- 1Department of Clinical Laboratory, The First Affiliated Hospital of Wenzhou Medical University, Wenzhou, China
- 2Key Laboratory of Clinical Laboratory Diagnosis and Translational Research of Zhejiang Province, Wenzhou, China
- 3Department of Medical Laboratory Science, School of Laboratory Medicine and Life Science, Wenzhou Medical University, Wenzhou, China
Colistin is used as the “last line of defense” against multidrug-resistant (MDR) Gram-negative bacteria (GNB). However, improper use of colistin may further lead to an increasing number of colistin-resistant (Col-R) strains worldwide, which greatly limits antibiotic treatment options. In this study, we investigated the antibacterial and antibiofilm activities of naringenin (NG) combined with colistin against Col-R GNB in vitro and in vivo. The checkerboard method and time-kill test showed that NG combined with colistin has better antibacterial activity (FICI < 0.5) compared with NG and colistin alone. Biofilm formation inhibition tests demonstrated that combining the two drugs could inhibit biofilm formation; scanning electron microscopy (SEM) confirmed that the combination of the two significantly reduces the number of cells in the biofilm compared with the drug alone. The in vivo experiment showed that the combination of NG and colistin can improve the survival rate of the Galleria mellonella (G. mellonella) and reduce the microbial load in the mouse thigh infection model. Mechanistically, the combination of NG and colistin synergistically enhances the antibacterial activity and changes the permeability of the bacterial outer membrane. More importantly, cytotoxicity tests showed no cell cytotoxicity of NG in combination with colistin. In conclusion, our data revealed that NG combined with colistin exhibited good synergistic effects in vivo and in vitro, thus providing a new therapeutic option for clinical Col-R GNB infections.
Introduction
Antibiotic resistance and the spread of multidrug-resistant (MDR) pathogens pose a serious threat to global health (El-Sayed Ahmed et al., 2020; Antimicrobial Resistance Collaborators, 2022). Colistin (polymyxin E) has re-emerged as a last-resort treatment option for bacterial infections, especially Gram-negative pathogens (Falagas and Kasiakou, 2005; Kaye et al., 2016). Yet, the improper use of colistin may further lead to an increasing number of colistin-resistant (Col-R) strains worldwide, which greatly limits antibiotic treatment options (Antoniadou et al., 2007; Liu et al., 2016). Over the years, several Col-R Gram-negative bacteria (GNB) have emerged worldwide and LPS, efflux pump, and PmrAB PhoPQ two-component system mutation have been considered the main factors regulating colistin resistance (Cai et al., 2012; Olaitan et al., 2014; Zhang et al., 2021a). A combination of natural compounds and antibiotics has been considered the main treatment option for Col-R GNB.
Colistin combined with fosfomycin, tigecycline, carbapenems, and rifampicin has shown a significant synergistic effect on treating GNB (Nordqvist et al., 2016; Abdelsalam et al., 2018; Wang et al., 2018a; Sato et al., 2021). Compared with synthetic antibiotics, phytochemicals have low toxicity and are not expensive. To date, resveratrol, eugenol, and astragalus, in combination with colistin, have been approved by the FDA for treating Col-R GNB (Cannatelli et al., 2018; Wang et al., 2018c; Zhou et al., 2018).
Biofilm formation is one of the main mechanisms through which bacteria develop antibiotic resistance. Biofilms are embedded in self-produced polymers such as polysaccharides, proteins, nucleic acids, and lipids, which maintain the stability of biofilms, mediate their adhesion to material surfaces, and help microorganisms colonize human organs or medical devices (Flemming and Wingender, 2010). In recent years, with the widespread use of antimicrobial drugs, nosocomial infections caused by biofilm-forming GNB, such as pneumonia, urinary tract infections, endocarditis, wound infections, and bacteremia, have substantially increased (Dijkshoorn et al., 2007; Prowle et al., 2011). Therefore, it is imperative to find effective drugs that can inhibit biofilms.
Recent studies have also shown that naringenin (NG), a natural flavonoid compound found in fruits and vegetables, may be used as a potential immunomodulator to treat sepsis, fulminant hepatitis, fibrosis, and cancer (Zeng et al., 2018). A recent study showed the synergistic effect of NG in combination with ceftazidime against ceftazidime-resistant Enterobacter cloacae (Eumkeb and Chukrathok, 2013). However, it remains unclear whether NG can be used in combination with colistin to treat Col-R GNB. In the present study, we evaluated the antibacterial and anti-biofilm effects of NG combined with colistin against GNB with different colistin resistance mechanisms and different strains, including Klebsiella pneumoniae, Escherichia coli, and Acinetobacter baumannii in vitro. In addition, we performed an in vivo experiment to further explore the synergistic effect of NG and colistin combination in the G. mellonella infection model and a mouse infection model.
Materials and methods
Bacterial isolates and growth conditions
In this study, 21 Gram-negative clinical Col-R isolates, including K. pneumoniae (n = 7), E. coli (n = 7), and A. baumannii (n = 7), were isolated from the First Affiliated Hospital of Wenzhou Medical University during 2015–2017. Strains were collected from different patients. Mechanisms of colistin resistance were identified by the experimental team (Chen et al., 2020; Zhang et al., 2021c). Identification of isolates was performed using the matrix-assisted laser desorption/ionization time-of-flight mass spectrometry (MALDI-TOF-MS, BioMerieux, France). Upon collection, isolates were snap-frozen in Luria Bertani (LB) (Thermo Fisher Scientific, United States) broth medium supplemented with 30% glycerol at −80°C for later use. Pseudomonas aeruginosa ATCC 27853 and E. coli ATCC 25922 were purchased as quality controls from the National Center for Clinical Laboratories (NCCL).
Antibiotics and solvent
Naringenin was purchased from MedChemExpress (MCE) Co., Ltd. (NJ, United States). The antibiotics used in this study, including amikacin, ceftazidime, cefepime, imipenem, ciprofloxacin, aminotransim, levofloxacin, gentamicin, tobramycin, and colistin, were purchased from Wenzhou Kangtai Biotechnology Co., Ltd., Zhejiang China.
Antimicrobial susceptibility testing
The minimum inhibitory concentration (MIC) of NG and commonly used antibiotics on 21 clinical GNB isolates was determined by the micro-broth dilution method with a simple modification (Andrews, 2001; Ogunniyi et al., 2017). A 96-well flat-bottomed microtitration plate with diluted antibiotics was prepared, and 100 μl of bacteria suspension were added to each well of the plate and incubated at 37°C for 16–20 h. Breakpoints for antibiotics were interpreted according to the CLSI guidelines. Each experiment was performed independently three times.
Checkerboard assays
In total, 21 Col-R GNB isolates were selected for the checkerboard assays, in which effects of twofold serial dilutions of drug positioned in a 12 × 8 matrix were tested (MacNair et al., 2018; Zhang et al., 2021c). Each drug was diluted in cation-adjusted Mueller-Hinton broth (CAMHB) to a range of MIC concentrations for each experimental strain. Single bacterial colonies were cultured overnight and then diluted to the 0.5 McFarland standard in sterile saline. Next, samples were diluted in CAMHB in a 1:100 ratio. The final bacterial concentration in each well was approximately 7.5 × 105 CFU/ml. The results were observed at 16–20 h at 37°C. The absorbance of the bacterial culture at 600 nm was measured with a microplate reader. All experiments were performed in triplicate with the average used for FIC calculations. The synergistic effect of NG in combination with colistin was assessed by using the fractional inhibition concentration index (FICI). The formula for calculating FICI is as follows: FICI = (MIC of drug A in combination/MIC of drug A alone) + (MIC of drug B in combination/MIC of drug B alone), where FICI ≤ 0.5 indicates synergism; FICI > 0.5 and ≤ 4 indicate irrelevant; and FICI > 4 was considered antagonistic (Fadwa et al., 2021).
Time-kill assay
The synergistic effect of NG and colistin was further assessed using a time-killing assay based on the published articles with simple modifications (Berard et al., 2016). Six Col-R isolates (including two K. pneumoniae, two E. coli, and two A. baumannii) were selected to investigate their synergistic effects. Tubes containing LB alone served as the negative control. Briefly, bacteria were exposed to NG and colistin alone or NG + colistin at 1 × 106 CFU/ml. The culture was shaken at 200 rpm at 37°C, and the number of bacteria was counted on LB agar plates at 0, 2, 4, 6, 12, and 24 h. The synergistic effect was defined as a reduction of 2 log10 CFU/ml (Fadwa et al., 2021). All studies were conducted in duplicate.
