- 1Department of Food Science and Technology, Biotechnical Faculty, University of Ljubljana, Ljubljana, Slovenia
- 2Department of Microbiology, Biotechnical Faculty, University of Ljubljana, Ljubljana, Slovenia
- 3Veterinary Diagnostic and Production Animal Medicine, College of Veterinary Medicine, Iowa State University, Ames, IA, United States
- 4Veterinary Microbiology and Preventive Medicine, College of Veterinary Medicine, Iowa State University, Ames, IA, United States
Campylobacter jejuni is the leading cause of bacterial gastroenteritis, or campylobacteriosis, in humans worldwide, and poultry serves as a major source of infection. To reduce the risk associated with C. jejuni transmission via poultry meat, effective interventions during poultry production are needed, and the use of probiotics is a promising approach. In this study, 15 Bacillus subtilis strains were initially screened for their anti-Campylobacter activities. B. subtilis PS-216 strain demonstrated the best anti-Campylobacter activity against 15 C. jejuni isolates when examined using in vitro co-cultures. To evaluate the suitability of B. subtilis PS-216 for probiotic use, its susceptibility to eight clinically important antimicrobials and simulated gastric conditions was investigated. B. subtilis PS-216 was sensitive to all of the tested antibiotics. Although vegetative cells were sensitive to gastric conditions, B. subtilis PS-216 spores were highly resistant. We further evaluated the use of a B. subtilis PS-216 spore preparation (2.5 × 106 CFU/mL water) to prevent and/or reduce C. jejuni colonization in broiler chickens in vivo. Compared to the untreated group, significantly lower Campylobacter counts were detected in caeca of broilers continuously treated with B. subtilis PS-216 spores in their drinking water. Furthermore, broilers continuously treated with B. subtilis PS-216 spores showed improved weight gain, compared to the control group. Together, these results demonstrate the potential of B. subtilis PS-216 for use in poultry to reduce C. jejuni colonization and improve weight gain.
Introduction
Campylobacteriosis is among the most frequently reported bacterial foodborne infection in both the European Union (EU) and the United States, with Campylobacter jejuni as the major causative agent. In the last decade, the number of confirmed infections in the EU exceeded 200,000/year. Symptoms of campylobacteriosis include diarrhea, fever and cramps. Death rarely occurs, but complications can increase more than fivefold due to infections with antibiotic-resistant strains (Helms et al., 2005; EFSA and ECDC, 2021a,b).
As C. jejuni is a common avian commensal, efforts are being made to tackle the pathogen at its primary source, the reservoir in chickens. Once Campylobacter is introduced onto a farm, its fast spread is imminent (Berndtson et al., 1996), and therefore effective control measures are of great importance. These can include pre-harvest measures (e.g., biosecurity, hygiene measures) to prevent Campylobacter from entering a farm and to limit its spread, and post-harvest measures (e.g., freezing, hot-water treatment, irradiation, chemical decontamination) to reduce Campylobacter after animal slaughter (Sahin et al., 2015). Reduction of Campylobacter in the chicken intestine can significantly reduce the public health risk, and thus the control of Campylobacter in poultry production is considered one of the most effective strategies for intervention (Meunier et al., 2016; Dogan et al., 2019; Koutsoumanis et al., 2020).
Probiotics can be used as a pre-harvest measure for pathogen control on poultry farms (Sahin et al., 2015; Alagawany et al., 2018; Koutsoumanis et al., 2020). Probiotics can have multiple beneficial effects on poultry, such as growth promotion, immunomodulation and inhibition of pathogens. The modes of action of probiotic bacteria against pathogens can include production of organic acids and antibacterial substances, competitive exclusion of pathogens, modulation of the host immune system, and others (reviewed by El-Hack et al., 2020; Jha et al., 2020). The main bacteria studied and used as probiotics in poultry are Lactobacillus spp., Bifidobacterium spp., Bacillus spp., Streptococcus spp., and Enterococcus spp. (Hong et al., 2005; Lutful Kabir, 2009; Alagawany et al., 2018).
Bacillus subtilis as a poultry probiotic that is included in commercial formulations, such as GalliPro (Chr Hansen), Calsporin (ORFFA), and Alterion (Novozymes). The administration in chickens of various B. subtilis strains either alone or in combination with other bacteria can improve feed conversion and body weight, reduce lesions caused by Clostridium perfringens, elongate intestinal villi in necrotic enteritis, modulate the microbiota to improve intestinal Lactobacillus numbers, and reduce the number of pathogens such as C. perfringens, Escherichia coli, Salmonella enteritidis and others (Fritts et al., 2000; Jayaraman et al., 2013; Zhou et al., 2015; Hmani et al., 2017; Park et al., 2017; Hayashi et al., 2018). Probiotic effects of different B. subtilis strains against C. jejuni in poultry have been well documented, although their anti-Campylobacter effects were shown to be variable and strain specific (Saint-Cyr et al., 2016), which indicates that not every B. subtilis treatment will result in reduced C. jejuni counts in the chicken intestine. Therefore, effective and reliable B. subtilis probiotics that carry anti-Campylobacter traits remain to be developed.
The aim of this study was to initially test different B. subtilis strains for their in vitro anti-Campylobacter activities, and then to apply the best one to in vivo testing in broiler chickens. From the 15 B. subtilis strains tested against C. jejuni NCTC 11168 in co-cultures, B. subtilis PS-216 showed the best anti-Campylobacter activity and was chosen for further evaluation. In addition to examining its anti-Campylobacter activity against different C. jejuni strains, B. subtilis PS-216 was evaluated for its antimicrobial resistance profile and its survival in the gut environment, using simulated intestinal conditions in vitro. Finally, we evaluated the use of B. subtilis PS-216 spores to prevent or reduce C. jejuni colonization in broiler chickens, and as well as their influence on the broiler weight gain. These results show that when given continuously as a water additive in broilers, B. subtilis PS-216 spores can reduce C. jejuni colonization in the broiler intestine and increased broiler body weight.
Materials and Methods
Animal Ethics
All of the animal protocols and procedures used in this study were reviewed and approved by the Institutional Animal Care and Use Committee at Iowa State University before the start of the experiments (IACUC Protocol IACUC-18-322).
Strains and Growth Conditions
Campylobacter jejuni isolates were derived from human feces, surface water and slaughter house environments, and were previously characterized by Kovač et al. (2018). B. subtilis strains were isolated from riverbank soil from Sava River in Slovenia, and were characterized by Štefanič and Mandic Mulec (2009). B. subtilis strains from tomato rhizosphere were characterized by Oslizlo et al. (2015) (Supplementary Table 1).
The bacterial strains were stored in 20% glycerol (Kemika, Croatia) and 80% Mueller Hinton (MH) broth (Oxoid, United Kingdom) at –80°C. C. jejuni strains were revitalized on Karmali agar (Oxoid, United Kingdom) supplemented with Karmali selective supplement (SR0167E; Oxoid, United Kingdom), with incubation at 42°C in a anaerobic jar flushed with tri-gas mixture (5% O2, 10% CO2, 85% N2) to provide microaerobic conditions for 24 h. B. subtilis strains were revitalized on MH agar (BD Difco, United States), with incubation at 37°C under aerobic conditions. The second passage was prepared as an overnight culture (16 h incubation) and was used in the experiments, as described below. To enumerate C. jejuni in monocultures and co-cultures, Karmali agar (Oxoid, United Kingdom) supplemented with Karmali selective supplement (SR0167E; Oxoid, United Kingdom) was used. For enumeration of C. jejuni from fecal samples, MH agar was supplemented with Bolton broth selective supplement (SR0183; Oxoid, United Kingdom) and growth supplement (SR0232E; Oxoid, United Kingdom) (MH-BSS). For enumeration of Bacillus sp. from fecal samples, HiChrom Bacillus agar (Himedia, United States) was used.
Co-cultivation of Bacillus subtilis With Campylobacter jejuni in Mueller Hinton Broth
Fifteen different Bacillus subtilis strains (Supplementary Table 1) were co-cultivated in MH broth with C. jejuni 11168 at a 1:10 starting ratio (5 × 104 CFU/mL: 5 × 105 CFU/mL) in favor of C. jejuni. Co-cultures and control monocultures were cultivated at 42°C under microaerobic conditions for 24 h. B. subtilis strain PS-216 was chosen for the further experiments on the basis of showing the greatest anti-Campylobacter effects, as shown by the highest C. jejuni reduction in co-culture.
The anti-Campylobacter effects of B. subtilis PS-216 were further tested on 15 different C. jejuni strains isolated from a slaughterhouse environment (S1–5), human feces (H1–5), and surface water (W1–5) (Kovač et al., 2018; Supplementary Table 1). The B. subtilis and C. jejuni were co-cultivated at 42°C under microaerobic conditions for 24 h in static cultures. The densities (CFU/mL) of C. jejuni and B. subtilis in the monocultures and co-cultures were determined by plating the cultures on selective media and incubating the plates at the appropriate temperatures under microaerobic and aerobic conditions, respectively. Co-cultivation experiments were carried out as three biological replicates, with up to three technical replicates.
