- Gastrointestinal Microbiology Research Group, Institute of Microbiology, Infectious Diseases and Immunology, Charité – Universitätsmedizin Berlin, Corporate Member of Freie Universität Berlin, Humboldt-Universität zu Berlin, and Berlin Institute of Health, Berlin, Germany
Food-borne Campylobacter jejuni infections constitute serious threats to human health worldwide. Since antibiotic treatment is usually not indicated in infected immune-competent patients, antibiotic-independent treatment approaches are needed to tackle campylobacteriosis. To address this, we orally applied carvacrol, deferoxamine, deoxycholate, and 2-fucosyl-lactose either alone or all in combination to human microbiota-associated IL-10−/− mice from day 2 until day 6 following oral C. jejuni infection. Neither treatment regimen affected C. jejuni loads in the colon, whereas carvacrol lowered the pathogen numbers in the ileum on day 6 post-infection (p.i.). The carvacrol and combination treatment regimens resulted in alleviated diarrheal symptoms, less distinct histopathological and apoptotic epithelial cell responses in the colon, as well as diminished numbers of colonic neutrophils and T lymphocytes on day 6 p.i., whereas the latter cells were also decreased upon deferoxamine, deoxycholate, or 2-fucosyl-lactose application. Remarkably, the carvacrol, deferoxamine, and combination treatment regimens dampened ex-vivo IFN-γ secretion in the colon, the kidneys, and even in the serum to basal concentrations on day 6 p.i. In conclusion, carvacrol alone and its combination with deferoxamine, deoxycholate, and 2-fucosyl-lactose constitute promising antibiotics-independent treatment options to fight acute campylobacteriosis.
1 Introduction
Campylobacteriosis is an acute infectious enteritis, primarily caused by foodborne Campylobacter species transmitted through contaminated chicken and turkey products, with Campylobacter jejuni being the most common pathogen (Price et al., 1979; Walker et al., 1986; Moore et al., 2005; Young et al., 2007; Kaakoush et al., 2015; Wagenaar et al., 2023). Given that the intestinal tract of poultry represents the major pathogen reservoir, the global surge in consumption of poultry products has contributed significantly to the continuous rise of human C. jejuni infections. As a result, campylobacteriosis has emerged as a critical global health problem with a high socioeconomic burden (Kaakoush et al., 2015; WHO, 2020; European Food Safety Authority, European Centre for Disease Prevention and Control, 2022). The asymptomatic colonization of avian intestinal tracts by the highly virulent enteropathogen has far-reaching implications for their transmission to humans. The commensal lifestyle in birds is the major reason for the tolerance of C. jejuni contaminations in poultry production chains and favors its adaptation to the intestines (Moore et al., 2005; Young et al., 2007; Kaakoush et al., 2015). The lack of disease symptoms in birds can be attributed to the avian innate immune system’s non-responsiveness to the pathogen. Additionally, birds display a resistance to lipopolysaccharide (LPS), a cell wall structure of Gram-negative bacteria, including Campylobacter species. C. jejuni produces a truncated version of LPS known as lipo-oligosaccharide (LOS), which further complicates pathogen recognition. Previous studies suggest that these variations in pathogen recognition may arise from species-specific differences in how Campylobacter LOS interacts with the specific Toll-like receptor (TLR)-4 (de Zoete et al., 2010).
In contrast to the avian hosts, humans exhibit heightened sensitivity to TLR-4 ligands such as LPS and LOS of Gram-negative bacteria. Consequently, when C. jejuni infects the human intestinal tract, LOS triggers host immune responses and induces hyperactivation of the innate and adaptive immune system via TLR-4 and mammalian target of rapamycin (mTOR) signaling (Sun et al., 2012; Callahan et al., 2021). This cascade results in damage to intestinal cells such as apoptosis and dissolution of the tight junctions (Lobo de Sá et al., 2021). Subsequently, patients experience acute enterocolitis characterized by bloody diarrhea, intestinal cellular apoptosis, compromised barrier function, malabsorption, and ultimately, severe tissue destruction (Sun et al., 2013; Lobo de Sá et al., 2021).
Although the majority of human campylobacteriosis cases resolve without residues within 1 to 2 weeks post-infection (p.i.), the potential to develop post-infectious autoimmune diseases, including Guillain-Barré syndrome, reactive arthritis, and intestinal diseases, including chronic inflammatory bowel diseases and irritable bowel syndrome remains (Nachamkin et al., 1998; Mortensen et al., 2009; Omarova et al., 2023). Importantly, the risk for these secondary complications correlates with the severity of the initial enteritis (Mortensen et al., 2009). Therefore, severe and invasive C. jejuni induced enterocolitis presenting with bloody diarrhea may require antibiotic therapy, particularly in immunocompromised and multimorbid individuals (Manfredi et al., 1999; Acheson and Allos, 2001). However, the increasing prevalence of antibiotic resistance in Campylobacter strains, including resistance to commonly used quinolones and macrolides, restricts the efficacy of antimicrobial therapy for human campylobacteriosis (Mouftah et al., 2021). Hence, there is an urgent need to develop novel antibiotic-independent strategies to combat and/or prevent C. jejuni infections, aligning with the One-Health approach (Zhang et al., 2023).
To promote global health and address pressing issues like antibiotic resistance and foodborne infections, the One Health concept seeks to balance and improve the health of humans, animals, and ecosystems (Anholt and Barkema, 2021). According to the One Health approach, multi-sectoral (i.e., humans, animals, and the associated environments) and inter-disciplinary strategies (involving farming, human and veterinary medicine for instance) are crucial to combat antibiotic resistance and their collateral damages (Robinson et al., 2016). Therefore, it is important to develop pharmaceutical intervention strategies to treat human campylobacteriosis with antibiotic-independent compounds. Additionally, it is important to identify alternative measures to control antibiotic resistance in both human hosts and animal reservoirs. These actions would enhance safety aspects along the food chains and reduce morbidities in humans caused by transmitted pathogens.
In fact, current research efforts have shifted towards identifying natural compounds with both anti-microbial and anti-inflammatory properties that do not trigger resistance. These compounds hold promise for future therapeutic and preventive applications in the context of enteropathogenic infections, including those caused by Campylobacter species (Kreling et al., 2020).
Carvacrol, a phenolic monoterpenoid and main component in essential oils derived from oregano (Origanum vulgare) and thyme (Thymus vulgaris) (Gholami-Ahangaran et al., 2022), is commonly used for flavoring and food-preservation within the food industry. Furthermore, it is known for its anti-microbial, −oxidant, −diabetic, −inflammatory, and anti-carcinogenic properties, making this compound a promising candidate in the treatment of several human morbidities (Sharifi-Rad et al., 2018; Mączka et al., 2023). The anti-bacterial effects of carvacrol against food-borne pathogens, such as Gram-positive Bacillus cereus or Gram-negative bacteria like Escherichia coli, Salmonella species, and C. jejuni have already been reported in the past (Ultee et al., 2002; Obaidat and Frank, 2009; van Alphen et al., 2012; Mączka et al., 2023). Additionally, our previous pre-clinical intervention studies assessed the anti-inflammatory and immune-modulatory properties of singular carvacrol application during murine campylobacteriosis (Mousavi et al., 2020; Foote et al., 2023).
Deferoxamine B (deferoxamine, trade name Desferal®) is an iron chelator and natural siderophore produced by microorganisms such as Streptomyces pilosus. This compound exhibits anti-microbial, anti-inflammatory, and cell-protective properties (Jurado, 1997; Bellotti and Remelli, 2021). Given that iron is essential for the survival of most pathogens, iron binding by deferoxamine can reduce the risk of infections (Jurado, 1997). Additionally, several studies revealed synergistic effects between deferoxamine and anti-microbial agents such as gentamicin, metronidazole, and hydrogen peroxide (Van Asbeck et al., 1983; van Asbeck et al., 1983; Moon et al., 2011). Previously we reported that the oral application of deferoxamine significantly improved the clinical outcome of C. jejuni infected mice, which was accompanied by dampened inflammatory responses such as less pronounced colonic epithelial cell apoptosis as compared to placebo-treated infected mice (Bereswill et al., 2022).
The secondary bile acid deoxycholic acid has been shown to exhibit bactericidal effects against several bacteria, including Staphylococcus aureus (Zhao et al., 2023) and Helicobacter pylori (Itoh et al., 1999). Previous studies revealed that C. jejuni alter the global gene transcription in response to the bile salt deoxycholate (Gourley et al., 2017; Negretti et al., 2017). Remarkably, deoxycholic acid effectively reduced C. jejuni growth in vitro (Negretti et al., 2017) and its colonization capacity in the intestinal tract of broiler chickens (Alrubaye et al., 2019). Furthermore, deoxycholic acid possesses immune-modulatory activities such as suppressing LPS-induced expression of pro-inflammatory cytokines such as interleukin (IL)-1, IL-6, and tumor necrosis factor-alpha (TNF-α) in murine bone marrow-derived dendritic cells (Hu et al., 2021; Su et al., 2023).
Human breast milk is the main source of energy in the early stage of life. However, the lack of enzymes responsible for the release of monosaccharides makes the human milk oligosaccharides (HMOs) indigestible by the newborn (Engfer et al., 2000; Sela and Mills, 2010). Interestingly, distinct bacteria such as Bifidobacterium and Lactobacillus species use HMOs as nutrients, which indicates the prebiotic properties of HMOs by promoting intestinal colonization by a “healthy” gut microbiota (Davis et al., 2016; Garrido et al., 2016). Additionally, 2-fucosyl-lactose, one of the most abundant oligosaccharides in human breast milk is known for its immune-modulatory effects and has been shown to prevent the binding of pathogens such as Pseudomonas aeruginosa, E. coli, and C. jejuni to the host epithelial cells (Newburg, 2005; Facinelli et al., 2019).
