- 1Faculty of Education, Mie University, Tsu, Mie, Japan
- 2Mie University Zebrafish Drug Screening Center, Tsu, Japan
- 3Graduate School of Regional Innovation Studies, Mie University, Tsu, Mie, Japan
- 4Department of Integrative Pharmacology, Mie University Graduate School of Medicine, Tsu, Japan
- 5Department of Bioinformatics, Mie University Advanced Science Research Promotion Center, Tsu, Japan
Interventions to the gut microbiome manipulate the gut–brain axis and could be useful in the treatment of anxiety and depression. In this study, we demonstrated that administration of the bacterium Paraburkholderia sabiae reduces anxiety-like behavior in adult zebrafish. P. sabiae administration increased the diversity of the zebrafish gut microbiome. Linear discriminant analysis Effect Size (LEfSe) analysis revealed that the populations of Actinomycetales including Nocardiaceae, Nocardia, Gordoniaceae, Gordonia, Nakamurellaceae, and Aeromonadaceae were reduced, whereas those of Rhizobiales including Xanthobacteraceae, Bradyrhizobiaceae, Rhodospirillaceae, and Pirellulaceae were increased in the gut microbiome. Functional analysis using Phylogenetic Investigation of Communities by Reconstruction of Unobserved States (PICRUSt2) predicted that P. sabiae administration altered taurine metabolism in the zebrafish gut, and we demonstrated that P. sabiae administration increased the taurine concentration in the brain. Since taurine functions as an antidepressant neurotransmitter in vertebrates, our results suggest that P. sabiae could improve anxiety-like behavior in zebrafish via the gut-brain axis.
1. Introduction
The gut microbiome affects brain development, myelination patterns (Gacias et al., 2016; Hoban et al., 2016), and host behaviors (Vuong et al., 2017) by modulating neurotransmitter synthesis in the gut and brain (Needham et al., 2020; Huang and Wu, 2021). Metabolites produced by the gut microbiome are distributed throughout all organs, including the brain, thereby affecting neuronal cell development and behaviors (Swann et al., 2017). For example, Lactobacillus rhamnosus JB-1, Lactobacillus helveticus R0052, and Bifidobacterium longum R0175 reduce stress-induced corticosterone as well as anxiety- and depression-related behaviors in rodents (Bravo et al., 2011; Messaoudi et al., 2011). Lactobacillus helveticus R0052 and Bifidobacterium longum R0175 also alleviated psychological distress in human subjects (Messaoudi et al., 2011). Moreover, consumption of fermented milk containing Bifidobacterium animalis subsp. lactis, Streptococcus thermophiles, Lactobacillus bulgaricus, and Lactococcus lactis subsp. lactis altered the activity of brain regions that control the central processing of emotion and sensation in healthy women (Tillisch et al., 2013). In addition to probiotic studies, Needham et al. (2022) reported that a gut-derived molecule, 4-ethylphenyl sulfate, modulates anxiety-like behavior in mice by affecting oligodendrocyte function and myelin patterning in the mouse brain. These studies suggest the possibility that interventions to the gut microbiome manipulate the gut–brain axis and could be useful in the treatment of anxiety and depression.
Zebrafish (Danio rerio) is becoming a major animal model for neurobehavioral research. Zebrafish exhibit numerous behaviors that correlate with human neurological processes and disorders, such as anxiety (Li et al., 2015), learning (Andersson et al., 2015), fear (Colwill and Creton, 2011), sociability (Engeszer et al., 2007), and psychosis (Giacomini et al., 2006). The zebrafish neurotransmitter system is similar to that of other vertebrates, including rodents and humans (Rico et al., 2011). Several zebrafish neurobehavioral tests have been developed and validated using chemicals and natural compounds. The novel tank diving test, which is suitable for evaluating anxiety-like behavior, is commonly used for drug testing, chemical screening, and mechanism identification (Wong et al., 2013). Similar to humans, the gut microbiome also affects the normal neurobehavioral phenotypes in zebrafish (Stagaman et al., 2020). For example, impairment of microbial colonization in zebrafish larvae using antibiotics caused hyperactivity (Phelps et al., 2017). Dietary administration of L. rhamnosus IMC 501 affects brain-derived neurotrophic factor (BDNF) levels, serotonin metabolism, and shoaling behavior in zebrafish (Borrelli et al., 2016). Lactobacillus plantarum administration alters GABAergic and serotonergic signaling in the brain and reduces anxiety-like behavior in zebrafish (Davis et al., 2016).
In this study, we focused on the bacterium Paraburkholderia, whose functions in the human and rodent gut microbiome have been previously discussed (Haonon et al., 2021; Zhang et al., 2021; Ning et al., 2022). Among these, we identified that administration of Paraburkholderia sabiae reduced anxiety traits in zebrafish. P. sabiae administration increased the diversity of the zebrafish gut microbiome and altered the taurine metabolism in zebrafish.
2. Materials and methods
2.1. Paraburkholderia sabiae exposure to the zebrafish
Wild-type zebrafish (Danio rerio; AB strain, Zebrafish International Research Center, Eugene, OR, United States) were raised in a flow-through system at 28 ± 0.5°C under a 14/10 h light/dark cycle at the Zebrafish Drug Screening Center, Mie University, Japan. Ten AB wild type 6 months-old zebrafish were transferred to 2 L of rearing water at 28°C. The fish were fed at 9:00 a.m. and 5:00 p.m. on weekdays. For 1 month, P. sabiae cells (1.0 × 109 cell/L) were administered in the rearing water twice a day at feeding times. Paraburkholderia sabiae was purchased from the German Collection of Microorganisms and details for the culture is described in Supplementary online materials. The experimental procedures were performed in accordance with the Japanese Animal Welfare Regulatory Practice Act on Welfare and Management of Animals (Ministry of the Environment of Japan) and in compliance with ARRIVE guidelines1. Ethical approval from the local Institutional Animal Care and Use Committee was not sought, because this law does not mandate the protection of zebrafish.
2.2. Evaluation of the anxiety-like behavior in zebrafish
Anxiety in zebrafish was evaluated using the novel tank diving test (Haghani et al., 2019; Anwer et al., 2021) from 14:00 p.m. to 15:30 p.m. An observation tank (149 mm × 38 mm × 100 mm) was filled with rearing water at a height of 10 cm. The zebrafish were transferred from the rearing tank to a restraint tank (37 mm × 7 mm × 65 mm) with a net. The zebrafish were placed in a restraint tank for 5 min to cause anxiety and transferred to the observation tank. When the zebrafish were transferred to the observation tank, their movements were immediately captured as videos using an iPad Pro for 2 min. The average speed, acceleration, total travel distance, and exploration rate in the observation tank were analyzed using the ToxTrac software (Rodriguez et al., 2018). Supplementary Figure S1 shows a schematic of the evaluation of zebrafish anxiety.