Propidium iodide staining
The propidium iodide (PI) staining method was used to determine cell membrane permeability with a few modifications (Liu et al., 2017). Exponential phase cells of K. pneumoniae FK1913 were treated with test drugs alone (colistin 2 μg/ml; NG 64 and 128 μg/ml) or in combination for 2 h and placed in PI (50 μg/ml) for 10 min at room temperature. A fluorescent microscope (Nikon, Japan) was used to investigate the fluorescence at 561 nm, and photographs were recorded.
Biofilm formation inhibition test
The effect of NG combined with colistin on biofilm formation was investigated by biofilm formation inhibition assays, with some minor modifications (Chen et al., 2021). Col-R K. pneumoniae (n = 3), E. coli (n = 3), and A. baumannii (n = 3) were selected for the experiment. First, to stimulate biofilm formation in bacteria, the starter cultures were diluted in 0.25% glucose-containing LB medium. Next, the strain was adjusted to 0.5 McFarland turbidity with sterile saline and then diluted to 1:100 in LB broth and transferred into 96-well plates. NG and colistin were added to the 96-well plates at final concentrations of 16–128 and 0.25–1 μg/ml, respectively. After incubation at 37°C for 24 h, the 96-well plates were washed three times with sterile phosphate-buffered saline (PBS) (Sigma-Aldrich, Milan, Italy) to remove planktonic bacteria. Next, the plates were dried at room temperature and incubated for 15 min at 37°C with 1% crystalline violet (Beijing Sun Biotechnology Co., Ltd., Beijing, China). The CVs were removed after staining, and the wells were washed three times with 1 × PBS. After that, 200 μl of ethanol-acetone were used to solubilize the bound CV (96:5 v/v), and the absorbance values taken at 595 nm were measured on an enzyme marker (Multiskan FC). All tests were performed in triplicate.
Scanning electron microscopy
The biofilm inhibitory effect of NG combined with colistin was further investigated by scanning electron microscopy (SEM) (Zhang et al., 2021b). Reference experiments were performed with simple modifications. Briefly, FK1913 was randomly selected as the experimental strain. Overnight cultures of FK1913 were adjusted to 0.5 McFarland by sterile saline. Round sterile coverslips were placed in six-well cell culture plates. Diluted cultures (100 μl) were aliquoted into each well of a six-well plate containing 1,900 μl NG (64 μg/ml) and colistin (2 μg/ml) alone or in a combination of the two and incubated for 18–24 h at 37°C in a constant temperature incubator. The culture solution was aspirated from the wells, and the planktonic bacteria were washed away with PBS. Then, coverslips were fixed containing 2.5% glutaraldehyde for 4 h at 4°C. The samples were dried at 37°C for 15 min and then observed by SEM (S-3000N, Japan).
In vivo evaluation of synergy in Galleria mellonella infection model
We investigated the in vivo efficacy of combination of NG and colistin by calculating the survival rate of the Galleria mellonella (G. mellonella) (Zhang et al., 2021c). As previously mentioned with simple modifications, K. pneumoniae (FK1913), E. coli (DC90), and A. baumannii (BM2349) were randomly selected as experimental strains. A single colony was selected and adjusted to 0.5 McFarland. FK1913 and DC90 were diluted to 1 × 106 CFU/ml, and BM2349 was diluted to 1 × 107 CFU/ml.
Insects weighing between 250 and 350 mg were chosen. Insects were then divided into four groups, namely, control group, NG monotherapy group, colistin monotherapy group, and combined drug treatment group. First, 10 μl of the bacterial solution was aspirated and injected into the left lower foot. The control group was only injected with a bacterial solution; the colistin single-drug group was injected with bacterial suspension and colistin (2, 4 μg/ml × 7); the NG group was injected with bacterial suspension and NG (16, 64 μg/ml × 7); the combination group was injected with the bacterial suspension and NG + colistin (2 + 64 μg/ml × 7, 4 + 64 μg/ml × 7, and 2 + 16 μg/ml × 7, respectively). Each group was injected with the bacterial suspension 2 h before antibiotic administration. The larvae were incubated at 37°C, and the survival rate was recorded for 7 days. When larvae do not respond to stimuli, they are considered to be dead. The survival rate of G. mellonella was analyzed by Kaplan-Meier analysis and log-rank test. All experiments were repeated three times.
In vivo evaluation of synergy in the mouse infection model
To further evaluate the in vivo efficacy of NG and colistin, the neutropenic mouse thigh infection model was established with slight modifications (Lin et al., 2021). Female ICR mice, 5–6 weeks old (Charles River, Hangzhou, China), were used. Mice were cared for in accordance with the China’s National Standards for Laboratory Animals (GB 14925–2010). All animal studies were approved by the Zhejiang Association for Science and Technology (ID: SYXK [Zhejiang] 2018-0017) and carried out according to the guideline of the Wenzhou Laboratory Animal Welfare and Ethics.
FK1913 was randomly selected as the experimental strain. Neutropenic (neutrophils ≤100/mm3) thigh infections were induced by intraperitoneal injection of cyclophosphamide (Yuanye Biotechnology Co., Ltd., Shanghai, China) at 4 days (150 mg/kg) and 1 day (100 mg/kg). Each mouse was injected with 100 μl of bacterial suspension (1.5 × 107 CFU/ml) in the posterior thigh muscle index. After 2 h of bacterial inoculation, colistin 7.5 mg/kg was injected intraperitoneally every 24 h, alone or in combination with NG (50 mg/kg every 24 h) (Jia et al., 2022). Mice were euthanized after 24 h of treatment. Bacterial load was quantified by CFU counts of post-thigh homogenates. Groups of three mice (six thigh infections) were included in each dosing regimen.
In vitro cytotoxicity assays
To investigate the safety of NG, we performed a cytotoxicity assay using RAW264.7 with a few modifications (Chen et al., 2021). Briefly, RAW264.7 cells were cultured in Dulbecco’s Modified Eagle Medium (DMEM) supplemented with 10% heat-inactivated fetal bovine serum (FBS) and incubated in a 5% CO2 incubator at 37°C until fusion. Trypsin treatment was used to harvest confluent cells. Three groups were set up in the experiment, namely, the control group, the NG group, and the combined group. First, a 100 μl cell suspension containing 1 × 105 cells was inoculated into a sterile 96-well plate. Then, 10 μl of different concentrations of NG (16, 32, 64, 128, 256, and 512 μg/ml) were added to the single-drug group. The combination group added 5 μl of NG and 5 μl of colistin (1 + 16 μg/ml, 1 + 32 μg/ml, 1 + 128 μg/ml, 2 + 64 μg/ml, 4 + 32 μg/ml, 4 + 64 μg/ml). The group without reagents was used as a control group. After that, the plate was incubated for 12 h. Next, 10 μl CCK-8 was added to each well and incubated for 1 h at room temperature in dark. Finally, the absorbance was recorded at 450 nm using a microplate reader.
Statistical analysis
The results were expressed as mean ± standard deviation. The significance was determined using Student’s t-test and log-rank test. For all analyses, *P < 0.05, **P < 0.01, and ***P < 0.001. Statistical analysis was performed using Prism 8.
Results
Antimicrobial susceptibility assay
The MICs of different isolates against common clinical antibiotics and NG are listed in Table 1. Most strains showed an MDR phenotype of 95.2% (20/21). The MICs of colistin against all strains were 4–64 μg/ml. The MICs of all strains for NG were > 512 μg/ml, which suggested that NG has no antibacterial activity against Col-R GNB.
