- 1Program in Pathobiology, the Fifth Affiliated Hospital, Zhongshan School of Medicine, Sun Yat-sen University, Guangdong, China
- 2Advanced Medical Technology Center, The First Affiliated Hospital, Zhongshan School of Medicine, Sun Yat-sen University, Guangzhou, China
- 3Key Laboratory of Tropical Diseases Control, Ministry of Education, Sun Yat-sen University, Guangzhou, China
- 4Microbiome Medicine Center, Department of Laboratory Medicine, Zhujiang Hospital, Southern Medical University, Guangzhou, China
- 5School of Basic Medical Sciences, Xinxiang Medical University, Xinxiang, China
- 6School of Public Health, Shandong University, Jinan, China
- 7School of Laboratory Medicine, Chengdu Medical College, Chengdu, China
- 8Department of Neonatal Surgery, Guangzhou Women and Children's Medical Center, Guangzhou, China
- 9Faculty of Forensic Medicine, Zhongshan School of Medicine, Sun Yat-sen University, Guangzhou, China
- 10Guangdong Province Translational Forensic Medicine Engineering Technology Research Center, Sun Yat-sen University, Guangzhou, China
Objectives: Tigecycline is recognized as one of the last-line antibiotics to treat serious bacterial infection caused by carbapenem-resistant Klebsiella pneumoniae (CRKP). The plasmid-borne gene tet(X4) mediates high resistance to tigecycline. However, the prevalence and genetic context of tet(X4) in K. pneumoniae from various sources are not fully understood. Here, we investigated the prevalence of tet(X4)-positive K. pneumoniae and characterized the genetic context of tet(X4)-bearing plasmids in K. pneumoniae isolates.
Methods: Polymerase chain reaction (PCR) was used to detect the tet(X4) gene. The transferability of the tet(X4)-carrying plasmids was tested by conjugation assays. The Galleria mellonella infection model was used to test virulence of tet(X4)-positive strains. Whole-genome sequencing and genome-wide analysis were performed to identify the antimicrobial resistance and the virulence genes, and to clarify the genetic characteristics of the tet(X4)-positive isolates.
Results: Among 921 samples, we identified two tet(X4)-positive K. pneumoniae strains collected from nasal swabs of two pigs (0.22%, 2/921). The two tet(X4)-positive isolates exhibited high minimum inhibitory concentrations to tigecycline (32–256 mg/L) and tetracycline (256 mg/L). The plasmids carrying the tet(X4) gene can transfer from the donor strain K. pneumoniae to the recipient strain Escherichia coli J53. Genetic analysis of the complete sequence of two tet(X4)-carrying plasmids pTKPN_3-186k-tetX4 and pTKPN_8-216k-tetX4 disclosed that the tet(X4) gene was flanked by delta ISCR2 and IS1R, which may mediate the transmission of the tet(X4) gene.
Conclusion: The prevalence of tet(X4)-positive K. pneumoniae among different sources was low. ISCR2 and IS1R may contribute to the horizontal transfer of tet(X4) gene. Effective measures should be taken to prevent the transmission of tet(X4)-producing K. pneumoniae in humans or animals.
Introduction
Klebsiella pneumoniae is a common cause of antimicrobial-resistant opportunistic infections in hospitalized patients (Wyres et al., 2020). In clinical, K. pneumoniae can cause a variety of infection diseases, including bacteremia, urinary tract infections, pneumonia, and liver abscesses (Hansen et al., 1998; Siu et al., 2012). In recent years, carbapenem resistant K. pneumoniae (CRKP) infection rates have increased alarmingly (Low et al., 2018). Tigecycline has been regarded as one of the “last resort” antimicrobials to fight against CRKP (Seifert et al., 2018).
Tigecycline is the first generation of glycylcycline, which has been used since 2005. It is one of the last choice of treatment for serious infection, especially those caused by extensively drug-resistant Enterobacteriaceae (Sun et al., 2019). Shortly after the first usage, a multi-drug resistant (MDR) K. pneumoniae strain (reduced tigecycline sensitivity, MIC = 4 μg/ml) was isolated in a hospital, significantly compromising the efficacy of tigecycline (Ruzin et al., 2005). To date, there are several known mechanisms associated with tigecycline resistance in K. pneumoniae, including the enhanced expression of resistance–nodulation–cell division (RND)-type efflux pumps such as AcrAB-TolC and OqxAB, mutations in the ribosomal S10 protein (encoded by rpsJ and lon genes; Ruzin et al., 2005; Villa et al., 2014; He et al., 2015; Fang et al., 2016), acquisition of plasmid-mediated tmexCD1-toprJ1 efflux pump (Lv et al., 2020), mutation of tet(A) gene (Du et al., 2018).
In recently, four plasmid-borne tet(X) variants mediating tigecycline resistance [tet(X3), tet(X4), tet(X5), and tet(X6)] have been detected in various species of bacteria obtained from animals, animal-derived foods, humans and environment samples in China (He et al., 2019; Chen et al., 2020; Dong et al., 2022). Among these tet(X) variants, tet(X4) gene is widely identified in E. coli isolates from food animals (Li Y. et al., 2021; Yu et al., 2021; Wang et al., 2022). To date, only a few studies have reported tet(X4)-positive K. pneumoniae isolated from pork and clinical sources (Li et al., 2022; Liu et al., 2022). Overall, the epidemiology and characteristics of tet(X)s-positive K. pneumoniae isolates are not fully understood. Here, we investigated the prevalence of tet(X) genes in K. pneumoniae isolates and further described the tet(X4)-harboring plasmids in K. pneumoniae isolates.
