- Research Center for Microbial Metabolites, Shaanxi Institute of Microbiology, Xi'an, China
Plant growth-promoting bacteria (PGPB) have been considered promising biological agents to increase crop yields for years. However, the successful application of PGPB for biocontrol of sharp eyespot in wheat has been limited, partly by the lack of knowledge of the ecological/environmental factors affecting the colonization, prevalence, and activity of beneficial bacteria on the crop. In this study, an endophytic bacterium XN08 with antagonistic activity against Rhizoctonia cerealis (wheat sharp eyespot pathogenic fungus), isolated from healthy wheat plants, was identified as Burkholderia ambifaria according to the sequence analysis of 16S rRNA. The antibiotic synthesis gene amplification and ultra-performance liquid chromatography-quadrupole time-of-flight mass spectrometry (UPLC-QTOF-MS) analyses were used to characterize the secondary metabolites. The results showed that the known powerful antifungal compound named pyrrolnitrin was produced by the strain XN08. In addition, B. ambifaria XN08 also showed the capacity for phosphate solubilization, indole-3-acetic acid (IAA), protease, and siderophore production in vitro. In the pot experiments, a derivate strain carrying the green fluorescent protein (GFP) gene was used to observe its colonization in wheat plants. The results showed that GFP-tagged B. ambifaria could colonize wheat tissues effectively. This significant colonization was accompanied by an enhancement of wheat plants' growth and an induction of immune resistance for wheat seedlings, which was revealed by the higher activities of polyphenol oxidase (PPO), peroxidase (POD), and phenylalanine ammonia-lyase (PAL). As far as we know, this is the first report describing the colonization traits of B. ambifaria in wheat plants. In addition, our results indicated that B. ambifaria XN08 might serve as a new effective biocontrol agent against wheat sharp eyespot disease caused by R. cerealis.
Introduction
The wheat sharp eyespot, caused predominately by the necrotrophic fungus Rhizoctonia cerealis, is one of the most destructive soil-borne fungal diseases in wheat (Triticum aestivum L.) and results in yield losses of 10%−40% in the regions of Asia, Oceania, Europe, North America, and Africa (Wang et al., 2018; Zhao et al., 2021). This fungal pathogen can survive in soils or the infected crop residues for a long time, and it reinfects the stems and sheaths of wheat plants in the favorable environmental conditions, blocks the transportation of nutrients, and eventually leads to host death (Su et al., 2020). Traditional agrochemicals, which were still widely used for the effective control of wheat sharp eyespot, had led to an increase in environmental pollution and induced pesticide resistance (Zhang et al., 2017). Therefore, the biological control of wheat sharp eyespot as a green and sustainable agricultural biotechnology has attracted lots of attention (Raymaekers et al., 2020; Xu et al., 2020).
Plant growth-promoting bacteria (PGPB) have been considered promising biological agents for years (Dimkić et al., 2022). They have shown multifunctional plant-promoting ways including the facilitation of nutrient uptake, nitrogen fixation for plant use, the production of plant hormones, direct antagonism against pathogens, and the induction of systemic resistance throughout the plant (Jing et al., 2019). Therefore, many researchers have focused on the exploration of new PGPB with varied beneficial effects in recent years. For example, Pantoea dispersa-AA7 and Enterobacter asburiae-BY4, which were isolated from sugarcane rhizosphere soils, showed the capacity for nitrogenase and ACC deaminase production (Jing et al., 2019). Saad et al. (2020) isolated 18 strains from the rhizosphere soils of red silk-cotton tree and Chinese banyan and found that Bacillus thuringiensis MN419208 exhibited the capacity for plant growth promotion by producing indole-3-acetic acid (IAA) and exopolysaccharides and exerting the capacity of nitrogen fixation, while Bacillus sonorensis MN419205, Bacillus wiedmannii MN419207, and Bacillus subtilis MN419218 showed the antagonistic properties against root rot in fava beans. In contrast, a rhizosphere isolated strain of Pseudomonas sp. 23S showed antagonistic activity against Clavibacter michiganensis subsp. michiganensis in vitro and reduced the severity of tomato bacterial canker by inducing systemic resistance (Takishita et al., 2018). However, it is inadequate to excavate the wheat association PGPB, especially in screening the biological control agents against sharp eyespot caused by R. cerealis.
On the contrary, although many PGPB have shown excellent antagonistic characteristics under laboratory and greenhouse conditions, the successful application of PGPB under field conditions has been limited by its poor colonization capacity (Rilling et al., 2019). In fact, the effective root colonization of PGPB is considered to be a critical factor in achieving successful plant–microbe interaction (Bo et al., 2022). Compared with the plant rhizobacteria, bacterial endophytes have more opportunities to be in contact with the plant cells, so they could readily exert a direct beneficial effect (Morales-Cedeno et al., 2021).
In our previous study, an endophytic bacterium XN08, which showed great antagonistic activities against varied phytopathogenic fungi including R. cerealis, was isolated from healthy wheat plants. The purpose of this study was to evaluate its biocontrol potential against sharp eyespot in wheat. The strain XN08 was identified via 16S rRNA analysis, and a known antifungal compound produced by the strain was confirmed by gene amplification and ultra-performance liquid chromatography-quadrupole time-of-flight mass spectrometry (UPLC-QTOF-MS) analyses. To observe its colonization in wheat plants, a derivate strain carrying the green fluorescent protein (GFP) gene was constructed. The immune resistance of wheat seedlings was also monitored in this study.
Materials and methods
Bacterial strains and growth conditions
The strain XN08 used in this study was obtained from healthy wheat plants in our laboratory. The strain was cultivated in a nutrient broth medium (NB) and maintained at −80°C in a 20% glycerol solution. GFP-tagged Burkholderia ambifaria was cultivated in an NB medium containing 100 μg/ml tetracycline (Tc) for the maintenance of plasmids. Rhizoctonia cerealis, which was kindly provided by the Center of Biological Pesticide Research, Northwest Agricultural and Forestry University, was maintained on potato dextrose agar (PDA) slants. Candida albicans, which was derived from the Center of Microbiological Detection, Shaanxi Institute of Microbiology, was used as indicator fungi to detect the antifungal activity. The pyrrolnitrin was purchased from ChengDu TongChuangYuan Pharmaceutical Co. Ltd. (Chengdu, China). The Xiaoyan 22 (T. aestivum L.) seeds were directly purchased from the market.
