- 1College of Land and Environment, Shenyang Agricultural University, Shenyang, China
- 2National Engineering Research Center for Efficient Utilization of Soil and Fertilizer Resources, Shenyang, China
- 3Monitoring and Experimental Station of Corn Nutrition and Fertilization in Northeast Region, Ministry of Agriculture, Shenyang, China
- 4Department of Foreign Languages, Shenyang Agricultural University, Shenyang, China
Soil microorganisms play crucial roles in nutrient cycling and determining soil quality and fertility; thus, they are important for agricultural production. However, the impacts of long-term fertilization on soil microbial community remain ambiguous due to inconsistent results from different studies. The objective of this study was to characterize changes in bacterial and fungal diversity and community structures after 12 years of different fertilization in aeolian sandy soil by analyzing 16S rRNA and ITS rRNA gene sequences and the soil properties to discover the driving factors. Eight different fertilizer treatments have been set up since 2009: no fertilizer (CK), chemical N fertilizer (N), chemical N and P fertilizer (NP), chemical N, P and K fertilizer (NPK), pig manure only (M), pig manure plus chemical N fertilizer (MN), pig manure plus chemical N and P fertilizer (MNP), pig manure plus chemical N, P, and K fertilizer (MNPK). The results indicated that the long-term application of chemical fertilizer reduced soil pH, whereas the addition of pig manure alleviated a decrease in soil pH value. Chemical fertilizer plus pig manure significantly improved soil available nutrients and soil organic carbon. Long-term MNPK fertilization resulted in changes in bacterial diversity due to effects on specific bacterial species; by contrast, all fertilization treatments resulted in changes in fungal diversity due to changes in soil properties. Principal component analysis indicated that fertilization had a significant effect on soil microbial community structure, and the effect of chemical fertilizer combined with pig manure was greater than that of chemical fertilizer alone. Soil available phosphorus, total phosphorus, and pH were the most important factors that influenced bacterial taxa, whereas soil pH, total phosphorus, organic carbon, ammonium nitrogen and nitrate nitrogen were the most important factors influencing fungal taxa after 12 years of fertilization in aeolian sandy soil.
Introduction
Soil microorganisms are the crucial drivers of soil organic matter formation and decomposition (Zhang et al., 2012), and play critical roles in maintaining soil health, productivity and sustainability (Chaparro et al., 2012; Su et al., 2015), as well as in regulating crop production or disease suppression (Van Der Heijden et al., 2008; Berg, 2009). Even though microorganisms account for only a small part of soil quality, they play an essential role in the cycling of soil carbon (C), nitrogen (N), phosphorus (P), and sulfur (S), and are important components in maintaining soil quality and fertility (Wei et al., 2019; Zhang et al., 2019). Changes in soil microbial community also affect the productivity and function of agroecosystems. Due to the importance of microorganisms in agroecosystems, researchers have paid increasing attention to the study of soil microorganisms in recent years. Soil microbial communities are affected by human activities such as fertilization and tillage (Ding et al., 2017). Previous studies have shown that soil microorganisms could be influenced by many factors, such as soil type (Nicolitch et al., 2016), tillage system (Hartmann et al., 2015; Wang et al., 2020), fertilizer regime (Wang et al., 2021), sampling period (Wang et al., 2018), and crop species (Li et al., 2021).
Fertilization is an important agricultural practice to improve soil fertility and increase crop yield in agroecosystems (Francioli et al., 2016; Hu et al., 2017). Fertilization can indirectly impact soil microorganisms by changing soil properties or directly by input of nutrients (Pan et al., 2020; Yan et al., 2021). A previous study indicated that long-term application of chemical fertilizer resulted in a significant decline in soil bacterial diversity due to a decrease in soil pH value, while the addition of manure effectively alleviated this decline (Sun et al., 2015). However, some studies yielded contradictory results. For instance, some studies revealed that application of organic fertilizer could increase soil microbial diversity compared with application of chemical fertilizer, whereas others indicated that application of organic fertilizer could reduce microbial diversity (Hu et al., 2018; Xu et al., 2020; Yang et al., 2020). Soil pH is generally considered to be the main factor affecting soil bacterial diversity, while fungal diversity was generally less affected by soil pH (Rousk et al., 2010). Zhou et al. (2016) indicated that long-term chemical N and P fertilization reduced the soil fungal diversity and altered the fungal community distribution in a black soil. Sun et al. (2016) revealed that the application of organic matter significantly reduced the relative abundance of potentially pathogenic fungi and changed the soil fungal community structure. The responses of soil microbial community to fertilization have been reported widely (Hu et al., 2017; Yu et al., 2019; Mei et al., 2021). Understanding the changes in soil microbial communities under different fertilization regimes in different soils is needed for determining the optimal fertilization methods to improve soil conditions and crop productivity.
Aeolian sandy soils have poor soil structure, low organic matter and nutrient content, water leakage and poor fertilizer retention ability. Fertilization is an effective method for improving aeolian sandy soils. Although the effects of long-term inorganic fertilizer application combined with organic fertilizer on soil microbial communities have been investigated frequently, few studies have been reported on aeolian sandy soils, and the potential links between soil properties and microbial communities remain unclear in aeolian sandy soils in northeast China. Therefore, this study aimed to investigate the changes in soil microbial diversity and community structure in an aeolian sandy soil due to differential fertilization for 12 years and to determine the relationship between soil microbial diversity and community structure and soil properties.
High-throughput sequencing was used to analyze soil microbial diversity and community structure in the aeolian sandy soil after 12-year fertilization. Our objectives were: (i) to evaluate the effects of various fertilization regimes on soil microbial diversity and community structure in the aeolian sandy soil, and (ii) to discover the most important factors affecting soil microbial communities. We hypothesized that the combined application of chemical fertilizer and pig manure would be more favorable than chemical fertilizer or pig manure alone in improving soil nutrient status and enhancing soil microbial diversity, and 12-year fertilization would change soil microbial community structure by altering soil properties.
Materials and methods
Site description and experimental design
The long-term fertilization experiment was established in 2009 at the Soil and Fertilizer Test Base of the National Peanut Industry Technology System in Shenyang Agricultural University, Liaoning Province, northeastern China (40°48′N, 123°33′E). This region has a temperate sub-humid continental climate with annual precipitation 574∼684 mm. The frost-free period is 164 days, and the average annual temperature is 7.0∼8.1°C. The field experiment was a continuous cropping system of peanut. The tested soil is aeolian sandy soil, which is classified as Entisols according to the USDA Soil Taxonomy classification systems.
Eight treatments were arranged in a randomized block design in triplicate under the peanut continuous cropping system. Each plot was 2 × 1 m in size and separated by 0.2 m wide and 2 m deep cement walls. The treatments included: (1) control treatment (CK); (2) chemical N fertilizer (N); (3) chemical N and P fertilizer (NP); (4) chemical N, P, and K fertilizer (NPK); (5) pig manure only (M); (6) pig manure plus chemical N fertilizer (MN); (7) pig manure plus chemical N and P fertilizer (MNP); (8) pig manure plus chemical N, P, and K fertilizer (MNPK). Urea, calcium superphosphate and potassium sulfate were applied as chemical fertilizers to supply N, P, and K, respectively. Fertilizer application rates of the eight treatments are shown in Supplementary Table 1. The average nutrient contents in pig manure were as follows: 7.2 g⋅kg–1 N; 8.7 g⋅kg–1 P2O5; 10.0 g⋅kg–1 K2O; and 83.5 g⋅kg–1 organic C. The field was plowed (20 cm deep) before planting. The whole process (including hoeing, ridging, fertilizing, sowing, weeding, and harvesting) was done manually.
Peanuts (Arachis hypogaea L.) were sown on May 12 and harvested on September 20, 2020. The width of the ridge was 60 cm; two rows of peanuts were planted in each ridge and the distance between two rows was 30 cm. Two seeds were sown in each hole, and the distance between two holes was 14 cm. The planting density of peanut was 30,000 plants ha–1.
Sample collection and soil property measurements
Rhizosphere soil samples were collected during the peanut harvest in 2020. Eight peanut plants were randomly selected in each plot, and the whole plants with their roots were dug out with a spade (approximately 20 cm depth). The roots were shaken gently to remove the loosely adhering soil and carefully brushed to collect the remaining rhizosphere soil. The visible plant roots and residues were removed from fresh rhizosphere soil samples, sieve (<2 mm) and divided into three subsamples. One subsample was air-dried to determine soil properties; the second subsample was used for determining soil ammonium nitrogen (NH4+-N) and soil nitrate nitrogen (NO3–-N), and the third subsample was stored at –80°C for deoxyribonucleic acid (DNA) extraction.