Materials and methods
Sample collection and identification of tet(X)s-positive Klebsiella pneumoniae strains
To determine the prevalence of tet(X)s-positive K. pneumoniae strains in animal-associated and clinical samples, 921 samples from pigs and humans in China were collected (Supplementary Table S1). Specifically, a total of 590 samples from a pig farm (56 swine nasal swabs; Guangdong province, China) and a swine slaughter house (419 swine nasal swabs, 67 swine anal swabs, and 48 skin swabs of workers; Guangdong province, China) were collected from October to November 2020. Besides, 184 human anal swab specimens including 171 hospitalized patients and 13 healthy individuals from hospital A collected in 2019 were also included. All samples were subjected to selection on brain heart infusion (BHI) plates containing tigecycline (2 mg/L). After incubation at 37°C for 16–18 h, the tigecycline-resistant colonies were selected. Then detection of the tet(X) genes was carried out by PCR using specific primers in Supplementary Table S2. Species identification was achieved by Matrix-assisted laser desorption/ionization time-of-flight mass spectrometry (MALDI-TOF MS). In addition, 147 clinical nonduplicate K. pneumoniae isolates were collected from bloodstream samples in four hospitals (named as B, C, D, and E) located in Guangdong province in China during the period 2008–2018, and all strains were tested for the presence of tet(X) genes.
Antimicrobial susceptibility testing
The susceptibility to tetracycline (TET), chloramphenicol (CHL), ciprofloxacin (CIP), ceftazidime (CAZ), gentamicin (GEN), amikacin (AMK), cefotaxime (CTX), trimethoprim-sulfamethoxazole (SXT), imipenem (IMP), ampicillin (AMP), fosfomycin (FOS), and rifampin (RIF) was determined by the agar dilution method according to the Clinical and Laboratory Standards Institute (CLSI) guidelines (CLSI, 2019). MICs of MICs of tigecycline (TGC) and colistin (CT) were determined by the broth dilution method according to the guidelines of EUCAST (2021). The E. coil ATCC25922 served as quality control.
Plasmid conjugation
Conjugation experiments were performed to test the transferability of the tet(X4)-harboring plasmids, using a sodium azide resistant E. coli J53 as the recipient. Briefly, a culture of tet(X4)-producing isolates and the recipient strain E. coli J53 were mixed (ratio of 1:9) in BHI broth and subjected to incubation for 6–8 h. The mixture was then spread on BHI agar plates containing 100 mg/L sodium azide and 1 mg/L tigecycline to select transconjugants that had acquired the tet(X4)-harboring plasmid. Colonies that grew on selective plates after incubation for 16–24 h at 37°C were further confirmed by PCR and Sanger sequencing.
Galleria mellonella infection model
The Galleria mellonella infection model was used to test virulence and pathogenesis of tet(X4)-positive K. pneumoniae strains, which was carried out as described previously with slight modifications (Buckner et al., 2018). Overnight cultures of K. pneumoniae strains were washed with phosphate-buffered saline (PBS) and further adjusted with PBS to concentrations of 1 × 106 CFU/ml. The larvae were injected with 10 uL of bacterial solution and negative control groups were inoculated with 10 uL of PBS, the hvKP4 K. pneumoniae strain was used as the hypervirulent control (Gu et al., 2018). Then the larvae were incubated at 37°C in the dark and the survival rates of the larvae were recorded. Kaplan–Meier survival curves were plotted using GraphPad Prism, and the log rank (Mantel-Cox) test was used to analyze the significant difference (p < 0.05) of the survival rates in G. mellonella infection model.
Genome sequencing and bioinformatics analysis
Genomic DNA of tet(X)s-positive K. pneumoniae isolates was extracted using the Qiagen Blood & Tissue kit (Qiagen, Hilden, Germany). DNA libraries were constructed with 350-bp paired-end fragments and sequenced using an Illumina HiSeq 2000 platform. In addition, the PacBio Sequel System was performed to long-read sequencing the TKPN_8 strain. The sequencing reads were assembled into contigs using SPAdes version 3.10 (Bankevich et al., 2012). Genome sequences were annotated using Prokka (version 1.13.3; Seemann, 2014). Multilocus sequence typing (MLST) of isolates was conducted using MLST v2.11 based on assembled contigs (Raisanen et al., 2020). The core genes of bacterial genomes were extracted and aligned using Roary (Page et al., 2015). RAxML v8.2.10 was used to construct a maximum likelihood phylogeny of the strains (Stamatakis, 2006), then the phylogenetic tree was visualized by iTOL (Letunic and Bork, 2016). The SNP (sequence-based) and core-genome-based MLST (cgMLST) strategies on BacWGSTdb 2.0 were used for source tracking bacterial pathogens, and the phylogenetic tree was generated and visualized by Grapetree (Feng et al., 2021). Capsule (K) loci was identified using Kaptive (Wick et al., 2018). To obtain the complete sequence of tet(X)s-carrying plasmid, we combined the sequencing data from the genomic DNA and the plasmids, and predicted gaps were closed by PCR and Sanger sequencing using primers listed in Supplementary Table S3. Plasmid replicons, insertion sequences, antimicrobial resistance determinants and virulence factors were determined using online tools.1 Easyfig was used to visualize the genetic comparisons.
Results
Identification of Klebsiella pneumoniae isolates harboring tet(X4)
In this study, the prevalence of tet(X)s-positive K. pneumoniae was 0.22% (2/921). The two tet(X4)-carrying strains, designated as TKPN_3 and TKPN_8, were collected from different swine nasal swabs (one was isolated from the pig farm; the other was isolated from a swine slaughter house). No other tet(X) variants were detected in K. pneumoniae strains.