Phylogenetic analysis
The bacterial genomic DNA was isolated and purified using the TaKaRa MiniBEST Bacteria Genomic DNA Extraction Kit (Dalian, China). Genomic DNA was then used as the template for PCR amplification of 16S rRNA gene fragments using the bacterial universal primers (27F-5′-AGAGTTGATCCTGGCTCAG-3′ and 1492R-5′-GGTTACCTTGTTACGACTT-3′). The final amplified reaction volume was 50 μl, containing 5.0 μl of 10 × Taq buffers, 4.0 μl of 200 mmol/L dNTPs, 2.0 μl of each primer at 10 μM, 0.5 μl of Ex Taq enzyme (TaKaRa, Dalian), 5.0 μl of genomic DNA, and 31.5 μl of sterilized distilled water. PCR amplification was performed using the Professional Standard 96 Gradient (Biometra, Jena, Germany) with the following cycling parameters: initial denaturation of DNA for 5 min at 95°C, then 30 cycles of denaturation of DNA for 1 min at 94°C, annealing for 1 min at 53°C, extension for 1.5 min at 72°C, and final incubation for 5 min at 72°C (Vasiee et al., 2018). The PCR products were subsequently purified and sequenced using BGI Biotechnology (Shenzhen, China). DNA sequence alignment was performed using BLAST (https://blast.ncbi.nlm.nih.gov/Blast.cgi). Finally, phylogenetic trees were constructed using the neighbor-joining (NJ) method implemented in MEGA 5.05 (Arizona State University, Tempe, United States).
Detection of genes associated with antibiotic biosynthesis using the PCR method
The Burkholderia spp. have been reported to produce antimicrobial compounds such as siderophore (required Cep gene), pyrrolnitrin (required Prn gene), and phenazine acid (required Pca gene). We designed three sets of low-degeneracy primers for PCR amplification of these genes. These primers are shown in Supplementary material 1. According to the manufacturer's instructions, the amplification was performed in 50 μl reactions with Taq polymerase (TaKaRa Biotechnology, Dalian, China). The PCR products were detected using gel electrophoresis detection. Finally, the PCR products obtained in this study were sequenced by BGI Biotechnology (Shenzhen, China), and the phylogenetic tree was constructed using the neighbor-joining (NJ) method.
Evaluation of antifungal activity in vitro and ex vivo
Antifungal activity of the Burkholderia sp. XN08 was evaluated using both the dual plate confrontation assay and the agar diffusion method. The inhibition ratio was calculated as follows (Cui et al., 2019):
The strain XN08, which was pre-cultured on a nutrient agar medium (NA) plate for 24 h, was inoculated into a 500-ml conical flask containing 200 ml of sterile LB broth and then cultured at 230 rpm for 48 h at 37°C. After cultivation, the cells were removed by centrifugation at 8,000 rpm for 10 min at 4°C. The supernatant was added into preheated PDA medium at 55°C. Rhizoctonia cerealis was respectively inoculated on the pure PDA medium plate and PDA medium supplemented with the fermentation broth supernatant of the strain XN08.
Plant leaf tissue was used to determine the antifungal activity of fermentation broth supernatant ex vivo as previously described (Fu et al., 2019) with minor modifications. In brief, the top leaves of 14-day-old wheat seedlings with four- to five-leaf stages were used for evaluating the biocontrol efficacy of the fermentation broth supernatant of the strain XN08 against R. cerealis. The leaves were wounded at the equator (0.5 mm wide) and inoculated with 5 μl of conidial suspension (2 × 105 spores/ml) of R. cerealis. Then, the fermentation broth supernatant of the strain XN08 was sprayed onto the leaf surface. To keep the plant growing, the petioles were wrapped in cotton that contained water to provide nutrients. The leaves were kept in greenhouse system at 23 ± 2°C with a relative humidity (RH) of 95% for 6 days. Daily observations were carried out.
Extraction and identification of antifungal compounds by UPLC-QTOF-MS
The n-butanol extracts from the fermentation broth supernatant of XN08 were dissolved with methanol at a concentration of 1 mg/ml, and 50 μl of the solution was added into a 6-mm well in Sabouraud agar medium plates, which were inoculated with C. albicans and cultured overnight at 37°C. Then, the plates were incubated at 28°C for 72 h, and the inhibition zones around the wells were observed to determine the antimicrobial activity. The redissolved sample was further purified using reverse-phase high-performance liquid chromatography (Waters 2695, PDA detector 2998) with a C18 column (YMC-Pack Pro C18, 250 × 4.6 mm S-5 μm, 12 nm; YMC CO., LTD, Japan) and eluted with a methanol–water mixture (methanol:water = 8:2) at a flow rate of 1.0 ml/min. The OD at 254 nm was monitored. The MS was operated in negative ion mode and was set to total ion chromatogram mode with the following parameter settings: capillary voltage, 1.0 kV; low collision energy, 6V; source temperature, 100°C; desolvation temperature, 500°C; and desolvation gas flow, 800 L/h. Data acquisition and processing were conducted using Masslynx version 4.1 (Waters, Manchester, United Kingdom).
Detection of plant growth-promoting traits in vitro
IAA detection
The strain XN08 was propagated overnight in 100 ml of LB medium and then supplemented with 1 ml of L-tryptophan solution with a concentration of 50 μg/ml. After incubation for 42 h, 1 ml aliquot of the supernatant was mixed vigorously with 4 ml of Salkowski's reagent (150 ml of concentrated H2SO4, 250 ml of distilled H2O, and 7.5 ml of 0.5 M FeCl3∙6H2O) and allowed to stand at room temperature for 20 min, and then the color changes were observed (Patten and Glick, 2002).
Phosphate solubilization detection
A single colony of strain XN08 was spot inoculated onto Pikovskaya's agar plate at 28°C and incubated at 28°C for 48 h. The formation of a halo-zone around the colony was observed (Patten and Glick, 2002).
Siderophore detection
A single colony of strain XN08 was spot inoculated on CAS medium [medium component (1L): chrome azurol S (CAS), 60.5 mg; hexadecyltrimetyl ammonium bromide (HDTMA), 72.9 mg; piperazine-1, 4-bis (2-ethanesulfonic acid; PIPES), 30.24 g; and 1 mM FeCl3∙6H2O in 10 mM HCl 10 ml agarose (0.9%, w/v)], and then color changes around the colonies were observed visually (Shahid et al., 2012).
Proteinase detection
A single colony of the strain XN08 was spot inoculated on a modified tryptic soy broth medium and then the zones of proteolysis around the colonies were observed visually (Shahid et al., 2012).
Pot experiments
Wheat seeds were surface-sterilized in 2.5% sodium hypochlorite for 5 min and in 75% ethanol for 2 min and then soaked in sterile-distilled water for 24 h. The sterilized wheat seeds were put into a sterile 10-ml glass bottle and then cultured for 7 days in the artificial climate box with a temperature of 28°C and 90% RH. The seedlings with consistent growth were selected for the pot experiments. All experiments have been conducted in a 10-ml glass bottle, and each experiment group consisted of four bottles, each of which contains three seedlings.
