- Department of Pathology, Microbiology and Immunology, New York Medical College, Valhalla, NY, United States
Age-related illnesses, including hypertension and accompanying metabolic disorders, compromise immunity and exacerbate infection-associated fatalities. Renin-angiotensin system (RAS) is the key mechanism that controls blood pressure. Upregulation of RAS through angiotensin receptor type 1 (AT1R), a G-protein coupled receptor, contributes to the pathophysiological consequences leading to vascular remodeling, hypertension, and end-organ damage. Genetic variations that increase the expression of human AT1R may cause the above pathological outcomes associated with hypertension. Previously we have shown that our chronically hypertensive transgenic (TG) mice containing the haplotype-I variant (Hap-I, hypertensive genotype) of human AT1R (hAT1R) gene are more prone to develop the metabolic syndrome-related disorders as compared to the TG mice containing the haplotype-II variant (Hap-II, normotensive genotype). Since aging and an increased risk of hypertension can impact multiple organ systems in a complex manner, including susceptibility to various infections, the current study investigated the susceptibility and potential effect of acute bacterial infection using a Gram-negative intracellular bacterial pathogen, Francisella tularensis in our hAT1R TG mice. Our results show that compared to Hap-II, F. tularensis-infected aged Hap-I TG mice have significantly higher mortality post-infection, higher bacterial load and lung pathology, elevated inflammatory cytokines and altered gene expression profile favoring hypertension and inflammation. Consistent with worsened phenotype in aged Hap-I mice post-Francisella infection, gene expression profiles from their lungs revealed significantly altered expression of more than 1,400 genes. Furthermore, bioinformatics analysis identified genes associated with RAS and IFN-γ pathways regulating blood pressure and inflammation. These studies demonstrate that haplotype-dependent over-expression of the hAT1R gene leads to enhanced susceptibility and lethality due to F. tularensis LVS infection, which gets aggravated in aged animals. Clinically, these findings will help in exploring the role of AT1R-induced hypertension and enhanced susceptibility to infection-related respiratory diseases.
Introduction
The severity of an infectious disease is primarily associated with an effect of pathogen or host factors. Underlying comorbidities like diabetes, hypertension, obesity, cardiovascular, renal, and chronic lung diseases have the worst outcomes. These comorbidities, mostly associated with old age, compromise immunity, exacerbate organ damage, and may result in death. The immune mechanisms contributing to enhanced susceptibility to infections in an aged individual with these underlying conditions are not very well known. Hypertension is one of the major predisposing factors responsible for increased susceptibility to infections (Harrison et al., 2011). The renin-angiotensin-aldosterone system (RAAS) plays a major role in blood pressure regulation (Muñoz-Durango et al., 2016; Mirabito Colafella et al., 2019). Angiotensin receptor type 1 (AT1R), a G-protein coupled receptor, mediates the effect of angiotensin-II and contributes to pathophysiological consequences such as vascular remodeling, hypertension, and end-organ damage (Mehta and Griendling, 2007; Forrester et al., 2018). Genetic variations that increase human AT1R (hAT1R) expression can lead to these pathological outcomes due to RAAS overactivity. In previous studies, we have developed unique transgenic (TG) mouse models that express the human AT1R (hAT1R) gene (Jain et al., 2013). Mice TG for haplotype-I (Hap-I) of hAT1R are hypertensive, while those with haplotype-II (Hap-II) are normotensive and exhibit normal blood pressure. Using our Hap-I TG mouse model, we have demonstrated that aging is associated with an enhanced trans-regulation of the hAT1R gene via stronger binding of STAT3, USF1, and Pol-II transcription factors (Jain et al., 2018b). We have also shown that higher hAT1R expression leads to elevated blood pressure and age-associated metabolic complications in Hap-I TG mice (Jain et al., 2018a).
Francisella tularensis is a Gram-negative, intracellular bacterial pathogen responsible for causing an acutely fatal disease known as tularemia (Ellis et al., 2002; Sjöstedt, 2007). F. tularensis is highly virulent and only 1–10 organisms, if inhaled, are sufficient to cause severe disease and death. Due to its extremely high virulence, Francisella was used in bioweapon programs in the past and now poses a threat as a potential bioterror agent (Altman and Wachs, 2002). The Centers for Disease Control has categorized F. tularensis as a Tier 1 Category A select agent. Of the four subspecies of F. tularensis, subspecies tularensis and holarctica are capable of causing tularemia in humans. The live vaccine strain (LVS) derived from the F. tularensis subspecies holarctica serves as an excellent surrogate for the highly virulent F. tularensis subspecies tularensis strains to study the immunopathogenesis of tularemia in a mouse model (Mörner et al., 1993). The clinical manifestations of tularemia depend on the route of bacterial entry and may present in ulceroglandular, typhoidal, or pneumonic form if the bacteria are acquired through the skin, orally, or through inhalation. Pneumonic tularemia is an acute and fatal form of the disease (Sjöstedt, 2007). An increased incidence of tularemia has been reported in several mid-western states of the United States in the past decade. A higher incidence of tularemia is reported among white males of all age groups. However, individuals over the age of 65 mainly exhibit the highest incidence of tularemia in the United States (Bishop et al., 2023).
Although hypertension is considered a major risk factor for diminished immunity and increased susceptibility to infections, the exact pathophysiology of an infectious disease in an aged hypertensive model is not well studied. In this study, we used pneumonic tularemia as an infectious disease model and Hap-I and Hap-II TG mice to study the impact of hypertension and old age on susceptibility and immunopathogenesis of F. tularensis infection. Our results identify unique immunopathogenic mechanisms that may contribute to the increased susceptibility of aged hypertensive individuals to acute infectious diseases.
Initially, we looked at the dose-dependent effect of intranasal (i.n.) F. tularensis LVS infection on morbidity and mortality in our transgenic strains. We also studied the lung pathology and pro-inflammatory cytokine profile in the serum and tissue post-F. tularensis LVS infection. RNA sequencing was performed to analyze alterations in gene expression profiles in canonical pathways related to hypertension and inflammation. Our results demonstrate increased susceptibility of hypertensive, aged Hap-I TG mice to F. tularensis LVS infection as evidenced by increased mortality; enhanced lung pathology and bacterial burden; higher levels of cytokines, and an upregulated expression of the components of the RAS pathway.
Materials and methods
Bacterial strains and media
The F. tularensis subsp. holarctica LVS (ATCC 29684; American Type Culture Collection, Rockville, MD) was used in this work. All the work was carried out under biosafety level 2 (BSL2) containment conditions. All bacterial stock cultures were grown at 37°C with 5% CO2 on Mueller-Hinton (MH) chocolate agar plates. After 48 h of growth, individual colonies were inoculated into MH broth (MHB) supplemented with anhydrous calcium chloride, hydrous magnesium chloride, glucose, ferric pyrophosphate, and isovitalex (BD Biosciences, San Jose, CA). The cultures were grown at 37°C for 12–16 h with constant shaking. Aliquots of mid-log-phase bacteria grown in MHB were stored at −80°C. Before use, the frozen vial of bacterial stock culture was thawed in a water bath, subculture and grown at 37°C with 5% CO2 on Mueller-Hinton (MH) chocolate agar plates for 12–16 h.
In vivo studies
Transgenic mice with either Hap-I (hypertensive) or Hap-II (normotensive) haplotypes were generated as described previously (Jain et al., 2013). Briefly, the hAT1R BAC plasmid was modified by replacing the 1.7-kb promoter region from the DNA of a subject containing hap-I of the hAT1R gene. Hap-II was generated from Hap-I by replacing a 1.2-kb promoter fragment from a human subject containing Hap-II of the hAT1R gene. These BAC plasmids were linearized by NotI and microinjected in mice. The blood pressure measurement in the conscious state by radio telemetry confirmed the hypertensive phenotype of Hap-I and normal blood pressure of Hap-II transgenic lines. Adult (12–16 weeks) and old (72–80 weeks) AT1R Hap-I and Hap-II mice were maintained in the animal facility of New York Medical College. Mice were administered an anesthetic cocktail consisting of ketamine (5 mg/kg) and xylazine (4 mg/kg) intraperitoneally and underwent experimental manipulation only after they failed to exhibit a toe pinch reflex. The deeply anesthetized mice were infected intranasally (i.n.) with 1 × 104 CFU or 1 × 105 CFUs of F. tularensis LVS. The bacterial infection dose was quantified by plating serial dilutions of inoculum onto MH chocolate agar plates. Mice were observed for a period of 15 days for morbidity and mortality. The body weights of all mice were recorded daily post-infection to monitor morbidity. Lung tissues on days three and five post-infection were homogenized in 1 mL of PBS, centrifuged, and the supernatant was used to quantify the bacterial burden as Log10 CFU/mL. All animal procedures were conducted in accordance with the Institutional Animal Care and Use Committee (IACUC) guidelines approved by New York Medical College.
Histology
Lung and spleen of control and F. tularensis LVS-infected mice were fixed in 10% neutral buffered formalin, paraffin embedded, and 3-micron sections were prepared using Leica Microtome. The sections were stained with hematoxylin–eosin (H & E) and the images were captured at 20× magnification using a Nikon Eclipse 90i microscope (Nikon, United States).
Cytokine analysis
The blood from F. tularensis-infected mice was collected on day three and day five post-infection by retro-orbital bleeding. The serum was separated and used for cytokine analysis as per the manufacturer’s instructions (Quantibody® Mouse Cytokine Array-Ray Biotech, GA).
RNA extraction and qRT-PCR analysis
The left lobe of the lung collected from control and F. tularensis-infected mice on days zero and five post-infection was stored in RNA Later at −80°C. RNA was isolated using an RNAeasy Kit (Qiagen) from each lung sample. Two hundred nanograms of the total RNA was converted to cDNA using the High-Capacity cDNA Reverse Transcription Kit (Applied biosystem, Thermo Fisher Scientific). The quantitative real-time PCRs (qRT-PCR) were performed using the QuantStudio 3 Real-Time PCR System (ThermoFisher), with 40 cycles of denaturation (95°C for 15 s), annealing (55°C for 30s), and extension (72°C for 30s). Primers of human AT1R and mouse At1ar, Stat3, Usf, Ace, Ccl2, Tnf-α, Il-17, Ifn-γ, and Il-6 were purchased from Integrated DNA Technologies (IDT, IA).
RNA-sequencing and gene expression analysis
RNA isolated from the lungs of control and F. tularensis LVS-infected mice on day five post-infection was quality was checked by 4200 TapeStation (Agilent Technologies, Santa Clara, CA) and RNA quantity was determined by Qubit 2 fluorometer (Life Technologies, Carlsbad, CA). For each sample, 400 ng of RNA was used to construct a cDNA sequencing library using the TruSeq Stranded Total RNA Library Preparation Kit (Illumina, San Diego, CA) in accordance with the manufacturer’s protocol using the polyadenylated RNA extraction. Sequencing of paired end reads (75 bp × 2) was performed in Illumina NextSeq 550 system.
Raw sequence reads were de-multiplexed and trimmed for adapters by using the Illumina bcl2fastq conversion software (v2.19). Sequence reads of each sample were pseudo-aligned to Ensembl mouse GRCm38 (release 100) reference transcriptome and the gene transcript abundance was quantified by using Kallisto (v0.43.1) algorithm (Bray et al., 2016). The differential expression of genes and transcripts was achieved in paired groups by using DESeq2 package (doi:10.1186/s13059-014-0550-8) in the RStudio platform (v1.3.1073, with R v4.1.0). The gene-enriched gene ontology and pathway analyses were achieved by using Ingenuity Pathway Analysis (IPA, Qiagen).
Statistical analysis
All results were expressed as mean ± SEM. Statistical comparisons between the groups were made using two-way ANOVA with the Tukey–Kramer test. Differences between the experimental groups were considered statistically significant at a p < 0.05 level. F-values representing the ratio of variance of two independent variables and their interaction effect were also determined. Survival results were plotted as Kaplan–Meier survival curves, and significance was calculated using the Log-rank (Mantel-Cox) test. All statistical comparisons were performed using GraphPad Prism software version 9.