Bacillus subtilis Spore Preparation
Bacillus subtilis spores were prepared according to Warriner and Waites (1999), with some modifications. Briefly, an overnight culture of B. subtilis prepared in LB media (200 rpm, 37°C) was inoculated (at 1% inoculum) into sporulation medium, which contained 16 g/L Nutrient broth (Oxoid, United Kingdom), 2 g/L KCl (Thermo Fisher Scientific, United States), 1 mM MgSO4 (Oxoid, United Kingdom), 1 mM CaCl2 (Merck, Germany), 1 μM FeSO4 (Sigma Aldrich, Germany), 10 μM MnCl2 (Sigma Aldrich, Germany) and 2.8 mM D-(+)-glucose (Sigma Aldrich, Germany), and incubated for 4 days (200 rpm, 37°C). The culture was treated at 80°C for 30 min and washed with 0.9% NaCl (10,000 × g, 10 min) three times before being stored in 10% glycerol at –20°C. The spore concentrations (as CFU/mL) were determined before freezing and after thawing, prior to the start of the experiments.
Determination of Bacillus subtilis Susceptibility to Antimicrobials
Minimal inhibitory concentrations (MICs) for eight antimicrobials of human or veterinary importance were determined against two B. subtilis strains, B. subtilis PS-216 and B. subtilis ATCC 6633, by the broth microdilution method using MH broth. Twofold serial dilutions of the antibiotics tetracycline (Fluka, Switzerland), chloramphenicol (Calbiochem, United States), kanamycin (Calbiochem, United States), erythromycin (Sigma, United States), streptomycin (Sigma, United States), gentamycin (Glentham Life Science, United Kingdom), tylosin tartrate (Sigma, United States) and ampicillin (Roche, Germany) were prepared at concentrations ranging from 0.5 to 64 μg/mL. The assays were performed in 96-well microtiter plates. For each well, 50 μL of the corresponding dilutions of the antibiotics were added to 50 μL bacterial suspension previously diluted in MH broth to 105 CFU/mL from an overnight culture (LB broth, 37°C, 16 h, aerobic conditions, 200 rpm). The MICs are expressed as the lowest concentration of antibiotic at which no visible growth of bacteria occurred (i.e., absence of turbidity). Breakpoint values as described by the European Food Safety Agency (EFSA, 2012) were used to interpret the MIC results of various antimicrobials against Bacillus spp.
Acid and Bile Salt Tolerance of Bacillus subtilis Vegetative Cells and Spores
Resistance of vegetative cells and spores to simulated gastric conditions and bile salts was determined as described previously (Barbosa et al., 2005; Mingmongkolchai and Panbangred, 2018), with some modifications. Briefly, B. subtilis overnight cultures (16 h) in Luria-Bertani (LB) medium (Conda, Spain) containing 1% glucose to prevent sporulation were sub-cultured (at 1% inoculum) into LB medium containing 1% glucose, and grown at 37°C with shaking (110 rpm) for 3 h. Vegetative cells were diluted to an OD600 of 0.1 in LB medium acidified to pH 2.5 with 2 M HCl and supplemented with 1 mg/mL pepsin from porcine gastric mucosa (Sigma-Aldrich, Switzerland), or in LB medium supplemented with 0.3% (w/v) bile salts (Oxoid, United Kingdom). For spore assays, the spores were diluted in 5 mL 0.85% NaCl adjusted to pH 2.5 and supplemented with 1 mg/mL pepsin, or isotonic buffer (Oxoid, United Kingdom) supplemented with 0.3% bile salts (Oxoid, United Kingdom). Cultures were incubated at 37°C with agitation (110 rpm), and aliquots were removed after 30, 60, and 90 min for acid tolerance, and after 60 and 180 min for bile salt tolerance. Bacterial cell survival (%) was calculated as follows: NA/NB × 100, where NA = log CFU/mL after incubation and NB = log CFU/mL before incubation.
Broiler Chicken Colonization
One-day-old broiler chicks (n = 45) were obtained from a commercial hatchery and were divided randomly into four groups with 11 or 12 chicks per group. The broilers were kept in tubs with soft bedding, and water (regular city water) and feed (Purina non-medicated starter feed with nutritional content presented in Supplementary Table 2) provided ad libitum. The animals were housed in a room with BSL-2 practices in place at laboratory animal facilities at Iowa State University with standard temperature and lightning recommended by commercial broilers in the region (Supplementary Table 3).
At the age of 8 d, all of the broilers were inoculated with 4 × 106 CFU C. jejuni 11168 by oral gavage. Successful colonization was confirmed by cloacal swabs 5 days after inoculation.
To evaluate the use of B. subtilis PS-216 spores to prevent and/or reduce C. jejuni colonization, the broilers were administered one of the following B. subtilis treatment regimens: (i) No treatment Control (n = 12), as inoculated with C. jejuni, but not treated with B. subtilis PS-216 spores; (ii) Pre-treatment group (n = 11), as treated with B. subtilis PS-216 spores before inoculation with C. jejuni, from an age of 1 to 7 days; (iii) Continuous treatment (n = 11), as treated with B. subtilis PS-216 spores throughout the experiment, from an age of 1 to 20 days; and (iv) Post-treatment group (n = 11), as treated with B. subtilis PS-216 spores 5 days after inoculation with C. jejuni, from an age of 13–20 days. The B. subtilis PS-216 spore treatments were administered in the drinking water at approximately 2.5 × 106 CFU/mL water B. subtilis PS-216 spores on the corresponding days as given above. The spore-containing drinking water was given to the broiler chickens ad libitum.
Cloacal swabs were collected from each broiler prior to C. jejuni inoculation (day 0) to confirm the absence of C. jejuni, 5 days after inoculation (day 5) to confirm colonization with C. jejuni, and 8 and 11 days after inoculation. At 21 days of age, all of the broilers were sacrificed and weighted, and the cecum contents were collected. To enumerate C. jejuni in feces, all of the swabs and cecum contents collected were diluted 10-fold in MH broth, and then plated onto MH-BSS and treated at 80°C for 30 min, and plated onto HiChrom Bacillus agar to enumerate the Bacillus spores.
Statistical Analysis
One-way ANOVA with Tukey’s post-hoc tests was used to analyze the effects of different B. subtilis strains on C. jejuni NCTC 11168. To evaluate the effects of co-culture on B. subtilis growth, Student’s t-tests (paired) were used. Two-way ANOVA with Bonferroni post-hoc tests was used to analyze the effects of B. subtilis PS-216 on multiple C. jejuni strains. The differences between treated and untreated broiler groups were analyzed using Student’s t-tests. Statistical analysis was performed with the SPSS software, version 21 (IBM Corp., NY, United States) and GraphPad Prism software, version 8 (GraphPad Software Inc., CA, United States). A P-value < 0.05 was considered statistically significant.
Results
Bacillus subtilis Reduces Growth of Campylobacter jejuni in Co-culture
To determine and select the most suitable B. subtilis strain against C. jejuni, we initially analyzed the anti-Campylobacter activities against C. jejuni NCTC11168 of 15 B. subtilis strains isolated from riverbank soil in Slovenia (Štefanič and Mandic Mulec, 2009) and from tomato rhizosphere (Oslizlo et al., 2015). The majority of the B. subtilis strains showed substantial anti-Campylobacter effects, with significant reductions in CFU/mL of C. jejuni (P < 0.05). Only three B. subtilis strains had no effects on the growth of C. jejuni (P > 0.05): PS-218, PS-18, and T16-10. Compared to C. jejuni mono-cultures, the co-cultures with B. subtilis for 24 h reduced C. jejuni by 1.03–3.01 log CFU/mL (Figure 1A). Among the B. subtilis strains, PS-216 showed the highest reduction of C. jejuni 11168 (3.01 log CFU/mL), and was therefore selected for the further experiments (Figure 1A).