• Group 1: The seedlings were poured with a 2-ml suspension of GFP-tagged B. ambifaria (107 CFU/ml).
• Group 2: The control group consisted of seedlings soaked in 2 ml of sterile water.
• Group 3: The seedlings soaked in a 2-ml of sterile water were scratched and inoculated with 500 μl of the suspension of R. cerealis containing 1 × 108 CFU/ml spores.
• Group 4: The seedlings were poured with 2 ml suspension of GFP-tagged B. ambifaria (107 CFU/mL) and inoculated with 500 μl of suspension of R. cerealis containing 1 × 108 CFU/ml spores.
All of the treated seedlings were further cultured in an artificial climate box (RH 90% and temperature 28°C) and then tested for plant growth-promoting and biocontrol properties. Three seedlings were sampled for each treatment at different growth stages. The samples were washed and wiped dry, and then their fresh weight and shoot height were measured.
Colonization of XN08 in wheat tissues
The seedlings of group 1 were scanned and imaged by CLSM (Laser Scanning Confocal Microscopy; DM6000, Leica Microsystems). The STED beam was generated by a 592-nm depletion beam. All images were detected using hybrid (HyD) detectors controlled by LAS-AX imaging software. All STED images were deconvolved using Huygens software (Scientific Volume Imaging) and analyzed offline using LAS AF Lite (Leica).
The activities of defense enzymes in wheat
The activity levels of polyphenol oxidase (PPO), peroxidase (POD), and phenylalanine ammonia-lyase (PAL) were determined according to the instructions provided by the manufacturer with the respective enzyme activity assay kits (Nanjing Jiancheng Bioengineering Institute, China). The article numbers of the kits are A136-1-1 (PPO), A084-3-1 (POD), and A137-1-1 (PAL).
Results
Identification of the strain XN08
As shown in Figures 1A,B, the strain XN08 exhibited off-white colony morphology and secreted viscoelastic substances on the NA plate. The cells appeared as short rod shapes under the light microscope (Figure 1C). A 1,438 bp region of the 16S rDNA gene was amplified from the genomic DNA of the strain XN08, and the sequence analysis indicated that the strain XN08 shared 99.85% identity with B. ambifaria AMMD (Genebank Accessions: CP00040) in the NCBI nr database. The phylogenetic tree was constructed (Figure 1D) using the neighbor-joining method. The result also showed that the strain XN08 had a close relationship with B. ambifaria AMMD. Therefore, the strain XN08 was identified as B. ambifaria.
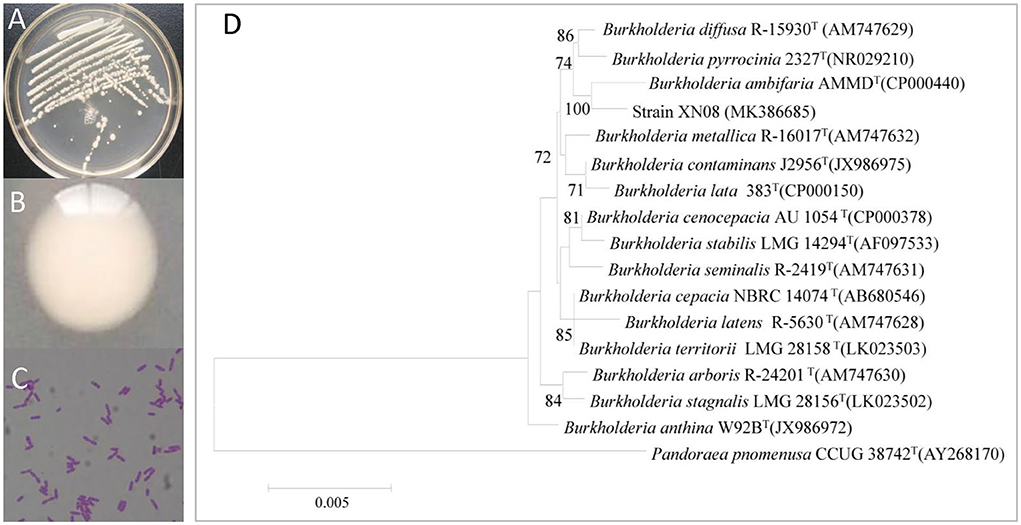
Figure 1. Morphological and molecular identification of the endophytic bacterium XN08. The colonies (A), colony characters (B) of the strain XN08 on LB medium and microscopic characters (C) under a light microscope (×400), phylogenetic tree of the strain XN08 based on 16S rRNA sequence (D).
Biocontrol potential of the strain XN08 against R. cerealis
Compared with the control group (Figure 2A), B. ambifaria XN08 showed dramatically a high antagonistic activity against R. cerealis under the co-cultural condition (Figure 2B). In addition, no obvious R. cerealis growth was observed on the PDA medium added with the fermentation supernatants of the strain XN08 (Figure 2C). Moreover, the plant leaves sprayed with the supernatant of strain XN08 maintained a healthy green color, and no obvious disease symptoms were observed after inoculation with R. cerealis (Figure 2D). Usually, the leaves would become yellow after pathogen infection. The above results demonstrated that the strain XN08 may produce extracellular antifungal compounds.