The MLST analysis declared that K. pneumoniae TKPN_3 and TKPN_8 belong to ST35 and ST193, respectively (Table 1). To conduct the phylogenetic analysis of TKPN_3 and TKPN_8, the whole-genome sequencing data of 45 K. pneumoniae strains were download from NCBI database, including 40 tet(X4)-negative strains of ST35 and ST193, and five tet(X4)-positive strains belonging to ST727, ST43, ST1418, ST35, and ST327. A phylogenetic tree for 47 K. pneumoniae strains based on SNPs of core genomes was constructed. It showed that the K. pneumoniae strains were clustered into three distinct clusters, and tet(X4)-positive strains were dispersed on different branches, all of which were isolated from China between 2019 and 2021 (Figure 1). For source tracking bacterial pathogens, the genome sequences of TKPN_3 and TKPN_8 were submitted to BacWGSTdb and none close strains were found based on SNP (sequence-based) strategy. The core-genome-based MLST (cgMLST) analysis showed that 86 and 8 strains were, respectively, closed to TKPN_3 and TKPN_8, but had more than 340 different alleles (Supplementary Figure S1). In order to explore the evolution and transmission of the tet(X)s-positive K. pneumoniae, more sequencing data may be needed for phylogenetic analysis.
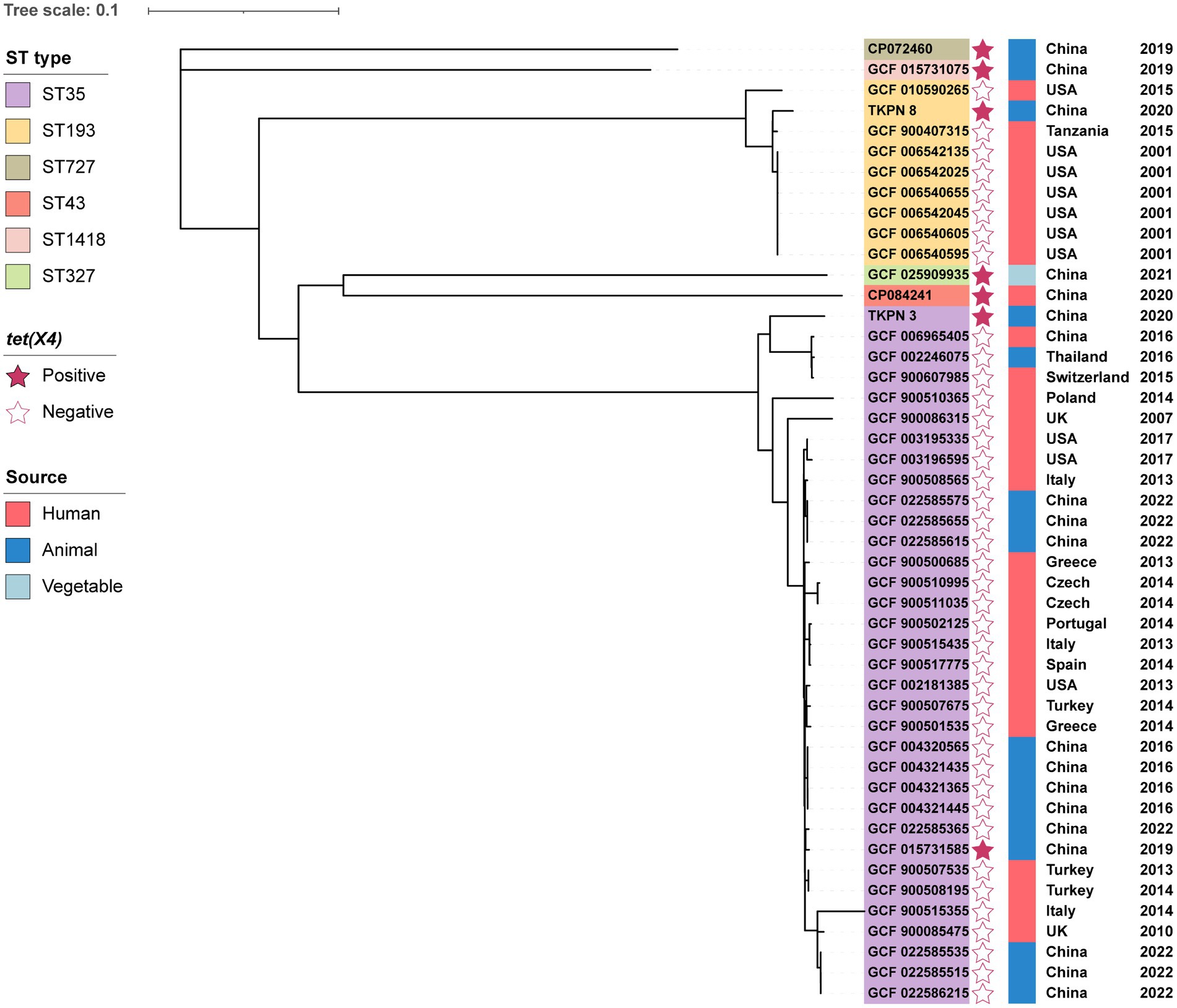
Figure 1. Phylogenetic analysis of 47 K. pneumoniae from different sources and their basic characterization. The phylogenetic tree was constructed by RAxML based on SNPs of core genomes.
The antimicrobial resistance phenotype and genotype of tet(X4)-positive Klebsiella pneumoniae
Klebsiella pneumoniae TKPN_3 and TKPN_8 were resistant to tigecycline, tetracycline, rifampin, chloramphenicol and ampicillin, but were susceptible to gentamicin, imipenem, amikacin, fosfomycin and colistin. TKPN_3 and TKPN_8 showed resistance to tigecycline with MIC of 256 and 32 mg/L, respectively. In addition, TKPN_3 was also resistant to ciprofloxacin, ceftazidime, cefotaxime and trimethoprim-sulfamethoxazole, while TKPN_8 was susceptible to them (Table 1).
