- Key Laboratory of Chemical Biology and Molecular Engineering of Ministry of Education, Institute of Biotechnology, Shanxi University, Taiyuan, China
Bacillus halotolerans DS5 was isolated and identified as a halophilic microbe according to 16S rRNA analysis and the physical and chemical indices of the strain. A new alkaline protease (designated as prot DS5) from Bacillus halotolerans DS5 was produced, purified, and characterized. After 12 h incubation in the medium with 1% dextrin, 0.5% NaCl, 2% soluble starch, and 1% yeast extract (pH 7.0), it could reach the maximum enzyme activity (279.74 U/ml). The prot DS5 was stable in the pH range of 6.0–12.0 and the temperature range of 40–60°C, with maximal hydrolytic activities at pH 9 and at 50°C. In the presence of Ca2+, Mn2+, Ba2+, Mg2+, and Fe3+, protease activity was enhanced. The prot DS5 was maintained highly stable in NaCl (up to 2.5 mol/L), reducing and oxidizing agents. The prot DS5 also exhibited compatibility in other detergent ingredients, such as non-ionic and anionic surfactants. These properties of prot DS5 make this enzyme suitable for various industrial applications (e.g., detergents and leather).
HIGHLIGHTS
- Bacillus halotolerans DS5 produces extracellular alkaline protease (prot DS5) and achieves the highest activity after 12 h incubation.
- Prot DS5 is a manganese ion-activated protease and exhibits stability to salt, reducing agents, and oxidizing agents.
- As a novel biological additive, prot DS5 has great potential in the detergent industry.
Introduction
Protease, an enzyme that catalyzes the hydrolysis of proteins, has become one of the most widely used enzyme preparations in the world (Nandan and Nampoothiri, 2020). It has an enormous range of applications in detergents, leather, textiles, dairy products, food, feather processing, and other industrial fields, as well as in the biopharmaceutical-cosmetics industry (Mechri et al., 2017). According to the position of the protease action site in the peptide chain, it can be preliminarily divided into exopeptidase and endopeptidase, and based on the catalytic mechanism, endopeptidases are divided into four subclasses, namely, serine proteases, aspartic proteases, cysteine proteases, and metalloproteases (Mothe and Sultanpuram, 2016). According to the optimum reaction pH of protease, it is divided into alkaline, neutral, and acidic proteases (Maruthiah et al., 2013). Alkaline protease is an enzyme that hydrolyzes protein peptide bonds under the condition of alkaline pH, and its optimum pH value is generally 9–11 (Mirzapour Kouhdasht et al., 2018). Many bacterial alkaline proteases used as detergents are commercially available, such as subtilisin Carlsberg, savinase, and subtilisin BPN (Gupta et al., 2002).
Microorganisms have become the main source of enzymes due to their advantages, such as simple culture methods, wide sources, and rapid reproduction. At present, Bacillus is a kind of bacteria that is widely used in microbial resources, because it can produce resistant spores and has strong survival adaptability. It has been widely used in agriculture, animal husbandry, industry, medicine, environmental protection, and other fields. To date, the main method for obtaining stable proteins is to screen microorganisms from extreme environmental conditions (Yang et al., 2014). Salt-tolerant bacteria, also known as facultative halophilic bacteria, can grow and reproduce in both salt and salt-free environments. They are a rich source of salt-tolerance genes and are also a resource guarantee for the study of the physiological mechanism of salt-tolerance and the isolation and application of salt-tolerance genes. The large-scale application of enzymes is limited by harsh industrial environments, such as high salt, high temperature, and strong acid/alkali. The enzymes produced by halophilic bacteria can maintain high activity and stability under high-salt conditions, so they have extremely high application potential (Karray et al., 2021).
The old name for Bacillus halotolerans is Brevibacterium halotolerans (Ben-Gad and Gerchman, 2017). Bacillus halotolerans is a rhizosphere-promoting bacterium with the ability to promote plant growth and enhance plant resistance to drought and salinity stress (Zhang et al., 2018). Bacillus halophila QTH8 isolated from the Cotinus coggygria rhizosphere soil against wheat crown rot (Li et al., 2022). Bacillus halotolerans strain MS50-18A was performed by Jaime Sagredo-Beltrán and co-workers (Sagredo-Beltrán et al., 2018), followed by the analysis of antifungal activity against root rot causal phytopathogens. Recently, a serine alkaline protease from Bacillus halotolerans CT2 (Dorra et al., 2018) has been purified and characterized. To date, alkaline proteases from Bacillus halotolerans have been characterized or explored for potential industrial applications, but the production of commercial enzymes from them was totally unsighted. The fermentation cycle, as well as the relatively low stability and catalytic activity under relevant operating conditions (i.e., high temperature, high pH, and high salt), often hinders the widespread application of enzymes from Bacillus halotolerans.
During the last few years, many extreme enzymes have been studied, including thermophilic (Dai et al., 2022), psychrophilic (Santiago et al., 2016), acidophilic (Parashar and Satyanarayana, 2018), alkalophilic (Ito et al., 1998), halophilic (Mokashe et al., 2018), radiophilic, and barophilic enzymes (Raddadi et al., 2015), but the number of extreme enzymes is currently insufficient to meet growing industrial demands (Mesbah, 2022). Halophilic microorganisms have natural advantages in protease production, and many new extreme enzymes can be developed.
In this context, we obtained a novel alkaline protease named prot DS5 from Bacillus halotolerans with fast growth rate and short fermentation period. Then, the main properties of protease including temperature and pH optima and stability, metal ion, salt, reducing agents, oxidizing agents, and surfactants have been determined. Moreover, the application of extracellular proteases produced by the isolate Bacillus halotolerans DS5 as a detergent additive in the washing industry was evaluated.
Materials and methods
Materials
The soil samples used for screening are from the vegetation surface rich in withered grass leaves in the mountainous area of Zuoquan, Shanxi Province, China (37°07′ north latitude, 113°37′ east longitude). Dextrin, casein, soluble starch, and borax were purchased from Sinopharm Reagent. Other reagents are analytical pure.
Screening of the protease-producing strain
In the preliminary screening, the soil samples are dispersed in a sterile petri dish and then placed at about 80°C for 24 h to kill most heat-labile bacteria. Then, 1.0 g of the heat-treated sample was weighed into 10 ml of sterile water to make a soil suspension, which was then serially diluted with sterile water to make six concentration gradients ranging from 10–1 to 10–6, with each dilution 100 μl of the suspension was evenly spread on the milk plate. After incubating for 12 h at 37°C, a clear transparent circle is formed around the colony-producing protease, and the diameter of the transparent circle/colony diameter (D/d) is measured.
The strains obtained from the preliminary screening were inoculated into the liquid enzyme-production medium and cultured in a constant temperature shaker at 37°C for 12 h. The medium was then centrifuged, and the activity of the supernatant was measured. The microbial strains with high enzyme activity were screened for subsequent analysis.
Identification of the protease-producing strain
The protease-producing strains were identified according to their biochemical and physiological characteristics; morphological, including gram staining, shape, motility, production of catalase, amylase, and hydrogen sulfide; carbohydrate fermentation patterns; and the growth ability at different salt concentrations (Zhao et al., 2016). The PCR amplification template was the genomic DNA. Universal primers for bacterial 16S rRNA amplification were 5′-AGAGTTTGATCCTGGCTCAG-3′ and 5′-TACCTTGTTACGACTT-3′. PCR program was 95°C for 30 s denaturation, 55°C for 30 s annealing, 72°C for 10 min extension, and 30 cycles (Hu et al., 2013). Its products were recovered using a Biomiga DNA Gel Purification Miniprep Kit (Biomiga, Hangzhou, China) and sequenced by Sangon Biotech (Shanghai, China). The sequence obtained by sequencing was aligned online using Blast in NCBI, and the neighbor-joining method in MEGA 6.0 was used to build a phylogenetic tree.
Production and purification of prot DS5
A single Bacillus halotolerans DS5 was inoculated into 5 ml LB medium and shaken overnight at 37°C and 200 rpm. The seed liquid was transferred to 300 ml of fermentation medium and fermented under the above conditions for about 24 h. During the incubation, samples were determined the enzyme activity and nucleic acid amount for every 2 h (OD260). Cell growth was monitored by the amount of nucleic acid according to reference (Prasetyo et al., 2011).
The secreted prot DS5 in the clear cell-free supernatant was precipitated using 75% (w/v) NH4SO2 precipitation. The precipitated protease was obtained by centrifugation at 13,000 rpm for 30 min at 4°C, resuspended in 50 mM Tris–HCl, 100 mM NaCl (pH 9.0), and centrifuged again. The supernatant was loaded onto a Sephadex G-25 desalting column equilibrated with the above buffer. Prot DS5 was concentrated using a 10 kDa MWCO membrane and applied onto a Superdex-75 column equilibrated with the same buffer. Each 2 ml fraction was collected and analyzed for the alkaline protease activity. Each step of purification was checked by 12% SDS-PAGE.
Protein mass spectrometry identification
The MALDI-TOF mass spectra were used to analyze peptide mass fingerprinting and MS/MS ion search. The excised protein bands are enzymolyzed and then put into the mass spectrometer for data collection. Peptidecalibstandard II was used for instrument calibration. The data acquisition mode of the primary mass spectrum adopts positive ion reflection mode and automatic data acquisition mode; 5–10 peaks with better signal intensity were selected from the primary mass spectrum peaks for secondary mass spectrometry analysis. The primary and secondary mass spectrometry data were integrated using the Bio Tools software. The Mascot software was used for mass spectrometry data search. The search database was NCBI, the species was the whole species, the cutting enzyme was pancreatin, and the Mascot score was higher than 55. The identification was considered successful.
Estimation of enzymatic activity
Protease assays were performed according to methods described by Carrie Cupp-Enyard with minor modifications (Hiraga et al., 2005). Briefly, 500 μl of enzyme solution and 500 μl of casein solution (10 mg/ml) were mixed and reacted for 10 min at 40°C; 1 ml of trichloroacetic acid (TCA; 0.4 mol/L) was added to stop the reactions. The absorbance measures 680 nm. The control was treated the same as above, except TCA needs to be added before the enzyme. Under standard conditions, the amount of enzyme required to produce 1 μg of tyrosine/min was defined as 1 unit of enzyme activity.
