- 1The Second Affiliated Hospital and Yuying Children’s Hospital, Wenzhou Medical University, Wenzhou, China
- 2Key Laboratory of Medical Genetics of Zhejiang Province, Key Laboratory of Laboratory Medicine, Ministry of Education, School of Laboratory Medicine and Life Sciences, Wenzhou Medical University, Wenzhou, China
- 3Medical Molecular Biology Laboratory, School of Medicine, Jinhua Polytechnic, Jinhua, China
A novel chromosome-encoded aminoglycoside O-nucleotidyltransferase AadA33 was identified in Providencia vermicola strain P13. The AadA33 shares the highest amino acid identity of 51.28% with the function characterized AadA31. Antibiotic susceptibility testing and enzyme kinetics analysis revealed that the function of AadA33 is to mediate spectinomycin and streptomycin resistance. The recombinant strain harboring aadA33 (pUCP20-aadA33/Escherichia coli DH5α) displayed >256- and 128-fold increases in the minimum inhibitory concentration levels to spectinomycin and streptomycin, respectively, compared with the control strains pUCP20/DH5α. Enzyme kinetic parameters manifested the substrate of AadA33 including spectinomycin and streptomycin, with kcat/Km of 3.28 × 104 (M−1 s−1) and 3.37 × 104 (M−1 s−1), respectively. Bioinformatics analysis revealed its structural mechanism of antimicrobial resistance, genetic context, and phylogenetic relationship with other aminoglycoside O-nucleotidyltransferases. This study of AadA33 contributed to understanding the function and resistance mechanism of aminoglycoside O-nucleotidyltransferase.
Introduction
Genus Providencia is Gram-negative opportunistic pathogens of the family Morganellaceae, which could be isolated from a wide range of organisms and environments. Providencia vermicola was first isolated from a nematode Steinernema thermophilum in New Delhi, India (Somvanshi et al., 2006). However, unlike the problematic ESKAPEE pathogens (Enterococcus faecium, Staphylococcus aureus, Klebsiella pneumoniae, Acinetobacter baumannii, Pseudomonas aeruginosa, Enterobacter species, and Escherichia coli) (Rice, 2008), Providencia Providencia rettgeri (Tshisevhe et al., 2016; Shin et al., 2018) and Providencia stuartii (Douka et al., 2015; Oikonomou et al., 2016), P. vermicola is rarely involved in the nosocomial outbreak (Lupande-Mwenebitu et al., 2021). At the time of writing, there are three genomes of P. vermicola deposited in the NCBI database and only one is the complete genome. Aminoglycoside antibiotics are traditional broad-spectrum Gram-negative antibacterial medications that inhibit protein synthesis. Mechanisms of resistance to aminoglycosides mainly include aminoglycoside modifying enzymes (Ramirez and Tolmasky, 2010), increased efflux (Aires et al., 1999), reduced uptake, or decreased permeability (Over et al., 2001), and alterations of 16S rRNA (Doi et al., 2016). At present, the most common mechanism of resistance to aminoglycosides is the inactivation of these antibiotics mediated by various aminoglycoside modifying enzymes (Ramirez and Tolmasky, 2010). Based on the site of modification (Shaw et al., 1993), aminoglycoside modifying enzymes could be classified into aminoglycoside N-acetyltransferases (AACs), aminoglycoside O-nucleotidyltransferases (ANTs), and aminoglycoside O-phosphotransferases (APHs).
ANTs mediate the covalent modification of aminoglycoside antimicrobial hydroxyl group by ATP-dependent transfer of AMP. There are five classes of ANTs, namely ANT(6), ANT(9), ANT(4′), ANT(3″), and ANT(2″), which catalyze nucleotidylylation at the hydroxyl group at positions 6, 9, 4, 3, and 2, respectively. ANT(3″)-Ia (also commonly named AadA) is the most common ANT enzyme of the ANT(3″) family. Up to date, more than 20 types of genes encoding ANT(3″)-Ia enzymes have been identified.
In this study, the function and molecular characteristics of a novel aminoglycoside O-nucleotidyltransferase gene (designated aadA33) encoded on the chromosome of a P. vermicola strain were characterized.
Materials and methods
Bacterial strains and plasmids
Providencia vermicola P13 was isolated from the blood of an inpatient diagnosed with idiopathic thrombocytopenic purpura at a hospital in Wenzhou, China. Species identification of P. vermicola P13 was conducted by the VITEK 2 Compact instrument (bioMerieux, Inc., Craponne, France), 16S rRNA gene sequence, and whole-genome average nucleotide identity (ANI) (Konstantinidis and Tiedje, 2005; Richter and Rosselló-Móra, 2009). The strains and plasmids used in this work are listed in Table 1.
Antibiotic susceptibility testing
The minimum inhibitory concentrations (MICs) were determined with the agar dilution method recommended by the Clinical and Laboratory Standards Institute (CLSI) guidelines, and the results were interpreted according to the CLSI M100 (31st Edition, 2021), the European Committee on Antimicrobial Susceptibility Testing (version 11.0, 2021) and National Antimicrobial Resistance Monitoring System for Enteric Bacteria breakpoint criteria.1 E. coli ATCC 25922 was used as a reference strain for quality control. All tested antimicrobials in this work were listed in Table 2, including one aminocyclitol (spectinomycin); ten aminoglycosides (streptomycin, neomycin, sisomicin, ribostamycin, tobramycin, gentamicin, amikacin, kanamycin, paromomycin and micronomicin); six β-lactams (ampicillin, cefoxitin, cefepime, ceftazidime, meropenem and aztreonam); one quinolone (levofloxacin); one phenicol (chloramphenicol); two tetracyclines (tetracycline and tigecycline); one phosphonic acid (fosfomycin), and one polymyxin (polymyxin E). All the antimicrobials were human drugs bought from a pharmacy or hospital.
Cloning of the aadA33 gene
The aadA33 gene along with its promoter region was amplified by PCR with the primers listed in Table 3. The PCR product was digested with BamHI and HindIII and ligated into the pUCP20 vector with a T4 DNA ligase cloning kit (Takara Bio, Inc., Dalian, China). The recombinant plasmid was transformed into E. coli DH5α by the calcium chloride method, and then the transformant was cultured on Luria-Bertani (LB) agar plates supplemented with 100 μg/ml ampicillin. The size and sequence of the cloned insert were verified by restriction enzyme digestion and Sanger sequencing, respectively.