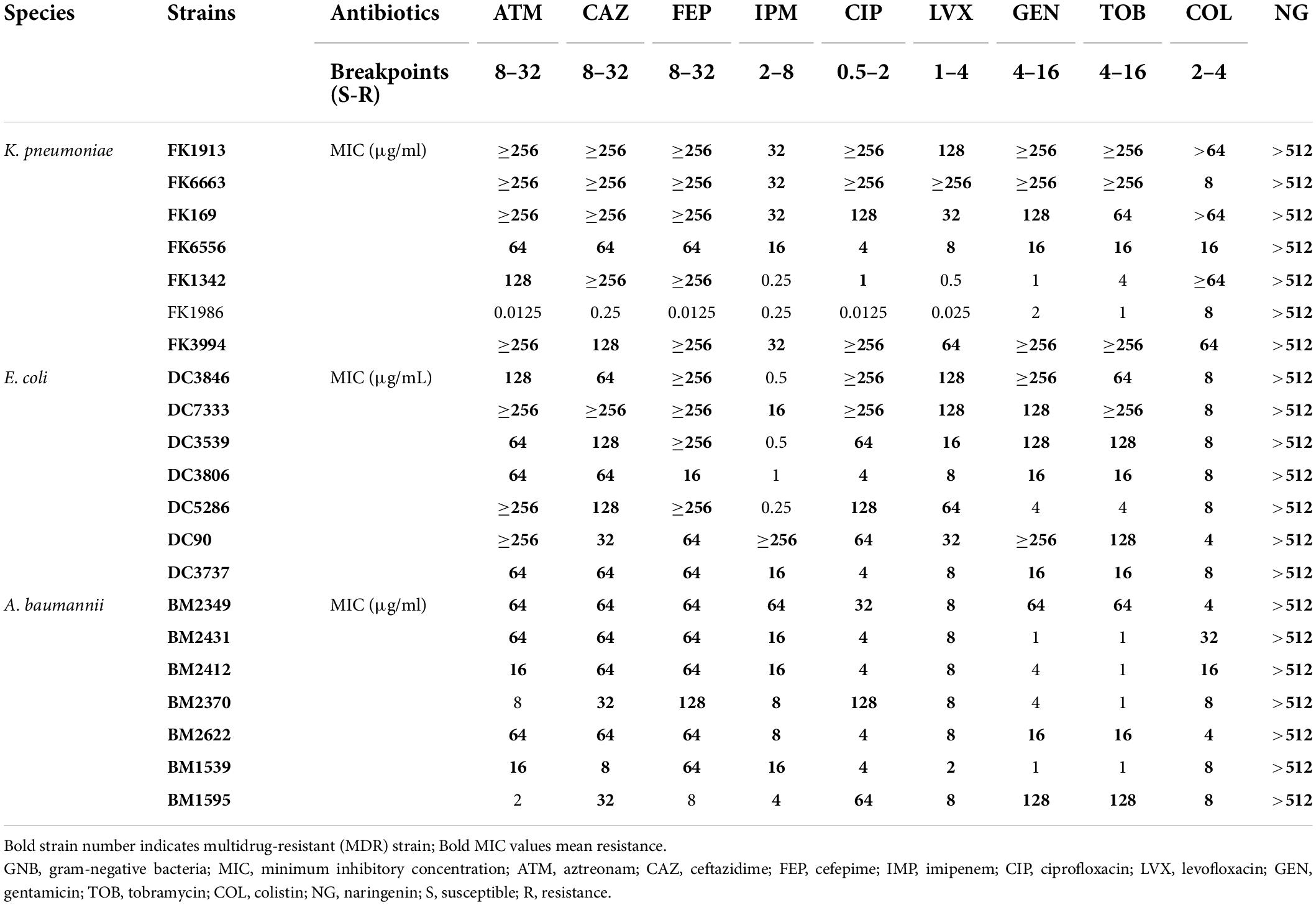
Table 1. Minimum inhibitory concentration (MIC) values of commonly used clinical antibiotics and NG against 21 colistin-resistant GNB.
Checkerboard assays
The MICs of colistin and NG were tested against a group of 21 strains of three different GNB. As shown in Table 2 and Supplementary Figure 1, the MIC of the Col-R strain was between 4 and 64 μg/ml. The MIC value of NG was >512 μg/ml. Mechanisms of colistin resistance were identified in these studied strains by the experimental team (Chen et al., 2020; Zhang et al., 2021c). The resistance mechanism of E. coli to colistin was attributed to mcr-1 mobile colistin resistance gene. K. pneumoniae mediated colistin resistance through substitution of MgrB, PmrB, and PhoQ. In addition, mcr-1 was also detected in four strains of K. pneumoniae. A. baumannii mediated colistin resistance by substituting LpxA, LpxC, LpxD, PmrB, and upregulation of the AdeABC/AdeIJK efflux pump. Checkerboard analysis showed that the combination of colistin and NG showed a significant synergistic effect on Col-R strains with different colistin resistance mechanisms (FICI ≤ 0.5), and the synergistic effect was independent of the resistance mechanism. In addition, MIC values of NG and colistin were significantly reduced when colistin was used in combination with NG. More importantly, the presence of NG resulted in the change of Col-R phenotype to colistin-sensitive phenotype in all strains.
Time−kill assay
As shown in Figure 1, the synergistic effect of NG and colistin on Col-R GNB was further analyzed using a time-kill curve. Drug concentrations were selected from results determined by the checkerboard method with FICI < 0.5. The experimental results showed that the monotherapy group had fewer time-dependent bactericidal activities than the control group. In addition, NG in combination with higher concentrations of colistin resulted in faster killing, and the strains treated with the combination exhibited a significant reduction in viable cells [more than >3log10 (CFU/ml)] compared with the control group. In summary, NG and colistin had very strong bactericidal activity when used together.
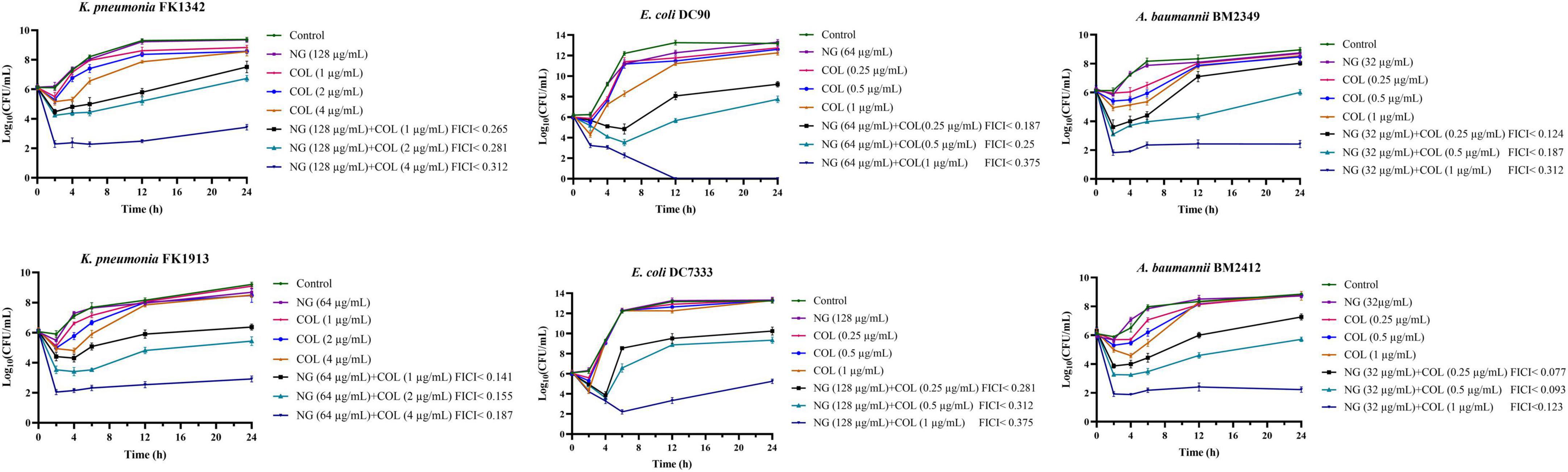
Figure 1. Colistin and naringenin time-killing curves against colistin-resistant Klebsiella pneumonia, colistin-resistant Escherichia coli, and colistin-resistant Acinetobacter baumannii when used alone or in combination. Naringenin (NG) and colistin (COL).
Mechanisms for drug synergy
We evaluated the cell membrane permeability of K. pneumoniae FK1913 using PI. As revealed by fluorescence microscopic analysis (Figure 2), after pre-incubating cells with colistin at 2 μg/ml, a minimal effect on cell membrane permeability was observed. However, pre-incubation of the cells with NG resulted in a concentration-dependent enhancement in fluorescence intensity due to PI uptake and DNA binding, which indicated that the integrity of the cell membrane gradually decreased.