2.3. Gut microbiome analysis in zebrafish
DNA was extracted from the zebrafish intestine using a Quick-DNA Fecal/Soil Microbe Miniprep Kit (Zymo Research, Irvine, CA, United States). The 16S rRNA V3/V4 region in the DNA was amplified by PCR, and Library preparation and sequencing were performed using the MiSeq Reagent Kit v3 (Illumina, San Diego, CA, USA) at 2 × 300 bp. The microbiome data were analyzed by phylogenetic investigation of communities by reconstruction of unobserved states 2 (PICRUSt2) ver. 2.3.0 b 32 (Douglas et al., 2020). Linear discriminant analysis effect size (LEfSe; Segata et al., 2011). For details, please see the Supplementary online materials.
2.4. Measurement of taurine and serotonin concentrations in the zebrafish brains
Zebrafish brains were thoroughly homogenized using a pestle in 100 μl PBS cooled on ice. The supernatant was collected by centrifugation at 14,500 rpm for 2 min. One microliter of the 10-fold diluted supernatant was used to measure taurine and serotonin concentrations using the Taurine Assay Kit (Cell Biolabs, San Diego, CA, USA) and Serotonin ELISA Kit (Immusmol, Bordeaux, France), respectively, according to the manufacturers’ protocols.
3. Results
3.1. Paraburkholderia sabiae administration reduced anxiety-like behavior in zebrafish
Six months-old zebrafish were exposed to P. sabiae bacterial cells for 1 month, and their anxiety-like behavior was evaluated using a novel tank diving test, which has become a standard test for determining anxiety-like behavior in zebrafish (Haghani et al., 2019; Supplementary Figure S1; Supplementary movies S1, S2). The average speed, average acceleration, and total distance tended to be higher in P. sabiae-exposed zebrafish. The average speeds were 45.1 mm/s and 60.8 mm/s, the average accelerations were 137.8 mm/s2 and 190.0 mm/s2, and the total distances were 5,566 mm and 7,501 mm in the control and the P. sabiae-exposed zebrafish, respectively (Figure 1A). Furthermore, P. sabiae-exposed zebrafish migrated significantly more extensively in the observation tank. The exploration rate in the observation tank was 32% for the control zebrafish, whereas that for the P. sabiae-exposed zebrafish was 49% (p < 0.05; Figures 1A,B). There was no significant difference in exploration rate between the genders (Supplementary Figure S2). The response of adult zebrafish in the novel tank environment, that is, to initially stay at the bottom of the tank and eventually acclimating to the rest of the tank, is interpreted as a precautionary anti-predator response and anxiety reduction, respectively (Haghani et al., 2019). Our results indicated that P. sabiae administration reduced anxiety in zebrafish.
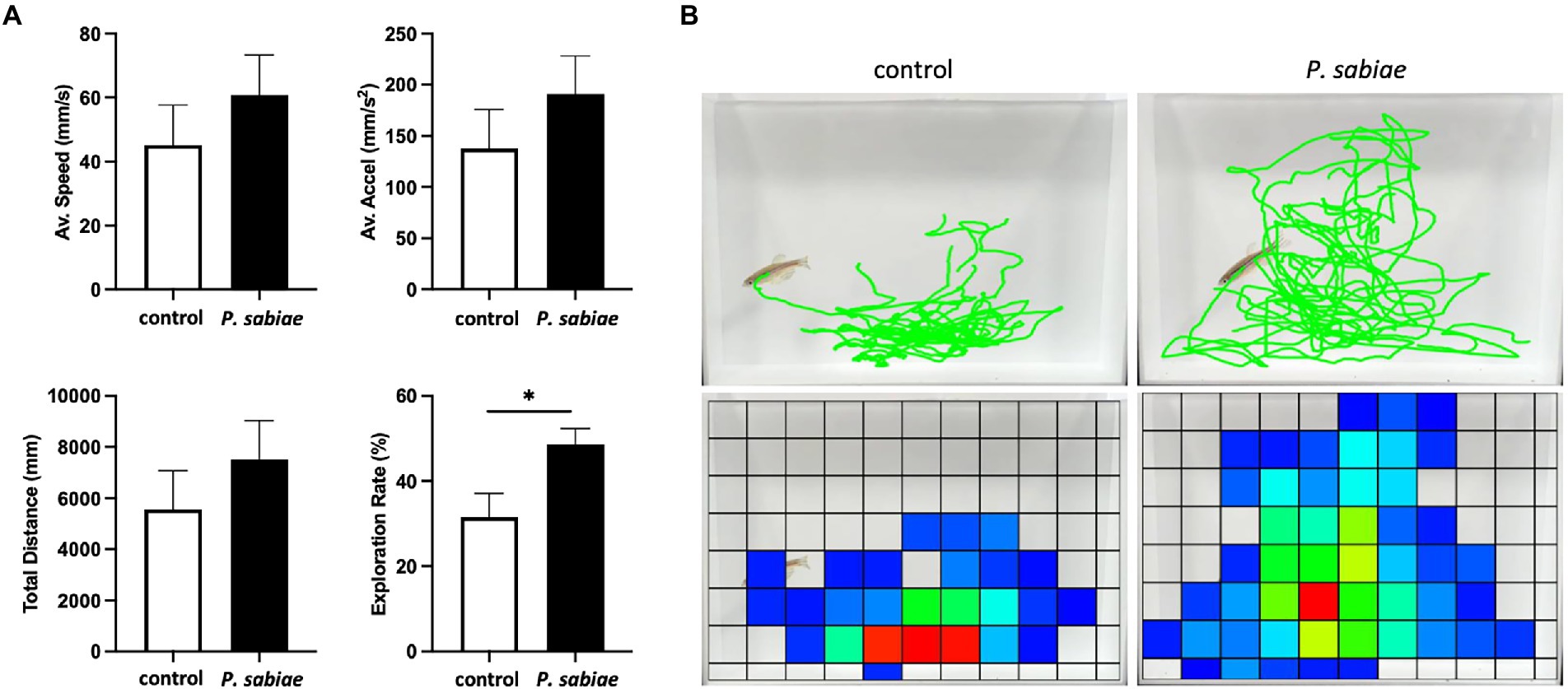
Figure 1. The zebrafish anxiety behaviors evaluated by the novel tank diving test. (A) Movements of the zebrafish following the transfer into the observation tank were traced for 2 min. The average speed (mm/s), average acceleration (mm/s2), total travel distance (mm), and exploration rate in the observation tank (%) of the zebrafish are presented. The error bars indicate standard error. N = 5, *p < 0.05. (B) The representative images of the traced movement of the P. sabiae-exposed and the control zebrafish.