Except for the tet(X4) gene, WGS data revealed that TKPN_3 harbored other 18 antibiotic-resistant genes (ARGs), including three quinolone resistance genes (oqxB, oqxA, qnrS1), two β-lactams resistance genes (blaCTX-M-3, blaSHV-33), one sulphonamide resistance gene (sul2), one trimethoprim resistance gene (dfrA27), one rifampicin resistance gene (arr-3), one fosfomycin resistance gene (fosA6), one macrolide resistance gene [mph(A)], one tetracycline resistance gene [tet(A)], five aminoglycoside resistance genes [aac(6′)-Ib-cr, aadA16, aph(3″)-Ib, aph(6)-Id, ant(3″)-Ia], one chloramphenicol resistance gene (floR) and one lincomycin resistance gene [lnu(G)]. In the isolate TKPN_8, multiple resistance genes were also identified, including tet(X4) along with other 11 ARGs including three quinolone resistance gene (oqxB, oqxA, qnrS1), one β-lactam resistance gene (blaSHV-61), one sulphonamide resistance gene (sul2), one fosfomycin resistance gene (fosA6), one tetracycline resistance gene [tet(A)], two aminoglycoside resistance genes [aph(3″)-Ib, aph(6)-Id], chloramphenicol resistance gene (floR) and one lincomycin resistance gene [lnu(G); Table 1]. In general, these two tet(X4)-positive K. pneumoniae strains have similar antimicrobial resistance phenotype and genotype. Moreover, TKPN_3 showed higher level of tigecycline resistance compared with TKPN_8, and correspondingly, mutations involving regulators of multidrug efflux pump were also found in strain TKPN_3. Amino acid mutations were identified in RamR at T141I and in Lon at A299T.
The virulence phenotype and genotype in Klebsiella pneumoniae TKPN_3 and TKPN_8
In addition to resistance genes, a total of 78 different virulence factors were identified among these two tet(X4) positive isolates. These genes include various siderophores such as enterobactin (encoded by entABCEF, fepABCDG, fes, and ybdA genes), aerobactin (encoded by iucABCD and iutA genes) and salmochelin (encoded by iroE gene). Moreover, the gene clusters tssABCD and tssFGHIJKLM encoding type VI secretion system, which was proved to be beneficial to bacterial competition, cell invasion, type-1 fimbriae expression and in vivo colonization in K. pneumoniae (Hsieh et al., 2019), were detected in TKPN_3 and TKPN_8. In addition, the capsular serotypes of the TKPN_3 and TKPN_8 strains were identified as KL71 and KL63, respectively. Then the virulence of two strains was demonstrated by Galleria mellonella infection model. After 12 h post-infection, the survival rate of G. mellonella larvaes injected with TKPN_3 and TKPN_8 was 50%. Overall, no difference in survival was observed for G. mellonella infected by TKPN_3 and TKPN_8 compared with HvKP4 (TKPN_3 vs. HvKP4, p = 0.606; TKPN_8 vs. HvKP4, p = 0.326; Supplementary Figure S2).
The transferability of tet(X4)-harboring plasmid from Klebsiella pneumoniae
To further determine the transferability of tet(X4) gene, conjugation assays were conducted. It showed that the tet(X4)-carrying plasmids form TKPN_3 and TKPN_8 could be successfully transferred from K. pneumoniae to E. coli J53. The two tet(X4)-harboring plasmids were denoted as pTKPN_3-186k-tetX4 and pTKPN_8-216k-tetX4. The conjugation frequencies of pTKPN_3-186k-tetX4 and pTKPN_8-216k-tetX4 were (1.0 ± 0.1) × 10−4 and (5.4 ± 0.7) × 10−5 cells per recipient, respectively. Compared with the recipient strain E. coli J53, the MICs of the transconjugants J53/pTKPN_3-186k-tetX4 and J53/pTKPN_8-216k-tetX4 for tigecycline and tetracycline increased by 128-fold, respectively.
Genetic characteristics of tet(X4) in pTKPN_3-186k-tetX4 and pTKPN_8-216k-tetX4
The genetic features of tet(X4)-carrying plasmid pTKPN_3-186k-tetX4 of TKPN_3 was analyzed in depth. To obtain the complete sequence of this plasmid, the sequencing data of the genomic DNA and the plasmids DNA were combined, and predicted gaps were closed by PCR and Sanger sequencing. pTKPN_3-186k-tetX4 was a 186,211 bp plasmid and encoded 218 predicted open reading frames (ORFs). The backbone of the tet(X4)-carrying plasmid pTKPN_3-186k-tetX4 showed a typical mosaic structure with multiple replicon types including IncHI1B(R27), IncHI1A and IncFIA (HI1). The genes involved in conjugation were identified including traC, traD, traG, traI, traJ, traU, and mobI. The tet(X4) gene and another five antimicrobial resistance genes blaTEM-1B, ant(3″)-Ia, qnrS1, floR, and lnu(G) were found in pTKPN_3-186k-tetX4. The mobile element IS1R (IS1 family, 768 bp) was located upstream of tet(X4) and delta ISCR2 (IS91 family, 977 bp) was found in downstream of tet(X4), which may contribute to the transmission of the tet(X4) gene (Figure 2). Additionally, two complete insertion sequence IS26 and two truncated transposons Tn2 were identified downstream of tet(X4) in pTKPN_3-186k-tetX4. The pTKPN_3-186k-tetX4 shared a similar plasmid backbone (99% coverage and 100% identity) against p1919D62-1 (GenBank accession number: CP046007.1), which isolated from a swine E.coli strain 1919D62 in China. The other two plasmids with high similarity with our plasmid were pSZ6R-tetX4 (GenBank accession number: MW940627.1) and pAB4-4-tetX4 (GenBank accession number: MW940615.1). The two plasmids pSZ6R-tetX4 and pAB4-4-tetX4 were isolated from Citrobacter sp. and Klebsiella sp. strains, respectively, (Figure 2).