Soil pH was measured using a pH meter (Mettler-Toledo 320) with a 1:2.5 ratio of soil to water. Total nitrogen (TN) and soil organic carbon (SOC) were determined by an Element Auto-Analyzer (Vario MAX CN; Elementar, Hanau, Germany). Soil ammonium nitrogen (NH4+-N) and nitrate nitrogen (NO3–-N) were extracted with 1 M KCl and measured by an autoanalyzer (AutoAnalyzer3, SEAL Analytical, Germany). Available phosphorus (AP) and available potassium (AK) was extracted using 0.5 M NaHCO3 solution and 1 M NH4OAc, respectively, and determined by molybdenum-blue colorimetry and flame photometry (M410, Sherwood Scientific Ltd., United Kingdom), respectively (Bao, 2000). Soil total phosphorus (TP) and total potassium (TK) was determined according to Lu (1999).
Soil deoxyribonucleic acid extraction, polymerase chain reaction amplification, and Illumina sequencing
Soil DNA was extracted using a Powersoil® DNA isolation kit (Mo Bio Laboratories, Inc., Carlsbad, CA, United States) according to the manufacturer’s instructions. The concentration and purity of extracted DNA were assessed by a NanoDrop ND-1000 (NanoDrop Technologies Inc., Wilmington, DE, United States).
The hypervariable region V3-V4 of the bacterial 16S ribosomal RNA (rRNA) gene was amplified with the primer pair 338F (5′-ACTCCTACGGGAGGCAGCAG-3′) and 806R (5′-GGACTACHVGGGTWTCTAAT-3′) (Xu et al., 2016). The 20 μL polymerase chain reaction (PCR) reaction mixture contained 4 μL of 5 × FastPfu Buffer, 2 μL of dNTPs (2.5 mM), 0.2 μL of bovine serum albumin (BSA), 0.8 μL of forward and reverse primers (5 μM), 0.4 μL of FastPfu DNA polymerase, 10 ng of template DNA, and was adjusted with ddH2O to 20 μL. The fungal ITS rRNA genes were amplified with the primer pair ITS1F (5′-CTTGGTCATTTAGAGGAAGTAA-3′) and ITS2R (5′-GCTGCGTTCTTCATCGATGC-3′) (Adams et al., 2013). The 20 μL PCR reaction mixture contained 2 μL of 10 × Buffer, 2 μL of dNTPs (2.5 mM), 0.2 μL of BSA, 0.8 μL of forward and reverse primers (5 μM), 0.2 μL of rTaq DNA Polymerase, 10 ng of template DNA, and was adjusted with ddH2O to 20 μL. The PCR thermal cycle conditions were: an initial denaturation step at 95°C for 3 min, followed by 27 cycles (for bacteria) or 35 cycles (for fungi) of denaturing at 95°C for 30 s, annealing at 55°C for 30 s, extension at 72°C for 45 s, and a final extension at 72°C for 10 min. The purified amplicons were sequenced on an Illumina MiSeq PE300 platform (Illumina, San Diego, CA, United States) by Majorbio Bio-Pharm Technology Co., Ltd. (Shanghai, China). The raw sequence data were deposited in the NCBI Sequence Read Archive database with the SRA accession numbers of PRJNA838988 and PRJNA839049 for bacteria and fungi, respectively.
Statistical analyses
Statistical analyses were performed using SPSS 19.0 (SPSS Inc., Chicago, IL, United States). The differences between treatments were analyzed by one-way analysis of variance (ANOVA), followed by Least Significant Difference (LSD) test. Spearman’s correlation analysis was used to evaluate the relationship between soil microbial diversity and soil properties. The online Circos software was used to perform the Circos graphs for bacterial and fungal community compositions.1 Principal coordinates analysis (PCoA) was performed to analyze beta-diversity in different treatments based on Bray-Curtis distance. Permutational multivariate analysis of variance (PERMAVONA) was calculated using the R package “vegan” to determine the significant differences in beta-diversity among the treatments. The linear discriminant analysis effect size (LEfSe) method was used to estimate potential microbial markers under different treatments. Redundancy analysis (RDA) with Monte Carlo permutation tests (n = 999) and Mantel test were performed using R to analyze the relationship between soil microbial communities and soil properties.
Results
Soil properties
The changes in soil properties under various treatments after 12 years of fertilization are shown in Table 1. Overall, fertilization increased the contents of SOC, TN, TP, NH4+-N, NO3–-N, AP, and AK (P < 0.05), except the N treatment. The contents of SOC, TN, TP, NO3–-N, AP, and AK were significantly higher in manure treatments (M, MN, MNP, and MNPK) than in chemical fertilizer treatments (N, NP, and NPK) (P < 0.05). The soil pH varied from 5.34 to 6.18. Long-term chemical fertilizer application significantly decreased the soil pH (P < 0.05), whereas the application of pig manure effectively alleviated soil acidification (P < 0.05).
Soil bacterial and fungal alpha-diversity
A total of 745,702 bacterial and 1,313,236 fungal sequences were detected in the 24 soil samples (eight treatments in biological triplicates) and were clustered into 5804 and 1541 operational taxonomic units (OTUs) based on 97% sequence similarity. The OTUs were assigned to 31 phyla and 849 genera for bacteria, and 17 phyla and 443 genera for fungi. The rarefaction curves approached saturation with the increasing number of sequences (Supplementary Figure 1), indicating that the depth of sequencing was sufficient to characterize the soil microbial community in this study.
Long-term fertilization resulted in variations in alpha-diversity of rhizosphere soil microbial community, including species richness [ACE (Abundance-based Coverage Estimator) indices] and diversity (Shannon indices) (Table 2). Soil bacterial ACE index was highest in the MN treatment and lowest in the NPK treatment. The ACE index was higher in the manure treatments (M, MN, MNP, and MNPK) than the chemical fertilizer treatments (N, NP, and NPK). Correlation analysis showed that bacterial ACE index was significantly positively correlated with SOC and TN (P < 0.01), and was positively correlated with TP, AP, and pH (P < 0.05, Table 3). Soil bacterial Shannon index was higher in the CK, M, and MN compared with the other treatments, and was lowest in the MNPK treatment.

Table 3. Spearman correlation coefficient between soil properties and soil microbial alpha-diversity.
Regarding soil fungal community diversity, the ACE index was lowest in the N treatment and highest in the M treatment. Compared with the CK treatment, ACE indices increased in the other fertilization treatments, except the N treatment. Correlation analysis showed that soil fungal ACE index was negatively correlated with NH4+-N (P < 0.01), and was positively correlated with other soil properties (Table 3). The Shannon index was highest in the MNPK treatment and lowest in the N treatment. The Shannon indices were higher in the manure treatments (M, MN, MNP, and MNPK) than the other treatments, except the NPK treatment. The soil fungal Shannon index was negatively correlated with NH4+-N (P < 0.01), and was positively correlated with the other soil properties, except NO3–-N (Table 3).
Soil bacterial and fungal community composition and structure
Twelve bacterial phyla with the relative abundance >1% were detected in all soil samples, including Actinobacteria, Proteobacteria, Firmicutes, Acidobacteria, Chloroflexi, Gemmatimonadetes, Bacteroidetes, Rokubacteria, Planctomycetes, Verrucomicrobiota, Nitrospirae, and Patescibacteria (Figure 1A). The most dominant phylum was Actinobacteria (29.98–38.40%), followed by Proteobacteria (22.40–30.85%) and Firmicutes (4.50–17.84%). The relative abundances of Actinobacteria and Proteobacteria in the no-manure treatments (CK, N, NP, NPK) were higher than those in the manure treatments (M, MN, MNP, and MNPK), whereas the relative abundance of Firmicutes in the no-manure treatments was significantly lower than that in the manure treatments (P < 0.05; Supplementary Figure 2A). The relative abundances of Gemmatimonadetes, Rokubacteria, and Nitrospirae decreased with fertilization. No statistically significant differences among the treatments were detected in the relative abundances of Actinobacteria, Chloroflexi, Planctomycetes, and Verrucomicrobiota. For fungal communities, three phyla and one unidentified phylum with the relative abundance > 1% were detected, including Ascomycota (71.43–84.13%), Basidiomycota (8.95–20.41%) and Mortierellomycota (3.71–9.79%) (Figure 1B). The relative abundance of Ascomycota was lowest in the MNPK treatments. The relative abundance of Basidiomycota was higher in the all fertilization treatments (except MN) compared with the CK treatment. The relative abundance of Mortierellomycota was lowest in the N treatment, and was significantly higher in the treatments with than without pig manure (Supplementary Figure 2B).