Expression and purification of the AadA33 enzyme
AadA33 was overexpressed in E. coli BL21/pCold I-aadA33 and purified as described previously (Qing et al., 2004; Shi et al., 2015). The aadA33 gene was cloned with a thrombin cleavage site into the pCold I vector under the control of the cspA promoter using the cold-shock system (Qing et al., 2004). When the OD600 of the culture reached 0.6–0.8 at 37°C, induction of protein expression was triggered by the addition of 1 mM isopropyl-β-D-thiogalactoside, and additional incubation was carried out for 20 h at 16°C. Cells were collected by centrifugation (5,000 × g, 10 min) at 4°C, resuspended in lysis buffer (20 mM Tris–HCl, 150 mM NaCl, 3 mM β-mercaptoethanol, 0.5% Nonidet-P-40; pH 8.0), and lysed by sonication. After removing cellular debris by centrifugation (12,000 × g, 30 min) at 4°C, lysates were incubated with pre-equilibrated nickel-nitrilotriacetic acid (Ni-NTA) agarose resin (Beyotime Biotechnology, Shanghai, China) for 8 h at 4°C under gentle shaking. Then the recombinant protein was purified by standard Ni-NTA affinity chromatography. The His6 tag was removed by incubation with thrombin for 24 h at 37°C. The purity of AadA33 protein was validated by SDS-PAGE, and the protein concentration was examined by a BCA protein assay kit (Thermo Fisher Scientific, Rockford, IL, United States).
Enzyme kinetics
The kinetic assay used to monitor enzyme activity was performed as reported previously (Kim et al., 2006). The AadA33 activity was measured by coupling the enzymatic reaction to the reactions of UDP-glucose pyrophosphorylase, phosphoglucomutase, and glucose-6-phosphate dehydrogenase. The catalytic activity of AadA33 was assayed by monitoring the accumulation of NADPH at 340 nm with a Synergy™ Neo2 Multi-Mode Microplate Reader (BioTek Instruments, Inc., United States). The reaction mixtures contained 50 mM HEPES (pH 7.5), 10 mM MgCl2, 0.2 mM UDP-glucose, 0.2 mM glucose 1,6-bisphosphate, 0.2 mM NADP, 0.2 mM dithiothreitol, 2 units/ml UDP-glucose pyrophosphorylase, 20 units/ml phosphoglucomutase, 20 units/ml glucose-6-phosphate dehydrogenase, 1 mM ATP, 3.41 × 10−8 mM of AadA33, and variable concentrations of aminoglycoside (1–150 μM) in a total volume of 0.2 ml. Reactions were initiated by the addition of the AadA33.
Whole genome sequencing and bioinformatic analysis
Genomic DNA of P. vermicola P13 was sequenced by the Illumina NovaSeq and PacBio RS II platforms (Shanghai Personal Biotechnology Co., Ltd., Shanghai, China). The Illumina short reads were assembled by SKESA v2.4.0 (Souvorov et al., 2018). The PacBio long reads were assembled by Trycycler v0.5.1 (Wick et al., 2021) and Flye v2.9-b1768 (Kolmogorov et al., 2019). The quality of the draft genome assembly was improved by Pilon by mapping Illumina short reads to the assembly to correct possible misassembled bases (Walker et al., 2014). ANI was computed using FastANI (Jain et al., 2018). Genes were predicted by Prokka v1.14.6 (Seemann, 2014). DIAMOND v2.0.11 (Buchfink et al., 2021) and NCBI non-redundant protein databases were used to annotate deduced proteins. Resistance Gene Identifier v5.2.02 and the comprehensive antibiotic resistance database (CARD, McArthur et al., 2013) were used to identify antimicrobial resistance genes. Multiple sequence alignment, phylogenetic tree construction, and visualization were conducted using MAFFT v7.490 (Katoh and Standley, 2013), IQ-TREE v 2.0.7 (Minh et al., 2020), and ggtree v3.2.0 (Yu et al., 2017), respectively. The conserved domain of AadA33 was discovered by CD-search.3 Visualization of genome map and features was generated in GView Server (Petkau et al., 2010). The figure of the genetic environment surrounding the aadA33 and aadA33-like genes was generated by clinker v0.0.24 (Gilchrist and Chooi, 2021). The molecular weight and pI value of AadA33 were predicted using ProtParam.4 GNU Parallel (Tange, 2021) and Entrez Direct5 were used to access resources in the NCBI databases.
Results
General features of the Providencia vermicola P13 genome
P13 was initially identified as P. stuartii by VITEK 2 Compact instrument. The 16S rRNA gene sequence of the isolate P13 has the highest similarity (98.76% coverage and 99.21% identity) with P. vermicola OP1 (NR_042415.1). Furthermore, ANI analysis revealed that P. vermicola P13 shares the highest ANI (99.16%) with P. vermicola P8538 (NZ_CP048796.1). ANI is the proposed genomic gold standard for prokaryotic species classification (Richter and Rosselló-Móra, 2009) and is used at the NCBI to review taxonomy for prokaryotic genomes (Ciufo et al., 2018). Therefore, along with the clinical laboratory result of the VITEK 2 Compact instrument, P13 was finally classified into P. vermicola and named P. vermicola P13.
The whole genome of P. vermicola P13 consists of one chromosome (plasmid-free), approximately 4.32 Mb in length, with 41.0% GC content, and encodes 3,919 open reading frames (Table 4). Comparative genomic analysis revealed that the genomes of P. vermicola P8538 (93.0% coverage and 99.33% identity) and P. vermicola LLDRA6 (78.0% coverage and 90.20% identity) showed the highest similarities with that of P. vermicola P13 (Figure 1).