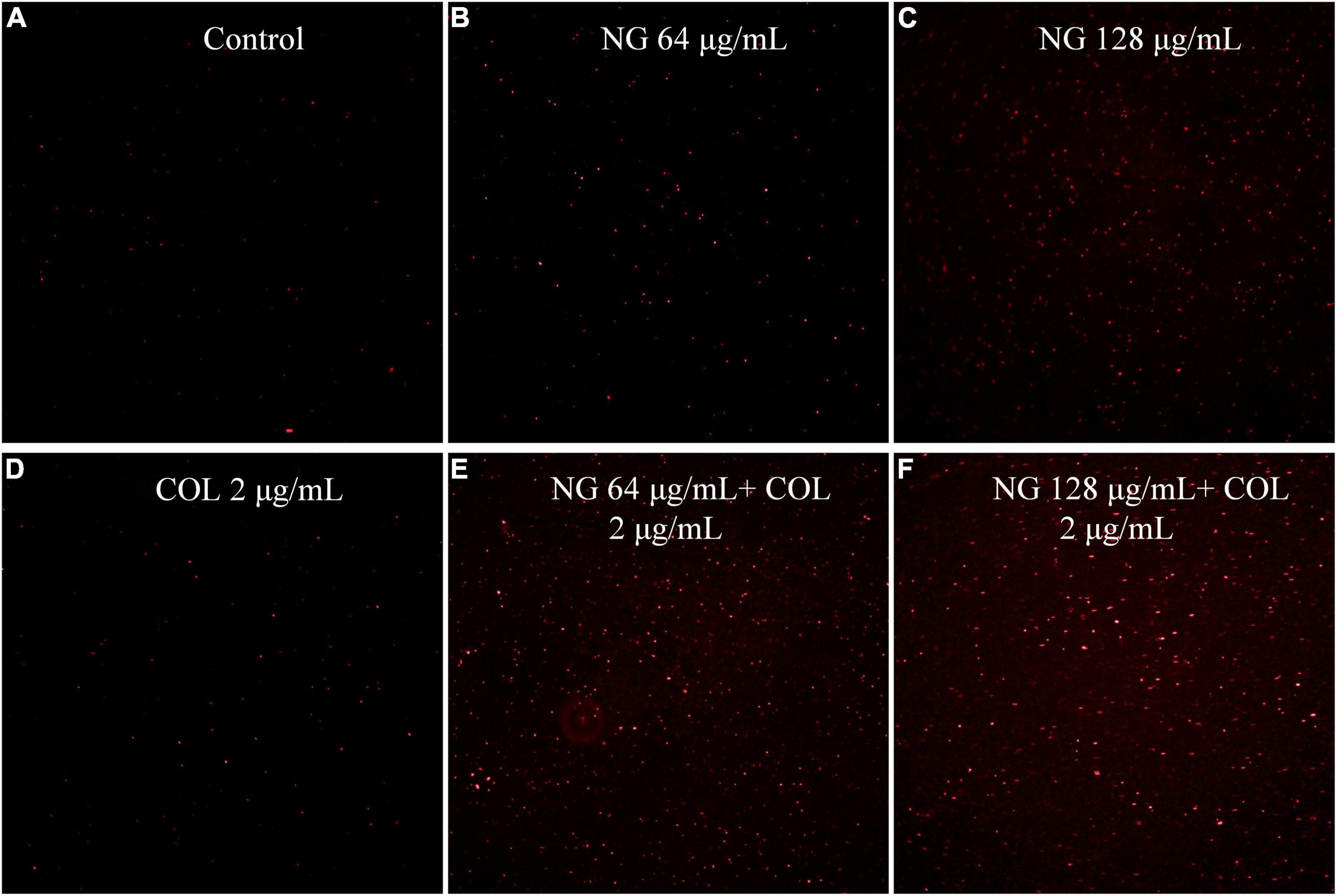
Figure 2. Fluorescence microscopy imaging of exponential-phase Klebsiella pneumonia FK1913, which were treated with naringenin and colistin alone or in combination, and incubated with 50 μg/ml PI for 10 min before imaging. (A) LB broth control; (B,C) cells treated with naringenin at 64, 128 μg/ml; (D) cells treated with colistin with 2 μg/ml; (E,F) cells exposed to a combination of naringenin and colistin. Naringenin (NG) and colistin (COL).
The antibiofilm activity of colistin/naringenin combinations
We investigated the ability of NG and colistin to inhibit biofilms when used alone or in combination. As shown in Figure 3, NG + colistin inhibited the biofilm of Col-R GNB compared with the blank control and NG or colistin alone (P < 0.05). The concentrations selected were derived from the synergistic concentrations of each strain in the checkerboard method.
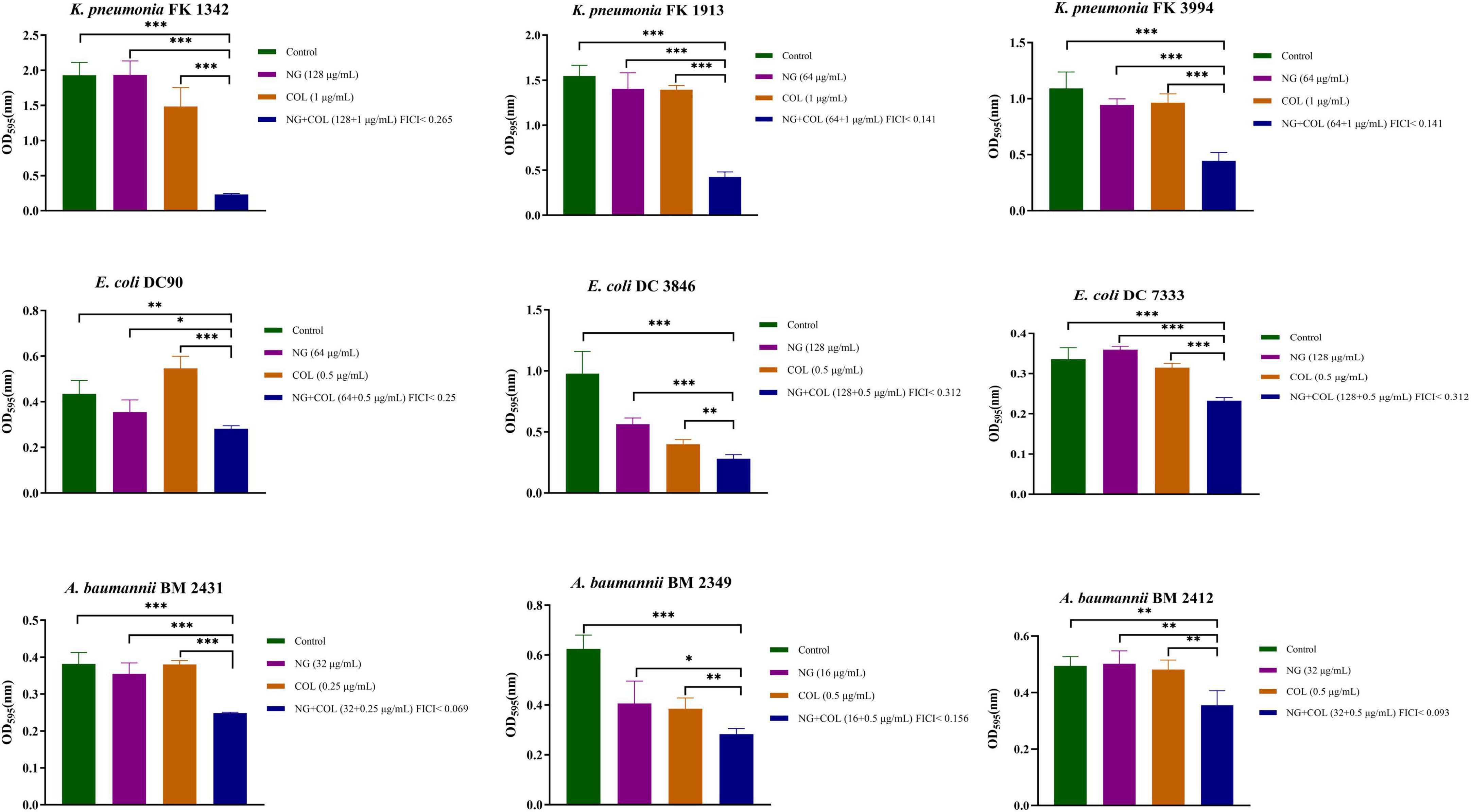
Figure 3. Biofilm inhibitory effects of colistin combined with naringenin on colistin-resistant Klebsiella pneumonia, colistin-resistant Escherichia coli, and colistin-resistant Acinetobacter baumannii. Drug concentration was chosen from the checkerboard method with FICI < 0.5. *P < 0.05, **P < 0.01, and ***P < 0.001 analyzed by Student’s t-test. Naringenin (NG) and colistin (COL).
