- 1Inner Mongolia Key Laboratory of Microbial Ecology of Silage, Inner Mongolia Engineering Research Center of Development and Utilization of Microbial Resources in Silage, Inner Mongolia Academy of Agriculture and Animal Husbandry Science, Hohhot, China
- 2College of Foreign Languages, Inner Mongolia University of Finance and Economics, Hohhot, China
- 3Inner Mongolia Youran Animal Husbandry Co., Ltd., Hohhot, China
- 4Institute of Grassland Research, Chinese Academy of Agricultural Sciences, Hohhot, China
The aim of this study was to determine the effects of six common commercial lactic acid bacteria (LAB) additives [A1, Lactobacillus plantarum, L. buchneri, and Enterococcus faecalis; A2, L. plantarum and L. casei; A3, L. plantarum and L. buchneri; A4, L. plantarum, L. buchneri, L. casei, and Pediococcus acidilactici; A5, L. plantarum (producing feruloyl esterase); and A6, L. buchneri, P. acidilactici, β-glucanase, and xylanase] on the bacterial community and fermentation quality of alfalfa silage. Alfalfa was harvested at the squaring stage, wilted in the field for 24 h, and ensiled without any additives (Control) or with A1, A2, A3, A4, A5, or A6. Microbial counts, bacterial community, fermentation parameters, and nutritional composition were determined after ensiling for 90 days. The total abundance of LAB genera on alfalfa pre-ensiling was 0.38% in bacterial community. The abundances of Lactobacillus, Enterococcus, and Pediococcus in the Control silage were 42.18, 40.18, and 8.09% of abundance, respectively. The abundances of Lactobacillus in A1-, A2-, A3-, A4-, and A5-treatments were 89.32, 92.93, 92.87, 81.12, and 80.44%, respectively. The abundances of Pediococcus and Lactobacillus in A6-treatment were 70.14 and 24.86%, respectively. Compared with Control silage, LAB-treated silage had lower pH and less ammonia nitrogen and water-soluble carbohydrates concentrations (p < 0.05). Further, the A5- and A6-treatments contained lower neutral detergent fiber, acid detergent fiber, and hemicellulose than other treatments (p < 0.05). Overall, LAB genera were presented as minor taxa in alfalfa pre-ensiling and as dominant taxa in alfalfa silage. Adding LAB additives improved the fermentation quality and altered the bacterial community of alfalfa silage. The main bacterial genera in Control silage were Lactobacillus, Enterococcus, and Pediococcus. Lactobacillus dominated the bacterial communities of A1-, A2-, A3-, A4-, and A5-treatments, while Pediococcus and Lactobacillus were dominant bacterial genera in A6-treatment. Inoculating A5 and A6 degraded the fiber in alfalfa silage. It is necessary to ensile alfalfa with LAB inoculants.
Introduction
Ensiling has become a common and effective method for the long-term preservation of forage for livestock (Sun et al., 2021a). Silage enables anaerobic microbial fermentation to be dominated by lactic acid bacteria (LAB), which utilize water-soluble carbohydrates (WSC) to produce lactic acid (LA), reduce pH, and inhibit harmful microorganisms during ensilage process (Yang et al., 2020). Alfalfa (Medicago sativa L.) is a preferred perennial legume forage for livestock producers owing to its high nutritional value, especially its high crude protein (CP) concentration (Hartinger et al., 2019; Besharati et al., 2021; Netthisinghe et al., 2021). However, the second and third cuts of alfalfa in northern China are generally harvested during July and August, a period with an unreliable weather for alfalfa hay processing as it is in the rainy season. As a result, ensiling is the preferable method for conserving alfalfa during this period. Nevertheless, ensiling alfalfa with satisfactory fermentation quality is difficult because of the low dry matter (DM) and WSC concentrations and high buffering capacity (BC) (Sun et al., 2021b). Thus, wilting and applying additives to ensiled alfalfa are necessary to improve the fermentation quality and optimize microbial communities (Gao et al., 2021; Zhang et al., 2021).
Microbial composition, particularly LAB populations, plays a crucial role in the ensiling fermentation quality of silage (Bai et al., 2021). The development of next-generation sequencing technologies has helped to understand the differences in microbial communities and fermentation quality among silages (Wang C. et al., 2021). Previous studies revealed that inoculating LAB additives at ensiling alfalfa promotes bacterial community dynamics (especially Lactobacillus dynamics) during the fermentation process (Guo et al., 2018, 2020; Hu et al., 2020; Zhao S. et al., 2021). Other studies have also reported that Lactobacillus dominates the bacterial community in terminal alfalfa silage and in the mixing silage of alfalfa and whole-plant corn (Wang et al., 2020; Luo et al., 2021; Wang M. et al., 2021; Yang et al., 2021).
Inoculating LAB at ensiling optimizes the bacterial community and improves the fermentation quality of the terminal silage (Schmidt et al., 2009; Silva et al., 2016; Zhang et al., 2021; Zhao S. et al., 2021). Previous studies reported that the inoculation of ensiling alfalfa with self-screened LAB can promote the succession of Lactobacillus during the fermentation process and increase the abundance of Lactobacillus in terminal silage with good fermentation quality (Guo et al., 2018; Hu et al., 2020; Yang et al., 2021). Fermentation quality is improved in alfalfa silage treated with functional LAB screened to produce 3-phenyllactic acid (Wu et al., 2020), ferulic acid esterase (Su et al., 2019; Xie et al., 2021), and class IIa bacteriocin (Li et al., 2020). Sun et al. (2021b) revealed that alfalfa silage inoculated with LAB from ensiling material had greater fermentation quality than that inoculated with LAB from other forage sources. However, LAB screening has a low degree of commercialization, and the effect of common commercial LAB additives on fermentation quality and microbial communities of alfalfa silage has rarely been reported.
In the present study, six commercial LAB additives commonly used for ensiling alfalfa silage in northern China were collected. We hypothesized that the application of these additives at ensiling would improve the fermentation quality and optimize the bacterial community of alfalfa silage. The objective of this study was to determine the fermentation quality and bacterial community in alfalfa silage treated with commercial LAB additives.
Materials and Methods
Silage Preparation
Alfalfa was grown for 3 years on an experimental farm (40°46.265 N, 111°39.851E) at the Inner Mongolia Academy of Agricultural and Animal Husbandry Science, Hohhot, China, and harvested from four fields as replicates. The second-cut alfalfa was harvested at the squaring stage at 1 p.m. on June 1, 2019, and wilted in the fields for 24 h. The wilted forages from the four fields were separately chopped to 10–20 mm lengths using a chaffcutter (Hongguang Industry and Trade Co., Ltd., Zhejiang, China), thoroughly mixed, and then randomly divided into seven batches for seven treatments. After each additive (5 g) was mixed with distilled water (2,000 ml), the resulting mixture was allowed to rest for 2 h. The seven treatments were as follows: CK (control): 2 ml/kg fresh weight (FW) of distilled water; A1: 2 ml/kg FW of distilled water and 2 g/t FW (recommended amount, RA) of the first additive [L. plantarum LP28 (≥1.0 × 1011 CFU/g), L. buchneri LBC136 (≥1.0 × 109 CFU/g), and Enterococcus faecalis EF08 (≥1.0 × 109 CFU/g); Xinlaiwang I-HL for ensiling straw; Xinlaiwang Biotechnology Co., Ltd., Yangzhou, China]; A2, 2 ml/kg FW of distilled water and 2 g/t FW (RA) of the second additive [L. plantarum (≥6.0 × 1010 CFU/g) and Lactobacillus casei (≥4.0 × 1010 CFU/g); Xinlaiwang I for ensiling alfalfa. Xinlaiwang Biotechnology Co., Ltd., Yangzhou, China]; A3, 2 ml/kg FW of distilled water and 5 g/t FW (RA) of the third additive [L. plantarum 550 and 360 (≥1.3 × 1010 CFU/g) and L. buchneri 225 (≥7.0 × 109 CFU/g); Zhuanglemei; Sichuan Gaofuji Biotechnology Co., Ltd., Chengdu, China]; A4, 2 ml/kg FW of distilled water and 1 g/t FW (RA) of the fourth additive [L. plantarum, L. buchneri, L. casei, and Pediococcus acidilactici (≥1.0 × 1011 CFU/g); BONSILAGE; Schaumann Agricultural Trading Co., Ltd., Shanghai, China]; A5, 2 ml/kg FW of distilled water and 1 g/t FW (RA) of the fifth additive [L. plantarum MF0932189 (producing feruloyl esterase, ≥ 1.0 × 1011 CFU/g); QXMG; Gansu Aopujintai Biological Engineering Co., Ltd., Lanzhou, China]; and A6, 2 ml/kg FW of distilled water and 1 g/t FW (RA) of the sixth additive [L. buchneri NCIMB 40788 (≥7.5 × 1010 CFU/g), P. acidilactici CNCM MA 18/5 M (≥5.0 × 1010 CFU/g), β-glucanase de Aspergillus niger MUCL 39199 (≥5,750 IU/g), and xylanase de Trichoderma longibrachiatum MUCL 39203 (≥30,000 IU/g), ≥ 1.25 × 1011 CFU/g; LaLSiL Dry; Lallemand Biotechnology Co., Ltd., Beijing, China]. After spraying distilled water with or without additives on chopped alfalfa and performing uniform mixing, approximately 500 g of forage was packed into a plastic bag (food grade, 300 mm × 400 mm; Qingye, Beijing, China) and sealed using a vacuum sealer (DZ-300; Qingye, Beijing, China). The bags were stored in a dark room for 90 days and then sampled for analysis. After sampling, the alfalfa pre-ensiling and silages were dried in a forced-air oven (BPG-9240A, Shanghai Yiheng Scientific Instrument Co., Ltd., Shanghai, China) at 65°C for 48 h, ground using a mill (FS-6D; Fichi Machinery Equipment Co., Ltd., Shandong, China) with a 1-mm screen, and dried in the same forced-air oven at 105°C until a constant mass was achieved. The dry matter (DM) content of the silages was corrected for the loss of volatiles during drying according to Weissbach and Strubelt (2008).
