; Malla et al., 2019; Crank et al., 2020; Tandukar et al., 2020), sludge (Wu et al., 2020), and other fecal polluted waters (Jennings et al., 2020). However, no information was available to date on whether crAssphage would serve as indicator for the potential infectivity of enteric viruses in wastewater. In influent wastewater, we found crAssphage strongly correlated to intact capsid norovirus GII and moderately to norovirus GI. In effluent wastewater, crAssphage moderately correlated with potentially infectious HAstV and norovirus GI.
Overall, the consistent detection of crAssphage in all influent and effluent samples corroborates the phage as an indicator for fecal contamination in wastewater. However, correlation readouts do not solidly support the use of crAssphage as indicator for the presence of potentially infectious enteric virus in wastewater, which was the primary hypothesis tested in this study. Thus, a strategy that targets each viral contaminant should be preferred to the sole detection of phages and this applies for both investigation and monitoring purposes.
The results of the present study also demonstrated that E. coli, adopted in the current regulation as fecal biomarker, and physicochemical parameters are not well suited as indicators for the viral contamination of wastewater, according to previous reports (Stachler et al., 2018; Ahmed et al., 2020).
Limitation, Perspective, and Future Research
This study did not take into account environmental variables, such as rainfall and temperature, among others, that could have affected reported results.
Although analyzing the samples by RT-qPCR alone could have served as baseline to check the performance of PMAxx, previous studies already investigated the relationship of capsid integrity treatment on viral amplification signal reduction (Randazzo et al., 2016, 2019; Cuevas-Ferrando et al., 2020). Following a one-size capsid integrity treatment fits all approach and assuming it could lead to lower signal reduction (e.g., virus and matrix specificity: length and structure of genome targeted by the qPCR assays, the influence of co-concentrated inhibitory substances, etc.), we tested the hypothesis to adapt existent workflows for improving risk assessment.
Also, the comparison of molecular results with cell culture would have soundly confirmed our findings. However, viral cell culture of environmental samples presents technical challenges that are difficult to overcome (e.g., contamination, toxicity, sensitivity), especially in a longitudinal monitoring study such this one. A similar consideration can be done for crAssphage (Shkoporov et al., 2018).
Our findings based on capsid integrity assays could boost the development of advanced quantitative microbial risk assessment (QMRA) models for determining the risk of infection in case of treated wastewater reuse. This warrants further investigation and constitutes the gap to fill in the future in order to better quantify the human health risk, provide robust information for decision-making, and support water quality regulation.
In conclusion, this work provides insights on the quantitative occurrence of crAssphage and intact capsid enteric viruses in influent and effluent wastewater, while correlation outcomes indicated that crAssphage is a poor indicator for enteric virus infectivity in reclaimed wastewater.
Data Availability Statement
The original raw dataset of the study are included as Supplementary Material in the article. Any further inquiries can be directed to the corresponding author.
Author Contributions
GS and WR: conceptualization and funding acquisition. EC-F, AP-C, and IF: methodology. EC-F and WR: writing—original draft preparation. EC-F, AP-C, IF, WR, and GS: review and editing. All authors have read and agreed to the published version of the manuscript.
Funding
This research was supported by the “VIRIDIANA” (AGL2017-82909, AEI/FEDER, UE) and “PREVISION” (PID2019-105509RJ-I00, AEI/FEDER, UE) projects funded by the Spanish Ministry of Science, Innovation and Universities, and the 2018CPS10 project funded by the Center for Produce Safety. AP-C was recipient of a postdoctoral grant from Generalitat Valenciana (APOSTD/2021/292). EC-F was recipient of a predoctoral contract from the MICINN, Call 2018. The funding sources had no rule in the study design, data collection, analysis of data, writing, or in the decision to publish.
Conflict of Interest
The authors declare that the research was conducted in the absence of any commercial or financial relationships that could be construed as a potential conflict of interest.
Publisher’s Note
All claims expressed in this article are solely those of the authors and do not necessarily represent those of their affiliated organizations, or those of the publisher, the editors and the reviewers. Any product that may be evaluated in this article, or claim that may be made by its manufacturer, is not guaranteed or endorsed by the publisher.