So far, research on C. jejuni-host interactions has been limited by standardized in vivo models. The commensal gut microbiome of conventional laboratory mice exerts a strong physiological colonization resistance, which prevents infections with enteropathogens like C. jejuni (Mullineaux-Sanders et al., 2018; Herzog et al., 2023). Therefore, an antibiotic-pretreatment regimen of mice has been established to generate proper enteropathogenic infection models. After depleting the murine microbiome, a stable colonization of C. jejuni through oral gavage can be assured (Bereswill et al., 2011). However, even though C. jejuni colonizes in secondary abiotic wildtype mice, characteristic clinical symptoms of acute human campylobacteriosis remain absent (Bereswill et al., 2011). This is due to the resistance against TLR-4 ligands like LPS and LOS, which is 10,000 times stronger in rodents as compared to humans (Mortensen et al., 2009). To overcome this challenge, the murine il10 gene has been knocked out to make the animals more susceptible to C. jejuni LOS (Mansfield et al., 2007). In consequence, secondary abiotic IL-10−/− mice can be colonized by the enteropathogen and also display C. jejuni-induced acute enterocolitis within a week post infection (p.i.) mimicking key features of severe and invasive human campylobacteriosis (Bereswill et al., 2011; Haag et al., 2012). Our previous preclinical placebo-controlled intervention studies using this acute C. jejuni infection and inflammation model provided evidence for anti-pathogenic and immune-modulatory properties of several natural compounds such as carvacrol (Mousavi et al., 2020), deferoxamine (Bereswill et al., 2022), and 2-fucosyl-lactose (Mousavi et al., 2023).
Previous studies also revealed that the host-specific intestinal microbiota impacts the host’s susceptibility to and resistance against distinct enteropathogens including C. jejuni (Bereswill et al., 2011), Campylobacter coli (Heimesaat et al., 2020), and Salmonella enterica (Chung et al., 2012). In order to elucidate the triangular relationship between enteropathogens on one side and the murine immunity and human gut microbiota on the other, the host side, we generated a human gut microbiota associated (hma) mouse model for C. jejuni induced inflammation. Therefore, secondary abiotic IL-10−/− mice were subjected to oral transplantation of a fecal microbiota from healthy human donors (Foote et al., 2023; Shayya et al., 2023). Following oral C. jejuni infection, human gut microbiota associated IL-10−/− mice were shown not only to carry the pathogen in their intestines at high loads but also to present with key symptoms of acute campylobacteriosis like secondary abiotic mice do (Shayya et al., 2023).
This preclinical placebo-controlled intervention study assesses carvacrol, deferoxamine, deoxycholic acid, and 2′-fucosyl-lactose alone and as a combination in hma IL-10−/− mice with acute C. jejuni induced enterocolitis. The following parameters will be addressed: (i) human gut microbiota compositions in recipient mice following engraftment; (ii) gastrointestinal pathogens burdens; (iii) clinical outcome; (iv) intestinal, (v) extra-intestinal, and also (vi) the systemic inflammatory immune responses upon oral infection.
2 Methods
2.1 Ethics
All animal procedures were performed in accordance with protocols approved by the local commission for animal experiments (“Landesamt für Gesundheit und Soziales,” LaGeSo, Berlin; registration number G0104/19). Clinical conditions of the mice were assessed daily following the European animal welfare guidelines (2010/63/EU).
2.2 Secondary abiotic IL-10−/− mice
IL-10−/− C57BL/6j mice were obtained from the Forschungsinstitute für Experimentelle Medizin of the Charité – Universitätsmedizin Berlin, Germany. Animals were bred and kept under a specified pathogen-free environment, housed in autoclaved cages with filter tops within an experimental semi-barrier, and given free access to standard chow (food pellets: ssniff R/M-H, V1534-300, Sniff, Soest, Germany) as well as autoclaved tap water. In all experiments, age- and sex-matched littermates were used. Mice were 3-week-old when subjected to an 8-week antibiotic treatment consisting of ampicillin plus sulbactam (2 g/L plus 1 g/L, respectively; Dr. Friedrich Eberth Arzneimittel, Ursensollen, Germany) ad libitum. To avoid cross-contamination, secondary abiotic mice were handled under aseptic conditions throughout the experiment. After eradication of the murine microbiome as described previously (Heimesaat et al., 2022), and 2 days prior to human fecal microbiota transplantation (FMT), the antibiotic treatment was substituted with autoclaved tap water to ensure proper washout.
2.3 Human fecal microbiota transplantation
Human fecal samples were collected from five healthy donors free of viruses, parasites, as well as enteropathogenic bacteria and stored at −80°C. After thawing and resuspending in sterile phosphate buffered saline (PBS, Thermo Fisher Scientific, Waltham, MA, United States), the samples were pooled to ensure at least 0.3 mL gavage volume per mouse. The microbiota composition of the pooled FMT sample can be found in Supplementary Figure S1. One week before the first C. jejuni infection (respectively on days 0 and 1), secondary abiotic mice were introduced to the complex human FMT on 3 days (respectively on days −7, −6 and −5) (Figure 1) as described previously (Shayya et al., 2023).
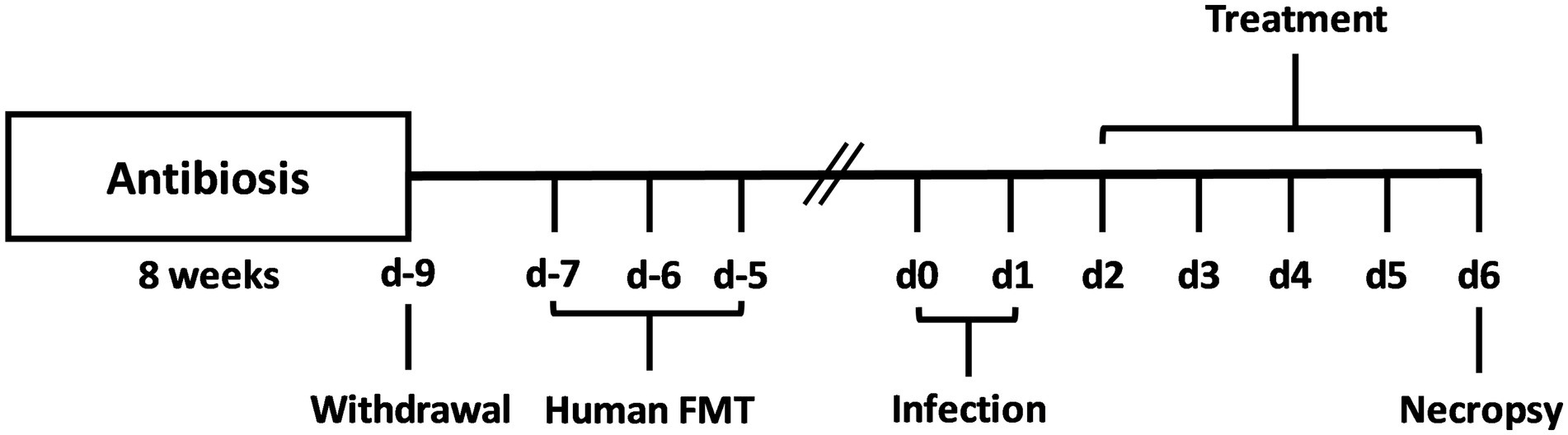
Figure 1. Experimental set-up. For gut microbiota depletion, conventionally raised IL-10−/− mice were subjected to an 8-week pretreatment with ampicillin plus sulbactam. Two days before oral human fecal microbiota transplantation (FMT) of secondary abiotic IL-10−/− mice on day (d)-7, d-6, and d-5, the antibiotic compounds were replaced by sterile tap water. On d0 and d1, human microbiota associated (hma) mice were perorally infected with C. jejuni strain 81–176, and treated with synthetic carvacrol, deferoxamine, deoxycholic acid, and 2-fucosyl-lactose alone, with a combination of all four compounds or with placebo (via the drinking water) from d2 until necropsy on d6 post-infection.
2.4 Campylobacter jejuni cultures and infections
Viable cells of C. jejuni strain 81–176 were thawed from −80°C and grown on Campylobacter-selective Karmali agar plates (Oxoid, Wesel, Germany) under microaerophilic conditions (CampyGen gas packs, Oxoid, Wesel, Germany) at 37°C (Bereswill et al., 2011). After 48 h, plates were harvested and bacterial cells were resuspended in sterile PBS to achieve an inoculum of 109 colony forming units (CFU). Then, 0.3 mL per mouse were gavaged on days 0 and 1 as shown previously (Bereswill et al., 2011).
2.5 Course of treatment
Treatment through oral administration via drinking water of carvacrol, deferoxamine, deoxycholic acid (all from Sigma-Aldrich, Munich, Germany), 2′-fucosyl-lactose (Chr. Hansen HMO GmbH, Rheinbreitbach, Germany), and the quadruple combination commenced 2 days following the onset of C. jejuni infection. Dosages were calculated considering an average body weight of approximately 25 g per mouse and an estimated drinking volume of 5 mL per day. All compounds were dissolved in autoclaved tap water. The daily doses and their previously determined minimal inhibitory concentrations (MICs) are illustrated in Table 1. To enhance the water solubility of carvacrol, 50 mg of the compound were dissolved in 250 μL Tween® 80 (Sigma-Aldrich, Munich, Germany). The placebo group received vehicle dissolved in autoclaved tap water.
2.6 Campylobacter jejuni loads in the gastrointestinal tract
Intraluminal samples from defined parts of the gastrointestinal tract (stomach, duodenum, ileum, and colon) were taken upon necropsy of the mice. The samples were homogenized in PBS, serial diluted and streaked on Karmali agar plates (Oxoid, Wesel, Germany). After 48 h of incubation at 37°C under microaerophilic conditions, C. jejuni was quantified by counting CFU (Bereswill et al., 2011). The detection limit of viable bacterial cells was 100 CFU per g intestinal sample.