Microbial Counts and Bacterial Community
The counts of LAB, coliforms, aerobic bacteria, and yeast were determined via culture on Man, Rogosa, Sharpe agar, violet red bile agar, nutrient agar, and potato dextrose agar, respectively, in an incubator (LRH-70, Shanghai Yiheng Science Instruments Co., Ltd., Shanghai, China) at 30°C for 72 h (Cai, 1999).
The bacterial communities of alfalfa pre-ensiling and silages were analyzed by Hangzhou Lianchuan Biotechnology Co., Ltd., Hangzhou, China, according to the method described by Sun et al. (2021a). The E.Z.N.A.® Stool DNA Kit (D4015, Omega Inc., Norcross, GA, United States) was used to extract DNA from the bacteria according to the manufacturer’s instructions. Polymerase chain reaction (PCR) was carried out to amplify the V3–V4 region of the bacterial rRNA gene with primers 341F (5′-CCTACGGGNGGCWGCAG-3′) and 805R (5′-GACTACHVGGGTATCTAATCC-3′) (Logue et al., 2016), and the following cycling conditions: 98°C for 30 s, followed by 32 cycles of denaturation at 98°C for 10 s, annealing at 54°C for 30 s, and extension at 72°C for 45 s, and a final extension at 72°C for 10 min. The PCR products were purified using AMPure XT beads (Beckman Coulter Genomics, Danvers, MA, United States), quantified using Qubit (Invitrogen, Carlsbad, CA, United States), and then sequenced on an Illumina NovaSeq PE250 platform according to the manufacturer’s recommendations. High-quality clean tags were obtained from raw reads via quality filtering according to fqtrim (v0.94), and then filtered using Vsearch software (v2.3.4). Bacterial community diversity was calculated using QIIME2. Further, the sequence alignment of species annotation was performed using BLAST; the alignment databases were SILVA and NT-16S. Principal component analysis (PCA) of the bacterial community (at the genus level) of silages and bubble plot of the bacterial community (genus level) of silages were derived using R (version 3.2.1).1 Sequencing data were submitted to the NCBI Sequence Read Archive database (accession number: PRJNA744283).
Fermentation Quality and Nutrition Composition
Fresh silage (25 g) was homogenized with sterile water (225 ml) using a flap-type sterile homogenizer (JX-05, Shanghai Jingxin Industrial Development Co., Ltd., Shanghai, China) for 100 s and filtered through four layers of cheesecloth to obtain the silage extract (Sun et al., 2021a). The pH of the silage extract was measured using a pH meter (PB-10; Sartorius, Gottingen, Germany). The organic acids [lactic acid (LA), acetic acid (AA), propionic acid, and butyric acid] were assessed using high-performance liquid chromatography (DAD, 210 nm, SPD-20A, Shimadzu Co., Ltd., Kyoto, Japan) and the following conditions: detector, SPD-20A diode array detector (210 nm); column, Shodex RS Pak KC-811 (50°C, Showa Denko K.K., Kawasaki, Japan); and mobile phase, 3 mM HClO4 (1.0 ml/min) (Bai et al., 2021). The concentrations of ammonia nitrogen (NH3-N) and total nitrogen (TN) were determined using the Kjeldahl method with a Kjeltec autoanalyzer (8400; Foss Co., Ltd., Hillerød, Denmark) (AOAC, 2005). Water-soluble carbohydrates (WSC) were assessed using anthrone-sulfuric acid colorimetry with a spectrophotometer (UV1102II, Shanghai Tianmei Scientific Instrument Co., Ltd., Shanghai, China), according to the method described by McDonald and Henderson (1964). The buffering capacity (BC) was assessed using acid-base titration, as described by Playne and McDonald (1966).
Crude protein (CP) concentration was calculated by multiplying the TN concentration by 6.25. Neutral detergent fiber (NDF) and acid detergent fiber (ADF) were assessed using an Ankom 2000 fiber analyzer (Ankom, Macedon, NY, United States) according to the method described by Van Soest et al. (1991). Hemicellulose concentration was calculated by the NDF concentration minus the ADF concentration. Crude ash was assessed using a muffle roaster (SX-4-10N, Shanghai Jingqi Instrument Co., Ltd., Shanghai, China) at 550°C for 5 h after carbonization.
Statistical Analysis
The differences in microbial counts, sequencing data, alpha diversity, fermentation quality, and nutrition composition among treatments were analyzed with seven treatments and four repetitions using one-factor analysis of variance via the general linear model (GLM) procedure of SAS (version 9.1.3; SAS Institute Inc., Cary, NC, United States). The differences were compared using the least significant difference test, and significance was determined at p ≤ 0.05.
Results
Fermentation Quality and Nutrition Composition
The silage had lower pH and WSC concentration and higher BC content than fresh materials (p < 0.05; Table 1). The LAB-treatments had lower pH, NH3-N, and WSC than Control silage. Further, A2- and A6-treatments contained higher NH3-N than other LAB-treatments (p < 0.05). Compared with the Control silage and A1-treatment, the A4-, A5-, and A6-treatments contained lower AA (p < 0.05); A4- and A6-treatments had higher LA/AA (p < 0.05). The BC was the lowest in A4-treatment and the highest in A5-treatment among LAB-treatments (p < 0.05). No propionic and butyric acids were detected in alfalfa silages.
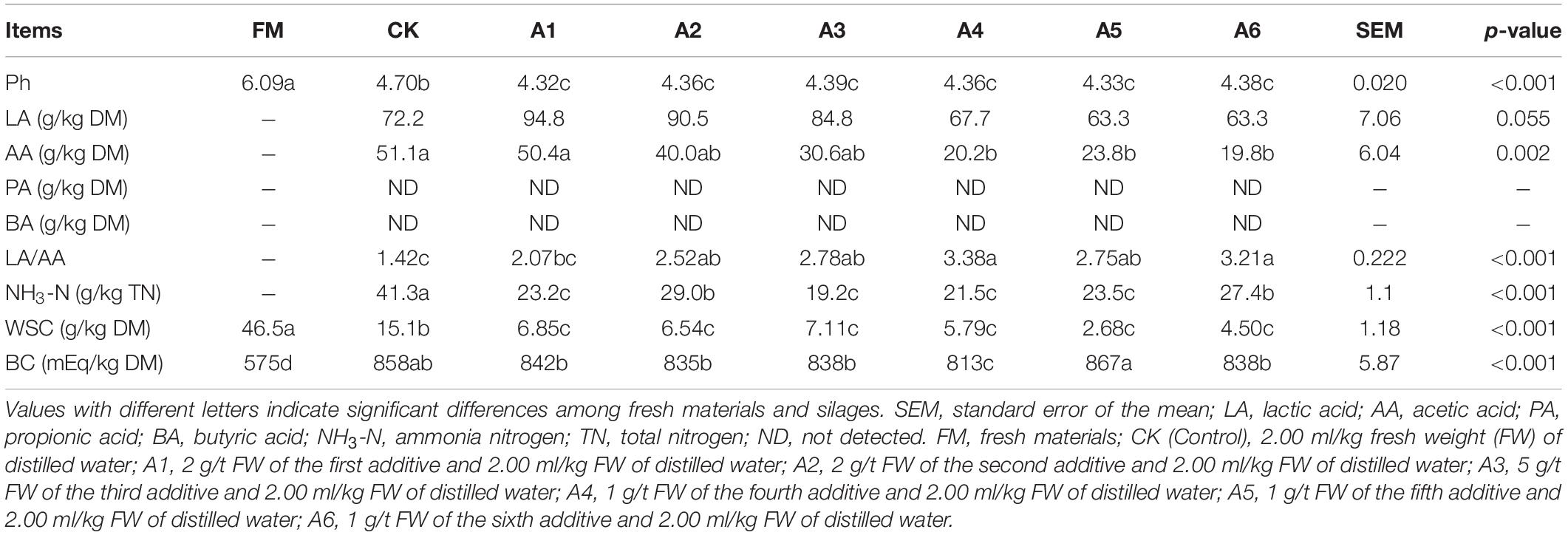
Table 1. Fermentation quality, water-soluble carbohydrates (WSC), and buffering capacity (BC) of alfalfa silages (n = 4).