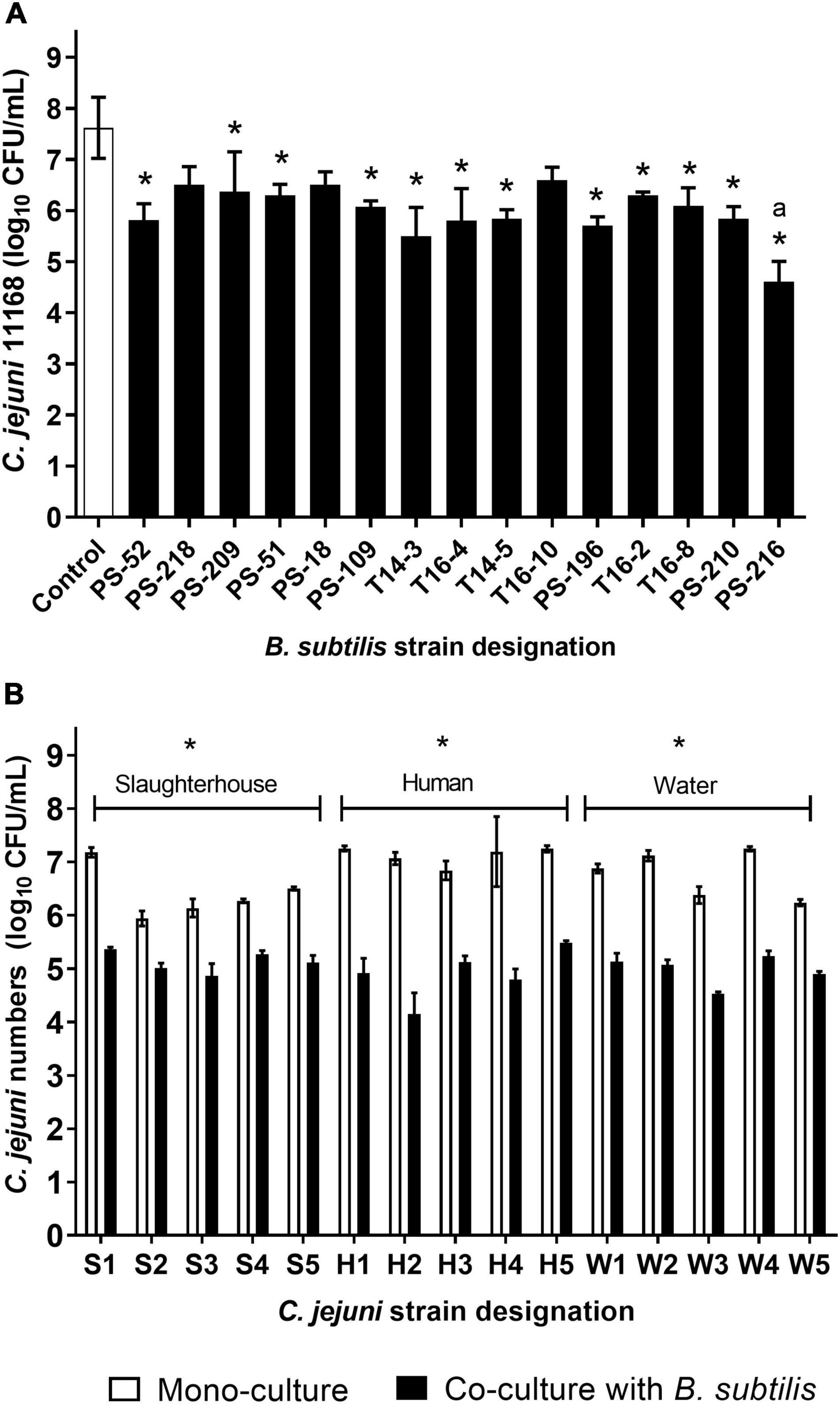
Figure 1. Anti-Campylobacter activities of the 15 B. subtilis strains against C. jejuni NCTC11168 (A) and of B. subtilis PS-216 against 15 C. jejuni strains (B). Data are means ± standard deviation from three replicates. *P < 0.05, vs. relevant control (one-way ANOVA with Tukey’s post-hoc tests).
To confirm the efficacy of B. subtilis PS-216 against C. jejuni, it was further tested by co-culture against 15 C. jejuni strains isolated from various sources. Significant reductions in C. jejuni by B. subtilis PS-216 were seen, which ranged from 0.93 to 2.81 log CFU/mL (P < 0.05) across the different C. jejuni strains (Figure 1B). B. subtilis PS-216 was most effective against C. jejuni strains isolated from human feces, with an average reduction of 2.22 ± 0.45 log CFU/mL, and was least effective against strains from the slaughterhouse environment, with an average reduction of 1.28 ± 0.32 log CFU/mL (Figure 1B). No significant differences in the growth of B. subtilis were seen when the B. subtilis strains were grown in co-cultures with Campylobacter or grown in monocultures (Supplementary Figure 1). Although the co-cultivation conditions were favorable for C. jejuni according to the higher starting numbers and microaerobic environment, these results showed that B. subtilis PS-216 had broad anti-Campylobacter activity against these different C. jejuni strains of various origins.
Bacillus subtilis PS-216 Is Susceptible to Antimicrobials
For a probiotic to be considered safe for use in human and animal nutrition, it should not harbor any transferable antibiotic resistance genes (FEEDAP, 2012; Pariza et al., 2015). Thus, the antimicrobial susceptibilities of B. subtilis PS-216 to eight antimicrobials relevant to human and veterinary medicine were examined. The antibiotics tested were tetracycline, chloramphenicol, kanamycin, erythromycin, streptomycin, gentamycin, tylosin tartrate and ampicillin, and the reference strain B. subtilis ATCC 6633 was used as a control in the MIC assays. Both B. subtilis PS-216 and the reference strain were susceptible to all of the antimicrobials tested, with the MICs for tetracycline, streptomycin and ampicillin against B. subtilis PS-216 > 16-fold, 2-fold, and > 4-fold higher than those in the reference strain (Table 1).

Table 1. Susceptibility of B. subtilis PS-216 and reference strain B. subtilis ATCC 6633 to antibiotics.
Bacillus subtilis PS-216 Spores Are Highly Resistant to Simulated Gastric Conditions
For a probiotic to be effective against C. jejuni, it must survive the harsh gastrointestinal conditions (i.e., acid and bile salts) to reach the colonization site of C. jejuni in the intestine. The acid and bile salt tolerance assays showed that the vegetative cells of B. subtilis PS-216 were very susceptible to simulated gastric conditions, with 100% loss of cell viability when exposed to simulated gastric conditions at 37°C for 30 min (Table 2). In contrast, the spores of B. subtilis PS-216 showed 100% survival after 90 min of exposure to simulated gastric conditions and after 180 min in 0.3% bile salts. This showed that the spores of B. subtilis PS-216 have excellent resistance to simulated gastric conditions and to 0.3% bile salts, which suggests that the spore form of B. subtilis PS-216 can be used as a probiotic in chickens.
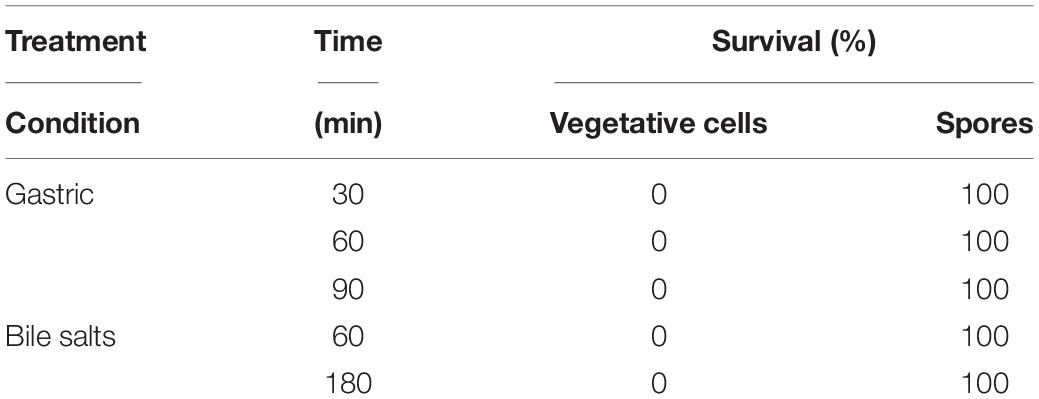
Table 2. Survival of B. subtilis PS-216 vegetative cells and spores under simulated gastric conditions (1 mg/mL pepsin, pH 2.5) and with 0.3% bile salts, presented as % of cells/spores after treatment.
Bacillus subtilis PS-216 Reduces Campylobacter jejuni Colonization in Broiler Chickens
To evaluate the effects of B. subtilis PS-216 on C. jejuni colonization in broiler chickens, B. subtilis spore solutions (2.5 × 106 CFU/mL water) were administered to broilers via their drinking water. The broilers underwent the following treatment regimens (Figure 2A): (i) Control group, inoculated with C. jejuni but not treated with B. subtilis PS-216 spores; (ii) Pre-treatment group, treated with B. subtilis PS-216 spores 7 days prior to C. jejuni inoculation (B. subtilis PS-216 as a preventive measure); (iii) Continuous treatment group, treated with B. subtilis PS-216 spores for the duration of the experiment (21 days); and (iv) Post-treatment group, treated with B. subtilis PS-216 spores 8 days after the broilers were colonized with C. jejuni (B. subtilis PS-216 as a curative measure).