Enzyme characteristics
Effects of temperature and pH on prot DS5 activity
To determine the optimal temperature for prot DS5, the enzyme activity was measured at 20–80°C under standard experimental conditions. Prot DS5 was incubated at 50°C for various times (30–150 min) to measure protease activity to determine the stability of the enzyme at 50°C.
The optimum pH for prot DS5 was studied in the range of 4.0–12.0. Casein was prepared in different buffers, including citrate (pH 4.0–5.0), Na2HPO4-NaH2PO4 (pH 6.0–7.0), Tris–HCl (pH 8.0–9.0), and glycine-NaOH (pH 10.0–12.0).
The pH stability of prot DS5 was studied by the following. The protease (0.4 mg/ml) was mixed with different buffers (pH 6.0–12.0) and incubated for 2.5 h, and then, the enzyme activity was determined. Untreated prot DS5 from the above study served as a control (100%).
Effects of metal ions and chemical reagents on enzyme activity
Prot DS5 was preincubated for 1 h with the following metal ions at a final concentration of 1, 5, 10, and 15 mM, including Ca2+, Ba2+, Mg2+, Mn2+, Ni2+, Fe3+, Fe2+, and Cu2+, and the enzymatic activity was determined.
To study the influence of surfactants agent on prot DS5, the enzyme was preincubated with different chemical reagents, including dithiothreitol (DTT), phenylmethanesulfonyl fluoride (PMSF), ethylenediaminetetraacetic acid (EDTA), β-mercaptoethanol (β-ME), Tween-20, Tween-80, TritonX-114, TritonX-100, alcohol ethoxylate, hydrogen peroxide, sodium dodecyl sulfate (SDS), and cetyltrimethylammonium bromide (CTAB) for 1 h. Then, the enzymatic activity was determined. Prot DS5 activity determined without any additives was used as a control (100%).
Effect of salinity on prot DS5
The salinity stability of the enzyme was studied by preincubating the prot DS5 for 1 h under the 0.1 M, 0.5 M, 1.0 M, 1.5 M, 2.0 M, and 2.5 M NaCl. The absence of NaCl was used as a control.
Effect of different substrates on prot DS5
Gelatin, casein, skimmed milk, bovine hemoglobin, and BSA as substrates were prepared at 1% concentration. The enzymes were incubated at 40°C for 10 min in different substrates, and then, the activity was determined.
Determination of kinetic parameters
The prot DS5 activity from casein with different concentrations of 0.36–4.85 mmol/L was measured at 40°C and pH 10.5. Kinetic parameters, Km (Michaelis-Menten constant), Kcat (turnover number), Vmax (maximal velocity), and Kcat/Km (catalytic efficiency), are determined according to the Lineweaver-Burk plot. [E] is the protease concentration in the reaction mixture used for determining the Kcat value. The calculation formula is as follows: Kcat = Vmax/[E].
Decontamination ability analysis
The prot DS5 washing ability test was evaluated according to Jian Zhang et al. (2019). We use the following detergents: a (2 g Chinese national standard detergent), b (2 g Chinese national standard detergent + 1% PB92 alkaline protease), and c (2 g Chinese national standard detergent + 1% prot DS5). The test cloth was JB-02 protein test cloth (3 cm × 3 cm). The detergency of the detergent was evaluated according to the method of GB/T13174-2008 (China National Standard). All the assays were conducted in triplicates.
Statistical analysis
All the assays were carried out in triplicate, and data are presented as mean ± standard error using Student’s t-test.
Results
Screening and identification of DS5 strain
In total, 80 strains were screened from soil samples to produce transparent circles on milk plates and then rescreened with fermentation culture. There were 3 strains with higher enzyme activity (Supplementary Table 1), and the DS5 strain that produced the highest protease activity was selected for follow-up research.
The Bacillus halotolerans DS5 was characterized as Gram positive. The cells were short rod-shaped, individually arranged with flagella. The colony was milky white, opaque, rough and wrinkled, and round in shape and exhibits catalase and amylase activities (Figure 1). It was able to grow at 37°C and tolerate up to 10% (w/v) NaCl. In addition, it produced hydrogen sulfide. The fermentation curve showed that it could utilize carbon sources, such as glucose, sucrose, lactose, fructose, maltose, xylose, arabinose, Inositol, sorbitol, mannitol, citrate, and cellulose. The results of its taxonomical characterization are summarized in Table 1.
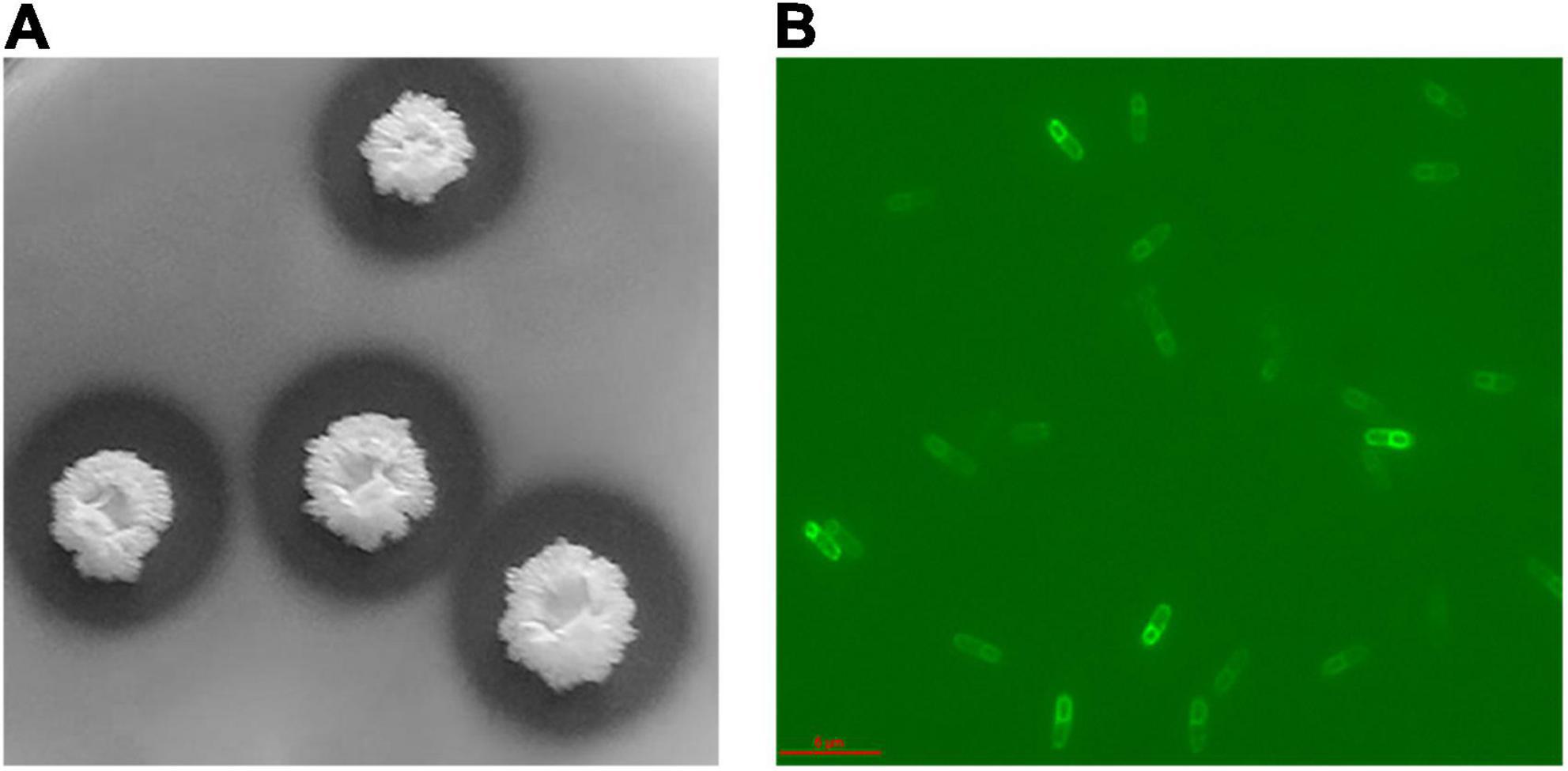
Figure 1. The morphological characteristics of Bacillus halotolerans DS5. (A) The hydrolyzed transparent circle of Bacillus halotolerans DS5; (B) Fluorescence microscope image of Bacillus halotolerans DS5.
Bacillus halotolerans DS5 was identified by 16S rRNA. As shown in Figure 2, phylogenetic tree analysis found that the 16S rRNA nucleotide sequence of this strain was 100% identical to Bacillus halotolerans strain ZB201702 (GeneBank: CP029364.1; Zhang et al., 2018).