Acknowledgments
We acknowledge GAMASER and Global Omnium S.L. for authorizing, arranging wastewater sampling, and performing physical-chemical analyses; Agustín Garrido (IATA-CSIC) for providing technical assistance in water processing; and the Central Service for Experimental Research (SCSIE) (University of Valencia, Spain) for supporting and supervising statistical analyses.
Supplementary Material
The Supplementary Material for this article can be found online at: https://www.frontiersin.org/articles/10.3389/fmicb.2022.836193/full#supplementary-material
References
AAVV (2018). Detection of Enteric Viruses. in Standard Methods For the Examination of Water and Wastewater. (Standard Methods for the Examination of Water and Wastewater). Washington DC: American Public Health Association. doi: 10.2105/SMWW.2882.202
Ahmed, W., Kitajima, M., Tandukar, S., and Haramoto, E. (2020). Recycled water safety: current status of traditional and emerging viral indicators. Curr. Opin. Environ. Sci. Health 16, 62–72.
Ali, M. (1997). Ultraviolet, chlorine and ozone disinfection of some enteroviruses in drinking water and treated effluents. J. Egypt. Public Health Assoc. 72, 411–423.
Amarasiri, M., Kitajima, M., Nguyen, T. H., Okabe, S., and Sano, D. (2017). Bacteriophage removal efficiency as a validation and operational monitoring tool for virus reduction in wastewater reclamation: review. Water Res. Engl. 121, 258–269. doi: 10.1016/j.watres.2017.05.035
Asghar, H., Diop, O. M., Weldegebriel, G., Malik, F., Shetty, S., El Bassioni, L., et al. (2014). Environmental surveillance for polioviruses in the global polio eradication initiative. J. Infect. Dis. 210, S294–S303. doi: 10.1093/infdis/jiu384
Barcelo, D., and Petrovic, M. (2011). The Handbook of Environmental Chemistry, eds D. Barcel and A. G. Kostianoy, Berlin: Springer.
Bivins, A., North, D., Ahmad, A., Ahmed, W., Alm, E., Been, F., et al. (2020b). Wastewater-based epidemiology: global collaborative to maximize contributions in the fight against COVID-19. Environ. Sci. Technol. 54, 7754–7757. doi: 10.1021/acs.est.0c02388
Bivins, A., Crank, K., Greaves, J., North, D., Wu, Z., and Bibby, K. (2020a). Cross-assembly phage and pepper mild mottle virus as viral water quality monitoring tools—potential, research gaps, and way forward. Curr. Opin. Environ. Sci. Health 16, 54–61. doi: 10.1016/j.coesh.2020.02.001
Borchardt, M. A., Boehm, A. B., Salit, M. L., Spencer, S. K., Wigginton, K. R., and Noble, R. T. (2021). The environmental microbiology minimum information (EMMI) guidelines: QPCR and dPCR quality and reporting for environmental microbiology. Environ. Sci. Technol. 55, 10210–10223.
