- 1College of Life Science and Technology, Guangxi University, Nanning, China
- 2Guangxi Key Laboratory of Veterinary Biotechnology, Guangxi Veterinary Research Institute, Nanning, China
- 3Key Laboratory of Ecology of Rare and Endangered Species and Environmental Protection, Ministry of Education, Guangxi Normal University, Guilin, China
- 4College of Animal Science and Technology, Guangxi University, Nanning, China
As one of the most environmentally toxic heavy metals, cadmium (Cd) has attracted the attention of researchers globally. In particular, Guangxi, a province in southwestern China, has been subjected to severe Cd pollution due to geogenic processes and anthropogenic activities. Cd can be accumulated in aquatic animals and transferred to the human body through the food chain, with potential health risks. The aim of the present study was to explore the effects of waterborne Cd exposure (0.5 mg/L and 1.5 mg/L) on the intestinal microbiota of mudsnail, Cipangopaludina cathayensis, which is favored by farmers and consumers in Guangxi. Gut bacterial community composition was investigated using high-throughput sequencing of the V3–V4 segment of the bacterial 16S rRNA gene. Our results indicated that C. cathayensis could tolerate low Cd (0.5 mg/L) stress, while Cd exposure at high doses (1.5 mg/L) exerted considerable effects on microbiota composition. At the phylum level, Proteobacteria, Bacteroidetes, and Firmicutes were the dominant phyla in the mudsnail gut microbiota. The relative abundances of Bacteroidetes increased significantly under high Cd exposure (H14) (p < 0.01), with no significant change in the low Cd exposure (L14) treatment. The dominant genera with significant differences in relative abundance were Pseudomonas, Cloacibacterium, Acinetobacter, Dechloromonas, and Rhodobacter. In addition, Cd exposure could significantly alter the pathways associated with metabolism, cellular processes, environmental information processing, genetic information processing, human diseases, and organismal systems. Notably, compared to the L14 treatment, some disease-related pathways were enriched, while some xenobiotic and organic compound biodegradation and metabolism pathways were significantly inhibited in the H14 group. Overall, Cd exposure profoundly influenced community structure and function of gut microbiota, which may in turn influence C. cathayensis gut homeostasis and health.
Introduction
In the wake of rapid industrialization, aquatic ecosystem pollution is becoming severe (Ali et al., 2022). As ubiquitous hazardous pollutants, heavy metals have attracted the attention or researchers globally due to their environmental toxicity. Cadmium (Cd), a non-essential element, usually exists as Cd (II). As one of the most toxic heavy metals, Cd is released into the environment mainly through anthropogenic activities, including electroplating, battery manufacturing, soldering, mining, and agriculture (Burger, 2008). Cd has numerous negative impacts on aquatic animals, including triggering histopathological changes, inducing oxidative stress, causing metabolic disorders, and altering gut microbial community structure (Chang et al., 2019; Liu et al., 2019; Cheaib et al., 2020; Wang et al., 2020a,b). Moreover, Cd is not easily degradable, and can be accumulated in aquatic animals followed by in the human body through the food chain, with potential human health risks (Wang et al., 2022).
Heavy metal pollution is a major environmental issue in China, and heavy metal pollution in aquatic environment is increasing in severity (Chen et al., 2022; Wang et al., 2022). Cd has been identified as one of the major soil contaminants in China (Ministry of Ecology and Environment of the People’s Republic of China, 2014). In particular, Cd contamination in Guangxi province is significantly higher than in other regions in China due to high background geochemical concentrations in the region (Zhao et al., 2015; Wen et al., 2020). In addition, Guangxi province is a key non-ferrous metal production area in China, so that Cd pollution is a major challenge in the province. In early January 2012, the Longjiang River of Guangxi was exposed to serious Cd contamination following an accident, with long-term impacts on the regional aquatic ecosystems (Zhao et al., 2018; Cui et al., 2022). Cd is also the primary heavy metal pollutant in Chinese agricultural land, including paddy soils (Song et al., 2019; Yang et al., 2021). Indeed, people inhabiting such areas with high levels of Cd pollution may be exposed to Cd toxicity, with potential threats to human health (Xu et al., 2018).
The mudsnail, Cipangopaludina cathayensis (phylum Mollusca, Gastropoda, Prosobranchia, Mesogastropoda, Viviparidae, and Cipangopaludina), is a widely distributed species that can be found in Chinese rivers, lakes, ponds, and other water bodies (Lu et al., 2014). C. cathayensis has high protein and low fat content, is rich in umami amino acid, and has high nutritional value, so that it is highly favored among consumers and farmers in China (Luo et al., 2021). Moreover, C. cathayensis flesh has been reported to have diverse biological and physiological properties that are beneficial in human disease prevention and treatment (Wang et al., 2016; Zhao et al., 2021).
Indeed, C. cathayensis is one of the most popular aquatic animals in China. Particularly in Guangxi province, the snail family Viviparidae is a source of key components of a famous snack, “snail rice noodle,” which represents one of the intangible cultural heritages in China (Luo et al., 2021). In recent years, with the continued increase in “snail rice noodle” consumption, demand for mudsnail and its production has been increasing. Paddy field culture is one of the major ways of mudsnail production in Guangxi. However, Cd pollution has been identified as a serious problem in Guangxi paddy soils and aquatic environments (Zhao et al., 2018; Song et al., 2019; Yang et al., 2021). In addition, considering mudsnail is a benthic organism that is closely associated with paddy soil, it could be exposed to high Cd concentrations, with major threats to food safety (Wang P. et al., 2019). At present, only a few studies had explored the adverse impacts of Cd exposure on the snail family Viviparidae. In addition, current studies have largely focused on the oxidative stress caused by Cd exposure (Hu and Tang, 2012; Zhou and Luo, 2018), so that further investigations on other adverse effects on snails need to be carried out.
The microbiomes associated with aquatic animals, particularly their gut systems, not only participate in digestion but also influence nutrition, growth, reproduction, the immune system, and host vulnerability to disease (Talwar et al., 2018; Chang et al., 2019; Paul and Small, 2019; Duan et al., 2020; Wang et al., 2020a; Diwan et al., 2021). Cd exposure has been reported to significantly affect the gut microbiota of numerous aquatic organisms (Chang et al., 2019; Wang et al., 2020b; Zhang Y. et al., 2020). However, the effects of Cd exposure on the intestinal microbiota of C. cathayensis remain unclear. To address the knowledge gap, in the present study, C. cathayensis individuals were exposed to two doses (0.5 mg/L and 1.5 mg/L) of cadmium chloride (CdCl2⋅2.5H2O) for > 14 days. The aim of the present study was to investigate the effect of Cd on C. cathayensis gut microbiota composition and diversity.
Materials and methods
Ethics statement
The experimental protocol for snail acclimation and experimentation was approved by the Animal Ethics committee of Guangxi Normal University, Guilin, Guangxi, China (No. 202207-02).
Experimental snail and treatment
Adult snails (C. cathayensis) were obtained from Juhe Agricultural Development Cooperatives (25.75° N, 109.38° E), Sanjiang District, Liuzhou City, Guangxi, China. They were then transferred to the laboratory, and acclimated to the experimental conditions at a temperature 24.0 ± 1.0°C, under a 12-h/12-h light/dark cycle in a 50-L (65 × 41 × 20 cm) plastic tank for 2 weeks. During the acclimation period, specimens were fed with commercial ground fish food (Tongwei, Chengdu, Sichuan, China) once a day at 0.5% of their body weight. The tank water was changed partially (30%) every day.
After a 2-week acclimation period, 225 snails were divided randomly into three groups and placed in plastic tanks, with three replicates (25 snails per tank) in each treatment. CdCl2⋅2.5H2O (Silong, Shantou, Guangdong, China) was dissolved in deionized water to prepare stock solution with a final concentration of 900 mg/L. The 0.5 mg/L and 1.5 mg/L Cd doses were selected according to previous studies (Hu and Tang, 2012). The three treatments in the present study included the control treatment (CK14: with no Cd supplementation), low Cd concentration exposure treatment (L14: 0.5 mg/L), and high Cd concentration exposure treatment (H14: 1.5 mg/L). Other experimental conditions were consistent with those in the acclimation phase. During the experimental period, one-third of the water in the tank was replaced every day by adding fresh water or water with a similar concentration. The experiment lasted 2 weeks, as severe mortality occurred at 14 -day in the H14 treatment (Supplementary Table S1).
Sample collection
Snail intestine samples were collected on day 14 and used to determine gut microbiota composition and diversity. The guts of three snails were pooled as a single sample, to ensure sample adequacy, with three biological replicates in each treatment. Briefly, the samples were wiped with 75% ethanol before the snails were removed from the shell. Subsequently, the snails were dissected and the guts extracted and rinsed with sterile water three times. The gut samples were flash frozen using liquid nitrogen and stored at −80°C for subsequent analyses.