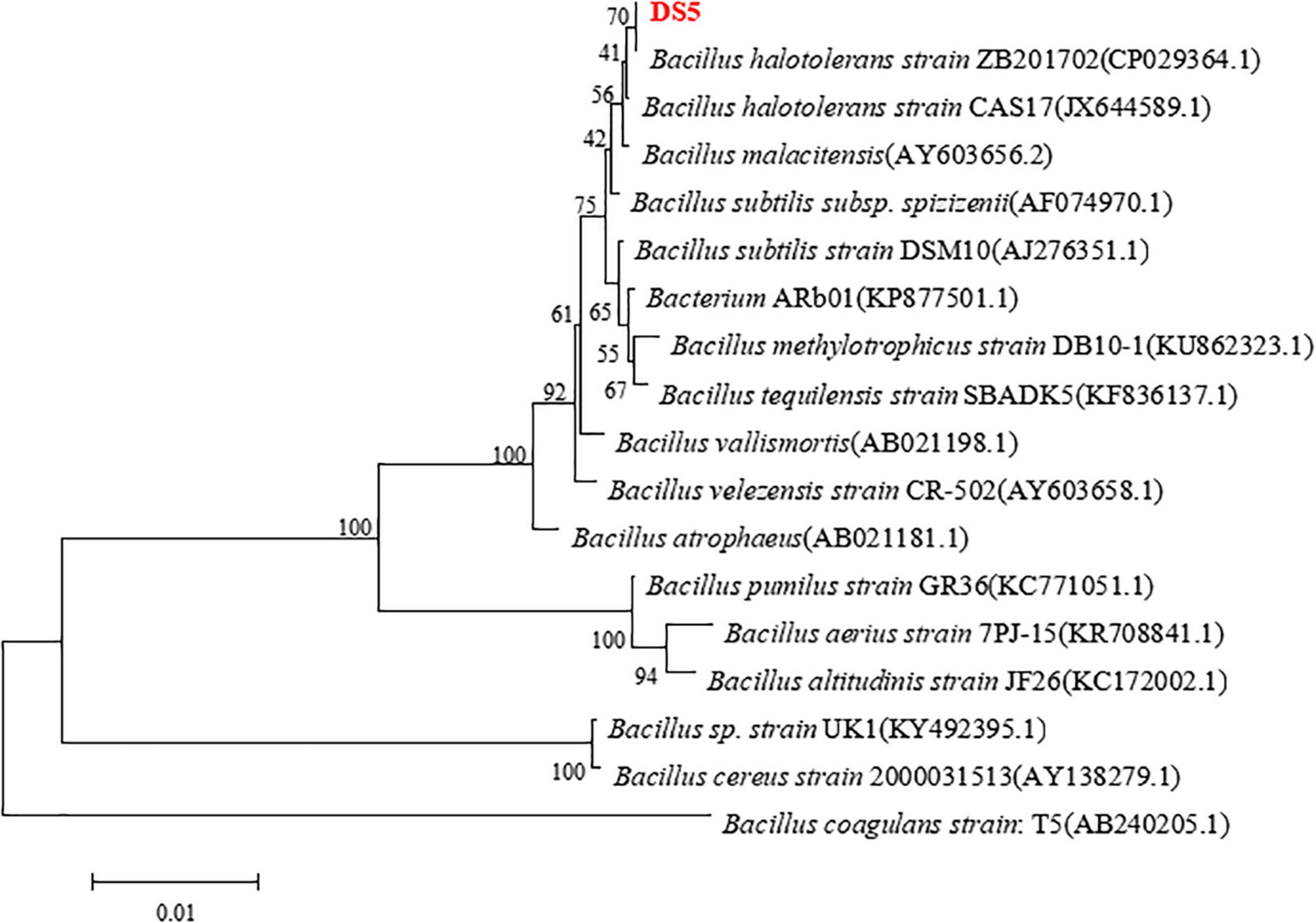
Figure 2. Phylogenetic tree analysis of Bacillus halotolerans DS5. Bootstrap values (expressed as percentages of 1,000 replications) are listed at branching points. The scale bar represented 1% divergence. The strains of each genome are as follows: Bacillus halotolerans strain ZB201702 (GenBank: CP029364.1), Bacillus halotolerans strain CAS17 (GenBank: JX644589.1), Bacillus malacitensis (GenBank: AY603656.2), Bacillus subtilis subsp. spizizenii (GenBank: AF074970.1), Bacillus subtilis strain DSM 10 (GenBank: AJ276351.1), Bacterium ARb01 (GenBank: KP877501.1), Bacillus methylotrophicus strain DB10-1 (GenBank: KU862323.1), Bacillus tequilensis strain SBADK5 (GenBank: KF836137.1), Bacillus vallismortis (GenBank: AB021198.1), Bacillus velezensis strain CR-502 (GenBank: AY603658.1), Bacillus atrophaeus (GenBank: AB021181.1), Bacillus pumilus strain GR36 (GenBank: KC771051.1), Bacillus aerius strain 7PJ-15 (GenBank: KR708841.1), Bacillus altitudinis strain JF26 (GenBank: KC172002.1), Bacillus sp. strain UK1 (GenBank:KY492395.1), Bacillus cereus strain 2000031513 (GenBank: AY138279.1), and Bacillus coagulans strain:T5 (GenBank: AB240205.1).
Production, purification, and identification of prot DS5
The cell growth and protease production of the DS5 strain after culture are shown in Figure 3. The enzyme production started at 4 h, and the enzyme production reached the highest at 12 h in a medium containing 1% dextrin, 0.5% NaCl, 2% soluble starch, and 1% yeast extract (pH 7.0). However, with the extension of fermentation time, the enzyme activity gradually decreased. Therefore, the optimal fermentation time for alkaline protease production was 12 h for Bacillus halotolerans DS5.
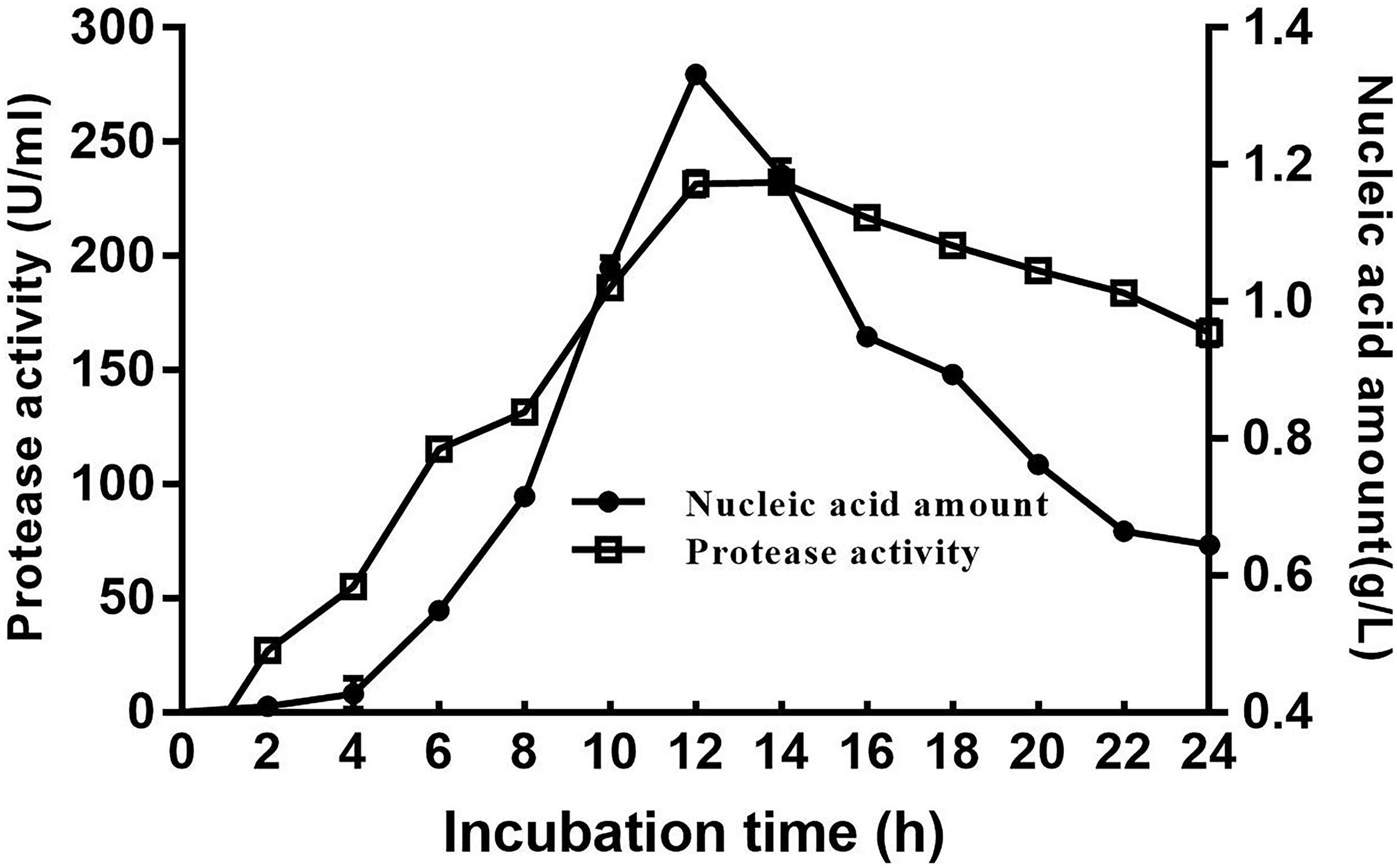
Figure 3. Time course of DS5 cell growth and protease production. Cell growth was detected by measuring the nucleic acid quantity at 260 nm. Each point represents the mean (n = 3) ± standard deviation.
The purification data and steps of prot DS5 are summarized in Table 2. After the crude enzyme solution was precipitated by ammonium sulfate and subjected to Superdex-75 gel chromatography, prot DS5 pure enzyme was obtained with a yield of 16% and a purification multiple of 5.6, and a specific activity of 1,501 U/mg. DS5 has successfully achieved homogeneity, and the molecular mass is approximately 27.0 kDa (Figure 4).
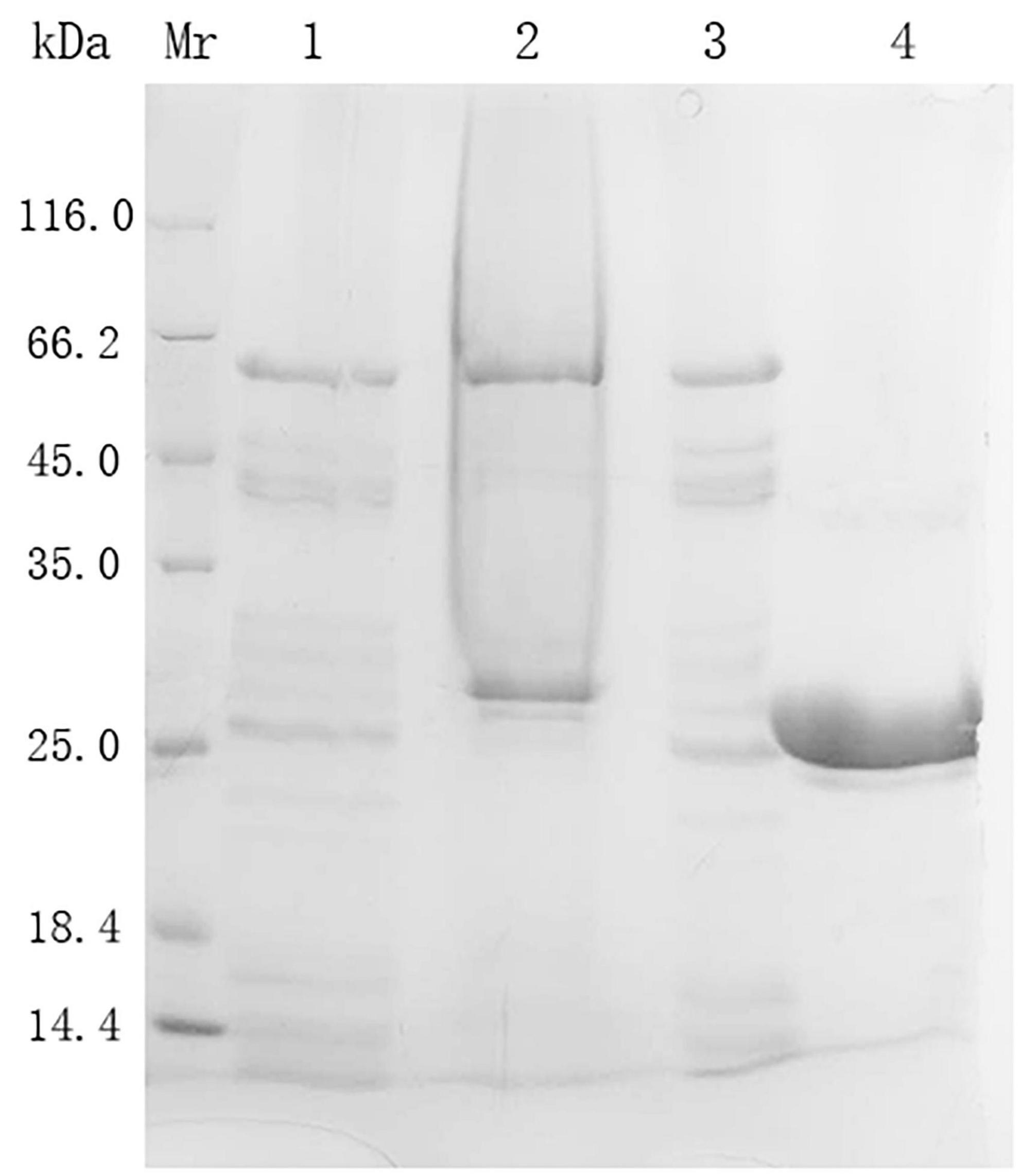
Figure 4. SDS-PAGE analysis of the expression and purification of prot DS5. Mr, protein marker; Lane 1, Cell-free supernatant; Lane 2, 70% (NH4)2SO4 precipitation sample; Lane 3, Sephadex G-25 desalted sample; Lane 4, sample after Superdex-75 gel chromatography.