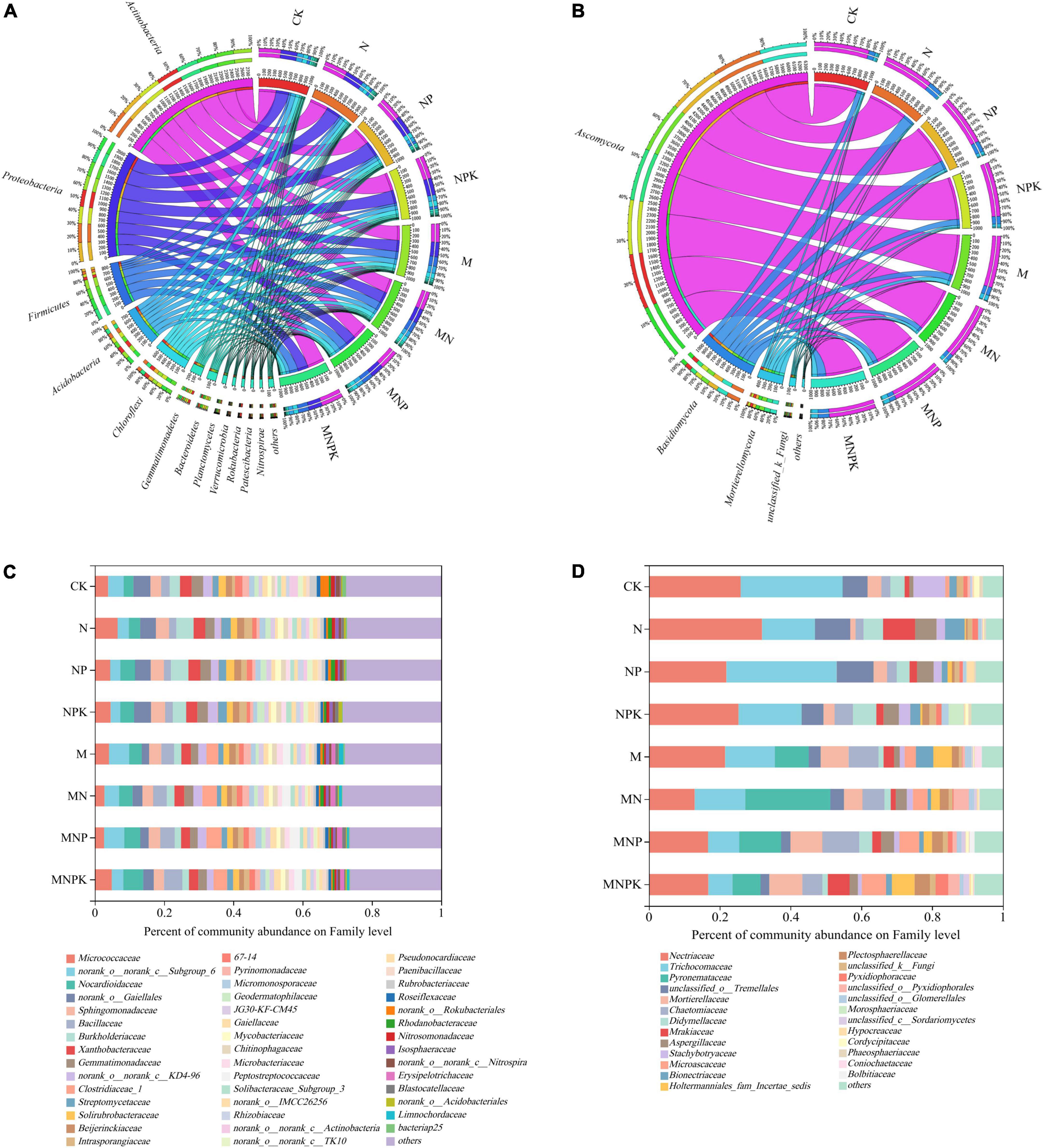
Figure 1. Relative abundances of the rhizosphere microbial community composition on phylum and family levels under different treatments. (A) Bacterial community on phylum level. (B) Fungal community on phylum level. (C) Bacterial community on family level. (D) Fungal community on family level. CK, no fertilizer; N, chemical N fertilizer; NP, chemical N and P fertilizer; NPK, chemical N, P, and K fertilizer; M, pig manure only; MN, pig manure plus chemical N fertilizer; MNP, pig manure plus chemical N and P fertilizer; MNPK, pig manure plus chemical N, P, and K fertilizer.
The soil bacterial and fungal compositions at the family level are detailed in Figure 1. For bacteria, the relative abundances of norank_o__Gaiellales, Streptomycetaceae (belonging to Actinobacteria), Sphingomonadaceae, and Burkholderiaceae (belonging to Proteobacteria) in the chemical fertilizer treatments were significantly higher than those in the manure treatments. The relative abundances of Bacillaceae and Clostridiaceae_1 (belonging to Firmicutes) increased significantly in the manure treatments (Figure 1C). Regarding fungal families, the relative abundances of Nectriaceae, Trichocomaceae, Didymellaceae, Stachybotryaceae (belonging to Ascomycota) and unclassified_o__Tremellales (belonging to Basidiomycota) were higher in the chemical fertilizer treatments compared with those that received pig manure. The relative abundances of Pyronemataceae, Chaetomiaceae, Microascaceae (belonging to Ascomycota) and Mortierellaceae (Mortierellomycota) increased significantly in the manure treatments (Figure 1D).
The variations in soil bacterial and fungal communities under different treatments were assessed by principal coordinate analysis (PCoA) based on Bray-Curtis distance measure (Figure 2). The first two principal components (PC1 and PC2) together explained 57.91% of variation in bacterial community and 63.20% of variation in fungal community. Soil bacterial and fungal communities were divided into two groups, whereby manure treatments (M, MN, MNP, and MNPK) represented one group, and no manure treatments (CK, N, NP, and NPK) were clustered into the other group. The PERMANOVA analysis also demonstrated significant differences among the treatments regarding soil bacterial and fungal community compositions (P < 0.01; Supplementary Table 2).
Taxonomic biomarkers of soil bacterial and fungal communities
The LEfSe was performed to identify high-dimensional biomarker taxa of soil bacterial and fungal communities with significantly different abundances among different treatments (Figure 3). In total, 29 bacterial and 50 fungal differentially abundant taxa were identified with an LDA threshold of 4.0. For the bacterial communities, Gaiellales (from class to its genus norank_f__norank_o__Gaiellales), Deltaproteobacteria class, and Rokubacteria (from phylum to its genus norank_f__norank_o__Rokubacteriales) were significantly higher in the CK treatment compared with all other treatments. Actinobacteria and Proteobacteria were the most enriched bacterial phyla in the N and NP treatments, respectively. The Acidobacteriia class within Acidobacteria and the Frankiales order within Actinobacteria were significantly enriched in the NPK treatment. The Clostridia class (from class to its genera Clostridium_sensu_stricto_1 and Terrisporobacter) within Firmicutes was significantly enriched in the MNP treatment. The Firmicutes (from phylum to its family Bacillaceae) and Propionibacteriales (from order to its genus Nocardioides) within Actinobacteria were significantly enriched in the MNPK treatment (Figure 3A).
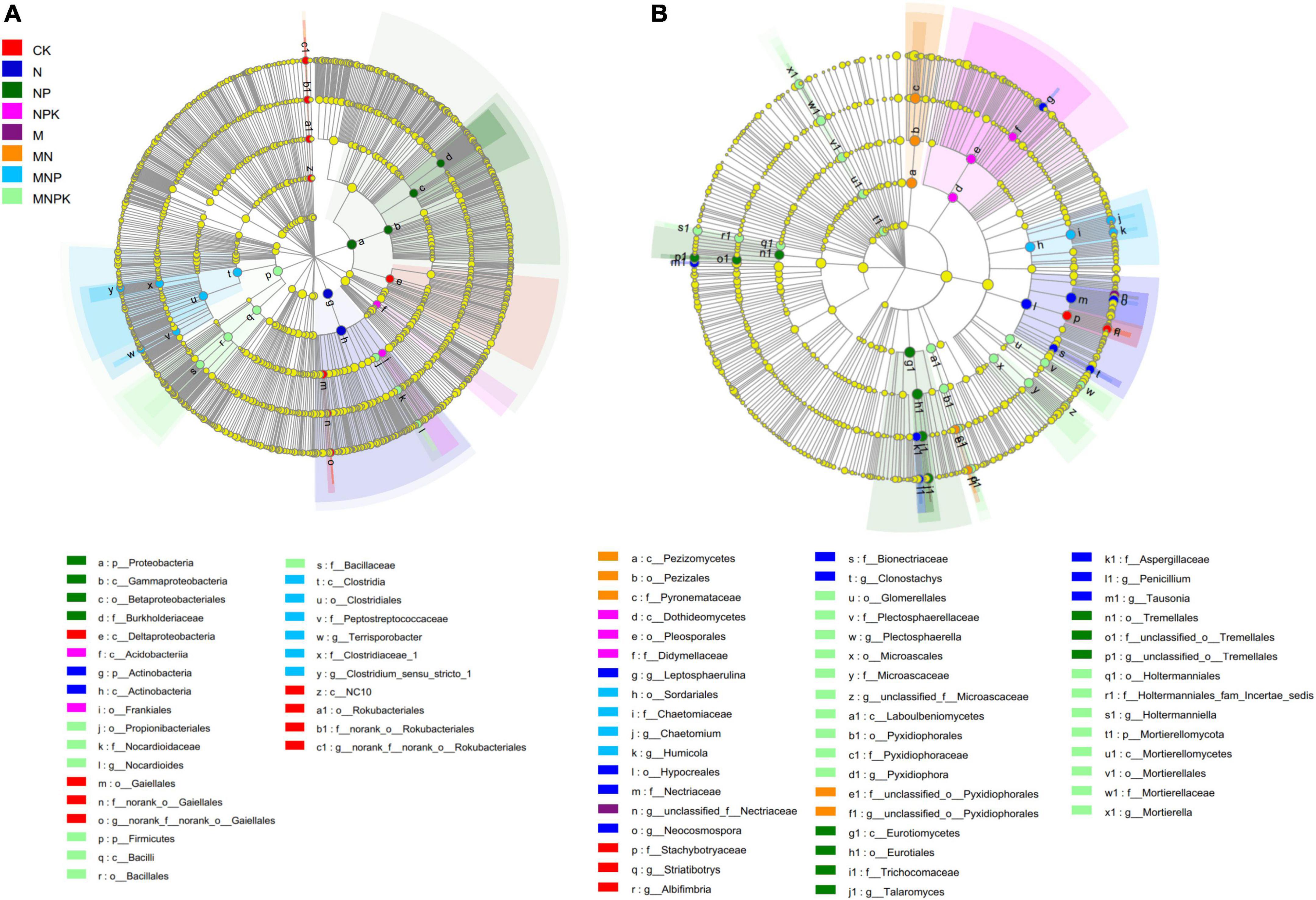
Figure 3. The linear discriminant analysis effect size (LEfSe) showed the significantly different taxa of bacterial communities (A) and fungal communities (B) under different treatments. The threshold of LDA score was 4.0.