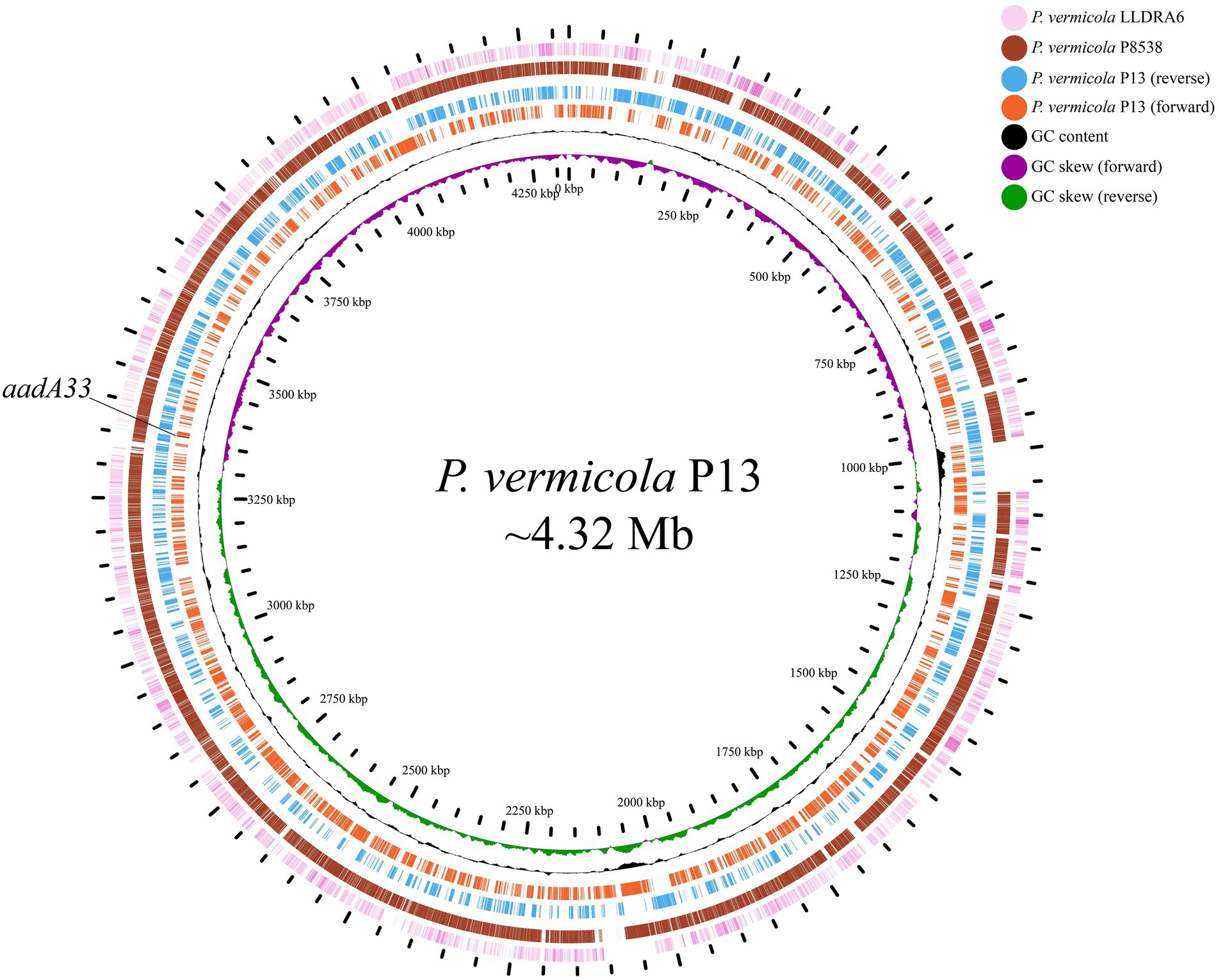
Figure 1. Genome maps of Providencia vermicola P13 and its close relatives. Circles from inside to outside represent GC skew, GC content, genes encoded in the forward and the reverse strands of the chromosome of P. vermicola P13, the P. vermicola P8538 chromosome (CP048796.1), and the P. vermicola LLDRA6 chromosome (CP067099.1), respectively. Regions with <80% nucleotide identities with that of the P. vermicola P13 chromosome are left blank.
Phenotypic and genotypic characterization of antibiotic resistance of Providencia vermicola P13
The in vitro susceptibility test showed that P. vermicola P13 exhibited resistance to many tested antimicrobials, including aminoglycosides (such as spectinomycin, streptomycin, tobramycin, gentamicin, and kanamycin), β-lactams (ampicillin, cefoxitin, ceftazidime, and meropenem), quinolones (levofloxacin), chloramphenicol, tetracycline, fosfomycin, and polymyxin E. A total of 18 genes (15 genotypes) with ≥95% similarity to the antibiotic resistance genes in the CARD database were identified on the chromosome, including four genotypes of aminoglycoside modifying enzymes (aadA2, aph(3′)-Ia, aph(4)-Ia and aac(3)-IV) and two genotypes of β-lactamase (blaNDM-1 and blaOXA-10) (Table 5). It should be noticed that although the strain showed resistance to spectinomycin (MIC >2048 μg/ml) and streptomycin (MIC 256 μg/ml) (Table 2), no function-characterized gene which conferred resistance to spectinomycin or/and streptomycin was identified. There might be a novel mechanism responsible for the resistance phenotype against the two antimicrobials of the bacterium. Spectinomycin and streptomycin were usually substrates of aadA (Ramirez and Tolmasky, 2010). When analyzing the annotation result of the genome, we found that one predicted hypothetical gene encoding a protein (finally designated aadA33) which shares 91.57% coverage and 49.37% amino acid identity of AadA5 (AAF17880.1) in the CARD database, a protein that mediated resistance to spectinomycin and streptomycin (Sandvang, 1999), was found. To figure out whether this hypothetical gene was related to the phenotype of the bacterium resistant to spectinomycin and streptomycin, the gene was cloned, and its function was determined.
aadA33 confers resistance to spectinomycin and streptomycin
Compared with the control strain (pUCP20/DH5α), the recombinant carrying aadA33 (pUCP20-aadA33/E. coli DH5α) exhibited >256- and 128-fold increase in MIC levels of spectinomycin and streptomycin, respectively. However, no significant increase in the MIC level was identified for the other tested aminoglycosides (Table 2). The enzyme can catalyze adenylylation of spectinomycin and streptomycin with kcat/Km of 3.28 × 104 (M−1 s−1) and 3.37 × 104 (M−1 s−1), respectively (Table 6). The kinetic parameters displayed that the substrates of AadA33 are consistent with its MIC patterns.
Comparative analysis of the aadA33 gene and its relatives
Phylogenetic analysis of the AadA33 with ANT(3″)-Ia family and other function characterized ANTs revealed that AadA33 has a close relationship with AadA14 and AadA31. It suggests that AadA33 is a novel lineage of the ANT(3″)-Ia family (Figure 2). Located on the chromosome, the aadA33 gene is 786 bp in length and encodes a 261 aa protein with a molecular weight of 29.3 kDa (Supplementary Figure S1) and a pI value of 5.59. When searching the homologous sequences of aadA33 in the NCBI non-redundant nucleotide and protein database. 25 protein sequences with >70% identity were found and they were all from the genus Providencia (Figure 3). The deduced protein sequence of AadA33 shared the highest amino acid similarity (100% coverage and 98.85% identity) with the DUF4111 domain-containing protein (WP_163861668.1) encoded on the chromosome of P. vermicola P8538 (NZ_CP048796). The other 24 sequences with similarities ranging from 73.64% to 98.08% were from P. stuartii (83.3%, 20/24), unclassified Providencia (12.5%, 3/24), and P. thailandensis (4.2%, 1/24). Besides, protein sequences with >90% identity against AadA33 were also found in 4 out of 25 whole-genome sequenced clinical Providencia isolates (data not shown). However, AadA33 only shared highest identity of 51.28%, 51.09%, 49.37%, 47.22% and 45.77% amino acid identities with the function characterized AadA31 (AUX81654.1), AadA (Q8ZPX9), AadA5 (AAF17880.1), AadA10 (AAL36430.1) and AadA13 (ABW91178.1), respectively. To analyze the resistance function-related structural mechanism of AadA33, multiple sequence alignment of AadA33 and the function-characterized AadA proteins including the structure-characterized AadA (Q8ZPX9) was built (Figure 4). It turns out that AadA33 contains four amino acid residues (E88, W113, D183, and N186) responsible for the adenylation of spectinomycin, and two residues (W174 and D179) for streptomycin (Stern et al., 2018).