Results
Hypertension and age increase susceptibility to respiratory infection caused by Francisella tularensis LVS
The association of hypertension and old age with susceptibility to respiratory tularemia was determined using hypertensive Hap-I TG adult (12–16 weeks old) and old (72–80 weeks old) mice. Age-matched normotensive Hap-II TG adult and old mice were used as controls. Hap-I and Hap-II TG adult and old mice were infected intranasally with 1 × (1 × 104 CFU) and 10× (1 × 105 CFU) lethal doses of F. tularensis LVS and were observed for morbidity and mortality for 15 days post-infection. The infection doses selected were based on our several previous studies (Alqahtani et al., 2018; Ma et al., 2019; Marghani et al., 2021; Suresh et al., 2021). No differences between the survival proportions of Hap-II TG adult and old mice were observed, and 60 % of mice from both groups succumbed to infection with 1 × 104 CFUs of F. tularensis LVS with a median survival time (MST) of 12 days. Eighty percent of the Hap-I TG adult mice died with an MST of 11 days. However, 100% of the Hap-I TG old mice succumbed to infection with a significantly lower (p < 0.05) MST of 9 days as compared to Hap-I adult (11 days) mice (Figure 1A). A significant difference (p < 0.05) in MST between Hap-I old (9 days) and Hap-II old (12 days) mice was also observed. Mice from all the groups lost their body weight, indicating that all mice received the infection (Figure 1B). At a higher infection dose of 1 × 105 CFU, 100% mortality was observed in all groups of infected mice. All Hap-I TG old mice died by day six post-infection with a significantly lower (p < 0.05) MST of 4.5 days as compared to Hap-I TG adult mice (6.5 days). Hap-II TG adult and old mice succumbed to infection by day 8–9 post-infection (MST 6.5–8 days). As observed with the lower dose, a significant difference (p < 0.05) in MST between Hap-I old (4.5 days) and Hap-II old (6.5 days) mice was also observed. All infected mice lost their body weights, and their weight loss was more rapid than that observed for those infected with 1×104 CFU of F. tularensis LVS (Figures 1C,D). Together, these results demonstrate that hypertensive (Hap-I) old mice are more susceptible to acute respiratory infection caused by F. tularensis than hypertensive adults and the normotensive (Hap-II) adult and old mice.
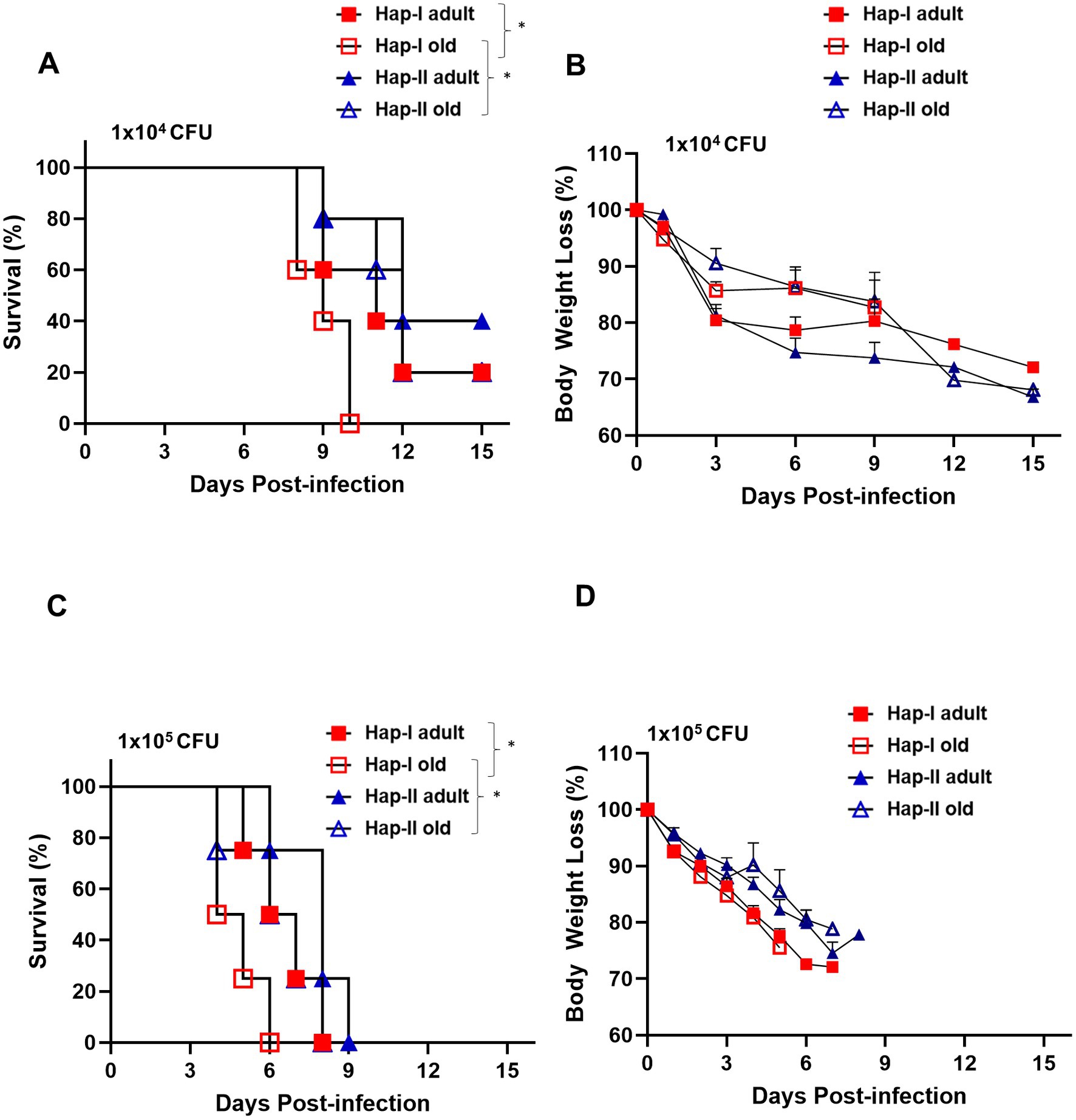
Figure 1. Susceptibility of Hap-I and Hap-II transgenic (TG) mice to Francisella tularensis LVS infection. Adult and old Hap-I (hypertensive) and Hap-II (normotensive-control) TG mice (n = 5 per group) were infected intranasally with 1 × LD100 (1 × 104 CFU) (A,B) or 10 × LD100 (1 × 105 CFU) (C,D) dose of F. tularensis LVS and were observed for morbidity and mortality for 15 days post-infection. Survival results are plotted as Kaplan-Meir survival curves (A,C) and the body weights are represented as percent initial body weight (B,D). The survival data were analyzed using the Log-rank test. *p < 0.05.
Hypertension and age are associated with impaired clearance of bacteria during an acute respiratory infection caused by Francisella tularensis LVS
It was observed that the hypertensive Hap-I TG old mice were more susceptible to F. tularensis LVS infection than their adult and normotensive counterparts. We next determined if the enhanced susceptibility of Hap-I old mice is associated with their reduced ability to clear bacteria from the site of infection. We infected age-matched Hap-I and Hap-II adult and old mice with 1 × 104 CFUs of F. tularensis LVS intranasally. Mice were sacrificed on days 3 and 5 post-infection, and their lungs were collected aseptically. The homogenates of the lungs were prepared, diluted 10-fold, and plated on MH chocolate agar plates to quantitate the bacterial load. The bacterial load was significantly higher (p < 0.01) in the Hap-I old than in Hap-I adult and Hap-II old mice on day three post-infection. The bacterial load increased in all groups of mice by day five post-infection. However, similar to day three, significantly higher (p < 0.001) numbers of bacteria were recovered from the lungs of Hap-I old than Hap-I adult and Hap-II adult and old mice. Further, like day three, on day five post-infection, the bacterial burdens were also significantly higher (p < 0.01) in Hap-I adult than in Hap-II adult mice. However, no statistical differences were observed in the bacterial load between Hap-II adult and old mice on days three and five post-infection (Figure 2). Overall, a statistically significant interaction was observed between bacterial burden in Hap-I and Hap-II mice with age and F. tularensis LVS infection [F (3.32) = 3.644, p = 0.0229]. Collectively, these results demonstrate that a hypertensive phenotype alone or along with old age impaired the ability to control bacterial growth from the site of infection which ultimately results in enhanced susceptibility to infection and adverse outcomes.
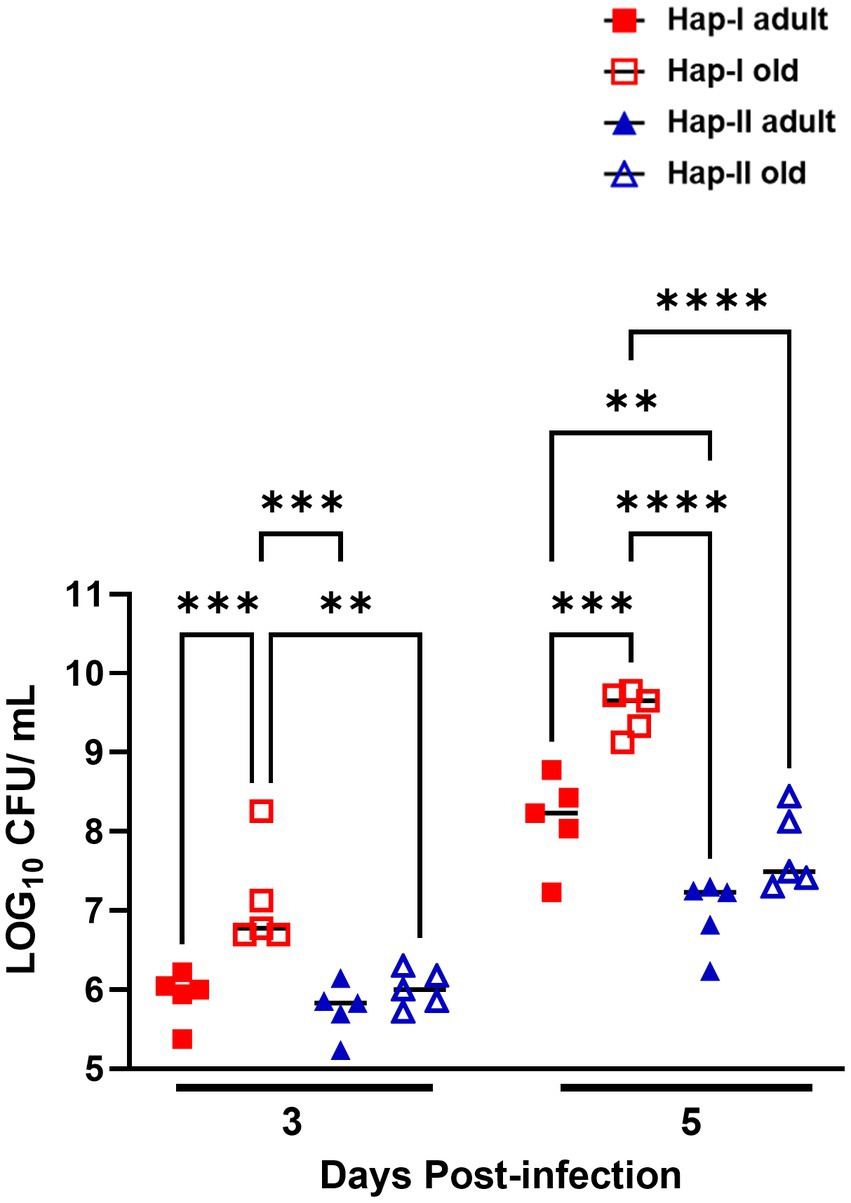
Figure 2. Bacterial load in the lungs of Hap-I and Hap-II mice. Adult and old Hap-I (hypertensive) and Hap-II (normotensive-control) TG mice (n = 4 per group) were infected intranasally with 1 × LD100 (1 × 104 CFU) dose of F. tularensis LVS. The bacterial burdens were quantitated in the lungs on days three and five post-infection. Bacterial numbers are expressed as Log10 CFU/gram of tissue. The p-values were determined using two-way ANOVA. **p < 0.01; ***p < 0.001; ****p<0.0001.