Scanning electron microscopy
To further demonstrate the inhibitory effect of NG/colistin combination on biofilms, SEM experiments were performed. We selected a strain of Col-R K. pneumoniae FK1913 for observation. As shown in Figure 4, the results showed that untreated K. pneumoniae, treated with NG (64 μg/ml) and colistin (2 μg/ml) alone, exhibited a dense number of biofilms and complete morphology. However, the combination of NG and colistin greatly reduced the number of biofilms and formed a single colony. It is further proved that the combination of NG and colistin can inhibit the formation of biofilm.
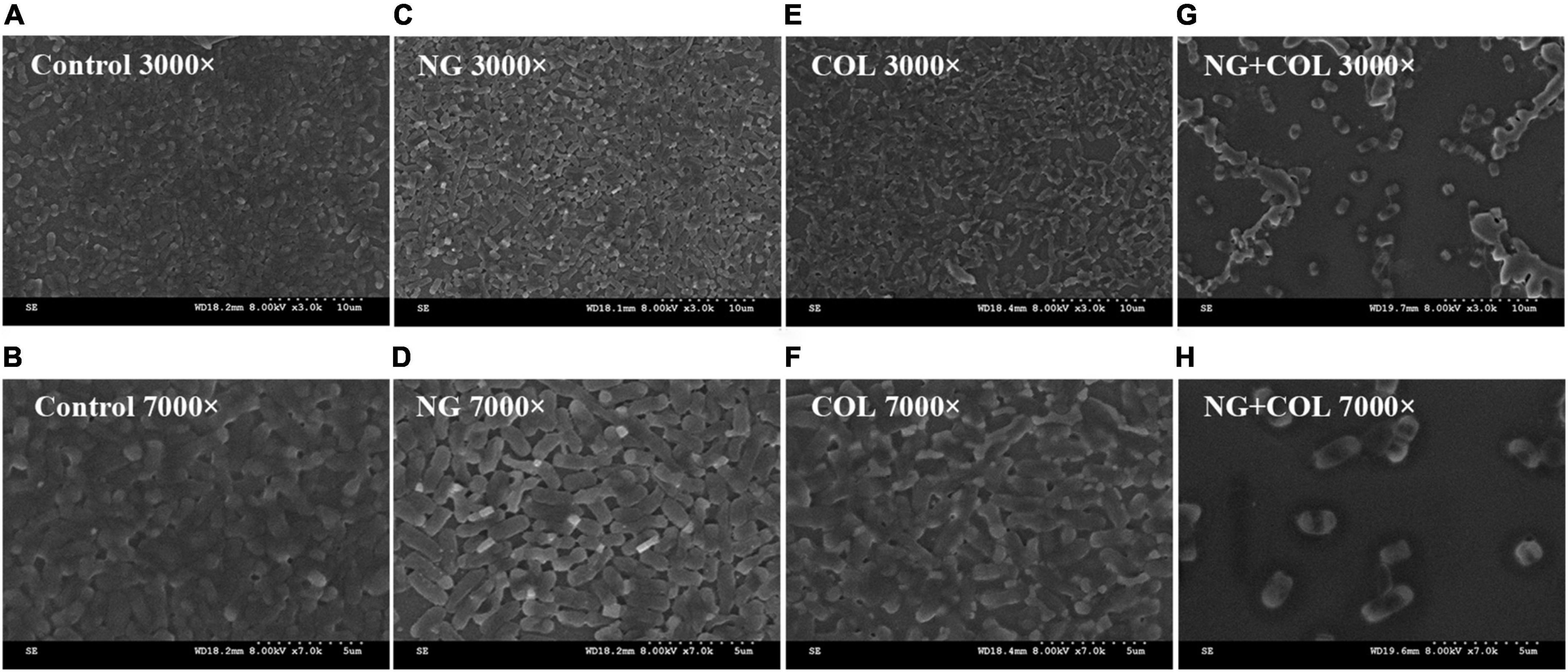
Figure 4. Scanning electron microscopy image. Klebsiella pneumonia FK1913 treated with 64 μg/ml naringenin (C,D), 2 μg/ml colistin (E,F), or combined treatment (G,H) for 2 h. (A,B) The control condition. Naringenin (NG) and colistin (COL).
In vivo study
To demonstrate the combined effect of NG and colistin in vivo, we conducted the G. mellonella test. We selected one strain of Col-R K. pneumoniae, E. coli, and A. baumannii for the experiment. As shown in Figure 5, almost all strains of G. mellonella injected with only the bacterial suspension died after 72 h. The survival rate of G. mellonella was significantly lower with monotherapy than with combination therapy (P < 0.05).

Figure 5. Survival rate of Galleria mellonella after 7 days of monotherapy or combination therapy against colistin-resistant Klebsiella pneumoniae FK1913, Escherichia coli DC90, and Acinetobacter baumannii BM2349. Naringenin (NG) and colistin (COL).
Meanwhile, the in vivo efficacy of the NG/colistin combination was further evaluated by a mouse thigh infection model. As shown in Figure 6, 50 mg/kg NG and 7.5 mg/kg colistin had a slight inhibition of K. pneumoniae FK1913. In addition, NG combined with colistin showed a higher efficacy for 24 h compared with NG and colistin alone (P < 0.05). In vivo experiments showed that the combination of the two drugs had a significant synergistic antibacterial effect.
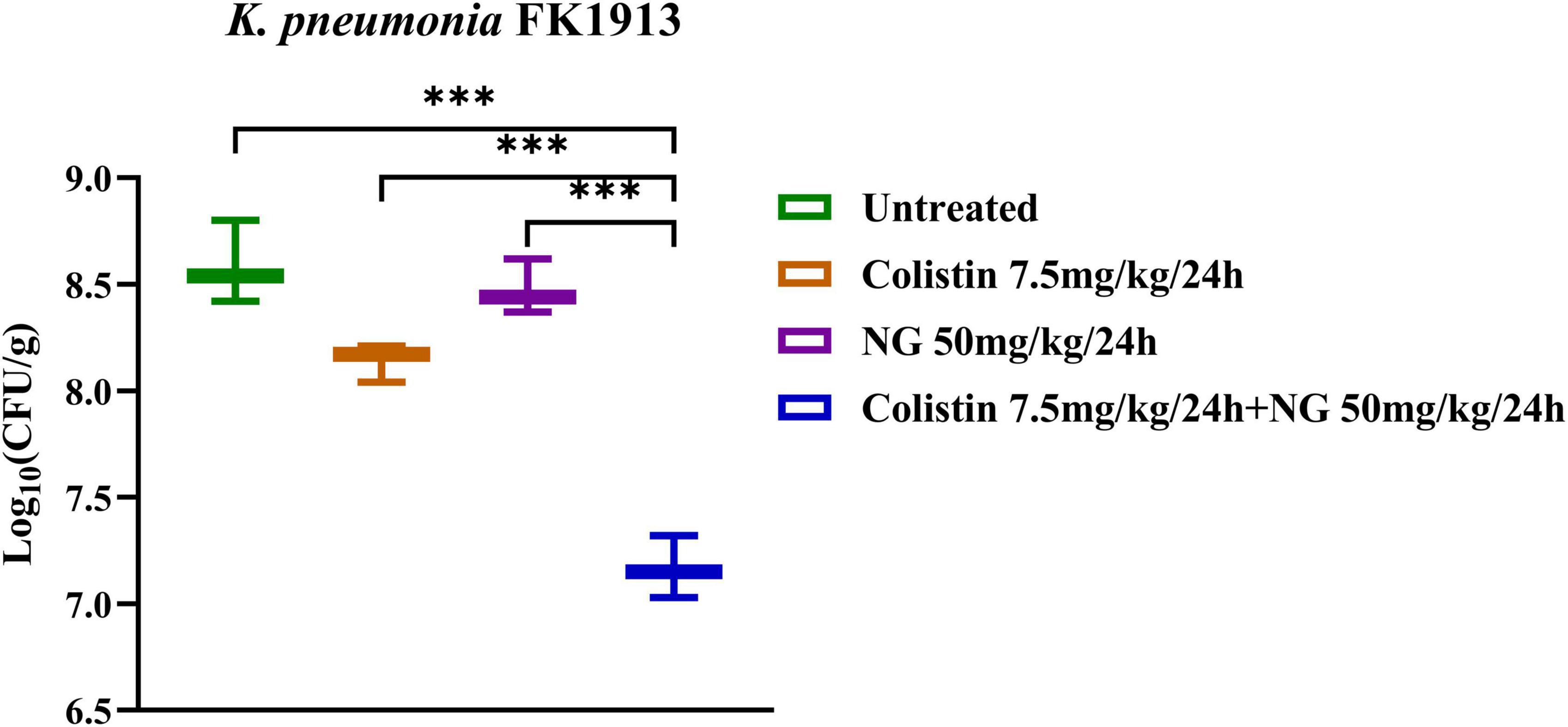
Figure 6. Changes in Log10 of thigh muscle (Δlog10 CFU/thigh) 24 h after monotherapy (naringenin 50 mg/kg, colistin 7.5 mg/kg) or combination therapy (50 mg/kg NG + 7.5 mg/kg colistin) for colistin-resistant Klebsiella pneumoniae FK1913. Naringenin (NG) and colistin (COL).