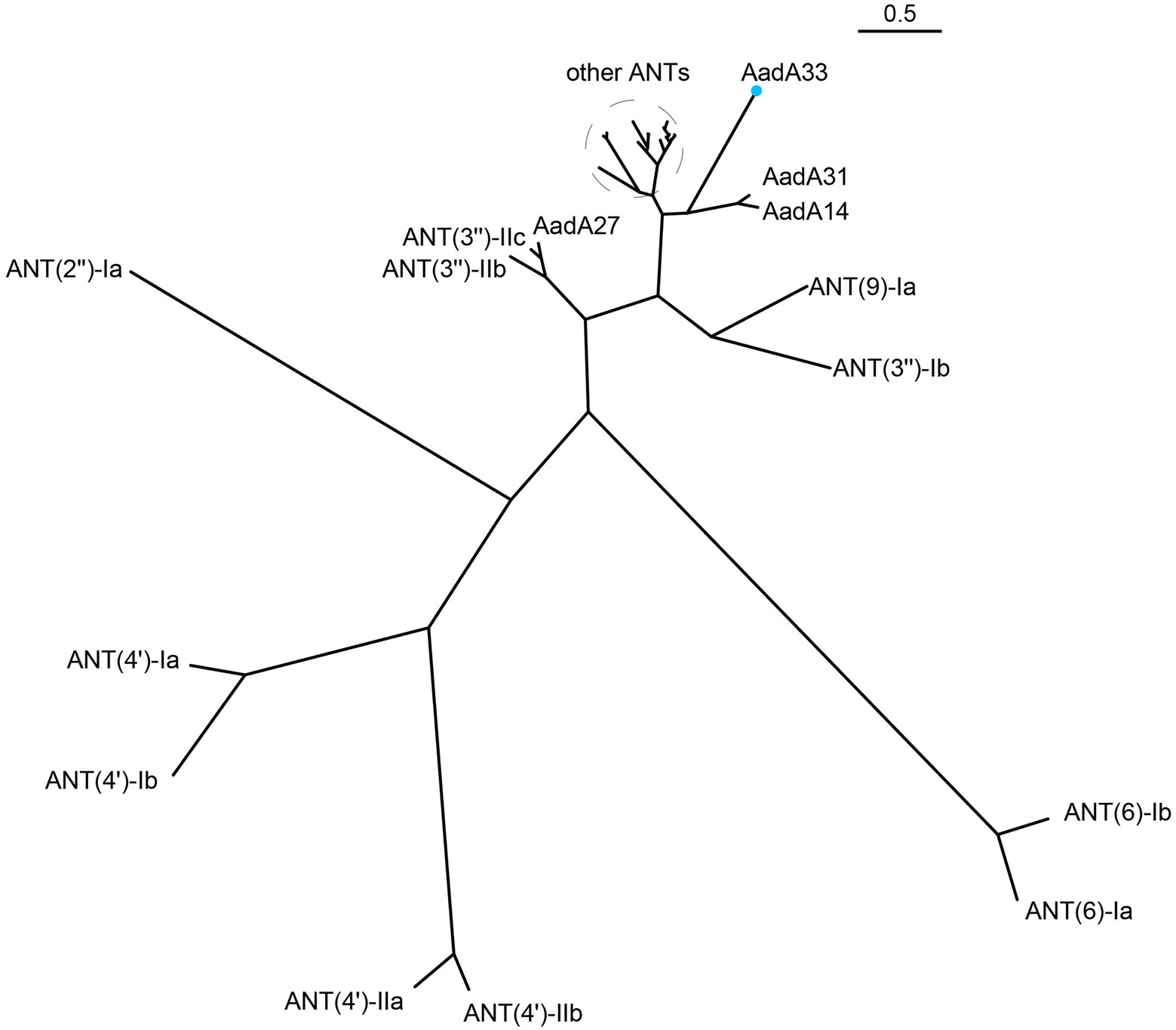
Figure 2. A phylogenetic tree showing the relationship of AadA33 with other functionally characterized ANTs. AadA33 is highlighted with a blue dot. Other ANTs include: AadA1, AadA4, AadA5, AadA6, AadA7, AadA8, AadA9, AadA10, AadA11, AadA13, AadA17, AadA21, AadA23, AadA24, AadA25, AadA28, AadA29, AadA30, ANT(3″)-IIa.
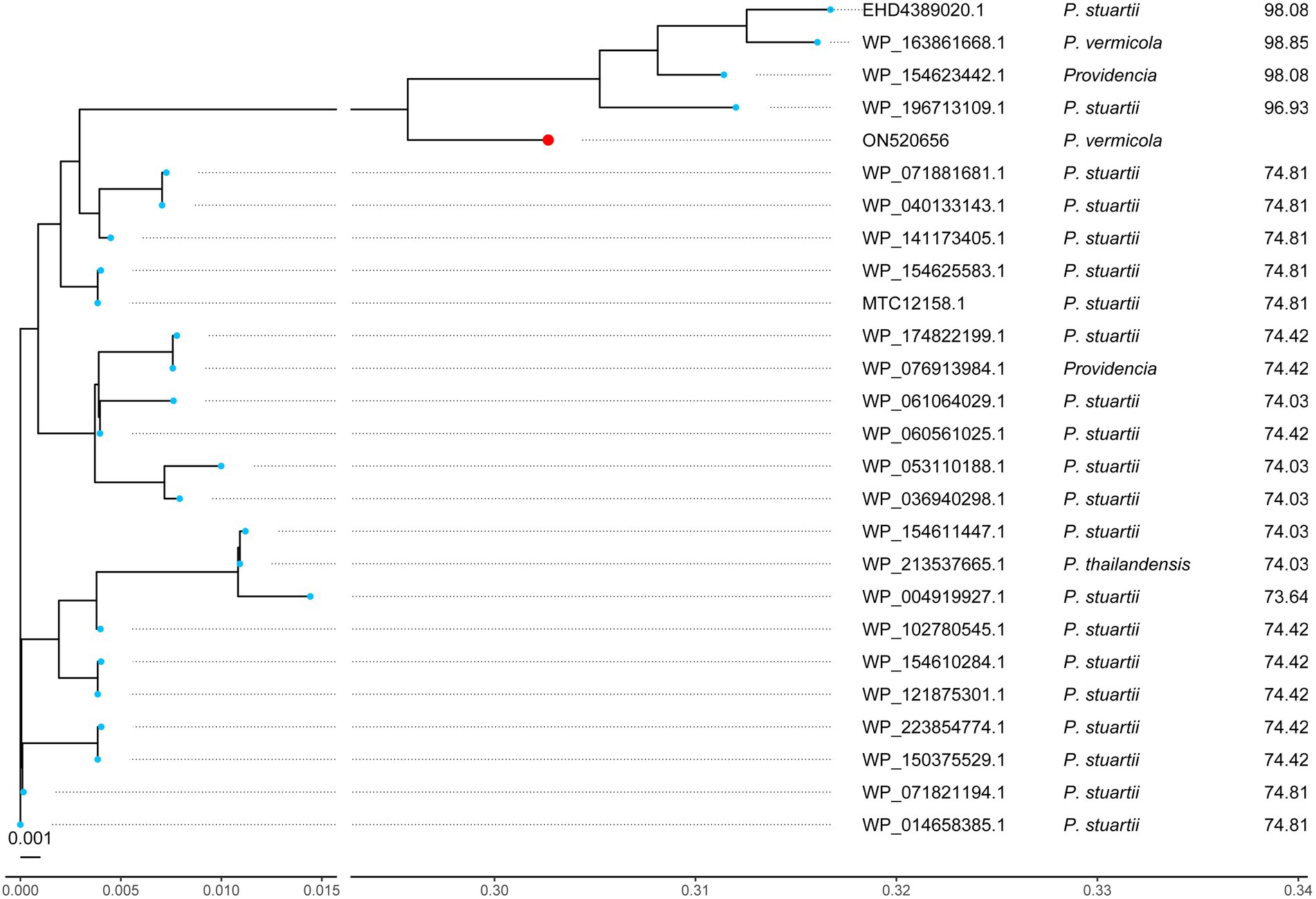
Figure 3. A phylogenetic tree showing the relationship of AadA33 with other putative ANTs. AadA33 is highlighted with a red dot. The three columns on the right represent accession numbers, the taxonomy of that sequence, and amino acid identity (%) with AadA33, respectively.