Multiple behaviors, including stress and anxiety, are modulated by neuropeptides (Rana et al., 2022). The altered expression of bdnf in distinct brain areas and BDNF signaling associated with social behavior have been frequently demonstrated in gut-brain axis models in mice (Bercik et al., 2010; Diaz Heijtz et al., 2011; Desbonnet et al., 2015). We also evaluated the expression levels of anxiety-related genes, neuropeptide Y npy (Shiozaki et al., 2020), isotocin (oxtI) (Godwin and Thompson, 2012), and bdnf (Bjorkholm and Monteggia, 2016). oxti expression tended to increase in the brains of P. sabiae-exposed fish, whereas npy expression decreased (no significance, Supplementary Figure S3). bdnf expression also tended to increase in the P. sabiae-exposed fish brain, similar to the results of a previous zebrafish study (Borrelli et al., 2016).
3.2. Paraburkholderia sabiae administration increased the microbial diversity in the zebrafish gut
The gut microbiomes of the five P. sabiae-exposed zebrafish were analyzed using the 16S rRNA V3/V4 region of their gut microbial DNAs. The 30,000–50,000 pair-reads, 93.1–94.1 Q20 values, and 83.5–85.3 Q30 values were obtained for each sample. A total of 450 OTUs were generated from the analysis using Qiime2 (Supplementary Table S2). Despite the continuous feeding of the bacterial cells to the zebrafish for 1 month, the observed OTUs in the P. sabiae-exposed zebrafish gut microbiome tended to be higher than those in the control zebrafish. Chao1, the richness index of alpha diversity in the gut microbiome, was significantly higher in the P. sabiae-exposed zebrafish than in the control zebrafish (p < 0.05; Figure 2A). No significant difference in the evenness index of the Shannon diversity index and the indicator of phylogenetic diversity, Faith_pd, was observed between these zebrafish gut microbiomes. P. sabiae was not detected in the gut microbiome analysis (Supplementary Table S2); thus, P. sabiae functioned without colonizing the zebrafish gut.
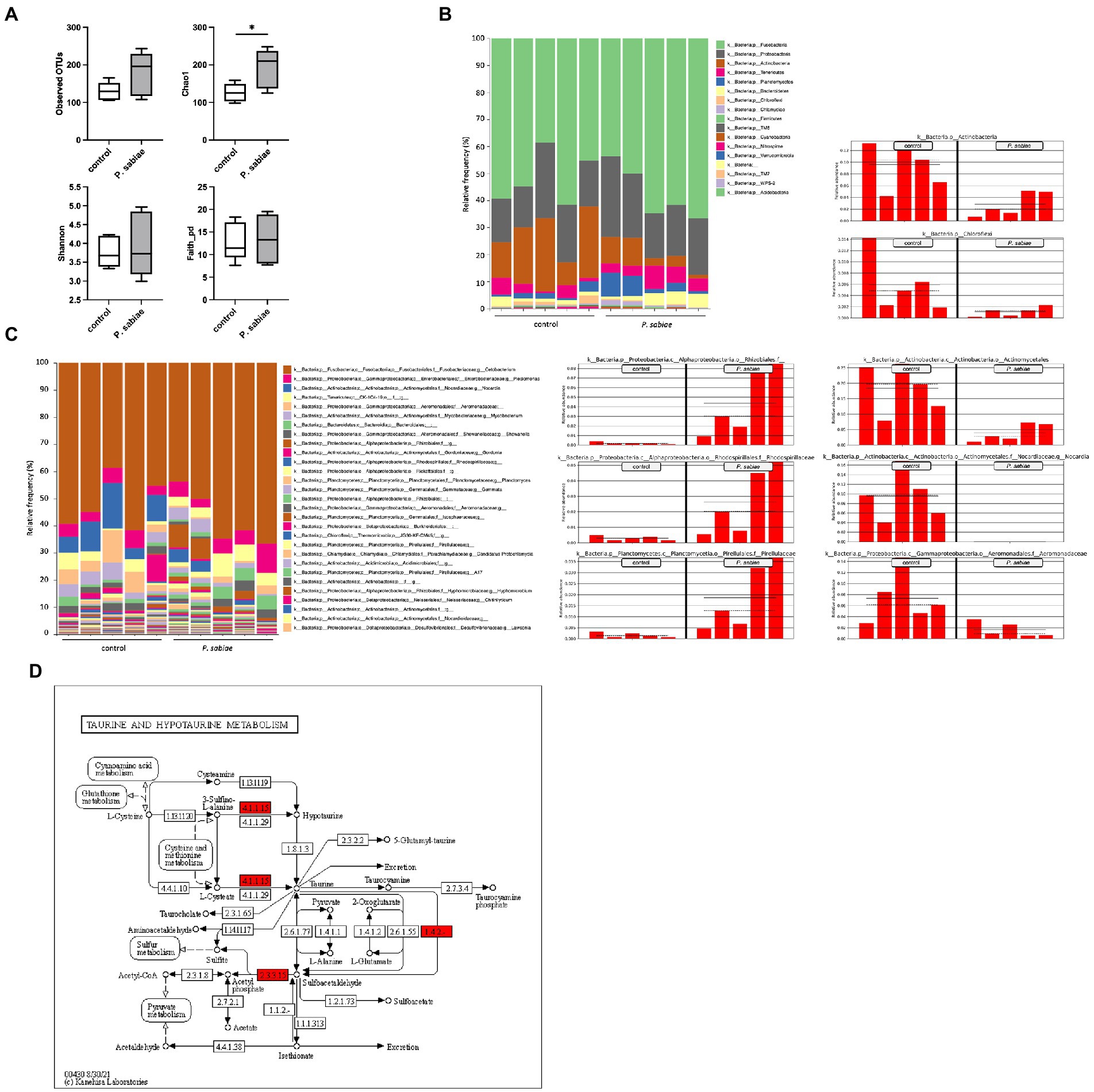
Figure 2. The gut microbiome analysis of the P. sabiae-exposed zebrafish. (A) The diversity indices, Observed OTUs, Chao1, and Shannon, Faith_pd in the P. sabiae-exposed and the control zebrafish gut microbiomes. All OTUs detected by the microbiome analysis are summarized in Supplementary Table S2. (B) The phylum level and (C) genus level analyses of the zebrafish gut microbiome were conducted. The representative OTUs which increased and decreased in the P. sabiae-exposed zebrafish gut microbiomes are extracted by the LEfSe analysis. All OTUs extracted by the LEfSe analysis are summarized in Supplementary Table S3. (D) The PICRUSt2 functional analysis with METACYC database revealed that the functions of superpathway of taurine degradation (PWY-1541) was significantly reduced in the P. sabiae-exposed zebrafish gut microbiome (Supplementary Table S7). The predicted taurine metabolism pathways are represented with KEGG Mapper. Reduced functions in the taurine metabolic pathway in the P. sabiae-exposed zebrafish gut microbiome are shown as red. N = 5, *p < 0.05.