2.7 Microbiota analysis
Fecal samples of hma mice were collected before (i.e., day 0) and 6 days after C. jejuni infection for microbiota composition analysis in comparison to the original fecal donor suspensions (Heimesaat et al., 2006). Samples were homogenized in sterile PBS, diluted in series and incubated on solid media under aerobic, microaerobic, and anaerobic conditions for 48 h. Total bacterial loads and bacterial species were identified according to their colony morphology, Gram-staining, and biochemical analysis. In addition, genomic DNA extraction and real-time polymerase chain reaction (PCR) of the fecal samples have been performed culture-independently; this is to assess the abundance of fastidious and non-cultivable bacteria from the human microbiome quantitatively. 16S variable regions were targeted using species-, genera- or group-specific 16S rRNA primers (Tib MolBiol, Berlin, Germany) (Heimesaat et al., 2006). Results are illustrated as 16S rRNA gene copies per ng DNA.
2.8 Clinical outcomes
Health outcome of mice was monitored before and for 6 days after C. jejuni infection. Total clinical score (maximum 12 points) constituted of clinical aspect (i.e., wasting symptoms; 0: normal; 1: ruffled fur; 2: less locomotion; 3: isolation; 4: severely compromised locomotion, pre-final aspect), fecal blood (0: none; 2: microscopic detection using the Guajac method (Haemoccult, Beckman Coulter/PCD, Germany); 4: visible blood spots), and stool appearance (0: normal/firm; 2: pasty; 4: liquid), as stated previously (Heimesaat et al., 2014).
2.9 Dissection and sampling
Six days p.i., mice were sacrificed by carbon dioxide asphyxiation and dissected in an aseptic environment. Ex vivo tissue samples from liver, kidneys, mesenteric lymph nodes (MLN), colon, in addition to luminal samples (from stomach, duodenum, ileum, and colon) were collected for microbiological, immunological, and immunohistopathological analyses. Heart blood was used for cytokine measurements.
2.10 Histopathological changes
Colonic tissue samples were fixed in 5% formalin and embedded in paraffin. 5-μm-sections were then stained with hematoxylin and eosin (H&E) to evaluate histopathological changes. The colonic mucosa was assessed under light microscopy (100 × magnification) using the following scoring scheme (Erben et al., 2014): Score 0, normal/no inflammatory cell infiltrates in epithelium. Score 1, minimal inflammatory cell infiltrates in mucosa but still intact epithelium. Score 2, mild inflammatory cell infiltrates in mucosa and submucosa with mild hyperplasia and mild goblet cell loss. Score 3, moderate inflammatory cell infiltrates in mucosa with moderate goblet cell loss. Score 4, extensive inflammatory cell infiltration into mucosa and submucosa with marked goblet cell loss, multiple crypt abscesses, and crypt loss.
2.11 In situ immunohistochemistry
5-μm-sections of colonic tissue samples were fixed in 5% formalin and embedded in paraffin as previously described (Heimesaat et al., 2018; Foote et al., 2023). Primary antibodies against cleaved caspase-3 (Asp175, Cell Signaling, Beverly, MA, United States, 1:200), MPO7 (No. A0398, Dako, Glostrup, Denmark, 1:500), CD3 (no. N1580, Dako, 1:10), and FOXP3 (clone FJK-165, no. 14-5773, eBioscience, 1:100) were used to count apoptotic epithelial cells, neutrophils, T lymphocytes, and regulatory T cells under light microscopy. The mean number of detected cells in each blinded sample was determined within at least six high power fields (HPF, 0.287 mm2, 400 × magnification).
2.12 Mouse inflammation cytometric bead assay
Ex vivo tissue samples from colon, liver (both approximately 1 cm3), kidney (one half after the longitudinal cut) were collected and washed in sterile PBS. Samples were incubated at 37°C for 18 h in 24-flat-bottom well culture plates (Thermo Fisher Scientific, Waltham, MA, United States) containing 500 μL serum-free RPMI 1640 medium (Thermo Fisher Scientific, Waltham, MA, United States), penicillin (100 μg/mL; Biochrom, Berlin, Germany) and streptomycin (100 μg/mL; Biochrom, Berlin, Germany). The culture supernatants and serum samples were then tested for interferon-gamma (IFN-γ) and IL-6 by applying the Mouse Inflammation Cytometric Bead Assay (BD Biosciences, Germany) in a BD FACS Canto II flow cytometer (BD Biosciences).
2.13 Statistical analysis and reproducibility
Results from animal experiments are representative of three independent repetitions. Statistical analysis was performed using GraphPad Prism (version 9; San Diego, CA, United States). The Anderson-Darling test was used to normalize data sets. The Student’s t-test and Mann–Whitney test were applied for pairwise comparisons of normally and not normally distributed data. Multiple comparisons were performed using the one-way ANOVA with Tukey post-correction (for normally distributed data) and Kruskal-Wallis test with Dunn’s post-correction (for not normally distributed data). p values of less than 0.05 were considered significant. Outliers were identified by the Grubb’s test.
3 Results
3.1 Fecal microbiota composition in hma IL-10−/− mice immediately before Campylobacter jejuni infection
We first assessed whether the human fecal microbiota transplants had comparably engrafted in the intestinal tract of secondary abiotic mice within 1-week after triple human FMTs. To address this, we performed quantitative gut microbiota analyses immediately before C. jejuni infection. Both, cultural and culture-independent (i.e., molecular) analyses proved that the fecal loads of the tested intestinal bacterial groups, genera, and species were comparably high in the six prospective treatment cohorts of the hma IL-10−/− mice pointing towards similar engraftment of the human fecal transplants in the murine hosts (Supplementary Figure S2; Figure 2).
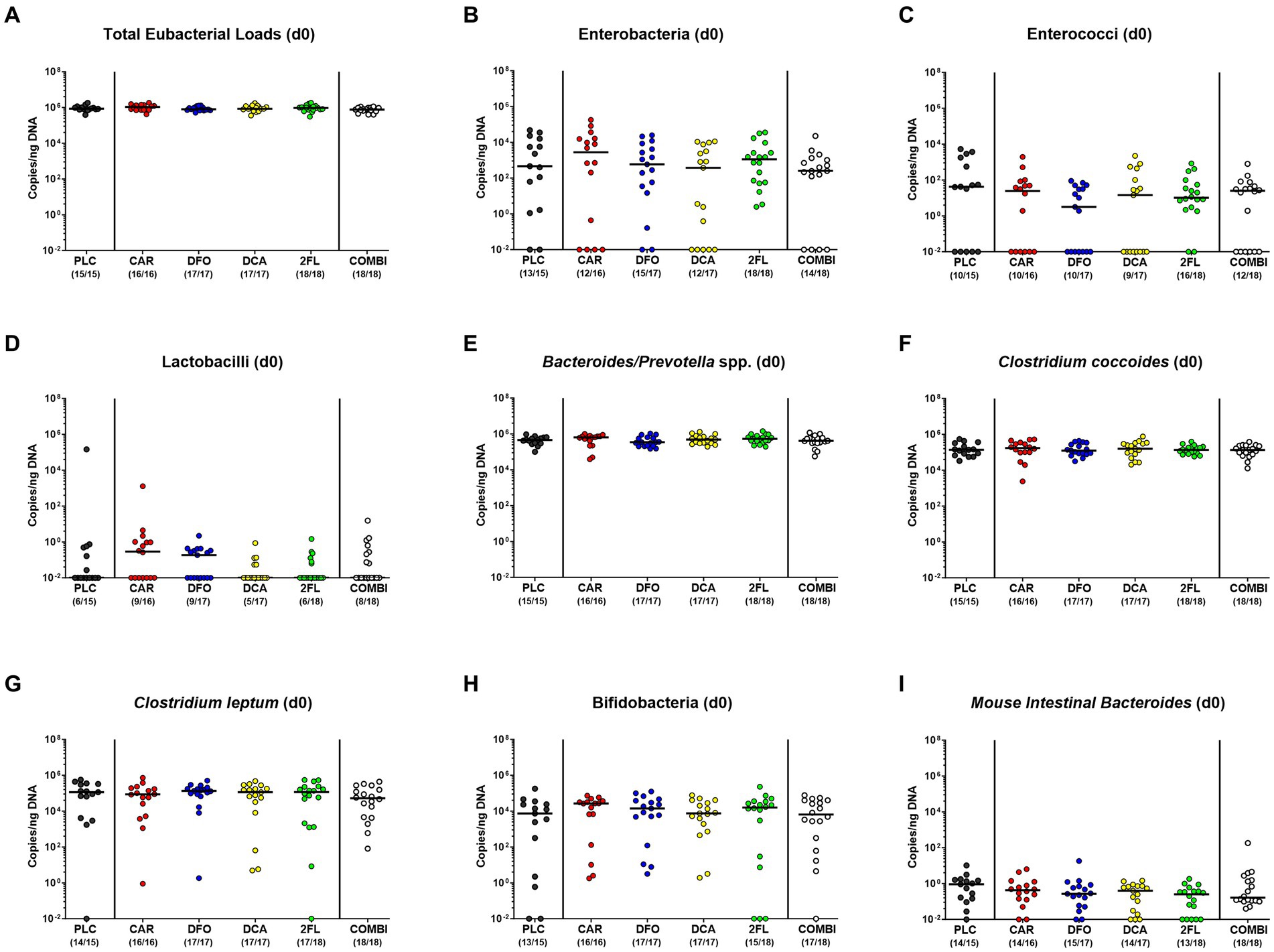
Figure 2. Culture-independent analysis of fecal microbiota composition in human microbiota associated IL-10−/− mice immediately before C. jejuni infection. A week before C. jejuni infection, secondary abiotic IL-10−/− mice (that had been generated by antibiotic pretreatment) were subjected to human fecal microbiota transplantation on three consecutive days by oral gavage [i.e., on day (d)-7, d-6, and d-5]. Immediately before C. jejuni infection on d0, the fecal microbiota composition was surveyed in mice from the prospective treatment cohorts (PLC, placebo; CAR, carvacrol; DFO, deferoxamine; DCA, deoxycholic acid; 2FL, 2-fucosyl-lactose; COMBI, a combination of all four compounds) by culture-independent, 16S rRNA real-time PCR (see methods) assessing the (A) total eubacterial loads, (B) enterobacteria, (C) enterococci, (D) lactobacilli, (E) Bacteroides/Prevotella species (spp.), (F) Clostridium coccoides group, (G) Clostridium leptum group, (H) bifidobacteria, and (I) Mouse Intestinal Bacteroides (expressed as copies per ng DNA). Medians (black bars) and numbers of mice with bacteria-positive detection out of the total number of analyzed animals (in parentheses) are indicated.