In vitro evaluation of cytotoxicity
To verify the safety of NG, we investigated the potential toxicity of NG alone and in combination on RAW264.7 cells. As depicted in Figure 7, the results indicated that NG concentration was 128 μg/ml without a toxic effect on RAW264.7 cells compared with the CCK-8 treated group. In addition, all concentrations of NG and colistin combined in this experiment showed no cytotoxic effects. This result demonstrates that the combined regimen can be used within a safe range in humans.
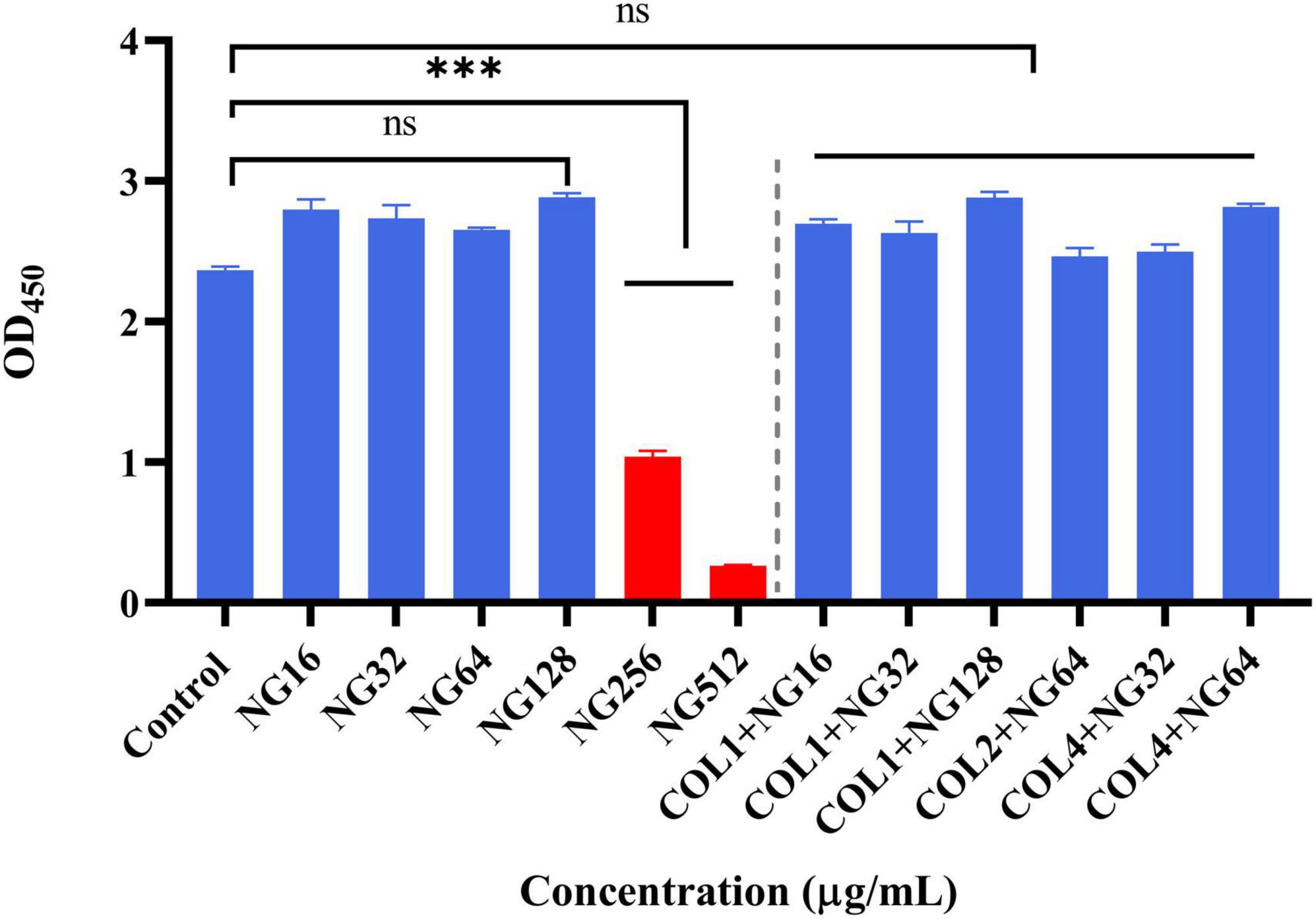
Figure 7. Cytotoxic effect of naringenin alone and in combination with different concentrations against the RAW 264.7 murine macrophage cell line (absorbance values at 450 nm). Data were analyzed by Student’s t-test; ns, not statistically significant; *P < 0.05, **P < 0.01, and ***P < 0.001. Naringenin (NG) and colistin (COL).
Discussion
In recent years, the massive and inappropriate use of broad-spectrum antibiotics has led to the emergence of MDR GNB (Rice, 2009). These drug-resistant pathogens pose a serious threat to the health and safety of hospitalized patients, as they are usually associated with a higher mortality rate (Brummett and Fox, 1989). Colistin has been used as a “last resort” to treat GNB infections. However, with its widespread use, the number of Col-R strains has significantly increased (Cannatelli et al., 2013; Olaitan et al., 2014). Therefore, developing and discovering new therapeutic means to treat these Col-R GNB strains remain crucial (Bush, 2010). To the best of our knowledge, this is the first study that reported how NG combined with colistin exhibited good synergistic effects in vivo and in vitro, thus providing a new therapeutic option for clinical Col-R GNB infections.
Global data indicate that colistin resistance is rising, especially with the discovery of mcr-1 plasmid found worldwide (Wang et al., 2018b). Previous studies have shown that colistin has significant neurotoxicity and nephrotoxicity and has been abandoned for use (Fiaccadori et al., 2016). Therefore, it is extremely important for us to restore the activation of colistin and reduce the dosage of colistin. In this study, we used the checkerboard method and found that most strains have an MDR phenotype of 95.2% (20/21), and that colistin shows different drug resistance mechanisms. The presence of NG significantly reduces the MIC value of colistin, which manifests as FICI < 0.5, and restores the colistin sensitivity of GNB with different colistin resistance mechanisms. The time-killing assay confirmed further that the combined use of NG and colistin reduces the number of bacteria compared with a single drug, confirming synergistic effect of NG and colistin on Col-R GNB.
In addition, the inhibition test of biofilm formation showed that the combination of NG and colistin could inhibit the biofilm formation of Col-R GNB. To further prove the combination of the two effects on biofilm formation, we randomly selected a Col-R GNB strain (FK1913) for SEM. The results showed no change in biofilm morphology when NG or colistin was used alone. However, the combination of colistin and NG significantly disrupted the formation of K. pneumoniae biofilm, and the number of bacteria was significantly reduced. In this study, NG alone did not significantly inhibit the biofilm of GNB. We speculate that this may be due to the concentration of NG used in this study, which was not the concentration required to inhibit GNB.
To investigate the effects of NG and colistin in vivo, we used the G. mellonella test and mouse thigh infection model. The results showed that NG combined with colistin could significantly improve the survival rate of G. mellonella. Notably, NG combined with colistin significantly reduced the number of bacteria in mice. NG has been reported to increase membrane permeability and change the morphology of Staphylococcus aureus (Wang et al., 2017). On this basis, we further explored the synergistic mechanism of NG and colistin by PI staining. The results showed that NG could overcome colistin resistance by increasing membrane permeability, which allows more colistin to cross cell membranes and act as an antibacterial agent.