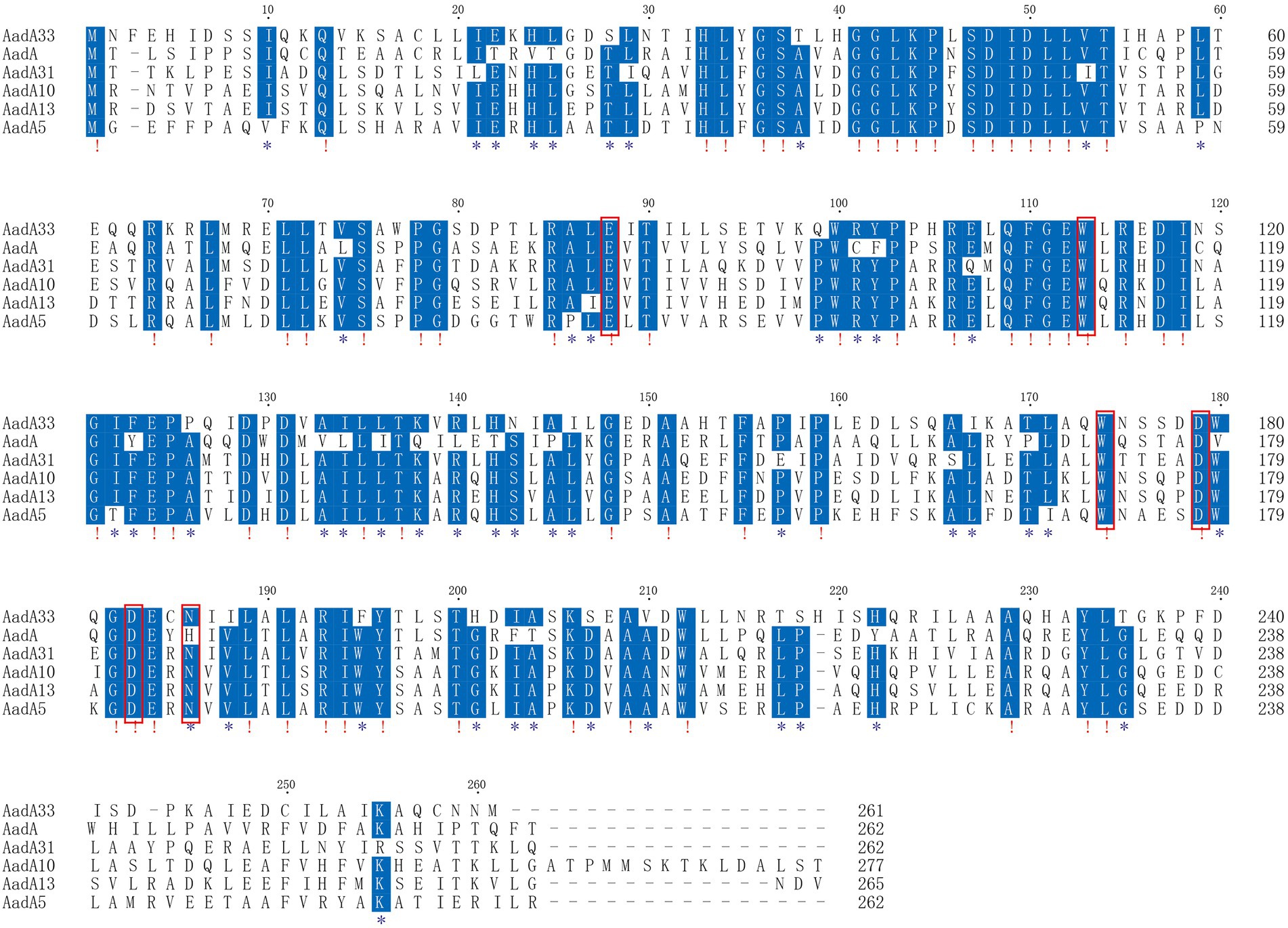
Figure 4. Multiple sequence alignment of AadA33 with other close relatives. Exclamations indicate fully conserved residues; asterisks indicate strongly similar residues; gaps are represented using hyphens. The numbers on the right represent the corresponding sequence length. The red frames indicate functional residues. Accession numbers of AadA proteins: AadA (Q8ZPX9), AadA31 (AUX81654.1), AadA10 (AAL36430.1), AadA13 (ABW91178.1) and AadA5 (AAF17880.1).
To figure out the genetic context of aadA33, the sequences of about 20 kb in length with an aadA33-like gene (with >70 identity to aadA33) at the center were retrieved from the NCBI non-redundant nucleotide database (Figure 5). No mobile genetic element was found in the adjacent regions of aadA33. Among the 16 fragments containing the aadA33-like sequences, most (87.5%, 14/16) were from P. stuartii. The aadA33 encoding fragment of P. vermicola P13 is particularly similar (100% coverage and 99.31% identity) to that of P. vermicola P8538 and P. stuartii CMC-4104.