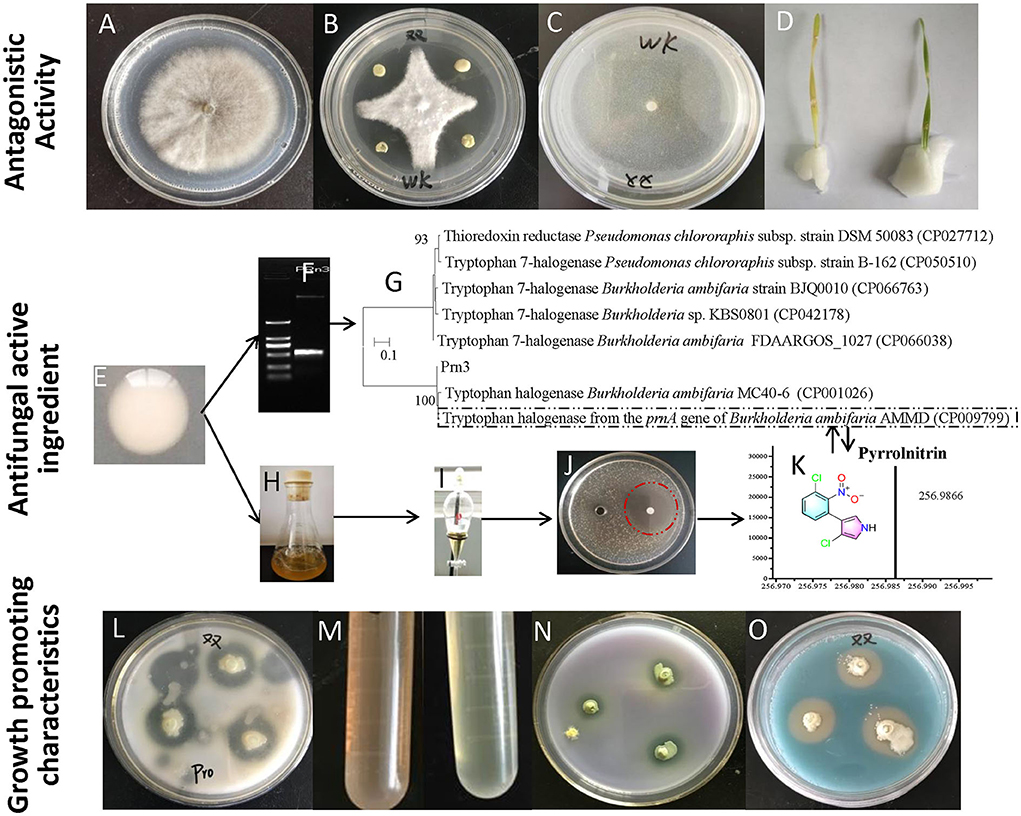
Figure 2. Antagonistic activities and plant growth-promoting characteristics of the strain XN08. R. cerealis grown on PDA medium (A), in vitro antagonism of B. ambifaria XN08 against R. cerealis (B), R. cerealis grown on PDA medium supplemented with the fermentation broth supernatant of B. ambifaria XN08 (C), inhibition of the fermentation broth supernatant of the strain XN08 against R. cerealis on detached leaves of wheat plants (D), amplification and sequence comparison of the antibiotic synthesis genes (E–G), fermentation of the strain XN08 (H), extraction of antifungal compounds (I), detection of antifungal activities (J), identification of antifungal compounds by UPLC-QTOF-MS method (K), and plant growth-promoting traits of B. ambifaria XN08 in vitro [(L), proteinase production; (M), IAA production; (N), the capacity of phosphate solubilization; (O), siderophore production].
Identification of potential antifungal compounds
To predict the potential antifungal substances produced by B. ambifaria XN08, PCR was used to detect the biosynthetic genes of antifungal compounds. Three genes, namely, Cep R, Prn, and Pca, were amplified with predesigned three primers pairs, respectively. A key gene fragment sequence (Prn 3, 503 bp) involved in pyrrolnitrin synthesis was successfully amplified (Figures 2E,F). A phylogenetic tree was constructed by sequence alignment using the neighbor-joining method. The sequence had a close relationship with tryptophan halogenase from the prn A gene of B. ambifaria AMMD (CP009799; Figure 2G). The result indicated that B. ambifaria XN08 had the potential to synthesize pyrrolnitrin. An antifungal activity assay was performed to identify the fraction containing the antifungal compound. The n-butanol-extracted fraction of B. ambifaria XN08 fermentation broth (Figures 2H,I) exhibited obvious antifungal activity against C. albicans (Figure 2J). Subsequently, pyrrolnitrin was detected in the n-butanol-extracted fraction using the UPLC-QTOF-MS method (Figure 2K).
In vitro plant growth-promoting traits of the strain XN08
As shown in Figures 2L–O, the strain XN08 exhibited a series of potential plant growth-promoting traits including protease, IAA, and siderophore production. Also, the strain could solubilize phosphate.
Evaluation of plant colonization of GFP-tagged B. ambifaria
A derivative strain carrying the GFP gene was successfully constructed, and CLSM was used to observe the cells with green fluorescence as shown in Figure 3A. The GFP-tagged B. ambifaria showed a slightly weaker antagonistic activity (inhibition ratio of 70.14%) against R. cerealis compared with the wild strain XN08 (inhibition ratio of 75.45%; Figures 3B,C). In the pot experiment (Figure 3D), a small number of GFP-tagged B. ambifaria cells were found to colonize the root of wheat when the strain was inoculated into the rhizosphere soil of plants for 2 days (Figures 3E,F). At 7 days after inoculation, large numbers of bacterial cells were observed in the root tips (Figures 3G,H).
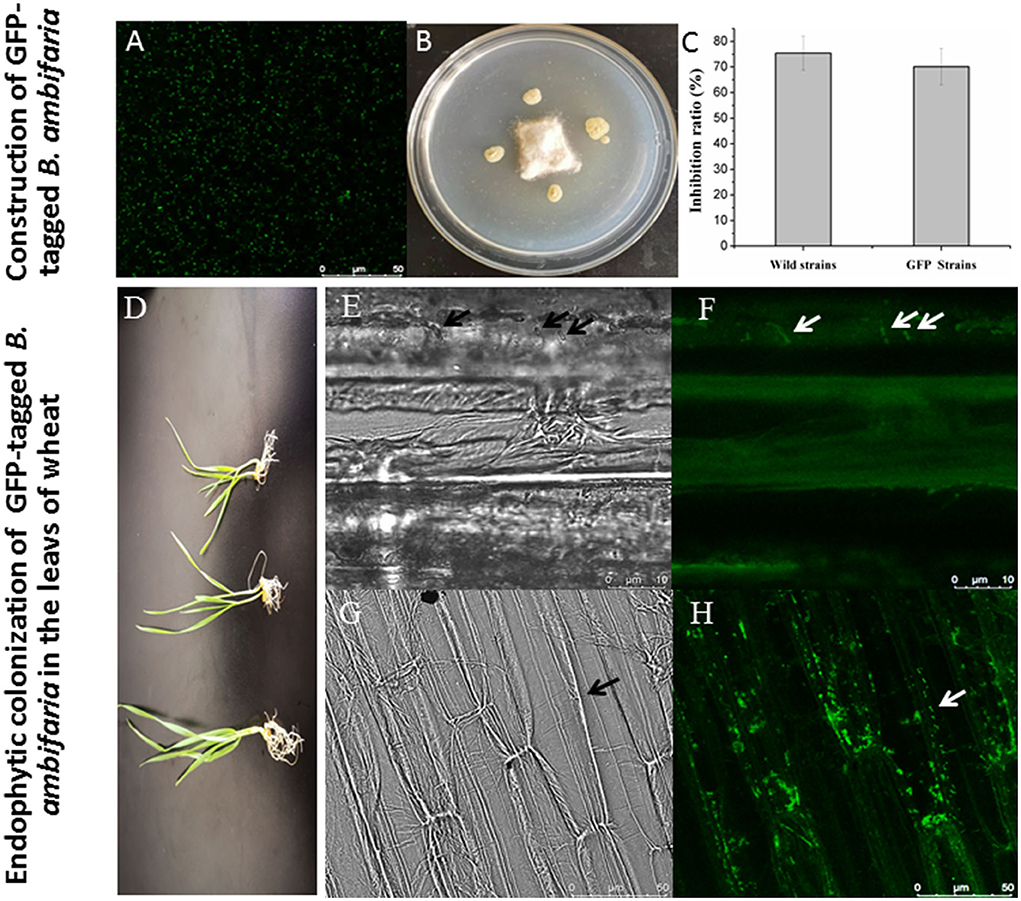
Figure 3. Colonization of GFP-tagged B. ambifaria in the seedlings of wheat. The observation of GFP-tagged B. ambifaria by CLSM (A), antagonistic activities against R. cerealis (B,C), the wheat seedlings inoculated GFP-tagged B. ambifaria at different growing stages (D), colonization of GFP-tagged B. ambifaria XN08 at 2 days after inoculation (photographs under dark (E) and bright (F) fields) and 7 days after inoculation (photographs under dark (G) and bright (H) fields).