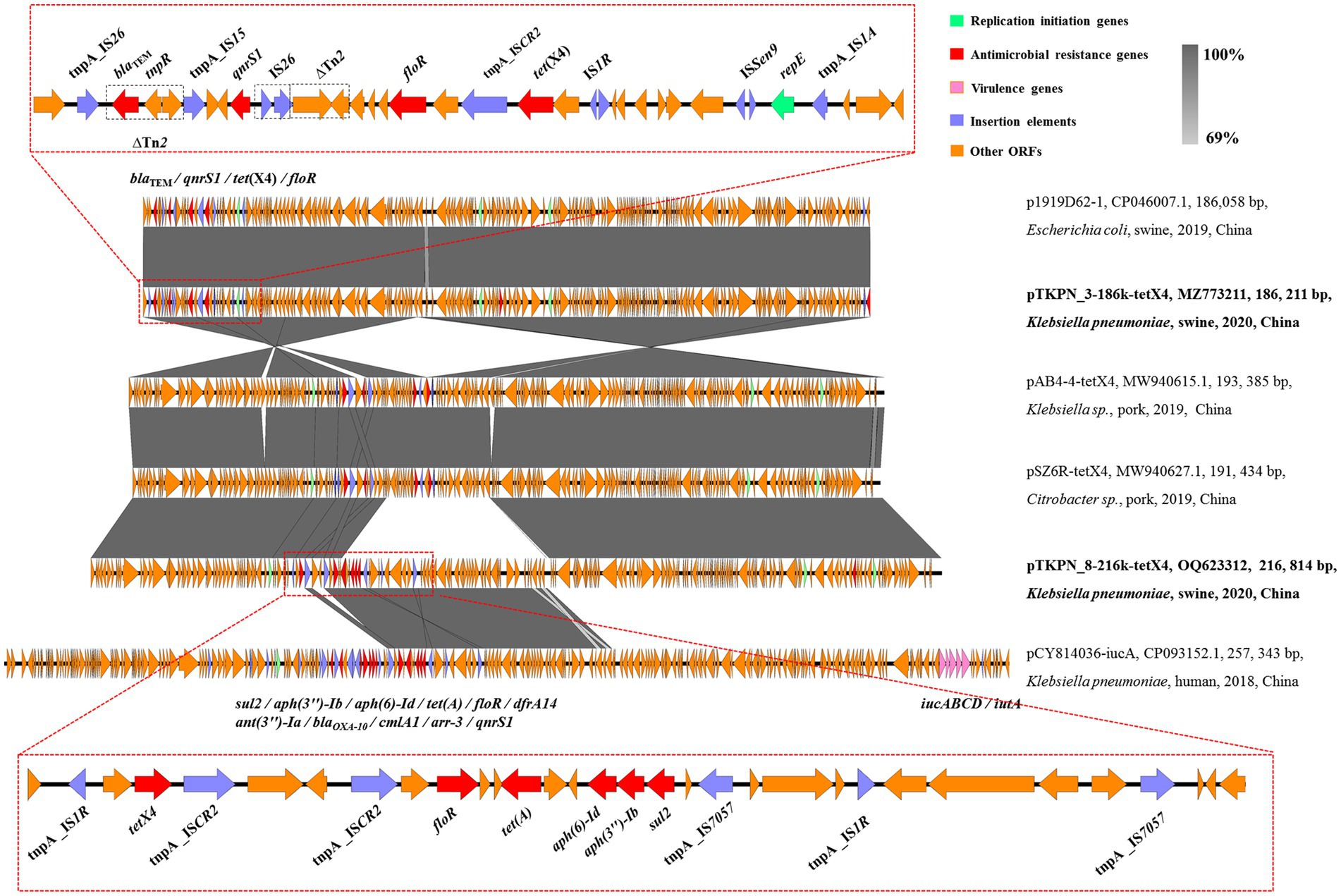
Figure 2. Structure analysis of plasmids pTKPN_3-186k-tetX4 and pTKPN_8-216k-tetX4. Major structural features of pTKPN_3-186k-tetX4 and pTKPN_8-216k-tetX4 were compared with those of plasmids p1919D62-1 (GenBank accession no. CP046007.1), pAB4-4-tetX4 (GenBank accession no. MW940615.1), pSZ6R-tetX4 (GenBank accession no. MW940627.1), and pCY814036-iucA (GenBank accession no. CP093152.1). Gray shading indicates shared regions with a high degree of homology (>69%). Red and pink represent the antimicrobial resistance genes and virulence genes, respectively, and purple is the insertion elements.
The complete sequence of another tet(X4)-positive plasmid pTKPN_8-216k-tetX4 was obtained by long-read sequencing. pTKPN_8-216k-tetX4 was 216,814 bp in size and also belongs to an IncHI1B(R27)/IncHI1A/IncFIA(HI1) type hybrid plasmid. pTKPN_8-216k-tetX4 exhibited a high similarity (74% coverage, 100% identity) with pTKPN_3-186k-tetX4, in particular, it had an additional ~30 kp fragment. This fragment was similar to pCY814036-iucA (GenBank accession number: CP093152.1; Figure 2). pCY814036-iucA was a multi-drug resistant and hypervirulent hybrid plasmid and isolated from a K. pneumoniae strain in China. pTKPN_8-216k-tetX4 also carried the conjugation-related genes traC, traD, traG, traI, traJ, traU, and mobI. In addition to tet(X4) gene, aph(3″)-Ib, aph(6)-Id, floR, sul2 and tet(A) were identified in pTKPN_8-216k-tetX4. It was worth noting that similar to pTKPN_3-186k-tetX4, tet(X4) gene was flanked by mobile elements IS1R and ISCR2 in pTKPN_8-216k-tetX4 (Figure 2).
Discussion
To date, the plasmid-borne tet(X) genes have been identified in >15 different Gram-negative species, with tet(X4)-positive E. coli isolated from food-producing animals being the most common (He et al., 2019; Sun et al., 2019). The tet(X4)-carrying K. pneumoniae strains has so far been rarely reported. A recently investigation showed 58 tet(X4)-positive strains were isolated from 139 fresh pork samples and the most strains were identified as E. coli (55/58), only two were K. pneumoniae (Li R. et al., 2021). In this study, two tet(X4)-harbouring tigecycline resistant K. pneumoniae were detected from swine nasal swabs in China. According to previous study, the prevalence of tet(X) genes in K. pneumoniae was significantly lower than in Escherichia coli (3.84%, 95/2475) and Acinetobacter spp. (5.02%, 193/3846; Chen et al., 2020; Sun et al., 2020). Although the tet(X4) gene was present in K. pneumoniae at a low prevalence, the TKPN_3 and TKPN_8 strains also contained many important virulence factors (Hsieh et al., 2019; Wang et al., 2020), such as enterobactin and aerobactin, and showed high virulence phenotype. Given the harmfulness of K. pneumoniae in clinical infections, the emergence of a tet(X4)-positive strain should be alarmed.