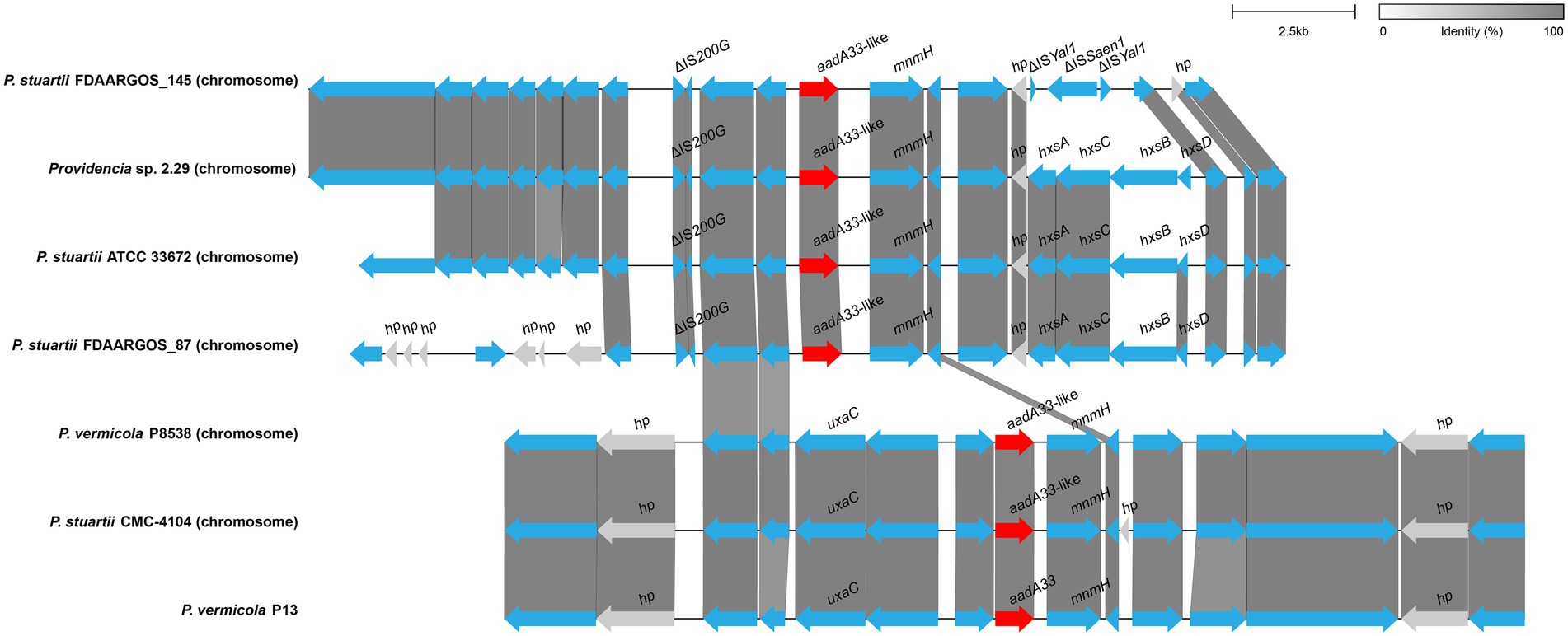
Figure 5. Genetic environment of the aadA33 and aadA33-like genes. Regions with ≥80% amino acid identity were colored grey. Accession numbers: Providencia stuartii FDAARGOS_145 (NZ_CP014024.2), Providencia sp. 2.29 (NZ_CP065420.1), P. stuartii ATCC 33672 (NZ_CP008920.1), P. stuartii FDAARGOS_87 (NZ_CP031508.1), P. vermicola P8538 (NZ_CP048796.1) and P. stuartii CMC-4104 (CP095443.1). hp: hypothetical protein.
Discussion
The aadA33 gene is intrinsic in the genus Providencia. The 25 predicted (hypothetical) homologous ANT protein sequences of AadA33 in NCBI non-redundant protein database and 4 homologous sequences in clinical strains are all from genus Providencia. While some other aadA genes such as aadA5 (Sandvang, 1999), aadA13 (Revilla et al., 2008), and aadA14 (Kehrenberg et al., 2005) were found related to mobile genetic elements (MGEs) encoded on plasmids or the chromosomes, aadA33 was not associated with the MGEs, which suggested that it might be intrinsic in this strain.
AadA belongs to the ANT(3″) gene family. The resistance profile of AadA33 is consistent with the members in the ANT(3″)-Ia group. Like the other AadA enzymes such as AadA10 (Partridge et al., 2002), AadA13 (Revilla et al., 2008), and AadA14 (Kehrenberg et al., 2005), it confers resistance to streptomycin and spectinomycin. Besides, four aminoglycoside 3″-nucleotidyltransferases in the ANT(3″) family with the identities from 33.04% to 47.62% with AadA33, including ANT(3″)-IIa (CAA26199.1), ANT(3″)-IIb (ENU91137.1), ANT(3″)-Ib (QEQ43477.1) and ANT(3″)-IIc (ENU37733.1) were also confirmed to be resistant to streptomycin and spectinomycin (Zhang et al., 2017; Ruiz et al., 2019). It has been shown that ANTs of the different groups showed different resistance spectra. ANT(6)-Ia and ANT(6)-Ib mediate resistance to streptomycin, while ANT(9)-Ia, however, showed resistance to spectinomycin. ANT(2″) confers resistance to gentamicin, dibekacin, kanamycin, sisomicin, and tobramycin, while ANT(4′) mediates resistance to amikacin, dibekacin, isepamicin, and tobramycin. Although AACs and APHs mediate modification of a wide range of aminoglycosides including amikacin, gentamicin, dibekacin, kanamycin, tobramycin, and neomycin, only APH(6)-Ic, APH(6)-Id and APH(3″)-Ic confer resistance to streptomycin, and APH(9) to confer resistance to spectinomycin.
AadA33 contains one conserved protein domain family PRK13746 (aminoglycoside resistance protein). It has been validated that the determinants of spectinomycin and streptomycin resistance of AadA (Q8ZPX9) conferring adenylation on spectinomycin were E87, W112, D182, and H/N185, and on streptomycin are W173 and D178 (Stern et al., 2018). 86E, 180D, and 183N in ANT(9) is essential for its spectinomycin resistance (Kanchugal and Selmer, 2020). Although AadA33 shares an overall low identity of 51.09% with this AadA protein sequence (Q8ZPX9), the six amino acid residues are conserved in AadA33 (with N186 in AadA33). This further confirms the novel resistance gene of this work to be a member of the AadA group.
Conclusion
In this work, we reported a novel aminoglycoside modifying enzyme named AadA33 from the chromosome of P. vermicola P13 isolated from a patient. Encoded in the chromosome, aadA33 was not related to a mobile genetic element. It belongs to the ANTs family and shares the highest amino acid identity with an aminoglycoside O-nucleotidyltransferase AadA31. The novel aminoglycoside modifying enzyme confers strong resistance to streptomycin and spectinomycin, which will be beneficial for the study of the intrinsic resistance mechanism against aminoglycosides in opportunistic pathogens.
Data availability statement
The datasets presented in this study can be found in online repositories. The names of the repository/repositories and accession number(s) can be found in the article/Supplementary material.
Ethics statement
Individual patient data was not involved, and only anonymous clinical residual samples during routine hospital laboratory procedures were used in this study. It was approved by the ethics committee of the Second Affiliated Hospital and Yuying Children’s Hospital of Wenzhou Medical University, Wenzhou, Zhejiang, China.
Author contributions
KL, HZ, YH, QB, and XL: conceived and designed the experiments. CF, MG, WJ, WS, AL, SL, LZ, and JL: performed the experiments. CF, MG, XZ, QL, HL, QB, and XL: data analysis and interpretation. CF, MG, QB, and XL: drafting of the manuscript. All authors contributed to the article and approved the submitted version.
Funding
This study was supported by the Science & Technology Project of Wenzhou City, China (N20210001 and Y2020112), Zhejiang Provincial Natural Science Foundation of China (LY19C060002 and LQ17H190001), and the Natural Science Foundation of China (81973382).
Acknowledgments
The authors would like to acknowledge all study participants and individuals who contributed to this study.