Growth-promoting effects of B. ambifaria XN08 on wheat in the pot experiments
After 7 days of inoculation, the biological characteristics of the plant changed significantly as shown in Figure 4A. First, shoot height and fresh weight of the seedlings inoculated with both R. cerealis and GFP-tagged B. ambifaria reached 15.18 ± 2.54 cm and 0.28 ± 0.07 g for each seedling, which were higher than those of the seedlings inoculated with equal volumes of water (14.35 ± 2.78 cm, 0.26 ± 0.06 g) and the seedlings inoculated with R. cerealis (6.98 ± 1.34 cm and 0.12 ± 0.03 g) (Figures 4B,C). No significant difference was observed between the wheat seedlings with or without R. cerealis when inoculated with GFP-tagged B. ambifaria. In addition, the seedlings inoculated with R. cerealis had obvious sharp eyespot symptoms, while those plants inoculated with both R. cerealis and GFP-tagged B. ambifaria had no obvious symptoms.
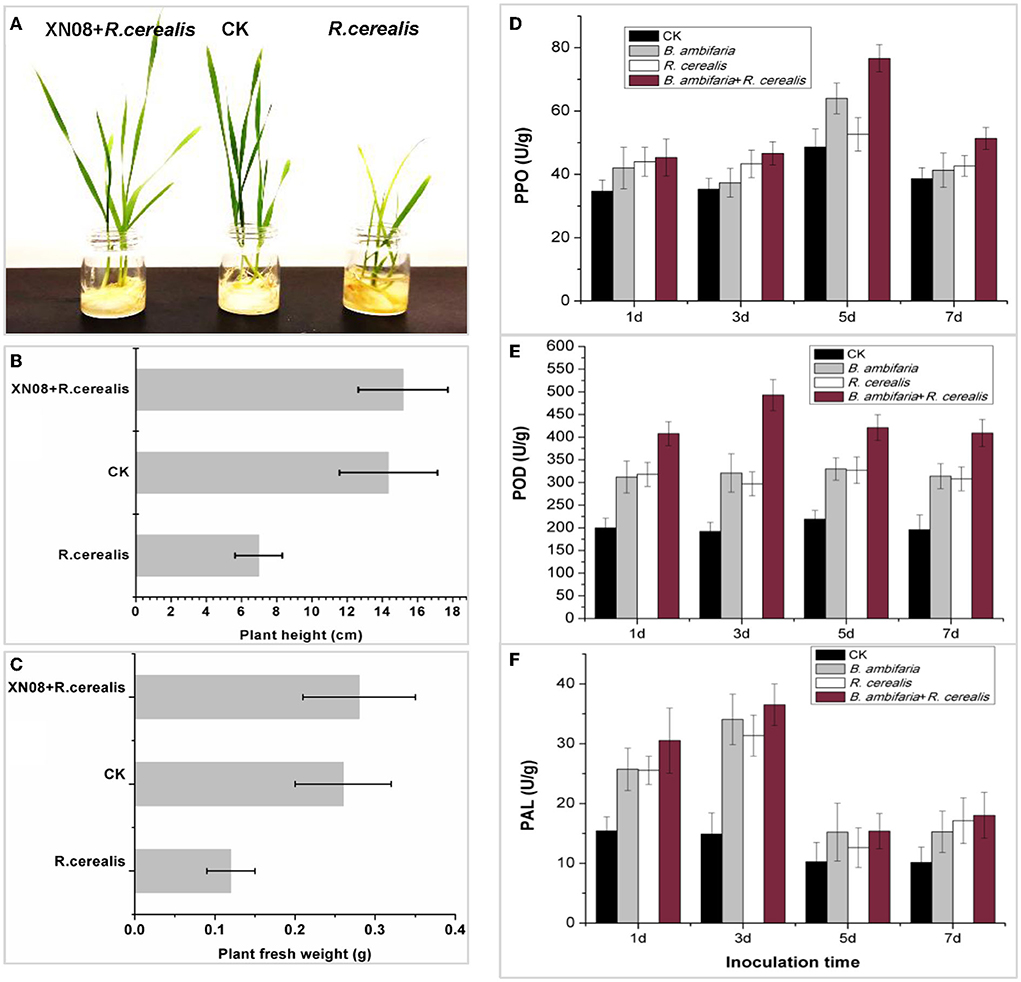
Figure 4. Antagonistic activities and plant growth-promoting characteristics of the strain XN08 in the pot experiments. Morphological changes in wheat seedlings (A), plant heights (B), plant fresh weights (C), and the activities of defense enzymes, including PPO (D), POD (E), and PAL (F) under different treatments.
The PPO, POD, and PAL enzyme activities of wheat seedlings at different growth stages for different treatment groups were tested. As shown in Figures 4D–F, the activities of PPO, POD, and PAL in the roots of wheat inoculated with both GFP-tagged B. ambifaria and R. cerealis were higher than those of the other groups and showed peaks at 5, 3, and 3 days after inoculation, respectively.
Discussion
Wheat hosts a high diversity of endophytic bacteria, including different genera such as Achromobacter, Acinetobacter, Arthrobacter, Bacillus, Burkholderia, Chitinophaga, Enterobacter, Erwinia, Flavobacterium, Klebsiella, Leifsonia, Microbispora, Micrococcus, Micromonospora, Mycobacterium, Paenibacillus, Pantoea, Pseudomonas, Roseomonas, Staphylococcus, Streptomyces, and Xanthomonas (Rana et al., 2020). Several studies have exploited wheat-associated PGPB. It was reported that 13 endophytic bacteria isolated from wheat showed multifarious plant beneficial traits with the capacity of P-solubilization, nitrogenase, and IAA production (Rana et al., 2020). Larran et al. (2016) isolated the endophytes from wheat cultivars and found that the endophytes of Penicillium sp., Bacillus sp., and Paecilomyces lilacinus significantly suppressed the growth of pathogens in vitro and have the potential to be developed as new biological agents against the tan spot of wheat. In this study, the endophytic bacterium B. ambifaria XN08, which was isolated from healthy wheat plants, showed potential as a novel biological control agent against the wheat sharp eyespot. After all, only Bacillus spp. were exploited as biocontrol agents against R. cerealis in the previous reports (Ji et al., 2019; Yi et al., 2022).