DNA extraction, bacterial 16S rRNA amplification, and sequencing
Total genomic DNA (gDNA) of the gut microbiota were extracted using a Fast DNA SPIN Extraction Kit (MP Biomedicals, United States) according to the manufacturer’s protocol. The V3–V4 regions of the bacterial 16S rRNA genes were amplified by PCR using universal bacterial primers (338F: 50-ACTCCTACGGGAGGGAGCA-30, 806R: 50-GGACTACHVGGGTWTCTAAT-30). The PCR cycle conditions for each sample were as follows: an initial denaturation at 95°C for 5 min; 25 cycles of denaturation at 95°C for 30 s, annealing at 55°C for 30 s, and extension at 72°C for 30 s, with a final extension at 72°C for 5 min. PCR products were purified and quantified using an AxyPrep DNA Gel Extraction Kit (Axygen, Union City, NJ, United States) and a Quant-iT PicoGreen dsDNA Assay Kit (Invitrogen, Waltham, MA, United States), respectively. A TruSeq Nano DNA LT Library Prep Kit (Illumina, United States) was used to establish the DNA library. The library was sequenced using a MiSeq Reagent Kit v3 (6,000-cycles-PE) (Illumina, United States) on a MiSeq platform by Personal Biotechnology Co., Ltd. (Shanghai, China). The raw reads were deposited into the NCBI Sequence Read Archive database (PRJNA837347).
Sequence processing
The sequencing data were processed using Quantitative Insights Into Microbial Ecology 2 (QIIME2 v2019.41). Briefly, Cutadapt (version 3.7) was used to filter and trim PCR primers from the raw reeds. DADA2 was used for quality control (Callahan et al., 2016), removing chimera sequences, and determining the sequence variants. Taxonomy was assigned using the DADA2 pipeline, which implements the Naive Bayesian Classifier using the DADA2 default parameters based on the Greengenes database (Release 13.82). Subsequently, the sequences were rarefied using the feature-table rarefy command in QIIME2.
Data analysis
All sequence analysis steps were performed using QIIME2 and R v3.2.0 (R Foundation for Statistical Computing, Vienna, Austria). The rarefaction curve was generated based on Amplicon Sequence Variants (ASVs) at a 97% similarity cut-off level. For alpha diversity analyses, Chao 1, Observed_species, Shannon, and Simpson indices were calculated using QIIME2 (for calculation methods3). Significance between groups was tested using the Kruskal–Wallis H test and the Dunn test. Beta diversity was calculated using weighted Bray-Curtis distance matrix and visualized with Principal Coordinates Analysis (PCoA). Hierarchical clustering using Bray–Curtis distances based on the relative abundances of species was performed to cluster the dataset. A Venn diagram was drawn using the “VennDiagram” package in R v3.2.0 (R Statistical Foundation). The functional profiles of microbial communities were predicted using PICRUSt2 (Phylogenetic Investigation of Communities by Reconstruction of Unobserved States4). The predicted genes and their respective functions were annotated using the Kyoto Encyclopedia of Genes and Genomes (KEGG) database5. Differences between populations were analyzed using one-way Analysis of Variance. Results were considering statistically significant at p < 0.05. The values are expressed as mean ± SD (Standard deviation).
Results
Relative abundance
After normalization, there were 833,989 sequences across all snail gut contents sampled, with an average of 92,665 sequences per sample (minimum of 55,824 sequences per sample and maximum of 145,373 sequences per sample, see Supplementary Table S2). Rarefaction curves indicated that all samples reached the saturation phase (Supplementary Figure S1). There were 14,951 ASVs derived from all samples; the CK14, L14, and H14 treatments had 4,625, 5,121, and 5,205 ASVs per sample, respectively. Moreover, 859 ASVs were shared among the three treatments, while 2,010, 2,029, and 2,386 ASVs were unique to the CK14, L14, and H14 treatments, respectively (Figure 1).
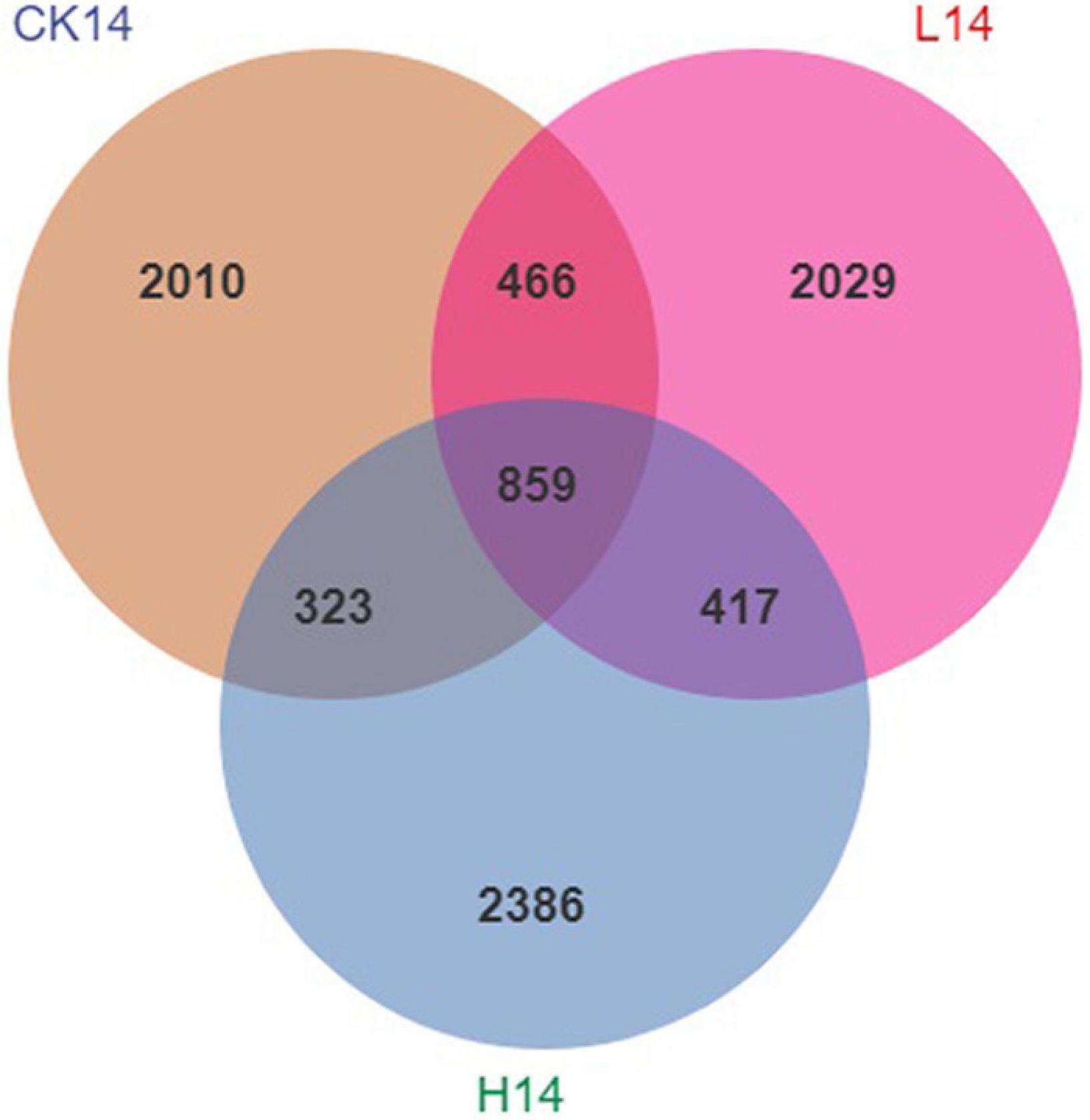
Figure 1. Venn diagram analysis depicting the numbers of shared and unique Amplicon Sequence Variants (ASVs) among the control (CK14), 0.5 mg/L (L14), and H14 (1.5 mg/L) treatments.
Intestinal microflora diversity
To compare bacterial community diversity across different groups, alpha-diversity and beta-diversity were evaluated. There were no significant differences in Chao 1 index, Observed_species index, Shannon index, and Simpson index among the three groups (p > 0.05) (Figure 2A and Supplementary Table S3). In a beta-diversity analysis (PCoA based on Bray–Curtis), the L14 and CK14 treatments were clustered together and could not be distinguished, whereas the H14 group was distinct from the L14 and CK14 groups, with the following main principal component (PC) scores: PC1 = 48.1%, PC2 = 25.7% (Figure 2B). In addition, according to the hierarchical clustering tree results, ASVs from C. cathayensis in the high Cd exposure group were clustered in one group based on similarity, while the control and low Cd exposure groups clustered into one independent group, excluding one control sample (Figure 2C). The results indicate that the high Cd exposure treatment had more severe effects on the diversity of the C. cathayensis microbiome than the low Cd exposure treatment.