Conflict of interest
The authors declare that the research was conducted in the absence of any commercial or financial relationships that could be construed as a potential conflict of interest.
Publisher’s note
All claims expressed in this article are solely those of the authors and do not necessarily represent those of their affiliated organizations, or those of the publisher, the editors and the reviewers. Any product that may be evaluated in this article, or claim that may be made by its manufacturer, is not guaranteed or endorsed by the publisher.
Supplementary material
The Supplementary material for this article can be found online at: https://www.frontiersin.org/articles/10.3389/fmicb.2022.990739/full#supplementary-material
SUPPLEMENTARY FIGURE S1 | SDS-PAGE of AadA33. Lane 1: PageRuler Prestained Protein adder (Thermo Fisher Scientific, product code: 26616); lane 2 and 3: uncleaved AadA33 with His6 tag; lane 4: cleaved AadA33 with thrombin.
Footnotes
1. ^https://www.cdc.gov/narms/antibiotics-tested.html
2. ^https://github.com/arpcard/rgi
3. ^https://www.ncbi.nlm.nih.gov/Structure/cdd/wrpsb.cgi
References
Aires, J. R., Köhler, T., Nikaido, H., and Plésiat, P. (1999). Involvement of an active efflux system in the natural resistance of Pseudomonas aeruginosa to aminoglycosides. Antimicrob. Agents Chemother. 43, 2624–2628. doi: 10.1128/AAC.43.11.2624
Buchfink, B., Reuter, K., and Drost, H.-G. (2021). Sensitive protein alignments at tree-of-life scale using DIAMOND. Nat. Methods 18, 366–368. doi: 10.1038/s41592-021-01101-x
Ciufo, S., Kannan, S., Sharma, S., Badretdin, A., Clark, K., Turner, S., et al. (2018). Using average nucleotide identity to improve taxonomic assignments in prokaryotic genomes at the NCBI. Int. J. Syst. Evol. Microbiol. 68, 2386–2392. doi: 10.1099/ijsem.0.002809
Doi, Y., Wachino, J.-I., and Arakawa, Y. (2016). Aminoglycoside resistance: the emergence of acquired 16S ribosomal RNA Methyltransferases. Infect. Dis. Clin. N. Am. 30, 523–537. doi: 10.1016/j.idc.2016.02.011
Douka, E., Perivolioti, E., Kraniotaki, E., Fountoulis, K., Economidou, F., Tsakris, A., et al. (2015). Emergence of a pandrug-resistant VIM-1-producing Providencia stuartii clonal strain causing an outbreak in a Greek intensive care unit. Int. J. Antimicrob. Agents 45, 533–536. doi: 10.1016/j.ijantimicag.2014.12.030
Gilchrist, C. L. M., and Chooi, Y.-H. (2021). Clinker & clustermap. Js: automatic generation of gene cluster comparison figures. Bioinformatics 37, 2473–2475. doi: 10.1093/bioinformatics/btab007
Jain, C., Rodriguez-R, L. M., Phillippy, A. M., Konstantinidis, K. T., and Aluru, S. (2018). High throughput ANI analysis of 90K prokaryotic genomes reveals clear species boundaries. Nat. Commun. 9:5114. doi: 10.1038/s41467-018-07641-9
Kanchugal, P. S., and Selmer, M. (2020). Structural recognition of spectinomycin by resistance enzyme ANT(9) from enterococcus faecalis. Antimicrob. Agents Chemother. 64:e00371-20. doi: 10.1128/AAC.00371-20
Katoh, K., and Standley, D. M. (2013). MAFFT multiple sequence alignment software version 7: improvements in performance and usability. Mol. Biol. Evol. 30, 772–780. doi: 10.1093/molbev/mst010
Kehrenberg, C., Catry, B., Haesebrouck, F., de Kruif, A., and Schwarz, S. (2005). Novel spectinomycin/streptomycin resistance gene, aadA14, from Pasteurella multocida. Antimicrob. Agents Chemother. 49, 3046–3049. doi: 10.1128/AAC.49.7.3046-3049.2005
Kim, C., Hesek, D., Zajícek, J., Vakulenko, S. B., and Mobashery, S. (2006). Characterization of the bifunctional aminoglycoside-modifying enzyme ANT(3)-ii/AAC(6)-IId from Serratia marcescens. Biochemistry 45, 8368–8377. doi: 10.1021/bi060723g
Kolmogorov, M., Yuan, J., Lin, Y., and Pevzner, P. A. (2019). Assembly of long, error-prone reads using repeat graphs. Nat. Biotechnol. 37, 540–546. doi: 10.1038/s41587-019-0072-8
Konstantinidis, K. T., and Tiedje, J. M. (2005). Genomic insights that advance the species definition for prokaryotes. Proc. Natl. Acad. Sci. U. S. A. 102, 2567–2572. doi: 10.1073/pnas.0409727102
Lupande-Mwenebitu, D., Khedher, M. B., Khabthani, S., Rym, L., Phoba, M.-F., Nabti, L. Z., et al. (2021). First genome description of Providencia vermicola isolate bearing NDM-1 from blood culture. Microorganisms 9:1751. doi: 10.3390/microorganisms9081751
McArthur, A. G., Waglechner, N., Nizam, F., Yan, A., Azad, M. A., Baylay, A. J., et al. (2013). The comprehensive antibiotic resistance database. Antimicrob. Agents Chemother. 57, 3348–3357. doi: 10.1128/AAC.00419-13
Minh, B. Q., Schmidt, H. A., Chernomor, O., Schrempf, D., Woodhams, M. D., von Haeseler, A., et al. (2020). IQ-TREE 2: new models and efficient methods for phylogenetic inference in the genomic era. Mol. Biol. Evol. 37, 1530–1534. doi: 10.1093/molbev/msaa015
Oikonomou, O., Liakopoulos, A., Phee, L. M., Betts, J., Mevius, D., and Wareham, D. W. (2016). Providencia stuartii isolates from Greece: co-carriage of cephalosporin (blaSHV-5, blaVEB-1), Carbapenem (blaVIM-1), and aminoglycoside (rmtB) resistance determinants by a multidrug-resistant outbreak clone. Microb. Drug Resist. 22, 379–386. doi: 10.1089/mdr.2015.0215
Over, U., Gür, D., Unal, S., and Miller, G. H., Aminoglycoside Resistance Study Group (2001). The changing nature of aminoglycoside resistance mechanisms and prevalence of newly recognized resistance mechanisms in Turkey. Clin. Microbiol. Infect. 7, 470–478. doi: 10.1046/j.1198-743x.2001.00284.x
Partridge, S. R., Collis, C. M., and Hall, R. M. (2002). Class 1 integron containing a new gene cassette, aadA10, associated with Tn1404 from R151. Antimicrob. Agents Chemother. 46, 2400–2408. doi: 10.1128/AAC.46.8.2400-2408.2002
Petkau, A., Stuart-Edwards, M., Stothard, P., and Van Domselaar, G. (2010). Interactive microbial genome visualization with GView. Bioinformatics 26, 3125–3126. doi: 10.1093/bioinformatics/btq588