Bosch, A., Guix, S., Sano, D., and Pintó, R. M. (2008). New tools for the study and direct surveillance of viral pathogens in water. Curr. Opin. Biotechnol. 19, 295–301. doi: 10.1016/j.copbio.2008.04.006
Canh, V. D., Torii, S., Furumai, H., and Katayama, H. (2021a). Application of capsid integrity (RT-)qPCR to assessing occurrence of intact viruses in surface water and tap water in Japan. Water Res. 189:116674. doi: 10.1016/j.watres.2020.116674
Canh, V. D., Torii, S., Yasui, M., Kyuwa, S., and Katayama, H. (2021b). Capsid integrity RT-qPCR for the selective detection of intact SARS-CoV-2 in wastewater. Sci. Total Environ. 791:148342. doi: 10.1016/j.scitotenv.2021.148342
Carter, M. J. (2005). Enterically infecting viruses: pathogenicity, transmission and significance for food and waterborne infection. J. Appl. Microbiol. 98, 1354–1380. doi: 10.1111/j.1365-2672.2005.02635.x
Coudray-Meunier, C., Fraisse, A., Martin-Latil, S., Guillier, L., and Perelle, S. (2013). Discrimination of infectious hepatitis A virus and rotavirus by combining dyes and surfactants with RT-qPCR. BMC Microbiol. 13:216. doi: 10.1186/1471-2180-13-216
Crank, K., Li, X., North, D., Ferraro, G. B., Iaconelli, M., Mancini, P., et al. (2020). CrAssphage abundance and correlation with molecular viral markers in Italian wastewater. Water Res. 184:116161. doi: 10.1016/j.watres.2020.116161
Cuevas-Ferrando, E., Randazzo, W., Pérez-Cataluña, A., and Sánchez, G. (2020). HEV occurrence in waste and drinking water treatment plants. Front. Microbiol. 10:2937. doi: 10.3389/fmicb.2019.02937
Cuevas-Ferrando, E., Randazzo, W., Pérez-Cataluña, A., Falcó, I., Navarro, D., Martin-Latil, S., et al. (2021). Platinum chloride-based viability RT-qPCR for SARS-CoV-2 detection in complex samples. Sci. Rep. 11:18120. doi: 10.1038/s41598-021-97700-x
Da Silva, A. K., Le Saux, J. C., Parnaudeau, S., Pommepuy, M., Elimelech, M., and Le Guyader, F. S. (2007). Evaluation of removal of noroviruses during wastewater treatment, using real-time reverse transcription-PCR: different behaviors of genogroups I and II. Appl. Environ. Microbiol. 73, 7891–7897. doi: 10.1128/AEM.01428-07
de Oliveira, M., Frihling, B. E. F., Velasques, J., Filho, F. J. C. M., Cavalheri, P. S., and Migliolo, L. (2020). Pharmaceuticals residues and xenobiotics contaminants: occurrence, analytical techniques and sustainable alternatives for wastewater treatment. Sci. Total Environ. 705:135568. doi: 10.1016/j.scitotenv.2019.135568
Falcó, I., Randazzo, W., Rodríguez-Díaz, J., Gozalbo-Rovira, R., Luque, D., Aznar, R., et al. (2019). Antiviral activity of aged green tea extract in model food systems and under gastric conditions. Int. J. Food Microbiol. 292, 101–106. doi: 10.1016/j.ijfoodmicro.2018.12.019
Farkas, K., Adriaenssens, E. M., Walker, D. I., McDonald, J. E., Malham, S. K., and Jones, D. L. (2019). Critical evaluation of CrAssphage as a molecular marker for human-derived wastewater contamination in the aquatic environment. Food Environ. Virol. 11, 113–119. doi: 10.1007/s12560-019-09369-1
Farkas, K., Marshall, M., Cooper, D., McDonald, J. E., Malham, S. K., Peters, D. E., et al. (2018). Seasonal and diurnal surveillance of treated and untreated wastewater for human enteric viruses. Environ. Sci. Pollut. Res. 25, 33391–33401. doi: 10.1007/s11356-018-3261-y
Farkas, K., Walker, D. I., Adriaenssens, E. M., McDonald, J. E., Hillary, L. S., Malham, S. K., et al. (2020). Viral indicators for tracking domestic wastewater contamination in the aquatic environment. Water Res. 181:115926. doi: 10.1016/j.watres.2020.115926