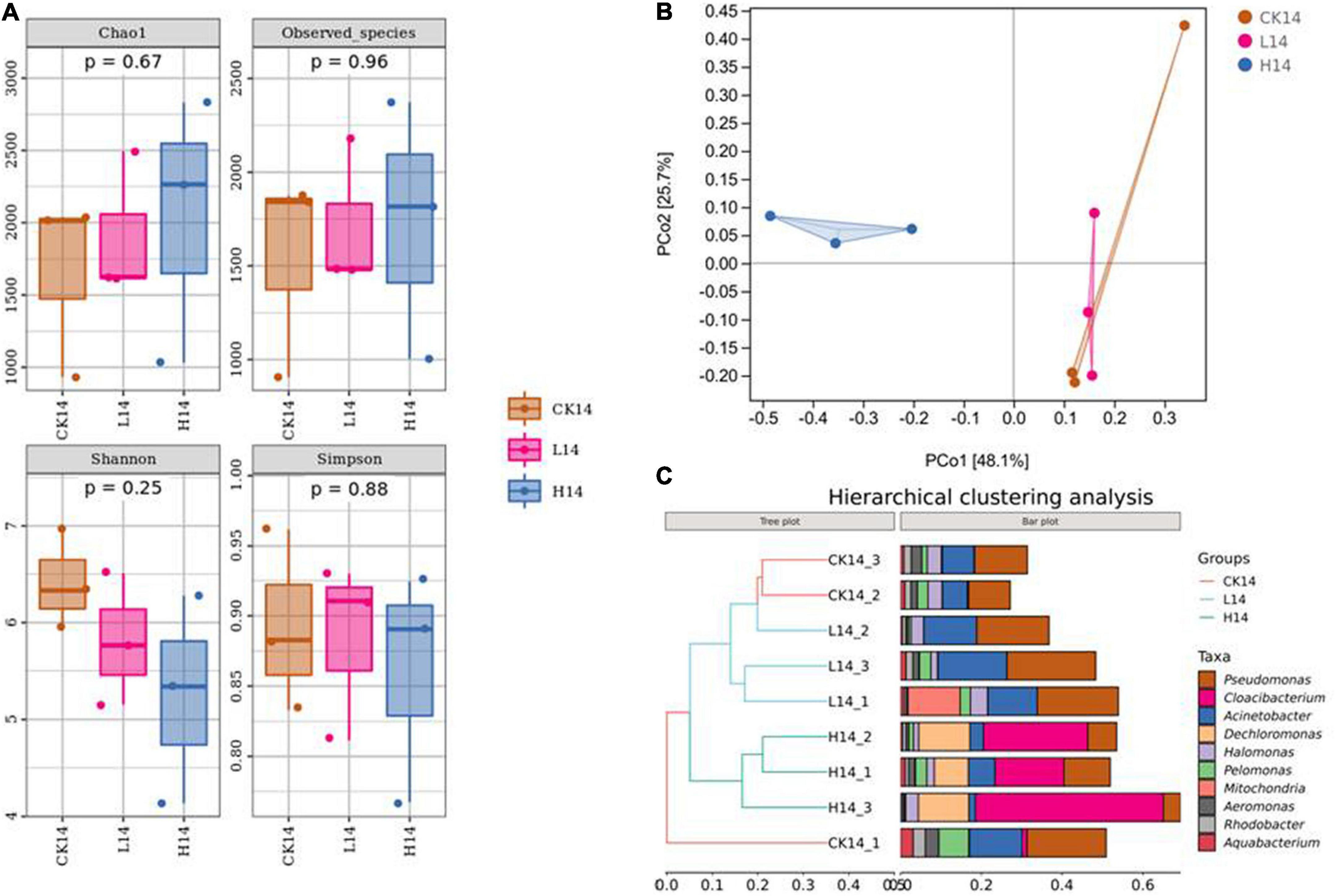
Figure 2. Intestinal microbiome diversity in the control (CK14), 0.5 mg/L (L14) and H14 (1.5 mg/L) groups. (A) α-diversity comparisons in the intestinal microflora among the CK14, L14, and H14 groups. (B) Bray–Curtis distances were calculated and visualized through Principal Coordinate Analysis (PCoA) (Ellipses were drawn with 95% confidence intervals). (C) Hierarchical cluster analysis of the Bray–Curtis distances generated from taxa tables showed Amplicon Sequence Variant (ASV) similarity across microbial communities among different groups.
Gut microbiota community structure
In total, 25 phyla, 50 classes, 115 orders, 185 families, 324 genera, and 90 species were identified. At the phylum level, Proteobacteria was the most abundant phylum across all three treatments (51.9% in CK14, 55.2% in L14, and 38.9% in H14), the other two prevalent phyla were Bacteroidetes and Firmicutes (Figure 3A and Supplementary Table S4). In addition, Bacteroidetes abundance in the H14 treatment was significantly higher than that in the C14 treatment (p < 0.01), although there was no significant difference between the L14 and control treatments (Figure 3A and Supplementary Figure S2a). At the genus level, Pseudomonas, Cloacibacterium, Acinetobacter, Dechloromonas, Halomonas, Pelomonas, Mitochondria, Aeromonas, Rhodobacter, and Aquabacterium were the dominant (Figure 3B and Supplementary Table S5). Pseudomonas relative abundance was higher in the L14 treatment than in the C14 treatment, although the difference was not significant (Figure 3B and Supplementary Figure S2b). Conversely, Pseudomonas relative abundance was lower in the H14 treatment than in the C14 treatment, although the difference was not significant (Figure 3B and Supplementary Figure S2b). However, Pseudomonas relative abundance decreased with an increase in Cd concentration (p < 0.01) in the H14. Acinetobacter exhibited a similar trend (Figure 3B and Supplementary Figure S2c). In addition, Rhodobacter relative abundance was significantly lower in the H14 treatment than in the C14 treatment (p < 0.05). Rhodobacter relative abundance was also lower in the L14 treatment than in the C14 treatment, although the difference was not significant (Figure 3B and Supplementary Figure S2d). On the contrary, Cloacibacterium and Dechloromonas were significantly enriched in the H14 treatment (p < 0.05), although there was no significant indifference between the L14 treatment and the CK14 treatment (Figure 3B and Supplementary Figures S2e,f). The results were consistent with beta diversity analysis results (Figures 2B,C). Cd exposure at low doses had minimal effect on snail gut microbial diversity, whereas high Cd stress influenced snail gut microbial diversity considerably.
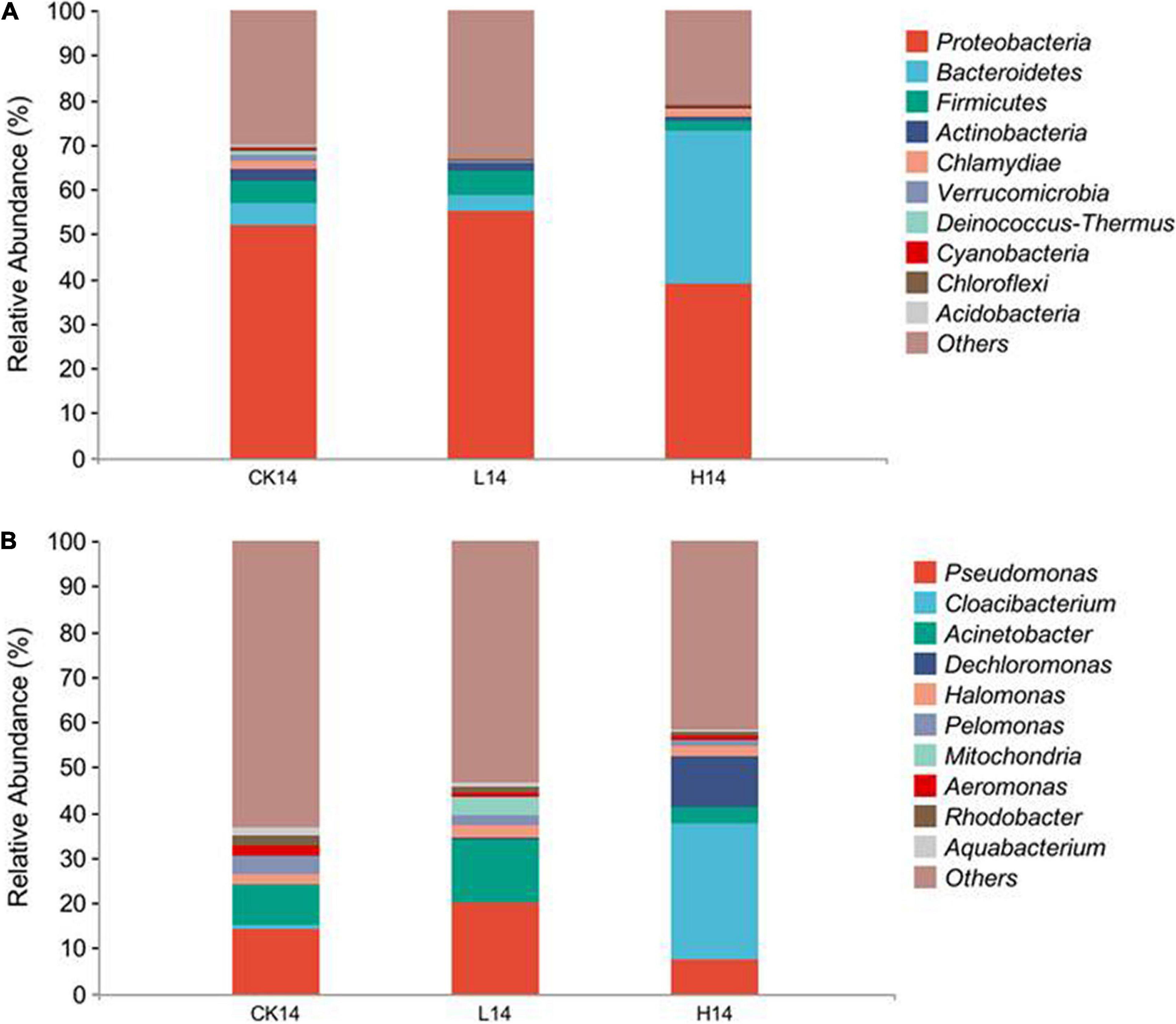
Figure 3. Compositions of the intestinal microflora among the control (CK14), 0.5 mg/L (L14), and H14 (1.5 mg/L) treatments. (A) Compositions of the intestinal microflora at the phylum level. (B) Compositions of the intestinal microflora at the genus level. The top ten abundant genera (higher than 1% in at least one sample) are shown in the figure and the rest are indicated as “Others.”