Burkholderia is a genus of gram-negative bacteria with a wide environmental and geographic distribution (Estrada-De los Santos et al., 2001). Over the last years, there was an increasing interest in the genus Burkholderia due to its great potential value in plant growth promotion, biocontrol of plant pathogens, and phytoremediation (Bach et al., 2021). It was reported that bacterial endophytes belonging to Bacillus and Burkholderia genera were the most effective isolates in controlling bacterial and fungal pathogens in vitro (Morales-Cedeno et al., 2021). In fact, Burkholderia genus has rich antibiotic synthesis genes (Kim et al., 2021), and it has been reported to produce a large number of antifungal substances such as pyrrolnitrin, siderophores, and phenazines (Mullins et al., 2019), all of which play important roles in controlling fungal diseases in plants. In this study, a known powerful antifungal compound named pyrrolnitrin, production by XN08, was found, proving that the strain is valuable in controlling diseases including the wheat sharp eyespot. In addition, it is quite interesting that pyrrolnitrin production is quorum-sensing regulated, which indicates that efficient environmental colonization is crucial for the effective control of the wheat sharp eyespot disease (Chapalain et al., 2013).
Plant growth promotion and environmental colonization characteristics also determine the great potential of the Burkholderia genus as biocontrol agents (Paungfoo-Lonhienne et al., 2016). To observe the bacterial colonization directly and conveniently, a derivate strain carrying GFP gene was constructed in this study. The GFP-tagged B. ambifaria showed a similar inhibition ratio against R. cerealis as the wild-type strain, which indicated that the derivate strain could be used to efficiently observe its colonization in wheat plant tissue. Fluorescent labeling technology was the most intuitive way of representing the colonization of strain and was widely used in studying the plants and microorganisms interaction (Rilling et al., 2019; Sa et al., 2021). A number of potential biocontrol strains, including Pseudomonas fluorescens, Paenibacillus glycanilyticus, Burkholderia tropica, and Bacillus velezensis, labeled with a green fluorescent protein have been used to study their colonization capacity in plant tissue (Bernabeu et al., 2015; Kang et al., 2018; Li et al., 2019; Elsayed et al., 2020). However, as far as we know, this is the first time that B. ambifaria was labeled with green fluorescent protein to study the colonization characterized in this study.
It was reported that PGPB induced plants defense-related genes expression such as phenylalanine ammonia-lyase (PAL), catalase (CAT), polyphenol oxidase (PPO), peroxidase (POD), and superoxide dismutase (SOD), which might assist the plant to protect from or reduce the impact of pathogen attacks (Jiang et al., 2019; Kamou et al., 2019; Wu et al., 2019; Zhu et al., 2019; Ashajyothi et al., 2020; Singh et al., 2021). Our results showed that the significant colonization of XN08, visually observed with CLSM, was accompanied by the higher activities of PPO, POD, and PAL, which indicated that B. ambifaria XN08 was involved in the immune-induced resistance of wheat seedlings. In addition, the results also showed that the endophytic bacterial strain XN08 could significantly improve the growth of wheat inoculated with R. cerealis. The fresh weight and shoot height of the seedlings inoculated with both R. cerealis and GFP-tagged B. ambifaria were similar to those of the control group. Meanwhile, no obvious sharp eyespot symptom was observed for the group seedlings inoculated with both R. cerealis and GFP-tagged B. ambifaria. It is obvious that XN08 may provide an adequate protection against wheat disease.
On a whole, the results obtained in this study revealed that XN08 had great potential for biological control against the wheat sharp eyespot. However, it is worth noting that the successful application of XN08 for biocontrol of sharp eyespot in wheat needs knowledge of the ecological/environmental factors affecting the colonization of crops. Therefore, further observation using GFP-tagged B. ambifaria under different environmental conditions should be performed.
Conclusion
Taken together, it was concluded that B. ambifaria XN08 was able to efficiently inhibit the growth of R. cerealis by producing antifungal compounds and showed the capacity to enhance plant growth by synthesizing a series of plant growth regulators. In addition, this strain exhibited significant colonization in wheat plants, which was accompanied by an enhancement of wheat plants' growth and an induction of immune resistance for wheat seedlings. These data indicated that the strain XN08 might be used as a new biocontrol agent against wheat sharp eyespot.
Data availability statement
The original contributions presented in the study are included in the article/Supplementary materials, further inquiries can be directed to the corresponding author.
Author contributions
CA performed the experiments, wrote, and edited the manuscript. WX designed and supervised the project. All experiments were also performed by SM, CL, and HD. All authors contributed to the article and approved the submitted version.
Funding
This study was simultaneously supported by the National Natural Science Foundation of China (21576160) and the Scientific and Technologic Research Program of Shaanxi Academy of Sciences, China (No. 2018K-09).
Acknowledgments
The authors thank the National Natural Science Foundation of China for financial support. They also thank Shaanxi Province Academy of Sciences for financial support, authors acknowledge technical support of Dr. Chenlin for the construction of a derivate Burkholderia ambifaria strain carrying GFP gene used in this study.
Conflict of interest
The authors declare that the research was conducted in the absence of any commercial or financial relationships that could be construed as a potential conflict of interest.
Publisher's note
All claims expressed in this article are solely those of the authors and do not necessarily represent those of their affiliated organizations, or those of the publisher, the editors and the reviewers. Any product that may be evaluated in this article, or claim that may be made by its manufacturer, is not guaranteed or endorsed by the publisher.