Regarding the fungal communities, genera Albifimbria and Striatibotrys within Ascomycota were significantly enriched in the CK treatment. Hypocreales (from order to its genera Clonostachys and Neocosmospora), Aspergillaceae (from family to its genus Penicillium), Leptosphaerulina within Ascomycota, and Tausonia within Basidiomycota were significantly enriched in the N treatment. Eurotiomycetes (from class to its genus Talaromyces) within Ascomycota and Tremellales within Basidiomycota were significantly enriched in the NP treatment. Dothideomycetes and Pezizomycetes (both Ascomycota) were enriched in the NPK and MN treatments, respectively. Sordariales (from order to its genera Chaetomium and Humicola) were significantly higher in the MNP treatment. Only the MNPK treatment had a phylum-level marker, namely Mortierellomycota. Some taxa within Ascomycota and Basidiomycota were significantly enriched in the MNPK treatment (Figure 3B).
Relationships between soil microbial communities and soil properties
To assess how soil properties impacted soil bacterial and fungal community structure in different treatments, Spearman’s correlations using the Mantel test (Table 4) between soil microbial communities and soil properties, and RDA (Figure 4) were performed. The Mantel test results showed soil bacterial community was significantly correlated with SOC, TN, TP, AP, and pH, and soil fungal community was significantly correlated with SOC, TN, TP, NH4+-N, NO3–-N, AP, AK, and pH. The soil properties included in RDA were selected on the basis of their collinearity and strength of their correlations with the soil microbial community structure by VIF < 20 (Lin et al., 2018) and significance (≤0.05) in Monte Carlo permutation test. The first two axes of the RDA explained 57.15 and 50.51% of the total variance in the bacterial and fungal communities, respectively. For the bacterial communities, the most important influencing factors were TP, AP, and pH (Figure 4A). For the fungal communities, the most important influencing factors were pH, SOC, NH4+-N, NO3–-N, and TP (Figure 4B).
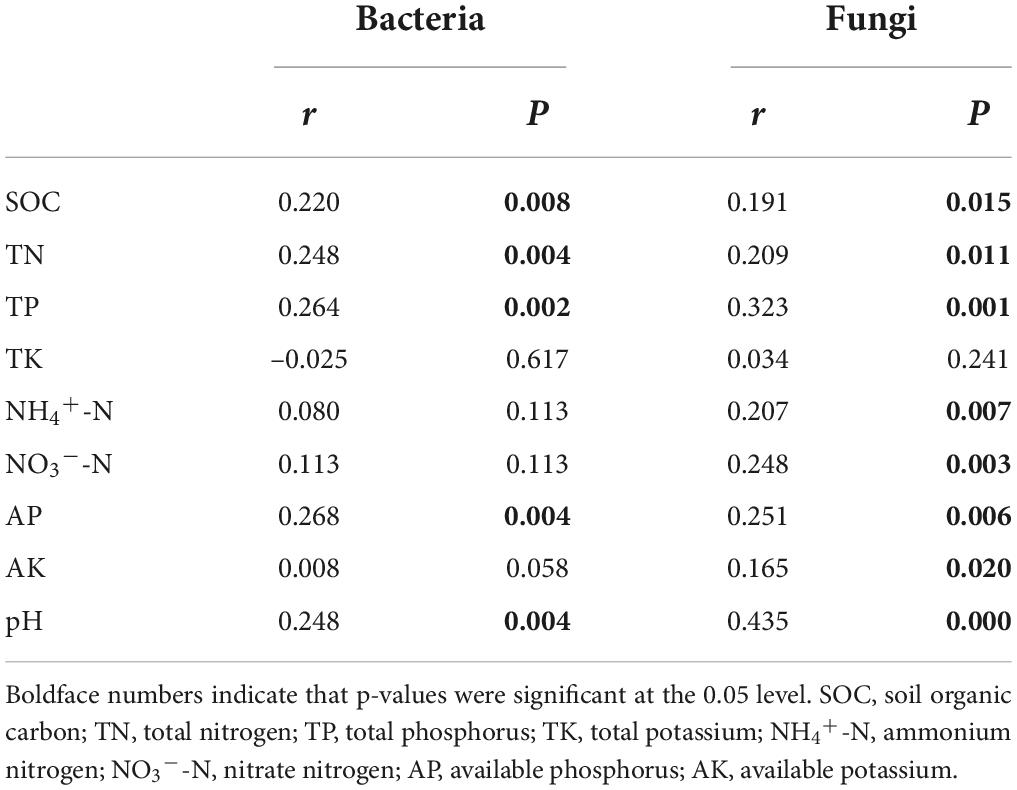
Table 4. Mantel analysis of the relationships between the soil microbial community structure and soil properties.
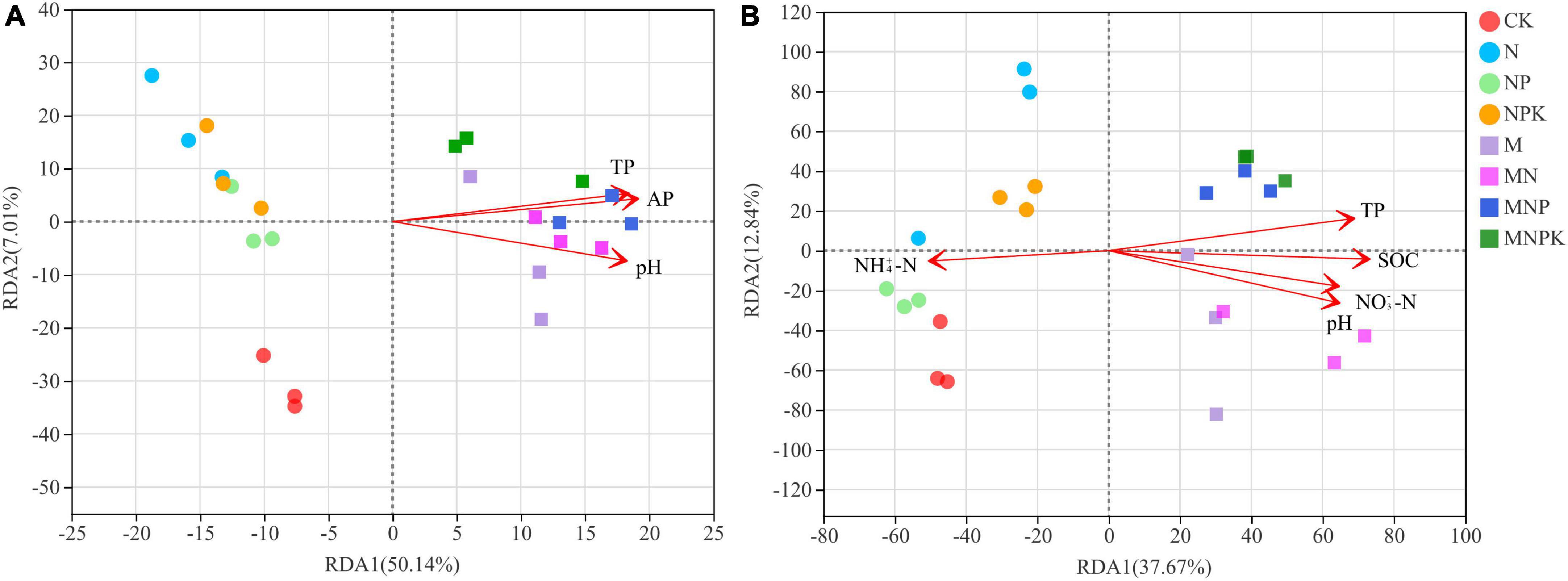
Figure 4. Redundancy analysis (RDA) of the relationship between microbial communities and the soil properties. (A) Bacterial community, (B) fungal community. SOC, soil organic carbon; TP, total phosphorus; NH4+-N, ammonium nitrogen; NO3–-N, nitrate nitrogen; AP, available phosphorus.