Until now, tet(X4)-bearing plasmids ranged from 9 to 315 kb and were categorized as ColE2-like, IncQ, IncX1, IncX4, IncA/C2, IncFII, IncFIB, IncI1, and hybrid plasmids with different replicons (Li R. et al., 2020), which suggested that tet(X4) gene could be captured by a range of mobile genetic elements circulating among bacterial strains. Moreover, the mobile elements, particularly ISCR2 and IS1R, could assist the integration and spread of tet(X4) gene between different plasmids. The host range of the tet(X4) gene is likely to be further expanded. According to tet(X4)-bearing plasmid type distribution, IncFIB(K)/IncFIA(HI1)/IncX1 hybrid plasmids were the most widespread in the sequenced plasmids (Li R. et al., 2020). In this study, both pTKPN_3-186k-tetX4 and pTKPN_8-216k-tetX4 belonged to IncHI1B(R27)/IncHI1A/IncFIA(HI1) hybrid plasmid type, which have been reported in several different species such as E. coli, Salmonella enterica and Citrobacter sp. Correspondingly, pTKPN_3-186k-tetX4 and pTKPN_8-216k-tetX4 was confirmed to be able to transfer from K. pneumoniae to E. coli. In contrast, IncFII-type plasmid carrying tet(X4) in K. pneumoniae was found to be non-self-transferable and could be only co-transferred with the help of other conjugative plasmids (Zhai et al., 2022). It is possible that pTKPN_3-186k-tetX4-like plasmids will become more widespread in the near future.
Worrisomely, the tet(X)-mediated tigecycline resistance has been detected in carbapenem- and colistin-resistant Acinetobacter spp. and Escherichia spp. strains (Chen et al., 2020; Li Y. et al., 2020). Future efforts are needed to prevent and monitor the emergence of tet(X)-mediated tigecycline- and carbapenem- resistant K. pneumoniae from all related sectors. Our data contributes to understanding of the genetic characteristic of tet(X4) and their transferabilities in K. pneumoniae.
Data availability statement
The names of the repository/repositories and accession number(s) can be found at: https://www.ncbi.nlm.nih.gov/genbank/, MZ773211 and OQ623312.
Author contributions
L-NQ, BY, and MD: conceptualization. YY and RH: data curation. YY, RH, YW, and MQ: investigation. YY, RH, YW, MQ, JC, YF, RZ, LX, and XG: methodology. G-BT, L-NQ, BY, and MD: resources. YY and RH: visualization. YY, RH, YW, and MQ: writing original draft. L-NQ, BY, MD, and G-BT: writing, review and editing. All authors contributed to the article and approved the submitted version.
Funding
This work was supported by the National Natural Science Foundation of China (grant number 82061128001 and 81830103 to G-BT), Guangdong Natural Science Foundation (grant number 2017A030306012 to G-BT), National Key Research and Development Program (grant number 2017ZX10302301 to G-BT), Project of High-level Health Teams of Zhu hai in 2018 (The Innovation Team for Antimicrobial Resistance and Clinical Infection to G-BT), and the Project 111 (grant number B12003 to G-BT).
Conflict of interest
The authors declare that the research was conducted in the absence of any commercial or financial relationships that could be construed as a potential conflict of interest.
Publisher’s note
All claims expressed in this article are solely those of the authors and do not necessarily represent those of their affiliated organizations, or those of the publisher, the editors and the reviewers. Any product that may be evaluated in this article, or claim that may be made by its manufacturer, is not guaranteed or endorsed by the publisher.
Supplementary material
The Supplementary material for this article can be found online at: https://www.frontiersin.org/articles/10.3389/fmicb.2023.1130708/full#supplementary-material
Footnotes
References
Bankevich, A., Nurk, S., Antipov, D., Gurevich, A. A., Dvorkin, M., Kulikov, A. S., et al. (2012). SPAdes: a new genome assembly algorithm and its applications to single-cell sequencing. J. Comput. Biol. 19, 455–477. doi: 10.1089/cmb.2012.0021
Buckner, M. M. C., Saw, H. T. H., Osagie, R. N., Mcnally, A., Ricci, V., Wand, M. E., et al. (2018). Clinically relevant plasmid-host interactions indicate that transcriptional and not genomic modifications ameliorate fitness costs of Klebsiella pneumoniae carbapenemase-carrying plasmids. MBio 9, e02303–e02317. doi: 10.1128/mBio.02303-17
Chen, C., Cui, C. Y., Yu, J. J., He, Q., Wu, X. T., He, Y. Z., et al. (2020). Genetic diversity and characteristics of high-level tigecycline resistance Tet(X) in Acinetobacter species. Genome Med. 12:111. doi: 10.1186/s13073-020-00807-5
CLSI (2019). Performance standards for antimicrobial susceptibility testing, 29th. Annapolis Junction, MD: Clinical and Laboratory Standards Institute.
Dong, N., Zeng, Y., Cai, C., Sun, C., Lu, J., Liu, C., et al. (2022). Prevalence, transmission, and molecular epidemiology of tet(X)-positive bacteria among humans, animals, and environmental niches in China: an epidemiological, and genomic-based study. Sci. Total Environ. 818:151767. doi: 10.1016/j.scitotenv.2021.151767
Du, X., He, F., Shi, Q., Zhao, F., Xu, J., Fu, Y., et al. (2018). The rapid emergence of tigecycline resistance in blaKPC-2 harboring Klebsiella pneumoniae, as mediated in vivo by mutation in tetA during tigecycline treatment. Front. Microbiol. 9:648. doi: 10.3389/fmicb.2018.00648
EUCAST (2021). European committee on antimicrobial susceptibility testing breakpoint tables for interpretation of MICs and zone diameters European committee on antimicrobial susceptibility testing breakpoint tables for interpretation of MICs and zone diameters, version 11.0. Sweden: EUCAST.