The prot DS5 protein band was excised from a 12% SDS-PAGE gel and analyzed for protein mass spectra. As described in Supplementary Table 2, six major peptides were obtained by MALDI-TOF mass spectrometry. These peptides share 100% similarity to subtilisin E (39.0 kDa) from Bacillus subtilis, indicating that prot DS5 belongs to endopeptidase.
Enzyme characterization
Effect of temperature and pH on the activity and stability of prot DS5
Prot DS5 activity was measured at 20–80°C. Prot DS5 had the highest enzyme activity at 50°C (Figure 5A), and the optimal pH is 9.0 (Figure 5B). Prot DS5 was incubated at 50°C for 1 h, the enzyme activity can retain more than 90%, and the half-life is 135 min (Figure 5C). Alkaline proteases in the detergent industry are typically active at around 40–50°C (Mothe and Sultanpuram, 2016).
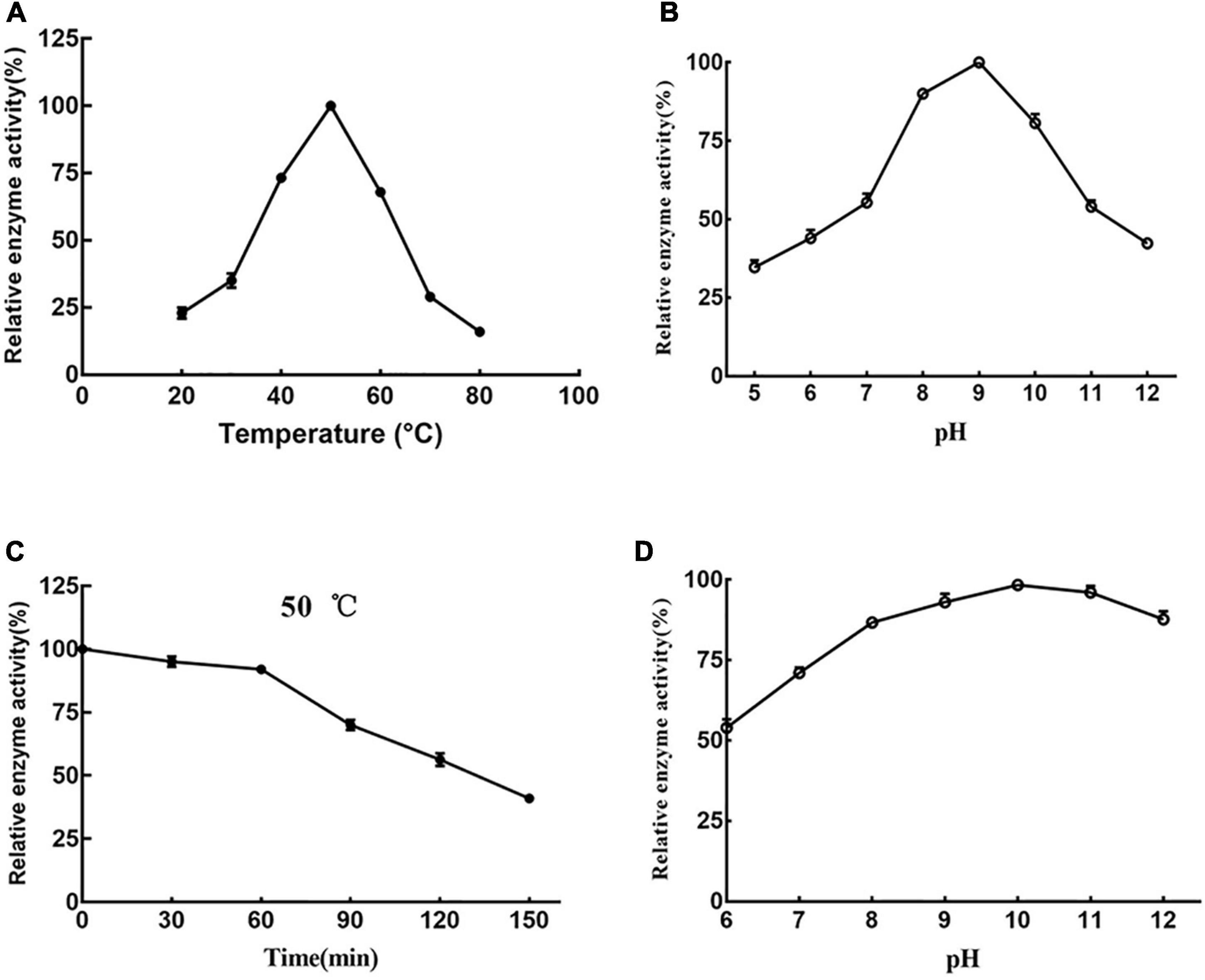
Figure 5. Effects of temperature and pH on prot DS5. Optimum reaction temperature (A) and pH (B) of the enzyme; (C) the stability of the enzyme at 50°C; (D) stability of the enzyme at different pH. Each point represents the mean (n = 3) ± standard deviation.
The prot DS5 was highly stable in the range of pH 7–12 (Figure 5D). We interestingly noticed that the enzyme activity of prot DS5 was basically maintained above 85% after 2.5 h of incubation in the pH range of 8–12. The optimal activity at higher pH and stability for a longer duration at alkaline pH make proteases highly attractive to the detergent industry, since laundry detergents generally operate at a pH of 7–11 (Ramkumar et al., 2018).
Effects of metal ions on the activity of prot DS5
The sensitivity of prot DS5 to metal ions was examined. Table 3 revealed that 1 mM of Ba2+, Mg2+, and Ni2+ ions slightly negatively influenced the enzyme activity, and Cu2+ severely inhibited the enzyme activity. However, Ca2+, Ba2+, Mg2+, Ni2+, Ca2+, Fe2+, Mn2+, and Fe3+ ions at 5 mM improved prot DS5 activity, except for Cu2+. The most obvious metal ions were 10 mM Ca2+ and 15 mM Mn2+ ions, which increased the protease activity by 26 and 86.42%, respectively.
Effects of chemical reagents on the activity of prot DS5
As shown in Table 4, non-ionic surfactants (e.g., 1% AEO, 1% Tween-80, 1% Triton X-114, and 1% Tween-20) have almost no effect on the prot DS5. Both anionic surfactant 0.1% SDS and 0.1% cationic surfactant cetyltrimethylammonium bromide (CTAB) have a severe inhibitory effect on enzyme activity. When the concentration is 0.5% for SDS and CTAB, the enzyme activity drops to under 10%. However, the enzyme activity remained above 85.76% at the concentration of 1% H2O2. In the presence of reducing agents DTT and β-mercaptoethanol, the activity of the enzyme increased or remained basically unchanged, indicating that the enzyme had strong tolerance to oxidants and reducing agents. On the contrary, the protease activity of prot DS5 was inhibited by PMSF and EDTA. Our protease activity was significantly inhibited by PMSF, suggesting that it might be a serine-type protease. The inhibitory effect of EDTA on the enzyme increased with the increase of EDTA concentration. The inhibitory effect of EDTA on the enzyme indicated that the enzyme could obtain the best activity only with the participation of metal ions (like Mn2+).
Salt tolerance analysis of prot DS5
The effect of NaCl concentration on enzyme activity was shown in Figure 6. When the NaCl concentration was 0–2.5 M, the enzyme activity remained basically unchanged. When the salt concentration reached 2.5 M, prot DS5 maintained a relative enzyme activity of 97.5%. This enzyme may have a protective mechanism corresponding to the high concentration of NaCl environment, so that it can still maintain a high enzyme activity under high salt conditions. The above result indicates that the prot DS5 can keep its properties stable in a high-concentration salt environment and has strong salt tolerance. The salt tolerance mechanism of prot DS5 may be related to secondary structure ratio, salt bridge, and hydrophobicity. Its strong salt tolerance can solve the problem of protease inactivation during the processing of some high-salt foods.
Prot DS5 substrate specificity
In all tested substrates (gelatin, casein, skim milk, BSA, and bovine hemoglobin), the enzyme hydrolyzes almost all the above substrates, which also makes it an excellent property for a detergent additive. From the results of substrate specificity detection (Figure 7), it could be seen that the optimal substrate of prot DS5 was casein. Its hydrolysis efficiency is approximately 10-fold that of the substrate BSA.