The Spearman’s correlation analysis between the soil bacterial community and soil properties showed that the relative abundances of Actinobacteria and Proteobacteria were significantly negatively correlated with SOC (P < 0.001), TN (P < 0.001), TP (P < 0.01), NO3–-N (P < 0.01), AP (P < 0.01), AK (P < 0.01), and pH (P < 0.001). By contrast, the relative abundance of Firmicutes was significantly positively correlated with SOC (P < 0.001), TN (P < 0.001), TP (P < 0.001), NO3–-N (P < 0.001), AP (P < 0.001), AK (P < 0.001), and pH (P < 0.001, Figure 5A). For fungal community, the Spearman’s correlation analysis indicated that the relative abundance of the two most dominant phyla Ascomycota and Basidiomycota did not correlate with soil properties. Nevertheless, the relative abundance of Mortierellomycota had significant positive correlations with SOC (P < 0.001), TN (P < 0.001), TP (P < 0.001), NO3–-N (P < 0.001), AK (P < 0.001), AP (P < 0.001), and pH (P < 0.001), and a significant negative correlation with NH4+-N (P < 0.01, Figure 5B).
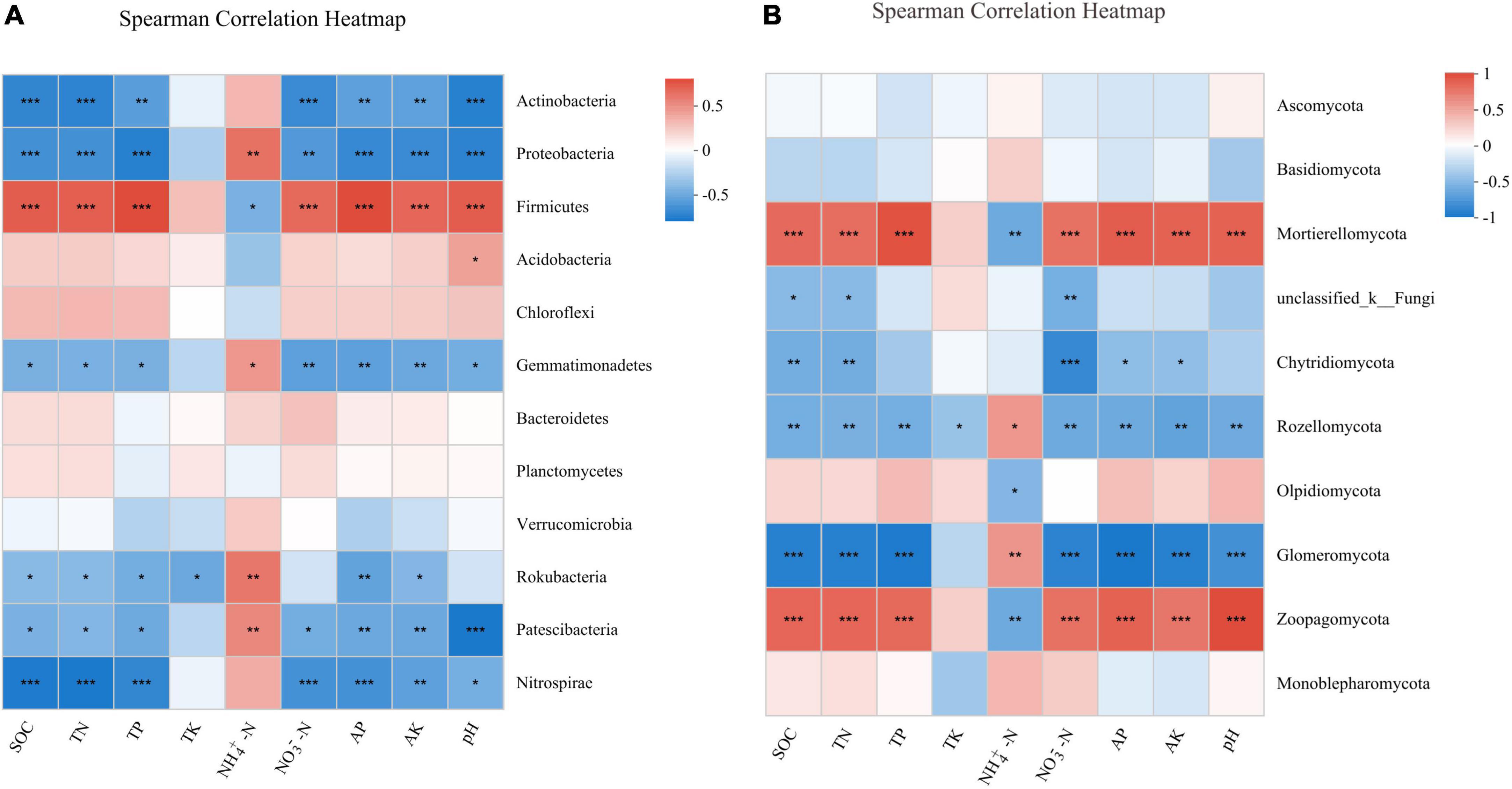
Figure 5. Spearman’s correlation analysis between soil properties and the relative abundance of bacterial (A) and fungal (B) phylum under different treatments. *P < 0.05; **P < 0.01; ***P < 0.001.
Discussion
Long-term fertilization improved soil fertility
As showed in Table 1, the contents of the major soil nutrients (N, P and K) and soil organic carbon were increased after the 12-year application of fertilizers. The contents of SOC, TN, TP, NO3–-N, AP, and AK were significantly higher in the manure treatments than in the chemical fertilizer treatments (P < 0.05), indicating that the effects of pig manure alone or combined with chemical fertilizer on improving soil fertility and accelerating soil nutrient turnover were more significant than those of chemical fertilizer alone, which was in line with previous studies (Wei et al., 2017; Pan et al., 2020). The 12-year chemical fertilization significantly decreased soil pH (P < 0.05), and the soil pH value was lowest in the N treatment. These findings were unsurprising because the ammonium-based N fertilizers (such as urea) are known to acidify soils with poor pH buffering capacity (typical for sandy soils). However, pig manure could effectively alleviate soil acidification (Bei et al., 2018); in the present study, the M treatment had the highest pH value. Pig manure is enriched with organic matter and nutrients including nitrogen, phosphorus, and potassium (Ding et al., 2017; Li et al., 2022); thus our results indicated general improvements in soil fertility after 12 years of combined application of pig manure and chemical fertilizers on aeolian sandy soil.
Effects of fertilization on soil bacterial and fungal diversity
Many previous studies showed that the application of manure alone or combined with chemical fertilizer could enhance soil bacterial diversity (Shen et al., 2015; Chen et al., 2016). In the present study, the ACE indices of bacterial abundance were increased in the manure treatments, which was in line with previous study (Chaudhry et al., 2012). This might be due to the fact that manure application enhanced the availability of carbon substrates in soil and promoted root exudation (Bittman et al., 2005). The bacterial ACE indices were positively correlated with SOC and TN, which also confirmed the above explanation. However, soil bacterial Shannon index was lowest in the MNPK treatment in the present study, followed by the N treatment (Table 2). It might have been due to the disappearance of many bacterial taxa with the application of manure, resulting in a decrease in diversity. The 12-year application of chemical nitrogen fertilizer significantly decreased bacterial diversity in the rhizosphere soil, which might be related to the effects of soil acidification caused by soil N enrichment on microorganisms (Geisseler and Scow, 2014). In addition, nitrogen levels could affect some physiological characteristics of plants, thereby altering root exudates or signals and thus altering diversity of rhizosphere microorganisms (Basal and Szabó, 2018). Contrary to predictions, although fertilization changed soil bacterial diversity, there was no significant correlation between bacterial diversity and soil properties.