The fresh material had a lower DM content than silages (p < 0.05; Table 2). The A4-treatment contained higher DM content than other treatments, with Control silage displaying a lower content than A3-treatment (p < 0.05). The NDF, ADF, and hemicellulose concentrations in A5- and A6-treatments were lower than those in other treatments and fresh materials (p < 0.05). The A3-tretment had higher NDF than Control silage and A1-treatment, with A1-treatment having a lower NDF than Control silage (p < 0.05). The A4-treatment contained the highest ADF, and A3-treatment had the greatest hemicellulose (p < 0.05). The crude ash concentration in A1-treatment was lower than that in A2- and A3-treatmetns (p < 0.05).
Microbial Counts and Bacterial Community
The Control silage and A2-treatment had greater LAB count than other treatments and fresh materials (p < 0.05). Further, A1- and A3-treatments contained higher LAB count than A4-, A5-, and A6-treatments (p < 0.05), and the A4-treatment displayed the lowest LAB count (p < 0.05; Table 3). The aerobic bacterial count in fresh materials was higher than that in the silages (p < 0.05), and the aerobic bacterial count in Control silage was higher than that in LAB-treatments (p < 0.05). Moreover, the A4- and A6-treatments had lower yeast count than other treatments and fresh materials (p < 0.05). Coliforms were detected in fresh materials but not in silages.
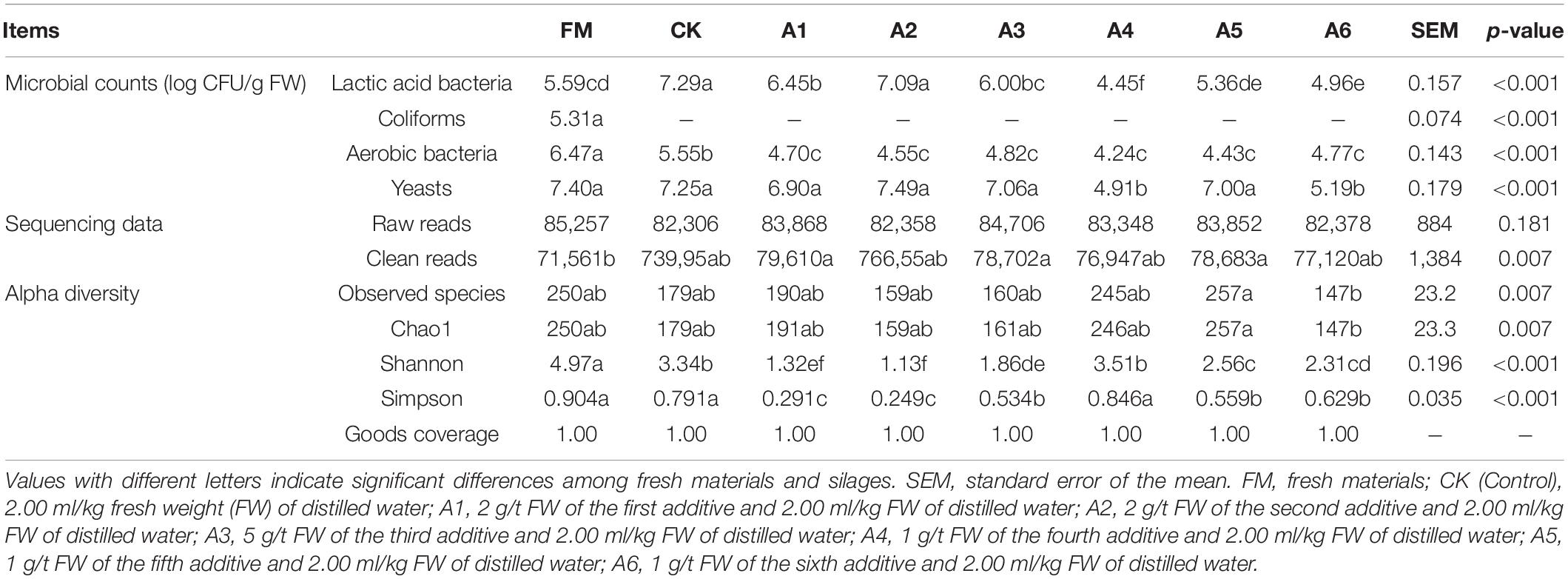
Table 3. Microbial counts, sequencing data, and alpha diversity of bacteria in alfalfa silages (n = 4).
A total of 2,672,280 raw reads and 2,453,088 clean reads of the 16S rRNA gene were obtained from the 32 samples (Table 3). There were no differences in the number of raw reads among all silages and fresh materials (p > 0.05), and the fresh materials had lower clean reads than A1-, A3-, and A5-treatments (p < 0.05). More than 83,000 raw reads and 76,000 clean reads were derived for each sample.
The A5-treatment had higher observed species and Chao1 index than A6-treatment (p < 0.05; Table 3). Fresh materials had a higher Shannon index than silages (p < 0.05), and Shannon index in Control silage and A4-treatment were higher than those in the other treatments (p < 0.05). The Simpson index for the A3-, A5-, and A6-treatments was higher than that for the A1- and A2-treatments and lower than that for the fresh materials, Control silage, and A4-treatment (p < 0.05).
According to PCA, the bacterial communities of Control silage and A6-treatment were cleanly separated from each other and from other treatments (Figure 1). However, the A1-, A2-, A3-, A4-, and A5-treatments had aggregated bacterial community. In addition, the fresh materials contained a separate bacterial community from that of the silages.
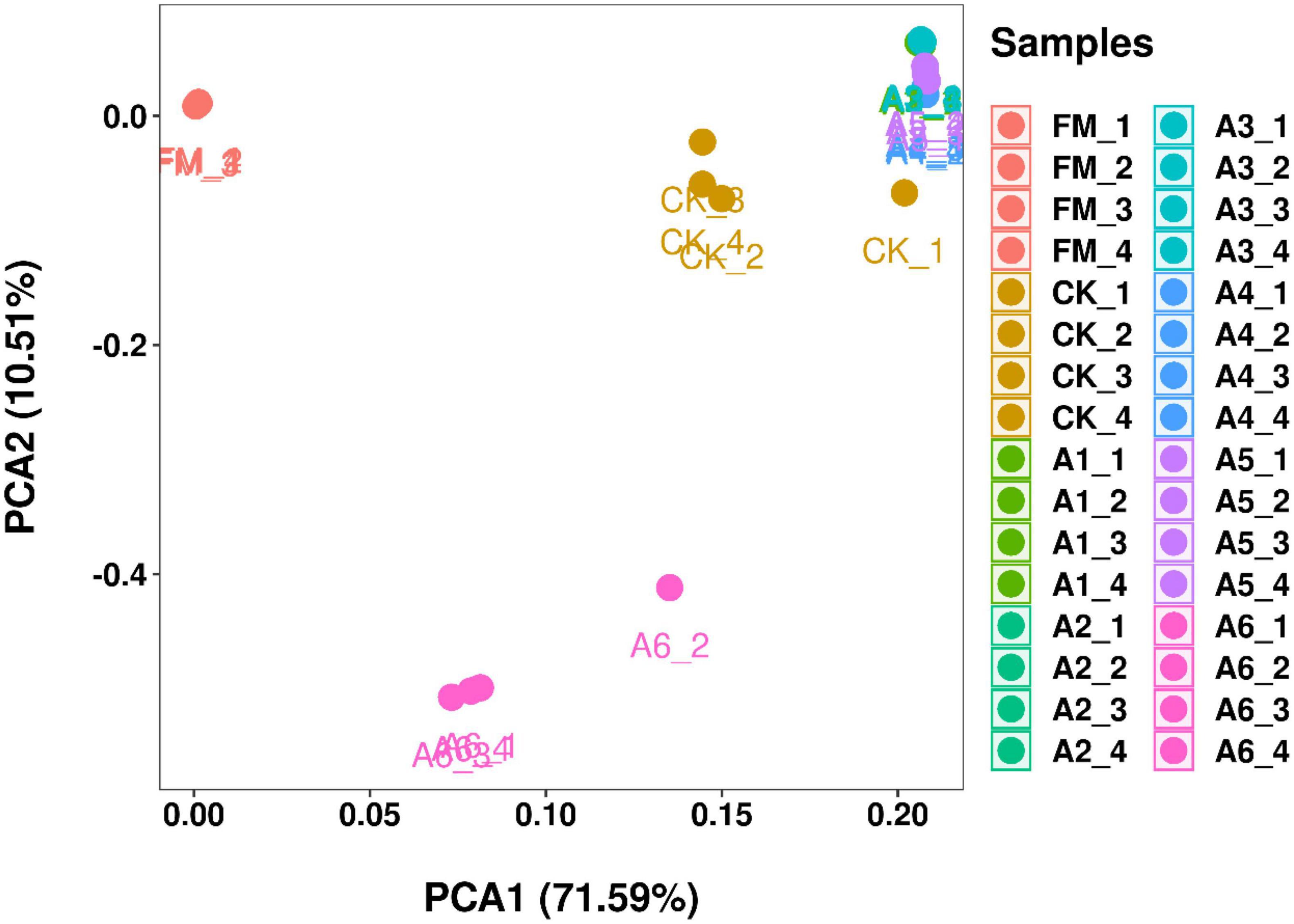
Figure 1. Principal component analysis (PCA) of the bacterial communities in silage and fresh materials (n = 4). FM, fresh materials; CK (Control), 2.00 ml/kg fresh weight (FW) of distilled water; A1, 2 g/t FW of the first additive and 2.00 ml/kg FW of distilled water; A2, 2 g/t FW of the second additive and 2.00 ml/kg FW of distilled water; A3, 5 g/t FW of the third additive and 2.00 ml/kg FW of distilled water; A4, 1 g/t FW of the fourth additive and 2.00 ml/kg FW of distilled water; A5, 1 g/t FW of the fifth additive and 2.00 ml/kg FW of distilled water; A6, 1 g/t FW of the sixth additive and 2.00 ml/kg FW of distilled water.