Supplementary material
The Supplementary Material for this article can be found online at: https://www.frontiersin.org/articles/10.3389/fmicb.2022.906724/full#supplementary-material
References
Ashajyothi, M., Kumar, A., Sheoran, N., Ganesan, P., Gogoi, R., Subbaiyan, G. K., et al. (2020). Black Pepper (Piper Nigrum L.) associated endophytic Pseudomonas putida BP25 alters root phenotype and induces defense in rice (Oryza Sativa L.) against blast disease incited by Magnaporthe oryzae. Biol. Control. 143, 104181. doi: 10.1016/j.biocontrol.2019.104181
Bach, E., Passaglia, L. M. P., Jiao, J. J., and Gross, H. (2021). Burkholderia in the genomic era: from taxonomy to the discovery of new antimicrobial secondary metabolites. Crit. Rev. Microbiol. 48, 121–160. doi: 10.1080/1040841X.2021.1946009
Bernabeu, P. R., Pistorio, M., Torres-Tejerizo, G., Estrada-De los Santos, P., Galar, M. L., Boiardi, J. L., et al. (2015). Colonization and plant growth-promotion of tomato by Burkholderia tropica. Sci. Hortic-amsterdam. 191, 113–120. doi: 10.1016/j.scienta.2015.05.014
Bo, T. T., Kong, C. X., Zou, S. X., Mo, M. H., and Liu, Y. J. (2022). Bacillus nematocida B16 enhanced the rhizosphere colonization of Pochonia chlamydosporia ZK7 and controlled the efficacy of the root-knot nematode Meloidogyne incognita. Microorganisms. 10, 218. doi: 10.3390/microorganisms10020218
Chapalain, A., Vial, L., Laprade, N., atacha., Dekimpe, V., Perreault, J., and Déziel, E. (2013). Identification of quorum sensing-controlled genes in Burkholderia ambifaria. MicrobiologyOpen 2, 226–242. doi: 10.1002/mbo3.67
Cui, W. Y., He, P. J., Munir, S., He, P. B., Li, X. Y., Li, Y. M., et al. (2019). Efficacy of plant growth promoting bacteria Bacillus amyloliquefaciens B9601-Y2 for biocontrol of southern corn leaf blight. Biol. Control. 139, 104080. doi: 10.1016/j.biocontrol.2019.104080
Dimkić, I., Janakiev, T., Petrović, M., Degrassi, G., and Fira, D. (2022). Plant-associated Bacillus and Pseudomonas antimicrobial activities in plant disease suppression via biological control mechanisms-a review. Physiol. Mol. Plant. P. 117, 101754. doi: 10.1016/j.pmpp.2021.101754
Elsayed, T. R, Jacquiod, S., Nour, E. H., Sørensen, S. J., and Smalla, K. (2020). Biocontrol of bacterial wilt disease through complex interaction between tomato plant, antagonists, the indigenous rhizosphere microbiota, and Ralstonia solanacearum. Front. Microbiol. 10, 2835. doi: 10.3389/fmicb.2019.02835
Estrada-De los Santos, P., Bustillos-Cristales, R., and Caballero-Mellado, J. (2001). Burkholderia, a genus rich in plant-associated nitrogen fixers with wide environmental and geographic distribution. Appl. Environ. Microbiol. 67, 2790–2798. doi: 10.1128/AEM.67.6.2790-2798.2001
Fu, M. R., Zhang, X. M., Jin, T., Li, B. Q., Zhang, Z. Q., Tian, S., et al. (2019). Inhibitory of grey mold on green pepper and winter jujube by chlorine dioxide (ClO2) fumigation and its mechanisms. LWT 100, 335–340. doi: 10.1016/j.lwt.2018.10.092
Ji, P., Li, W. G., Zheng, Y. X., Wang, Z. H., Huo, Q. X., Hua, C. Y., and Han, C. (2019). Isolation and identification of four novel biocontrol Bacillus strains against wheat sharp eyespot and their growth-promoting effect on wheat seedling. Int. J. Agric. Biol. 21, 282–288. doi: 10.17957/IJAB/15.0892
Jiang, C. H., Yao, X. F., Mi, D. D., Li, Z. J., Yang, B. Y., Zheng, Y., et al. (2019). Comparative transcriptome analysis reveals the biocontrol mechanism of Bacillus velezensis F21 against Fusarium Wilt on Watermelon. Front. Microbiol. 10, 652. doi: 10.3389/fmicb.2019.00652
Jing, L. M., Jeong, J. C., Lee, J. S, Park, J. M., Yang, J. W., Lee, M. H., et al. (2019). Potential of Pantoea dispersa as an effective biocontrol agent for black rot in sweet potato. Sci. Rep. 9, 16354. doi: 10.1038/s41598-019-52804-3
Kamou, N. N., Cazorla, F., Kandylas, G., and Lagopodi, A. L. (2019). Induction of defense-related genes in tomato plants after treatments with the biocontrol agents Pseudomonas chlororaphis Toza7 and Clonostachys rosea Ik726. Arch. Microbiol. 202, 257–267. doi: 10.1007/s00203-019-01739-4
Kang, X. X., Zhang, W. L., Cai, X. C., Zhu, T., Xue, Y. R., and Liu, C. H. (2018). Bacillus velezensis CC09: a potential 'vaccine' for controlling wheat diseases. Mol. Plant. Microbe. Interact. 31, 623–632. doi: 10.1094/MPMI-09-17-0227-R
Kim, S., Jo, S., Kim, M. S., and Shin, D. H. (2021). A study of inhibitors of D-glycero-β-d-manno-heptose-1-phosphate adenylyltransferase from Burkholderia pseudomallei as a potential antibiotic target. J. Enzyme. Inhib. Med. Chem. 36, 776–784. doi: 10.1080/14756366.2021.1900166
Larran, S., Simón, M. R., Moreno, M. V., Santamarina Siurana, M. P., and Perell,ó, A. (2016). Endophytes from wheat as biocontrol agents against tan spot disease. Bio. Control. 92, 17–23. doi: 10.1016/j.biocontrol.2015.09.002
Li, L. M., Zhang, Z., Pan, S. Y., Li, L., and Li, X. Y. (2019). Characterization and metabolism effect of seed endophytic bacteria associated with peanut grown in South China. Front. Microbiol. 10, 2659. doi: 10.3389/fmicb.2019.02659
Morales-Cedeno, L. R., Orozco-Mosqueda, M. D., Loeza-Lara, P. D., Parra-Cota, F. I., de los Santos-Villalobos, S., and Santoyo, G. (2021). Plant growth-promoting bacterial endophytes as biocontrol agents of pre- and post-harvest diseases: fundamentals, methods of application and future perspectives. Microbiol. Res. 242, 126612. doi: 10.1016/j.micres.2020.126612
Mullins, A. J., Murray, J. A. H., Bull, M. J., Jenner, M., Jones, C., Webster, G., et al. (2019). Genome mining identifies cepacin as a plant-protective metabolite of the biopesticidal bacterium Burkholderia ambifaria. Nat. Microbiol. 4, 996–1005. doi: 10.1038/s41564-019-0383-z
Patten, C. L., and Glick, B. R. (2002). Role of Pseudomonas putida indoleacetic acid in development of the host plant root system. Appl. Environ. Microb. 68, 3795–3801. doi: 10.1128/AEM.68.8.3795-3801.2002