Hypertension and age are associated with severe organ damage during an acute respiratory infection caused by Francisella tularensis
It was next determined if the higher bacterial load in the lungs of Hap-I TG adult and old mice is also associated with lung and spleen damage. Lungs and spleen from Hap-I and Hap-II adult and old mice infected intranasally with 1 × 104 CFUs of LVS were collected on day five post-infection, embedded in paraffin, sectioned, and stained with H&E to observe histopathological changes. No pathological changes were observed in the lungs of control uninfected Hap-I adult and old mice, indicating that the hypertensive phenotype perse does not cause any effect in the lungs of Hap-I adult or old mice. The lungs of Hap-I adult mice infected with F. tularensis LVS showed patchy alveolar and peribronchial inflammatory foci. However, in infected Hap-I old mice, these foci of inflammation were more severe and developed into necrotizing pneumonia at several places (Figure 3A). Similar to uninfected Hap-I mice, lungs of uninfected Hap-II adult and old mice did not reveal any pathological lesions. Only mild and small patchy inflammatory foci were observed in the lungs of infected Hap-II adult and old mice, while most of the lung parenchyma was free of inflammatory lesions (Figure 3B).
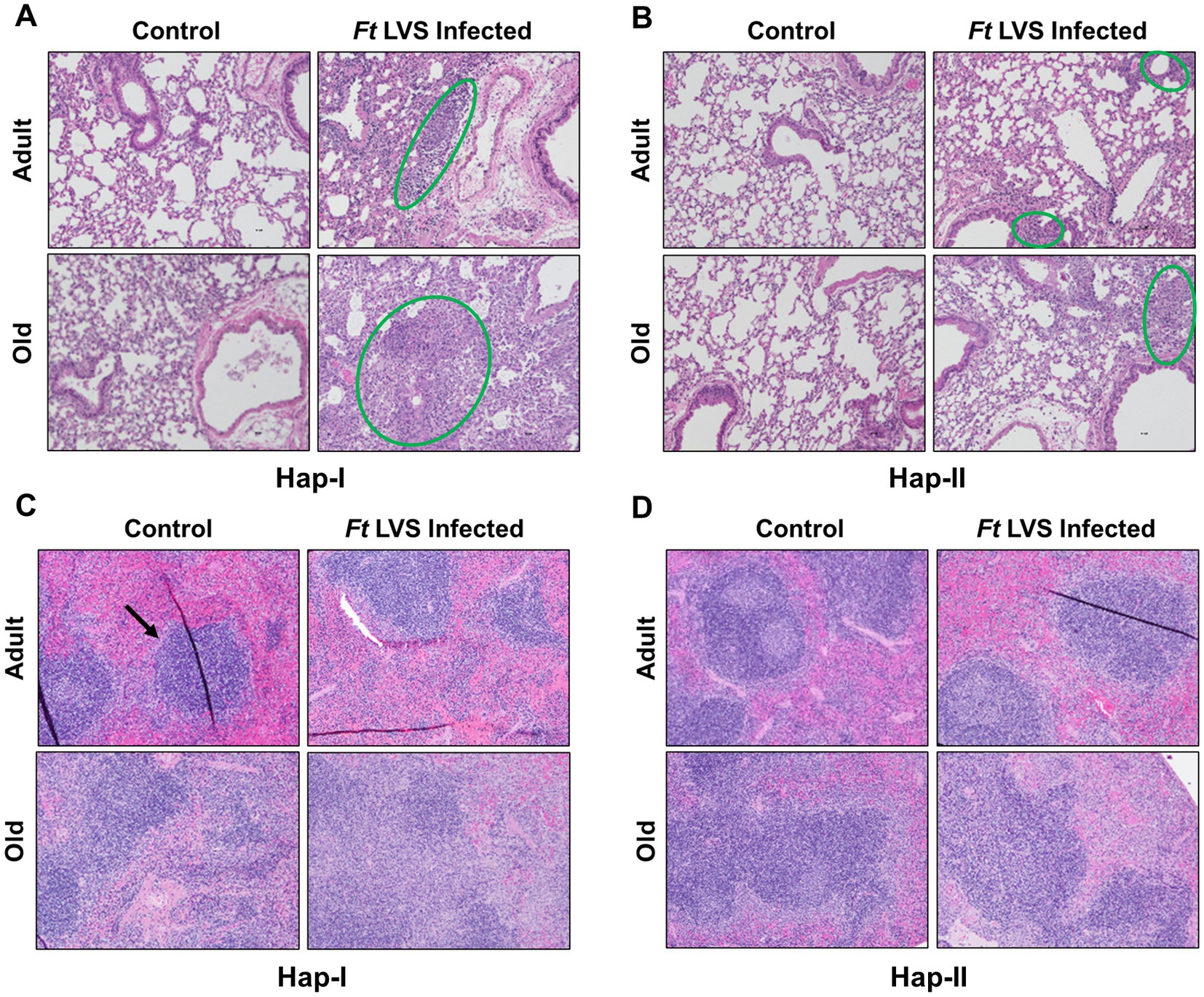
Figure 3. Histopathological lesions in the lungs and spleen of Hap-I and Hap-II mice. Adult and old Hap-I (hypertensive) and Hap-II (normotensive-control) TG mice (n = 4 per group) were infected intranasally with 1 × LD100 (1 × 104 CFU) dose of F. tularensis (Ft) LVS. Lungs (A,B) and spleens (C,D) were excised on day five post-infection, preserved in 10% formalin, paraffin-embedded, sliced into 3 μ thin sections and stained with Hematoxylin & Eosin. Stained sections were observed for histopathological lesions under a light microscope (Magnification 20×). The green circles (A,B) show alveolar and peribronchial inflammatory foci. The black arrow (C) shows normal splenic architecture.
Unlike lungs, the spleens of uninfected Hap-I old but not Hap-I adult mice revealed inflammatory, proliferative responses within the germinal centers. The lesions in the spleens of infected Hap-I adult mice revealed disrupted splenic red pulp. In F. tularensis LVS-infected Hap-I old mice, the lesions in the spleen presented with coalescing areas of neutrophilic to pyogranulomatous necrotic splenitis that resulted in complete disruption of splenic architecture (Figure 3C). The spleens of Hap-II adult mice showed normal architecture with minimal evidence of inflammatory response on day five post-infection, while a proliferative response was observed in the spleen of Hap-II old mice (Figure 3D). Taken together, results shown in Figures 1–3 demonstrate that failure to control the bacterial load by Hap-I old mice results in a hyperinflammatory response in the lungs and spleen causing severe organ damage, enhanced susceptibility, and early death when infected with F. tularensis LVS.
Increased levels of pro-inflammatory cytokines are observed in Hap-I old mice infected with Francisella tularensis LVS
Initially, we determined the basal expression levels of il-6, Tnf-α, Il-17a and ifn-γ transcripts in uninfected Hap-I and Hap-II, adult and old mice by qRT-PCR. No differences were observed in the transcript levels of these cytokines (Supplementary Figure S1). We further determined the transcripts level of these cytokines on day five post-infection. As shown in Figure 4, the transcript levels of all the cytokines tested were significantly increased in old Hap-I mice as compared to adult Hap-I (except il-17a) as well as their Hap-II counterparts on day five post-infection (Figures 4A1–A4). We further confirmed the results of qRT-PCR by determining the serum cytokine levels on day three and day five post-infection in these mice. No significant changes were observed in cytokine levels on day three post-infection in adult and old, Hap-I or Hap-II mice. However, as observed for the transcript levels, all the cytokine levels were significantly higher in old Hap-I mice as compared to their Hap-II counterparts on day five post-infection (Figures 4B1–B4). Overall, these results indicate that F. tularensis LVS infection leads to a haplotype and age-dependent augmentation of an inflammatory response which may cause increased susceptibility to infection and organ damage in hAT1R Hap-I TG mice.
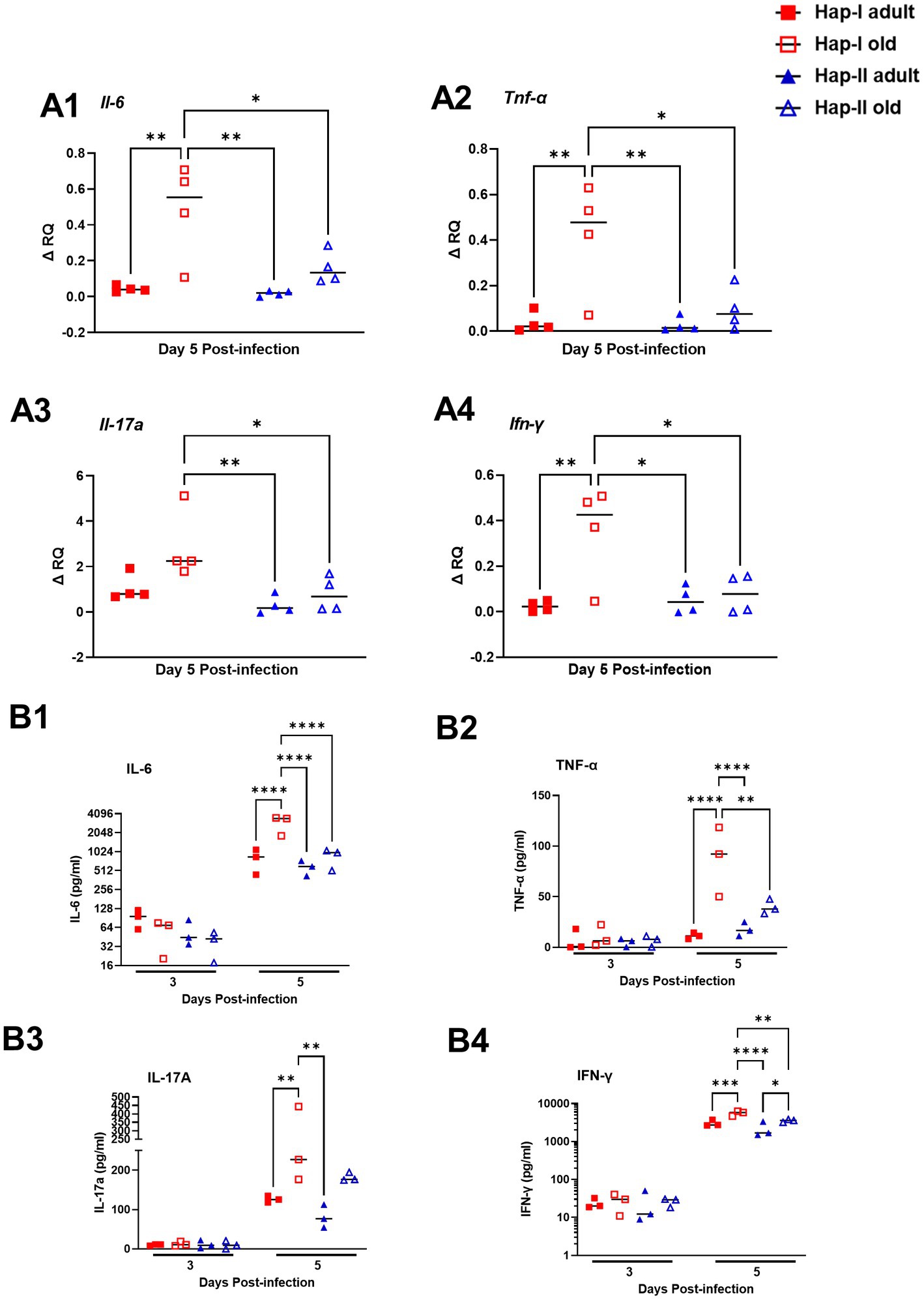
Figure 4. Expression of inflammatory cytokines in the lungs of F. tularensis LVS-infected Hap-I and Hap-II TG mice. Adult and old Hap-I (hypertensive) and Hap-II (normotensive-control) TG mice were infected intranasally with 1 × 104 CFU of F. tularensis LVS. QRT-PCR performed using RNA extracted from the lungs (n = 4 per group) on day five post-infection (A1–A4). The serum levels of the indicated cytokines (n = 3 mice per group) were assayed by ELISA days three and five post-infection with F. tularensis LVS (B1–B4). The p-values were determined using two-way ANOVA. *p < 0.05, **p < 0.01, ***p < 0.001; ****p < 0.0001.