The most predominant bacterial genus in the A1-, A2-, A3-, A4-, and A5-treatments was Lactobacillus, with abundances of 89.32, 92.93, 92.87, 81.12, and 80.44%, respectively (Figure 2). The other main genera (>1%) were Enterococcus, Cedecea, and Devosia in A1-treatment; Enterococcus in A2- and A3-treatments; Pediococcus, Paracoccus, Devosia, and Allorhizobium-Neorhizobium-Pararhizobium-Rhizobium in A4-treatment; and Pediococcus, Enterococcus, Paracoccus, Devosia, Allorhizobium-Neorhizobium-Pararhizobium-Rhizobium, and Falsirhodobacter in A5-treatment. The main bacterial genera in Control silage and A6-treatment were Lactobacillus (42.18 and 24.86%, respectively), Pediococcus (8.09 and 70.14%, respectively), and Enterococcus (40.18 and 1.40%, respectively), followed by Pantoea, Paracoccus, and Weissella in Control silage (>1%). Further, Pantoea, Enterobacter, and Pseudomonas were the dominant bacterial genera in fresh materials, with abundances of 37.59, 21.20, and 15.74%, respectively (Figure 2).
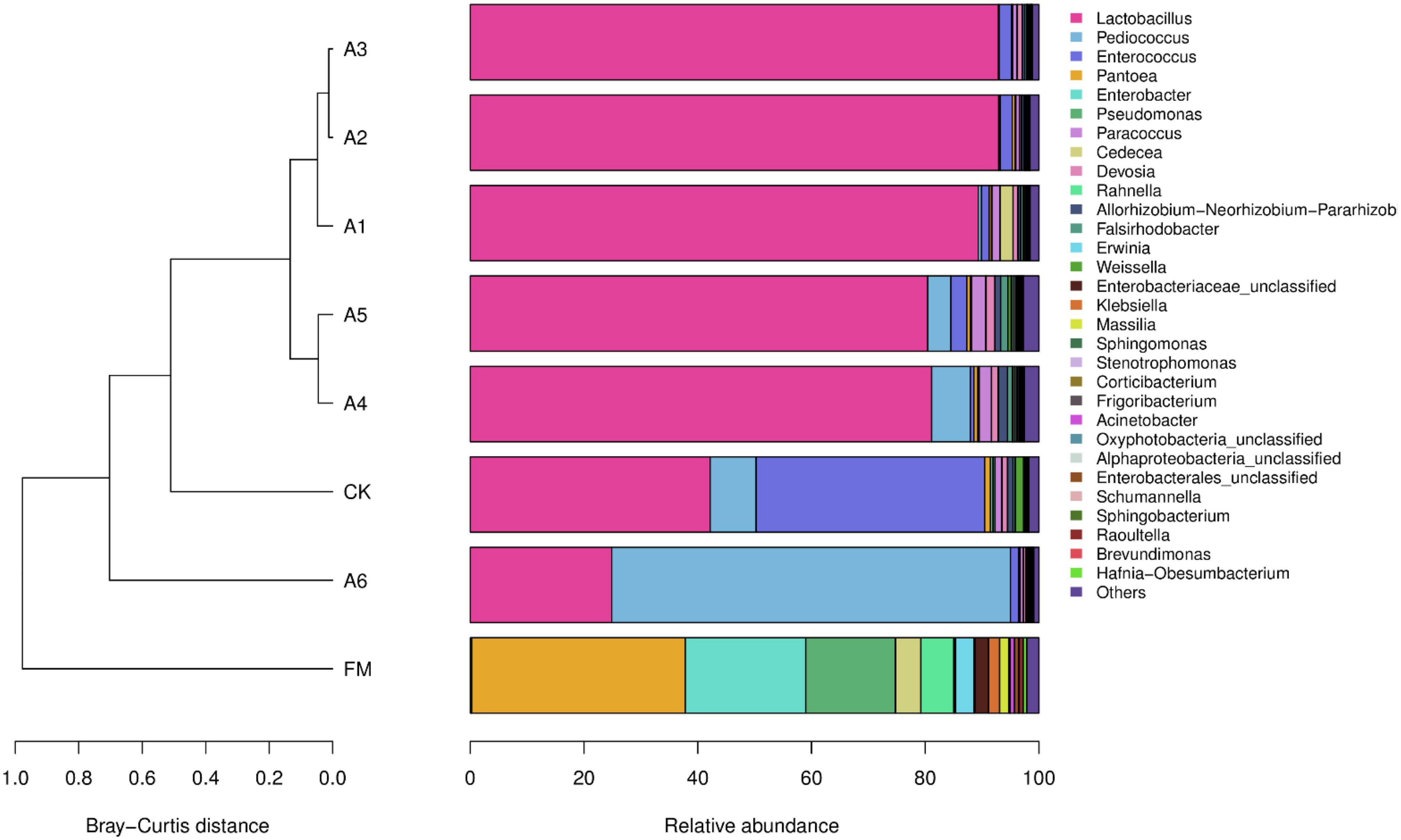
Figure 2. Relative abundance of the bacterial community (genus level) in silages and fresh materials (FM) (n = 4). FM, fresh materials; CK (Control), 2.00 ml/kg fresh weight (FW) of distilled water; A1, 2 g/t FW of the first additive and 2.00 ml/kg FW of distilled water; A2, 2 g/t FW of the second additive and 2.00 ml/kg FW of distilled water; A3, 5 g/t FW of the third additive and 2.00 ml/kg FW of distilled water; A4, 1 g/t FW of the fourth additive and 2.00 ml/kg FW of distilled water; A5, 1 g/t FW of the fifth additive and 2.00 ml/kg FW of distilled water; A6, 1 g/t FW of the sixth additive and 2.00 ml/kg FW of distilled water.
Difference in Bacterial Community Among Silages and Fresh Materials
Compared with fresh materials, silages had higher Lactobacillus, Pediococcus, and Enterococcus (p < 0.05) and lower Pantoea, Enterobacter, Pseudomonas, Cedecea, and Rahnella (p < 0.05) (Figure 3). Control silage and A6-treatment contained less Lactobacillus than other treatments, with Control silage having higher than the A6-treatments (p < 0.05). The A6-treatment had higher Pediococcus than other treatments, with A1-, A2-, and A3-treatments displaying a lower than A4- and A5-treatments and Control silage (p < 0.05). Control silage had higher Enterococcus than other treatments (p < 0.05).