LEfSe analysis was used to compare the bacterial populations between the P. sabiae-exposed and control zebrafish gut microbiomes (Supplementary Table S3; Segata et al., 2011). At the phylum level, Fusobacteria and Proteobacteria were dominant in the zebrafish gut microbiome, which is consistent with previous reports (Borrelli et al., 2016; Davis et al., 2016). Actinobacteria and Chloroflexi populations were smaller in the gut microbiome of P. sabiae-exposed zebrafish than in that of control zebrafish (Figure 2B). At the genus level, Cetobacterium was detected as the major bacterium in the gut microbiome of zebrafish. The populations of Actinomycetales, including Nocardiaceae (p < 0.01), Nocardia (p < 0.01), Gordoniaceae (p < 0.01), Gordonia (p < 0.01), Nakamurellaceae (p < 0.01), and Aeromonadaceae (p < 0.05) were significantly reduced, whereas those of Rhizobiales, including Xanthobacteraceae (p < 0.05) and Bradyrhizobiaceae (p < 0.05), Rhodospirillaceae (p < 0.01), and Pirellulaceae (p < 0.01) were significantly increased in the P. sabiae group (Figure 2C; Supplementary Table S3).
Functional composition tables based on EC number, Kyoto Encyclopedia of Genes and Genomes (KEGG) ortholog, cluster of orthologous groups, and METACYC were generated using PICRUSt2 (Supplementary Tables S4–S7; Douglas et al., 2020). Functional analysis based on METACYC revealed that the mevalonate pathway (PWY-6174), superpathway of polyamine biosynthesis (PWY-6565), and chitin derivative degradation (PWY-6906) were detected only in the P. sabiae-exposed zebrafish gut microbiome. In contrast, the functions of the superpathways of taurine degradation (PWY-1541) (p < 0.01), creatinine degradation (PWY-4722) (p < 0.01), and aerobic toluene degradation via catechol (PWY-5178) (p < 0.001) were significantly reduced in the P. sabiae-exposed zebrafish gut microbiome (Supplementary Table S7). Regarding the neurotransmitter taurine, glutamate decarboxylase [EC:4.1.1.15] (p < 0.01; Supplementary Table S4) and the functions of tauY: taurine dehydrogenase large subunit [EC:1.4.2.-] (K07256) (p < 0.05), tauA: taurine transport system substrate-binding protein (K07256) (p < 0.05; Supplementary Table S5), ABC-type taurine transport system, periplasmic component (COG4521; p < 0.05), and ATPase component (COG4525) (p < 0.01; Supplementary Table S6) were also reduced in the P. sabiae-exposed zebrafish gut microbiome. The reduced functions in the metabolic pathways of taurine and hypotaurine were represented by the KEGG Mapper (Kanehisa et al., 2022; Figure 2D).
3.3. Effects of Paraburkholderia sabiae on taurine levels in the zebrafish brain
Because PICRUSt2 predicted downregulation of the superpathway of taurine degradation (PWY-1541) in the zebrafish gut microbiome, we next evaluated taurine levels in zebrafish. The concentration of taurine (2-aminoethanesulfonic acid) in the P. sabiae group was significantly (p < 0.01) higher than that in the control zebrafish brain:32.2 and 10.4 nmol/mg-brain, respectively (Figure 3A). We also measured the taurine level of intestinal contents (microbiota) and blood, and found that P. sabiae administration shows a tendency (p < 0.2) to increase taurine in the intestinal contents (Supplementary Figure S4). The expression level of the taurine biosynthesis gene encoding cysteine dioxygenase cdo, was 2.0 times higher than that in the P. sabiae-exposed zebrafish brains, compared with that in the control zebrafish brains (p < 0.1), while no difference was observed in those of the other taurine biosynthesis genes encoding cysteamine dioxygenase ado, cysteine sulfinic acid decarboxylase csad, and taurine transporter tauT (Figures 3B,C).
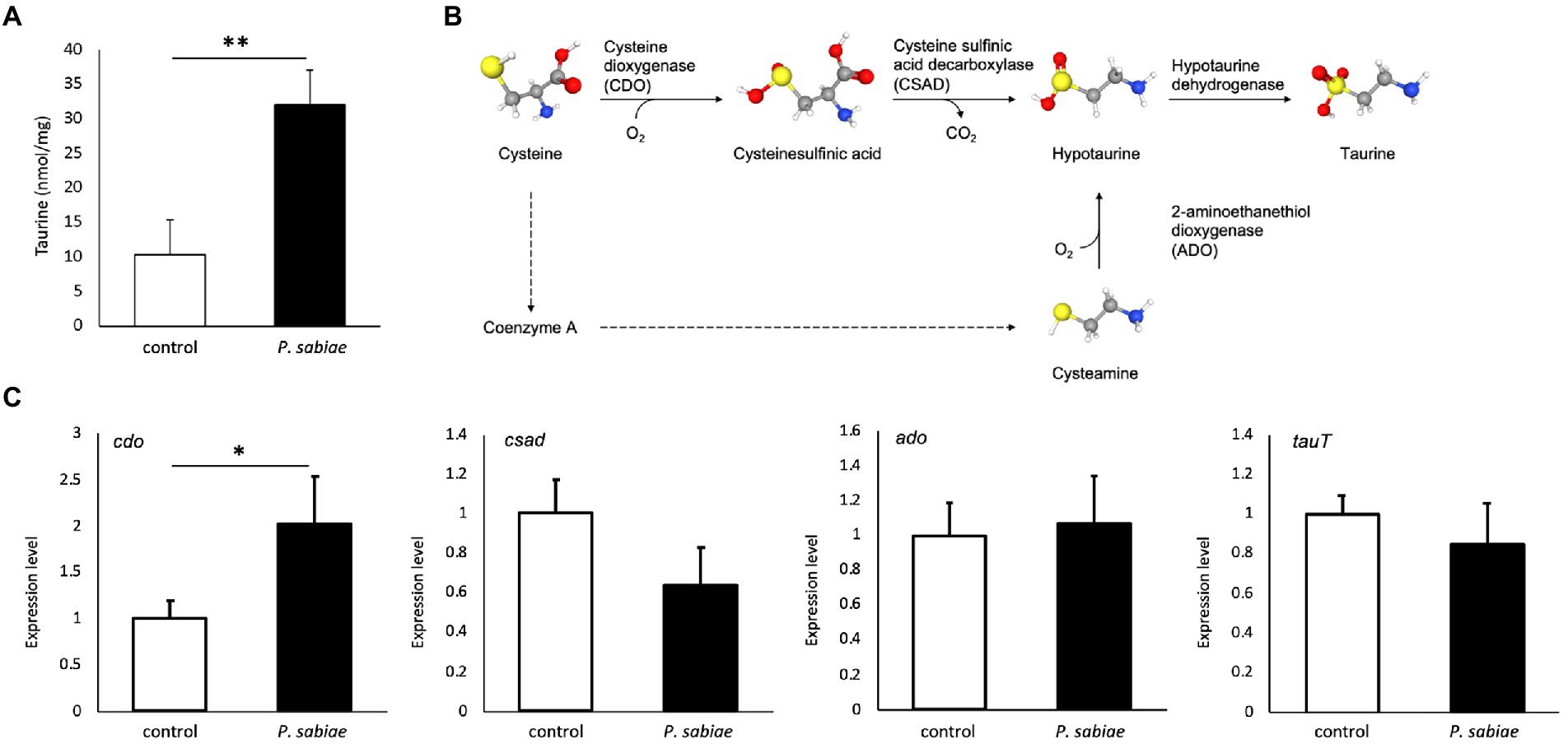
Figure 3. Taurine concentration and expression of taurine synthesis genes in zebrafish brains. (A) Taurine concentrations in zebrafish brains evaluated using the Taurine Assay Kit (Cell Biolabs.). N = 5, **p < 0.01. (B) Taurine biosynthesis pathways, and (C) the expression levels of taurine biosynthesis genes (ado, cdo, csad, tauT) in the zebrafish brain. N = 8, *p < 0.1.