3.2 Gastrointestinal Campylobacter jejuni loads following oral infection and treatment of hma IL-10−/− mice with carvacrol, deferoxamine, deoxycholic acid, and 2-fucosyl-lactose alone or in combination
Following the start of oral treatment in C. jejuni infected hma IL-10−/− mice with carvacrol, deferoxamine, deoxycholic acid, and 2-fucosyl-lactose alone or all four in combination on day 2 p.i., we surveyed the C. jejuni pathogen loads in fecal samples over time. Analyses by culture revealed median fecal C. jejuni counts of approximately 109 viable bacteria per gram that did not differ between the six treatment cohorts on days 2, 3, 4, and 5 p.i. [not significant (n.s.); Supplementary Figure S3]. Furthermore, we enumerated viable C. jejuni bacteria in luminal samples taken from defined gastrointestinal parts upon necropsy. Whereas the pathogen counts in the stomach and colon did not differ between the respective groups (n.s.; Figures 3A,D), approximately two orders of magnitude lower pathogen numbers could be cultured from the ileum lumen of carvacrol as compared to placebo treated mice on day 6 p.i. (p < 0.05; Figure 3C). Furthermore, the carvacrol and combination groups exhibited lower C. jejuni burdens in their duodenum as compared to 2-fucosyl-lactose treated mice (p < 0.01; Figure 3B). Therefore, all treatment regimens, whether applied individually or in combination did not affect C. jejuni loads in the colon. It is notable that only the carvacrol treatment lowered pathogen loads in the ileal tracts of infected hma IL-10−/− mice.
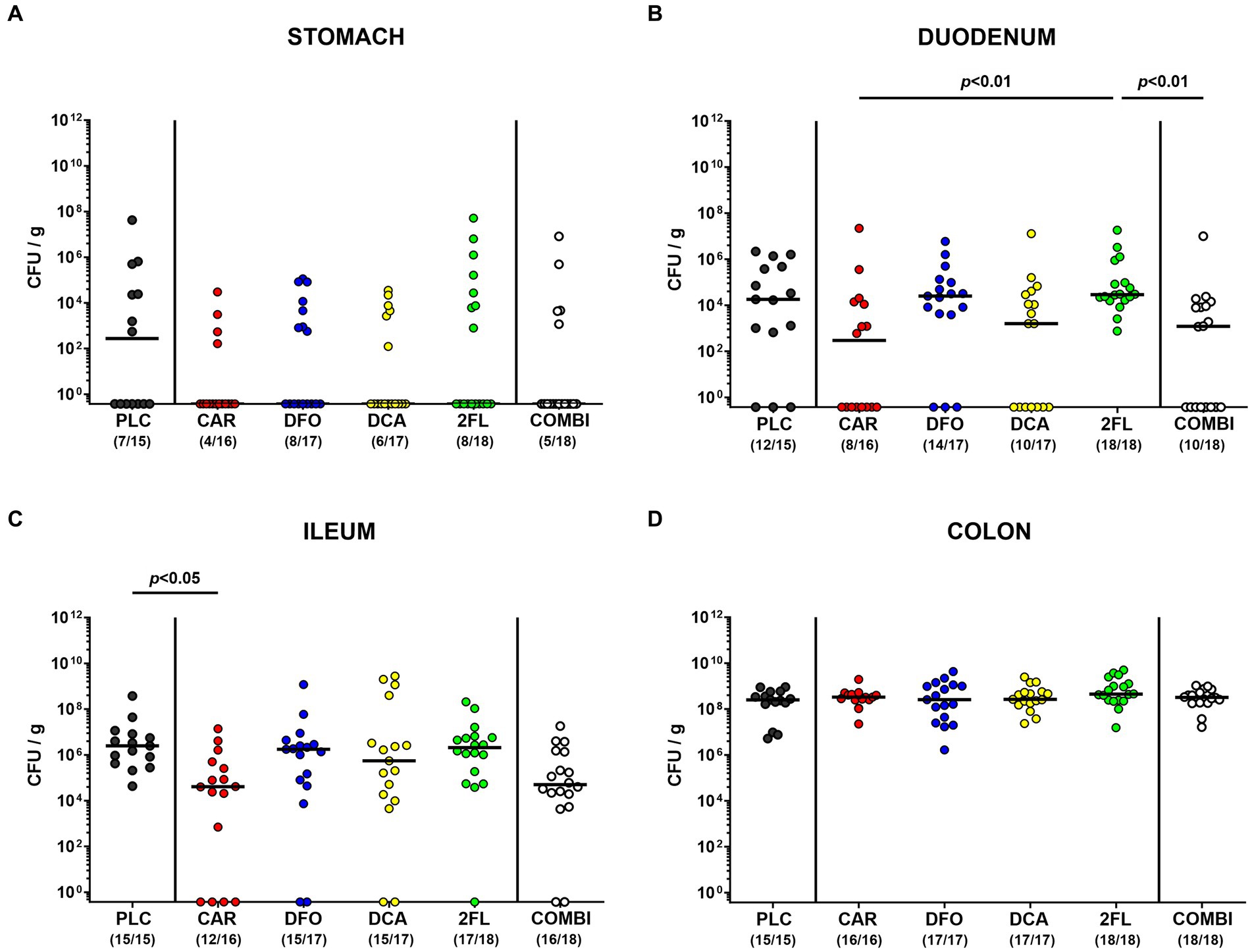
Figure 3. Establishment of C. jejuni in the gastrointestinal tract of infected hma IL-10−/− mice. Hma IL-10−/− mice were orally infected with C. jejuni strain 81–176 on days 0 and 1. From day 2 until day 6 post-infection, mice were treated with synthetic carvacrol (CAR), deferoxamine (DFO), deoxycholic acid (DCA), 2-fucosyl-lactose (2FL), a combination of all four compounds (COMBI) or placebo (PLC) via the drinking water. Upon sacrifice on d6 post-infection, C. jejuni were quantitated in luminal samples taken from the (A) stomach, (B) duodenum, (C) ileum, and (D) colon by culture and expressed as colony-forming units per gram (CFU/g). Individual data pooled from three experiments, medians (black bars), the numbers of culture-positive mice out of the total number of analyzed animals (in parentheses), and the significance levels (p values) determined by the Kruskal-Wallis test and Dunn’s post-correction are shown.
3.3 Clinical outcomes of hma IL-10−/− mice following Campylobacter jejuni infection and treatment with carvacrol, deferoxamine, deoxycholic acid, and 2-fucosyl-lactose alone or in combination
We further surveyed the effect of the applied treatment regimens on C. jejuni induced disease with campylobacteriosis scores (assessing wasting symptoms plus diarrhea plus fecal blood) over time p.i. As early as 24 h after initiation of the treatments (i.e., on day 3 p.i.), increased clinical scores were determined in the deferoxamine, deoxycholic acid, 2-fucosyl-lactose, and placebo treated groups (p < 0.01–0.001 versus naive; Supplementary Figure S4A), whereas carvacrol and combination treated hma mice displayed basal values (n.s. versus naive; Supplementary Figure S4A). This also held true for basal scores in mice from the combination group on days 4 and 5 p.i. (n.s. versus naive; Supplementary Figures S4B,C). Immediately before necropsy on day 6 p.i., mice from all infected cohorts exhibited similarly increased overall campylobacteriosis scores (p < 0.05–0.001 versus naive; Figure 4A), but with a trend towards lower median scores in carvacrol and combination treated mice versus placebo counterparts (n.s. due to relatively high standard deviations; Figure 4A). When specifically quantitating wasting symptoms on day 6 p.i., no differences in wasting scores could be observed between treated mice (p < 0.05–0.001 versus naive; Figure 4B), whereas the scores for fecal blood were increased in all cohorts except the carvacrol treatment group (n.s. versus naive; Figure 4C). Remarkably, carvacrol and combination treated mice suffered from less severe diarrheal symptoms as indicated by lower diarrhea scores if compared to placebo control mice on day 6 p.i. (p < 0.05; Figure 4D). Of note, mice from the carvacrol, deoxycholic acid, 2-fucosyl-lactose, and combination cohorts exhibited basal diarrheal scores at the end of the experiment (n.s. versus naive; Figure 4D). Hence, carvacrol and combination treatment alleviated diarrheal symptoms in C. jejuni infected hma mice.