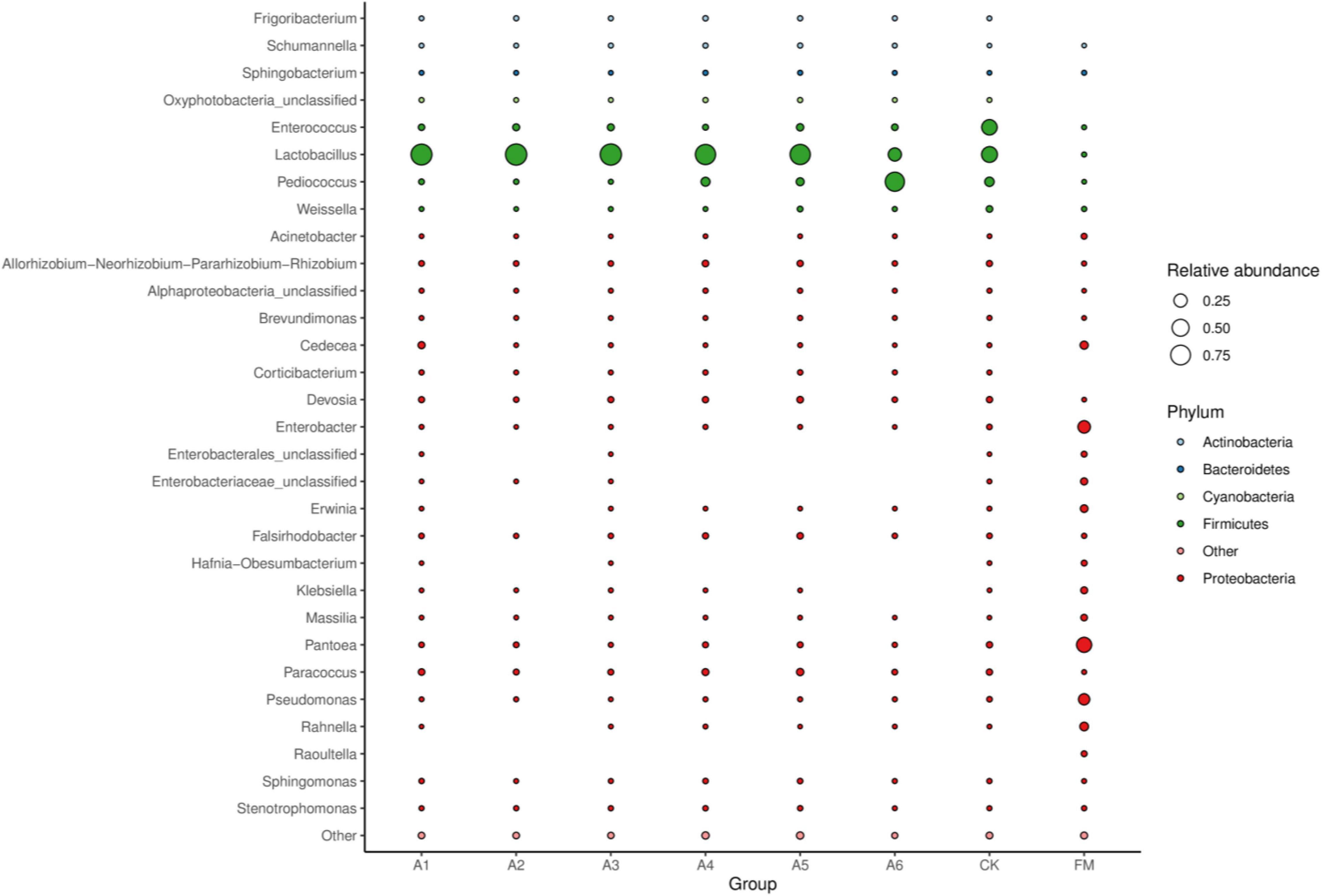
Figure 3. Bubble plot of the bacterial community (genus level) among silages and fresh material (n = 4, p < 0.05). FM, fresh materials; CK (Control), 2.00 ml/kg fresh weight (FW) of distilled water; A1, 2 g/t FW of the first additive and 2.00 ml/kg FW of distilled water; A2, 2 g/t FW of the second additive and 2.00 ml/kg FW of distilled water; A3, 5 g/t FW of the third additive and 2.00 ml/kg FW of distilled water; A4, 1 g/t FW of the fourth additive and 2.00 ml/kg FW of distilled water; A5, 1 g/t FW of the fifth additive and 2.00 ml/kg FW of distilled water; A6, 1 g/t FW of the sixth additive and 2.00 ml/kg FW of distilled water.
Discussion
Characteristics of Alfalfa Pre-ensiling
A previous study reported that ensiling alfalfa with satisfactory fermentation quality is difficult because of low LAB count, less WSC concentration, and higher BC in fresh forage (Sun et al., 2021b). In the present study, the epiphytic LAB count (5.59 log CFU/g FW, Table 3) in alfalfa pre-ensiling met the criteria (105 log CFU/g FW) required for adequate fermentation (McDonald et al., 1991). However, the alfalfa pre-ensiling contained less WSC and moisture contents (46.5 g/kg DM and 515 g/kg) and higher BC (575 mEq/kg DM) (Tables 1, 2), resulting in higher pH (4.70), more NH3-N (41.3 g/kg), and lower LA/AA (1.42) in Control silage than those in LAB-treatments (Table 1). These suggest that it is necessary to ensile alfalfa with LAB additive for good fermentation quality. The LAB genera (Weissella, Lactobacillus, Enterococcus, and Pediococcus) were detected in alfalfa pre-ensiling with total abundance of 0.38% (Figure 2). Other studies found that the LAB genera have total abundance of less than 1.0% in alfalfa pre-ensiling (Hu et al., 2020; Zhao S. et al., 2021) and fresh whole-plant corn (Xu et al., 2019; Guan et al., 2020). The LAB genera were demonstrated to be generally presented as minor taxa in forage pre-ensiling. The main bacterial genera in alfalfa pre-ensiling were Pantoea (37.59%), Enterobacter (21.20%), and Pseudomonas (15.74%) (Figure 2). The findings agreed with those reported by Zhao S. et al. (2021) for high-moisture alfalfa [Enterobacter (33.93%), Pseudomonas (16.67%), and Pantoea (7.09%)]. However, other studies reported that the main bacterial genera (>10% of abundance) in alfalfa pre-ensiling were Pseudomonas, Exiguobacterium, and Massilla (Yang et al., 2020), Sphingobium (Hu et al., 2020), Xanthomonas and Cyanobacteria (Guo et al., 2020), and Exiguobacterium (Wang et al., 2019). The different bacterial communities in alfalfa pre-ensiling among those studies might be due to the differences in geographical locations (Wang C. et al., 2021).
Fermentation Quality and Nutrition Composition of Silage
Ensiling alfalfa with LAB inoculants improves fermentation quality, as demonstrated by the increased LA content and decreased pH and NH3-N (Guo et al., 2020; Hu et al., 2020). In the present study, LAB inoculation at ensiling decreased pH and NH3-N in alfalfa silage. However, no difference in LA content was detected among all treatments, and the AA content in the Control silage and A1-treatment were higher than that in A4-, A5-, and A6-treatments. In addition, Control silage contained more WSC than LAB-treatments (15.1 vs. 2.86–7.11 g/kg DM); however, BC did not differ between Control silage and LAB-treatments (except A4) (Table 1). Lactobacillus, as the principal component of the additives used in the present study, was negatively correlated with WSC content in alfalfa silage (Supplementary Figure 1). The results suggest that other fermentation products (valeric acid, caproic acid, succinic acid, citric acid, ethanol, propanol, and 1,2-propandiol) might be generated during the ensiling process in LAB-treatments, and inoculating LAB at ensiling increased the utilization of WSC in silage during the fermentation process. This phenomenon was also observed in alfalfa silage (Xie et al., 2021), whole-plant corn silage (Jiang et al., 2020), and whole-plant sweet sorghum silage (Diepersloot et al., 2021). Inoculating heterofermentative LAB at ensiling reduces LA/AA in terminal silage by decreasing LA and increasing AA (Kung et al., 2018). The additives (except A5) used in the present study contained heterofermentative LAB (A1, L. buchneri; A2, L. casei; A3, L. buchneri; A4, L. buchneri, L. casei; A6, L. buchneri). However, A5-treatment had no difference in LA relative to other treatments; AA relative to A2-, A3-, A4-, and A6-treatments; and LA/AA relative to other LAB-treatments (Table 1). The finding might be due to homofermentative LAB dominating the fermentation process, as reflected by the total abundance of L. plantarum, E. mundtii, E. faecium, and P. acidilactici in Control silage and LAB-treatments (58.50, 85.50, 88.76, 73.21, 64.16, 74.42, and 77.28%, respectively) (Supplementary Table 1). Moreover, Guo et al. (2020) reported the undifferenced LA and AA concentrations between alfalfa silages treated with homo- and hetero-fermentative LAB.
During fermentation, proteolysis in silage is inevitable, owing to the presence of plant and microbial proteases (Thomas et al., 1980; Hassanat et al., 2007). The NH3-N, as part of the non-protein, shows the extent of silage preservation during the ensiling process, owing to its low utilization in the rumen (Xue et al., 2017; Yin et al., 2017). In the present study, NH3-N (41.3 g/kg TN) in Control silage was lower than the suggested concentrations in legume silage (< 120 g/kg TN) (Kung et al., 2018), indicating that the Control silage was well preserved. This finding might be because the higher DM content (480 g/kg) and the ideal anaerobic environment during the ensilage process cause a decrease in the activity of undesired microorganisms during ensiling. Propionic acid, butyric acid, coliforms, and Clostridia were not detected in any of the silages (Table 1 and Figure 2). Furthermore, compared with Control silage, the LAB-treatments displayed lower pH (4.70 vs. 4.32– to 4.39), NH3-N (41.3 vs. 19.2– to 29 g/kg TN), Enterobacteriaceae (2.43% vs. 0.1–% to 0.43%), and potentially pathogenic bacteria (6.50% vs. 1.05–% to 1.86%, expect A1) (Table 1 and Supplementary Figures 2, 3). This finding indicated that the fermentation products of the LAB additives used in the present study contributed more to the preservation of silage and the inhibition of undesired microorganisms during fermentation under low pH, low moisture, and ideal anaerobic conditions. Previous studies reported that LAB inoculation at ensiling decreased NH3-N content in alfalfa silage (Hu et al., 2020; Sun et al., 2021b).