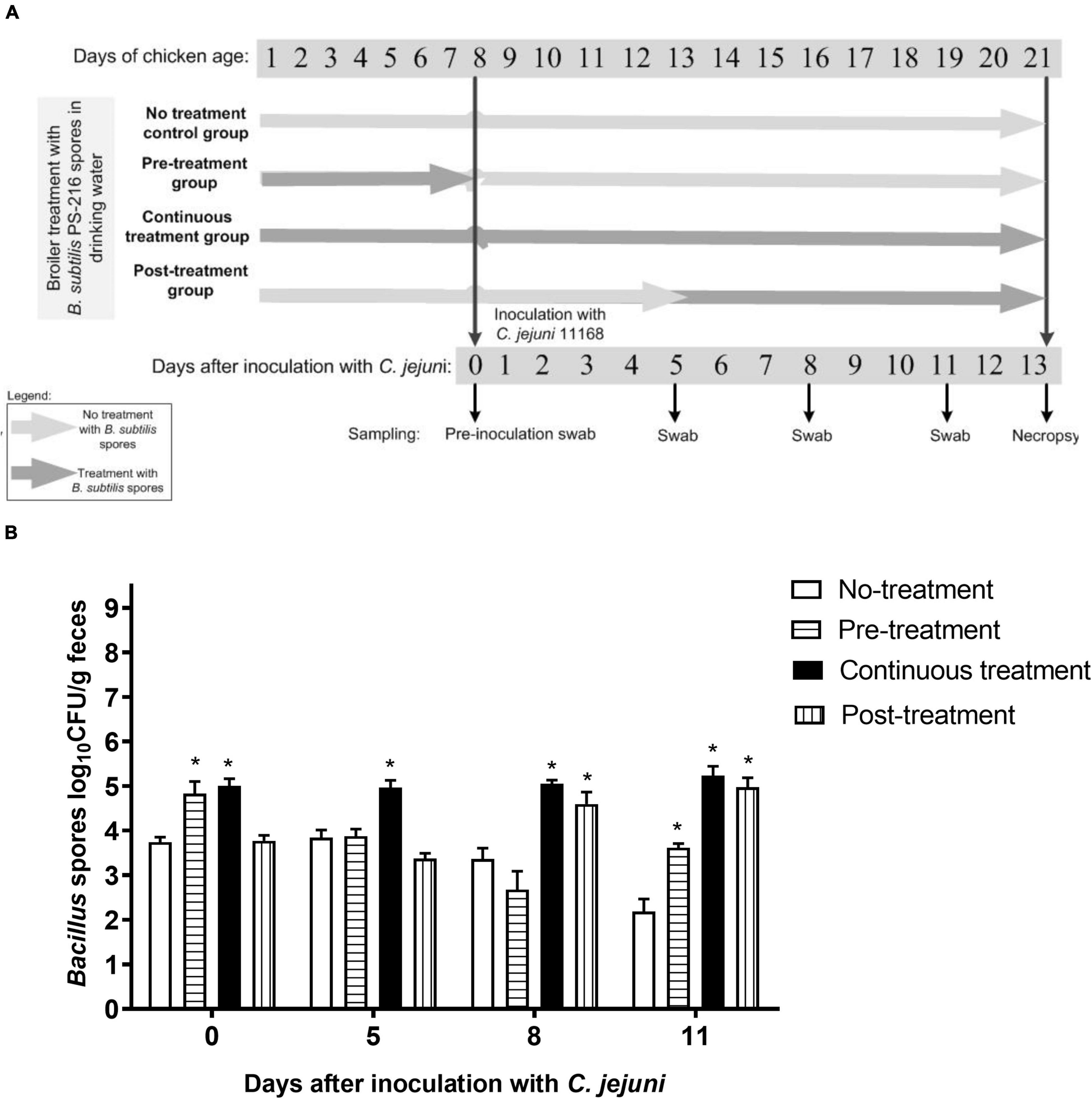
Figure 2. Schematic representation of broiler colonization with C. jejuni 11168 and B. subtilis PS-216 treatments (A); and detection of Bacillus spores in the broiler feces according to the B. subtilis PS-216 treatments given. Data are means ± standard deviation. *P < 0.05 vs. untreated controls (Student’s t-tests) (B).
All of the broilers had baseline Bacillus spore counts in their feces (3.8 × 103 CFU/g feces) at placement when they were 1 day old, and as expected, the Bacillus spore counts were higher when the broilers were given B. subtilis PS-216 spores in their drinking water (Figure 2B). Furthermore, these higher Bacillus spore counts (> 5 × 104 CFU/g feces) dropped abruptly after treatment cessation. This is exemplified by the Pre-treatment group of broilers, where high Bacillus spore counts were detected at first sampling during the B. subtilis PS-216 spore treatment (day 0 after inoculation with C. jejuni), with a drop to baseline (comparable to untreated Control group) after treatment cessation (second sampling, day 5 after inoculation with C. jejuni) (Figure 2B). For the last feces sampling day (day 11 after inoculation with C. jejuni), all of the treated groups (Pre-treatment, Continuous treatment, Post-treatment) showed higher Bacillus spore counts compared to the untreated Control group (by 1.57, 3.19, 2.94 log CFU/g feces, respectively). Campylobacter counts showed no significant differences between groups at cloacal swab samplings (Supplementary Figure 2).
At 21 days of age, all of the broilers were sacrificed, and their cecum contents were examined for C. jejuni and for Bacillus spores. C. jejuni was detected in all of the broilers, regardless of the treatment regimens (Figure 3A). However, compared to the untreated Control group, there was a significant decrease in C. jejuni counts in the cecum contents in the group continuously treated with B. subtilis PS-216 spores (P = 0.002), with a mean decrease of 1.2 log CFU/g feces in the C. jejuni counts. In contrast, the C. jejuni counts in the Pre-treatment and Post-treatment groups were comparable to the untreated Control group. This indicates that the continuous supplementation of broilers with B. subtilis PS-216 spores is an effective measure to lower the CFU counts of C. jejuni in the caeca.
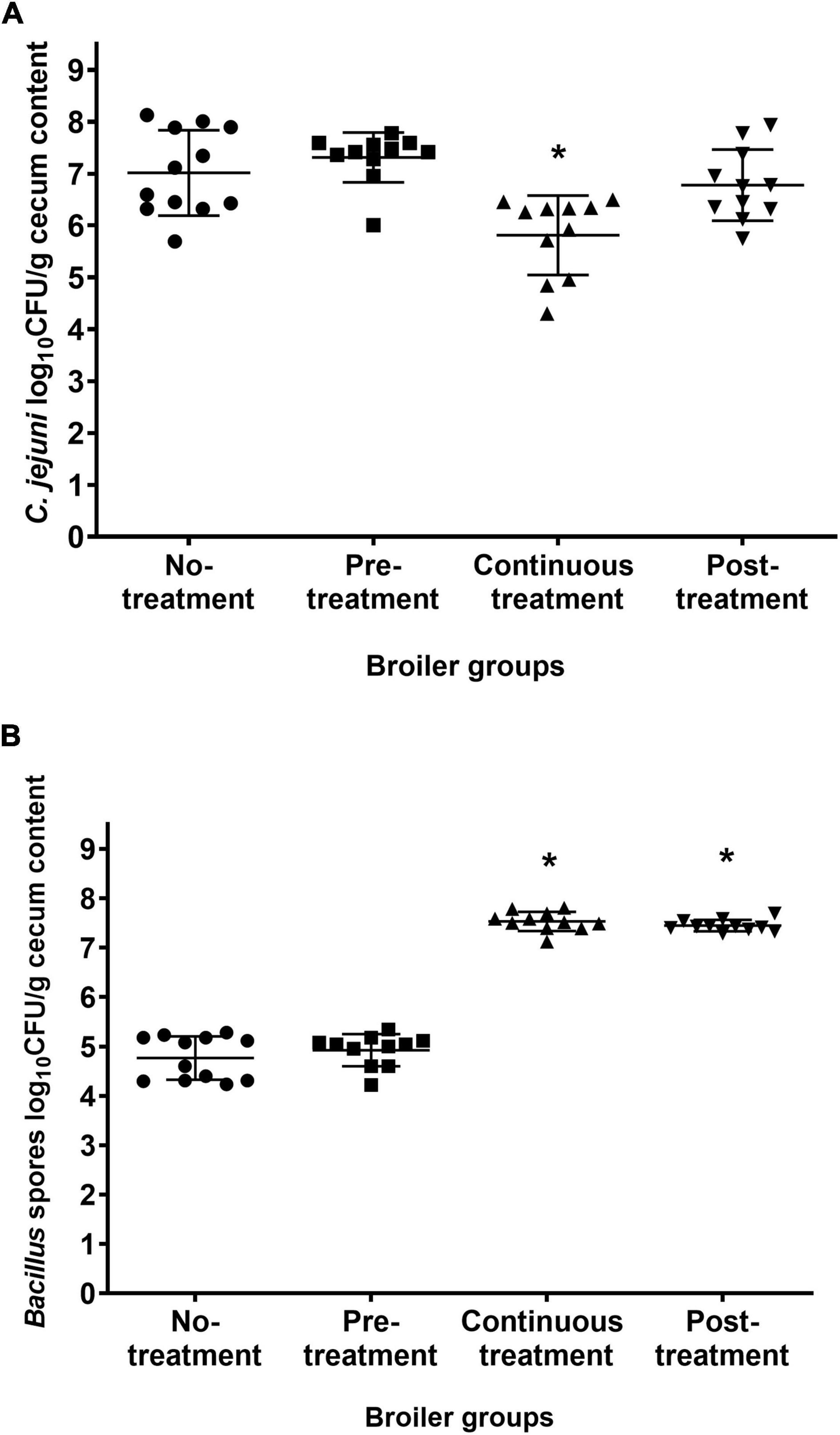
Figure 3. Cecum contents of C. jejuni (A) and Bacillus spores (B) of the broilers 21 days of age according to the B. subtilis PS-216 treatments given. Each symbol represents an individual broiler chicken. Horizontal line, means ± standard deviations. *P < 0.01 vs. untreated control (Student’s t-tests).