Qing, G., Ma, L.-C., Khorchid, A., Swapna, G. V. T., Mal, T. K., Takayama, M. M., et al. (2004). Cold-shock induced high-yield protein production in Escherichia coli. Nat. Biotechnol. 22, 877–882. doi: 10.1038/nbt984
Ramirez, M. S., and Tolmasky, M. E. (2010). Aminoglycoside modifying enzymes. Drug Resist. Updat. 13, 151–171. doi: 10.1016/j.drup.2010.08.003
Revilla, C., Garcillán-Barcia, M. P., Fernández-López, R., Thomson, N. R., Sanders, M., Cheung, M., et al. (2008). Different pathways to acquiring resistance genes illustrated by the recent evolution of IncW plasmids. Antimicrob. Agents Chemother. 52, 1472–1480. doi: 10.1128/AAC.00982-07
Rice, L. B. (2008). Federal funding for the study of antimicrobial resistance in nosocomial pathogens: no ESKAPE. J. Infect. Dis. 197, 1079–1081. doi: 10.1086/533452
Richter, M., and Rosselló-Móra, R. (2009). Shifting the genomic gold standard for the prokaryotic species definition. Proc. Natl. Acad. Sci. U. S. A. 106, 19126–19131. doi: 10.1073/pnas.0906412106
Ruiz, C., McCarley, A., Espejo, M. L., Cooper, K. K., and Harmon, D. E. (2019). Comparative genomics reveals a well-conserved intrinsic resistome in the emerging multidrug-resistant pathogen Cupriavidus gilardii. mSphere 4:e00631-19. doi: 10.1128/mSphere.00631-19
Sandvang, D. (1999). Novel streptomycin and spectinomycin resistance gene as a gene cassette within a class 1 integron isolated from Escherichia coli. Antimicrob. Agents Chemother. 43, 3036–3038. doi: 10.1128/AAC.43.12.3036
Seemann, T. (2014). Prokka: rapid prokaryotic genome annotation. Bioinformatics 30, 2068–2069. doi: 10.1093/bioinformatics/btu153
Shaw, K. J., Rather, P. N., Hare, R. S., and Miller, G. H. (1993). Molecular genetics of aminoglycoside resistance genes and familial relationships of the aminoglycoside-modifying enzymes. Microbiol. Rev. 57, 138–163. doi: 10.1128/mr.57.1.138-163.1993
Shi, J., Jin, Y., Bian, T., Li, K., Sun, Z., Cheng, Z., et al. (2015). SuhB is a novel ribosome associated protein that regulates expression of MexXY by modulating ribosome stalling in Pseudomonas aeruginosa. Mol. Microbiol. 98, 370–383. doi: 10.1111/mmi.13126
Shin, S., Jeong, S. H., Lee, H., Hong, J. S., Park, M.-J., and Song, W. (2018). Emergence of multidrug-resistant Providencia rettgeri isolates co-producing NDM-1 carbapenemase and PER-1 extended-spectrum β-lactamase causing a first outbreak in Korea. Ann. Clin. Microbiol. Antimicrob. 17:20. doi: 10.1186/s12941-018-0272-y
Somvanshi, V. S., Lang, E., Sträubler, B., Spröer, C., Schumann, P., Ganguly, S., et al. (2006). Providencia vermicola sp. nov., isolated from infective juveniles of the entomopathogenic nematode Steinernema thermophilum. Int. J. Syst. Evol. Microbiol. 56, 629–633. doi: 10.1099/ijs.0.63973-0
Souvorov, A., Agarwala, R., and Lipman, D. J. (2018). SKESA: strategic k-mer extension for scrupulous assemblies. Genome Biol. 19:153. doi: 10.1186/s13059-018-1540-z
Stern, A. L., Van der Verren, S. E., Kanchugal, P. S., Näsvall, J., Gutiérrez-de-Terán, H., and Selmer, M. (2018). Structural mechanism of AadA, a dual-specificity aminoglycoside adenylyltransferase from Salmonella enterica. J. Biol. Chem. 293, 11481–11490. doi: 10.1074/jbc.RA118.003989
Tshisevhe, V. S., Lekalakala, M. R., Tshuma, N., Janse van Rensburg, S., and Mbelle, N. (2016). Outbreak of carbapenem-resistant Providencia rettgeri in a tertiary hospital. S. Afr. Med. J. 107, 31–33. doi: 10.7196/SAMJ.2016.v107.i1.12002
Walker, B. J., Abeel, T., Shea, T., Priest, M., Abouelliel, A., Sakthikumar, S., et al. (2014). Pilon: an integrated tool for comprehensive microbial variant detection and genome assembly improvement. PLoS One 9:e112963. doi: 10.1371/journal.pone.0112963
Wick, R. R., Judd, L. M., Cerdeira, L. T., Hawkey, J., Méric, G., Vezina, B., et al. (2021). Trycycler: consensus long-read assemblies for bacterial genomes. Genome Biol. 22, 266. doi: 10.1186/s13059-021-02483-z
Yu, G., Smith, D. K., Zhu, H., Guan, Y., and Lam, T. T. (2017). GGTREE: an R package for visualization and annotation of phylogenetic trees with their covariates and other associated data. Methods Ecol. Evol. 8, 28–36. doi: 10.1111/2041-210X.12628
Zhang, G., Leclercq, S. O., Tian, J., Wang, C., Yahara, K., Ai, G., et al. (2017). A new subclass of intrinsic aminoglycoside nucleotidyltransferases, ANT(3)-II, is horizontally transferred among Acinetobacter spp. by homologous recombination. PLoS Genet. 13:e1006602. doi: 10.1371/journal.pgen.1006602
Keywords: aminoglycoside O-nucleotidyltransferase, AadA33, Providencia vermicola, enzyme kinetics, novel antimicrobial resistance gene
Citation: Feng C, Gao M, Jiang W, Shi W, Li A, Liu S, Zhang L, Zhang X, Li Q, Lin H, Lu J, Li K, Zhang H, Hu Y, Bao Q and Lin X (2022) Identification of a novel aminoglycoside O-nucleotidyltransferase AadA33 in Providencia vermicola. Front. Microbiol. 13:990739. doi: 10.3389/fmicb.2022.990739
Edited by:
Chang-Wei Lei, Sichuan University, ChinaReviewed by:
Yingshun Zhou, Southwest Medical University, ChinaShangshang Qin, Zhengzhou University, China
Copyright © 2022 Feng, Gao, Jiang, Shi, Li, Liu, Zhang, Zhang, Li, Lin, Lu, Li, Zhang, Hu, Bao and Lin. This is an open-access article distributed under the terms of the Creative Commons Attribution License (CC BY). The use, distribution or reproduction in other forums is permitted, provided the original author(s) and the copyright owner(s) are credited and that the original publication in this journal is cited, in accordance with accepted academic practice. No use, distribution or reproduction is permitted which does not comply with these terms.
*Correspondence: Qiyu Bao, YmFvcXlAZ2Vub21pY3MuY24=; Xi Lin, MTg3NTE4ODAxNTBAMTYzLmNvbQ==