In addition to taurine, serotonin (5-hydroxytryptamine) and GABA are also well-known key players in anxiety in human and zebrafish (Davis et al., 2016; Marcinkiewcz et al., 2016). Several probiotic treatments affect serotonin-related gene expressions in zebrafish (Borrelli et al., 2016; Davis et al., 2016). The expression level of the serotonergic gene tph2 was significantly increased in the P. sabiae group, however that of the other serotonergic genes slc4a6a, slc4a6b, htr1aa, mao, tph1a, and tph1b were not significantly different between these zebrafish brains (Supplementary Figure S5). No significant difference of the serotonin concentrations was observed in these zebrafish brains: (6.0 and 6.2 pmol/mg-brain), according not to be predicted by PICRUSt2. For GABAergic genes, the expression levels of gabra1 and gad1 were not affected by P. sabiae administration (Supplementary Figure S6).
4. Discussion
4.1. The characteristics of Paraburkholderia in gut microbiome
Paraburkholderia is a new genus in gram-negative β-proteobacteria, which can be distinguished from Burkholderia (Sawana et al., 2014). Paraburkholderia is often isolated from the rhizosphere and plant organs, such as root nodules, and it functions in nitrogen fixation and plant growth promotion (Esmaeel et al., 2018). Furthermore, Paraburkholderia has been detected in guts, ranging from those of insects to humans (Takeshita and Kikuchi, 2020; Chen et al., 2022; Tang et al., 2022). The presence of Burkholderia-Caballeronia-Paraburkholderia in human gut microbiomes remarkably correlated with body mass index, osteoarthritis, and cholangiocarcinoma (Zhang et al., 2021; Ning et al., 2022). Opisthorchis viverrini infection is a key risk factor for cholangiocarcinoma and increases Paraburkholderia in the gut microbiomes of hamsters (Haonon et al., 2021). The abundance of Paraburkholderia has also been discussed with regards to ulcerative colitis (Liu et al., 2021) and breast cancer-related fatigue (Lv et al., 2022).
In this study, we focused on the function of Paraburkholderia and demonstrated that zebrafish exposed to the Paraburkholderia type strain, P. sabiae DSM 23623 (Chen et al., 2008), moved more extensively in the novel tank diving test (Figure 1), suggesting the possibility that the P. sabiae-exposed treatment reduced anxiety in zebrafish. This is the first report to describe the function of Paraburkholderia in animal behavior.
4.2. Paraburkholderia sabiae administration affected the diversity of zebrafish gut microbiome
A loss in the diversity of the gut microbiome and dysbiosis caused by diet, infection, stress, etc., leads to abnormalities in brain function and mental health (Rogers et al., 2016). Antibiotic dysbiosis is also associated with an elevated risk of anxiety (Lurie et al., 2015). Borrelli et al. (2016) and Davis et al. (2016) reported that bacterial administration modulates gut microbial communities in zebrafish. Here, we show that P. sabiae administration increased the richness of zebrafish gut microbiomes, as shown by the high values of the diversity index, Chao1 (Figure 2A). In addition to the higher richness, we observed a reduction of Actinobacteria in the gut microbiome in the P. sabiae-exposed zebrafish, which represented reduced anxiety-like behavior (Figures 1, 2), which was similar to the case of L. rhamnosus administration (Borrelli et al., 2016). Paraburkholderia exposure treatment unexpectedly worked as a paraprobiotic (Tsilingiri and Rescigno, 2013), because P. sabiae did not colonize zebrafish guts (Supplementary Table S2).
4.3. The increased taurine concentration in the Paraburkholderia sabiae-exposed zebrafish brain
Metagenomic profiling using PICRUSt revealed functional alterations in the KEGG pathway involved in taurine metabolism (Figure 2B; Supplementary Table S7), and we demonstrated that taurine concentration was significantly (p < 0.05) increased in the P. sabiae-exposed zebrafish brain (Figure 3A). The presence of taurine in the colon depends on the gut microbiome, and it is transported from the colon into the systemic circulation (Matsumoto et al., 2017; Sharon et al., 2019; Li et al., 2020), including the central nervous system, through the blood–brain-barrier via the taurine transporter TauT (Benrabh et al., 1995). In addition, taurine is synthesized in the brain neurons and astrocytes (Vitvitsky et al., 2011). In this study, P. sabiae exposure upregulated the taurine synthesis gene, cdo, in zebrafish brains (Figure 3C), suggesting the existence of indirect mechanisms induced by P. sabiae ingredients, which activate taurine synthesis in the brain.
Taurine, a small organic compound, is a major constituent of bile that exists mainly in the large intestine. It possesses many biological functions, including osmoregulation, membrane stability, intracellular calcium metabolism, anti-oxidation, and anti-inflammation in various tissues (Marcinkiewicz and Kontny, 2014; Menzie et al., 2014). In vertebrates, taurine is thought to have anxiolytic properties in vertebrates (Zhang and Kim, 2007). For example, mouse administered 0.5 mmol/kg taurine for 7 days showed anxiolytic-like properties in the plus-maze test (Chen et al., 2004). The taurine-deficient mice without CSAD exhibited increased anxiety-like behavior in the plus-maze test, which was compensated by the oral treatment with 0.2% taurine (Park et al., 2019). In our results (Figure 3), taurine concentration was significantly (p < 0.05) higher in the P. sabiae-exposed zebrafish brains, suggesting that the increased level of taurine is the cause of reduced anxiety in the P. sabiae-exposed zebrafish. Consistent with our results, Mezzomo et al. reported that treatment with taurine (3.2 mmol/L) decreased anxiety-like behaviors induced by acute exposure to conspecific alarm substances in zebrafish (Mezzomo et al., 2016, 2019). Interestingly, Mezzomo et al. detected anxiolytic effects only in the light and dark test, not in the novel tank test as we did in the present study, in the case of acute taurine administration. In other words, the light and dark test may also provide greater improvement in the P. sabiae group.