Fang, L., Chen, Q., Shi, K., Li, X., Shi, Q., He, F., et al. (2016). Step-wise increase in tigecycline resistance in Klebsiella pneumoniae associated with mutations in ramR, lon and rpsJ. PLoS One 11:e0165019. doi: 10.1371/journal.pone.0165019
Feng, Y., Zou, S., Chen, H., Yu, Y., and Ruan, Z. (2021). BacWGSTdb 2.0: a one-stop repository for bacterial whole-genome sequence typing and source tracking. Nucleic Acids Res. 49, D644–D650. doi: 10.1093/nar/gkaa821
Gu, D., Dong, N., Zheng, Z., Lin, D., Huang, M., Wang, L., et al. (2018). A fatal outbreak of ST11 carbapenem-resistant hypervirulent Klebsiella pneumoniae in a Chinese hospital: a molecular epidemiological study. Lancet Infect. Dis. 18, 37–46. doi: 10.1016/s1473-3099(17)30489-9
Hansen, D. S., Gottschau, A., and Kolmos, H. J. (1998). Epidemiology of Klebsiella bacteraemia: a case control study using Escherichia coli bacteraemia as control. J. Hosp. Infect. 38, 119–132. doi: 10.1016/s0195-6701(98)90065-2
He, F., Fu, Y., Chen, Q., Ruan, Z., Hua, X., Zhou, H., et al. (2015). Tigecycline susceptibility and the role of efflux pumps in tigecycline resistance in KPC-producing Klebsiella pneumoniae. PLoS One 10:e0119064. doi: 10.1371/journal.pone.0119064
He, T., Wang, R., Liu, D., Walsh, T. R., Zhang, R., Lv, Y., et al. (2019). Emergence of plasmid-mediated high-level tigecycline resistance genes in animals and humans. Nat. Microbiol. 4, 1450–1456. doi: 10.1038/s41564-019-0445-2
Hsieh, P. F., Lu, Y. R., Lin, T. L., Lai, L. Y., and Wang, J. T. (2019). Klebsiella pneumoniae type VI secretion system contributes to bacterial competition, cell invasion, type-1 fimbriae expression, and in vivo colonization. J. Infect. Dis. 219, 637–647. doi: 10.1093/infdis/jiy534
Letunic, I., and Bork, P. (2016). Interactive tree of life (iTOL) v3: an online tool for the display and annotation of phylogenetic and other trees. Nucleic Acids Res. 44, W242–W245. doi: 10.1093/nar/gkw290
Li, Y., Li, Y., Bu, K., Wang, M., Wang, Z., and Li, R. (2022). Antimicrobial resistance and genomic epidemiology of tet(X4)-bearing bacteria of pork origin in Jiangsu, China. Genes 14:36. doi: 10.3390/genes14010036
Li, R., Li, Y., Peng, K., Yin, Y., Liu, Y., He, T., et al. (2021). Comprehensive genomic investigation of tigecycline resistance gene tet(X4)-bearing strains expanding among different settings. Microbiol. Spectr. 9:e0163321. doi: 10.1128/spectrum.01633-21
Li, R., Lu, X., Peng, K., Liu, Z., Li, Y., Liu, Y., et al. (2020). Deciphering the structural diversity and classification of the mobile tigecycline resistance gene tet(X)-bearing plasmidome among bacteria. mSystems 5, e00134–e00120. doi: 10.1128/mSystems.00134-20
Li, Y., Wang, Q., Peng, K., Liu, Y., Li, R., and Wang, Z. (2020). Emergence of carbapenem- and tigecycline-resistant proteus cibarius of animal origin. Front. Microbiol. 11:1940. doi: 10.3389/fmicb.2020.01940
Li, Y., Wang, Q., Peng, K., Liu, Y., Xiao, X., Mohsin, M., et al. (2021). Distribution and genomic characterization of tigecycline-resistant tet(X4)-positive Escherichia coli of swine farm origin. Microb. Genom. 7:000667. doi: 10.1099/mgen.0.000667
Liu, C., Dong, N., Zeng, Y., Lu, J., Chen, J., Wang, Y., et al. (2022). Co-transfer of last-line antibiotic resistance and virulence operons by an IncFIBk-FII-X3-ColKP3 hybrid plasmid in Klebsiella pneumoniae. J. Antimicrob. Chemother. 77, 1856–1861. doi: 10.1093/jac/dkac121
Low, Y. M., Chong, C. W., Yap, I. K. S., Chai, L. C., Clarke, S. C., Ponnampalavanar, S., et al. (2018). Elucidating the survival and response of carbapenem resistant Klebsiella pneumoniae after exposure to imipenem at sub-lethal concentrations. Pathog. Glob. Health 112, 378–386. doi: 10.1080/20477724.2018.1538281
Lv, L., Wan, M., Wang, C., Gao, X., Yang, Q., Partridge, S. R., et al. (2020). Emergence of a plasmid-encoded resistance-nodulation-division efflux pump conferring resistance to multiple drugs, including tigecycline, in Klebsiella pneumoniae. MBio 11, e02930–e02919. doi: 10.1128/mBio.02930-19
Page, A. J., Cummins, C. A., Hunt, M., Wong, V. K., Reuter, S., Holden, M. T., et al. (2015). Roary: rapid large-scale prokaryote pan genome analysis. Bioinformatics 31, 3691–3693. doi: 10.1093/bioinformatics/btv421
Raisanen, K., Lyytikainen, O., Kauranen, J., Tarkka, E., Forsblom-Helander, B., Gronroos, J. O., et al. (2020). Molecular epidemiology of carbapenemase-producing Enterobacterales in Finland, 2012-2018. Eur. J. Clin. Microbiol. Infect. Dis. 39, 1651–1656. doi: 10.1007/s10096-020-03885-w