For the Bacillus spore counts, the 7-days pre-treatment of the broilers with B. subtilis PS-216 spores had no effects on the final Bacillus spore counts in the cecum, as this was comparable to the untreated Control group (Figure 3B). However, the Bacillus spore counts in the broilers treated with B. subtilis PS-216 spores until the necropsy time (Continuous treatment, Post-treatment) were higher than those in the untreated Control group (increases of 2.76, 2.68 log CFU/g feces, respectively; P < 0.05).
Bacillus subtilis PS-216 Spore Treatment Increases Weigh Gain in Broilers
The body weights of the broilers were measured at the end of the study to determine whether the water supplementation of B. subtilis PS-216 spores affected the weight gain of the broilers. Indeed, the weights of the broilers in the three groups treated with B. subtilis PS-216 spores were significantly higher than those of the untreated Control group (Figure 4; P < 0.05). An average increase of 158 g was measured for the Pre-treatment group, 134 g for the Continuous treatment group, and 124 g for the Post-treatment group, which represented further weight increases over the non-treated Control group of 37, 32, and 30%, respectively.
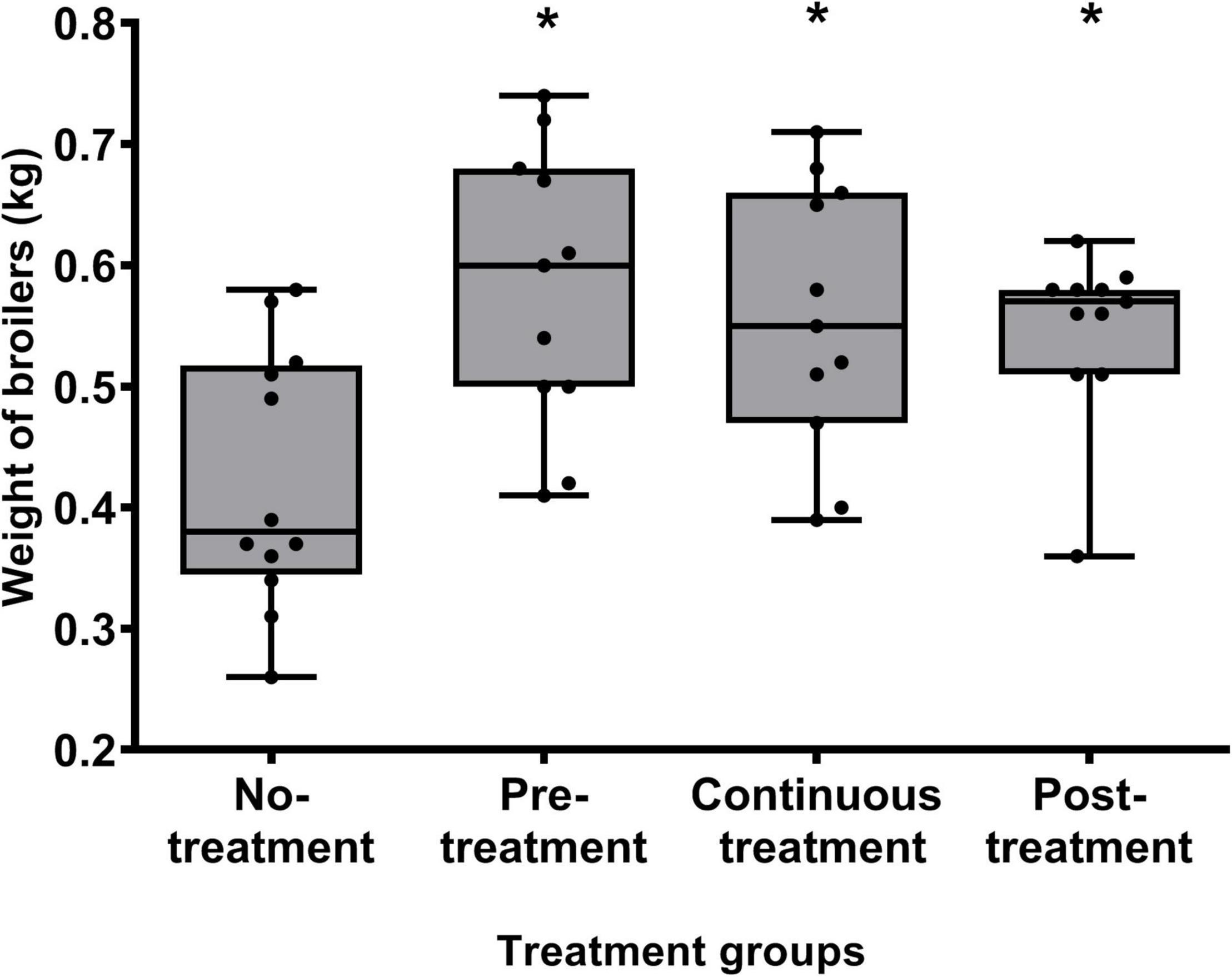
Figure 4. Body weights of the broilers at 21 days of age according to the B. subtilis PS-216 treatments given. Each symbol represents an individual broiler chicken. Data are shown as box plots with whiskers. *P < 0.05 vs. untreated control (Student’s t-tests).
Altogether, these data showed that treatment of the broilers with B. subtilis PS-216 spores in their drinking water significantly reduced the CFU/g of C. jejuni in cecum content and increased the bodyweight of these broiler chickens.
Discussion
The food-borne pathogen C. jejuni is among the most prevalent bacterial causes of gastroenteritis in the EU and the United States (Tam and O’Brien, 2016; Kuhn et al., 2020). Although C. jejuni can be spread through various routes, the main source of C. jejuni is the reservoir in chickens. Thus, control of C. jejuni at the farm level should help to reduce the risk of C. jejuni spread through the food chain. Probiotics (i.e., live-fed microbials) can provide benefits to the animal host, and if shown to be effective for the reduction of C. jejuni, they can be used as a pre-harvest intervention on farms (van Wagenberg et al., 2020). In the present study, we identified B. subtilis PS-216 as an additive that can be given to broiler chickens in the form of spores in the drinking water to reduce C. jejuni colonization and to increase the broiler weight gain.
Previous studies have reported that probiotic bacteria can show species-specific and strain-specific anti-Campylobacter activities both in vitro and in the chicken host (Mohan, 2015; Mortada et al., 2020). Moreover, Wine et al. (2009) reported that one Lactobacillus strain was effective for the inhibition of C. jejuni, while another was not, while the same two strains did not have the same effects on two different C. jejuni strains. We have shown a similar pattern here, although not to the same extent, in the anti-Campylobacter activity of different B. subtilis strains. Of the 15 B. subtilis strains tested, the majority (12 of 15) inhibited the growth of C. jejuni 11168 to various levels. Nevertheless, B. subtilis PS-216 had superior effects compared to all of the other B. subtilis stains tested, and thus the activity of B. subtilis PS-216 was additionally tested, and confirmed, against 15 different C. jejuni strains that originated from three different environments. Contrary to Wine et al. (2009), we observed a similar activity of PS-216 against all of the C. jejuni strains tested; i.e., all of the reductions in C. jejuni were significant regardless of the C. jejuni origin. This confirmed the broad anti-Campylobacter effects of B. subtilis PS-216 in vitro, and highlights the potential of this strain for use in high-load Campylobacter environments, such as the poultry intestinal tract.