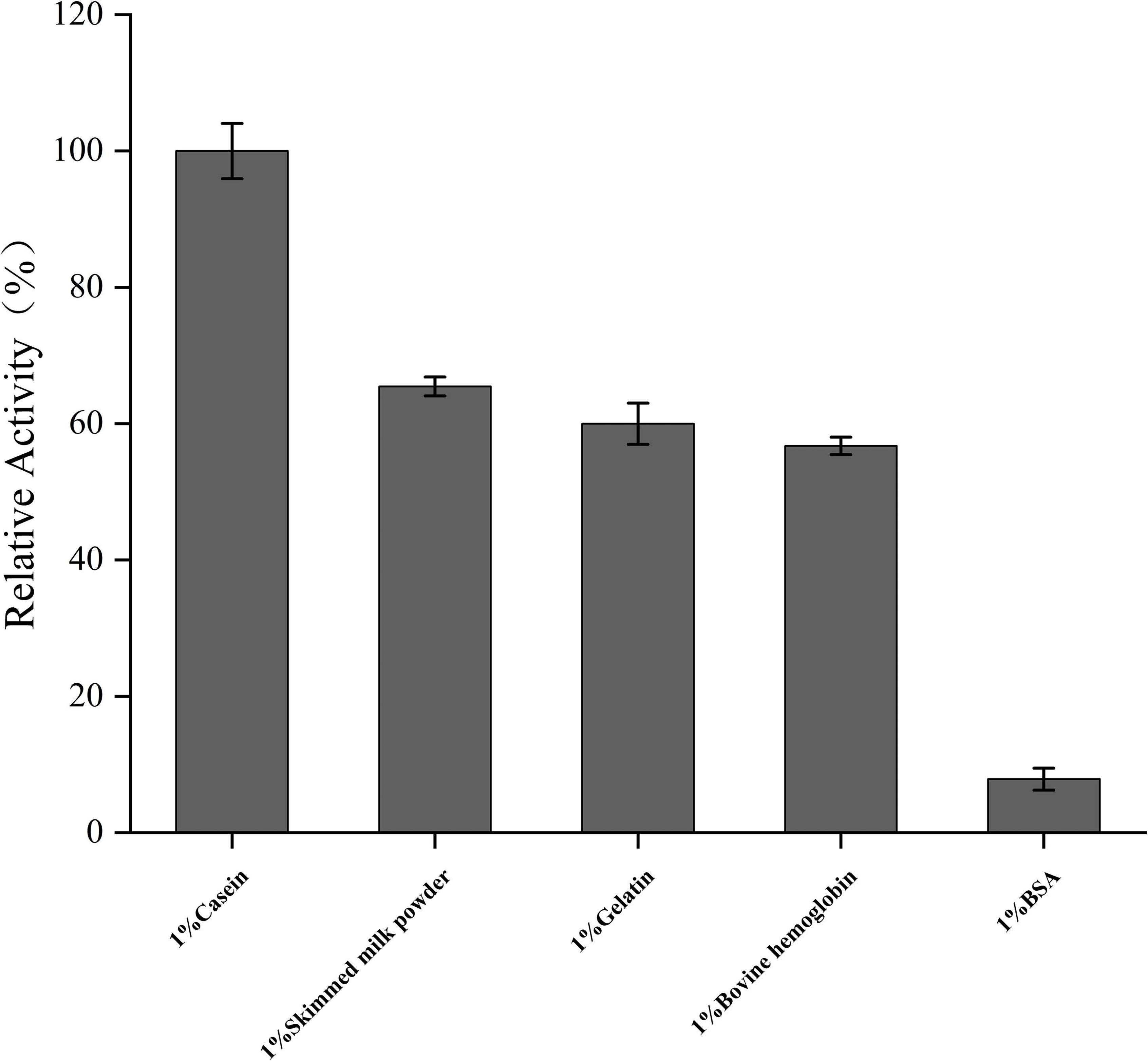
Figure 7. Analysis of substrate-dependent protease activity. Mean values are from three independent experiments.
Enzyme reaction kinetic parameters
The determination of prot DS5 kinetic parameters was studied. Using Lineweaver-Burk plot for visualization, we combined the equations generated from the curve fitting in Figure 8 with the computational rules of the Michaelis-Menten equation kinetics. Vmax, Km, Kcat, and Kcat/Km calculated for the purified enzyme were 2.644 μmol/min, 6.26 mmol/L, 2.3692/min, and 0.378 mmol/L/min, respectively.
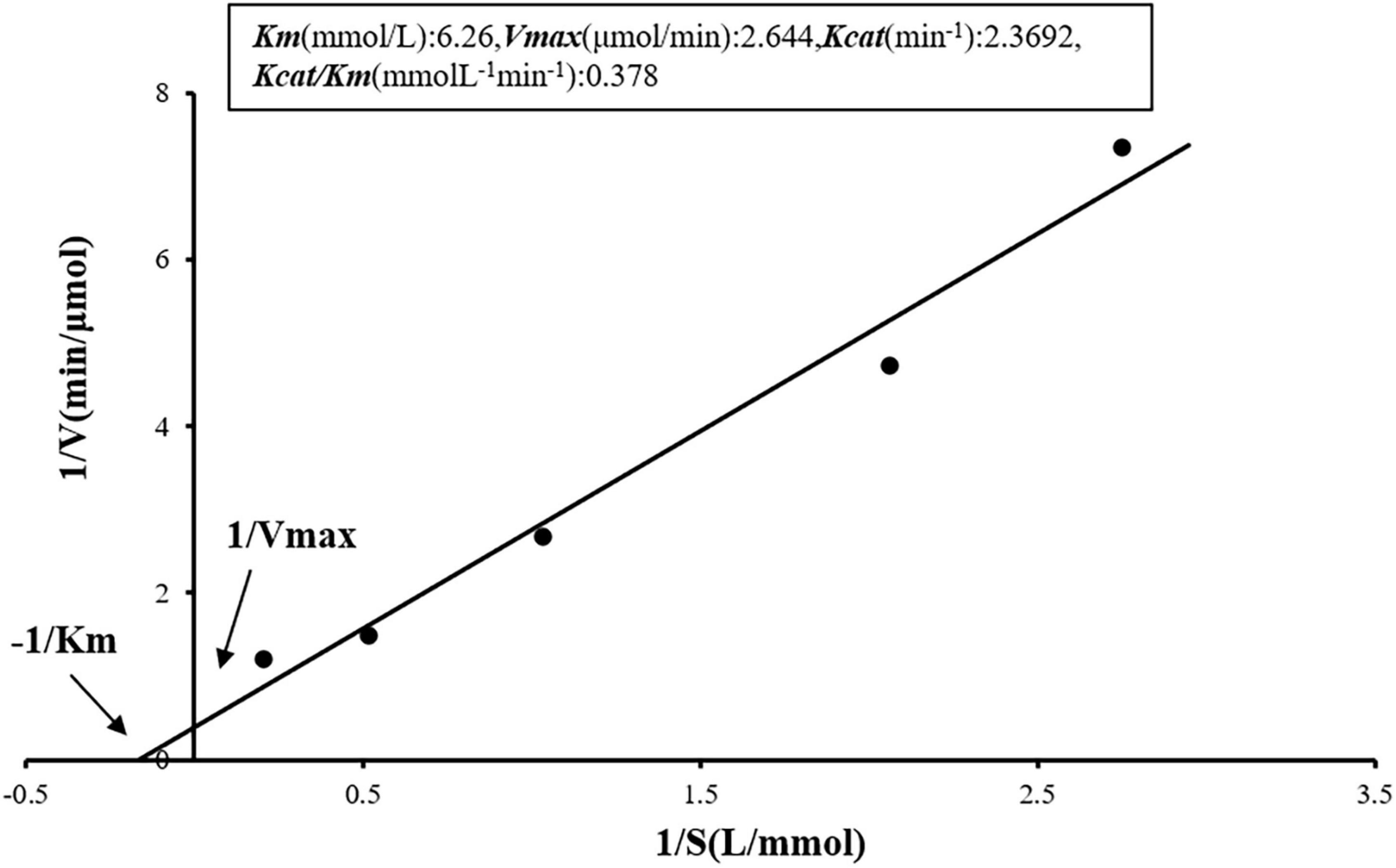
Figure 8. Kinetic analysis of the enzymatic reaction of prot DS5. The enzyme concentration (0.81 mg/ml) was used in the detection. Inset: Kinetic parameters of prot DS5.
Washing effect of prot DS5
By visual appearance evaluation, the addition of prot DS5 showed better stain removal ability compared with control or PB92 protein test cloth (Figure 9A). The visual appearance of better cleaning was supported by quantitative evaluation, and we compared whiteness values to evaluate detergent performance. Specifically, using the calculation method of the value of detergency (R) in GB/T13174-2008 (China National Standard), the R of detergents a, b, and c was 9.50, 18.68, and 23.93, respectively (Figure 9B). The results show that the washing performance of detergents b and c was higher than a. In conclusion, prot DS5 had an excellent stain removal effect.