Francisella tularensis LVS infection results in enhanced expression of RAS molecules in aged TG mice
Previously we have shown age and diet-dependent regulation of hAT1R gene expression in Hap-I and Hap-II hAT1R TG mice (Jain et al., 2018a,b). Therefore, in the present study, we examined the expression of the hAT1R gene following infection with F. tularensis LVS in adult and old hAT1R TG mice. RNA isolated from the lungs of F. tularensis LVS-infected old Hap-I mice showed significantly high hAT1R expression (p < 0.01) as compared to the adult and old Hap-II mice (Figure 5A1). However, the expression of endogenous mouse At1ar (mAt1ar) gene remained unaltered in both haplotypes (Figure 5A2). Higher levels of hAT1R in old Hap-I mice suggested a haplotype-specific increase in RAS, driven by F. tularensis LVS infection.
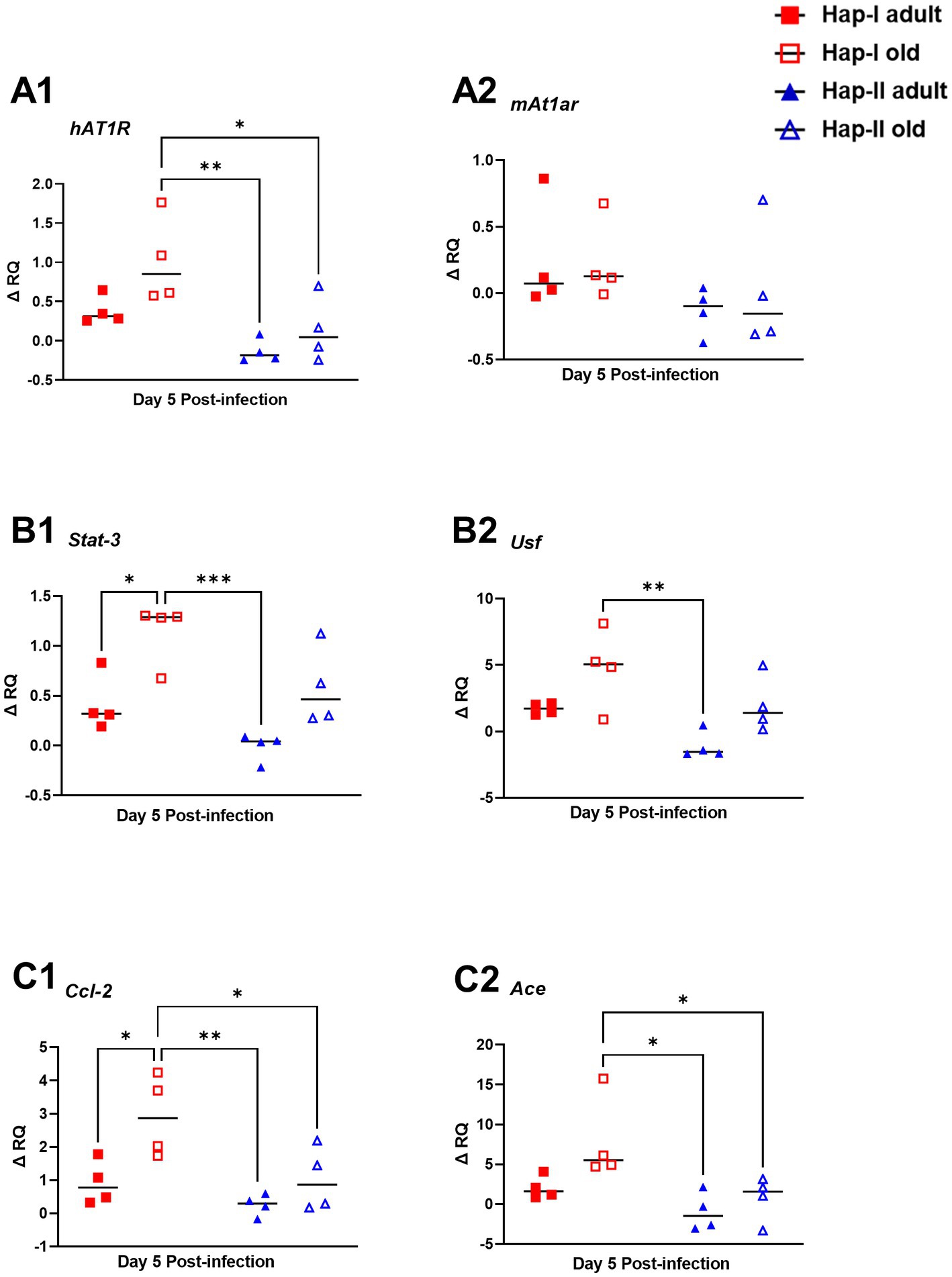
Figure 5. Expression of molecules involved in RAS signaling pathway in the lungs of F. tularensis LVS infected Hap-I and Hap-II mice. Adult and old Hap-I (hypertensive) and Hap-II (normotensive-control) TG mice (n = 4 per group/time) were infected intranasally with 1 × 104 CFU of F. tularensis LVS. RNA isolated from lungs on day five post-infection was used for quantitation of expression of human (A1) and mouse (A2) At12 genes, and other indicated molecules involved in RAS signaling pathway by qRT-PCR (B1,B2,C1,C2). Relative quantification of transcripts (ΔRQ) was done by subtracting the change in transcript levels of each control subject post-F. tularensis LVS infection. The p-values were determined using two-way ANOVA. *p < 0.05, **p < 0.01; ***p < 0.001.
Additionally, published data from our earlier studies suggest that age and diet potentiate transcriptional milieu in Hap-I TG mice and upregulate Usf and Stat3 genes, which bind strongly to promoter of the hAT1R gene (Jain et al., 2018a,b). To investigate the expression of Usf and Stat3 in F. tularensis LVS-infected adult and old Hap-I TG mice, we quantified them in the lungs by qRT-PCR. Our results indicate a highly significant age-dependent increase in Stat3 and Usf expression in Hap-I hypertensive as compared to Hap-II mice (Figures 5B1, B2). These results suggest that infection in aged subjects leads to an increased expression of Stat3 and Usf transcription factors which over-drive the transcription of hAT1R gene, specifically in Hap-I TG mice, and therefore may cause RAS augmentation.
Angiotensin convertase enzyme (ACE) catalyzes the conversion of angiotensinogen into a vasoactive octapeptide AngII. Overexpression of ACE, thus, leads to vasoconstriction and an increase in blood pressure, causing hypertension. Conversely, CCL2 is an inflammatory and immunomodulatory cytokine involved in disease pathogenesis. Therefore, we analyzed the expression of Ace and Ccl2 genes in the F. tularensis LVS-infected lungs of adult and old Hap-I and Hap-II mice. The qRT PCR results show a significant increase in Ace and Ccl2 gene expression in Hap-I old TG mice as compared to the adult or old Hap-II TG animals (p < 0.01). This expression is age dependent as we did not observe any significant difference in either Ace or Ccl2 gene expression in adult mice (Figures 5C1, C2). These results indicate the role of Ace and Ccl2 gene overexpression in the activation of the RAS pathway following infection with F. tularensis LVS.
Transcriptional analysis of lung tissues from Hap-I mice infected with Francisella tularensis LVS reveals modulations of hypertension and infection-associated canonical signaling pathways
Age-related hypertension and infection may alter the expression of genes in canonical pathways associated with blood pressure regulation and inflammation. Therefore, we next investigated if the altered profile of genes modulating RAS and IFN-γ pathways as the major regulatory mechanisms, respectively, influencing blood pressure and inflammation. RNA sequencing was performed on lung RNA from F. tularensis LVS-infected old Hap-I and age-matched uninfected control animals. The gene expression profile was analyzed by IPA software from Qiagen. As seen in the PCA plot, the results from RNA sequencing analysis indicate a clear segregation of gene expression profiles of uninfected control and F. tularensis LVS-infected Hap-I mice (Figure 6A). More than 1,400 genes were significantly altered in old Hap-I mice infected with F. tularensis LVS as shown in the Volcano plot (Figure 6B). Almost 50 percent of genes were upregulated. Canonical pathways associated with infection, including cytokine signaling and immune cell activation were significantly altered in hypertensive haplotype Hap-I (Figure 6C). Further, the results from gene expression analysis showed a higher level of cytokines genes including Il-1, Il-6, Il-17, Ifn-γ, Tnf-α and their receptors post-F. tularensis LVS-infection (Table 1A); a significant upregulation of genes involved in RAS pathway including Ccl2, Nfkb, p38 Mapk, Ras, Stat1, Stat3 and Tnf-α (Table 1B); and an upregulated expression of IFN-γ pathway genes such as Bak, Bax, Ifit, Ifn-γ, Irf, Isg, Oas1, Psmb8, Ptpn2, Stat and Tap1 (Table 1C). The RNA-Seq data are available in the GEO database under accession number GSE186016. Results from the RNA-seq gene expression analysis were corroborated by qRT-PCR analysis for the genes involved in the RAS pathway and pro-inflammatory cytokines of the IFN-γ pathway.
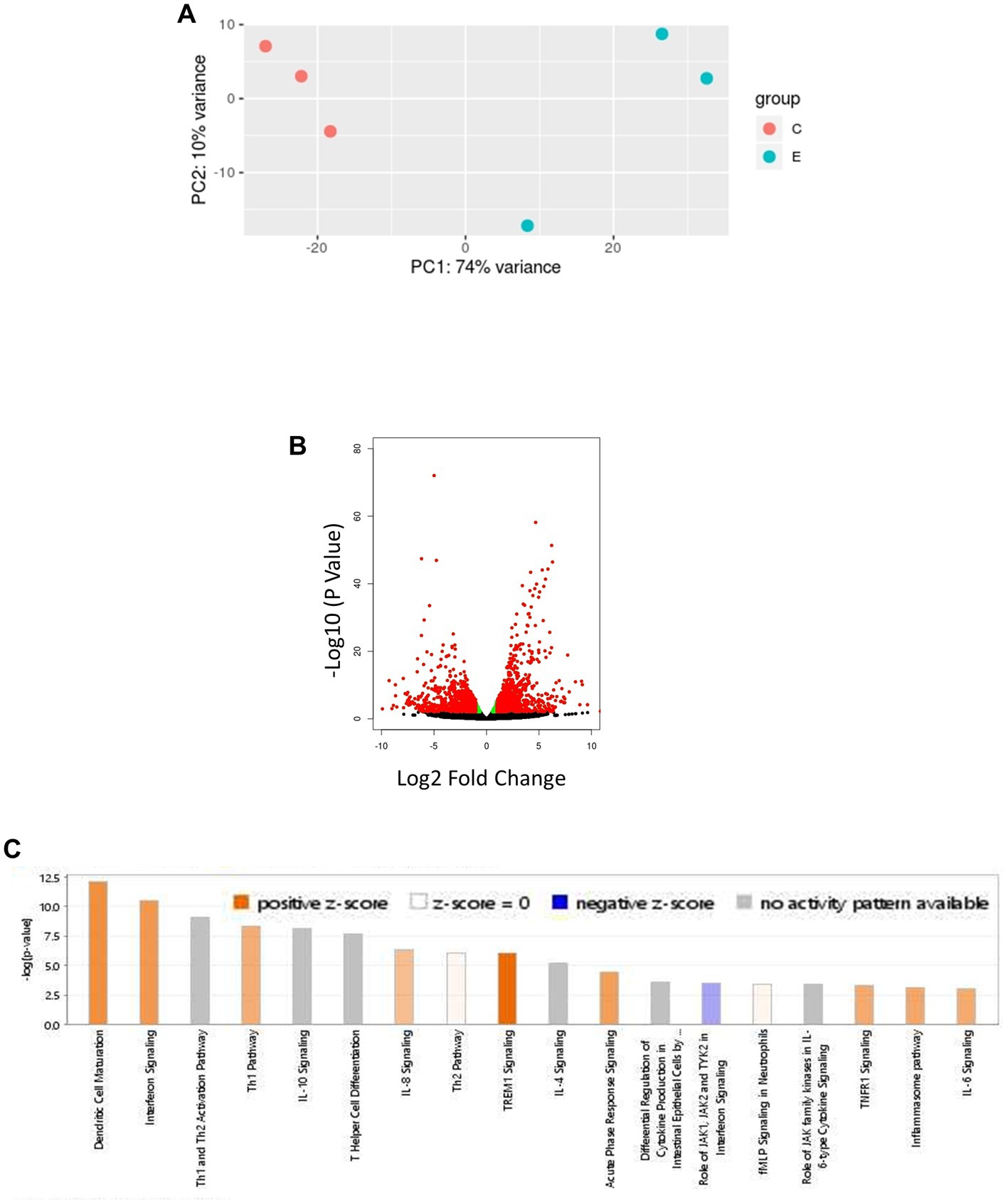
Figure 6. Bioinformatic analysis of transcriptomic data obtained from lungs of F. tularensis LVS-infected old Hap-I mice as compared to their age matched vehicle control group. PCA plot shows distinct isolation of uninfected control and F. tularensis LVS-infected Hap-I old mice (A). The volcano plot shows a significant alteration of >1,400 genes with p < 0.005 (B). Significant canonical pathways related to immune responses, including interferon signaling (C). n = 3, p < 0.005.