More importantly, the safety of NG was further confirmed by cytotoxicity assay. The results showed that 128 μg/ml NG alone had no cytotoxicity on RAW264.7 cells, and none of the combination concentrations used in this study showed any cytotoxicity. G. mellonella test, the mouse thigh infection model, and cytotoxicity test proved that NG combined with colistin is safe and effective. The safety and pharmacokinetics of NG have also been reported, and clinical trials have demonstrated the safety of 900 mg NG (Rebello et al., 2020). Especially now that coronavirus disease 2019 (COVID-19) is still at a critical juncture, it has been demonstrated that NG may have a therapeutic effect on COVID-19 by inhibiting COVID-19 major protease, 3CLpro, and reducing ACE2 receptor activity (Tutunchi et al., 2020). This suggests that a combination of NG and colistin might be a feasible option for patients with both COVID-19 infection and Col-R GNB. The potential medical value of NG was verified. NG has been shown to reduce methotrexate-induced allocytopenia, which is both nephrogenic and hepatotoxic (Dhanisha et al., 2022).
In summary, our study indicates that NG combined with colistin has a significant synergistic effect on Col-R GNB with different colistin resistance mechanisms. Furthermore, this study highlights the potential for reusing colistin and restoring the activity that maintains the last line of colistin. More importantly, NG combined with colistin can reduce the dose of colistin, thereby reducing its side effects of colistin (neurotoxicity and nephrotoxicity), and still maintaining the maximum therapeutic effect. Therefore, this study has great significance for the limited use of colistin in treating Col-R GNB bacterial infection, providing a prospective option for future treatment.
Data availability statement
The raw data supporting the conclusions of this article will be made available by the authors, without undue reservation.
Ethics statement
All animal studies were approved by the Zhejiang Association for Science and Technology (ID: SYXK [Zhejiang] 2018-0017) and carried out according to the guideline of the Wenzhou Laboratory Animal Welfare and Ethics.
Author contributions
MX wrote the first draft of the manuscript. MX, ZY, YZ, and SS prepared the material and collected the data. YS, LF, and XZ analyzed the data. CZ, JC, and TZ supervised the research. All authors contributed to the revisions of the manuscript and approved the final manuscript.
Funding
This study was supported by the Major Projects of Wenzhou Science and Technology Bureau (ZY2019011) and the Science and Technology Project of Wenzhou (no. Y20170204).
Acknowledgments
We acknowledge The First Affiliated Hospital of Wenzhou Medical University for its help.
Conflict of interest
The authors declare that the research was conducted in the absence of any commercial or financial relationships that could be construed as a potential conflict of interest.
Publisher’s note
All claims expressed in this article are solely those of the authors and do not necessarily represent those of their affiliated organizations, or those of the publisher, the editors and the reviewers. Any product that may be evaluated in this article, or claim that may be made by its manufacturer, is not guaranteed or endorsed by the publisher.
Supplementary material
The Supplementary Material for this article can be found online at: https://www.frontiersin.org/articles/10.3389/fmicb.2022.916587/full#supplementary-material
Supplementary Figure 1 | Checkerboard analysis of the synergistic antibacterial activity of the combination of colistin and naringenin against colistin-resistant Klebsiella pneumonia, colistin-resistant Escherichia coli, and colistin-resistant Acinetobacter baumannii. The absorbance of the bacterial cultures at 600 nm is shown at 16–20 h incubation. The dark blue area represents higher cell density. Data represent the mean OD600 of three biological replicates. Naringenin (NG) and colistin (COL).
References
Abdelsalam, M. F. A., Abdalla, M. S., and El-Abhar, H. S. E. (2018). Prospective, comparative clinical study between high-dose colistin monotherapy and colistin-meropenem combination therapy for treatment of hospital-acquired pneumonia and ventilator-associated pneumonia caused by multidrug-resistant Klebsiella pneumoniae. J. Glob. Antimicrob. Resist. 15, 127–135. doi: 10.1016/j.jgar.2018.07.003
Andrews, J. M. (2001). Determination of minimum inhibitory concentrations. J. Antimicrob. Chemother. 48, (Suppl. 1), 5–16. doi: 10.1093/jac/48.suppl_1.5
Antimicrobial Resistance Collaborators (2022). Global burden of bacterial antimicrobial resistance in 2019: a systematic analysis. Lancet 399, 629–655. doi: 10.1016/S0140-6736(21)02724-0
Antoniadou, A., Kontopidou, F., Poulakou, G., Koratzanis, E., Galani, I., Papadomichelakis, E., et al. (2007). Colistin-resistant isolates of Klebsiella pneumoniae emerging in intensive care unit patients: first report of a multiclonal cluster. J. Antimicrob. Chemother. 59, 786–790. doi: 10.1093/jac/dkl562
Berard, X., Stecken, L., Pinaquy, J. B., Cazanave, C., Puges, M., Pereyre, S., et al. (2016). Comparison of the antimicrobial properties of silver impregnated vascular grafts with and without triclosan. Eur. J. Vasc. Endovasc. Surg. 51, 285–292. doi: 10.1016/j.ejvs.2015.10.016
Brummett, R. E., and Fox, K. E. (1989). Aminoglycoside-induced hearing loss in humans. Antimicrob. Agents Chemother. 33, 797–800. doi: 10.1128/AAC.33.6.797
Bush, K. (2010). Alarming beta-lactamase-mediated resistance in multidrug-resistant Enterobacteriaceae. Curr. Opin. Microbiol. 13, 558–564. doi: 10.1016/j.mib.2010.09.006
Cai, Y., Chai, D., Wang, R., Liang, B., and Bai, N. (2012). Colistin resistance of Acinetobacter baumannii: clinical reports, mechanisms and antimicrobial strategies. J. Antimicrob. Chemother. 67, 1607–1615. doi: 10.1093/jac/dks084
Cannatelli, A., D’Andrea, M. M., Giani, T., Di Pilato, V., Arena, F., Ambretti, S., et al. (2013). In vivo emergence of colistin resistance in Klebsiella pneumoniae producing KPC-type carbapenemases mediated by insertional inactivation of the PhoQ/PhoP mgrB regulator. Antimicrob. Agents Chemother. 57, 5521–5526. doi: 10.1128/AAC.01480-13
Cannatelli, A., Principato, S., Colavecchio, O. L., Pallecchi, L., and Rossolini, G. M. (2018). Synergistic activity of colistin in combination with resveratrol against colistin-resistant gram-negative pathogens. Front. Microbiol. 9:1808. doi: 10.3389/fmicb.2018.01808
Chen, L., Lin, J., Lu, H., Zhang, X., Wang, C., Liu, H., et al. (2020). Deciphering colistin heteroresistance in Acinetobacter baumannii clinical isolates from Wenzhou, China. J. Antibiot. 73, 463–470. doi: 10.1038/s41429-020-0289-2
Chen, L., Yu, K., Chen, L., Zheng, X., Huang, N., Lin, Y., et al. (2021). Synergistic activity and biofilm formation effect of colistin combined with PFK-158 against colistin-resistant gram-negative bacteria. Infect. Drug Resist. 14, 2143–2154. doi: 10.2147/IDR.S309912
Dhanisha, S. S., Drishya, S., Gangaraj, K. P., Rajesh, M. K., and Guruvayoorappan, C. (2022). Molecular docking studies of naringenin and its protective efficacy against methotrexate induced oxidative tissue injury. Anticancer Agents Med. Chem. 22, 169–180. doi: 10.2174/1871520621666210322102915
Dijkshoorn, L., Nemec, A., and Seifert, H. (2007). An increasing threat in hospitals: multidrug-resistant Acinetobacter baumannii. Nat. Rev. Microbiol. 5, 939–951. doi: 10.1038/nrmicro1789
El-Sayed Ahmed, M. A. E., Zhong, L. L., Shen, C., Yang, Y., Doi, Y., and Tian, G. B. (2020). Colistin and its role in the Era of antibiotic resistance: an extended review (2000-2019). Emerg. Microbes Infect. 9, 868–885. doi: 10.1080/22221751.2020.1754133