In the present study, compared with Control silage, LAB-treatments had higher DM content, although the difference did not reach a significant level among Control silage and the A1-, A2-, A3-, and A6-treatments. Moreover, there were no differences in CP concentration among the silages (Table 2). Previous studies revealed that inoculating LAB at ensiling alfalfa increases the contents of DM and CP and improves the fermentation quality of terminal silage (Li et al., 2020; Wu et al., 2020). The results suggest that satisfactory fermentation quality contributes to increasing the DM content and preserving the CP of alfalfa silage. The concentrations of NDF, ADF, and hemicellulose in the A5- and A6-treatments were lower than those in the other treatments (Table 2), which might be related to the function of L. plantarum (producing feruloyl esterase) in A5 and the composition of A6 (β-glucanase and xylanase). Feruloyl esterase can promote cell wall degradation, especially in collaboration with cellulase and hemicellulose, by cleaving the ester or ether linkages between ferulic acid and sugars (Dilokpimol et al., 2016; Duan et al., 2021). Su et al. (2019) reported that inoculating feruloyl esterase-producing Lactobacillus fermentum at ensiling alfalfa decreased the NDF and ADF concentrations in terminal silage. β-glucanase, as one type of cellulase, can cleave glycosidic bonds in the amorphous regions of cellulose polymers (Takizawa et al., 2020). Moreover, xylanase contributes to the degradation of hemicellulose (Paës et al., 2012; Vucinic et al., 2021). Collectively, these findings indicate that A5 and A6, as additives, can degrade the cell wall during fermentation in alfalfa silage.
Microbial Counts and Bacterial Community of Silage
Inoculating LAB at ensiling can increase LAB count in the terminal silage (Hu et al., 2020; Zhang et al., 2020). However, in the present study, the Control silage and A2-treatment displayed more LAB counts than the other treatments (Table 3). Moreover, the Control silage had the highest pH and aerobic bacterial count (Tables 1, 3). These results suggest that the LAB in the A2-treatment might have better resistance to less moisture and a low pH environment (494 g/kg and 4.26, respectively), and the microorganisms in the Control silage had greater activity under less moisture and weakly acidic conditions (504 g/kg and 4.70, respectively). The A4- and A6-treatments contained lower yeast counts than the other treatments (Table 3), which might be related to the presence of P. acidilactici in A4 and A6 as additives used in the present study. Previous studies reported that P. acidilactici inhibits effects on other microorganisms by producing antimicrobial bacteriocins (Kaya and Simsek, 2020; Surachat et al., 2021).
In the present study, most bacteria were detected in all samples the goods’ coverages reached approximately 1 (Table 3). The bacterial diversity of the silages was lower than that of the alfalfa pre-ensiling (Table 3). Furthermore, the material had a clearly separated bacterial community from the silages (Figure 1); similar results were reported by Zheng et al. (2017) and Zhao S. et al. (2021). This finding might be due to the large increasing abundance of LAB genera as the main bacterial taxa in silages (87.90%–95.30%) (Figures 2, 3). The Shannon and Simpson indexes for A4-treatment were higher than those for other LAB-treatments and did not differ from those of Control silage. Moreover, A1- and A2-treatments had lower Shannon and Simpson indexes than the other treatments (Table 3). These results suggest that the bacterial diversity was higher in the Control silage and A4-treatment, but lower in the A1- and A2-treatments. Interestingly, the same trend was detected in the number of main bacterial species, with > 10% abundance (Supplementary Figure 4). The bacterial communities in the Control silage and A6-treatment separated clearly from those of other treatments (Figure 1) due to the less abundance of Lactobacillus detected in the former (Figures 2, 3). Moreover, Control silage contained more Lactobacillus and Enterococcus and less Pediococcus than A6-treatment (Figures 2, 3), resulting in the separation of the bacterial communities between them (Figure 1).
Inoculation of LAB at ensiling optimizes the bacterial community and improves the fermentation quality of the terminal silage (Zhang et al., 2021; Zhao S. et al., 2021). In general, Lactobacillus dominates the bacterial community in well-preserved silage owing to its great capacity to produce acid and reduce pH during ensiling (Zi et al., 2021). In the present study, compared with Control silage, the LAB-treatments contained different bacterial communities (Figure 1) and had lower pH and NH3-N/TN (Table 1). Moreover, Lactobacillus dominated the bacterial communities in the A1-, A2-, A3-, A4-, and A5-treatments (89.32, 92.93, 92.87, 81.12, and 80.44%, respectively) (Figure 2). Nevertheless, the most dominant genus in A6-treatment was Pediococcus (70.14%) (Figure 2), which also caused lower pH, AA, and NH3-N/TN, and higher LA/AA than those for Control silage (Table 1). Such finding indicates that alfalfa silage is also well-preserved, with Pediococcus being the dominant genus. The difference in the most dominant bacterial genus among LAB-treatments might be related to the composition of the commercial additives used in the present study. Lactobacillus plantarum had the highest composition in A1, A2, A3, A4, and A5. Additionally, previous studies revealed that silage treated with L. plantarum had a greater abundance of Lactobacillus than Control silage (Zhang et al., 2020; Zhao C. et al., 2021). These results demonstrate that ensiling forage with L. plantarum increases the abundance of Lactobacillus in the terminal silage. Inoculating P. acidilactici, as one of the two components of A6 (L. buchneri, ≥ 7.5 × 1010 CFU/g; P. acidilactici, ≥ 5.0 × 1010 CFU/g), increased the abundance of Pediococcus in A6-treatment compared with other LAB-treatments (70.14% vs. 0.11–6.81%) (Figure 2). Moreover, the A4-treatment contained higher Pediococcus than A1-, A2-, A3-, and A5-treatments (6.81% vs. 0.11–4.07%), and P. acidilactici is one of the four components of A4. These results indicate that ensiling alfalfa with P. acidilactici increases the abundance of Pediococcus in the terminal silage. Lactobacillus buchneri, as one of the components of A1, A3, A4, and A6, was only detected in A6-treatment, with 0.17% abundance (Supplementary Table 1). Previous studies reported that L. buchneri, as an inoculant at ensiling, was detected as a minor taxon in alfalfa silage (Guo et al., 2020) and whole-plant corn silage (Xu et al., 2020; Netthisinghe et al., 2021). Moreover, E. faecalis (one of compositions of A1) was only detected in A1- and A2-treatments, with 0.025 and 0.001% abundances, respectively, and L. casei (one of compositions of A2 and A4) was only present in A6-treatment (0.011%) (Supplementary Table 1). These results indicate that L. buchneri, L. casei, and E. faecalis might have weaker competitiveness than L. plantarum and P. acidilactici in alfalfa silage with less moisture and low pH environments (475–497 g/kg and 4.33–4.39, respectively). The role of these LAB as the main components of LAB additives during the fermentation process in silage requires further study. The main bacterial genera in Control silage were Lactobacillus (42.18%), Enterococcus (40.18%), and Pediococcus (8.09%), indicating that the LAB genera dominated the bacterial community in Control silage (DM = 496 g/kg). Previous studies reported that the LAB population dominates the bacterial community in low-moisture alfalfa silage (DM > 400 g/kg) without any treatment (Guo et al., 2018, 2020) and presents as minor taxa in high-moisture alfalfa silage (DM < 270 g/kg) without any inoculants (Yang et al., 2020; Zhao S. et al., 2021). These results suggest that wilting alfalfa pre-ensiling may increase the total abundance of LAB genera in the bacterial community of alfalfa silage.
Conclusion
The LAB genera are present as minor taxa in fresh alfalfa. Inoculating commercial LAB additives at ensiling alfalfa improved the fermentation quality, contributed to the preservation, and altered the bacterial community of the terminal silage. Lactobacillus, Enterococcus, and Pediococcus dominated the bacterial community in the Control silage. Lactobacillus was the most dominant bacterial genus in the A1-, A2-, A3-, A4-, and A5-treatments, and Pediococcus was the most dominant in A6-treatment. Further, addition of A5 and A6 decreased the concentrations of NDF, ADF, and hemicellulose in silage. Overall, the commercial lactic acid bacterial additives used in the present study can be employed to inoculate ensiling alfalfa in Northern China.
Data Availability Statement
The datasets presented in this study can be found in online repositories. The names of the repository/repositories and accession number(s) can be found in the article/Supplementary Material.
Author Contributions
NN, YX, and YT designed the study. NN wrote the manuscript. NN, MQ, NW, LS, YZ, and XW performed the experiments. HX, YX, and YT reviewed and edited the manuscript. NN, MQ, NW, HX, and YZ analyzed the data. YX and YT funded and supervised the experiments. All authors reviewed the manuscript.
Funding
This work was funded by the National Natural Science Foundation of China (32160342 and 31772674), the Inner Mongolia Science and Technology Plan (2020GG0049), and Inner Mongolia Agriculture and Animal Husbandry Innovation Fund (2022CYZX04).
Conflict of Interest
XW and YT were employed by Inner Mongolia Youran Animal Husbandry Co., Ltd.