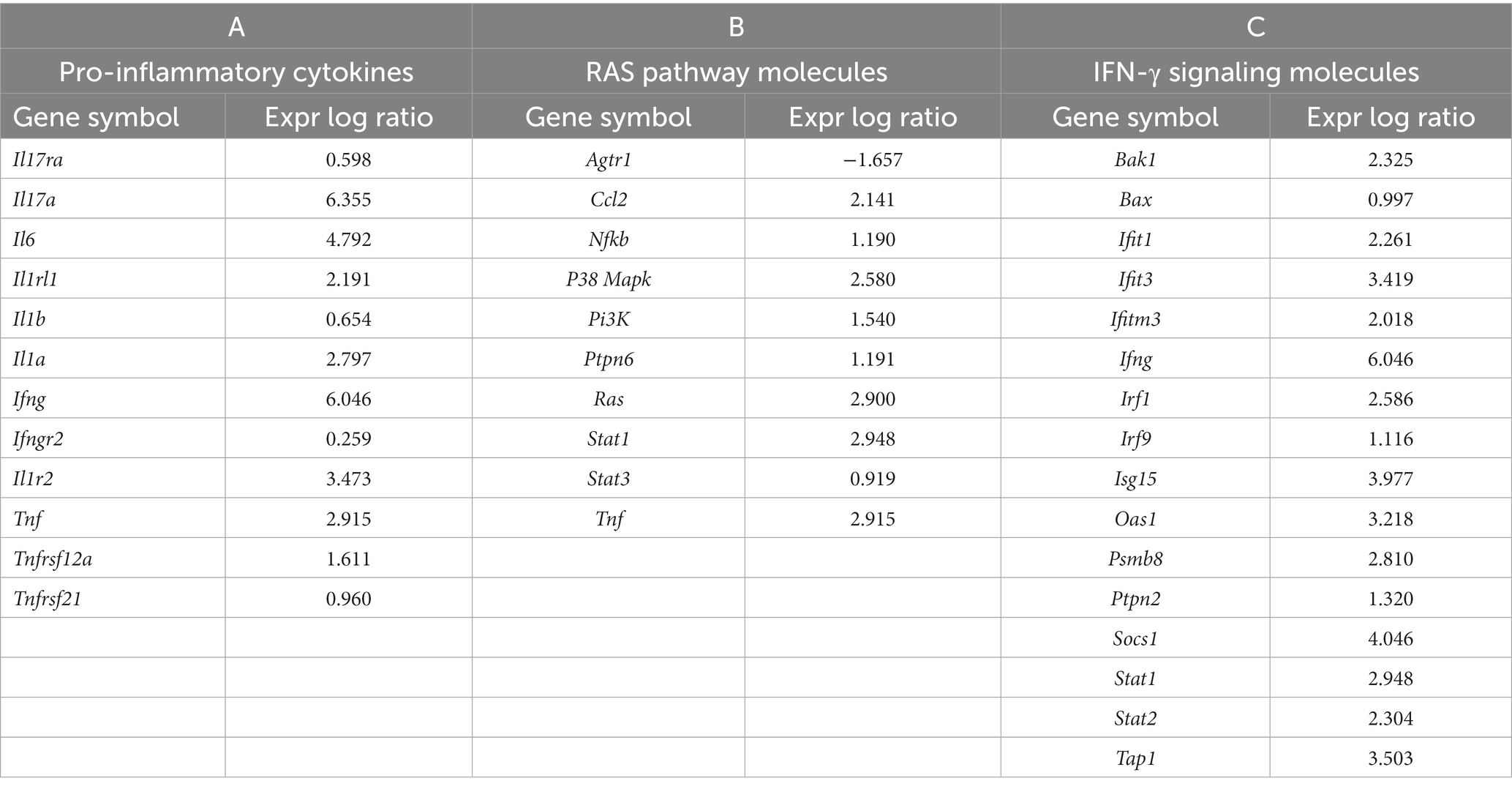
Table 1. Expression of RAS and IFN-γ pathway specific molecules as analyzed by IPA (Qiagen) in the lungs of aged hAT1R TG mice post-Francisella tularensis LVS infection.
Discussion
Old age as a contributing factor for increased susceptibility or the severity of the infection is not new. It has been demonstrated that age-related mortality is more commonly associated with pathogens capable of causing both acute and chronic infections (Kline and Bowdish, 2016). The increased susceptibility to infection in aged people is mostly associated with a decline in cell-mediated immune responses, changes in the structure and functions of the organs, especially the lungs, and degenerative diseases (Boe et al., 2017). Additionally, comorbidities such as hypertension, obesity, and diabetes also increase susceptibility to infectious diseases (Vasto et al., 2007; Shaw et al., 2013). Hypertension is the most prevalent disease and a leading cause of vascular disorders and deaths worldwide (Mills et al., 2020). Several parasitic, viral, and bacterial infectious diseases have been linked to hypertension. Higher susceptibility to malaria, COVID-19, HIV, dengue, herpesvirus, cytomegalovirus, Chlamydia and Helicobacter infections have been reported in individuals with hypertension (Cool et al., 2003; Sotiropoulos et al., 2006; Cheng et al., 2009; Mehta and Hotez, 2016; Fahme et al., 2018; Etyang et al., 2019). On the other hand, chronic bacterial periodontal diseases cause hypertension by increasing both systolic and diastolic blood pressures (Desvarieux et al., 2010). Chronic inflammation caused by hypertension has been proposed to be one of the major factors responsible for increased susceptibility and higher mortality due to these infectious diseases (Tseng et al., 2020). The complications related to hypertension get further aggravated in aged subjects. However, a lack of effective models to study the molecular mechanisms associated with hypertension and its contribution to enhanced susceptibility to infectious diseases during old age remain elusive. Previously, we generated TG mouse models of chronic hypertension exhibiting age and diet-related upregulation of RAS and associated renovascular disorders. The two lines of TG mice have variants of the human AT1R gene represented by haplotype-I (Hap-I, hypertensive genotype) and haplotype-II (Hap-II, normotensive genotype). In this study, we used pneumonic tularemia as an infectious disease model and old Hap-I and Hap-II TG mice to study the impact of hypertension and old age on susceptibility and immunopathogenesis of F. tularensis infection.
Immuosenescence, the changes that occur to immune system with aging, and inflammaging, the age-related increase of pro-inflammatory cytokine levels in blood and tissues are the hallmarks of the immune dysfunction associated with old age. Both immuosenescence and inflammaging diminish resistance to fight infection in old age. Immune dysfunctions associated with immuosenescence include defects in T and B cell development resulting in impaired innate and adaptive immune responses to pathogens and immunogens (Cohen et al., 2003; Arnold et al., 2011; Kogut et al., 2012). The number of neutrophils, monocytes and DCs as a result of immuosenescence remain unchanged or mildly decreased in aged individuals indicating that the increased basal inflammation associated with inflammaging is not due to increased cellularity (Chatta et al., 1994; Jing et al., 2009; Seidler et al., 2010). Impaired chemotaxis of neutrophils which accounts for both reduced migration as well as egression from the sites of inflammation, diminished capacity to phagocytose and kill phagocytosed bacteria by neutrophils are the most common features of immuosenescence (Wenisch et al., 2000; Butcher et al., 2001; Gomez et al., 2007). NK cell infiltration in lungs, cytotoxicity, IFN-γ production, and the effector functions of macrophages and DCs are also impaired with age (Moretto et al., 2008; Beli et al., 2011; Hearps et al., 2012).
A previous study conducted in old (20–24 months) C57BL/6 mice using F. tularensis LVS as a respiratory infection model demonstrated that old age alone does not impair the ability to clear bacteria from the lungs. In fact, old mice clear Francisella more efficiently than young mice. Although the histopathological lesions are more extensive in the lungs of old than young mice, no differences were observed in the survival of old and young mice (Mares et al., 2010a,b). Similar results have been reported for infections with several intracellular pathogens in young and old mice (Emmerling et al., 1979; Turner et al., 2002; Ehrchen et al., 2004), indicating that old age associated immuosenescence alone does not contribute to enhanced susceptibility to Francisella infection. The results obtained in the present study in normotensive Hap-II old mice agree with these results. No significant differences in bacterial burdens and survival were observed between Hap-II adult and Hap-II-old mice. However, the histopathological lesions in the lungs and spleen were more severe in Hap-II old than in the respective Hap-II adult mice. Contrary to this, hypertensive old Hap-I mice had a significantly higher bacterial load in the lungs than in their younger hypertensive or adult and old normotensive counterparts. This increased bacterial load in the lungs resulted in severe organ damage, leading to significantly increased mortality in old Hap-I mice. These results clearly demonstrate that old age and hypertension together, rather than old age alone or a hypertensive phenotype in young adults, increases the susceptibility and severity of respiratory tularemia caused by F. tularensis.
The inflammaging-associated immune dysfunction include elevated basal levels of IL-6, which transforms monocytes into antigen presenting cells to produce IL-1β and IL-23. Both IL-1β and IL-23 amplify the production of IL-17A, which is responsible for causing end-organ damage and hypertension. IL-17A has been reported to be a key pro-inflammatory cytokine associated with hypertension in individuals suffering from autoimmune disorders such as systemic lupus erythematosus (SLE) and Crohn’s disease (Toussirot, 2012; Lozovoy et al., 2014). Furthermore, IL-1β produced by the myeloid cells is also associated with exacerbation of hypertension (Alexander et al., 2019). In addition to these proinflammatory cytokines, the immune cells further exacerbate hypertension by releasing reactive oxygen species (ROS), and metalloproteinases (Madhur et al., 2010). Monocytes and macrophages, the cells primarily involved in innate immune response, also augment the development of hypertension. Mice that lack macrophage-colony stimulating factor are protected from the rise in blood pressure (De Ciuceis et al., 2005). Furthermore, monocyte-derived derived DCs from hypertensive mice produce increased amounts of ROS, IL-1β, TNF-α, IL-6, and IL-23 to promote hypertension and end-organ damage. The production of these inflammatory mediators by DCs in hypertensive individuals also skew the CD8+ T cells to produce IFN-γ and IL-17 (Kirabo et al., 2014). B and T lymphocytes cells are also linked to hypertension, as RAG1 and SCID mice that lack both the T and B cells, and BAFF-R mice, that lack mature B cells are protected from hypertension (Guzik et al., 2007; Crowley et al., 2010; Chan et al., 2015). These studies highlight the importance of inflammaging in augmenting hypertension and the associated end-organ damage.