Fenaux, H., Chassaing, M., Berger, S., Gantzer, C., Bertrand, I., and Schvoerer, E. (2019). Transmission of hepatitis E virus by water: an issue still pending in industrialized countries. Water Res. 151, 144–157. doi: 10.1016/j.watres.2018.12.014
García-Aljaro, C., Ballesté, E., Muniesa, M., and Jofre, J. (2017). Determination of crAssphage in water samples and applicability for tracking human faecal pollution. Microb. Biotechnol. 10, 1775–1780. doi: 10.1111/1751-7915.12841
Gerba, C. P., Betancourt, W. Q., and Kitajima, M. (2017). How much reduction of virus is needed for recycled water: a continuous changing need for assessment? Water Res. 108, 25–31. doi: 10.1016/j.watres.2016.11.020
Gerba, C. P., Betancourt, W. Q., Kitajima, M., and Rock, C. M. (2018). Reducing uncertainty in estimating virus reduction by advanced water treatment processes. Water Res. 133, 282–288. doi: 10.1016/j.watres.2018.01.044
Gyawali, P., and Hewitt, J. (2018). Detection of infectious noroviruses from wastewater and seawater using PEMAXTM treatment combined with RT-qPCR. Water (Switzerland) 10:841. doi: 10.3390/w10070841
Haramoto, E., Kitajima, M., Hata, A., Torrey, J. R., Masago, Y., Sano, D., et al. (2018). A review on recent progress in the detection methods and prevalence of human enteric viruses in water. Water Res. 135, 168–186. doi: 10.1016/j.watres.2018.02.004
Hellmér, M., Paxéus, N., Magnius, L., Enache, L., Arnholm, B., Johansson, A., et al. (2014). Detection of pathogenic viruses in sewage provided early warnings of hepatitis A virus and norovirus outbreaks. Appl. Environ. Microbiol. 80, 6771–6781. doi: 10.1128/AEM.01981-14
Honap, T. P., Sankaranarayanan, K., Schnorr, S. L., Ozga, A. T., Warinner, C., and Lewis, C. M. Jr. (2020). Biogeographic study of human gut-associated crAssphage suggests impacts from industrialization and recent expansion. PLoS One 15:e0226930. doi: 10.1371/journal.pone.0226930
Jennings, W. C., Gálvez-Arango, E., Prieto, A. L., and Boehm, A. B. (2020). CrAssphage for fecal source tracking in Chile: covariation with norovirus, HF183, and bacterial indicators. Water Res. X 9:100071. doi: 10.1016/j.wroa.2020.100071
Katayama, H., Haramoto, E., Oguma, K., Yamashita, H., Tajima, A., Nakajima, H., et al. (2008). One-year monthly quantitative survey of noroviruses, enteroviruses, and adenoviruses in wastewater collected from six plants in Japan. Water Res. 42, 1441–1448. doi: 10.1016/j.watres.2007.10.029
Kim, K., Katayama, H., Kitajima, M., Tohya, Y., and Ohgaki, S. (2011). Development of a real-time RT-PCR assay combined with ethidium monoazide treatment for RNA viruses and its application to detect viral RNA after heat exposure. Water Sci. Technol. 63, 502–507. doi: 10.2166/wst.2011.249
Kitajima, M., Iker, B. C., Pepper, I. L., and Gerba, C. P. (2014). Relative abundance and treatment reduction of viruses during wastewater treatment processes – Identification of potential viral indicators. Sci. Total Environ. 488–489, 290–296. doi: 10.1016/j.scitotenv.2014.04.087
Kongprajug, A., Mongkolsuk, S., and Sirikanchana, K. (2019). CrAssphage as a potential human sewage marker for microbial source tracking in Southeast Asia. Environ. Sci. Technol. Lett. 6, 159–164. doi: 10.1021/acs.estlett.9b00041
KWR (2020). New SARS-CoV-2 Graphs for Wastewater Samples of September. Available online at: https://www.kwrwater.nl/en/actueel/new-sars-cov-2-graphs-for-wastewater-samples-of-september/ (accessed December 29, 2020).