Eumkeb, G., and Chukrathok, S. (2013). Synergistic activity and mechanism of action of ceftazidime and apigenin combination against ceftazidime-resistant Enterobacter cloacae. Phytomedicine 20, 262–269. doi: 10.1016/j.phymed.2012.10.008
Fadwa, A. O., Alkoblan, D. K., Mateen, A., and Albarag, A. M. (2021). Synergistic effects of zinc oxide nanoparticles and various antibiotics combination against Pseudomonas aeruginosa clinically isolated bacterial strains. Saudi J. Biol. Sci. 28, 928–935. doi: 10.1016/j.sjbs.2020.09.064
Falagas, M. E., and Kasiakou, S. K. (2005). Colistin: the revival of polymyxins for the management of multidrug-resistant gram-negative bacterial infections. Clin. Infect. Dis. 40, 1333–1341. doi: 10.1086/429323
Fiaccadori, E., Antonucci, E., Morabito, S., d’Avolio, A., Maggiore, U., and Regolisti, G. (2016). Colistin use in patients with reduced kidney function. Am. J. Kidney Dis. 68, 296–306. doi: 10.1053/j.ajkd.2016.03.421
Flemming, H. C., and Wingender, J. (2010). The biofilm matrix. Nat. Rev. Microbiol. 8, 623–633. doi: 10.1038/nrmicro2415
Jia, Y., Zhang, L., Liu, Z., Mao, C., Ma, Z., Li, W., et al. (2022). Targeting macrophage TFEB-14-3-3 epsilon Interface by naringenin inhibits abdominal aortic aneurysm. Cell Discov. 8:21. doi: 10.1038/s41421-021-00363-1
Kaye, K. S., Pogue, J. M., Tran, T. B., Nation, R. L., and Li, J. (2016). Agents of last resort: polymyxin resistance. Infect. Dis. Clin. North Am. 30, 391–414. doi: 10.1016/j.idc.2016.02.005
Lin, Y., Zhang, Y., Liu, S., Ye, D., Chen, L., Huang, N., et al. (2021). Quercetin rejuvenates sensitization of colistin-resistant Escherichia coli and Klebsiella Pneumoniae clinical isolates to colistin. Front. Chem. 9:795150. doi: 10.3389/fchem.2021.795150
Liu, Y., Ding, S., Dietrich, R., Martlbauer, E., and Zhu, K. (2017). A biosurfactant-inspired heptapeptide with improved specificity to kill MRSA. Angew. Chem. Int. Ed Engl. 56, 1486–1490. doi: 10.1002/anie.201609277
Liu, Y. Y., Wang, Y., Walsh, T. R., Yi, L. X., Zhang, R., Spencer, J., et al. (2016). Emergence of plasmid-mediated colistin resistance mechanism MCR-1 in animals and human beings in China: a microbiological and molecular biological study. Lancet Infect. Dis. 16, 161–168. doi: 10.1016/S1473-3099(15)00424-7
MacNair, C. R., Stokes, J. M., Carfrae, L. A., Fiebig-Comyn, A. A., Coombes, B. K., Mulvey, M. R., et al. (2018). Overcoming mcr-1 mediated colistin resistance with colistin in combination with other antibiotics. Nat. Commun. 9:458. doi: 10.1038/s41467-018-02875-z
Nordqvist, H., Nilsson, L. E., and Claesson, C. (2016). Mutant prevention concentration of colistin alone and in combination with rifampicin for multidrug-resistant Acinetobacter baumannii. Eur. J. Clin. Microbiol. Infect. Dis. 35, 1845–1850. doi: 10.1007/s10096-016-2736-3
Ogunniyi, A. D., Khazandi, M., Stevens, A. J., Sims, S. K., Page, S. W., Garg, S., et al. (2017). Evaluation of robenidine analog NCL195 as a novel broad-spectrum antibacterial agent. PLoS One 12:e0183457. doi: 10.1371/journal.pone.0183457
Olaitan, A. O., Morand, S., and Rolain, J. M. (2014). Mechanisms of polymyxin resistance: acquired and intrinsic resistance in bacteria. Front. Microbiol. 5:643. doi: 10.3389/fmicb.2014.00643
Prowle, J. R., Echeverri, J. E., Ligabo, E. V., Sherry, N., Taori, G. C., Crozier, T. M., et al. (2011). Acquired bloodstream infection in the intensive care unit: incidence and attributable mortality. Crit. Care 15:R100. doi: 10.1186/cc10114
Rebello, C. J., Beyl, R. A., Lertora, J. J. L., Greenway, F. L., Ravussin, E., Ribnicky, D. M., et al. (2020). Safety and pharmacokinetics of naringenin: a randomized, controlled, single-ascending-dose clinical trial. Diabetes Obes. Metab. 22, 91–98. doi: 10.1111/dom.13868
Rice, L. B. (2009). The clinical consequences of antimicrobial resistance. Curr. Opin. Microbiol. 12, 476–481. doi: 10.1016/j.mib.2009.08.001
Sato, Y., Ubagai, T., Tansho-Nagakawa, S., Yoshino, Y., and Ono, Y. (2021). Effects of colistin and tigecycline on multidrug-resistant Acinetobacter baumannii biofilms: advantages and disadvantages of their combination. Sci. Rep. 11:11700. doi: 10.1038/s41598-021-90732-3
Tutunchi, H., Naeini, F., Ostadrahimi, A., and Hosseinzadeh-Attar, M. J. (2020). Naringenin, a flavanone with antiviral and anti-inflammatory effects: a promising treatment strategy against COVID-19. Phytother. Res. 34, 3137–3147. doi: 10.1002/ptr.6781
Wang, J., He, J. T., Bai, Y., Wang, R., and Cai, Y. (2018a). Synergistic activity of colistin/fosfomycin combination against carbapenemase-producing Klebsiella pneumoniae in an in vitro pharmacokinetic/pharmacodynamic model. Biomed Res. Int. 2018:5720417. doi: 10.1155/2018/5720417
Wang, Y. M., Kong, L. C., Liu, J., and Ma, H. X. (2018c). Synergistic effect of eugenol with Colistin against clinical isolated Colistin-resistant Escherichia coli strains. Antimicrob. Resist. Infect. Control 7:17. doi: 10.1186/s13756-018-0303-7
Wang, R., van Dorp, L., Shaw, L. P., Bradley, P., Wang, Q., Wang, X., et al. (2018b). The global distribution and spread of the mobilized colistin resistance gene mcr-1. Nat. Commun. 9:1179. doi: 10.1038/s41467-018-03205-z
Wang, L. H., Wang, M. S., Zeng, X. A., Xu, X. M., and Brennan, C. S. (2017). Membrane and genomic DNA dual-targeting of citrus flavonoid naringenin against Staphylococcus aureus. Integr. Biol. 9, 820–829. doi: 10.1039/c7ib00095b
Zeng, W., Jin, L., Zhang, F., Zhang, C., and Liang, W. (2018). Naringenin as a potential immunomodulator in therapeutics. Pharmacol. Res. 135, 122–126. doi: 10.1016/j.phrs.2018.08.002
Zhang, S., Abbas, M., Rehman, M. U., Wang, M., Jia, R., Chen, S., et al. (2021a). Updates on the global dissemination of colistin-resistant Escherichia coli: an emerging threat to public health. Sci. Total Environ. 799:149280. doi: 10.1016/j.scitotenv.2021.149280
Zhang, Y., Lin, Y., Zhang, X., Chen, L., Xu, C., Liu, S., et al. (2021c). Combining colistin with furanone C-30 rescues colistin resistance of gram-negative bacteria in vitro and in vivo. Microbiol. Spectr. 9:e0123121. doi: 10.1128/Spectrum.01231-21
Zhang, X., Zhao, Y., Feng, L., Xu, M., Ge, Y., Wang, L., et al. (2021b). Combined with mefloquine, resurrect colistin active in colistin-resistant Pseudomonas aeruginosa in vitro and in vivo. Front. Microbiol. 12:790220. doi: 10.3389/fmicb.2021.790220
Keywords: colistin resistance, synergistic effect, naringenin, biofilm, gram-negative bacteria
Citation: Xu M, Yao Z, Zhao Y, Shi S, Sun Y, Feng L, Zhou C, Zhang X, Cao J and Zhou T (2022) Naringenin restores colistin activation against colistin-resistant gram-negative bacteria in vitro and in vivo. Front. Microbiol. 13:916587. doi: 10.3389/fmicb.2022.916587
Received: 09 April 2022; Accepted: 04 July 2022;
Published: 03 August 2022.
Edited by:
Dinesh Mahajan, Translational Health Science and Technology Institute (THSTI), IndiaReviewed by:
Rasoul Mirzaei, Pasteur Institute of Iran (PII), IranChen Kaichao, City University of Hong Kong, Hong Kong SAR, China
Copyright © 2022 Xu, Yao, Zhao, Shi, Sun, Feng, Zhou, Zhang, Cao and Zhou. This is an open-access article distributed under the terms of the Creative Commons Attribution License (CC BY). The use, distribution or reproduction in other forums is permitted, provided the original author(s) and the copyright owner(s) are credited and that the original publication in this journal is cited, in accordance with accepted academic practice. No use, distribution or reproduction is permitted which does not comply with these terms.
*Correspondence: Jianming Cao, d3pjam1pbmdAMTYzLmNvbQ==; Tieli Zhou, d3l6dGxpQDE2My5jb20=