A dysregulated host response associated with elevated levels of pro-inflammatory cytokines and chemokines is a hallmark of the severity of Francisella infection, progression to sepsis and death (Mares et al., 2008; Sharma et al., 2011; Spencer et al., 2021). Elevated levels of pro-inflammatory cytokines TNF-α, IL-6 and chemokines are the markers of sepsis. It has been demonstrated in previous studies that both young and old mice can mount immune responses of similar magnitude to several intracellular pathogens, which potentially accounts for the lack of differences in susceptibility to infections as a function of old age (Emmerling et al., 1979; Turner et al., 2002; Ehrchen et al., 2004). It has been reported that the magnitude of the pro-inflammatory cytokine response is of a lower magnitude in old mice infected with F. tularensis LVS than in young mice (Mares et al., 2010a). This was proposed to be due to the early clearance of bacteria by the old mice. Since we observed a higher bacterial load and severe histopathological lesions in F. tularensis LVS-infected Hap-I old mice, we investigated whether these differences were due to an altered immune environment in the lungs of Hap-I hypertensive old mice.
The RNA sequencing analysis of the lung tissues of Hap-I old mice revealed the differential expression of several pro-inflammatory cytokine genes. The Tnf-α, Il-6, Ifn-γ, and l1-17a were significantly upregulated in the lungs of Hap-I old mice as compared to the Hap-I adult, Hap-II adult, and Hap-II old mice. The upregulated expression of the cytokine genes as well as protein levels are akin to the cytokine storm observed in the later stages of Francisella infection. It has been reported that moribund mice express higher levels of these cytokines resulting from excessive bacterial replication and severe organ damage and correlate with disease severity, sepsis, and mortality. It is probable that inflammaging in Hap-I old mice may further aggravate inflammation and organ damage due to Francisella infection. Upregulation of these inflammatory markers further support our preceding results and establish that hypertension and age, as observed in Hap-I old mice, will increase susceptibility to respiratory tularemia caused by F. tularensis. In addition to these immunological factors, oxidative stress and mitochondrial dysfunction associated with age also contributes to age-related hypertension (Griendling et al., 2021). Necroptosis caused by Francisella results in severe mitochondrial damage, increased mitochondrial oncosis, and oxidative stress, leading to the leakage of mitochondrial contents in the lungs of infected mice. The recognition of released mitochondrial contents results in elevated levels of pro-inflammatory cytokines, contributing to sepsis-like conditions observed during Francisella infection (Singh et al., 2017). The results from this study on day three post-infection demonstrate that despite significant, but small differences in bacterial loads between Hap-I adult and old mice in lungs, the cytokine levels remain more or less the same. These data support the notion that changes in pro-inflammatory cytokine levels in Hap-I old mice are not on account of the differences in the bacterial load observed on day five post-infection, but probably are caused by the immunoregulatory role of the AT1R gene. On the other hand, lower levels of these cytokines and chemokines in Hap-I adult, Hap-II adult, and old mice indicate that hypertensive phenotype or old age alone can tip the balance of this pro-inflammatory response to a beneficial side leading to bacterial clearance and enhanced survival.
Multiple studies have shown the activation of AT1R signaling in the process of aging (Benigni et al., 2009; Capettini et al., 2012; Harvey et al., 2016). Human AT1R expression significantly increases in Hap-I mice with age. Findings from current studies show that F. tularensis LVS infection results in a substantial increase in human AT1R expression in old Hap-I subjects as compared to their old Hap-II counterparts. However, the mouse At1r does not show a significant age-dependent shift post-F. tularensis LVS infection. Overexpression of hAT1R, in turn, significantly activates RAS signaling in Hap-I animals, thereby assisting an infection-associated phenotype. ACE directly influences various aspects of the immune response and is a chief regulator of the RAS pathway and cleaves angiotensin I into the vasoconstrictor angiotensin II. It has been shown that an ACE inhibitor reduces the bactericidal activity of human neutrophils in vitro and impairs mouse neutrophil activity in vivo (Cao et al., 2021). Besides regulatory molecules, a large number of chemokines also play a major role in hypertension and associated organ damage (Rudemiller and Crowley, 2017). CCL2 is a major chemokine that guides phagocyte infiltration to the sites of inflammation via chemokine receptor ligation (Fuentes et al., 1995; Johnston et al., 1999; Charo and Ransohoff, 2006). Additionally, the circulating levels of CCL2 are upregulated in patients with hypertension and correlate with the degree of hypertension-associated organ damage in humans (Tucci et al., 2006). The higher expression of Ace and Ccl2 in F. tularensis LVS-infected old Hap-I mice from our study (Figures 5C1,C2) further supports these findings.
Recent studies have revealed global and tissue-specific transcriptomic alterations related to aging and infections (Lee et al., 2002; Rodwell et al., 2004; Alfego et al., 2018; Suresh et al., 2021). However, these studies do not provide molecular insight into hypertension. Therefore, by exploiting hAT1R overexpressing TG animals, it is possible to investigate further the underlying mechanisms and essential molecules associated with hypertension and vulnerability to infection. Some of these age and infection-related molecules may be involved in our haplotype-specific regulation of canonical pathways involved in blood pressure regulation and inflammation. Previous studies from our group have shown that the hAT1R promoter interacts with some trans-regulatory elements, including USF2 and STAT3. Stronger binding of these transcription factors to the hAT1R promoter in Hap-I leads to higher expression of the hAT1R gene and, thus, upregulation of the RAS pathway. Earlier, we have shown a diet and age-dependent upregulation of Usf and Stat3, which is associated with an increase in hAT1R expression and increased blood pressure in Hap-I animals (Jain et al., 2018a,b). This study shows a significant increase in the expression of Usf and Stat3 transcription factors post-F. tularensis LVS infection in the lungs of Hap-I TG mice. A rise in these transcription factors suggests that the Hap-I mice would have been experiencing high BP due to the upregulation of hAT1R gene expression and RAS pathway, thus resulting in increased mortality following F. tularensis LVS infection.
Our results demonstrate an additive effect of aging and infection on inflammation and organ damage in a RAS-upregulated, chronically hypertensive mouse model. In the lungs, the Ang II-mediated classic RAS pathway regulates cell proliferation, hypoxia, angiogenesis, and several immune inflammatory responses, contributing to lung injury and pulmonary diseases, including those observed in the recent COVID-19 infection. These effects are exacerbated with aging (Monteonofrio et al., 2021). RAS inhibitors, primarily ACE and AT1R blockers, are critical in attenuating inflammation, vasoconstriction, and oxidative stress by reducing the availability and activity of vasoconstrictor Ang II. Previous studies suggest both beneficial and adverse effects of different RAS inhibitors on morbidity, mortality, and disease severity in subjects treated for infection (Tsampasian et al., 2022). As summarized in Figure 7, our results demonstrate that respiratory infection with F. tularensis LVS in old, hypertensive Hap-I mice results in upregulated expression of the hypertension-associated RAS pathway components, mediated by the hAT1R gene. Furthermore, upregulated expression of genes in the IFN-γ pathway via the gamma interferon activation site elements may contribute to the hyperinflammatory response and severe tularemia in old, hypertensive mice. The activation of these pathways eventually results in a hyperinflammatory response associated with enhanced organ damage and death of F. tularensis-infected old Hap-I hypertensive mice. Transcriptomic data from the lungs of old hypertensive animals provide us with novel gene targets associated with RAS and IFN-γ pathways to further explore the interaction of signaling mechanisms involved in aged hypertensive individuals in response to infection with other infections.
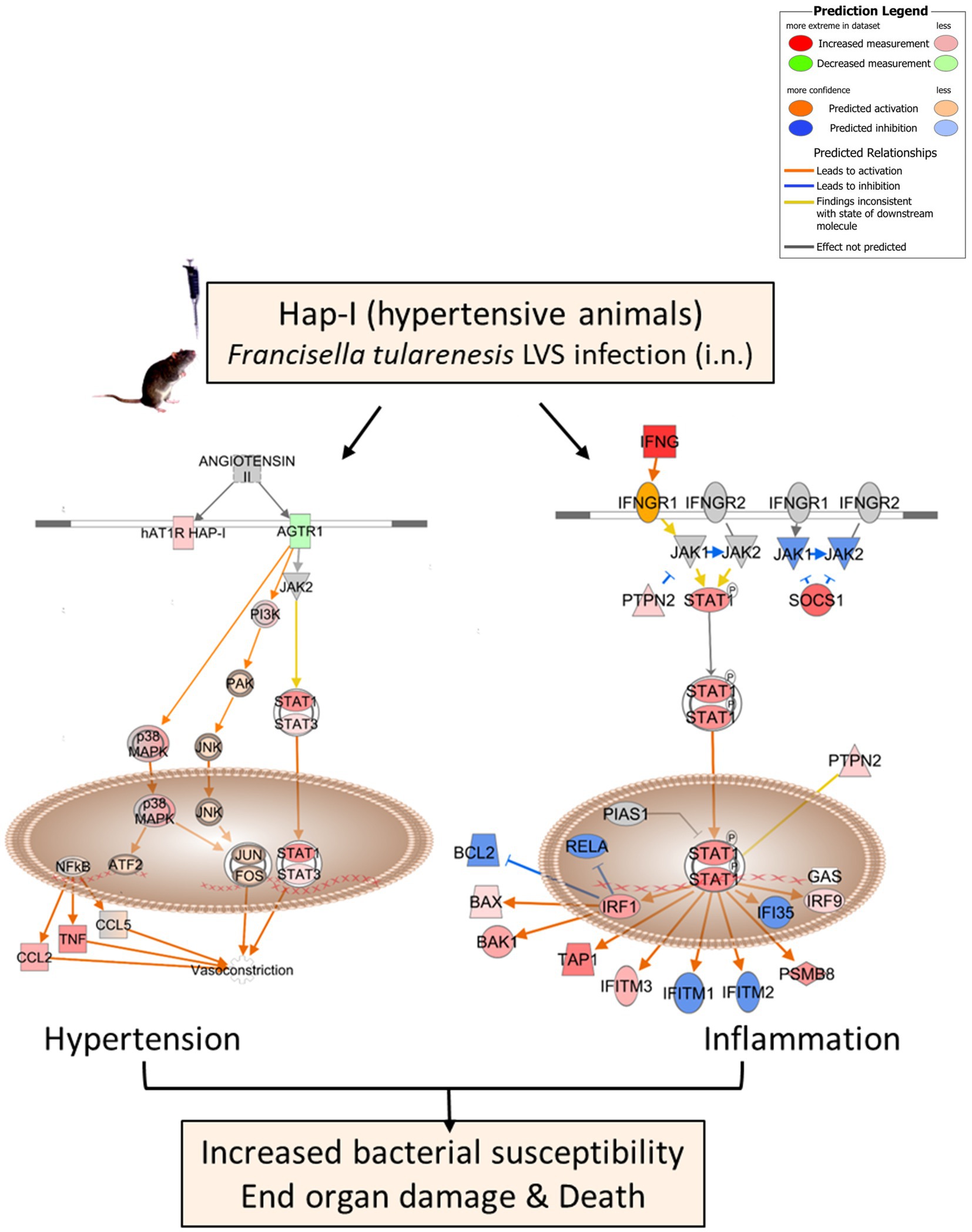
Figure 7. Potential mechanisms leading to increased susceptibility and end-organ damage in old Hap-I TG animals post-F. tularensis LVS infection. A schematic representation depicting upregulated expression of hypertension associated RAS and IFN-γ pathway mediated by overexpression of hAT1R gene in Hap-I hypertensive mice. The model was generated from the gene expression data obtained by RNA-sequencing utilizing IPA bioinformatic software (Qiagen).
Conclusion
Our results show that compared to the normotensive Hap-II mice expressing human AT1R, the hypertensive, transgenic Hap-I mice exhibit enhanced susceptibility to F. tularensis infection. This study demonstrates that severe organ damage and mortality are associated with aging in Hap-I mice due to an environment favoring the upregulation of components of the RAS signaling pathway following acute infection with F. tularensis LVS. Collectively, these findings pave the way for further studies investigating the association between age, hypertension, and other infections using a unique transgenic mouse model of chronic hypertension.
The impact of key components of the RAS pathway on proinflammatory cytokine signaling and the outcome of a bacterial infection is not well established. Our study, for the first time, addresses the association of the RAS pathway with hypertension, aging, and increased susceptibility to respiratory tularemia. Whether such an association is unique to F. tularensis infection or whether a similar response will be observed in our old, hypertensive Hap-I mouse model with other pathogens will remain the focus of our future investigations. It would also be of interest to evaluate the effect of various inhibitors on RAS signaling, immune response, and organ impairment in our mouse model in response to infection with other microbial pathogens.