Thus, additional research into the molecular mechanisms underlying the increase in the zebrafish gut microbiome diversity, along with the increase in taurine concentration in the zebrafish brain, following P. sabiae administration is required, and future studies will aim to explore the same.
5. Conclusion
We demonstrated that administering P. sabiae to adult zebrafish lowered their anxiety levels and improved the diversity of the gut microbiota in zebrafish. Functional analysis of PICRUSt2 predicted fluctuations in taurine metabolism in the gut microbiome, and our results demonstrated that P. sabiae treatment increased taurine concentration in the brain. These findings indicate that P. sabiae can reduce zebrafish anxiety via the gut-microbiota axis.
Data availability statement
The data presented in the study are deposited in the DDBJ repository, accession number PRJDB15161.
Ethics statement
Ethical review and approval was not required for the animal study because ethical approval from the local Institutional Animal Care and Use Committee was not sought, because Japanese law does not mandate the protection of zebrafish.
Author contributions
SI and YS designed the study, wrote, and revised the manuscript. SI, RA, HF, KH, LZ, HN, and IM contributed to the acquisition, analysis, and interpretation of the data. All authors contributed to the article and approved the submitted version.
Funding
This work was partially supported by the Japan Society for the Promotion of Science KAKENHI (grant numbers JP18K18218 and 21K14953). The funders had no role in the study design, data collection and interpretation, or decision to submit the work for publication.
Conflict of interest
The authors declare that the research was conducted in the absence of any commercial or financial relationships that could be construed as a potential conflict of interest.
Publisher’s note
All claims expressed in this article are solely those of the authors and do not necessarily represent those of their affiliated organizations, or those of the publisher, the editors and the reviewers. Any product that may be evaluated in this article, or claim that may be made by its manufacturer, is not guaranteed or endorsed by the publisher.
Supplementary material
The Supplementary material for this article can be found online at: https://www.frontiersin.org/articles/10.3389/fmicb.2023.1079187/full#supplementary-material
Footnotes
References
Andersson, M. A., Ek, F., and Olsson, R. (2015). Using visual lateralization to model learning and memory in zebrafish larvae. Sci. Rep. 5:8667. doi: 10.1038/srep08667
Anwer, H., Mason, D., Zajitschek, S., Noble, D. W. A., Hesselson, D., Morris, M. J., et al. (2021). An efficient new assay for measuring zebrafish anxiety: tall tanks that better characterize between-individual differences. J. Neurosci. Meth. 356:109138. doi: 10.1016/j.jneumeth.2021.109138
Benrabh, H., Bourre, J. M., and Lefauconnier, J. M. (1995). Taurine transport at the blood-brain barrier: an in vivo brain perfusion study. Brain Res. 692, 57–65. doi: 10.1016/0006-8993(95)00648-A
Bercik, P., Verdu, E. F., Foster, J. A., Macri, J., Potter, M., Huang, X., et al. (2010). Chronic gastrointestinal inflammation induces anxiety-like behavior and alters central nervous system biochemistry in mice. Gastroenterology 139, 2102–2112.e1. doi: 10.1053/j.gastro.2010.06.063
Bjorkholm, C., and Monteggia, L. M. (2016). BDNF – A key transducer of antidepressant effects. Neuropharmacology 102, 72–79. doi: 10.1016/j.neuropharm.2015.10.034
Borrelli, L., Aceto, S., Agnisola, C., De Paolo, S., Dipineto, L., Stilling, R. M., et al. (2016). Probiotic modulation of the microbiota-gut-brain axis and behaviour in zebrafish. Sci. Rep. 6:30046. doi: 10.1038/srep30046
Bravo, J. A., Forsythe, P., Chew, M. V., Escaravage, E., Savignac, H. M., Dinan, T. G., et al. (2011). Ingestion of lactobacillus strain regulates emotional behavior and central GABA receptor expression in a mouse via the vagus nerve. Proc. Natl. Acad. Sci. U. S. A. 108, 16050–16055. doi: 10.1073/pnas.1102999108
Chen, Z., Chen, J. Q., Liu, Y., Zhang, J., Chen, X. H., and Qu, Y. F. (2022). Comparative study on gut microbiota in three Anura frogs from a mountain stream. Ecol. Evol. 12:e8854. doi: 10.1002/ece3.8854
Chen, W. M., de Faria, S. M., Chou, J. H., James, E. K., Elliott, G. N., Sprent, J. I., et al. (2008). Burkholderia sabiae sp. nov., isolated from root nodules of Mimosa caesalpiniifolia. Int. J. Syst. Evol. Microbiol. 58, 2174–2179. doi: 10.1099/ijs.0.65816-0
Chen, S. W., Kong, W. X., Zhang, Y. J., Li, Y. L., Mi, X. J., and Mu, X. S. (2004). Possible anxiolytic effects of taurine in the mouse elevated plus-maze. Life Sci. 75, 1503–1511. doi: 10.1016/j.lfs.2004.03.010
Colwill, R. M., and Creton, R. (2011). Imaging escape and avoidance behavior in zebrafish larvae. Rev. Neurosci. 22, 63–73. doi: 10.1515/rns.2011.008
Davis, D. J., Doerr, H. M., Grzelak, A. K., Busi, S. B., Jasarevic, E., Ericsson, A. C., et al. (2016). Lactobacillus plantarum attenuates anxiety-related behavior and protects against stress-induced dysbiosis in adult zebrafish. Sci. Rep. 6:33726. doi: 10.1038/srep33726
Desbonnet, L., Clarke, G., Traplin, A., O'Sullivan, O., Crispie, F., Moloney, R. D., et al. (2015). Gut microbiota depletion from early adolescence in mice: implications for brain and behaviour. Brain Behav. Immun. 48, 165–173. doi: 10.1016/j.bbi.2015.04.004
Diaz Heijtz, R., Wang, S., Anuar, F., Qian, Y., Bjorkholm, B., Samuelsson, A., et al. (2011). Normal gut microbiota modulates brain development and behavior. Proc. Natl. Acad. Sci. U. S. A. 108, 3047–3052. doi: 10.1073/pnas.1010529108