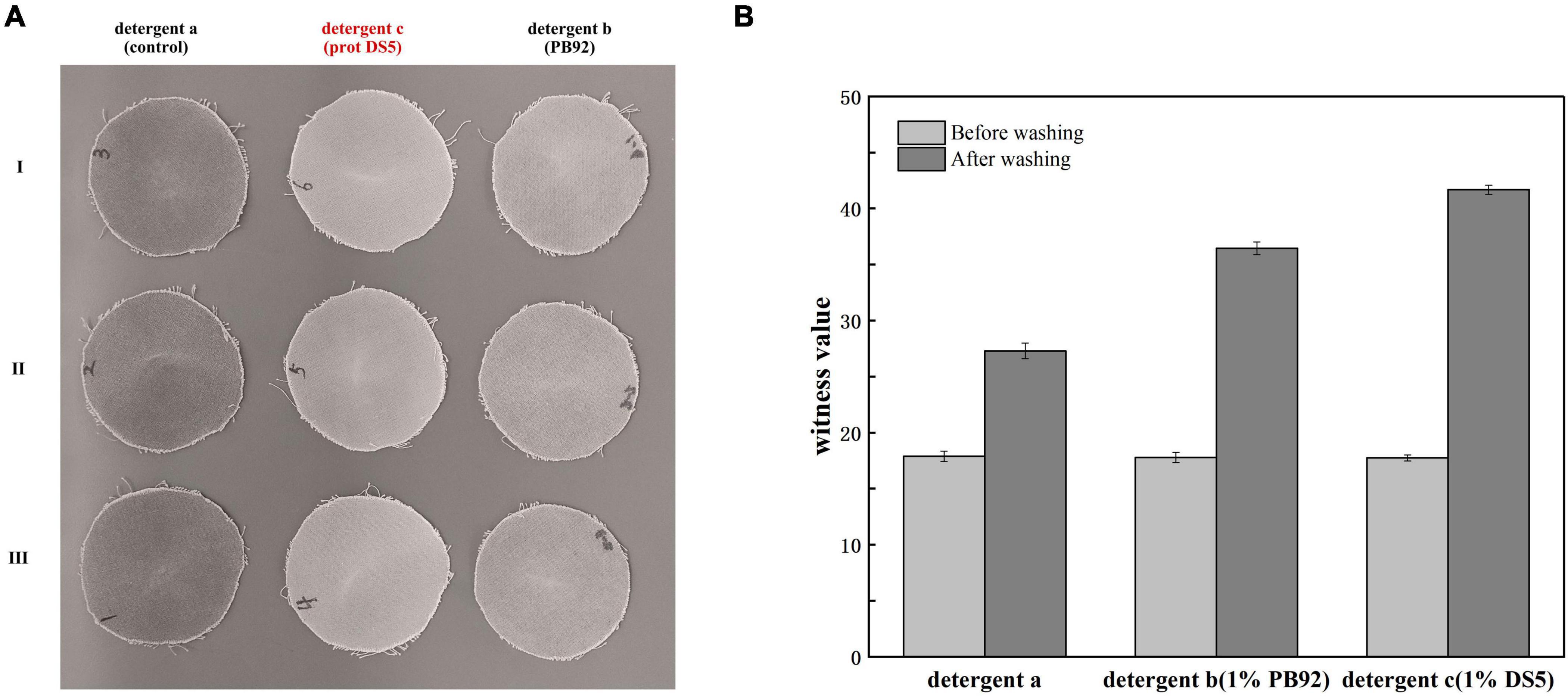
Figure 9. Determination of washing performance of prot DS5. (A) Visual appearance evaluation of wash performance. (B) Quantitative evaluation of wash performance. Detergent a: 2g Chinese national standard detergent. Detergent b: 2g Chinese national standard detergent + 1% PB92 alkaline protease. Detergent c: 2g Chinese national standard detergent + 1% prot DS5.
Discussion
In soil, microorganisms (Singh et al., 2011), animal excreta (Sánchez-Ramos et al., 2004), plants (Milton and Chawla, 1986), and dry-wet deposition (Rudenskaya and Pupov, 2008) constitute the main sources of alkaline proteases. Bacillus (Zhang et al., 2022), Actinomyces (Espersen et al., 2021), and Fungi (Damare et al., 2020) have been reported to produce alkaline protease. Among them, Bacillus is widely used in commercial to produce alkaline protease, such as Bacillus licheniformis used by NovoNordisk, a Danish enzyme manufacturer. A new Bacillus producing alkaline protease was found in the soil of mountain areas in Shanxi Province of China. It was identified as Bacillus halotolerans by 16S rRNA and named as Bacillus halotolerans DS5.
In general, the fermentation time of Bacillus producing alkaline protease is about 24 h–72 h. In previous studies, Bacillus B001 reached the highest enzyme activity when cultured for 30 h (Deng et al., 2010). Bacillus sp. APP1 (Chu, 2007), Bacillus aquimaris VITP4 strain (Thaz and Jayaraman, 2014), Bacillus licheniformis strain K7A (Hadjidj et al., 2018), Bacillus sp. ATCC 6633 (Dias et al., 2008), and endophytic Bacillus halotolerans strain CT2 (Dorra et al., 2018) reached the maximum protease production after 48 h incubation. The cell growth and protease production reached the highest value at 22 h for Bacillus safensis strain RH12 (Rekik et al., 2019). But for Bacillus safensis S406 (Mhamdi et al., 2017), Bacillus sp. SM2014 (Jain et al., 2012), Bacillus sp.DEM05 (Mohamadi et al., 2021), Bacillus pumilus ATCC7061 (Gomaa, 2013), and Bacillus pumilus SG2 (Sangeetha et al., 2011), the protease production reached the highest value at 72 h. Compared with these strains, DS5 strain has the greatest advantage of short fermentation time. The maximum output of protease can be reached after 12 h, which greatly shortens the fermentation time and saves the cost. With the continuous development of science and technology, people have made enormous progress in the field of industrial enzyme research, and a large flow of data on bacterial proteolytic enzymes is emerging, but there is still less report on the separation, purification, and identification of protease from Bacillus halotolerans (Dorra et al., 2018). In view of the limited information about this enzyme from Bacillus halotolerans, it is very valuable to purify and characterize it from a newly isolated bacterium.
Alkaline protease in the detergent industry usually has activity at about 40–50°C (Mothe and Sultanpuram, 2016). The optimum temperature of Savinase is 50–60°C (Beg and Gupta, 2003). The optimum temperature of prot DS5 was 50°C, and 90% of the initial activity was still observed after 60-min incubation at the same temperature. This result is consistent with the report from endophytic Bacillus halotolerans strain CT2 (50°C; Dorra et al., 2018), haloalkaliphilic Bacillus lehensis JO-26 (50°C; Bhatt and Singh, 2020), Geobacillus steelothermophilus (50°C; Chang et al., 2021), and Bacillus sp. DEM05 (50°C; Mohamadi et al., 2021). Prot DS5 has wide pH tolerance and high activity within 6–12. To be the standard for detergents, the enzyme should first be resistant to alkaline conditions (Jain et al., 2012). The optimum pH value of prot DS5 is 9.0. This result is consistent with the report from endophytic Bacillus halotolerans strain CT2 (Dorra et al., 2018), Bacillus safensis strain RH12 (Rekik et al., 2019), and Bacillus invictae AH1 strain (Hammami et al., 2020). These findings indicate that it meets the protease properties required by the detergent industry. To be the first choice of detergent, alkaline protease should have the ability to hydrolyze a variety of proteins. Casein is the best substrate for prot DS5 relative to other substrates. It is consistent with the optimal substrate for protease from Bacillus megaterium-TK1 (Manavalan et al., 2020) and Caldicoprobacter guelmensis (Bouacem et al., 2015), Geotrichum candidum QAUGC01 (Muhammad et al., 2019), and Geobacillus steelothermophilus (Chang et al., 2021). Prot DS5 can remove various protein stains, and the potential of these enzymes to prepare hydrolysates in some industries can also be explored (Tu et al., 2018).
As cofactors of enzymes, metal ions have obvious activating or inhibiting effects on proteases. Many researchers have reported that alkaline proteases require metal ions, such as Ca2+, Zn2+, and Fe2+ to maintain their stable properties at higher temperatures (Bouacem et al., 2015; Ibrahim et al., 2019). Ca2+ and other divalent ions, such as Mn2+ and Mg2+, are known to enhance protease activity. In our research, we found that Mg2+ and Ca2+ enhanced the activity of prot DS5, which further indicated that prot DS5 is suitable for washing in hard water (presence of Mg2+ and Ca2+). With the continuous increase of Mn2+ concentration, the activity of prot DS5 showed a gradually increasing trend. When the Mn2+ concentration reached 15 mM, the activity of prot DS5 increased to 186.42%. Mn2+ ions have been reported to activate proteases from organisms, such as endophytic Bacillus halotolerans strain CT2 (Dorra et al., 2018), Bacillus circulans MTCC 7942 (Patil et al., 2016), Bacillus pumilus TMS55 (Ibrahim et al., 2011), Stenotrophomonas maltophilia (MTCC 7528; Kuddus and Ramteke, 2009), Bacillus sp. ZJ1502 (Yu et al., 2019), and Bacillus amyloliquefaciens strain HM48 (Mushtaq et al., 2021). However, the exact mechanism of Mn2+ remains to be further elaborated. Hydrogen peroxide is a strong oxidant, and most enzymes will be inactivated within 1–2 min in the presence of hydrogen peroxide (Yu et al., 2019). Prot DS5 remained highly active (64.41%) in 5% H2O2. In addition, DTT and β-mercaptoethanol slightly activated prot DS5. Prot DS5 also keeps highly active (106.15%) in high salinity solution (2.5M). Studies on the effects of different surfactants have shown that non-ionic surfactants (Tween-80, Tween-20, AEO, and Triton X-114) slightly increased prot DS5 protease action. The alkaline protease of endophytic Bacillus halotolerans CT2 also has a similar effect (Dorra et al., 2018). Taken together, our results demonstrate that the resistance to salt, oxidants, reductants, surfactants, and metal ions of prot DS5 is better than those of prot CT2.
As an essential component in detergent, protease must be soluble with most detergent additives, including bleach, surfactant, oxidant, and other additives (Sharma et al., 2021). In recent years, researchers have screened alkaline proteases with their own characteristics from different new strains; however, the stability of most new alkaline proteases is reflected in one or several aspects. For example, some proteases have high resistance to NaCl, but poor resistance to oxidants and reductants. Protease from halotolerant alkaliphilic Bacillus sp. NPST-AK15 (Ibrahim et al., 2015) is highly stable in NaCl up to 20% (w/v) and slightly activated by hydrogen peroxide, but the reductants β-mercaptoethanol and DTT significantly inhibited the enzyme activity. In the presence of 10 mM Ca2+ and Tween-80, the enzyme activity of an alkaline protease from fermented bean curd (Yu et al., 2019) can be increased by 21 and 30%, while its tolerance to H2O2 is weak. The alkaline protease produced by Bacillus subtilis DR8806 (Farhadian et al., 2015) can improve the enzyme activity in the presence of Mg2+, Ca2+, K+, and Fe2+, but the temperature, pH stability, and tolerance to hydrogen peroxide are poor. Thus, compared with the reported Bacillus surfactant and detergent stabilizing enzyme, the prot DS5 was found to have better activity and stability, indicating that it has more application value, which may pave the way for its development in industry.
Conclusion
We proposed a novel alkaline protease derived from a novel Bacillus halotolerans strain. The extracellular protease (prot DS5) was also purified and characterized. Prot DS5 exhibits higher activity and stability at 50°C and a wider pH range. Prot DS5 not only is salt tolerant but also shows good tolerance to oxidants and reducing agents, which are the basic conditions for detergent enzymes. DS5 has a very amazing detergency ability, which can be used as a candidate enzyme for industrial applications. It needs further investigation into the structure-function relationship of the enzyme by genetic engineering and protein engineering.