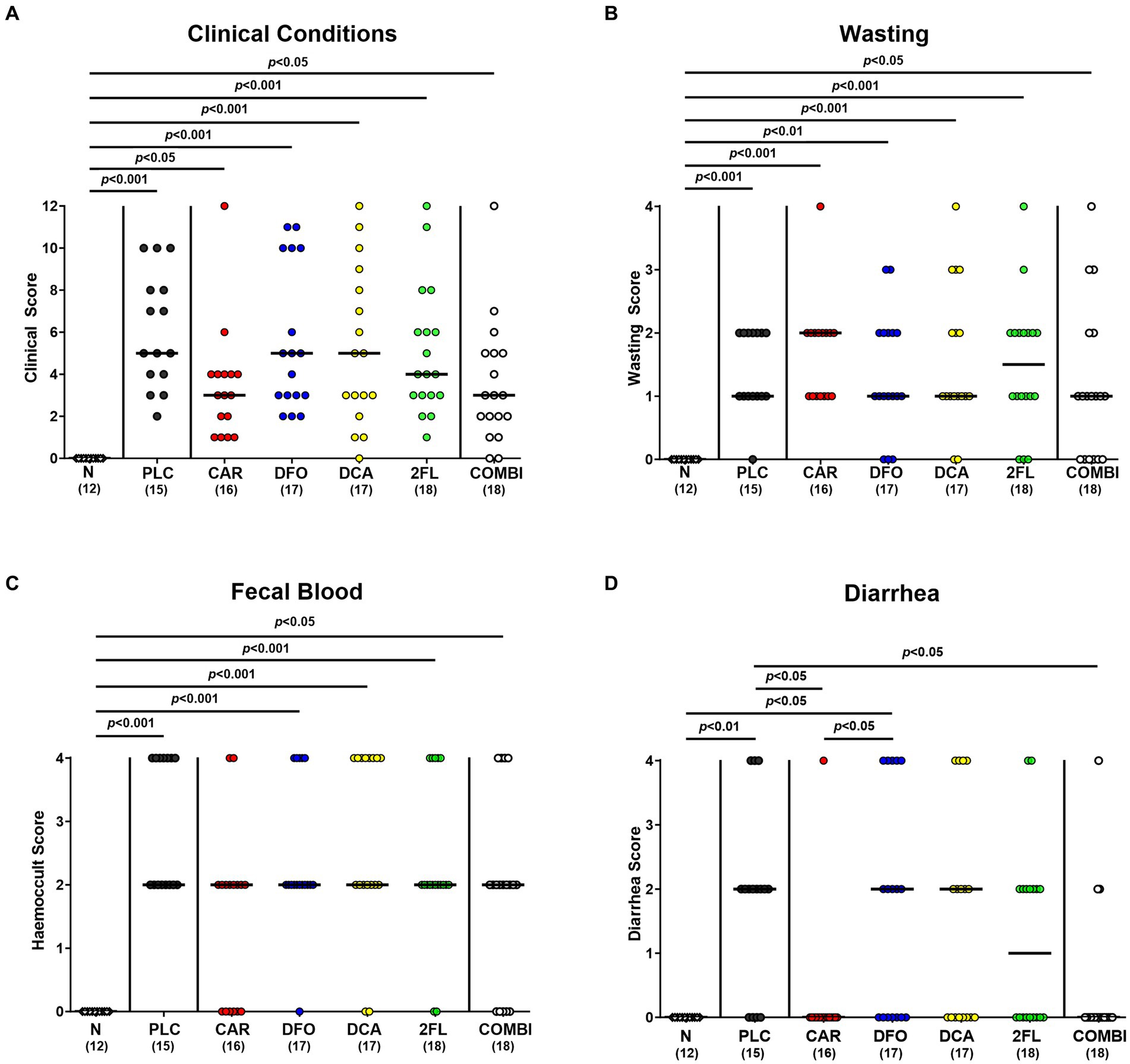
Figure 4. Clinical outcomes in hma IL-10−/− mice following C. jejuni infection and treatment with carvacrol, deferoxamine, deoxycholic acid, and 2-fucosyl-lactose alone or all in combination. C. jejuni infected hma IL-10−/− mice were perorally treated with synthetic carvacrol (CAR), deferoxamine (DFO), deoxycholic acid (DCA), 2-fucosyl-lactose (2FL), a combination of all four compounds (COMBI) or placebo (PLC) from day 2 until day 6 post-infection. At the end of the treatment (i.e., day 6 post-infection), the clinical outcomes were quantitated with clinical scores assessing the (A) overall clinical conditions and specifically, (B) wasting symptoms, (C) diarrhea, and (D) fecal blood. Naive (N) hma IL-10−/− mice served as non-infected and untreated controls. Individual data pooled from three experiments, the medians (black bars), the numbers of included mice (in parentheses), and the significance levels (p values) determined by the Kruskal-Wallis test and Dunn’s post-correction are shown.
3.4 Macroscopic and microscopic inflammatory changes in hma IL-10−/− mice following Campylobacter jejuni infection and treatment with carvacrol, deferoxamine, deoxycholic acid, and 2-fucosyl-lactose alone or in combination
Next, we assessed changes in colonic lengths, as enterocolitis is known to result in significantly shorter intestines due to inflammation (Heimesaat et al., 2006; Bereswill et al., 2011). Irrespective of the treatment, infected mice displayed shorter colons as compared to uninfected control animals (p < 0.05–0.001; Figure 5A). Furthermore, we quantitated the C. jejuni induced histopathological changes in the colon and determined lower histopathological scores in carvacrol and combination treated mice as compared to placebo counterparts on day 6 p.i., indicative for less severe pathogen-induced microscopic disease (p < 0.01 and p < 0.001, respectively; Figure 5B). In addition, the numbers of apoptotic colonic epithelial cells were lower in infected mice from the carvacrol, deferoxamine, 2-fucosyl-lactose, and combination cohorts versus placebo (p < 0.05–0.001; Figure 5C). The combination treatment dampened both, histopathological and apoptotic cell responses in the colon of C. jejuni infected mice to basal levels (n.s. versus naive; Figures 5B,C). Hence, carvacrol and combination treatment of hma IL-10−/− mice resulted in diminished microscopic (i.e., histopathological and apoptotic) sequelae of C. jejuni infection.
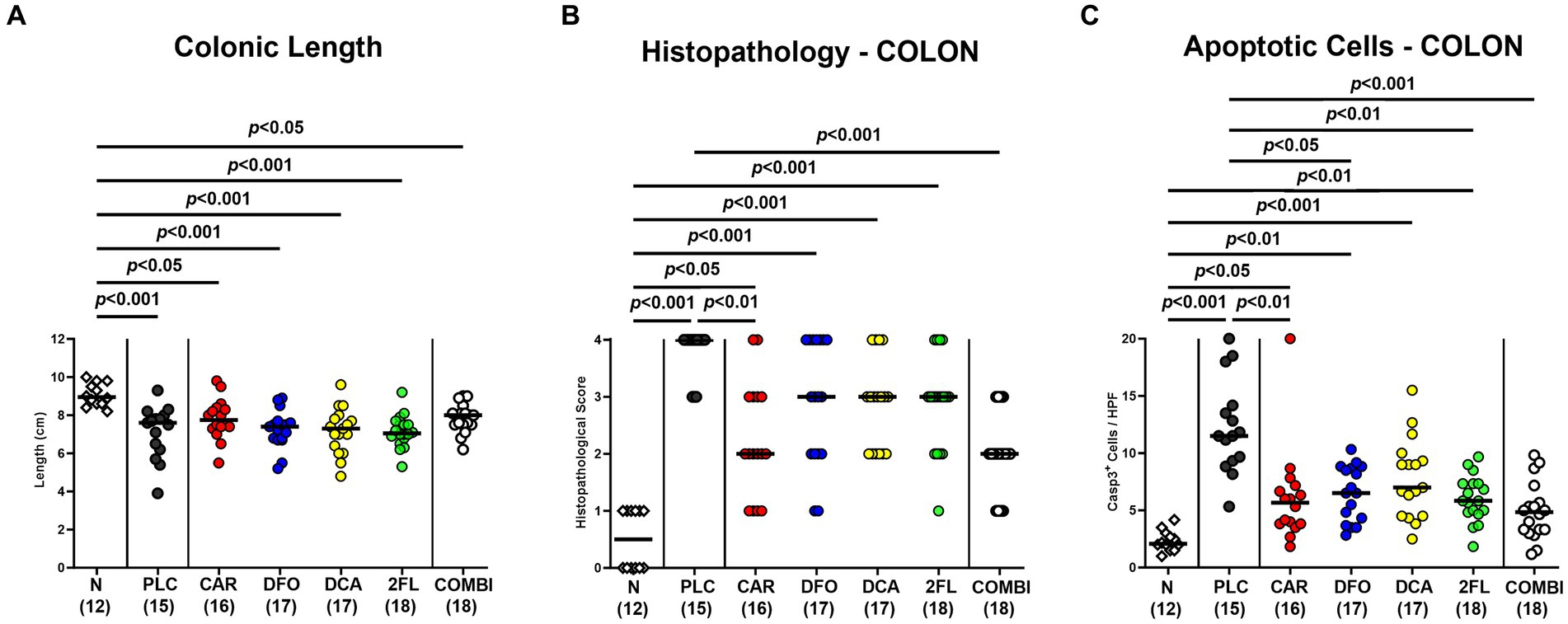
Figure 5. Macroscopic and microscopic inflammatory changes in hma IL-10−/− mice following C. jejuni infection and treatment with carvacrol, deferoxamine, deoxycholic acid, and 2-fucosyl-lactose alone or all in combination. C. jejuni infected hma IL-10−/− mice were perorally treated with synthetic carvacrol (CAR), deferoxamine (DFO), deoxycholic acid (DCA), 2-fucosyl-lactose (2FL), a combination of the four compounds (COMBI) or placebo (PLC) via the drinking water from day 2 until day 6 post-infection. On day 6, the (A) colonic lengths were measured, the (B) histopathological changes scored in hematoxylin and eosin-stained colonic paraffin sections, and the (C) numbers of apoptotic colonic epithelial cells determined in colonic paraffin sections stained with an antibody against cleaved caspase-3 (average numbers out of six representative high-power fields (HPF, 400 × magnification) per mouse). Naive (N) hma IL-10−/− mice served as non-infected and untreated controls. Individual data pooled from three experiments, the medians (black bars), the numbers of included mice (in parentheses), and the significance levels (p values) determined by the one-way ANOVA test with Tukey post-correction (A) and by the Kruskal-Wallis test and Dunn’s post-correction (B,C) are shown.
3.5 Immune cell subsets in the colon of hma IL-10−/− mice following Campylobacter jejuni infection and treatment with carvacrol, deferoxamine, deoxycholic acid, and 2-fucosyl-lactose alone or in combination
To test treatment impact on C. jejuni induced immune responses, we stained colonic paraffin sections with antibodies against surface markers of distinct innate and adaptive immune cells. On day 6 p.i., MPO7+ neutrophilic granulocytes were lower in the colonic mucosa and lamina propria of carvacrol and combination treated mice when compared to placebo counterparts (p < 0.001; Figure 6A). Remarkably, they did not even differ from uninfected controls (n.s. versus naive; Figure 6A). Irrespective of the treatment, C. jejuni infection resulted in colonic increases in adaptive CD3+ T lymphocytes and FOXP3+ regulatory T cells (p < 0.05–0.001 versus naive; Figures 6B,C). T cell numbers were, however, approximately 50% lower in the colon of all verum as compared to the control group on day 6 p.i. (p < 0.001; Figure 6B). Moreover, the combination of all four compounds did not further alleviate colonic T cell responses upon C. jejuni infection. Hence, oral carvacrol and combination treatment could diminish C. jejuni triggered immune responses.