Douglas, G. M., Maffei, V. J., Zaneveld, J. R., Yurgel, S. N., Brown, J. R., Taylor, C. M., et al. (2020). PICRUSt2 for prediction of metagenome functions. Nat. Biotechnol. 38, 685–688. doi: 10.1038/s41587-020-0548-6
Engeszer, R. E., Barbiano, L. A., Ryan, M. J., and Parichy, D. M. (2007). Timing and plasticity of shoaling behaviour in the zebrafish, Danio rerio. Anim Behav. 74, 1269–1275. doi: 10.1016/j.anbehav.2007.01.032
Esmaeel, Q., Miotto, L., Rondeau, M., Leclere, V., Clement, C., Jacquard, C., et al. (2018). Paraburkholderia phytofirmans PsJN-plants interaction: from perception to the induced mechanisms. Front. Microbiol. 9:2093. doi: 10.3389/fmicb.2018.02093
Gacias, M., Gaspari, S., Santos, P. M., Tamburini, S., Andrade, M., Zhang, F., et al. (2016). Microbiota-driven transcriptional changes in prefrontal cortex override genetic differences in social behavior. Elife 5:e13442. doi: 10.7554/eLife.13442
Giacomini, N. J., Rose, B., Kobayashi, K., and Guo, S. (2006). Antipsychotics produce locomotor impairment in larval zebrafish. Neurotoxicol. Teratol. 28, 245–250. doi: 10.1016/j.ntt.2006.01.013
Godwin, J., and Thompson, R. (2012). Nonapeptides and social behavior in fishes. Horm. Behav. 61, 230–238. doi: 10.1016/j.yhbeh.2011.12.016
Haghani, S., Karia, M., Cheng, R. K., and Mathuru, A. S. (2019). An automated assay system to study novel tank induced anxiety. Front. Behav. Neurosci. 13:180. doi: 10.3389/fnbeh.2019.00180
Haonon, O., Liu, Z., Dangtakot, R., Intuyod, K., Pinlaor, P., Puapairoj, A., et al. (2021). Opisthorchis viverrini infection induces metabolic and fecal microbial disturbances in association with liver and kidney pathologies in hamsters. J. Proteome Res. 20, 3940–3951. doi: 10.1021/acs.jproteome.1c00246
Hoban, A. E., Stilling, R. M., Ryan, F. J., Shanahan, F., Dinan, T. G., Claesson, M. J., et al. (2016). Regulation of prefrontal cortex myelination by the microbiota. Transl. Psychiatry 6:e774. doi: 10.1038/tp.2016.42
Huang, F., and Wu, X. (2021). Brain neurotransmitter modulation by gut microbiota in anxiety and depression. Front. Cell Dev. Biol. 9:649103. doi: 10.3389/fcell.2021.649103
Kanehisa, M., Sato, Y., and Kawashima, M. (2022). KEGG mapping tools for uncovering hidden features in biological data. Protein Sci. 31, 47–53. doi: 10.1002/pro.4172
Li, Q., Lin, J., Zhang, Y., Liu, X., Chen, X. Q., Xu, M. Q., et al. (2015). Differential behavioral responses of zebrafish larvae to yohimbine treatment. Psychopharmacology 232, 197–208. doi: 10.1007/s00213-014-3656-5
Li, H., Xu, H., Li, Y., Jiang, Y., Hu, Y., Liu, T., et al. (2020). Alterations of gut microbiota contribute to the progression of unruptured intracranial aneurysms. Nat. Commun. 11:3218. doi: 10.1038/s41467-020-16990-3
Liu, H., Liu, W., Huang, X., Feng, Y., Lu, J., and Gao, F. (2021). Intestinal flora differences between patients with ulcerative colitis of different ethnic groups in China. Medicine 100:e26932. doi: 10.1097/MD.0000000000026932
Lurie, I., Yang, Y. X., Haynes, K., Mamtani, R., and Boursi, B. (2015). Antibiotic exposure and the risk for depression, anxiety, or psychosis: a nested case-control study. J. Clin. Psychiatry 76, 1522–1528. doi: 10.4088/JCP.15m09961
Lv, Z., Liu, R., Su, K., Gu, Y., Fang, L., Fan, Y., et al. (2022). Acupuncture ameliorates breast cancer-related fatigue by regulating the gut microbiota-gut-brain axis. Front. Endocrinol. 13:921119. doi: 10.3389/fendo.2022.921119
Marcinkiewcz, C. A., Mazzone, C. M., D'Agostino, G., Halladay, L. R., Hardaway, J. A., DiBerto, J. F., et al. (2016). Serotonin engages an anxiety and fear-promoting circuit in the extended amygdala. Nature 537, 97–101. doi: 10.1038/nature19318
Marcinkiewicz, J., and Kontny, E. (2014). Taurine and inflammatory diseases. Amino Acids 46, 7–20. doi: 10.1007/s00726-012-1361-4
Matsumoto, M., Ooga, T., Kibe, R., Aiba, Y., Koga, Y., and Benno, Y. (2017). Colonic absorption of low-molecular-weight metabolites influenced by the intestinal microbiome: a pilot study. PLoS One 12:e0169207. doi: 10.1371/journal.pone.0169207
Menzie, J., Pan, C., Prentice, H., and Wu, J. Y. (2014). Taurine and central nervous system disorders. Amino Acids 46, 31–46. doi: 10.1007/s00726-012-1382-z
Messaoudi, M., Lalonde, R., Violle, N., Javelot, H., Desor, D., Nejdi, A., et al. (2011). Assessment of psychotropic-like properties of a probiotic formulation (Lactobacillus helveticus R0052 and Bifidobacterium longum R0175) in rats and human subjects. Br. J. Nutr. 105, 755–764. doi: 10.1017/S0007114510004319
Mezzomo, N. J., Fontana, B. D., Muller, T. E., Duarte, T., Quadros, V. A., Canzian, J., et al. (2019). Taurine modulates the stress response in zebrafish. Horm. Behav. 109, 44–52. doi: 10.1016/j.yhbeh.2019.02.006
Mezzomo, N. J., Silveira, A., Giuliani, G. S., Quadros, V. A., and Rosemberg, D. B. (2016). The role of taurine on anxiety-like behaviors in zebrafish: a comparative study using the novel tank and the light-dark tasks. Neurosci. Lett. 613, 19–24. doi: 10.1016/j.neulet.2015.12.037
Needham, B. D., Funabashi, M., Adame, M. D., Wang, Z., Boktor, J. C., Haney, J., et al. (2022). A gut-derived metabolite alters brain activity and anxiety behaviour in mice. Nature 602, 647–653. doi: 10.1038/s41586-022-04396-8
Needham, B. D., Kaddurah-Daouk, R., and Mazmanian, S. K. (2020). Gut microbial molecules in behavioural and neurodegenerative conditions. Nat. Rev. Neurosci. 21, 717–731. doi: 10.1038/s41583-020-00381-0
Ning, Y. J., Hu, M. H., Gong, Y., Huang, R. T., Xu, K., Chen, S. J., et al. (2022). Comparative analysis of the gut microbiota composition between knee osteoarthritis and Kashin-Beck disease in Northwest China. Arthritis Res Ther. May 24:24. doi: 10.1186/s13075-022-02819-5