Data availability statement
The original contributions presented in this study are included in the article/Supplementary material, further inquiries can be directed to the corresponding author.
Author contributions
YW and YS conceived and designed the study. YW, JQ, GZ, and XZ performed the experiments. YW performed the data analyses and wrote the manuscript. LW and YS participated in modifying the manuscript. All authors contributed to the article and approved the submitted version.
Funding
This work has been financially supported by the “1331” project in Shanxi Province (No. PT201812).
Conflict of interest
The authors declare that the research was conducted in the absence of any commercial or financial relationships that could be construed as a potential conflict of interest.
Publisher’s note
All claims expressed in this article are solely those of the authors and do not necessarily represent those of their affiliated organizations, or those of the publisher, the editors and the reviewers. Any product that may be evaluated in this article, or claim that may be made by its manufacturer, is not guaranteed or endorsed by the publisher.
Supplementary material
The Supplementary Material for this article can be found online at: https://www.frontiersin.org/articles/10.3389/fmicb.2022.935072/full#supplementary-material
References
Beg, Q. K., and Gupta, R. (2003). Purification and characterization of an oxidation-stable, thiol-dependent serine alkaline protease from Bacillus mojavensis. Enzyme Microb. Technol. 32, 294–304.
Ben-Gad, D., and Gerchman, Y. (2017). Reclassification of Brevibacterium halotolerans DSM8802 as Bacillus halotolerans comb. nov. Based on microbial and biochemical characterization and multiple gene sequence. Curr. Microbiol. 74, 1–5. doi: 10.1007/s00284-016-1143-4
Bhatt, H. B., and Singh, S. P. (2020). Cloning, expression, and structural elucidation of a biotechnologically potential alkaline serine protease from a newly isolated haloalkaliphilic Bacillus lehensis JO-26. Front. Microbiol. 11:941. doi: 10.3389/fmicb.2020.00941
Bouacem, K., Bouanane-Darenfed, A., Laribi-Habchi, H., Elhoul, M. B., Hmida-Sayari, A., Hacene, H., et al. (2015). Biochemical characterization of a detergent-stable serine alkaline protease from Caldicoprobacter guelmensis. Int. J. Biol. Macromol. 81, 299–307. doi: 10.1016/j.ijbiomac.2015.08.011
Chang, C., Gong, S., Liu, Z., Yan, Q., and Jiang, Z. (2021). High level expression and biochemical characterization of an alkaline serine protease from Geobacillus stearothermophilus to prepare antihypertensive whey protein hydrolysate. BMC Biotechnol. 21:21. doi: 10.1186/s12896-021-00678-7
Chu, W. H. (2007). Optimization of extracellular alkaline protease production from species of Bacillus. J. Ind. Microbiol. Biotechnol. 34, 241–245. doi: 10.1007/s10295-006-0192-2
Dai, C., Hou, Y., Xu, H., Umego, E. C., Huang, L., He, R., et al. (2022). Identification of a thermophilic protease-producing strain and its application in solid-state fermentation of soybean meal. J. Sci. Food Agric. 102, 2359–2370. doi: 10.1002/jsfa.11574
Damare, S., Mishra, A., D’Souza-Ticlo-Diniz, D., Krishnaswamy, A., and Raghukumar, C. (2020). A deep-sea hydrogen peroxide-stable alkaline serine protease from Aspergillus flavus. 3 Biotech 10:528. doi: 10.1007/s13205-020-02520-x
Deng, A., Wu, J., Zhang, Y., Zhang, G., and Wen, T. (2010). Purification and characterization of a surfactant-stable high-alkaline protease from Bacillus sp. B001. Bioresour. Technol. 101, 7111–7117. doi: 10.1016/j.biortech.2010.03.130
Dias, D. R., Vilela, D. M., Silvestre, M. P. C., and Schwan, R. F. (2008). Alkaline protease from Bacillus sp. isolated from coffee bean grown on cheese whey. World J. Microbiol. Biotechnol. 24, 2027–2034.
Dorra, G., Ines, K., Imen, B. S., Laurent, C., Sana, A., Olfa, T., et al. (2018). Purification and characterization of a novel high molecular weight alkaline protease produced by an endophytic Bacillus halotolerans strain CT2. Int. J. Biol. Macromol. 111, 342–351. doi: 10.1016/j.ijbiomac.2018.01.024
Espersen, R., Huang, Y., Falco, F. C., Hägglund, P., Gernaey, K. V., Lange, L., et al. (2021). Exceptionally rich keratinolytic enzyme profile found in the rare actinomycetes Amycolatopsis keratiniphila D2(T). Appl. Microbiol. Biotechnol. 105, 8129–8138. doi: 10.1007/s00253-021-11579-2
Farhadian, S., Asoodeh, A., and Lagzian, M. (2015). Purification, biochemical characterization and structural modeling of a potential htrA-like serine protease from Bacillus subtilis DR8806. J. Mol. Catal. B Enzym. 115, 51–58.
Gomaa, E. Z. (2013). Optimization and characterization of alkaline protease and carboxymethyl-cellulase produced by Bacillus pumillus grown on Ficus nitida wastes. Braz. J. Microbiol. 44, 529–537. doi: 10.1590/s1517-83822013005000048
Gupta, R., Beg, Q. K., and Lorenz, P. (2002). Bacterial alkaline proteases: Molecular approaches and industrial applications. Appl. Microbiol. Biotechnol. 59, 15–32. doi: 10.1007/s00253-002-0975-y
Hadjidj, R., Badis, A., Mechri, S., Eddouaouda, K., Khelouia, L., Annane, R., et al. (2018). Purification, biochemical, and molecular characterization of novel protease from Bacillus licheniformis strain K7A. Int. J. Biol. Macromol. 114, 1033–1048. doi: 10.1016/j.ijbiomac.2018.03.167
Hammami, A., Bayoudh, A., Hadrich, B., Abdelhedi, O., Jridi, M., and Nasri, M. (2020). Response-surface methodology for the production and the purification of a new H2O2 -tolerant alkaline protease from Bacillus invictae AH1 strain. Biotechnol. Prog. 36:e2965. doi: 10.1002/btpr.2965
Hiraga, K., Nishikata, Y., Namwong, S., Tanasupawat, S., Takada, K., and Oda, K. (2005). Purification and characterization of serine proteinase from a halophilic bacterium, Filobacillus sp. RF2-5. Biosci. Biotechnol. Biochem. 69, 38–44. doi: 10.1271/bbb.69.38
Hu, M., Zhao, H., Zhang, C., Yu, J., and Lu, Z. (2013). Purification and characterization of plantaricin 163, a novel bacteriocin produced by Lactobacillus plantarum 163 isolated from traditional Chinese fermented vegetables. J. Agric. Food Chem. 61, 11676–11682. doi: 10.1021/jf403370y
Ibrahim, A. S., Al-Salamah, A. A., El-Badawi, Y. B., El-Tayeb, M. A., and Antranikian, G. (2015). Detergent-, solvent- and salt-compatible thermoactive alkaline serine protease from halotolerant alkaliphilic Bacillus sp. NPST-AK15: Purification and characterization. Extremophiles 19, 961–971. doi: 10.1007/s00792-015-0771-0
Ibrahim, A. S. S., Elbadawi, Y. B., El-Tayeb, M. A., Al-Maary, K. S., Maany, D. A. F., Ibrahim, S. S. S., et al. (2019). Alkaline serine protease from the new halotolerant alkaliphilic Salipaludibacillus agaradhaerens strain AK-R: Purification and properties. 3 Biotech 9:391. doi: 10.1007/s13205-019-1928-9
Ibrahim, K. S., Muniyandi, J., and Karutha Pandian, S. (2011). Purification and characterization of manganese-dependent alkaline serine protease from Bacillus pumilus TMS55. J. Microbiol. Biotechnol. 21, 20–27. doi: 10.4014/jmb.1009.09001
Ito, S., Kobayashi, T., Ara, K., Ozaki, K., Kawai, S., and Hatada, Y. (1998). Alkaline detergent enzymes from alkaliphiles: Enzymatic properties, genetics, and structures. Extremophiles 2, 185–190. doi: 10.1007/s007920050059
Jain, D., Pancha, I., Mishra, S. K., Shrivastav, A., and Mishra, S. (2012). Purification and characterization of haloalkaline thermoactive, solvent stable and SDS-induced protease from Bacillus sp.: A potential additive for laundry detergents. Bioresour. Technol. 115, 228–236. doi: 10.1016/j.biortech.2011.10.081
Karray, A., Alonazi, M., Horchani, H., and Ben Bacha, A. (2021). A novel thermostable and alkaline protease produced from Bacillus stearothermophilus isolated from olive oil mill sols suitable to industrial biotechnology. Molecules 26:1139. doi: 10.3390/molecules26041139
Kuddus, M., and Ramteke, P. W. (2009). Cold-active extracellular alkaline protease from an alkaliphilic Stenotrophomonas maltophilia: Production of enzyme and its industrial applications. Can. J. Microbiol. 55, 1294–1301. doi: 10.1139/w09-089
Li, S., Xu, J., Fu, L., Xu, G., Lin, X., Qiao, J., et al. (2022). Biocontrol of wheat crown rot using Bacillus halotolerans QTH8. Pathogens 11:595. doi: 10.3390/pathogens11050595
Manavalan, T., Manavalan, A., Ramachandran, S., and Heese, K. (2020). Identification of a novel thermostable alkaline protease from Bacillus megaterium-TK1 for the detergent and leather industry. Biology 9:472. doi: 10.3390/biology9120472
Maruthiah, T., Esakkiraj, P., Prabakaran, G., Palavesam, A., and Immanuel, G. (2013). Purification and characterization of moderately halophilic alkaline serine protease from marine Bacillus subtilis AP-MSU 6. Biocatal. Agric. Biotechnol. 2, 116–119.