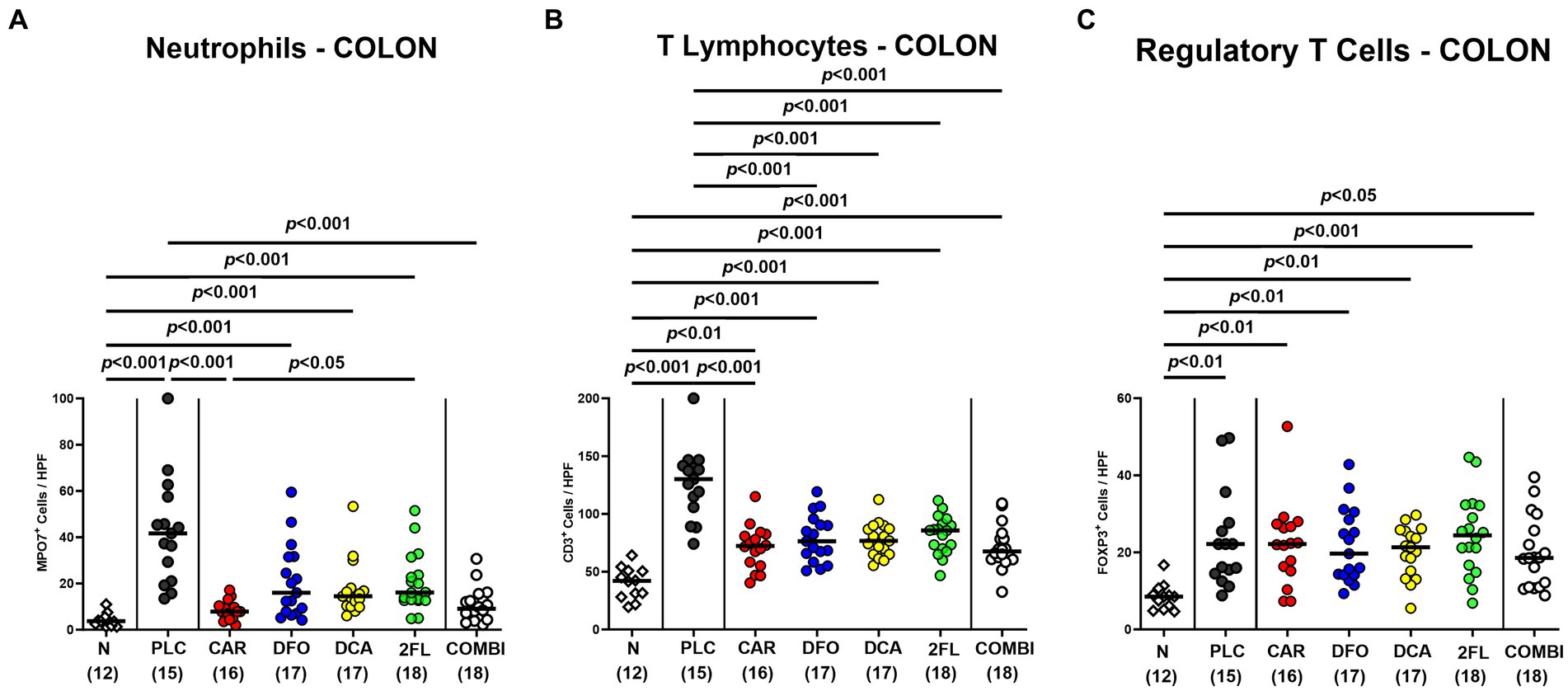
Figure 6. Immune cell subsets in the colon of hma IL-10−/− mice following C. jejuni infection and treatment with carvacrol, deferoxamine, deoxycholic acid, and 2-fucosyl-lactose alone or all in combination. C. jejuni infected hma IL-10−/− mice were perorally treated with synthetic carvacrol (CAR), deferoxamine (DFO), deoxycholic acid (DCA), 2-fucosyl-lactose (2FL), a combination of the four compounds (COMBI) or placebo (PLC) via the drinking water from day 2 until day 6 post-infection. (A) Neutrophils (MPO7+), (B) T lymphocytes (CD3+), and (C) regulatory T cells (FOXP3+) were enumerated in immunohistochemically stained colonic paraffin sections derived on day 6 post-infection (average numbers out of six representative high-power fields (HPF, 400 × magnification) per mouse). Naive (N) hma IL-10−/− mice served as non-infected and untreated controls. Individual data pooled from three experiments, the medians (black bars), the numbers of included mice (in parentheses), and the significance levels (p values) determined by the Kruskal-Wallis test and Dunn’s post-correction (A,C) and the one-way ANOVA test with Tukey post-correction (B) are shown.
3.6 Intestinal and extra-intestinal IFN-γ secretion in hma IL-10−/− mice following Campylobacter jejuni infection and treatment with carvacrol, deferoxamine, deoxycholic acid, and 2-fucosyl-lactose alone or in combination
C. jejuni infection resulted in enhanced IFN-γ secretion in colon and kidneys of placebo, deoxycholic acid and 2-fucosyl-lactose treated mice (p < 0.05–0.001; Figures 7A,B). In comparison, basal colonic and renal IFN-γ concentrations were measured in the carvacrol, deferoxamine, and combination treatment cohorts on day 6 p.i. (n.s. versus naive Figures 7A,B). This was also the case when determining IFN-γ levels in the liver of infected mice following carvacrol and combination treatment (n.s. versus naive Figure 7C). Hence, carvacrol and the combination treatment of hma IL-10−/− mice dampens C. jejuni induced IFN-γ responses in colon and extra-intestinal compartments, as observed in kidneys and liver.
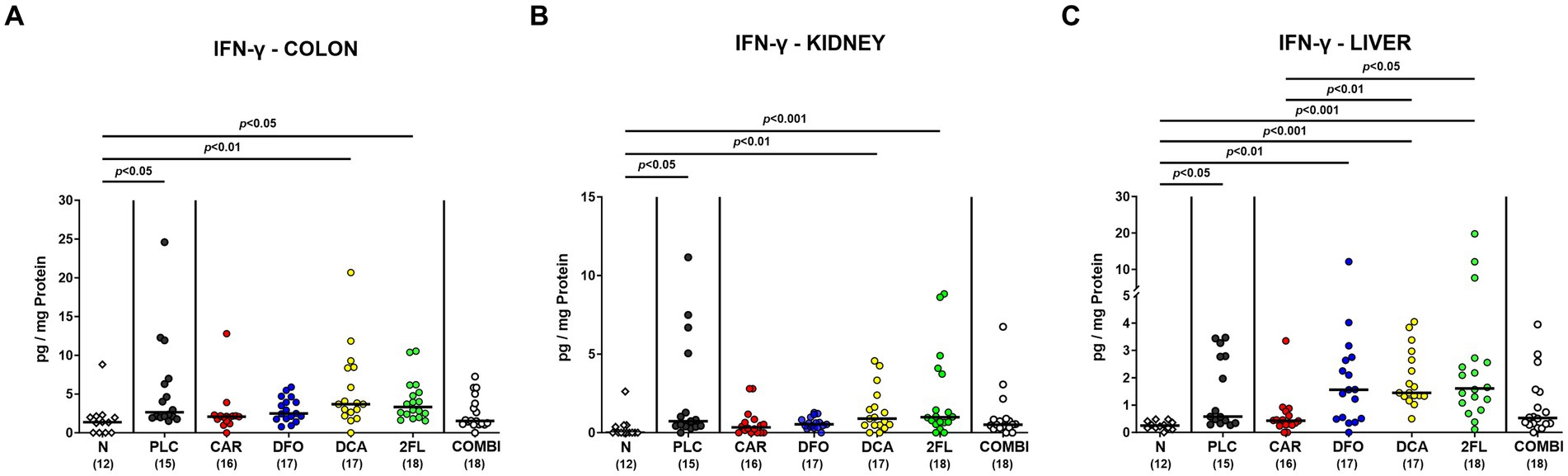
Figure 7. Intestinal and extra-intestinal IFN-γ concentrations in hma IL-10−/− mice following C. jejuni infection and treatment with carvacrol, deferoxamine, deoxycholic acid, and 2-fucosyl-lactose alone or all in combination. C. jejuni infected hma IL-10−/− mice were perorally treated with synthetic carvacrol (CAR), deferoxamine (DFO), deoxycholic acid (DCA), 2-fucosyl-lactose (2FL), a combination of the four compounds (COMBI) or placebo (PLC) via the drinking water from day 2 until day 6 post-infection. IFN-γ concentrations were determined in ex vivo biopsies taken from the (A) colon, (B) kidneys, and (C) liver on day 6 post-infection. Naive (N) hma IL-10−/− mice served as non-infected and untreated controls. Individual data pooled from three experiments, the medians (black bars), the numbers of included mice (in parentheses), and the significance levels (p values) determined by the Kruskal-Wallis test and Dunn’s post-correction are shown.
3.7 Pro-inflammatory cytokine secretion in the serum of hma IL-10−/− mice following Campylobacter jejuni infection and treatment with carvacrol, deferoxamine, deoxycholic acid, and 2-fucosyl-lactose alone or in combination
Further, we addressed whether the treatment regimens could even affect systemic C. jejuni induced cytokine responses. As for the kidneys, basal IFN-γ levels were measured in the serum of infected mice from the carvacrol, deferoxamine, and combination cohorts (n.s. versus naive: Figure 8A), which also held true for basal serum IL-6 concentrations determined in carvacrol and combination treated mice on day 6 p.i. (n.s. versus naive; Figure 8B). Hence, carvacrol and combination treatment of hma IL-10−/− mice could alleviate systemic pro-inflammatory immune responses upon C. jejuni infection.