A previous study found that long-term organic fertilizers, especially manure, could decrease soil fungal diversity (He et al., 2008). However, Ding et al. (2017) found that inorganic fertilizer combined with manure could weakly improve soil fungal diversity. In our study, the fungal ACE indices were increased in all fertilization treatments except N treatment. The Shannon index was increased in all fertilization treatments except N and NP treatments. Manure application led to higher fungal diversity than chemical fertilization (Table 2). On the one hand, differential results in different studies are likely due to diverse soil types. On the other hand, it might be the application of manure stimulated the growth of fungi. The application of manure provides organic substances, and fungi, as indispensable decomposers, could digest complex organic compounds into bioavailable forms (Xiang et al., 2020). The soil fungal Shannon index was lowest in the N treatment in our study, which was in line with the conclusion that chemical nitrogen fertilizer reduced fungal biodiversity (Zhou et al., 2016). A significant decreasing trend of soil fungal diversity with increasing NH4+-N (R2 = –0.693, P < 0.01) and decreasing pH (R2 = 0.503, P < 0.05) was revealed by Spearman correlation analysis (Table 3). The 12-year application of nitrogen fertilizer significantly reduced soil pH value and increased soil inorganic nitrogen (Table 1). The decrease of soil pH might have been associated with a reduction or disappearance of some fungal taxa due to diminishment of the original habitat suitable for the growth of fungi (Zhou et al., 2016), while the enrichment of nitrogen affected the adaptability of fungi, especially arbuscular mycorrhizal fungi, to the soil environment (Zhang et al., 2021). In contrast to no correlation between the bacterial Shannon index and soil properties, the fungal Shannon index was positively correlated with a range of soil properties (i.e., SOC, TN, TP, TK, AP, AK), indicating that long-term fertilization had a greater impact on rhizosphere soil fungal diversity than bacterial diversity in aeolian sandy soil.
Alterations in soil microbial community composition and structure
Soil microorganisms play an important role in soil nutrient turnover, which in turn affects soil microbial community. In this study, 12-year fertilization significantly affected soil microbial community composition (Figure 1). Twelve bacterial phyla were detected in soil samples, of which two phyla (Actinobacteria and Proteobacteria) dominated in abundance in all treatments, indicating that these bacterial phyla have strong adaptability to the environment. The relative abundance of Actinobacteria and Proteobacteria decreased with the application of manure, whereas that of Firmicutes increased in the manure treatments. The relative abundances of Actinobacteria and Proteobacteria were significantly negatively correlated with SOC, TN, TP, NO3–-N, AP, AK, and pH. By contrast, the relative abundance of Firmicutes was significantly positively correlated with these soil properties (Figure 5). Actinobacteria have high tolerance to environmental stress and can survive in low-pH soil environments (Dai et al., 2018). Therefore, the relative abundance of Actinobacteria was highest in the N treatment, and Actinobacteria was the indicator bacterial taxon for the N treatment (Figure 3A), which was consistent with other studies of Actinobacteria in the rhizosphere soil (e.g., Dai et al., 2018). Regarding different survival strategies in bacterial communities, Proteobacteria and Firmicutes are copiotrophic bacteria (Li et al., 2019). Proteobacteria are closely related to the C and N cycles, and Firmicutes express glycosylated enzymes involved in hydrolysis of cellulose and chitin (Wegner and Liesack, 2016). However, the relative abundance of Proteobacteria was negatively correlated with soil organic carbon content in our study (Figure 5), which might have been caused by the oligotrophic characteristics of its dominant family Burkholderiaceae (Sun et al., 2015; Purkamo et al., 2016), in the present study, the relative abundance of Burkholderiaceae decreased with the application of manure (Figure 1C). Phylogenetic network analysis showed that Firmicutes were negatively correlated with Actinobacteria and Proteobacteria at the family level (Supplementary Figure 3). With the application of manure, Firmicutes proliferated, which might have been another reason for the decrease of the relative abundance of Actinobacteria and Proteobacteria in the manure treatments (Mei et al., 2021). The members of Firmicutes were indicator bacteria of the MNP and MNPK treatments. Bacillaceae were the most abundant family of Firmicutes in the MNPK treatment, and they are well known to contribute to plant growth and health in numerous ways (Tu et al., 2018; Lee et al., 2021), indicating that long-term application of manure enhanced the microbial community of aeolian sandy soil.
For fungal communities, we detected three predominant phyla in soil samples, i.e., Ascomycota, Basidiomycota, and Mortierellomycota. In this study, the relative abundance of Ascomycota was highest in all treatments, and its members were the indicator fungi in each treatment (Figure 3B), which was consistent with Yan et al. (2021). Ascomycota are considered organic decomposers that generally increase in abundance with organic fertilizers application (Ye et al., 2020). However, in the present study, Ascomycota and Basidiomycota were found to have no correlation with soil properties. The relative abundance of Mortierellomycota was increased in the manure treatments and was significantly positively correlated with SOC, TN, TP, NO3–-N, AK, AP, and pH, indicating long-term application of pig manure could increase soil nutrient contents and provide a more suitable habitat for Mortierellomycota. Therefore, as saprophytic fungi, Mortierellomycota benefitted from the application of manure (Cong et al., 2020). Hence, Mortierellomycota (from phylum to genera) were indicator fungal taxa of the MNPK treatment. Narisawa et al. (1998) found that Mortierella were involved in plant pathogen inhibition, alleviating the incidence and severity of Chinese cabbage bulb disease and Fusarium wilt and root rot in banana. The present study proved again that long-term application of chemical fertilizer combined with manure was beneficial to the microbial community of aeolian sandy soil.
The PCoA revealed that fertilization significantly changed soil microbial community structure (Figure 2). Soil bacterial and fungal community compositions were different in the manure treatments and non-manure treatments, which was in agreement with Pan et al. (2020). The RDA results showed that soil properties had a large impact on the changes in soil bacterial and fungal community composition (Figure 4), indicating that these soil properties played a decisive role in the soil microbial community structure. Soil bacteria community structure was significantly influenced by soil properties, such as AP, TP, and pH (Figure 4A). Spearman’s correlation analysis demonstrated that most bacteria were associated with these soil properties (Figure 5A). Similarly, a range of soil properties (i.e., pH, TP, SOC, NH4+-N, and NO3–-N) contributed to shaping the fungal community structure (Figure 4B). In this study, soil TP and pH were the main driving factors influencing both bacterial and fungal communities. Phosphorus is an important nutrient for the growth of bacteria and fungi and can directly influence the composition and functioning of soil microbial community (Richardson et al., 2011; Liao et al., 2018). Many studies have shown that fungi generally grow optimally over a wide pH range and are therefore less affected by soil pH than bacteria (Rousk et al., 2010; Zhalnina et al., 2015). Contrary to these claims, the soil pH was the main environmental factor driving fungal community changes in our study. This might have been due to a significant positive correlation between the major fungal phylum Mortierellomycota and soil pH. Bradley et al. (2006) found N fertilization caused significant changes in the composition of soil fungal communities. Zhang et al. (2021) showed that an appropriate amount of N could improve the efficiency of mycorrhizal symbiosis. In our study, soil fungal community was affected by soil carbon and nitrogen, which is consistent with the saprophytic status of most fungi (Liu et al., 2015; Ding et al., 2017). To sum up, different fertilization methods altered soil microbial community composition by varying soil properties.
Conclusion
The contents of soil available nutrients and soil organic carbon were higher in the manure treatments compared with those that received chemical fertilizer only. Chemical fertilizer combined with manure increased the diversity of fungi. Long-term fertilization changed soil microbial community structure by altering soil properties. Soil TP, AP, and pH were the most important factors that influenced bacterial taxa, whereas soil pH, SOC, NH4+-N, NO3–-N, and TP were the most important factors influencing fungal taxa after 12-year fertilization in an aeolian sandy soil.
Data availability statement
The datasets presented in this study can be found in online repositories. The names of the repository/repositories and accession number(s) can be found in the article/Supplementary material.
Author contributions
SZ: conceptualization, methodology, investigation, data curation, writing – original draft and review and editing. XL, KC, and JS: investigation and data curation. YW: linguistic modification. PL and JY: visualization and funding acquisition. YuW: investigation, data curation, and supervision. XH: conceptualization, writing – review and editing, supervision, project administration, funding acquisition, and resources. All authors reviewed the manuscript.
Funding
This work was supported by the China Agriculture Research System of MOF and MARA (CARS-13) and the China Scholarship Council (No. 202108857001).
Acknowledgments
We are grateful to Fangfang Cai, Wei Hua, and Jing Peng for their insightful suggestions and comments on this manuscript who are not listed as co-authors.
Conflict of interest
The authors declare that the research was conducted in the absence of any commercial or financial relationships that could be construed as a potential conflict of interest.
Publisher’s note
All claims expressed in this article are solely those of the authors and do not necessarily represent those of their affiliated organizations, or those of the publisher, the editors and the reviewers. Any product that may be evaluated in this article, or claim that may be made by its manufacturer, is not guaranteed or endorsed by the publisher.