Prediction of microbial community function
PICRUSt functional prediction and KEGG pathway enrichment analysis results showed that the main functional categories included five cellular processes pathways, three environmental information processing pathways, four genetic information processing pathways, five human disease pathways, 11 metabolism pathways, and seven organismal system pathways (Figure 4). In the L14 treatment, the fluorobenzoate degradation pathway was enriched; on the contrary, carotenoid biosynthesis, steroid biosynthesis, and indole alkaloid biosynthesis pathways were significantly down-regulated in the L14 treatment compared with in the control treatment (Figures 5A,B). In the H14 treatment, five pathways (protein digestion and absorption, apoptosis, lysosome, other glycan degradation, and pathways in cancer) were significantly up-regulated, whereas shigellosis and endocytosis pathways were decreased relative to the control group (Figures 5A,C). In addition, notably, compared with in the L14 treatment, some xenobiotic and organic compound biodegradation and metabolism pathways were significantly reduced in the H14 treatment (Figures 5A,D).
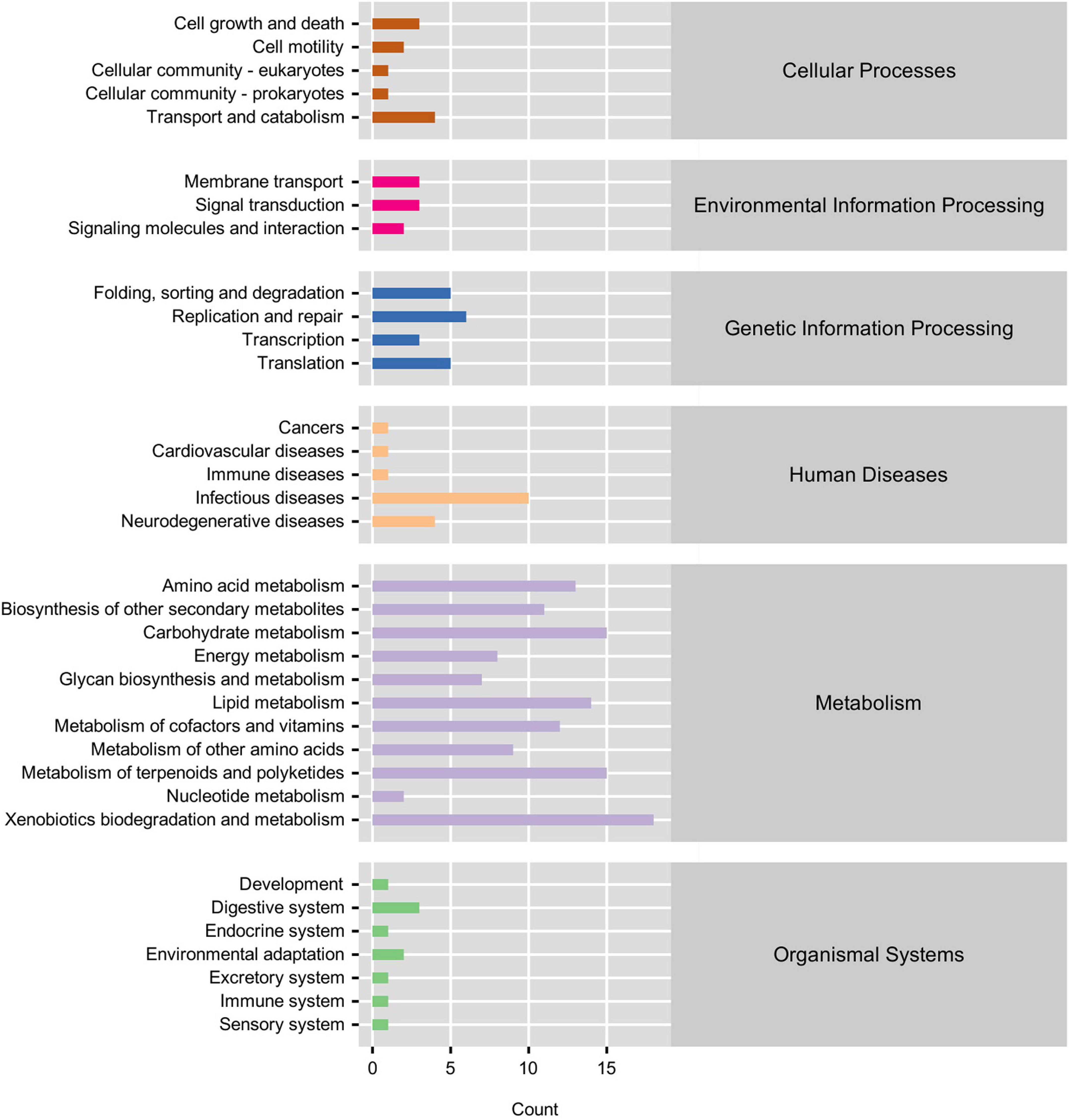
Figure 4. Functional annotations and abundance information about the intestinal microbiota at KEGG level 1 and level 2. The gene function is showed as color-bars (level 1). The detailed pathways are shown on the left side (level 2).
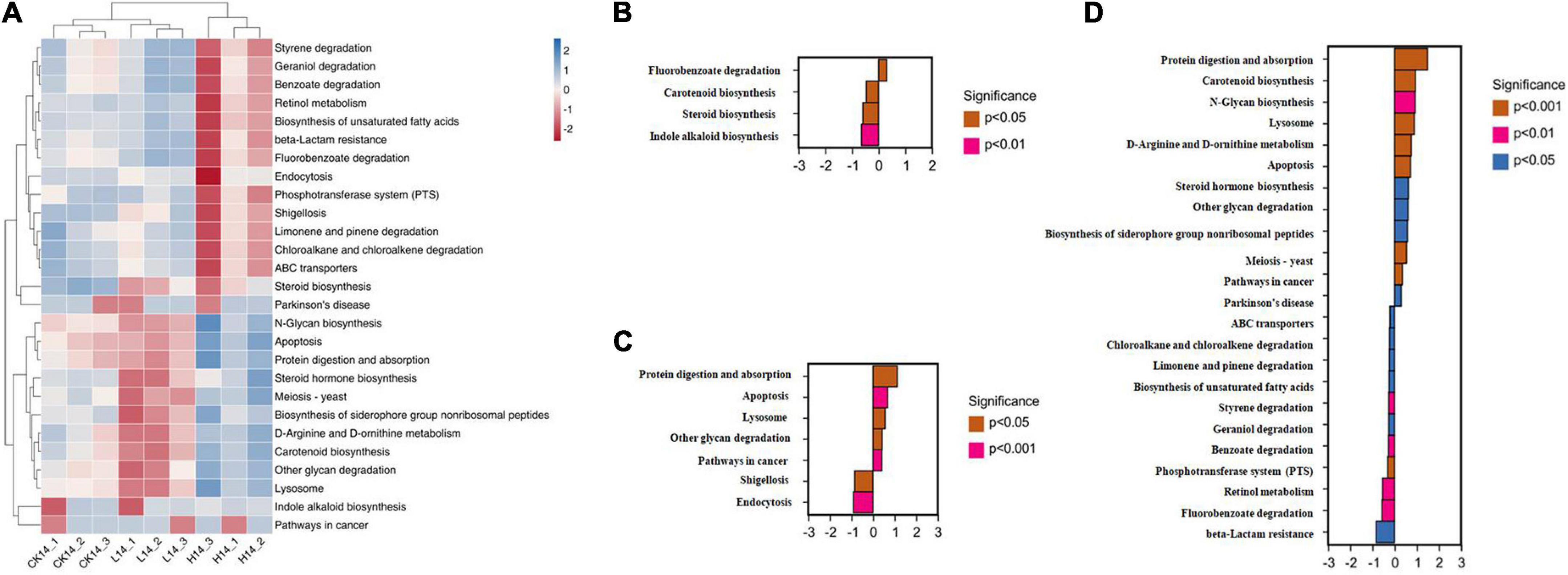
Figure 5. Intestinal microbiota predictive metabolic functions from the Kyoto Encyclopedia of Genes and Genomes (KEGG) database in all samples. (A) Heatmap of the significant differential pathways among the control (CK14), 0.5 mg/L (L14), and H14 (1.5 mg/L) treatments. (B) Significant different pathways between the control and L14 treatments. (C) Significantly different pathways between the control and H14 treatments. (D) Significantly different pathways between the L14 and H14 treatments.