The remaining authors declare that the research was conducted in the absence of any commercial or financial relationships that could be construed as a potential conflict of interest.
Publisher’s Note
All claims expressed in this article are solely those of the authors and do not necessarily represent those of their affiliated organizations, or those of the publisher, the editors and the reviewers. Any product that may be evaluated in this article, or claim that may be made by its manufacturer, is not guaranteed or endorsed by the publisher.
Supplementary Material
The Supplementary Material for this article can be found online at: https://www.frontiersin.org/articles/10.3389/fmicb.2022.836899/full#supplementary-material
Supplementary Figure 1 | Redundancy analysis of the bacterial community (top 10 genera) and fermentation quality of alfalfa silage (n = 4).
Supplementary Figure 2 | Relative abundance of the bacterial community (family level) in alfalfa silage (n = 4).
Supplementary Figure 3 | Relative abundance of potentially pathogenic bacteria in alfalfa silage (n = 4).
Supplementary Figure 4 | Relative abundance of the bacterial community (species level) in alfalfa silage (n = 4).
Supplementary Table 1 | Relative abundance of lactic acid bacteria (species level) in alfalfa silage (n = 4).
Footnotes
References
Bai, C., Wang, C., Sun, L., Xu, H., Jiang, Y., Na, N., et al. (2021). Dynamics of bacterial and fungal communities and metabolites during aerobic exposure in whole-plant corn silages with two different moisture levels. Front. Microbiol. 12:663895. doi: 10.3389/fmicb.2021.663895
Besharati, M., Palangi, V., Ghozalpour, V., Nemati, Z., and Ayaşan, T. (2021). Essential oil and apple pomace affect fermentation and aerobic stability of alfalfa silage. S. Afr. J. Anim. Sci. 51, 371–377. doi: 10.4314/sajas.v51i3.11
Cai, Y. (1999). Identification and characterization of Enterococcus species isolated from forage crops and their influence on silage fermentation. J. Dairy Sci. 82, 2466–2471. doi: 10.3168/jds.S0022-0302(99)75498-6
Diepersloot, E. C., Pupo, M. R., Ghizzi, L. G., Gusmão, J. O., Heinzen, C. Jr., McCary, C. L., et al. (2021). Effects of microbial inoculation and storage length on fermentation profile and nutrient composition of whole-plant sorghum silage of different varieties. Front. Microbiol. 12:660567. doi: 10.3389/fmicb.2021.660567
Dilokpimol, A., Makela, M. R., Aguilar-Pontes, M. V., Benoit-Gelber, I., Hilden, K. S., and de Vries, R. P. (2016). Diversity of fungal feruloyl esterases: updated phylogenetic classification, properties, and industrial applications. Biotechnol. Biofuels 9:231. doi: 10.1186/s13068-016-0651-6
Duan, X., Dai, Y., and Zhang, T. (2021). Characterization of feruloyl esterase from Bacillus pumilus SK52.001 and its application in ferulic acid production from de-starched wheat bran. Foods 10:1229. doi: 10.3390/foods10061229
Gao, R., Wang, B., Jia, T., Luo, Y., and Yu, Z. (2021). Effects of different carbohydrate sources on alfalfa silage quality at different ensiling days. Agriculture 11:58. doi: 10.3390/agriculture11010058
Guan, H., Shuai, Y., Yan, Y., Ran, Q., Wang, X., Li, D., et al. (2020). Microbial community and fermentation dynamics of corn silage prepared with heat-resistant lactic acid bacteria in a hot environment. Microorganisms 8:719. doi: 10.3390/microorganisms8050719
Guo, L., Yao, D., Li, D., Lin, Y., Bureenok, S., Ni, K., et al. (2020). Effects of lactic acid microbial community, and in vitro digestibility of alfalfa silage. Front. Microbiol. 10:2998. doi: 10.3389/fmicb.2019.02998
Guo, X. S., Ke, W. C., Ding, W. R., Ding, L. M., Xu, D. M., Wang, W. W., et al. (2018). Profiling of metabolome and bacterial community dynamics in ensiled Medicago sativa inoculated without or with Lactobacillus plantarum or Lactobacillus buchneri. Sci. Rep. 8:357. doi: 10.1038/s41598-017-18348-0
Hartinger, T., Gresner, N., and Südekum, K. (2019). Effect of wilting intensity, dry matter content and sugar addition on nitrogen fractions in lucerne silages. Agriculture 9:11. doi: 10.3390/agriculture9010011
Hassanat, F., Mustafa, A. F., and Seguin, P. (2007). Effects of inoculation on ensiling characteristics, chemical composition and aerobic stability of regular and brown midrib millet silages. Anim. Feed Sci. Technol. 139, 125–140. doi: 10.1016/j.anifeedsci.2007.01.005
Hu, Z., Niu, H., Tong, Q., Chang, J., Yu, J., Li, S., et al. (2020). The microbiota dynamics of alfalfa silage during ensiling and after air exposure, and the metabolomics after air exposure are affected by Lactobacillus casei and cellulase addition. Front. Microbiol. 11:519121. doi: 10.3389/fmicb.2020.519121
Jiang, F., Cheng, H., Liu, D., Wei, C., An, W., Wang, Y., et al. (2020). Treatment of whole-plant corn silage with lactic acid bacteria and organic acid enhances quality by elevating acid content, reducing pH, and inhibiting undesirable microorganisms. Front. Microbiol. 11:593088. doi: 10.3389/fmicb.2020.593088
Kaya, H. I., and Simsek, O. (2020). Characterization of Pediococcus acidilactici PFC69 and Lactococcus lactis PFC77 bacteriocins and their antimicrobial activities in tarhana fermentation. Microorganisms 8:1083. doi: 10.3390/microorganisms8071083
Kung, L. Jr., Shaver, R. D., Grant, R. J., and Schmidt, R. J. (2018). Silage review: Interpretation of chemical, microbial, and organoleptic components of silages. J. Dairy Sci. 101, 4020–4033. doi: 10.3168/jds.2017-13909
Li, F., Ding, Z., Adesogan, A. T., Ke, W., Jiang, Y., Bai, J., et al. (2020). Effects of class IIa bacteriocin-producing Lactobacillus species on fermentation quality and aerobic stability of alfalfa silage. Animals 10, 1575. doi: 10.3390/ani10091575
Logue, J. B., Stedmon, C. A., Kellerman, A. M., Nielsen, N. J., and Andersson, A. F. (2016). Experimental insights into the importance of aquatic bacterial community composition to the degradation of dissolved organic matter. ISME J. 10, 533–545. doi: 10.1038/ismej.2015.131
Luo, R., Zhang, Y., Wang, F., Liu, K., Huang, G., Zheng, N., et al. (2021). Effects of sugar cane molasses addition on the fermentation quality, microbial community, and tastes of alfalfa silage. Animals 11:355. doi: 10.3390/ani11020355
McDonald, P., and Henderson, A. R. (1964). Determination of water-soluble carbohydrates in grass. J. Sci. Food Agr. 15, 395–398. doi: 10.1002/jsfa.2740150609
McDonald, P., Henderson, A. R., and Heron, S. J. E. (1991). The Biochemistry of Silage, 2nd Edn. Wales: Cambrian Printers, Ltd.