Data availability statement
The data presented in the study are deposited in the GEO database under accession number GSE186016.
Ethics statement
Ethical review and approval was not required for the animal study because all animal procedures were conducted in accordance with the Institutional Animal Care and Use Committee (IACUC) guidelines approved by New York Medical College.
Author contributions
HK, MA, ST, and SP carried out the experiments. HK, SJ, and CB analyzed the data. SJ and CB conceived and coordinated the research and drafted the manuscript. All authors contributed to the article and approved the submitted version.
Funding
This research was funded by National Institutes of Health grant R01HL146628 to SJ.
Conflict of interest
The authors declare that the research was conducted in the absence of any commercial or financial relationships that could be construed as a potential conflict of interest.
Publisher’s note
All claims expressed in this article are solely those of the authors and do not necessarily represent those of their affiliated organizations, or those of the publisher, the editors and the reviewers. Any product that may be evaluated in this article, or claim that may be made by its manufacturer, is not guaranteed or endorsed by the publisher.
Supplementary material
The Supplementary material for this article can be found online at: https://www.frontiersin.org/articles/10.3389/fmicb.2023.1173577/full#supplementary-material
References
Alexander, M. R., Norlander, A. E., Elijovich, F., Atreya, R. V., Gaye, A., Gnecco, J. S., et al. (2019). Human monocyte transcriptional profiling identifies IL-18 receptor accessory protein and lactoferrin as novel immune targets in hypertension. Br. J. Pharmacol. 176, 2015–2027. doi: 10.1111/bph.14364
Alfego, D., Rodeck, U., and Kriete, A. (2018). Global mapping of transcription factor motifs in human aging. PLoS One 13:e0190457. doi: 10.1371/journal.pone.0190457
Alqahtani, M., Ma, Z., Ketkar, H., Suresh, R. V., Malik, M., and Bakshi, C. S. (2018). Characterization of a unique outer membrane protein required for oxidative stress resistance and virulence of Francisella tularensis. J. Bacteriol. 200:e00693-17. doi: 10.1128/JB.00693-17
Altman, G. B., and Wachs, J. E. (2002). Tularemia. AAOHN J. 50, 373–379. doi: 10.1177/216507990205000810
Arnold, C. R., Wolf, J., Brunner, S., Herndler-Brandstetter, D., and Grubeck-Loebenstein, B. (2011). Gain and loss of T cell subsets in old age – age-related reshaping of the T cell repertoire. J. Clin. Immunol. 31, 137–146. doi: 10.1007/s10875-010-9499-x
Beli, E., Clinthorne, J. F., Duriancik, D. M., Hwang, I., Kim, S., and Gardner, E. M. (2011). Natural killer cell function is altered during the primary response of aged mice to influenza infection. Mech. Ageing Dev. 132, 503–510. doi: 10.1016/j.mad.2011.08.005
Benigni, A., Corna, D., Zoja, C., Sonzogni, A., Latini, R., Salio, M., et al. (2009). Disruption of the Ang II type 1 receptor promotes longevity in mice. J. Clin. Investig. 119, 524–530. doi: 10.1172/JCI36703
Bishop, A., Wang, H.-H., Donaldson, T. G., Brockinton, E. E., Kothapalli, E., Clark, S., et al. (2023). Tularemia cases increase in the USA from 2011 through 2019. Curr. Res. Parasitol. Vector-Borne Dis. 3:100116. doi: 10.1016/j.crpvbd.2023.100116
Boe, D. M., Boule, L. A., and Kovacs, E. J. (2017). Innate immune responses in the ageing lung. Clin. Exp. Immunol. 187, 16–25. doi: 10.1111/cei.12881
Bray, N. L., Pimentel, H., Melsted, P., and Pachter, L. (2016). Near-optimal probabilistic RNA-seq quantification. Nat. Biotechnol. 34, 525–527. doi: 10.1038/nbt.3519
Butcher, S. K., Chahal, H., Nayak, L., Sinclair, A., Henriquez, N. V., Sapey, E., et al. (2001). Senescence in innate immune responses: reduced neutrophil phagocytic capacity and CD16 expression in elderly humans. J. Leukoc. Biol. 70, 881–886. doi: 10.1189/jlb.70.6.881
Cao, D. Y., Giani, J. F., Veiras, L. C., Bernstein, E. A., Okwan-Duodu, D., Ahmed, F., et al. (2021). An ACE inhibitor reduces bactericidal activity of human neutrophils in vitro and impairs mouse neutrophil activity in vivo. Sci. Transl. Med. 13:eabj2138. doi: 10.1126/scitranslmed.abj2138
Capettini, L. S. A., Montecucco, F., Mach, F., Stergiopulos, N., Santos, R. A., and Da Silva, R. F. (2012). Role of renin-angiotensin system in inflammation, immunity and aging. Curr. Pharm Des. 18, 963–970. doi: 10.2174/138161212799436593
Chan, C. T., Sobey, C. G., Lieu, M., Ferens, D., Kett, M. M., Diep, H., et al. (2015). Obligatory role for B cells in the development of angiotensin II-dependent hypertension. Hypertension 66, 1023–1033. doi: 10.1161/HYPERTENSIONAHA.115.05779
Charo, I. F., and Ransohoff, R. M. (2006). The many roles of chemokines and chemokine receptors in inflammation. N. Engl. J. Med. 354, 610–621. doi: 10.1056/nejmra052723
Chatta, G. S., Price, T. H., Stratton, J. R., and Dale, D. C. (1994). Aging and marrow neutrophil reserves. J. Am. Geriatr. Soc. 42, 77–81. doi: 10.1111/j.1532-5415.1994.tb06077.x
Cheng, J., Ke, Q., Jin, Z., Wang, H., Kocher, O., Morgan, J. P., et al. (2009). Cytomegalovirus infection causes an increase of arterial blood pressure. PLoS Pathog. 5, –e1000427. doi: 10.1371/journal.ppat.1000427
Cohen, H. J., Harris, T., and Pieper, C. F. (2003). Coagulation and activation of inflammatory pathways in the development of functional decline and mortality in the elderly. Am. J. Med. 114, 180–187. doi: 10.1016/S0002-9343(02)01484-5
Cool, C. D., Rai, P. R., Yeager, M. E., Hernandez-Saavedra, D., Serls, A. E., Bull, T. M., et al. (2003). Expression of human herpesvirus 8 in primary pulmonary hypertension. N. Engl. J. Med. 349, 1113–1122. doi: 10.1056/nejmoa035115
Crowley, S. D., Song, Y. S., Lin, E. E., Griffiths, R., Kim, H. S., and Ruiz, P. (2010). Lymphocyte responses exacerbate angiotensin II-dependent hypertension. Am. J. Physiol. Regul. Integr. Comp. Physiol. 298, R1089–R1097. doi: 10.1152/ajpregu.00373.2009
De Ciuceis, C., Amiri, F., Brassard, P., Endemann, D. H., Touyz, R. M., and Schiffrin, E. L. (2005). Reduced vascular remodeling, endothelial dysfunction, and oxidative stress in resistance arteries of angiotensin II-infused macrophage colony-stimulating factor-deficient mice: evidence for a role in inflammation in angiotensin-induced vascular injury. Arterioscler. Thromb. Vasc. Biol. 25, 2106–2113. doi: 10.1161/01.ATV.0000181743.28028.57
Desvarieux, M., Demmer, R. T., Jacobs, D. R. Jr., Rundek, T., Boden-Albala, B., Sacco, R. L., et al. (2010). Periodontal bacteria and hypertension: the oral infections and vascular disease epidemiology study (INVEST). J. Hypertens. 28, 1413–1421. doi: 10.1097/HJH.0b013e328338cd36
Ehrchen, J., Sindrilaru, A., Grabbe, S., Schönlau, F., Schlesiger, C., Sorg, C., et al. (2004). Senescent BALB/c mice are able to develop resistance to Leishmania major infection. Infect. Immun. 72, 5106–5114. doi: 10.1128/IAI.72.9.5106-5114.2004
Ellis, J., Oyston, P. C. F., Green, M., and Titball, R. W. (2002). Tularemia. Clin. Microbiol. Rev. 15, 631–646. doi: 10.1128/CMR.15.4.631
Emmerling, P., Hof, H., and Finger, H. (1979). Age-related defense against infection with intracellular pathogens. Gerontology 25, 327–336. doi: 10.1159/000212361
Etyang, A. O., Kapesa, S., Odipo, E., Bauni, E., Kyobutungi, C., Abdalla, M., et al. (2019). Effect of previous exposure to malaria on blood pressure in Kilifi, Kenya: a Mendelian randomization study. J. Am. Heart Assoc. 8, –e011771. doi: 10.1161/JAHA.118.011771
Fahme, S. A., Bloomfield, G. S., and Peck, R. (2018). Hypertension in HIV-infected adults: novel pathophysiologic mechanisms. Hypertension 72, 44–55. doi: 10.1161/HYPERTENSIONAHA.118.10893
Forrester, S. J., Booz, G. W., Sigmund, C. D., Coffman, T. M., Kawai, T., Rizzo, V., et al. (2018). Angiotensin II signal transduction: an update on mechanisms of physiology and pathophysiology. Physiol. Rev. 98, 1627–1738. doi: 10.1152/physrev.00038.2017
Fuentes, M. E., Durham, S. K., Swerdel, M. R., Lewin, A. C., Barton, D. S., Megill, J. R., et al. (1995). Controlled recruitment of monocytes and macrophages to specific organs through transgenic expression of monocyte chemoattractant protein-1. J. Immunol. 155, 5769–5776. doi: 10.4049/jimmunol.155.12.5769
Gomez, C. R., Hirano, S., Cutro, B. T., Birjandi, S., Baila, H., Nomellini, V., et al. (2007). Advanced age exacerbates the pulmonary inflammatory response after lipopolysaccharide exposure. Crit. Care Med. 35, 246–251. doi: 10.1097/01.CCM.0000251639.05135.E0
Griendling, K. K., Camargo, L. L., Rios, F. J., Alves-Lopes, R., Montezano, A. C., and Touyz, R. M. (2021). Oxidative stress and hypertension. Circ. Res. 128, 993–1020. doi: 10.1161/CIRCRESAHA.121.318063
Guzik, T. J., Hoch, N. E., Brown, K. A., McCann, L. A., Rahman, A., Dikalov, S., et al. (2007). Role of the T cell in the genesis of angiotensin II-induced hypertension and vascular dysfunction. J. Exp. Med. 204, 2449–2460. doi: 10.1084/jem.20070657
Harrison, D. G., Guzik, T. J., Lob, H. E., Madhur, M. S., Marvar, P. J., Thabet, S. R., et al. (2011). Inflammation, immunity, and hypertension. Hypertension 57, 132–140. doi: 10.1161/HYPERTENSIONAHA.110.163576
Harvey, A., Montezano, A. C., Lopes, R. A., Rios, F., and Touyz, R. M. (2016). Vascular fibrosis in aging and hypertension: molecular mechanisms and clinical implications. Can. J. Cardiol. 32, 659–668. doi: 10.1016/j.cjca.2016.02.070
Hearps, A. C., Martin, G. E., Angelovich, T. A., Cheng, W. J., Maisa, A., Landay, A. L., et al. (2012). Aging is associated with chronic innate immune activation and dysregulation of monocyte phenotype and function. Aging Cell 11, 867–875. doi: 10.1111/j.1474-9726.2012.00851.x
Jain, S., Prater, A., Pandey, V., Rana, A., Puri, N., and Kumar, A. (2013). A haplotype of angiotensin receptor type 1 associated with human hypertension increases blood pressure in transgenic mice. J. Biol. Chem. 288, 37048–37056. doi: 10.1074/jbc.M113.520023
Jain, S., Puri, N., Rana, A., Sirianni, N., Mopidevi, B., and Kumar, A. (2018a). Metabolic syndrome induces over expression of the human AT1R: A haplotype-dependent effect with implications on cardio-renal function. Am. J. Hypertens. 31, 495–503. doi: 10.1093/ajh/hpx176