Discussion
Intestinal microbial diversity
Cadmium is undoubtedly an environmental contaminant. Previous studies have demonstrated that Cd exposure could alter intestinal flora composition in aquatic animals (Chang et al., 2019; Wang et al., 2020b; Zhang Y. et al., 2020). In the present study, intestinal microbiota in C. cathayensis was investigated using high-throughput 16S rRNA gene sequencing. Our results suggested no significant difference in alpha diversity among the three treatments (Figure 2A). The results are inconsistent with the findings of previous studies that have reported that Cd exposure altered the alpha diversity of gut microbiota (Ya et al., 2019; Zhang Y. et al., 2020), even under relatively low Cd concentration (Chang et al., 2019). Furthermore, the PCoA analysis results showed that gut microbial community structure in the high Cd exposure treatment was distinct from that in the low Cd exposure and control treatments, whereas the taxonomic groups in the latter two treatments were clustered together (Figure 2B). In addition, hierarchical clustering tree construction revealed that the intestinal samples of the three exposure treatments were clustered into two independent groups, excluding one control sample (Figure 2C), implying that Cd exposure at high dose (1.5 mg/L) exerted greater effects on the microbiota composition in C. cathayensis. The results indicate that C. cathayensis could potentially tolerate low Cd stress. Gut microbiome systems of aquatic animals participate in various processes, including nutrition, growth, immunity, and disease resistance (Talwar et al., 2018; Chang et al., 2019; Paul and Small, 2019; Duan et al., 2020; Wang et al., 2020a; Diwan et al., 2021). In the present study, intestinal microbiota structure was altered in the C. cathayensis gut under high Cd exposure when compared with in the control treatment (Figures 2B,C). Consequently, alteration of intestinal microbial community structure following Cd exposure could induce adverse effects on C. cathayensis health.
Effects of Cd exposure on gut microbial community
In the present study, phylum Proteobacteria was the dominant phylum across all three groups. The results are consistent with the findings of recent studies in other Cipangopaludina species (Zhou K. Q. et al., 2022; Zhou Z. H. et al., 2022). The other two dominant phyla were Bacteroidetes and Firmicutes, which are consistent with the findings of previous studies that have reported that the major bacterial phyla in the gut of aquatic animals, including fish, crustaceans, and mollusks are Proteobacteria, Bacteroidetes, and Firmicutes (Chang et al., 2019; Liu et al., 2019; Wang et al., 2020b; Zhang Y. et al., 2020; Zhou Z. H. et al., 2022). However, the two phyla were not dominant in a closely related species, Cipangopaludina chinensis (Zhou K. Q. et al., 2022; Zhou Z. H. et al., 2022). The result implies that although the two species are closely related, they may have different strategies of responding to Cd stress. Nevertheless, further research is required to investigate the factors responsible for the difference between the two species. Compared to that in the control, the abundance of Bacteroidetes was significantly higher in the H14 treatment, although there was no significant difference between the L14 and control treatments. Bacteroidetes, the largest phylum of Gram-negative bacteria in the human gastrointestinal tract microbiome, has the potential to secrete surface lipopolysaccharides and toxic proteolytic peptides, which can cause inflammation in the gut (Lukiw, 2016). The elevated phylum Bacteroidetes abundance in H14 treatment indicated that high Cd exposure has potential adverse effects on C. cathayensis health.
Genus Pseudomonas, which has been identified as bacterial pathogen in teleosts, exists widely in aquatic environments and in the gut of aquatic animals (Llewellyn et al., 2014; Xu et al., 2015; Dehler et al., 2017; Diwan et al., 2021). Pseudomonas has also been observed to increase in the guts of different vertebrates, including fish and amphibians, following Cd exposure (Chang et al., 2019; Ya et al., 2019; Cheaib et al., 2020). Furthermore, Pseudomonas outbreaks have been reported in aquacultured animals (Llewellyn et al., 2014; Xu et al., 2015). However, Pseudomonas are also considered probiotics for application in aquaculture (Wang A. R. et al., 2019), that can chelate or oxidize heavy metals, thereby facilitating heavy metal excretion and minimizing the exposure of organisms to heavy metals (Duan et al., 2020; Arun et al., 2021). In the present study, Pseudomonas relative abundance in the L14 treatment was higher than that in the control treatment, although the difference was not significant. However, Pseudomonas relative abundance in the H14 treatment was lower than that in the control treatment, although not significant. Notably, Pseudomonas abundance decreased with an increase in Cd concentration, suggesting that Pseudomonas could play a role in Cd toxicity removal. However, probiotics contents decreased with an increase in Cd concentration, which could adversely affect Cd toxicity tolerance in mudsnail. Indeed, high snail mortality was observed in the H14 treatment but not in the L14 treatment. The results further confirm our postulation above that C. cathayensis could acclimate to low Cd concentration, potentially by accumulating Pseudomonas. Acinetobacter are putative pathogens. Their abundance increased significantly in the gut of Nile tilapia (Zhai et al., 2016) and common carp (Chang et al., 2019) following Cd exposure, and greatly increased in methyl-mercury (MeHg)-exposed fish (Bridges et al., 2018). Studies have shown that Acinetobacter may exert adverse effects on fish health (Wu et al., 2013; Wang et al., 2020c). Consistent with the previous findings, Acinetobacter increased in the L14 treatment, although not significantly. However, when Cd concentration reached 1.5 mg/L, Acinetobacter reduced considerably, which may be related to the extremely high Cd content (Wang et al., 2020c). Similarly, Rhodobacter significantly decreased in the H14 treatment. Decreased Rhodobacter abundance has been reported to reduce growth (Liu H. S. et al., 2021) and to have adverse effects on fish innate immunity (Wang et al., 2020c), resulting in increased vulnerability to disease (She et al., 2017; Liu F. P. et al., 2021). Indeed, Rhodobacter is a candidate probiotic for fish (Ye et al., 2019). However, some studies have found that higher abundances of such bacteria could be associated with diseased intestines (Tran et al., 2018), and they could cause neurotoxicity in the hosts (Bridges et al., 2018; Arun et al., 2021). Such findings illustrate the importance of intestinal bacterial community homeostasis in hosts. Decreased Rhodobacter abundance in the present study suggest that intestinal function could have been impaired in C. cathayensis exposed to Cd, which could result in disease outbreaks under natural conditions (She et al., 2017; Liu F. P. et al., 2021).
In the present study, Cloacibacterium, a key genus in the phylum Bacteroidetes implicated in xenobiotic metabolism and metal removal (Nouha et al., 2016; Duan et al., 2020), increased significantly in the H14 treatment. Cloacibacterium has been used to detoxify MeHg in MeHg-exposed fish (Bridges et al., 2018). Enrichment of Cloacibacterium has been reported to be an important feature under MeHg-induced neurotoxicity (Bridges et al., 2018). In addition, in the present study, Dechloromonas abundance increased in the H14 treatment. Dechloromonas, which belongs to the phylum Proteobacteria and is considered a Cd-resistant microorganism (Zhang et al., 2019), could efficiently degrade polycyclic aromatic hydrocarbons during sludge composting (Lu et al., 2019; Che et al., 2021), and participate in organic matter degradation in aquaculture pond sediment (Zhang K. K. et al., 2020). Cloacibacterium and Dechloromonas enrichment in C. cathayensis gut in the present study highlight their potential roles in Cd detoxification, which merit further study.