Paungfoo-Lonhienne, C., Lonhienne, T. G. A., Yeoh, Y. K., Donose, B. C., Webb, R. I., Parsons, J., et al. (2016). Crosstalk between sugarcane and a plant growth promoting Burkholderia species. Sci. Rep. 6, 37389. doi: 10.1038/srep37389
Rana, K. L., Kour, D., Kaur, T., Sheikh, T., Yadav, A. M., Kumar, V., et al. (2020). Endophytic microbes from diverse wheat genotypes and their potential biotechnological applications in plant growth promotion and nutrient uptake. Proc. Natl. Acad. Sci. India 90, 969–979. doi: 10.1007/s40011-020-01168-0
Raymaekers, K., Ponet, L., Holtappels, D., Berckmans, B., and Cammue, B. P. A. (2020). Screening for novel biocontrol agents applicable in plant disease management – a review. Biol. Control. 144, 104240. doi: 10.1016/j.biocontrol.2020.104240
Rilling, J. I., Acuña, J. J., Nannipieri, P., Cassan, F., Maruyama, F., and Jorquera, M. A. (2019). Current opinion and perspectives on the methods for tracking and monitoring plant growth–promoting bacteria. Soil. Biol. Biochem. 130, 205–219. doi: 10.1016/j.soilbio.2018.12.012
Sa, R. B., Zhang, J. L., Sun, J. Z., and Gao, Y. X. (2021). Colonization characteristics of poplar fungal disease biocontrol bacteria n6-34 and the inhibitory effect on pathogenic fungi by real-time fluorescence quantitative PCR detection. Curr. Microbiol. 78, 2916–2925. doi: 10.1007/s00284-021-02529-2
Saad, M. M. G., Kandil, M., and Mohammed, Y. M. M. (2020). Isolation and identification of plant growth-promoting bacteria highly effective in suppressing root rot in fava beans. Curr. Microbiol. 77, 2155–2165. doi: 10.1007/s00284-020-02015-1
Shahid, M., Hameed, S., Imran, A., Ali, S., and Elsas, J. D. V. (2012). Root colonization and growth promotion of sunflower (Helianthus Annuus L.) by phosphate solubilizing Enterobacter sp. Fs-11. World. J. Microb. Biot. 28, 2749-2758. doi: 10.1007/s11274-012-1086-2
Singh, P., Singh, R. K., Li, H. B., Guo, D. J., Sharma, A., Lakshmanan, P., et al. (2021). Diazotrophic bacteria Pantoea dispersa and Enterobacter asburiae promote sugarcane growth by inducing nitrogen uptake and defense-related gene expression. Front. Microbiol. 11, 600417. doi: 10.3389/fmicb.2020.600417
Su, Q., Wang, K., and Zhang, Z. Y. (2020). Ecotopic expression of the antimicrobial peptide dmamp1w improves resistance of transgenic wheat to two diseases: sharp eyespot and common root rot. Int. J. Mol. Sci. 21, 647. doi: 10.3390/ijms21020647
Takishita, Y., Charron, J. B., and Smith, D. L. (2018). Biocontrol rhizobacterium Pseudomonas sp. 23S induces systemic resistance in tomato (Solanum lycopersicum L.) against bacterial canker Clavibacter michiganensis subsp michiganensis. Front. Microbiol. 9, 2119. doi: 10.3389/fmicb.2018.02119
Vasiee, A., Behbahani, B. A., Yazdi, F. T., and Mortazavi, S. A. (2018). Diversity and probiotic potential of lactic acid bacteria isolated from Horreh, a traditional Iranian fermented food. Probiotics. Antimicrob. 10, 258–268. doi: 10.1007/s12602-017-9282-x
Wang, M., Zhu, X. L., Wang, K., Lu, C. G., Luo, M. Y., Shan, T. L., et al. (2018). Wheat caffeic acid 3-O-methyltransferase Tacomt-3d positively contributes to both resistance to sharp eyespot disease and stem mechanical strength. Sci. Rep. 8, 6543. doi: 10.1038/s41598-018-24884-0
Wu, Z. S., Huang, Y. Y., Li, Y., Dong, J. W., Liu, X. C., and Li, C. (2019). Biocontrol of Rhizoctonia solani via induction of the defense mechanism and antimicrobial compounds produced by Bacillus subtilis SL-44 on Pepper (Capsicum annuum L.). Front. Microbiol. 10, 2676. doi: 10.3389/fmicb.2019.02676
Xu, Y. L., Li, X. Y., Cong, C., Gong, G. L., Xu, Y. P., Che, J., et al. (2020). Use of resistant Rhizoctonia cerealis strains to control wheat sharp eyespot using organically developed pig manure fertilizer. Sci. Total. Environ. 726, 138568. doi: 10.1016/j.scitotenv.2020.138568
Yi, Y., Luan, P., Liu, S., Shan, Y., Hou, Z., Zhao, S., Jia, S., and Li, R. (2022). Efficacy of Bacillus subtilis XZ18-3 as a biocontrol agent against Rhizoctonia cerealis on wheat. Agriculture 12, 258. doi: 10.3390/agriculture12020258
Zhang, Z. X., Wang, H. Y., Wang, K. Y., Jiang, L. L., and Wang, D. (2017). Use of lentinan to control sharp eyespot of wheat, and the mechanism involved. J. Agr. Food. Chem. 65, 10891–10898. doi: 10.1021/acs.jafc.7b04665
Zhao, X. L., Song, P., Hou, D. Y., Li, Z. L., and Hu, Z. J. (2021). Antifungal activity, identification and iosynthetic potential analysis of fungi against Rhizoctonia cerealis. Ann. Microbiol. 71, 41. doi: 10.1186/s13213-021-01654-4
Keywords: Burkholderia ambifaria, biological control, Rhizoctonia cerealis, colonization, antifungal activity
Citation: An C, Ma S, Liu C, Ding H and Xue W (2022) Burkholderia ambifaria XN08: A plant growth-promoting endophytic bacterium with biocontrol potential against sharp eyespot in wheat. Front. Microbiol. 13:906724. doi: 10.3389/fmicb.2022.906724
Received: 29 March 2022; Accepted: 04 July 2022;
Published: 28 July 2022.
Edited by:
Daniela Trivella, National Center for Research in Energy and Materials, BrazilReviewed by:
Mohsen Mohamed Elsharkawy, Kafrelsheikh University, EgyptVipin Kumar Singh, Banaras Hindu University, India
Sueli Van Der Sand, Federal University of Rio Grande do Sul, Brazil
Wei-Liang Kong, Nanjing Forestry University, China
Yanglei Yi, Northwest A&F University, China
Copyright © 2022 An, Ma, Liu, Ding and Xue. This is an open-access article distributed under the terms of the Creative Commons Attribution License (CC BY). The use, distribution or reproduction in other forums is permitted, provided the original author(s) and the copyright owner(s) are credited and that the original publication in this journal is cited, in accordance with accepted academic practice. No use, distribution or reproduction is permitted which does not comply with these terms.
*Correspondence: Wenjiao Xue, eC13ZW5qaWFvQDE2My5jb20=