Mechri, S., Ben Elhoul Berrouina, M., Omrane Benmrad, M., Zaraî Jaouadi, N., Rekik, H., Moujehed, E., et al. (2017). Characterization of a novel protease from Aeribacillus pallidus strain VP3 with potential biotechnological interest. Int. J. Biol. Macromol. 94(Pt A), 221–232. doi: 10.1016/j.ijbiomac.2016.09.112
Mesbah, N. M. (2022). Industrial biotechnology based on enzymes from extreme environments. Front. Bioeng. Biotechnol. 10:870083. doi: 10.3389/fbioe.2022.870083
Mhamdi, S., Bkhairia, I., Nasri, R., Mechichi, T., Nasri, M., and Kamoun, A. S. (2017). Evaluation of the biotechnological potential of a novel purified protease BS1 from Bacillus safensis S406 on the chitin extraction and detergent formulation. Int. J. Biol. Macromol. 104(Pt A), 739–747. doi: 10.1016/j.ijbiomac.2017.06.062
Milton, D. K., and Chawla, R. K. (1986). Cotton dust contains proteolytic and elastolytic enzymes not inhibited by alpha-1-proteinase inhibitor. Am. J. Ind. Med. 9, 247–260. doi: 10.1002/ajim.4700090308
Mirzapour Kouhdasht, A., Moosavi-Nasab, M., and Aminlari, M. (2018). Gelatin production using fish wastes by extracted alkaline protease from Bacillus licheniformis. J. Food Sci. Technol. 55, 5175–5180. doi: 10.1007/s13197-018-3449-7
Mohamadi, S., Mehrabi, M., and Sajadimajd, S. (2021). Purification and characterization of an extracellular alkaline solvent-stable metalloprotease secreted from newly isolated Bacillus sp. DEM05: Optimization of protease production. Iran. J. Biotechnol. 19:e2866. doi: 10.30498/ijb.2021.247161.2866
Mokashe, N., Chaudhari, B., and Patil, U. (2018). Operative utility of salt-stable proteases of halophilic and halotolerant bacteria in the biotechnology sector. Int. J. Biol. Macromol. 117, 493–522. doi: 10.1016/j.ijbiomac.2018.05.217
Mothe, T., and Sultanpuram, V. R. (2016). Production, purification and characterization of a thermotolerant alkaline serine protease from a novel species Bacillus caseinilyticus. 3 Biotech 6:53. doi: 10.1007/s13205-016-0377-y
Muhammad, A., Bokhari, S. A. I., Vernoux, J. P., Ali, M. I., Faryal, R., Desmasures, N., et al. (2019). Purification, characterization and thermodynamic assessment of an alkaline protease by Geotrichum candidum of dairy origin. Iran. J. Biotechnol. 17:e2042. doi: 10.21859/ijb.2042
Mushtaq, H., Jehangir, A., Ganai, S. A., Farooq, S., Ganai, B. A., and Nazir, R. (2021). Biochemical characterization and functional analysis of heat stable high potential protease of Bacillus amyloliquefaciens strain HM48 from soils of Dachigam National Park in Kashmir Himalaya. Biomolecules 11:117. doi: 10.3390/biom11010117
Nandan, A., and Nampoothiri, K. M. (2020). Therapeutic and biotechnological applications of substrate specific microbial aminopeptidases. Appl. Microbiol. Biotechnol. 104, 5243–5257. doi: 10.1007/s00253-020-10641-9
Parashar, D., and Satyanarayana, T. (2018). An insight into ameliorating production, catalytic efficiency, thermostability and starch saccharification of acid-stable α-amylases from acidophiles. Front. Bioeng. Biotechnol. 6:125. doi: 10.3389/fbioe.2018.00125
Patil, U., Mokashe, N., and Chaudhari, A. (2016). Detergent-compatible, organic solvent-tolerant alkaline protease from Bacillus circulans MTCC 7942: Purification and characterization. Prep. Biochem. Biotechnol. 46, 56–64. doi: 10.1080/10826068.2014.979205
Prasetyo, J., Zhu, J., Kato, T., and Park, E. Y. (2011). Efficient production of cellulase in the culture of Acremonium cellulolyticus using untreated waste paper sludge. Biotechnol. Prog. 27, 104–110. doi: 10.1002/btpr.534
Raddadi, N., Cherif, A., Daffonchio, D., Neifar, M., and Fava, F. (2015). Biotechnological applications of extremophiles, extremozymes and extremolytes. Appl. Microbiol. Biotechnol. 99, 7907–7913. doi: 10.1007/s00253-015-6874-9
Ramkumar, A., Sivakumar, N., Gujarathi, A. M., and Victor, R. (2018). Production of thermotolerant, detergent stable alkaline protease using the gut waste of Sardinella longiceps as a substrate: Optimization and characterization. Sci. Rep. 8:12442. doi: 10.1038/s41598-018-30155-9
Rekik, H., Zaraî Jaouadi, N., Gargouri, F., Bejar, W., Frikha, F., Jmal, N., et al. (2019). Production, purification and biochemical characterization of a novel detergent-stable serine alkaline protease from Bacillus safensis strain RH12. Int. J. Biol. Macromol. 121, 1227–1239. doi: 10.1016/j.ijbiomac.2018.10.139
Rudenskaya, G. N., and Pupov, D. V. (2008). Cysteine proteinases of microorganisms and viruses. Biochemistry 73, 1–13. doi: 10.1134/s000629790801001x
Sagredo-Beltrán, J., De La Cruz-Rodríguez, Y., Alvarado-Rodríguez, M., Vega-Arreguín, J., Rodríguez-Guerra, R., Alvarado-Gutiérrez, A., et al. (2018). Genome sequence of Bacillus halotolerans strain MS50-18A with antifungal activity against phytopathogens, isolated from saline soil in San Luís Potosí, Mexico. Genome Announc. 6:e00135-18. doi: 10.1128/genomeA.00135-18
Sánchez-Ramos, I., Hernández, C. A., Castañera, P., and Ortego, F. (2004). Proteolytic activities in body and faecal extracts of the storage mite, Acarus farris. Med. Vet. Entomol. 18, 378–386. doi: 10.1111/j.0269-283X.2004.00518.x
Sangeetha, R., Geetha, A., and Arulpandi, I. (2011). Pongamia pinnata seed cake: A promising and inexpensive substrate for production of protease and lipase from Bacillus pumilus SG2 on solid-state fermentation. Indian J. Biochem. Biophys. 48, 435–439.
Santiago, M., Ramírez-Sarmiento, C. A., Zamora, R. A., and Parra, L. P. (2016). Discovery, molecular mechanisms, and industrial applications of cold-active enzymes. Front. Microbiol. 7:1408. doi: 10.3389/fmicb.2016.01408
Sharma, S., Kumar, S., Kaur, R., and Kaur, R. (2021). Multipotential alkaline protease from a novel Pyxidicoccus sp. 252: Ecofriendly replacement to various chemical processes. Front. Microbiol. 12:722719. doi: 10.3389/fmicb.2021.722719
Singh, S. K., Singh, S. K., Tripathi, V. R., Khare, S. K., and Garg, S. K. (2011). A novel psychrotrophic, solvent tolerant Pseudomonas putida SKG-1 and solvent stability of its psychro-thermoalkalistable protease. Process Biochem. 46, 1430–1435.
Thaz, C. J., and Jayaraman, G. (2014). Stability and detergent compatibility of a predominantly β-sheet serine protease from halotolerant B. aquimaris VITP4 strain. Appl. Biochem. Biotechnol. 172, 687–700. doi: 10.1007/s12010-013-0524-4
Tu, M., Liu, H., Zhang, R., Chen, H., Mao, F., Cheng, S., et al. (2018). Analysis and evaluation of the inhibitory mechanism of a novel angiotensin-I-converting enzyme inhibitory peptide derived from casein hydrolysate. J. Agric. Food Chem. 66, 4139–4144. doi: 10.1021/acs.jafc.8b00732
Yang, H., Li, J., Shin, H. D., Du, G., Liu, L., and Chen, J. (2014). Molecular engineering of industrial enzymes: Recent advances and future prospects. Appl. Microbiol. Biotechnol. 98, 23–29. doi: 10.1007/s00253-013-5370-3
Yu, P., Huang, X., Ren, Q., and Wang, X. (2019). Purification and characterization of a H2O2-tolerant alkaline protease from Bacillus sp. ZJ1502, a newly isolated strain from fermented bean curd. Food Chem. 274, 510–517. doi: 10.1016/j.foodchem.2018.09.013
Zhang, J., Wang, J., Zhao, Y., Li, J., and Liu, Y. (2019). Study on the interaction between calcium ions and alkaline protease of Bacillus. Int. J. Biol. Macromol. 124, 121–130. doi: 10.1016/j.ijbiomac.2018.11.198
Zhang, J., Zhu, B., Li, X., Xu, X., Li, D., Zeng, F., et al. (2022). Multiple modular engineering of Bacillus amyloliquefaciens cell factories for enhanced production of alkaline proteases from B. clausii. Front. Bioeng. Biotechnol. 10:866066. doi: 10.3389/fbioe.2022.866066
Zhang, Z., Yin, L., Li, X., Zhang, C., Liu, C., and Wu, Z. (2018). The complete genome sequence of Bacillus halotolerans ZB201702 isolated from a drought- and salt-stressed rhizosphere soil. Microb. Pathog. 123, 246–249. doi: 10.1016/j.micpath.2018.07.019
Zhao, S., Han, J., Bie, X., Lu, Z., Zhang, C., and Lv, F. (2016). Purification and characterization of plantaricin JLA-9: A novel bacteriocin against Bacillus spp. produced by Lactobacillus plantarum JLA-9 from Suan-Tsai, a traditional Chinese fermented cabbage. J. Agric. Food Chem. 64, 2754–2764. doi: 10.1021/acs.jafc.5b05717
Keywords: alkaline protease, identification, purification, enzymatic properties, Bacillus halotolerans
Citation: Wen Y, Qiang J, Zhou G, Zhang X, Wang L and Shi Y (2022) Characterization of redox and salinity-tolerant alkaline protease from Bacillus halotolerans strain DS5. Front. Microbiol. 13:935072. doi: 10.3389/fmicb.2022.935072
Received: 03 May 2022; Accepted: 26 July 2022;
Published: 18 August 2022.
Edited by:
Luis Henrique Guimarães, University of São Paulo, BrazilReviewed by:
Marcelo Franco, Universidade Estadual de Santa Cruz, BrazilLijing Xu, Shanxi Agricultural University, China
Copyright © 2022 Wen, Qiang, Zhou, Zhang, Wang and Shi. This is an open-access article distributed under the terms of the Creative Commons Attribution License (CC BY). The use, distribution or reproduction in other forums is permitted, provided the original author(s) and the copyright owner(s) are credited and that the original publication in this journal is cited, in accordance with accepted academic practice. No use, distribution or reproduction is permitted which does not comply with these terms.
*Correspondence: Yawei Shi, eWF3ZWlzaGlAc3h1LmVkdS5jbg==
†These authors have contributed equally to this work