Intestinal microbiome function
Our function prediction analysis of the gut microbiota showed that most of the genes encoded by the C. cathayensis gut microbiota were related to metabolism, followed by organismal systems, cellular processes pathways, human diseases pathways, genetic information processing pathways, and environmental information processing pathways (Figure 4). The intestines are essential organs involved in the metabolism of nutrients (Liu et al., 2022). The results suggest that Cd exposure may alter gut microbial function and host metabolism. In addition, function prediction results showed that, compared with the control treatment, only one pathway related to fluorobenzoate degradation was enriched in the L14 treatment, whereas more pathways were enriched in the H14 treatment (Figure 5), including protein digestion and absorption, apoptosis, lysosome, other glycan degradation, and pathways in cancer. Fluorobenzoate is the sole carbon and energy source for Pseudomonas (Kalpit et al., 1988). The fluorobenzoate degradation pathway was enriched in the L14 treatment, which is consistent with the increasing trends in Pseudomonas abundance observed in the treatment group. The cell apoptosis pathway is usually activated following disease infection (Qiu et al., 2020) or exposure to adverse environmental factors (Chen et al., 2021). Furthermore, lysosomes not only play a central role in cell decomposition but also participate in metabolism, membrane repair, and cell death (Serrano-Puebla and Boya, 2015), and lysosome metabolic pathways are closely related to cell apoptosis (Guo et al., 2017). In the present study, lysosome pathway and cell apoptosis pathway were both enriched in the H14 treatment, which suggests that high Cd exposure may exert more adverse effects on gut microbes of snails than low Cd exposure. Furthermore, pathways in cancer were also enriched in the H14 treatment. The results above partially explain our hypothesis above that C. cathayensis has a capacity to acclimate to low Cd stress.
It is also worth noting that pathways associated with xenobiotic and organic compound biodegradation and metabolism, including chloroalkane and chloroalkene degradation, benzoate degradation, fluorobenzoate degradation, styrene degradation, limonene and pinene degradation, geraniol degradation, were significantly inhibited in the H14 treatment in the present study, when compared to in the L14 treatment. Chloroalkane, chloroalkene, benzoate, fluorobenzoate and styrene are xenobiotics found in the environment (Vera et al., 2022). However, the pathways associated with the degradation of the xenobiotics were down-regulated in the H14 treatment, which suggested that the capacity of elimination of the compounds decreased following exposure to high Cd doses (Gu et al., 2017; Vera et al., 2022). Limonene and pinene are considered anti-inflammatory molecules; the down-regulation of the limonene and pinene degradation pathway in the H14 treatment could have increased the levels of limonene and pinene, which could have antagonized the inflammatory response caused by Cd stress (Han et al., 2021). Geraniol is another carbon and energy source for some Pseudomonas species (Vandenbergh and Wright, 1983; Zhu et al., 2020). The decline in the geraniol degradation pathway in the present study could be attributed to the decreased contents of the genus Pseudomonas. Overall, according to the results of the present study, Cd exposure disrupts gut microbial community structure and their potential functions, and could in turn, adversely influence C. cathayensis health.
Conclusion
Our results revealed that Cd exposure could significantly alter the structure and function of intestinal microbial communities, which may in turn influence C. cathayensis gut homeostasis and health. To the best of our knowledge, this is the first study to explore the effects of Cd exposure on the intestinal microbiota of C. cathayensis. The results obtained in this study provide insights into the mechanisms associated with the response of the intestinal microbiota of C. cathayensis to Cd pollution. However, obtaining the 16S rRNA gene sequences through the Illumina HiSeq platform has limitations. In the present study, we did not isolate and identify the putatively pathogenic and putatively beneficial bacteria, which warrants further research.
Data availability statement
The datasets presented in this study can be found in online repositories. The names of the repository/repositories and accession number(s) can be found below: https://www.ncbi.nlm.nih.gov/, PRJNA837347.
Ethics statement
The animal study was reviewed and approved by Animal Ethics Committee of Guangxi Normal University.
Author contributions
Z-XX, J-XF, and J-YJ conceived and designed the study. Z-XX, J-YJ, and W-HL contributed reagents and materials. J-YJ and Y-YW analyzed the data. Y-YW and C-XC performed the gut extraction. Y-YW, C-XC, and Q-QY cultured the snails. J-YJ and W-HL wrote the manuscript. All authors read and approved the final manuscript.
Funding
This study was supported by the Guangxi BaGui Scholars Program Foundation (2019A50) and the National Key R&D Program of China (2021YFD1100106).
Acknowledgments
We thank all participants in this work, including staff at the Key Laboratory of Ecology of Rare and Endangered Species and Environmental Protection (Guangxi Normal University), Ministry of Education, and staff at Guangxi Key Laboratory of Veterinary Biotechnology, Guangxi Veterinary Research Institute.
Conflict of interest
The authors declare that the research was conducted in the absence of any commercial or financial relationships that could be construed as a potential conflict of interest.
Publisher’s note
All claims expressed in this article are solely those of the authors and do not necessarily represent those of their affiliated organizations, or those of the publisher, the editors and the reviewers. Any product that may be evaluated in this article, or claim that may be made by its manufacturer, is not guaranteed or endorsed by the publisher.
Supplementary material
The Supplementary Material for this article can be found online at: https://www.frontiersin.org/articles/10.3389/fmicb.2022.984757/full#supplementary-material
Supplementary Figure S1 | Rarefaction curves for all the analyzed samples. Different samples are represented by curves of different colors.
Supplementary Figure S2 | Relative abundance of intestinal bacteria. (a) The relative abundance of phylum Bacteroidetes; (b) genus Pseudomonas; (c) genus Acinetobacter; (d) genus Rhodobacter; (e) genus Cloacibacterium; (f) and genus Dechloromonas. Asterisks (*) indicate p-values < 0.05.
Footnotes
- ^ http://qiime.org
- ^ http://greengenes.secondgenome.com/
- ^ http://scikit-bio.org/docs/latest/generated/skbio.diversity.alpha.html#module-skbio.diversity.alpha
- ^ https://github.com/picrust/picrust2
- ^ http://www.genome.jp/kegg/pathway.html
References
Ali, M. M., Rahman, S., Islam, M. S., Rakib, M., Hossen, S., Rahman, M. Z., et al. (2022). Distribution of heavy metals in water and sediment of an urban river in a developing country: a probabilistic risk assessment. Int. J. Sediment Res. 37, 173–187.
Arun, K. B., Madhavan, A., Sindhu, R., Emmanual, S., Binod, P., Pugazhendhi, A., et al. (2021). Probiotics and gut microbiome-prospects and challenges in remediating heavy metal toxicity. J. Hazard. Mater. 420:126676. doi: 10.1016/j.jhazmat.2021.126676
Bridges, K. N., Zhang, Y., Curran, T. E., Magnuson, J. T., Venables, B. J., Durrer, K. E., et al. (2018). Alterations to the intestinal microbiome and metabolome of pimephales promelas and mus musculus following exposure to dietary methylmercury. Environ. Sci. Technol. 52, 8774–8784. doi: 10.1021/acs.est.8b01150
Burger, J. (2008). Assessment and management of risk to wildlife from cadmium. Sci. Total Environ. 389, 37–45.
Callahan, B. J., McMurdie, P. J., Rosen, M. J., Han, A. W., Johnson, A. J. A., and Holmes, S. P. (2016). DADA2: High-resolution sample inference from Illumina amplicon data. Nat. Methods 13, 581–583.
Chang, X. L., Li, H., Feng, J. C., Chen, Y. Y., Nie, G. X., and Zhang, J. X. (2019). Effects of cadmium exposure on the composition and diversity of the intestinal microbial community of common carp (cyprinus carpio L.). Ecotoxicol. Environ. Saf. 171, 92–98. doi: 10.1016/j.ecoenv.2018.12.066
Che, J. G., Bai, Y. D., Li, X., Ye, J., Liao, H. P., Cui, P., et al. (2021). Linking microbial community structure with molecular composition of dissolved organic matter during an industrial-scale composting. J. Hazard. Mater. 405:124281. doi: 10.1016/j.jhazmat.2020.124281
Cheaib, B., Seghouani, H., Ijaz, U. Z., and Derome, N. (2020). Community recovery dynamics in yellow perch microbiome after gradual and constant metallic perturbations. Microbiome 8:14. doi: 10.1186/s40168-020-0789-0
Chen, G., Pang, M. X., Yu, X. M., Wang, J. R., and Tong, J. G. (2021). Transcriptome sequencing provides insights into the mechanism of hypoxia adaption in bighead carp (Hypophthalmichthys nobilis). Comp. Biochem. Physiol. D Genom. Proteom. 40:100891. doi: 10.1016/j.cbd.2021.100891
Chen, Y. F., Shi, Q. G., Qu, J. Y., He, M. X., and Liu, Q. (2022). A pollution risk assessment and source analysis of heavy metals in sediments: A case study of Lake Gehu, China. Chin. J. Anal. Chem. 50:100077.