Leifels, M., Cheng, D., Sozzi, E., Shoults, D. C., Wuertz, S., Mongkolsuk, S., et al. (2020). Capsid integrity quantitative PCR to determine virus infectivity in environmental and food applications – a systematic review. Water Res. X 11:100080. doi: 10.1016/j.wroa.2020.100080
Leifels, M., Jurzik, L., Wilhelm, M., and Hamza, I. A. (2015). Use of ethidium monoazide and propidium monoazide to determine viral infectivity upon inactivation by heat, UV- exposure and chlorine. Int. J. Hygiene Environ. Health 218, 686–693. doi: 10.1016/j.ijheh.2015.02.003
Leifels, M., Shoults, D. C., Wiedemeyer, A., Ashbolt, N. J., Sozzi, E., Hagemeier, A., et al. (2019). Capsid integrity qPCR—an azo-dye based and culture-independent approach to estimate adenovirus infectivity after disinfection and in the aquatic environment. Water 11:1196. doi: 10.3390/w11061196
López-Gálvez, F., Randazzo, W., Vásquez, A., Sánchez, G., Decol, L. T., Aznar, R., et al. (2018). Irrigating lettuce with wastewater effluent: does disinfection with chlorine dioxide inactivate viruses? J. Environ. Qual. 47, 1139–1145. doi: 10.2134/jeq2017.12.0485
Malla, B., Makise, K., Nakaya, K., Mochizuki, T., Yamada, T., and Haramoto, E. (2019). Evaluation of human- and animal-specific viral markers and application of CrAssphage, pepper mild mottle virus, and tobacco mosaic virus as potential fecal pollution markers to river water in Japan. Food Environ. Virol. 11, 446–452. doi: 10.1007/s12560-019-09398-w
Mercan, S., Kuloglu, M., and Asicioglu, F. (2019). Monitoring of illicit drug consumption via wastewater: development, challenges, and future aspects. Curr. Opin. Environ. Sci. Health 9, 64–72. doi: 10.1016/j.coesh.2019.05.002
Mezzanotte, V., Antonelli, M., Citterio, S., and Nurizzo, C. (2007). Wastewater disinfection alternatives: chlorine, ozone, peracetic acid, and UV light. Water Environ. Res. 79, 2373–2379. doi: 10.2175/106143007x183763
Miura, T., Lhomme, S., Le Saux, J. C., Le Mehaute, P., Guillois, Y., Couturier, E., et al. (2016). Detection of hepatitis E virus in sewage after an outbreak on a French Island. Food Environ. Virol. 8, 194–199. doi: 10.1007/s12560-016-9241-9
Montazeri, N., Goettert, D., Achberger, E. C., Johnson, C. N., Prinyawiwatkul, W., and Janes, M. E. (2015). Pathogenic enteric viruses and microbial indicators during secondary treatment of municipal wastewater. Appl. Environ. Microbiol. 81, 6436–6445. doi: 10.1128/AEM.01218-15
Okoh, A. I., Sibanda, T., and Gusha, S. S. (2010). Inadequately treated wastewater as a source of human enteric viruses in the environment. Int. J. Environ. Res. Public Health 7, 2620–2637. doi: 10.3390/ijerph7062620
Oude Munnink, B. B., and van der Hoek, L. (2016). Viruses causing gastroenteritis: the known, the new and those beyond. Viruses 8:42. doi: 10.3390/v8020042
Parshionikar, S., Laseke, I., and Fout, G. S. (2010). Use of propidium monoazide in reverse transcriptase PCR to distinguish between infectious and noninfectious enteric viruses in water samples. Appl. Environ. Microbiol. 76, 4318–4326. doi: 10.1128/AEM.02800-09
Prevost, B., Goulet, M., Lucas, F. S., Joyeux, M., Moulin, L., and Wurtzer, S. (2016). Viral persistence in surface and drinking water: suitability of PCR pre-treatment with intercalating dyes. Water Res. 91, 68–76. doi: 10.1016/j.watres.2015.12.049
Prevost, B., Lucas, F. S., Goncalves, A., Richard, F., Moulin, L., and Wurtzer, S. (2015). Large scale survey of enteric viruses in river and waste water underlines the health status of the local population. Environ. Int. 79, 42–50. doi: 10.1016/j.envint.2015.03.004
Rachida, S., and Taylor, M. B. (2020). Potentially infectious novel hepatitis a virus strains detected in selected treated wastewater discharge sources, South Africa. Viruses 12:1468.