Supplementary material
The Supplementary Material for this article can be found online at: https://www.frontiersin.org/articles/10.3389/fmicb.2022.979759/full#supplementary-material
Footnotes
References
Adams, R. I., Miletto, M., Taylor, J. W., and Bruns, T. D. (2013). Dispersal in microbes: Fungi in indoor air are dominated by outdoor air and show dispersal limitation at short distances. ISME J. 7, 1262–1273. doi: 10.1038/ismej.2013.28
Basal, O., and Szabó, A. (2018). The effects of drought and nitrogen on soybean (Glycine max (L.) Merrill) physiology and yield. Int. J. Agric. Biosyst. Engin. 12, 260–265. doi: 10.5281/zenodo.1474431
Bei, S. K., Zhang, Y. L., Li, T. T., Christie, P., Li, X. L., and Zhang, J. L. (2018). Response of the soil microbial community to different fertilizer inputs in a wheat-maize rotation on a calcareous soil. Agric. Ecosyst. Environ. 260, 58–69. doi: 10.1016/j.agee.2018.03.014
Berg, G. (2009). Plant-microbe interactions promoting plant growth and health: Perspectives for controlled use of microorganisms in agriculture. Appl. Microbiol. Biotechnol. 84, 11–18. doi: 10.1046/j.1439-037x.1999.00294.x
Bittman, S., Forge, T. A., and Kowalenko, C. G. (2005). Responses of the bacterial and fungal biomass in a grassland soil to multi-year applications of dairy manure slurry and fertilizer. Soil Biol. Biochem. 37, 613–623. doi: 10.1016/j.soilbio.2004.07.038
Bradley, K., Drijber, R. A., and Knops, J. (2006). Increased N availability in grassland soils modifies their microbial communities and decreases the abundance of arbuscular mycorrhizal fungi. Soil Biol. Biochem. 38, 1583–1595. doi: 10.1016/j.soilbio.2005.11.011
Chaparro, J. M., Sheflin, A. M., Manter, D. K., and Vivanco, J. M. (2012). Manipulating the soil microbiome to increase soil health and plant fertility. Biol. Fertil. Soils 48, 489–499. doi: 10.1007/s00374-012-0691-4
Chaudhry, V., Rehman, A., Mishra, A., Chauhan, P. S., and Nautiyal, C. S. (2012). Changes in bacterial community structure of agricultural land due to long-term organic and chemical amendments. Microb. Ecol. 64, 450–460. doi: 10.1007/s00248-012-0025-y
Chen, C., Zhang, J. N., Lu, M., Qin, C., Chen, Y. H., Yang, L., et al. (2016). Microbial communities of an arable soil treated for 8 years with organic and inorganic fertilizers. Biol. Fertil. Soils 52, 455–467. doi: 10.1007/s00374-016-1089-5
Cong, P., Wang, J., Li, Y. Y., Liu, N., Dong, J. X., Pang, H. C., et al. (2020). Changes in soil organic carbon and microbial community under varying straw incorporation strategies. Soil Tillage Res. 204:104735. doi: 10.1016/j.still.2020.104735
Dai, Z., Su, W., Chen, H., Barber’an, A., Zhao, H., Yu, M., et al. (2018). Long-term nitrogen fertilization decreases bacterial diversity and favors the growth of Actinobacteria and Proteobacteria in agroecosystems across the globe. Global Change Biol. 24, 3452–3461. doi: 10.1111/gcb.14163
Ding, J. L., Jiang, X., Guan, D. W., Zhao, B. S., Ma, M. C., Zhou, B. K., et al. (2017). Influence of inorganic fertilizer and organic manure application on fungal communities in a long-term field experiment of Chinese mollisols. Appl. Soil Ecol. 111, 114–122. doi: 10.1016/j.apsoil.2016.12.003
Francioli, D., Schulz, E., Lentendu, G., Wubet, T., Buscot, F., and Reitz, T. (2016). Mineral vs. organic amendments: Microbial community structure, activity and abundance of agriculturally relevant microbes are driven by long-term fertilization strategies. Front. Microbiol. 7:1446. doi: 10.3389/fmicb.2016.01446
Geisseler, D., and Scow, K. M. (2014). Long-term effects of mineral fertilizers on soil micro- organisms-a review. Soil Biol. Biochem. 75, 54–63. doi: 10.3389/fmicb.2016.01446
Hartmann, M., Frey, B., Mayer, J., Mäder, P., and Widmer, F. (2015). Distinct soil microbial diversity under long-term organic and conventional farming. ISME J. 9, 1177–1194. doi: 10.1038/ismej.2014.210
He, J. Z., Zheng, Y., Chen, C. R., He, Y. Q., and Zhang, L. M. (2008). Microbial composition and diversity of an upland red soil under long-term fertilization treatments as revealed by culture-dependent and culture-independent approaches. J. Soil Sediment. 8, 349–358. doi: 10.1007/s11368-008-0025-1
Hu, X. J., Liu, J. J., Wei, D., Zhu, P., Cui, X., Zhou, B. K., et al. (2017). Effects of over 30-year of different fertilization regimes on fungal community compositions in the black soils of northeast China. Agric. Ecosyst. Environ. 248, 113–122. doi: 10.1016/j.agee.2017.07.03
Hu, X. J., Liu, J. J., Zhu, P., Wei, D., Jin, J., Liu, X. B., et al. (2018). Long-term manure addition reduces diversity and changes community structure of diazotrophs in a neutral black soil of Northeast China. J. Soil Sediment. 18, 2053–2062. doi: 10.1007/s11368-018-1975-6
Lee, S. M., Kong, H. G., Song, G. C., and Ryu, C. M. (2021). Disruption of firmicutes and actinobacteria abundance in tomato rhizosphere causes the incidence of bacterial wilt disease. ISME J. 15, 330–347. doi: 10.1038/s41396-020-00785-x
Li, T., Li, Y. Z., Gao, X. C., Wang, S. N., Wang, Z. T., Liu, Y., et al. (2021). Rhizobacterial communities and crop development in response to long-term tillage practices in maize and soybean fields on the loess plateau of China. Catena 202:105319. doi: 10.1016/j.catena.2021.105319
Li, X., Wen, Q. X., Zhang, S. Y., Li, N., Yang, J. F., Romanyà, J., et al. (2022). Long-term changes in organic and inorganic phosphorus compounds as affected by long-term synthetic fertilisers and pig manure in arable soils. Plant Soil 472, 239–255. doi: 10.1007/s11104-021-05225-z
Li, Y., Nie, C., Liu, Y. H., Du, W., and He, P. (2019). Soil microbial community composition closely associates with specific enzyme activities and soil carbon chemistry in a long-term nitrogen fertilized grassland. Sci. Total Environ. 654, 264–274. doi: 10.1016/j.scitotenv.2018.11.031
Liao, H., Zhang, Y. C., Zou, Q. Y., Du, B. B., Chen, W. L., Wei, D., et al. (2018). Contrasting responses of bacterial and fungal communities to aggregate-size fractions and long-term fertilizations in soils of northeastern China. Sci. Total Environ. 635, 784–792. doi: 10.1016/j.scitotenv.2018.04.168
Lin, Y. X., Ye, G. P., Liu, D. Y., Ledgard, S., Luo, J. F., Fan, J. B., et al. (2018). Long-term application of lime or pig manure rather than plant residues suppressed diazotroph abundance and diversity and altered community structure in an acidic ultisol. Soil Biol. Biochem. 123, 218–228. doi: 10.1016/j.soilbio.2018.05.018
Liu, J. J., Sui, Y. Y., Yu, Z. H., Shi, Y., Chu, H. Y., Jin, J., et al. (2015). Soil carbon content drives the biogeographical distribution of fungal communities in the black soil zone of northeast China. Soil Biol. Biochem. 83, 29–39. doi: 10.1016/j.soilbio.2015.01.009
Lu, R. K. (1999). Analytical methods of soil agricultural chemistry. Beijing: China Agriculture Science and Technology Press.