However, before a probiotic can be used commercially, it must first meet additional standards. One of these requirements is susceptibility to antibiotics, as probiotics can carry antibiotic resistance determinants that can be transferred to other residential bacteria in the gut via horizontal gene transfer, which will amplify the growing problem of antibiotic resistance (Sharma et al., 2014; Zheng et al., 2017; EFSA and ECDC, 2019). Thus, it is of utmost importance that probiotics do not serve as genetic reservoirs for the emergence of antibiotic resistance, and consequently do not pose a risk to animal and human health. We tested the B. subtilis PS-216 susceptibility to antibiotics according to EFSA guidance (FEEDAP, 2012). B. subtilis PS-216 was indeed susceptible to the several antibiotics examined in this study, and therefore complies with the required probiotic standards.
Additionally, to be effective, probiotic strains must reach the gut, and thus they must withstand the harsh conditions of the gastrointestinal tract of an animal. Indeed, the spores of B. subtilis PS-216 were highly resistant to simulated conditions of the gastrointestinal tract in this study, and thus should pass through the gastrointestinal tract and into the intestine, where their action against pathogens needs to take place. On the contrary, the vegetative cells of B. subtilis PS-216 did not survive these gastric conditions, and are thus not suitable for such probiotic use. Cartman et al. (2008) showed that B. subtilis spores germinate and proliferate in the chicken intestine. Our results also suggest that B. subtilis PS-216 germinates and proliferates in the broiler intestine, as the Bacillus CFU/g counts of cecum samples at day 21 were approximately 1 log higher than in the inoculation solution. Thus, only the spores need to survive gastric conditions to have a probiotic effect.
A further requirement that a potential probiotic must meet is in vivo efficacy, as the efficacy of a probiotic cannot be judged by in vitro results alone (Robyn et al., 2013; Mortada et al., 2020). Here, both the in vitro and in vivo results showed a significant reduction in Campylobacter for the treatment with B. subtilis PS-216 spores. The B. subtilis PS-216 spores were added to broiler chicken drinking water as a treatment solution: (i) Before inoculation with C. jejuni, to test this as a preventive measure; (ii) after established colonization with C. jejuni, to test this as a therapeutic measure; and (iii) continuously from day 1. This animal study yielded three important conclusions: (i) The Bacillus spore counts in the feces increased during the B. subtilis PS-216 spore treatment, but decreased after the treatment ended; (ii) continuous B. subtilis PS-216 spore treatment reduced C. jejuni in the cecum of broilers, although the preventive (Pre-treatment) and therapeutic (Post-treatment) uses of B. subtilis were not effective at the B. subtilis PS-216 spore concentration used here (106 CFU/mL water); and (iii) short-term treatment at the beginning or end of the experiment increased the broiler weights compared to the untreated controls. Previously, Latorre et al. (2014) reported that only continuous treatments of chickens with B. subtilis spores led to high spore counts in the chicken intestine. This is in line with our results, where there was a decrease in the Bacillus spore counts in feces and cecum after short-term B. subtilis PS-216 spore treatment, but a constant Bacillus spore count when the B. subtilis PS-216 spores were added continuously. An increase in Bacillus spore counts after the addition of B. subtilis spores was also shown by Ciurescu et al. (2020); however, the level of Bacillus spore counts in the treated groups in the cecum content were different from the present study. We determined Bacillus spore counts of around 107 CFU/g feces, while Latorre et al. (2014) observed lower Bacillus spore counts, of approximately 105 CFU/g feces. Interestingly, the number of Bacillus spores in the cecum was also higher than in the feces, and higher than the concentration of B. subtilis PS-216 spores added to the drinking water (i.e., the treatment solution). This suggests that the B. subtilis PS-216 spores either germinate in the intestine into vegetative cells that then die off before being passed as feces, or the B. subtilis PS-216 spores somehow accumulate in the broiler cecum. Nevertheless, the high spore counts without continuous administration were not sufficient to reduce the number of C. jejuni in the cecum of the treated broilers. Continuous treatment with B. subtilis PS-216 spores (106 CFU/mL water) resulted in a significant reduction in C. jejuni (1.2 log CFU/g feces) that was comparable to multispecies probiotics containing B. subtilis tested by Arsi et al. (2015) (up to 3 log CFU/g feces reduction), multi-strain B. subtilis probiotics tested by Aguiar et al. (2013) (from 1 to 4 log CFU/g feces reduction), and a single species B. subtilis probiotic Calsporin (Guyard-Nicodème et al., 2016) (1.7 log CFU/g reduction). Differences in B. subtilis efficacy in broilers can be attributed to strain variability, as well as to treatment variability.
One possible mechanism of anti-Campylobacter action of B. subtilis is competitive exclusion, in which the probiotic occupies the C. jejuni attachment sites in the intestine, thus preventing C. jejuni adhesion (Wine et al., 2009). B. subtilis strains have been shown before to reduce pathogens like E. coli (La Ragione et al., 2001) and Salmonella (La Ragione and Woodward, 2003) in the intestine by means of competitive exclusion. Some B. subtilis strains have been shown to promote the colonization of Lactobacillus in chicken intestine (Park et al., 2017), thereby acidifying the intestine and making it an unfriendly environment for C. jejuni. B. subtilis is also known to produce antimicrobials (Tamehiro et al., 2002; Huang et al., 2009; Efremenkova et al., 2016) that might act in the gut, although it is not clear whether these would be expressed in the broiler intestine. In previous studies, we showed that B. subtilis PS-216 can act against the reference C. jejuni NCTC 11168 strain in variable settings by producing the antimicrobial substance bacillaene (Erega et al., 2021; Šimunović et al., 2022); however, we have no data at this stage to support the hypothesis that this antimicrobial affects the survival of C. jejuni in these broiler chickens.
Adding probiotics as feed/water supplements represents a good Campylobacter control strategy (Koutsoumanis et al., 2020). B. subtilis probiotics have been shown to have immunomodulatory effects in the gut and to modulate the gut microbiota (Hayashi et al., 2018), promote muscle development and meat quality (Zhou et al., 2015), and promote growth, feed conversion and body weight in chickens with potential anti-pathogenic effects, due to their good enzymatic activity (Fritts et al., 2000; Hmani et al., 2017; Park et al., 2017). Although such an intervention might increase production costs somewhat, and consequently the cost of the food on the market, it can also be a cost-effective intervention when the addition of probiotics reduces the risk of Campylobacter and simultaneously increases the growth performance (van Wagenberg et al., 2020). All of the B. subtilis PS-216 spore treatments in the present study resulted in increased body weight of these broiler chickens. Remarkably, even the 8-days treatment resulted in a significant weight improvement. Thus, this use of B. subtilis PS-216 spores offers the poultry industry different options: short-term use that can be implemented to increase the weight of broilers, and continuous use that on top of the weight increase, can also reduce C. jejuni counts and improve the safety of the food. In conclusion, our study suggests that B. subtilis PS-216 spores represent a new and attractive solution to enhance broiler chicken health and to increase poultry and food safety.
To conclude, these results underline the potential of B. subtilis PS-216 spores as a treatment for improving poultry production and food safety. The anti-Campylobacter activity of B. subtilis PS-216 was demonstrated by in vitro and in vivo experiments in this study. In addition to its anti-Campylobacter activity, B. subtilis PS-216 spore treatments also increased the weight gain of the broiler chickens. Although B. subtilis might also act against other pathogenic bacteria, this was not tested in the present study, and future studies are needed to confirm the overall beneficial effects of B. subtilis PS-216 spores for enhanced poultry health and performance. In the future, it will also be necessary to evaluate the anti-Campylobacter effects of B. subtilis PS-216 under different settings, to determine its reproducibility and broader applicability as a viable intervention measure for Campylobacter in primary poultry production.
Data Availability Statement
The original contributions presented in this study are included in the article/Supplementary Material, further inquiries can be directed to the corresponding author.
Ethics Statement
All of the animal protocols and procedures used in this study were reviewed and approved by the Institutional Animal Care and Use Committee at Iowa State University before the start of the experiments (IACUC Protocol IACUC-18-322).
Author Contributions
KŠ, PŠ, AK, QZ, OS, IMM, and SSM: conceptualization. KŠ, PŠ, and AK: methodology and validation. KŠ: formal analysis, data curation, writing—original draft preparation, and visualization. KŠ, AE, and OS: investigation. IMM, SSM, and QZ: resources. PŠ, AK, IMM, SSM, QZ, and OS: writing—review and editing and supervision. IMM, SSM and AK: project administration and funding acquisition. All authors have read and agreed to the published version of the manuscript.