Cui, Y. D., Wang, B. Q., Zhao, Y. J., Bond, N. R., and Wang, H. Z. (2022). Recovery time of macroinvertebrate community from Cd pollution in Longjiang River, Guangxi, China. J. Oceanol. Limnol. 40, 183–194.
Dehler, C. E., Secombes, C. J., and Martin, S. A. M. (2017). Environmental and physiological factors shape the gut microbiome of Atlantic salmon (Salmo salar L.). Aquaculture 467, 149–157.
Diwan, A. D., Harke, S. N., and Gopalkrishna, Panche, A. N. (2021). Aquaculture industry prospective from gut microbiome of fish and shellfish: An overview. J. Anim. Physiol. Anim. Nutr. 106, 441–469. doi: 10.1111/jpn.13619
Duan, H., Yu, L. L., Tian, F. W., Zhai, Q. X., Fan, L. P., and Chen, W. (2020). Gut microbiota: A target for heavy metal toxicity and a probiotic protective strategy. Sci. Total Environ. 742:140429. doi: 10.1016/j.scitotenv.2020.140429
Gu, X. Z., Fu, H. T., Sun, S. M., Qiao, H., Zhang, W. Y., Jiang, S. F., et al. (2017). Dietary cholesterol-induced transcriptome differences in the intestine, hepatopancreas, and muscle of Oriental River prawn Macrobrachium nipponense. Comp. Biochem. Physiol. D Genom. Proteom. 23, 39–48. doi: 10.1016/j.cbd.2017.06.001
Guo, Y. S., Ma, Y., Zhang, Y. W., Zhou, L., Huang, S. L., Wen, Y., et al. (2017). Autophagy-related gene microarray and bioinformatics analysis for ischemic stroke detection. Biochem. Biophys. Res. Commun. 489, 48–55. doi: 10.1016/j.bbrc.2017.05.099
Han, Y. J., Kang, L. L., Liu, X. H., Zhang, Y. H., Chen, X., and Li, X. Y. (2021). Establishment and validation of a logistic regression model for prediction of septic shock severity in children. Hereditas 158:45. doi: 10.1186/s41065-021-00206-9
Hu, R., and Tang, Z. Y. (2012). Effect of cadmium and mercury on superoxidedismutase activity of the mudsnail (Cipangopaludina cahayensis). J. Sichuan Normal Univ. 35, 690–693.
Kalpit, A. V., Singh, C., and Modi, V. V. (1988). Degradation of 2-fluorobenzoate by a pseudomonad. Curr. Microbiol. 17, 249–254.
Liu, H. S., Li, X., Lei, H. J., Li, D., Chen, H. X., Schlenk, D., et al. (2021). Dietary seleno-L-methionine alters the microbial communities and causes damage in the gastrointestinal tract of japanese medaka Oryzias latipes. Environ. Sci. Technol. 55, 16515–16525. doi: 10.1021/acs.est.1c04533
Liu, F. P., Xu, X. F., Chao, L., Chen, K., Shao, A., Sun, D. Q., et al. (2021). Alteration of the gut microbiome in chronic kidney disease patients and its association with serum free immunoglobulin light chains. Front. Immunol. 12:609700. doi: 10.3389/fimmu.2021.609700
Liu, J., Pang, J. J., Tu, Z. C., Wang, H., Sha, X. M., Shao, Y. H., et al. (2019). The accumulation, histopathology, and intestinal microorganism effects of waterborne cadmium on carassius auratus gibelio. Fish Physiol. Biochem. 45, 231–243. doi: 10.1007/s10695-018-0557-2
Liu, Y. Y., Cheng, J. X., Xia, Y. Q., Li, X. H., Liu, Y., and Liu, P. F. (2022). Response mechanism of gut microbiome and metabolism of European seabass (Dicentrarchus labrax) to temperature stress. Sci. Total Environ. 813:151786. doi: 10.1016/j.scitotenv.2021.151786
Llewellyn, M. S., Boutin, S., Hoseinifar, S. H., and Derome, N. (2014). Teleost microbiomes: The state of the art in their characterization, manipulation and importance in aquaculture and fisheries. Front. Microbiol. 5:207. doi: 10.3389/fmicb.2014.00207
Lu, H. F., Du, L. N., Li, Z. Q., Chen, X. Y., and Yang, J. X. (2014). Morphological analysis of the Chinese Cipangopaludina species (Gastropoda; Caenogastropoda: Viviparidae). Zool. Res. 35, 510–527.
Lu, Y., Zheng, G. Y., Zhou, W. B., Wang, J. J., and Zhou, L. X. (2019). Bioleaching conditioning increased the bioavailability of polycyclic aromatic hydrocarbons to promote their removal during co-composting of industrial and municipal sewage sludges. Sci. Total Environ. 665, 1073–1082. doi: 10.1016/j.scitotenv.2019.02.174
Lukiw, W. J. (2016). Bacteroides fragilis lipopolysaccharide and inflammatory signaling in alzheimer’s disease. Front. Microbiol. 7:1544. doi: 10.3389/fmicb.2016.01544
Luo, H., Chen, L. T., Jing, T. S., Sun, W. B., Li, Z., Zhou, M. R., et al. (2021). Muscle nutrition analysis of four snail species. J. Fish. China 2021. (in press). https://kns.cnki.net/kcms/detail/31.1283.S.20211018.1713.002.html.
Ministry of Ecology and Environment of the People’s Republic of China (2014). National soil pollution survey communique. Accessed online at: https://www.mee.gov.cn/gkml/sthjbgw/qt/201404/W020140417558995804588.pdf
Nouha, K., Kumar, R. S., and Tyagi, R. D. (2016). Heavy metals removal from wastewater using extracellular polymeric substances produced by cloacibacterium normanense in wastewater sludge supplemented with crude glycerol and study of extracellular polymeric substances extraction by different methods. Bioresour. Technol. 212, 120–129. doi: 10.1016/j.biortech.2016.04.021
Paul, J. S., and Small, B. C. (2019). Exposure to environmentally relevant cadmium concentrations negatively impacts early life stages of channel catfish (Ictalurus punctatus). Comp. Biochem. Physiol. C Toxicol. Pharmacol. 216, 43–51. doi: 10.1016/j.cbpc.2018.11.004
Qiu, Y., Yin, Y. H., Ruan, Z. Q., Gao, Y., Bian, C., Chen, J. M., et al. (2020). Comprehensive transcriptional changes in the liver of kanglang white minnow (anabarilius grahami) in response to the infection of parasite ichthyophthirius multifiliis. Animals 10:681. doi: 10.3390/ani10040681
Serrano-Puebla, A., and Boya, P. (2015). Lysosomal membrane permeabilization in cell death: New evidence and implications for health and disease. Ann. N.Y.Acad. Sci. 1371, 30–44. doi: 10.1111/nyas.12966
She, R., Li, T. T., Luo, D., Li, J. B., Yin, L. Y., Li, H., et al. (2017). Changes in the Intestinal Microbiota of Gibel Carp (Carassius gibelio) Associated with Cyprinid herpesvirus 2 (CyHV-2) Infection. Curr. Microbiol. 74, 1130–1136. doi: 10.1007/s00284-017-1294-y
Song, B., Wang, F. P., Zhou, L., Wu, Y., Pang, R., and Chen, T. B. (2019). Cd Content characteristics and ecological risk assessment of paddy soil in high cadmium anomaly area of Guangxi. Environ. Sci. 40, 2443–2452. doi: 10.13227/j.hjkx.201806202
Talwar, C., Nagar, S., Lal, R., and Negi, R. K. (2018). Fish gut microbiome: Current approaches and future perspectives. Indian J. Microbiol. 58, 397–414. doi: 10.1007/s12088-018-0760-y
Tran, N. T., Zhang, J., Xiong, F., Wang, G. T., Li, W. X., and Wu, X. G. (2018). Altered gut microbiota associated with intestinal disease in grass carp (ctenopharyngodon idellus). World J. Microb. Biotechnol. 34:71. doi: 10.1007/s11274-018-2447-2
Vandenbergh, P. A., and Wright, A. M. (1983). Plasmid involvement in acyclic isoprenoid metabolism by Preudomonas putida. Appl. Environ. Microbiol. 45, 1953–1955. doi: 10.1128/aem.45.6.1953-1955.1983
Vera, A., Wilson, F. P., and Cupples, A. M. (2022). Predicted functional genes for the biodegradation of xenobiotics in groundwater and sediment at two contaminated naval sites. Appl. Microbiol. Biotechnol. 106, 835–853. doi: 10.1007/s00253-021-11756-3
Wang, C., Liu, J., Huang, Y., and Zhang, X. (2016). In vitro polysaccharide extraction from cipangopaludina cathayensis and its pharmacological potential. J. Environ. Biol. 37, 1069–1072.