Ramírez-Castillo, F. Y., Loera-Muro, A., Jacques, M., Garneau, P., Avelar-González, F. J., Harel, J., et al. (2015). Waterborne pathogens: detection methods and challenges. Pathogens (Basel, Switzerland) 4, 307–334. doi: 10.3390/pathogens4020307
Randazzo, W., D’Souza, D. H., and Sanchez, G. (2018a). Norovirus: the burden of the unknown. Adv. Food Nutr. Res. 86, 13–53. doi: 10.1016/bs.afnr.2018.02.005
Randazzo, W., Piqueras, J., Rodríguez-Díaz, J., Aznar, R., and Sánchez, G. (2018c). Improving efficiency of viability-qPCR for selective detection of infectious HAV in food and water samples. J. Appl. Microbiol. 124, 958–964. doi: 10.1111/jam.13519
Randazzo, W., Khezri, M., Ollivier, J., Le Guyader, F. S., Rodríguez-Díaz, J., Aznar, R., et al. (2018b). Optimization of PMAxx pretreatment to distinguish between human norovirus with intact and altered capsids in shellfish and sewage samples. Int. J. Food Microbiol. 266, 1–7. doi: 10.1016/j.ijfoodmicro.2017.11.011
Randazzo, W., López-Gálvez, F., Allende, A., Aznar, R., and Sánchez, G. (2016). Evaluation of viability PCR performance for assessing norovirus infectivity in fresh-cut vegetables and irrigation water. Int. J. Food Microbiol. 229, 1–6. doi: 10.1016/j.ijfoodmicro.2016.04.010
Randazzo, W., Piqueras, J., Evtoski, Z., Sastre, G., Sancho, R., Gonzalez, C., et al. (2019). Interlaboratory comparative study to detect potentially infectious human enteric viruses in influent and effluent waters. Food Environ. Virol. 11, 350–363. doi: 10.1007/s12560-019-09392-2
Randazzo, W., Truchado, P., Cuevas-Ferrando, E., Simón, P., Allende, A., and Sánchez, G. (2020). SARS-CoV-2 RNA in wastewater anticipated COVID-19 occurrence in a low prevalence area. Water Res. 181:115942. doi: 10.1016/j.watres.2020.115942
Regulation (EU) 2020/741 (2020). Regulation (EU) 2020/741 of the European Parliament and of the Council of 25 May 2020 On Minimum Requirements for Water Reuse. Available online at: http://data.europa.eu/eli/reg/2020/741/oj (accessed December 29, 2020).
Sano, D., Amarasiri, M., Hata, A., Watanabe, T., and Katayama, H. (2016). Risk management of viral infectious diseases in wastewater reclamation and reuse: review. Environ. Int. 91, 220–229. doi: 10.1016/j.envint.2016.03.001
Santiso-Bellón, C., Randazzo, W., Pérez-Cataluña, A., Vila-Vicent, S., Gozalbo-Rovira, R., Muñoz, C., et al. (2020). Epidemiological surveillance of norovirus and rotavirus in Sewage (2016–2017) in Valencia (Spain). Microorganisms 8:458. doi: 10.3390/microorganisms8030458
Shah, A. D., Dotson, A. D., Linden, K. G., and Mitch, W. A. (2011). Impact of UV disinfection combined with chlorination/chloramination on the formation of halonitromethanes and haloacetonitriles in drinking water. Environ. Sci. Technol. 45, 3657–3664. doi: 10.1021/es104240v
Shirasaki, N., Matsushita, T., Matsui, Y., and Koriki, S. (2020). Suitability of pepper mild mottle virus as a human enteric virus surrogate for assessing the efficacy of thermal or free-chlorine disinfection processes by using infectivity assays and enhanced viability PCR. Water Res. 186:116409. doi: 10.1016/j.watres.2020.116409
Shkoporov, A. N., Khokhlova, E. V., Fitzgerald, C. B., Stockdale, S. R., Draper, L. A., Ross, R. P., et al. (2018). ΦCrAss001 represents the most abundant bacteriophage family in the human gut and infects Bacteroides intestinalis. Nat. Commun. 9:4781. doi: 10.1038/s41467-018-07225-7