Funding
This study was financed by the Slovenian Research Agency ARRS grants J4-7637 (awarded to IMM), J4-2542 (awarded to SSM), J4-9299 (awarded to AK), the ARRS National Research Program grant P4-0116 (coordinated by IMM), and bilateral grants USA-SI 2018/2019, BI-US/19-21-105, and BI-US/22-24-073 (awarded to SSM and AK).
Conflict of Interest
The authors declare that the research was conducted in the absence of any commercial or financial relationships that could be construed as a potential conflict of interest.
Publisher’s Note
All claims expressed in this article are solely those of the authors and do not necessarily represent those of their affiliated organizations, or those of the publisher, the editors and the reviewers. Any product that may be evaluated in this article, or claim that may be made by its manufacturer, is not guaranteed or endorsed by the publisher.
Acknowledgments
We thank the MSc student Katarina Krapež for technical support, and Melda Meral Ocal, Kritika Singh, Iris Yin, Changjun Xu, and Nada Pavlović for technical support.
Supplementary Material
The Supplementary Material for this article can be found online at: https://www.frontiersin.org/articles/10.3389/fmicb.2022.910616/full#supplementary-material
References
Aguiar, V. F., Donoghue, A. M., Arsi, K., Reyes-Herrera, I., Metcalf, J. H., de los Santos, F. S., et al. (2013). Targeting motility properties of bacteria in the development of probiotic cultures against Campylobacter jejuni in broiler chickens. Foodborne Pathog. Dis. 10, 435–441. doi: 10.1089/fpd.2012.1302
Alagawany, M., Abd El-Hack, M. E., Farag, M. R., Sachan, S., Karthik, K., and Dhama, K. (2018). The use of probiotics as eco-friendly alternatives for antibiotics in poultry nutrition. Environ. Sci. Pollut. Res. 25, 10611–10618. doi: 10.1007/s11356-018-1687-x
Arsi, K., Donoghue, A. M., Woo-Ming, A., Blore, P. J., and Donoghue, D. J. (2015). Intracloacal inoculation, an effective screening method for determining the efficacy of probiotic bacterial isolates against Campylobacter colonization in broiler chickens. J. Food Prot. 78, 209–213. doi: 10.4315/0362-028X.JFP-14-326
Barbosa, T. M., Serra, C. R., La Ragione, R. M., Woodward, M. J., and Henriques, A. O. (2005). Screening for bacillus isolates in the broiler gastrointestinal tract. Appl. Environ. Microbiol. 71, 968–978. doi: 10.1128/AEM.71.2.968-978.2005
Berndtson, E., Danielsson-Tham, M. L., and Engvall, A. (1996). Campylobacter incidence on a chicken farm and the spread of Campylobacter during the slaughter process. Int. J. Food Microbiol. 32, 35–47. doi: 10.1016/0168-1605(96)01102-6
Cartman, S. T., La Ragione, R. M., and Woodward, M. J. (2008). Bacillus subtilis spores germinate in the chicken gastrointestinal tract. Appl. Environ. Microbiol. 74, 5254–5258. doi: 10.1128/AEM.00580-08
Ciurescu, G., Dumitru, M., Gheorghe, A., Untea, A. E., and Drăghici, R. (2020). Effect of Bacillus subtilis on growth performance, bone mineralization, and bacterial population of broilers fed with different protein sources. Poult. Sci. 99, 5960–5971. doi: 10.1016/j.psj.2020.08.075
Dogan, O. B., Clark, J., Mattos, F., and Wang, B. (2019). A quantitative microbial risk assessment model of Campylobacter in broiler chickens: evaluating processing interventions. Food Control 100, 97–110. doi: 10.1016/j.foodcont.2019.01.003
Efremenkova, O. V., Gabrielyan, N. I., Malanicheva, I. A., Efimenko, T. A., Terekhova, L. P., Udalova, V. V., et al. (2016). Antibiotic activity of probiotic strain Bacillus subtilis 534 against clinical isolates of Acinetobacter baumannii. Antibiot. Khimioter. 61, 3–7.
EFSA (2012). Guidance on the assessment of bacterial susceptibility to antimicrobials of human and veterinary importance. EFSA J. 10:2740. doi: 10.2903/j.efsa.2012.2740
EFSA, and ECDC (2019). The European Union summary report on antimicrobial resistance in zoonotic and indicator bacteria from humans, animals and food in 2017. EFSA J. 17:5598. doi: 10.2903/j.efsa.2019.5598
EFSA and ECDC (2021a). The European Union One Health 2019 zoonoses report. EFSA J. 19:6406. doi: 10.2903/j.efsa.2021.6406
EFSA, and ECDC (2021b). The European Union summary report on antimicrobial resistance in zoonotic and indicator bacteria from humans, animals and food in 2018/2019. EFSA J. 19:6490. doi: 10.2903/j.efsa.2021.6490
El-Hack, M. E. A., El-Saadony, M. T., Shafi, M. E., Qattan, S. Y. A., Batiha, G. E., Khafaga, A. F., et al. (2020). Probiotics in poultry feed: a comprehensive review. J. Anim. Physiol. Anim. Nutr. 104, 1835–1850. doi: 10.1111/jpn.13454
Erega, A., Stefanic, P., Dogsa, I., Danevčič, T., Simunovic, K., Klančnik, A., et al. (2021). Bacillaene mediates the inhibitory effect of Bacillus subtilis on Campylobacter jejuni Biofilms. Appl. Environ. Microbiol. 87:e0295520. doi: 10.1128/AEM.02955-20
FEEDAP Panel on Additives and Products or Substances used in Animal Feed (2012). Guidance on the assessment of bacterial susceptibility to antimicrobials of human and veterinary importance. EFSA J. 10:2740.
Fritts, C. A., Kersey, J. H., Motl, M. A., Kroger, E. C., Yan, F., Si, J., et al. (2000). Bacillus subtilis C-3102 (Calsporin) improves live performance and microbiological status of broiler chickens. J. Appl. Poult. Res. 9, 149–155. doi: 10.1093/japr/9.2.149
Guyard-Nicodème, M., Keita, A., Quesne, S., Amelot, M., Poezevara, T., Le Berre, B., et al. (2016). Efficacy of feed additives against Campylobacter in live broilers during the entire rearing period. Poult. Sci. 95, 298–305. doi: 10.3382/ps/pev303
Hayashi, R. M., Lourenço, M. C., Kraieski, A. L., Araujo, R. B., Gonzalez-Esquerra, R., Leonardecz, E., et al. (2018). Effect of feeding Bacillus subtilis spores to broilers challenged with Salmonella enterica serovar Heidelberg Brazilian strain UFPR1 on performance, immune response, and gut health. Front. Vet. Sci 5:13 doi: 10.3389/fvets.2018.00013
Helms, M., Simonsen, J., Olsen, K. E. P., and Mølbak, K. (2005). Adverse health events associated with antimicrobial drug resistance in Campylobacter species: a registry-based cohort study. J. Infect. Dis. 191, 1050–1055. doi: 10.1086/428453
Hmani, H., Daoud, L., Jlidi, M., Jalleli, K., Ben Ali, M., Hadj Brahim, A., et al. (2017). A Bacillus subtilis strain as probiotic in poultry: selection based on in vitro functional properties and enzymatic potentialities. J. Ind. Microbiol. Biotechnol. 44, 1157–1166. doi: 10.1007/s10295-017-1944-x
Hong, H. A., Duc, L. H., and Cutting, S. M. (2005). The use of bacterial spore formers as probiotics. FEMS Microbiol. Rev. 29, 813–835. doi: 10.1016/j.femsre.2004.12.001
Huang, T., Geng, H., Miyyapuram, V. R., Sit, C. S., Vederas, J. C., and Nakano, M. M. (2009). Isolation of a variant of subtilosin A with hemolytic activity. J. Bacteriol. 191, 5690–5696. doi: 10.1128/JB.00541-09
Jayaraman, S., Thangavel, G., Kurian, H., Mani, R., Mukkalil, R., and Chirakkal, H. (2013). Bacillus subtilis PB6 improves intestinal health of broiler chickens challenged with Clostridium perfringens-induced necrotic enteritis. Poult. Sci. 92, 370–374. doi: 10.3382/ps.2012-02528
Jha, R., Das, R., Oak, S., and Mishra, P. (2020). Probiotics (direct-fed microbials) in poultry nutrition and their effects on nutrient utilization, growth and laying performance, and gut health: a Systematic Review. Animals 10:1863. doi: 10.3390/ani10101863