Mei, N., Zhang, X. Z., Wang, X. Q., Peng, C., Gao, H. J., Zhu, P., et al. (2021). Effects of 40 years applications of inorganic and organic fertilization on soil bacterial community in a maize agroecosystem in northeast China. Eur. J. Agron. 130:126332. doi: 10.1016/j.eja.2021.126332
Narisawa, K., Tokumasu, S., and Hashiba, T. (1998). Suppression of clubroot formation in Chinese cabbage by the root endophytic fungus, heteroconium chaetospira. Plant Pathol. 47, 206–210. doi: 10.1046/j.1365-3059.1998.00225.x
Nicolitch, O., Colin, Y., Turpault, M. P., and Uroz, S. (2016). Soil type determines the distribution of nutrient mobilizing bacterial communities in the rhizosphere of beech trees. Soil Biol Biochem. 103, 429–445. doi: 10.1016/j.soilbio.2016.09.018
Pan, H., Chen, M. M., Feng, H. J., Wei, M., Song, F. P., Lou, Y. H., et al. (2020). Organic and inorganic fertilizers respectively drive bacterial and fungal community compositions in a fluvo-aquic soil in northern China. Soil Till. Res. 198:104540. doi: 10.1016/j.still.2019.104540
Purkamo, L., Bomberg, M., Kietäväinen, R., Salavirta, H., Nyyssönen, M., Nuppunen-Puputti, M., et al. (2016). Microbial co-occurrence patterns in deep Precambrian bedrock fracture fluids. Biogeosciences 13, 3091–3108. doi: 10.5194/bg-13-3091-2016
Richardson, A. E., Lynch, J. P., Ryan, P. R., Delhaize, E., Smith, F. A., Smith, S. E., et al. (2011). Plant and microbial strategies to im- prove the phosphorus efficiency of agriculture. Plant Soil 349, 121–156. doi: 10.1007/s11104-011-0950-4
Rousk, J., Bååth, E., Brookes, P. C., Lauber, C. L., Lozupone, C., Caporaso, J. G., et al. (2010). Soil bacterial and fungal communities across a pH gradient in an arable soil. ISME J. 4, 1340–1351. doi: 10.1038/ismej.2010.58
Shen, Z. Z., Ruan, Y. Z., Chao, X., Zhang, J., Li, R., and Shen, Q. R. (2015). Rhizosphere microbial community manipulated by 2 years of consecutive biofertilizer application associated with banana fusarium wilt disease suppression. Biol. Fertil. Soils 51, 553–562. doi: 10.1007/s00374-015-1002-7
Su, J. Q., Ding, L. J., Xue, K., Yao, H. Y., Quensen, J., Bai, S. J., et al. (2015). Long-term balanced fertilization increases the soil microbial functional diversity in a phosphorus-limited paddy soil. Microb. Ecol. 24, 136–150. doi: 10.1111/mec.13010
Sun, R. B., Dsouza, M., Gilbert, J. A., Guo, X. S., Wang, D. Z., Guo, Z. B., et al. (2016). Fungal community composition in soils subjected to long-term chemical fertilization is most influenced by the type of organic matter. Environ. Microbiol. 18, 5137–5150. doi: 10.1111/1462-2920.13512
Sun, R. B., Zhang, X. X., Guo, X. S., Wang, D. Z., and Chu, H. Y. (2015). Bacterial diversity in soils subjected to long-term chemical fertilization can be more stably maintained with the addition of livestock manure than wheat straw. Soil Biol. Biochem. 88, 9–18. doi: 10.1016/j.soilbio.2015.05.007
Tu, J., Qiao, J., Zhu, Z. W., Li, P., and Wu, L. C. (2018). Soil bacterial community responses to long-term fertilizer treatments in paulownia plantations in subtropical China. Appl. Soil Ecol. 124, 217–326. doi: 10.1016/j.apsoil.2017.09.036
Van Der Heijden, M. G., Bardgett, R. D., and Van Straalen, N. M. (2008). The unseen majority: Soil microbes as drivers of plant diversity and productivity in terrestrial ecosystems. Ecol. Lett. 11, 296–310. doi: 10.1111/j.1461-0248.2007.01139.x
Wang, J., Rhodes, G., Huang, Q., and Shen, Q. (2018). Plant growth stages and fertilization regimes drive soil fungal community compositions in a wheat-rice rotation system. Biol. Fertil. Soils 54, 731–742. doi: 10.1007/s00374-018-1295-4
Wang, J. L., Liu, K. L., Zhao, X. Q., Zhang, H. Q., and Shen, R. F. (2021). Balanced fertilization over four decades has sustained soil microbial communities and improved soil fertility and rice productivity in red paddy soil. Sci. Total Environ. 793:148664. doi: 10.1016/j.scitotenv.2021.148664
Wang, Y. J., Liu, L., Yang, J. F., Duan, Y. M., Luo, Y., and Taherzadeh, M. J. (2020). The diversity of microbial community and function varied in response to different agricultural residues composting. Sci. Total Environ. 715:136983. doi: 10.1016/j.scitotenv.2020.136983
Wegner, C. E., and Liesack, W. (2016). Microbial community dynamics during the early stages of plant polymer breakdown in paddy soil. Environ. Microbiol. 18, 2825–2842. doi: 10.1111/1462-2920.12815
Wei, M., Hu, G. Q., Wang, H., Bai, E., Lou, Y. H., Zhang, A. J., et al. (2017). 35 years of manure and chemical fertilizer application alters soil microbial community composition in a Fluvo-aquic soil in northern China. Eur. J. Soil Biol. 82, 27–34. doi: 10.1016/j.ejsobi.2017.08.002
Wei, X. M., Zhu, Z. K., Wei, L., Wu, J. S., and Ge, T. D. (2019). Biogeochemical cycles of key elements in the paddy-rice rhizosphere: Microbial mechanisms and coupling processes. Rhizosphere 10:100145. doi: 10.1016/j.rhisph.2019.100145
Xiang, X. J., Liu, J., Zhang, J., Li, D. M., Xu, C. X., and Kuzyakov, Y. (2020). Divergence in fungal abundance and community structure between soils under long-term mineral and organic fertilization. Soil Tillage Res. 196:104491. doi: 10.1016/j.still.2019.104491
Xu, N., Tan, G. C., Wang, H. Y., and Gai, X. P. (2016). Effect of biochar additions to soil on nitrogen leaching, microbial biomass and bacterial community structure. Eur. J. Soil Biol. 74, 1–8. doi: 10.1016/j.ejsobi.2016.02.004
Xu, Q. C., Ling, N., Chen, H., Duan, Y. H., Wang, S., Shen, Q. R., et al. (2020). Long- term chemical-only fertilization induces a diversity decline and deep selection on the soil bacteria. mSystems 5, e337–e320. doi: 10.1128/mSystems.00337-20
Yan, T. T., Xue, J. H., Zhou, Z. D., and Wu, Y. B. (2021). Biochar-based fertilizer amendments improve the soil microbial community structure in a karst mountainous area. Sci. Total Environ. 794:148757. doi: 10.1016/j.scitotenv.2021.148757
Yang, Y. R., Li, X. G., Liu, J. G., Zhou, Z. G., Zhang, T. L., and Wang, X. X. (2020). Fungal community structure in relation to manure rate in red soil in southern China. Appl. Soil Ecol. 147:103442. doi: 10.1016/j.apsoil.2019.103442
Ye, G., Lin, Y., Luo, J., Di, H. J., Lindsey, S., Liu, D., et al. (2020). Responses of soil fungal diversity and community composition to long-term fertilization: Field experiment in an acidic Ultisol and literature synthesis. Appl. Soil Ecol. 145:103305. doi: 10.1016/j.apsoil.2019.06.008
Yu, Y. J., Wu, M., Petropoulos, E., Zhang, J. W., Nie, J., Liao, Y. L., et al. (2019). Responses of paddy soil bacterial community assembly to different long-term fertilizations in southeast China. Sci. Total Environ. 656, 625–633. doi: 10.1016/j.scitotenv.2018.11.359
Zhalnina, K., Dias, R., de Quadros, P. D., Davis-Richardson, A., Camargo, F. A., Clark, I. M., et al. (2015). Soil pH determines microbial diversity and composition in the park grass experiment. Microb. Ecol. 69, 395–406. doi: 10.1007/s00248-014-0530-2
Zhang, Q. C., Shamsi, I. H., Xu, D. T., Wang, G. H., Lin, X. Y., Jilani, G., et al. (2012). Chemical fertilizer and organic manure inputs in soil exhibit a vice versa pattern of microbial community structure. Appl. Soil Ecol. 57, 1–8. doi: 10.1016/j.apsoil.2012.02.012
Zhang, S. Y., Luo, P. Y., Yang, J. F., Irfan, M., Dai, J., An, N., et al. (2021). Responses of arbuscular mycorrhizal fungi diversity and community to 41-Year rotation fertilization in brown soil region of northeast China. Front. Microbiol. 12:742651. doi: 10.3389/fmicb.2021.742651
Zhang, Y., Li, P., Liu, X. J., Xiao, L., Shi, P., and Zhao, B. H. (2019). Effects of farmland conversion on the stoichiometry of carbon, nitrogen, and phosphorus in soil aggregates on the Loess Plateau of China. Geoderma 351, 188–196. doi: 10.1016/j.geoderma.2019.05.037
Keywords: bacterial community, fungal community, long-term fertilization, Illumina MiSeq sequencing, aeolian sandy soil
Citation: Zhang S, Li X, Chen K, Shi J, Wang Y, Luo P, Yang J, Wang Y and Han X (2022) Long-term fertilization altered microbial community structure in an aeolian sandy soil in northeast China. Front. Microbiol. 13:979759. doi: 10.3389/fmicb.2022.979759
Received: 27 June 2022; Accepted: 17 August 2022;
Published: 07 September 2022.
Edited by:
Jeanette M. Norton, Utah State University, United StatesReviewed by:
Jia Liu, Jiangxi Academy of Agricultural Sciences (CAAS), ChinaHuike Li, Northwest A&F University, China
Copyright © 2022 Zhang, Li, Chen, Shi, Wang, Luo, Yang, Wang and Han. This is an open-access article distributed under the terms of the Creative Commons Attribution License (CC BY). The use, distribution or reproduction in other forums is permitted, provided the original author(s) and the copyright owner(s) are credited and that the original publication in this journal is cited, in accordance with accepted academic practice. No use, distribution or reproduction is permitted which does not comply with these terms.
*Correspondence: Yue Wang, d2FuZ3l1ZTEwMjhAMTYzLmNvbQ==; Xiaori Han, aGFueHJAc3lhdS5lZHUuY24=