Netthisinghe, A., Woosley, P., Rowland, N., Willian, T., Gilfillen, B., and Sistani, K. (2021). Alfalfa forage production and nutritive value, fermentation characteristics and hygienic quality of ensilage, and soil properties after broiler litter amendment. Agronomy 11:701. doi: 10.3390/agronomy11040701
Paës, G., Berrin, J. G., and Beaugrand, J. (2012). GH11 xylanases: structure/function/properties relationships and applications. Biotechnol. Adv. 30, 564–592. doi: 10.1016/j.biotechadv.2011.10.003
Playne, M. J., and McDonald, P. (1966). The buffering constituents of herbage and silage. J. Sci. Food Agr. 17, 264–268. doi: 10.1002/jsfa.2740170609
Schmidt, R. J., Hu, W., Mills, J. A., and Kung, L. Jr. (2009). The development of lactic acid bacteria and Lactobacillus buchneri and their effects on the fermentation of alfalfa silage. J. Dairy Sci. 92, 5005–5010. doi: 10.3168/jds.2008-1701
Silva, V. P., Pereira, O. G., Leandro, E. S., Da Silva, T. C., Ribeiro, K. G., Mantovani, H. C., et al. (2016). Effects of lactic acid bacteria with bacteriocinogenic potential on the fermentation profile and chemical composition of alfalfa silage in tropical conditions. J. Dairy Sci. 99, 1895–1902. doi: 10.3168/jds.2015-9792
Su, R., Ni, K., Wang, T., Yang, X., Zhang, J., Liu, Y., et al. (2019). Effects of ferulic acid esterase-producing Lactobacillus fermentum and cellulase additives on the fermentation quality and microbial community of alfalfa silage. PeerJ 7:e7712. doi: 10.7717/peerj.7712
Sun, L., Bai, C., Xu, H., Na, N., Jiang, Y., Yin, G., et al. (2021a). Succession of bacterial community during the initial aerobic, intense fermentation, and stable phases of whole-plant corn silages treated with lactic acid bacteria suspensions prepared from other silages. Front. Microbiol. 12:655095. doi: 10.3389/fmicb.2021.655095
Sun, L., Jiang, Y., Ling, Q., Na, N., Xu, H., Vyas, D., et al. (2021b). Effects of adding pre-fermented fluid prepared from lucerne or red clover on fermentation quality and in vitro digestibility of the ensiled wilting-forages. Agriculture 11:454. doi: 10.3390/agriculture11050454
Surachat, K., Kantachote, D., Deachamag, P., and Wonglapsuwan, M. (2021). Genomic insight into Pediococcus acidilactici HN9, a potential probiotic strain isolated from the traditional Thai-Style fermented beef nhang. Microorganisms 9:50. doi: 10.3390/microorganisms9010050
Takizawa, S., Asano, R., Fukuda, Y., Feng, M., Baba, Y., Abe, K., et al. (2020). Change of endoglucanase activity and rumen microbial community during biodegradation of cellulose using rumen microbiota. Front. Microbiol. 11:603818. doi: 10.3389/fmicb.2020.603818
Thomas, P. C., Chamberlain, D. G., Kelly, N. C., and Wait, M. K. (1980). The nutritive value of silages digestion of nitrogenous constituents in sheep receiving diets of grass-silage and grass silage and barley. Br. J. Nutr. 43, 469–479. doi: 10.1079/BJN19800114
Van Soest, P. J., Roberts, J., and Lewis, B. A. (1991). Methods for dietary fibre neutral detergent fibre and nonstarch polysaccharides in relation to animal nutrition. J. Dairy Sci. 74, 3583–3594. doi: 10.3168/jds.S0022-0302(91)78551-2
Vucinic, J., Novikov, G., Montanier, C. Y., Dumon, C., Schiex, T., and Barbe, S. (2021). A comparative study to decipher the structural and dynamics determinants underlying the activity and thermal stability of GH-11 xylanases. Int. J. Mol. Sci. 22:5961. doi: 10.3390/ijms22115961
Wang, B., Sun, Z., and Yu, Z. (2020). Pectin degradation is an important determinant for alfalfa silage fermentation through the rescheduling of the bacterial community. Microorganisms 8:488. doi: 10.3390/microorganisms8040488
Wang, C., Han, H., Sun, L., Na, N., Xu, H., Chang, S., et al. (2021). Bacterial succession pattern during the fermentation process in whole-plant corn silage processed in different geographical areas of Northern China. Processes 9:900. doi: 10.3390/pr9050900
Wang, C., He, L., Xing, Y., Zhou, W., Yang, F., Chen, X., et al. (2019). Effects of mixing Neolamarckia cadamba leaves on fermentation quality, microbial community of high moisture alfalfa and stylo silage. Microb. Biotechnol. 12, 869–878. doi: 10.3390/10.1111/1751-7915.13429
Wang, M., Gao, R., Franco, M., Hannaway, D. B., Ke, W., Ding, Z., et al. (2021). Effect of mixing alfalfa with whole-plant corn in different proportions on fermentation characteristics and bacterial community of silage. Agriculture 11:174. doi: 10.3390/agriculture11020174
Weissbach, F., and Strubelt, C. (2008). Correcting the dry matter content of grass silages as a substrate for biogas production. J. Agric. Eng. 63:210.
Wu, Z., Xu, S., Yun, Y., Jia, T., and Yu, Z. (2020). Effect of 3-phenyllactic acid and 3-phenyllactic acid-producing lactic acid bacteria on the characteristics of alfalfa silage. Agriculture 10:10. doi: 10.3390/agriculture10010010
Xie, Y., Bao, J., Li, W., Sun, Z., Gao, R., Wu, Z., et al. (2021). Effects of applying lactic acid bacteria and molasses on the fermentation quality, protein fractions and in vitro digestibility of baled alfalfa silage. Agronomy 11:91. doi: 10.3390/agronomy11010091
Xu, D., Ding, W., Ke, W., Li, F., Zhang, P., and Guo, X. (2019). Modulation of metabolome and bacterial community in whole crop corn silage by inoculating homofermentative Lactobacillus plantarum and heterofermentative Lactobacillus buchneri. Front. Microbiol. 9:3299. doi: 10.3389/fmicb.2018.03299
Xu, D., Ding, Z., Wang, M., Bai, J., Ke, W., Zhang, Y., et al. (2020). Characterization of the microbial community, metabolome and biotransformation of phenolic compounds of sainfoin (Onobrychis viciifolia) silage ensiled with or without inoculation of Lactobacillus plantarum. Bioresour. Technol. 316:123910. doi: 10.1016/j.biortech.2020.123910
Xue, Y., Bai, C., Sun, J., Sun, L., Chang, S., Sun, Q., et al. (2017). Effects of locations and growth stages on nutritive value and silage fermentation quality of Leymus chinensis in Eurasian steppe of northern China. Grassl. Sci. 64, 40–50. doi: 10.1111/grs.12177
Yang, F., Wang, Y., Zhao, S., and Wang, Y. (2020). Lactobacillus plantarum Inoculants delay spoilage of high moisture alfalfa silages by regulating bacterial community composition. Front. Microbiol. 11:1989. doi: 10.3389/fmicb.2020.01989
Yang, F., Zhao, S., Wang, Y., Fan, X., Wang, Y., and Feng, C. (2021). Assessment of bacterial community composition and dynamics in alfalfa silages with and without Lactobacillus plantarum inoculation using absolute quantification 16S rRNA sequencing. Front. Microbiol. 11:629894. doi: 10.3389/fmicb.2020.629894
Yin, G., Bai, C., Sun, J., Sun, L., Xue, Y., Zhang, Y., et al. (2017). Fermentation quality and nutritive value of total mixed ration silages based on desert wormwood (Artemisia desertorum Spreng.) combining with early stage corn. Anim. Sci. J. 88, 1963–1969. doi: 10.1111/asj.12862
Zhang, G., Fang, X., Feng, G., Li, Y., and Zhang, Y. (2020). Silage fermentation, bacterial community, and aerobic stability of total mixed ration containing wet corn gluten feed and corn stover prepared with different additives. Animals 10:1775. doi: 10.3390/ani10101775
Zhang, M., Wang, L., Wu, G., Wang, X., Lv, H., Chen, J., et al. (2021). Effects of Lactobacillus plantarum on the fermentation profile and microbiological composition of wheat fermented silage under the freezing and thawing low temperatures. Front. Microbiol. 12:671287. doi: 10.3389/fmicb.2021.671287
Zhao, C., Wang, L., Ma, G., Jiang, X., Yang, J., Lv, J., et al. (2021). Cellulase interacts with lactic acid bacteria to affect fermentation quality, microbial community, and ruminal degradability in mixed silage of soybean residue and corn stover. Animals 11:334. doi: 10.3390/ani11020334
Zhao, S., Yang, F., Wang, Y., Fan, X., Feng, C., and Wang, Y. (2021). Dynamics of fermentation parameters and bacterial community in high-moisture alfalfa silage with or without lactic acid bacteria. Microorganisms 9:1225. doi: 10.3390/microorganisms9061225
Zheng, M. L., Niu, D. Z., Jiang, D., Zuo, S. S., and Xu, C. C. (2017). Dynamics of microbial community during ensiling direct-cut alfalfa with and without LAB inoculant and sugar. J. Appl. Microbiol. 122, 1456–1470. doi: 10.1111/jam.13456
Keywords: alfalfa silage, bacterial community, fermentation quality, lactic acid bacterial additives, microbial counts, nutrition composition
Citation: Na N, Qili M, Wu N, Sun L, Xu H, Zhao Y, Wei X, Xue Y and Tao Y (2022) Bacterial Community and Fermentation Quality of Ensiling Alfalfa With Commercial Lactic Acid Bacterial Additives. Front. Microbiol. 13:836899. doi: 10.3389/fmicb.2022.836899
Received: 16 December 2021; Accepted: 22 February 2022;
Published: 22 April 2022.
Edited by:
Zheng Zhang, Shandong University, ChinaReviewed by:
Xianjun Yuan, Nanjing Agricultural University, ChinaKimon Andreas Karatzas, University of Reading, United Kingdom
Tugay Ayasan, Osmaniye Korkut Ata University, Turkey
Copyright © 2022 Na, Qili, Wu, Sun, Xu, Zhao, Wei, Xue and Tao. This is an open-access article distributed under the terms of the Creative Commons Attribution License (CC BY). The use, distribution or reproduction in other forums is permitted, provided the original author(s) and the copyright owner(s) are credited and that the original publication in this journal is cited, in accordance with accepted academic practice. No use, distribution or reproduction is permitted which does not comply with these terms.
*Correspondence: Yanlin Xue, eHVleWFubGluXzE5NzlAMTYzLmNvbQ==; Ya Tao, dGFveWEyMDAxQDEyNi5jb20=