Park, E., Elidrissi, A., Schuller-Levis, G., and Chadman, K. K. (2019). Taurine partially improves abnormal anxiety in taurine-deficient mice. Adv. Exp. Med. Biol. 1155, 905–921. doi: 10.1007/978-981-13-8023-5_76
Phelps, D., Brinkman, N. E., Keely, S. P., Anneken, E. M., Catron, T. R., Betancourt, D., et al. (2017). Microbial colonization is required for normal neurobehavioral development in zebrafish. Sci. Rep. 7:7. doi: 10.1038/s41598-017-10517-5
Rana, T., Behl, T., Sehgal, A., Singh, S., Sharma, N., Abdeen, A., et al. (2022). Exploring the role of neuropeptides in depression and anxiety. Prog. Neuropsychopharmacol. Biol. Psychiatry 114:110478. doi: 10.1016/j.pnpbp.2021.110478
Rico, E. P., Rosemberg, D. B., Seibt, K., Capiotti, K. M., Da Silva, R. S., and Bonan, C. D. (2011). Zebrafish neurotransmitter systems as potential pharmacological and toxicological targets. Neurotoxicol. Teratol. 33, 608–617. doi: 10.1016/j.ntt.2011.07.007
Rodriguez, A., Zhang, H. Q., Klaminder, J., Brodin, T., Andersson, P. L., and Andersson, M. (2018). ToxTrac: a fast and robust software for tracking organisms. Methods Ecol. Evol. 9, 460–464. doi: 10.1111/2041-210X.12874
Rogers, G. B., Keating, D. J., Young, R. L., Wong, M. L., Licinio, J., and Wesselingh, S. (2016). From gut dysbiosis to altered brain function and mental illness: mechanisms and pathways. Mol. Psych. 21, 738–748. doi: 10.1038/mp.2016.50
Sawana, A., Adeolu, M., and Gupta, R. S. (2014). Molecular signatures and phylogenomic analysis of the genus Burkholderia: proposal for division of this genus into the emended genus Burkholderia containing pathogenic organisms and a new genus Paraburkholderia gen. nov. harboring environmental species. Front. Genet. 5:429. doi: 10.3389/fgene.2014.00429
Segata, N., Izard, J., Waldron, L., Gevers, D., Miropolsky, L., Garrett, W. S., et al. (2011). Metagenomic biomarker discovery and explanation. Genome Biol. 12:R60. doi: 10.1186/gb-2011-12-6-r60
Sharon, G., Cruz, N. J., Kang, D. W., Gandal, M. J., Wang, B., Kim, Y. M., et al. (2019). Human gut microbiota from autism spectrum disorder promote behavioral symptoms in mice. Cell 177:e1617, 1600–1618.e17. doi: 10.1016/j.cell.2019.05.004
Shiozaki, K., Kawabe, M., Karasuyama, K., Kurachi, T., Hayashi, A., Ataka, K., et al. (2020). Neuropeptide Y deficiency induces anxiety-like behaviours in zebrafish (Danio rerio). Sci. Rep. 10:5913. doi: 10.1038/s41598-020-62699-0
Stagaman, K., Sharpton, T. J., and Guillemin, K. (2020). Zebrafish microbiome studies make waves. Lab Anim. 49, 201–207. doi: 10.1038/s41684-020-0573-6
Swann, J. R., Garcia-Perez, I., Braniste, V., Wilson, I. D., Sidaway, J. E., Nicholson, J. K., et al. (2017). Application of (1)H NMR spectroscopy to the metabolic phenotyping of rodent brain extracts: a metabonomic study of gut microbial influence on host brain metabolism. J. Pharm. Biomed. Anal. 143, 141–146. doi: 10.1016/j.jpba.2017.05.040
Takeshita, K., and Kikuchi, Y. (2020). Genomic comparison of insect gut symbionts from divergent Burkholderia subclades. Genes (Basel) 11:744. doi: 10.3390/genes11070744
Tang, S., Li, Y., Huang, C., Yan, S., Li, Y., Chen, Z., et al. (2022). Comparison of gut microbiota diversity between captive and wild tokay gecko (Gekko gecko). Front. Microbiol. 13:897923. doi: 10.3389/fmicb.2022.897923
Tillisch, K., Labus, J., Kilpatrick, L., Jiang, Z., Stains, J., Ebrat, B., et al. (2013). Consumption of fermented milk product with probiotic modulates brain activity. Gastroenterology 144, 1394–1401. 1401.e1-14. doi: 10.1053/j.gastro.2013.02.043
Tsilingiri, K., and Rescigno, M. (2013). Postbiotics: what else? Benef. Microbes 4, 101–107. doi: 10.3920/BM2012.0046
Vitvitsky, V., Garg, S. K., and Banerjee, R. (2011). Taurine biosynthesis by neurons and astrocytes. J. Biol. Chem. 286, 32002–32010. doi: 10.1074/jbc.M111.253344
Vuong, H. E., Yano, J. M., Fung, T. C., and Hsiao, E. Y. (2017). The microbiome and host behavior. Annu. Rev. Neurosci. 40, 21–49. doi: 10.1146/annurev-neuro-072116-031347
Wong, R. Y., Oxendine, S. E., and Godwin, J. (2013). Behavioral and neurogenomic transcriptome changes in wild-derived zebrafish with fluoxetine treatment. BMC Genomics 14:348. doi: 10.1186/1471-2164-14-348
Zhang, C. G., and Kim, S. J. (2007). Taurine induces anti-anxiety by activating strychnine-sensitive glycine receptor in vivo. Ann. Nutr. Metab. 51, 379–386. doi: 10.1159/000107687
Keywords: Danio rerio, Burkholderiaceae, novel tank diving, stress, mental diseases
Citation: Ichikawa S, Abe R, Fujimoto H, Higashi K, Zang L, Nakayama H, Matsuoka I and Shimada Y (2023) Paraburkholderia sabiae administration alters zebrafish anxiety-like behavior via gut microbial taurine metabolism. Front. Microbiol. 14:1079187. doi: 10.3389/fmicb.2023.1079187
Edited by:
Reeta Goel, GLA University, IndiaReviewed by:
Kiran Veer Sandhu, University College Cork, IrelandAurelijus Burokas, Vilnius University, Lithuania
Copyright © 2023 Ichikawa, Abe, Fujimoto, Higashi, Zang, Nakayama, Matsuoka and Shimada. This is an open-access article distributed under the terms of the Creative Commons Attribution License (CC BY). The use, distribution or reproduction in other forums is permitted, provided the original author(s) and the copyright owner(s) are credited and that the original publication in this journal is cited, in accordance with accepted academic practice. No use, distribution or reproduction is permitted which does not comply with these terms.
*Correspondence: Yasuhito Shimada, ✉ c2hpbWFkYS55YXN1aGl0b0BtaWUtdS5hYy5qcA==