Wang, N., Jiang, M., Zhang, P. J., Shu, H., Li, Y. R., Guo, Z. Y., et al. (2020a). Amelioration of Cd-induced bioaccumulation, oxidative stress and intestinal microbiota by bacillus cereus in carassius auratus gibelio. Chemosphere 245:125613. doi: 10.1016/j.chemosphere.2019.125613
Wang, N., Guo, Z. Y., Zhang, Y. L., Zhang, P. J., Liu, J., Cheng, Y., et al. (2020b). Effect on intestinal microbiota, bioaccumulation, and oxidative stress of carassius auratus gibelio under waterborne cadmium exposure. Fish Physiol. Biochem. 46, 2299–2399. doi: 10.1007/s10695-020-00870-0
Wang, X. H., Hu, M. H., Gu, H. H., Zhang, L. B., Shang, Y. Y., Wang, T., et al. (2020c). Short-term exposure to norfloxacin induces oxidative stress, neurotoxicity and microbiota alteration in juvenile large yellow croaker Pseudosciaena crocea. Environ. Pollut. 267:115397. doi: 10.1016/j.envpol.2020.115397
Wang, P., Chen, H. P., Kopittke, P. M., and Zhao, F. J. (2019). Cadmium contamination in agricultural soils of China and the impact on food safety. Environ. Pollut. 249, 1038–1048.
Wang, A. R., Ran, C., Wang, Y. B., Zhang, Z., Ding, Q. W., Yang, Y. L., et al. (2019). Use of probiotics in aquaculture of china-a review of the past decade. Fish Shellfish Immunol. 86, 734–755. doi: 10.1016/j.fsi.2018.12.026
Wang, R., Xia, W., Eggleton, M. A., Qu, X., Liu, H., Xin, W., et al. (2022). Spatial and temporal patterns of heavy metals and potential human impacts in central yangtze lakes, China. Sci. Total Environ. 820:153368. doi: 10.1016/j.scitotenv.2022.153368
Wen, Y. B., Li, W., Yang, Z. F., Zhuo, X. X., Guan, D. X., Song, Y. X., et al. (2020). Evaluation of various approaches to predict cadmium bioavailability to rice grown in soils with high geochemical background in the karst region, Southwestern China. Environ. Pollut. 258:113645. doi: 10.1016/j.envpol.2019.113645
Wu, S. G., Tian, J. Y., Gatesoupe, F. J., Li, W. X., Zou, H., Yang, B. J., et al. (2013). Intestinal microbiota of gibel carp (Carassius auratus gibelio) and its origin as revealed by 454 pyrosequencing. World J. Microbiol. Biotechnol. 29, 1585–1595. doi: 10.1007/s11274-013-1322-4
Xu, J., Zeng, X. H., Jiang, N., Zhou, Y., and Zeng, L. B. (2015). Pseudomonas alcaligenes infection and mortality in cultured Chinese sturgeon, Acipenser sinensis. Aquaculture 446, 37–41.
Xu, X., Nie, S., Ding, H., and Hou, F. (2018). Environmental pollution and kidney diseases. Nat. Rev. Nephrol. 14, 313–324.
Ya, J., Ju, Z. Q., Wang, H. Y., and Zhao, H. F. (2019). Exposure to cadmium induced gut histopathological damages and microbiota alterations of Chinese toad (Bufo gargarizans) larvae. Ecotox. Environ. Saf. 180, 449–456. doi: 10.1016/j.ecoenv.2019.05.038
Yang, Q., Yang, Z. F., Zhang, Q., Liu, X., Zhuo, X. X., Wu, T. S., et al. (2021). Ecological risk assessment of Cd and other heavy metals in soil-rice system in the karst areas with high geochemical background of Guangxi. China. Sci. China Earth Sci. 64, 1126–1139.
Ye, Q., Feng, Y. Y., Wang, Z. L., Zhou, A. G., Xie, S. L., Fan, L. F., et al. (2019). Effects of dietary gelsemium elegans alkaloids on intestinal morphology, antioxidant status, immune responses and microbiota of megalobrama amblycephala. Fish Shellfish Immunol. 94, 464–478. doi: 10.1016/j.fsi.2019.09.048
Zhai, Q. X., Yu, L. L., Li, T. Q., Zhu, J. M., Zhang, C. C., Zhao, J. X., et al. (2016). Effect of dietary probiotic supplementation on intestinal microbiota and physiological conditions of Nile tilapia (‘) under waterborne cadmium exposure. Antonie van Leeuwenhoek 110, 501–513. doi: 10.1007/s10482-016-0819-x
Zhang, L. Q., Fan, J. J., Nguyen, H. N., Li, S. G., and Rodrigues, D. F. (2019). Effect of cadmium on the performance of partial nitrification using sequencing batch reactor. Chemosphere 222, 913–922.
Zhang, Y., Li, Z. Y., Kholodkevich, S., Sharov, A., Chen, C., Feng, Y. J., et al. (2020). Effects of cadmium on intestinal histology and microbiota in freshwater crayfish (procambarus clarkii). Chemosphere 242:125105. doi: 10.1016/j.chemosphere.2019.125105
Zhang, K. K., Zheng, X. F., He, Z. L., Yang, T., Shu, L. F., Xiao, F. S., et al. (2020). Fish growth enhances microbial sulfur cycling in aquaculture pond sediments. Microb. Biotechnol. 13, 1597–1610. doi: 10.1111/1751-7915.13622
Zhao, F. J., Ma, Y., Zhu, Y. G., Tang, Z., and McGrath, S. P. (2015). Soil contamination in China: Current status and mitigation strategies. Environ. Sci. Technol. 49, 750–759.
Zhao, T., Xiong, J. Q., Chen, W., Xu, A. H., Zhu, D., and Liu, J. T. (2021). Purification and characterization of a novel fibrinolytic enzyme from cipangopaludina cahayensis. Iran. J. Biotechnol. 19, 121–127. doi: 10.30498/IJB.2021.2805
Zhao, X., Yao, L. A., Ma, Q. L., Zhou, G., and Xu, Z. C. (2018). Distribution and ecological risk assessment of cadmium in water and sediment in Longjiang River, China: Implication on water quality management after pollution accident. Chemosphere 194:107. doi: 10.1016/j.chemosphere.2017.11.127
Zhou, K. Q., Qin, J. Q., Pang, H. F., Chen, Z., Huang, Y., Li, W. H., et al. (2022). Comparison of the composition and function of gut microbes between adult and juvenile Cipangopaludina chinensis in the rice snail system. PeerJ 10:e13042. doi: 10.7717/peerj.13042
Zhou, Z. H., Wu, H. W., Li, D. H., Zeng, W. L., Huang, J. L., and Wu, Z. J. (2022). Comparison of gut microbiome in the Chinese mudsnail (Cipangopaludina chinensis) and the invasive golden apple snail (Pomacea canaliculata). PeerJ 10:e13245. doi: 10.7717/peerj.13245
Zhou, Y. Y., and Luo, Z. M. (2018). Effects of cadmium on antioxidant enzymes activities and lipid peroxidation in mudsnail (Cipangopaludina cathayensis). Sci. Technol. Food Ind. 39, 43–47.
Keywords: cadmium, Cipangopaludina cathayensis, intestinal microbiota, high-throughput sequencing, microbial diversity
Citation: Jiang J-y, Li W-h, Wu Y-y, Cheng C-x, Ye Q-q, Feng J-x and Xie Z-x (2022) Effects of cadmium exposure on intestinal microflora of Cipangopaludina cathayensis. Front. Microbiol. 13:984757. doi: 10.3389/fmicb.2022.984757
Received: 02 July 2022; Accepted: 21 July 2022;
Published: 08 August 2022.
Edited by:
Punyasloke Bhadury, Indian Institute of Science Education and Research Kolkata, IndiaCopyright © 2022 Jiang, Li, Wu, Cheng, Ye, Feng and Xie. This is an open-access article distributed under the terms of the Creative Commons Attribution License (CC BY). The use, distribution or reproduction in other forums is permitted, provided the original author(s) and the copyright owner(s) are credited and that the original publication in this journal is cited, in accordance with accepted academic practice. No use, distribution or reproduction is permitted which does not comply with these terms.
*Correspondence: Zhi-xun Xie, eGllemhpeHVuQDEyNi5jb20=
†These authors have contributed equally to this work