Ruzin, A., Visalli, M. A., Keeney, D., and Bradford, P. A. (2005). Influence of transcriptional activator RamA on expression of multidrug efflux pump AcrAB and tigecycline susceptibility in Klebsiella pneumoniae. Antimicrob. Agents Chemother. 49, 1017–1022. doi: 10.1128/AAC.49.3.1017-1022.2005
Seemann, T. (2014). Prokka: rapid prokaryotic genome annotation. Bioinformatics 30, 2068–2069. doi: 10.1093/bioinformatics/btu153
Seifert, H., Blondeau, J., and Dowzicky, M. J. (2018). In vitro activity of tigecycline and comparators (2014-2016) among key WHO 'priority pathogens' and longitudinal assessment (2004-2016) of antimicrobial resistance: a report from the T.E.S.T. study. Int. J. Antimicrob. Agents 52, 474–484. doi: 10.1016/j.ijantimicag.2018.07.003
Siu, L. K., Yeh, K. M., Lin, J. C., Fung, C. P., and Chang, F. Y. (2012). Klebsiella pneumoniae liver abscess: a new invasive syndrome. Lancet Infect. Dis. 12, 881–887. doi: 10.1016/S1473-3099(12)70205-0
Stamatakis, A. (2006). RAxML-VI-HPC: maximum likelihood-based phylogenetic analyses with thousands of taxa and mixed models. Bioinformatics 22, 2688–2690. doi: 10.1093/bioinformatics/btl446
Sun, J., Chen, C., Cui, C. Y., Zhang, Y., Liu, X., Cui, Z. H., et al. (2019). Plasmid-encoded tet(X) genes that confer high-level tigecycline resistance in Escherichia coli. Nat. Microbiol. 4, 1457–1464. doi: 10.1038/s41564-019-0496-4
Sun, C., Cui, M., Zhang, S., Liu, D., Fu, B., Li, Z., et al. (2020). Genomic epidemiology of animal-derived tigecycline-resistant Escherichia coli across China reveals recent endemic plasmid-encoded tet(X4) gene. Commun. Biol. 3:412. doi: 10.1038/s42003-020-01148-0
Villa, L., Feudi, C., Fortini, D., Garcia-Fernandez, A., and Carattoli, A. (2014). Genomics of KPC-producing Klebsiella pneumoniae sequence type 512 clone highlights the role of RamR and ribosomal S10 protein mutations in conferring tigecycline resistance. Antimicrob. Agents Chemother. 58, 1707–1712. doi: 10.1128/AAC.01803-13
Wang, J., Lu, M. J., Wang, Z. Y., Jiang, Y., Wu, H., Pan, Z. M., et al. (2022). Tigecycline-resistant Escherichia coli ST761 carrying tet(X4) in a pig farm, China. Front. Microbiol. 13:967313. doi: 10.3389/fmicb.2022.967313
Wang, G., Zhao, G., Chao, X., Xie, L., and Wang, H. (2020). The characteristic of virulence, biofilm and antibiotic resistance of Klebsiella pneumoniae. Int. J. Environ. Res. Public Health 17:6278. doi: 10.3390/ijerph17176278
Wick, R. R., Heinz, E., Holt, K. E., and Wyres, K. L. (2018). Kaptive web: user-friendly capsule and lipopolysaccharide serotype prediction for Klebsiella genomes. J. Clin. Microbiol. 56, e00197–e00118. doi: 10.1128/JCM.00197-18
Wyres, K. L., Lam, M. M. C., and Holt, K. E. (2020). Population genomics of Klebsiella pneumoniae. Nat. Rev. Microbiol. 18, 344–359. doi: 10.1038/s41579-019-0315-1
Yu, Y., Cui, C. Y., Kuang, X., Chen, C., Wang, M. G., Liao, X. P., et al. (2021). Prevalence of tet(X4) in Escherichia coli from duck farms in Southeast China. Front. Microbiol. 12:716393. doi: 10.3389/fmicb.2021.716393
Keywords: Klebsiella pneumoniae , tigecycline resistance, tet(X4), horizontal transfer, swine
Citation: Yang Y, He R, Wu Y, Qin M, Chen J, Feng Y, Zhao R, Xu L, Guo X, Tian G-B, Dai M, Yan B and Qin L-N (2023) Characterization of two multidrug-resistant Klebsiella pneumoniae harboring tigecycline-resistant gene tet(X4) in China. Front. Microbiol. 14:1130708. doi: 10.3389/fmicb.2023.1130708
Edited by:
Tao Li, Shanghai Veterinary Research Institute (CAAS), ChinaReviewed by:
Tao He, Jiangsu Academy of Agricultural Sciences (JAAS), ChinaRuichao Li, Yangzhou University, China
Copyright © 2023 Yang, He, Wu, Qin, Chen, Feng, Zhao, Xu, Guo, Tian, Dai, Yan and Qin. This is an open-access article distributed under the terms of the Creative Commons Attribution License (CC BY). The use, distribution or reproduction in other forums is permitted, provided the original author(s) and the copyright owner(s) are credited and that the original publication in this journal is cited, in accordance with accepted academic practice. No use, distribution or reproduction is permitted which does not comply with these terms.
*Correspondence: Li-Na Qin, cWlubG5hQG1haWwuc3lzdS5lZHUuY24=; Bin Yan, eWFuYmluMjIyQGZveG1haWwuY29t; Min Dai, ZGFpbWluMTAxNUBjbWMuZWR1LmNu
†These authors have contributed equally to this work
‡Present Address: Li-Na Qin, Guangdong Province Translational Forensic Medicine Engineering Technology Research Center, Sun Yat-sen University, Guangzhou, China