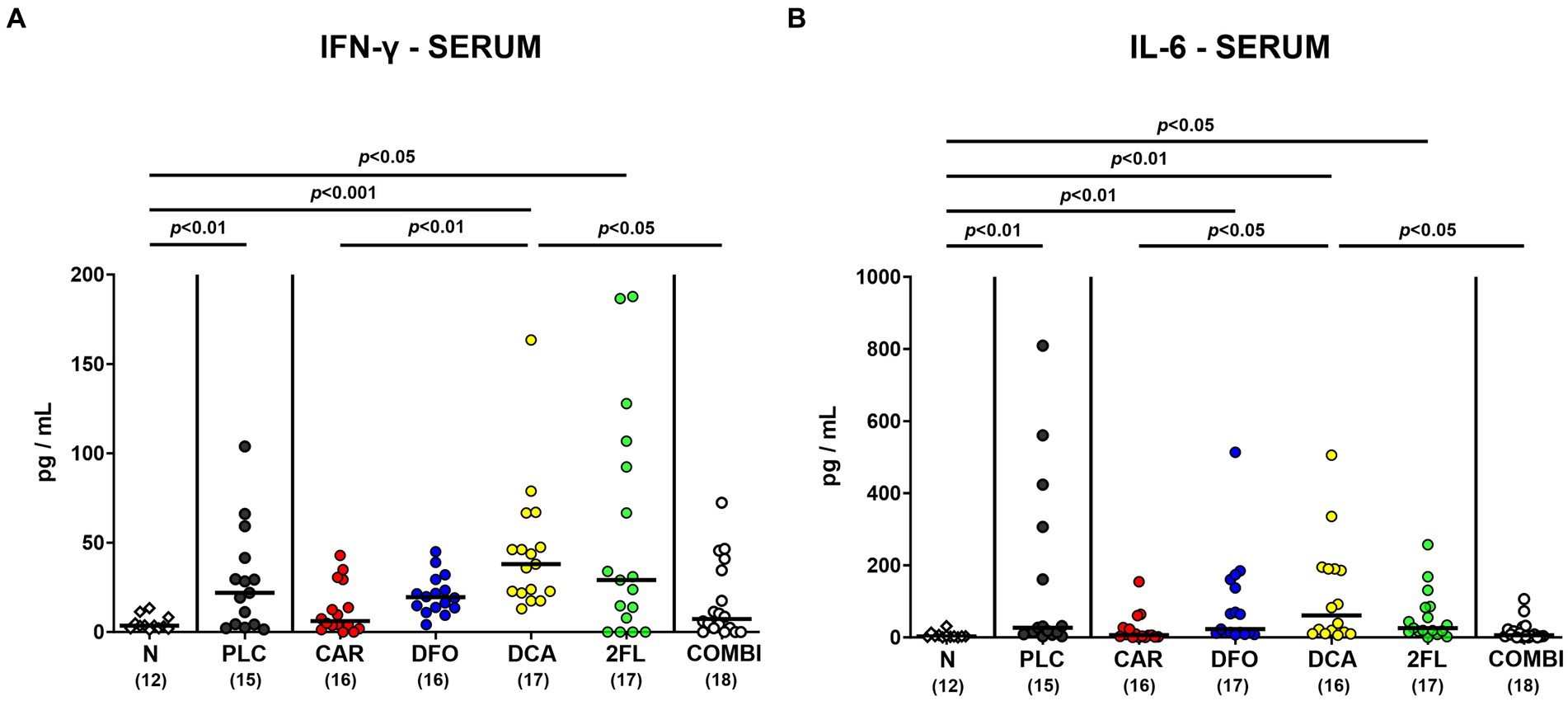
Figure 8. Systemic pro-inflammatory cytokine secretion in hma IL-10−/− mice following C. jejuni infection and treatment with carvacrol, deferoxamine, deoxycholic acid, and 2-fucosyl-lactose alone or all in combination. C. jejuni infected hma IL-10−/− mice were perorally treated with synthetic carvacrol (CAR), deferoxamine (DFO), deoxycholic acid (DCA), 2-fucosyl-lactose (2FL), a combination of the four compounds (COMBI) or placebo (PLC) via the drinking water from day 2 until day 6 post-infection. To assess systemic pro-inflammatory cytokine responses, (A) IFN-γ and (B) IL-6 concentrations were measured in serum samples taken on day 6 post-infection. Naive (N) hma IL-10−/− mice served as non-infected and untreated controls. Individual data pooled from three experiments, the medians (black bars), the numbers of included mice (in parentheses), and the significance levels (p values) determined by the Kruskal-Wallis test and Dunn’s post-correction are shown. Outliers were excluded following identification by the Grubb’s test.
4 Discussion
For our current preclinical placebo-controlled intervention trial, we used (with respect to the gut microbiota) “humanized” IL-10−/− mice that have been generated through triple oral human FMTs of secondary abiotic IL-10−/− mice. Like all experimental systems, the hma IL-10−/− mouse model has its own limitations, which need to be critically taken into consideration. For instance, distinct human bacterial phyla might have been affected by freezing and thawing of the human fecal donor suspensions. Furthermore, differences in engraftment of the human fecal transplants in the murine intestinal tract could affect the clinical and immunopathological course of infection (Shayya et al., 2023). Both, our cultural and molecular analyses of fecal samples taken 1-week following the human FMTs and hence, immediately before C. jejuni infection on day 0 revealed comparable fecal loads of the main intestinal commensal bacterial phyla in all six prospective treatment cohorts indicating similar intestinal bacterial conditions upon induction of campylobacteriosis. At the end of the observation period, no treatment regimens have affected C. jejuni loads in the colon, whereas on day 6 p.i. carvacrol treated hma IL-10−/− mice displayed approximately two orders of magnitude lower pathogen loads in the ileum, but not the colon when compared to placebo counterparts. One can speculate that most of the administered carvacrol might have already been absorbed by enterocytes before reaching the colon and exerting a direct pathogen-lowering effect in the colonic lumen. Previous in vitro and in vivo studies have proven anti-Campylobacter directed effects of carvacrol, but with varying efficacies depending on the experimental model, the applied carvacrol doses, and the duration of treatment (Johny et al., 2010; van Alphen et al., 2012; Kelly et al., 2017; Szott et al., 2020). Nevertheless, one could argue that the 2-log reduction in the ileal pathogen loads alone was not sufficient to explain the observed clinical effects upon oral carvacrol application. An in vitro study by Upadhyay et al. revealed that carvacrol treatment resulted in reduced adhesion invasion, and translocation of C. jejuni (Upadhyay et al., 2017). These results might explain the disease alleviating effect of oral carvacrol without pronounced antibacterial effects directed against C. jejuni infection in vivo. Nevertheless, other aspects such as the metabolization of carvacrol by members of the intestinal microbiota or direct immune-modulatory effects of the compound may have contributed to the better outcome of C. jejuni induced disease in treated mice.
In line with our results, even prophylactic application of deferoxamine in infected IL10−/− mice did not lower intestinal C. jejuni loads (Bereswill et al., 2022). Unexpectedly, deoxycholic acid and 2′-fucosyl-lactose did not exert antibacterial effects against C. jejuni, although numerous in vitro and in vivo studies have reported antimicrobial effects against various Gram-negative bacteria such as Pseudomonas aeruginosa, E. coli, Salmonella spp., and especially Campylobacter, for both substances (Yu et al., 2016; Gourley et al., 2017; Negretti et al., 2017; Alrubaye et al., 2019; Facinelli et al., 2019).
The lack of anti-Campylobacter effects of exogenous deoxycholic acid and 2′-fucosyl-lactose in our preclinical trial might be explained by concentrations in the drinking solutions that were below the measured MICs. Even though the concentrations of carvacrol, deferoxamine and all four combined compounds in the drinking solutions were exceeding the MIC of respective compounds measured in vitro, the treated mice harbored the enteropathogen at numbers that did not differ from those determined in placebo counterparts. This unexpected result might be explained due to mixing and dilution effects of the applied substances with secretory intestinal fluids, which have potentially resulted in a rather subtle or even absent antibacterial effect. This is further supported by the fact that mice do not exhibit continuous drinking behaviors during day and night.
Despite the high gastrointestinal pathogen burdens, carvacrol and the quadruple combination treatment did not only result in less severe diarrheal symptoms in C. jejuni infected hma mice, but also prevented diarrhea in over 80% of infected mice that did not present any changes in stool consistency on day 6 p.i. This could be confirmed by our previous study showing potent anti-diarrheal effects of prophylactic oral carvacrol in C. jejuni infected secondary abiotic IL10−/− mice (Mousavi et al., 2020). Furthermore, carvacrol was shown to inhibit the diarrheal toxin production by Bacillus cereus in vitro (Ultee et al., 2002) and to alleviate Clostridioides difficile associated diarrheal symptoms in mice (Mooyottu et al., 2017).
The marked improvement in diarrheal symptoms following carvacrol and combination treatment of hma IL-10−/− mice was accompanied by less pronounced microscopic inflammatory sequelae of C. jejuni infection like histopathological changes and apoptotic epithelial cell responses in the large intestine. In line with these findings, carvacrol strongly inhibited caspase-dependent apoptosis and down-regulated the mTOR-Signaling in vitro (Banik et al., 2019), whereas prophylactic carvacrol application attenuated C. jejuni-induced apoptosis in colonic epithelia of infected secondary abiotic IL-10−/− mice (Mousavi et al., 2020). Besides carvacrol, also oral deferoxamine and 2′-fucosyl-lactose alone diminished apoptotic colonic cell responses upon C. jejuni infection. In line with our findings, a study in adult rats showed that iron overload increased caspase-3 reactivity whereas, conversely, deferoxamine treatment ameliorated iron-induced intestinal apoptosis and inflammation (El-Sheikh et al., 2018). Furthermore, 2-fucosyl-lactose was shown to protect small intestinal epithelial cells against 5-fluorouracil-induced apoptosis (Zhao et al., 2022).