Sidhu, J. P. S., Ahmed, W., Palmer, A., Smith, K., Hodgers, L., and Toze, S. (2017). Optimization of sampling strategy to determine pathogen removal efficacy of activated sludge treatment plant. Environ. Sci. Pollut. Res. 24, 19001–19010. doi: 10.1007/s11356-017-9557-5
Simmons, F. J., and Xagoraraki, I. (2011). Release of infectious human enteric viruses by full-scale wastewater utilities. Water Res. 45, 3590–3598. doi: 10.1016/j.watres.2011.04.001
Stachler, E., Akyon, B., de Carvalho, N. A., Ference, C., and Bibby, K. (2018). Correlation of crAssphage qPCR markers with culturable and molecular indicators of human fecal pollution in an impacted urban watershed. Environ. Sci. Technol. 52, 7505–7512. doi: 10.1021/acs.est.8b00638
Stachler, E., Kelty, C., Sivaganesan, M., Li, X., Bibby, K., and Shanks, O. C. (2017). Quantitative CrAssphage PCR assays for human fecal pollution measurement. Environ. Sci. Technol. 51, 9146–9154. doi: 10.1021/acs.est.7b02703
Symonds, E. M., Rosario, K., and Breitbart, M. (2019). Pepper mild mottle virus: agricultural menace turned effective tool for microbial water quality monitoring and assessing (waste)water treatment technologies. PLoS Pathog. 15:e1007639. doi: 10.1371/journal.ppat.1007639
Tandukar, S., Sherchan, S. P., and Haramoto, E. (2020). Applicability of crAssphage, pepper mild mottle virus, and tobacco mosaic virus as indicators of reduction of enteric viruses during wastewater treatment. Sci. Rep. 10:3616. doi: 10.1038/s41598-020-60547-9
Tian, P., Yang, D., Shan, L., Li, Q., Liu, D., and Wang, D. (2018). Estimation of human norovirus infectivity from environmental water samples by in situ capture RT-qPCR Method. Food Environ. Virol. 10, 29–38. doi: 10.1007/s12560-017-9317-1
Wang, D., and Tian, P. (2014). Inactivation conditions for human norovirus measured by an in situ capture-qRT-PCR method. Int. J. Food Microbiol. 172, 76–82. doi: 10.1016/j.ijfoodmicro.2013.11.027
Wu, Z., Greaves, J., Arp, L., Stone, D., and Bibby, K. (2020). Comparative fate of CrAssphage with culturable and molecular fecal pollution indicators during activated sludge wastewater treatment. Environ. Int. 136:105452. doi: 10.1016/j.envint.2019.105452
Keywords: enteric viruses, capsid integrity RT-qPCR, wastewater, crAssphage, fecal contamination indicator
Citation: Cuevas-Ferrando E, Pérez-Cataluña A, Falcó I, Randazzo W and Sánchez G (2022) Monitoring Human Viral Pathogens Reveals Potential Hazard for Treated Wastewater Discharge or Reuse. Front. Microbiol. 13:836193. doi: 10.3389/fmicb.2022.836193
Received: 17 January 2022; Accepted: 21 February 2022;
Published: 08 April 2022.
Edited by:
Shubiao Wu, Aarhus Institute of Advanced Studies, DenmarkReviewed by:
Davey Jones, Bangor University, United KingdomJanet Mans, University of Pretoria, South Africa
Copyright © 2022 Cuevas-Ferrando, Pérez-Cataluña, Falcó, Randazzo and Sánchez. This is an open-access article distributed under the terms of the Creative Commons Attribution License (CC BY). The use, distribution or reproduction in other forums is permitted, provided the original author(s) and the copyright owner(s) are credited and that the original publication in this journal is cited, in accordance with accepted academic practice. No use, distribution or reproduction is permitted which does not comply with these terms.
*Correspondence: Walter Randazzo, d3JhbmRhenpvQGlhdGEuY3NpYy5lcw==