Jain, S., Rana, A., Jain, K., Perla, S. K., Puri, N., and Kumar, A. (2018b). Age-related expression of human AT1R variants and associated renal dysfunction in transgenic mice. Am. J. Hypertens. 31, 1234–1242. doi: 10.1093/ajh/hpy121
Jing, Y., Shaheen, E., Drake, R. R., Chen, N., Gravenstein, S., and Deng, Y. (2009). Aging is associated with a numerical and functional decline in plasmacytoid dendritic cells, whereas myeloid dendritic cells are relatively unaltered in human peripheral blood. Hum. Immunol. 70, 777–784. doi: 10.1016/j.humimm.2009.07.005
Johnston, B., Burns, A. R., Suematsu, M., Issekutz, T. B., Woodman, R. C., and Kubes, P. (1999). Chronic inflammation upregulates chemokine receptors and induces neutrophil migration to monocyte chemoattractant protein-1. J. Clin. Investig. 103, 1269–1276. doi: 10.1172/JCI5208
Kirabo, A., Fontana, V., De Faria, A. P. C., Loperena, R., Galindo, C. L., Wu, J., et al. (2014). DC isoketal-modified proteins activate T cells and promote hypertension. J. Clin. Investig. 124, 4642–4656. doi: 10.1172/JCI74084
Kline, K. A., and Bowdish, D. M. E. (2016). Infection in an aging population. Curr. Opin. Microbiol. 29, 63–67. doi: 10.1016/j.mib.2015.11.003
Kogut, I., Scholz, J. L., Cancro, M. P., and Cambier, J. C. (2012). B cell maintenance and function in aging. Semin. Immunol. 24, 342–349. doi: 10.1016/j.smim.2012.04.004
Lee, C. K., Allison, D. B., Brand, J., Weindruch, R., and Prolla, T. A. (2002). Transcriptional profiles associated with aging and middle age-onset caloric restriction in mouse hearts. Proc. Natl. Acad. Sci. U. S. A. 99, 14988–14993. doi: 10.1073/pnas.232308999
Lozovoy, M. A. B., Simão, A. N. C., Morimoto, H. K., Iryioda, T. M. V., Panis, C., Reiche, E. M. V., et al. (2014). Hypertension is associated with serologically active disease in patients with systemic lupus erythematosus: role of increased Th1/Th2 ratio and oxidative stress. Scand. J. Rheumatol. 43, 59–62. doi: 10.3109/03009742.2013.834963
Ma, Z., King, K., Alqahtani, M., Worden, M., Muthuraman, P., Cioffi, C. L., et al. (2019). Stringent response governs the oxidative stress resistance and virulence of Francisella tularensis. PLoS One 14:e0224094. doi: 10.1371/journal.pone.0224094
Madhur, M. S., Lob, H. E., McCann, L. A., Iwakura, Y., Blinder, Y., Guzik, T. J., et al. (2010). Interleukin 17 promotes angiotensin II-induced hypertension and vascular dysfunction. Hypertension 55, 500–507. doi: 10.1161/HYPERTENSIONAHA.109.145094
Mares, C. A., Ojeda, S. S., Li, Q., Morris, E. G., Coalson, J. J., and Teale, J. M. (2010a). Aged mice display an altered pulmonary host response to Francisella tularensis live vaccine strain (LVS) infections. Exp. Gerontol. 45, 91–96. doi: 10.1016/j.exger.2009.10.004
Mares, C. A., Ojeda, S. S., Morris, E. G., Li, Q., and Teale, J. M. (2008). Initial delay in the immune response to Francisella tularensis is followed by hypercytokinemia characteristic of severe sepsis and correlating with upregulation and release of damage-associated molecular patterns. Infect. Immun. 76, 3001–3010. doi: 10.1128/IAI.00215-08
Mares, C. A., Sharma, J., Ojeda, S. S., Li, Q., Campos, J. A., Morris, E. G., et al. (2010b). Attenuated response of aged mice to respiratory Francisella novicida is characterized by reduced cell death and absence of subsequent hypercytokinemia. PLoS One 5, –e14088. doi: 10.1371/journal.pone.0014088
Marghani, D., Ma, Z., Centone, A. J., Huang, W., Malik, M., and Bakshi, C. S. (2021). An AraC/XylS family transcriptional regulator modulates the oxidative stress response of Francisella tularensis. J. Bacteriol. 203:e0018521. doi: 10.1128/JB.00185-21
Mehta, P. K., and Griendling, K. K. (2007). Angiotensin II cell signaling: physiological and pathological effects in the cardiovascular system. Am. J. Physiol. Cell Physiol. 292, C82–C97. doi: 10.1152/ajpcell.00287.2006
Mehta, P., and Hotez, P. J. (2016). NTD and NCD co-morbidities: the example of dengue fever. PLoS Negl. Trop. Dis. 10, –e0004619. doi: 10.1371/journal.pntd.0004619
Mills, K. T., Stefanescu, A., and He, J. (2020). The global epidemiology of hypertension. Nat. Rev. Nephrol. 16, 223–237. doi: 10.1038/s41581-019-0244-2
Mirabito Colafella, K. M., Bovée, D. M., and Danser, A. H. J. (2019). The renin-angiotensin-aldosterone system and its therapeutic targets. Exp. Eye Res. 186:107680. doi: 10.1016/j.exer.2019.05.020
Monteonofrio, L., Florio, M. C., AlGhatrif, M., Lakatta, E. G., and Capogrossi, M. C. (2021). Aging- and gender-related modulation of RAAS: potential implications in COVID-19 disease. Vasc. Biol. 3, R1–R14. doi: 10.1530/VB-20-0014
Moretto, M. M., Lawlor, E. M., and Khan, I. A. (2008). Aging mice exhibit a functional defect in mucosal dendritic cell response against an intracellular pathogen. J. Immunol. 181, 7977–7984. doi: 10.4049/jimmunol.181.11.7977
Mörner, T., Mattsson, R., Forsman, M., Johansson, K.-E., and Sandström, G. (1993). Identification and classification of different isolates of Francisella tularensis. J. Veterinary Med. Ser. B 40, 613–620. doi: 10.1111/j.1439-0450.1993.tb00184.x
Muñoz-Durango, N., Fuentes, C. A., Castillo, A. E., González-Gómez, L. M., Vecchiola, A., Fardella, C. E., et al. (2016). Role of the renin-angiotensin-aldosterone system beyond blood pressure regulation: molecular and cellular mechanisms involved in end-organ damage during arterial hypertension. Int. J. Mol. Sci. 17:797. doi: 10.3390/ijms17070797
Rodwell, G. E. J., Sonu, R., Zahn, J. M., Lund, J., Wilhelmy, J., Wang, L., et al. (2004). A transcriptional profile of aging in the human kidney. PLoS Biol. 2:e427. doi: 10.1371/journal.pbio.0020427
Rudemiller, N. P., and Crowley, S. D. (2017). The role of chemokines in hypertension and consequent target organ damage. Pharmacol. Res. 119, 404–411. doi: 10.1016/j.phrs.2017.02.026
Seidler, S., Zimmermann, H. W., Bartneck, M., Trautwein, C., and Tacke, F. (2010). Age-dependent alterations of monocyte subsets and monocyte-related chemokine pathways in healthy adults. BMC Immunol. 11:30. doi: 10.1186/1471-2172-11-30
Sharma, J., Mares, C. A., Li, Q., Morris, E. G., and Teale, J. M. (2011). Features of sepsis caused by pulmonary infection with Francisella tularensis type A strain. Microb. Pathog. 51, 39–47. doi: 10.1016/j.micpath.2011.03.007
Shaw, A. C., Goldstein, D. R., and Montgomery, R. R. (2013). Age-dependent dysregulation of innate immunity. Nat. Rev. Immunol. 13, 875–887. doi: 10.1038/nri3547
Singh, A., Periasamy, S., Malik, M., Bakshi, C. S., Stephen, L., Ault, J. G., et al. (2017). Necroptotic debris including damaged mitochondria elicits sepsis-like syndrome during late-phase tularemia. Cell Death Discov. 3:17056. doi: 10.1038/cddiscovery.2017.56
Sjöstedt, A. (2007). Tularemia: history, epidemiology, pathogen physiology, and clinical manifestations. Ann. N. Y. Acad. Sci. 1105, 1–29. doi: 10.1196/annals.1409.009
Sotiropoulos, A., Gikas, A., Skourtis, S., Merkouris, P., Pentzeridis, P., Polydorou, A., et al. (2006). Seropositivity to Chlamydia pneumoniae or Helicobacter pylori and coronary artery disease. Int. J. Cardiol. 109, 420–421. doi: 10.1016/j.ijcard.2005.05.039
Spencer, C. T., Ramos Muniz, M. G., Setzu, N. R., and Sanchez Guillen, M. A. (2021). Francisella tularensis infection of mice as a model of sepsis. Methods Mol. Biol. 2321, 75–100. doi: 10.1007/978-1-0716-1488-4_8
Suresh, R. V., Bradley, E. W., Higgs, M., Russo, V. C., Alqahtani, M., Huang, W., et al. (2021). Nlrp3 increases the Host’s susceptibility to tularemia. Front. Microbiol. 12:725572. doi: 10.3389/fmicb.2021.725572
Toussirot, É. (2012). The IL23/Th17 pathway as a therapeutic target in chronic inflammatory diseases. Inflamm. Allergy Drug Targets 11, 159–168. doi: 10.2174/187152812800392805
Tsampasian, V., Corballis, N., and Vassiliou, V.-S. (2022). Renin–Angiotensin–Aldosterone Inhibitors and COVID-19 Infection. Curr. Hypertens Rep. 24, 425–433. doi: 10.1007/s11906-022-01207-3
Tseng, Y.-H., Yang, R.-C., and Lu, T.-S. (2020). Two hits to the renin-angiotensin system may play a key role in severe COVID-19. Kaohsiung J. Med. Sci. 36, 389–392. doi: 10.1002/kjm2.12237
Tucci, M., Quatraro, C., Frassanito, M. A., and Silvestris, F. (2006). Deregulated expression of monocyte chemoattractant protein-1 (MCP-1) in arterial hypertension: role in endothelial inflammation and atheromasia. J. Hypertens. 24, 1307–1318. doi: 10.1097/01.hjh.0000234111.31239.c3
Turner, J., Frank, A. A., and Orme, I. M. (2002). Old mice express a transient early resistance to pulmonary tuberculosis that is mediated by CD8 T cells. Infect. Immun. 70, 4628–4637. doi: 10.1128/IAI.70.8.4628-4637.2002
Vasto, S., Candore, G., Balistreri, C. R., Caruso, M., Colonna-Romano, G., Grimaldi, M. P., et al. (2007). Inflammatory networks in ageing, age-related diseases and longevity. Mech. Ageing Dev. 128, 83–91. doi: 10.1016/j.mad.2006.11.015
Keywords: Francisella, aging, transgenic, hypertension, inflammation, sepsis, cytokine storm, respiratory tularemia
Citation: Ketkar H, Alqahtani M, Tang S, Parambath SP, Bakshi CS and Jain S (2023) Chronically hypertensive transgenic mice expressing human AT1R haplotype-I exhibit increased susceptibility to Francisella tularensis. Front. Microbiol. 14:1173577. doi: 10.3389/fmicb.2023.1173577
Edited by:
Jason F. Huntley, University of Toledo, United StatesReviewed by:
Kenta Watanabe, Yamaguchi University, JapanThomas Robert Laws, Defence Science and Technology Laboratory, United Kingdom
Jerod Skyberg, University of Missouri, United States
Klara Kubelkova, University of Defence, Czechia
Copyright © 2023 Ketkar, Alqahtani, Tang, Parambath, Bakshi and Jain. This is an open-access article distributed under the terms of the Creative Commons Attribution License (CC BY). The use, distribution or reproduction in other forums is permitted, provided the original author(s) and the copyright owner(s) are credited and that the original publication in this journal is cited, in accordance with accepted academic practice. No use, distribution or reproduction is permitted which does not comply with these terms.
*Correspondence: Chandra Shekhar Bakshi, Shekhar_Bakshi@nymc.edu; Sudhir Jain, sjain5@nymc.edu