Koutsoumanis, K., Allende, A., Alvarez-Ordóñez, A., Bolton, D., Bover-Cid, S., Davies, R., et al. (2020). Update and review of control options for Campylobacter in broilers at primary production. EFSA J. 18:e06090. doi: 10.2903/j.efsa.2020.6090
Kovač, J., Stessl, B., Čadež, N., Gruntar, I., Cimerman, M., Stingl, K., et al. (2018). Population structure and attribution of human clinical Campylobacter jejuni isolates from central Europe to livestock and environmental sources. Zoonoses Public Health 65, 51–58. doi: 10.1111/zph.12366
Kuhn, K. G., Nygård, K. M., Guzman-Herrador, B., Sunde, L. S., Rimhanen-Finne, R., Trönnberg, L., et al. (2020). Campylobacter infections expected to increase due to climate change in Northern Europe. Sci. Rep. 10:13874. doi: 10.1038/s41598-020-70593-y
La Ragione, R. M., Casula, G., Cutting, S. M., and Woodward, M. J. (2001). Bacillus subtilis spores competitively exclude Escherichia coli O78:K80 in poultry. Vet. Microbiol. 79, 133–142. doi: 10.1016/s0378-1135(00)00350-3
La Ragione, R. M., and Woodward, M. J. (2003). Competitive exclusion by Bacillus subtilis spores of Salmonella enterica serotype Enteritidis and Clostridium perfringens in young chickens. Vet. Microbiol. 94, 245–256. doi: 10.1016/s0378-1135(03)00077-4
Latorre, J. D., Hernandez-Velasco, X., Kallapura, G., Menconi, A., Pumford, N. R., Morgan, M. J., et al. (2014). Evaluation of germination, distribution, and persistence of Bacillus subtilis spores through the gastrointestinal tract of chickens. Poult. Sci. 93, 1793–1800. doi: 10.3382/ps.2013-03809
Lutful Kabir, S. M. (2009). The role of probiotics in the poultry industry. Int. J. Mol. Sci. 10, 3531–3546. doi: 10.3390/ijms10083531
Meunier, M., Guyard-Nicodème, M., Dory, D., and Chemaly, M. (2016). Control strategies against Campylobacter at the poultry production level: biosecurity measures, feed additives and vaccination. J. Appl. Microbiol 120, 1139–1173. doi: 10.1111/jam.12986
Mingmongkolchai, S., and Panbangred, W. (2018). Bacillus probiotics: an alternative to antibiotics for livestock production. J. Appl. Microbiol. 124, 1334–1346. doi: 10.1111/jam.13690
Mohan, V. (2015). The role of probiotics in the inhibition of Campylobacter jejuni colonization and virulence attenuation. Eur. J. Clin. Microbiol. Infect. Dis. 34, 1503–1513. doi: 10.1007/s10096-015-2392-z
Mortada, M., Cosby, D. E., Shanmugasundaram, R., and Selvaraj, R. K. (2020). In vivo and in vitro assessment of commercial probiotic and organic acid feed additives in broilers challenged with Campylobacter coli. J. Appl. Poult. Res. 29, 435–446. doi: 10.1016/j.japr.2020.02.001
Oslizlo, A., Stefanic, P., Vatovec, S., Beigot Glaser, S., Rupnik, M., and Mandic Mulec, I. (2015). Exploring ComQXPA quorum-sensing diversity and biocontrol potential of Bacillus spp. isolates from tomato rhizoplane. Microb. Biotechnol. 8, 527–540. doi: 10.1111/1751-7915.12258
Pariza, M. W., Gillies, K. O., Kraak-Ripple, S. F., Leyer, G., and Smith, A. B. (2015). Determining the safety of microbial cultures for consumption by humans and animals. Regul. Toxicol. Pharmacol. 73, 164–171. doi: 10.1016/j.yrtph.2015.07.003
Park, J. H., Kim, Y. M., Kang, D. K., and Kim, I. H. (2017). Effect of Dietary Bacillus subtilis C14 and RX7 strains on growth performance, blood parameter, and intestinal microbiota in broiler chickens challenged with Salmonella gallinarum. J. Poult. Sci. 54, 236–241. doi: 10.2141/jpsa.0160078
Robyn, J., Rasschaert, G., Hermans, D., Pasmans, F., and Heyndrickx, M. (2013). In vivo broiler experiments to assess anti-Campylobacter jejuni activity of a live Enterococcus faecalis strain. Poult. Sci. 92, 265–271. doi: 10.3382/ps.2012-02712
Sahin, O., Kassem, I. I., Shen, Z., Lin, J., Rajashekara, G., and Zhang, Q. (2015). Campylobacter in poultry: ecology and potential interventions. Avian Dis. 59, 185–200. doi: 10.1637/11072-032315-Review
Saint-Cyr, M. J., Guyard-Nicodème, M., Messaoudi, S., Chemaly, M., Cappelier, J. M., Dousset, X., et al. (2016). Recent advances in screening of anti-Campylobacter activity in probiotics for use in poultry. Front. Microbiol 7:553. doi: 10.3389/fmicb.2016.00553
Sharma, P., Tomar, S. K., Goswami, P., Sangwan, V., and Singh, R. (2014). Antibiotic resistance among commercially available probiotics. Food Res. Int. 57, 176–195. doi: 10.1016/j.foodres.2014.01.025
Šimunović, K., Stefanic, P., Klančnik, A., Erega, A., Mandic-Mulec, I., and Smole Možina, S. S. (2022). Bacillus subtilis PS-216 antagonistic activities against Campylobacter jejuni NCTC 11168 are modulated by temperature, oxygen, and growth medium. Microorganisms 10:289. doi: 10.3390/microorganisms10020289
Štefanič, P., and Mandic Mulec, I. (2009). Social interactions and distribution of Bacillus subtilis pherotypes at microscale. J. Bacteriol. 191, 1756–1764. doi: 10.1128/JB.01290-08
Tam, C. C., and O’Brien, S. J. (2016). Economic cost of Campylobacter, norovirus and rotavirus disease in the United Kingdom. PLoS One 11:e0138526. doi: 10.1371/journal.pone.0138526
Tamehiro, N., Okamoto-Hosoya, Y., Okamoto, S., Ubukata, M., Hamada, M., Naganawa, H., et al. (2002). Bacilysocin, a novel phospholipid antibiotic produced by Bacillus subtilis 168. Antimicrob. Agents Chemother. 46, 315–320. doi: 10.1128/AAC.46.2.315-320.2002
van Wagenberg, C. P. A., van Horne, P. L. M., and van Asseldonk, M. A. P. M. (2020). Cost-effectiveness analysis of using probiotics, prebiotics, or synbiotics to control Campylobacter in broilers. Poult. Sci. 99, 4077–4084. doi: 10.1016/j.psj.2020.05.003
Warriner, K., and Waites, W. M. (1999). Enhanced sporulation in Bacillus subtilis grown on medium containing glucose:ribose. Lett. Appl. Microbiol. 29, 97–102. doi: 10.1046/j.1365-2672.1999.00593.x
Wine, E., Gareau, M. G., Johnson-Henry, K., and Sherman, P. M. (2009). Strain-specific probiotic (Lactobacillus helveticus) inhibition of Campylobacter jejuni invasion of human intestinal epithelial cells. FEMS Microbiol. Lett 300, 146–152. doi: 10.1111/j.1574-6968.2009.01781.x
Zheng, M., Zhang, R., Tian, X., Zhou, X., Pan, X., and Wong, A. (2017). Assessing the risk of probiotic detary supplements in the context of antibiotic resistance. Front. Microbiol 8:908 doi: 10.3389/fmicb.2017.00908
Keywords: Campylobacter jejuni, Bacillus subtilis, probiotic, alternative to antibiotics, spore-containing drinking water, broiler chicken
Citation: Šimunović K, Sahin O, Erega A, Štefanič P, Zhang Q, Mandic Mulec I, Smole Smole Možina S and Klančnikk A (2022) Bacillus subtilis PS-216 Spores Supplemented in Broiler Chicken Drinking Water Reduce Campylobacter jejuni Colonization and Increases Weight Gain. Front. Microbiol. 13:910616. doi: 10.3389/fmicb.2022.910616
Received: 01 April 2022; Accepted: 20 June 2022;
Published: 08 July 2022.
Edited by:
Octavio Luiz Franco, Catholic University of Brasilia (UCB), BrazilReviewed by:
Luyao Ma, University of California, Davis, United StatesMohamed K. Fakhr, University of Tulsa, United States
Copyright © 2022 Šimunović, Sahin, Erega, Štefanič, Zhang, Mandic Mulec, Smole Možina and Klančnik. This is an open-access article distributed under the terms of the Creative Commons Attribution License (CC BY). The use, distribution or reproduction in other forums is permitted, provided the original author(s) and the copyright owner(s) are credited and that the original publication in this journal is cited, in accordance with accepted academic practice. No use, distribution or reproduction is permitted which does not comply with these terms.
*Correspondence: Anja Klančnik, YW5qYS5rbGFuY25pa0BiZi51bmktbGouc2k=