In addition to alleviated diarrheal symptoms and colonic epithelial cell apoptosis, oral carvacrol and quadruple combination treatment dampened pro-inflammatory immune cell responses in C. jejuni infected hma mice as indicated by attenuated accumulation of neutrophils and T lymphocytes in the colonic mucosa and lamina propria. Moreover, C. jejuni induced colonic T cell responses were decreased upon deferoxamine, deoxycholic acid, and 2-fucosyl-lactose application alone. In support, our previous studies revealed that C. jejuni infected IL-10−/− mice pre-treated with carvacrol or deferoxamine exhibited lower numbers of T lymphocytes in the large intestines, as compared to placebo controls (Mousavi et al., 2020; Bereswill et al., 2022). Additionally, 2′-fucosyl-lactose pretreatment was shown to reduce CD3+ T cell infiltration of the colon in C. jejuni infected wildtype mice (Yu et al., 2016). When assessing pro-inflammatory cytokine secretion in the infected large intestines, mice from the carvacrol, the deferoxamine, and the combination cohorts exhibited colonic IFN-γ concentrations that did not differ from those determined in naive mice. The anti-inflammatory effects of the applied compounds could also be observed beyond the intestinal tract given only basal IFN-γ concentrations measured in the kidneys, the liver, and even in the serum of infected mice from the carvacrol, the deferoxamine, and the combination treatment cohorts. In support, carvacrol treatment was shown to down-regulate IFN-γ expression in splenocytes of asthmatic mice (Kianmehr et al., 2016) and to decrease IFN-γ and IL-6 secretion in mice suffering from multiple sclerosis (Mahmoodi et al., 2019). A very recent study revealed that oral treatment with the iron chelating compound deferasirox alleviated acute dextran sulfate sodium (DSS) induced colitis that was accompanied by decreased IFN-γ serum concentrations (Wu et al., 2023), further underscoring the immune-modulatory effects of iron deprivation in acute intestinal inflammation including campylobacteriosis as shown for deferoxamine in our present and earlier studies (Bereswill et al., 2022).
Although previous reports have highlighted that both deoxycholic acid and 2-fucosyl-lactose attenuated alleviated intestinal inflammation including C. jejuni induced colitis in mice (Yu et al., 2016; Sun et al., 2018), no overt immune-modulatory effects of both substances were observed in our present trial. These discrepancies might be due to differences in experimental settings including dosage and duration of treatment, gut microbiota composition, genetic background, and animal husbandry, for instance.
Furthermore, given the here applied 4-day therapeutic treatment regimens, the disease-alleviating effects of respective compounds alone or in combination might have been more prominent after longer application periods starting before infection (i.e., prophylactic regimen). This might be addressed in future investigations.
Finally, the here applied “humanized” IL-10−/− mouse model provides a useful experimental tool (i) to dissect the interactions between C. jejuni on the pathogen side and the immune system as well as the commensal (murine or human) gut microbiota on the host side and (ii) to test defined molecules and compounds for their potential disease-alleviating including anti-pathogenic and immune-modulatory properties (Shayya et al., 2023).
5 Conclusion
Our placebo-controlled preclinical intervention study provides evidence that oral treatment with carvacrol, deferoxamine, deoxycholate, and 2-fucosyl-lactose exerts disease-alleviating effects in acute campylobacteriosis. All substances are approved by the U.S. Food and Drug Administration (Food and Drug Administration, 2023) and have proven to exert disease-alleviating effects in acute campylobacteriosis. In particular, the highly potent intestinal, extra-intestinal, and systemic anti-inflammatory properties of the non-toxic natural compounds might point towards a promising alternative in the fight of acute campylobacteriosis with antibiotics-independent treatment options.
Data availability statement
The original contributions presented in the study are included in the article/Supplementary material, further inquiries can be directed to the corresponding author.
Ethics statement
The animal study was approved by “Landesamt für Gesundheit und Soziales”, LaGeSo, Berlin. The study was conducted in accordance with the local legislation and institutional requirements.
Author contributions
SM: Formal analysis, Investigation, Visualization, Writing – original draft. MF: Formal analysis, Investigation. KD: Investigation, Writing – review & editing. RB: Investigation. SB: Conceptualization, Funding acquisition, Supervision, Writing – review & editing. MMH: Conceptualization, Funding acquisition, Investigation, Supervision, Validation, Writing – original draft.
Funding
The author(s) declare financial support was received for the research, authorship, and/or publication of this article. The project received funding from by the German Federal Ministries of Education and Research (BMBF) in frame of the Zoonoses Research Consortium PAC-Campylobacter to MMH and SB (IP7/01KI2007D) and from the Federal Ministry for Economic Affairs and Energy following a resolution of the German National Parliament, Deutscher Bundestag to MMH and SB (ZIM, ZF4117908 AJ8).
Acknowledgments
We are very grateful for the excellent contribution to this project of Alexandra Bittroff-Leben, Ines Puschendorf, Ulrike Fiebiger, Sumaya Abdul-Rahman, Gernot Reifenberger, Nizar W. Shayya, and the animal technicians at FEM of Charité – University Medicine Berlin. We acknowledge the financial support from the Open Access Publication Fund of Charité – Universitätsmedizin Berlin and the German Research Foundation (DFG).
Conflict of interest
The authors declare that the research was conducted in the absence of any commercial or financial relationships that could be construed as a potential conflict of interest.
The author(s) declared that they were an editorial board member of Frontiers, at the time of submission. This had no impact on the peer review process and the final decision.
Publisher’s note
All claims expressed in this article are solely those of the authors and do not necessarily represent those of their affiliated organizations, or those of the publisher, the editors and the reviewers. Any product that may be evaluated in this article, or claim that may be made by its manufacturer, is not guaranteed or endorsed by the publisher.
Supplementary material
The Supplementary material for this article can be found online at: https://www.frontiersin.org/articles/10.3389/fmicb.2024.1290490/full#supplementary-material
References
Acheson, D., and Allos, B. M. (2001). Campylobacter jejuni infections: update on emerging issues and trends. Clin. Infect. Dis. 32, 1201–1206. doi: 10.1086/319760
Alrubaye, B., Abraha, M., Almansour, A., Bansal, M., Wang, H., Kwon, Y. M., et al. (2019). Microbial metabolite deoxycholic acid shapes microbiota against Campylobacter jejuni chicken colonization. PLoS One 14:e0214705. doi: 10.1371/journal.pone.0214705
Banik, S., Akter, M., Corpus Bondad, S. E., Saito, T., Hosokawa, T., and Kurasaki, M. (2019). Carvacrol inhibits cadmium toxicity through combating against caspase dependent/independent apoptosis in PC12 cells. Food Chem. Toxicol. 134:110835. doi: 10.1016/j.fct.2019.110835
Bellotti, D., and Remelli, M. (2021). Deferoxamine B: a natural, excellent and versatile metal chelator. Molecules 26:3255. doi: 10.3390/molecules26113255
Bereswill, S., Fischer, A., Plickert, R., Haag, L. M., Otto, B., Kuhl, A. A., et al. (2011). Novel murine infection models provide deep insights into the "menage a trois" of Campylobacter jejuni, microbiota and host innate immunity. PLoS One 6:e20953. doi: 10.1371/journal.pone.0020953
Bereswill, S., Mousavi, S., Weschka, D., Buczkowski, A., Schmidt, S., and Heimesaat, M. M. (2022). Iron deprivation by Oral Deferoxamine application alleviates acute Campylobacteriosis in a clinical murine Campylobacter jejuni infection model. Biomol. Ther. 13:71. doi: 10.3390/biom13010071
Bereswill, S., Plickert, R., Fischer, A., Kühl, A. A., Loddenkemper, C., Batra, A., et al. (2011). What you eat is what you get: novel Campylobacter models in the quadrangle relationship between nutrition, obesity, microbiota and susceptibility to infection. Eur. J. Microbiol. Immunol. (Bp). 1, 237–248. doi: 10.1556/EuJMI.1.2011.3.8
Callahan, S. M., Dolislager, C. G., and Johnson, J. G. (2021). The host cellular immune response to infection by Campylobacter spp. and its role in disease. Infect. Immun. 89, e00116–e00121. doi: 10.1128/IAI.00116-21
Chung, H., Pamp, S. J., Hill, J. A., Surana, N. K., Edelman, S. M., Troy, E. B., et al. (2012). Gut immune maturation depends on colonization with a host-specific microbiota. Cell 149, 1578–1593. doi: 10.1016/j.cell.2012.04.037
Davis, J. C., Totten, S. M., Huang, J. O., Nagshbandi, S., Kirmiz, N., Garrido, D. A., et al. (2016). Identification of oligosaccharides in feces of breast-fed infants and their correlation with the gut microbial community. Mol. Cell. Proteomics 15, 2987–3002. doi: 10.1074/mcp.M116.060665
de Zoete, M. R., Keestra, A. M., Roszczenko, P., and van Putten, J. P. (2010). Activation of human and chicken toll-like receptors by Campylobacter spp. Infect. Immun. 78, 1229–1238. doi: 10.1128/IAI.00897-09
El-Sheikh, A. A., Ameen, S. H., and Abd El-Fatah, S. S. (2018). Ameliorating iron overload in intestinal tissue of adult male rats: quercetin vs deferoxamine. J. Toxicol. 2018, 1–13. doi: 10.1155/2018/8023840
Engfer, M. B., Stahl, B., Finke, B., Sawatzki, G., and Daniel, H. (2000). Human milk oligosaccharides are resistant to enzymatic hydrolysis in the upper gastrointestinal tract. Am. J. Clin. Nutr. 71, 1589–1596. doi: 10.1093/ajcn/71.6.1589
Erben, U., Loddenkemper, C., Doerfel, K., Spieckermann, S., Haller, D., Heimesaat, M. M., et al. (2014). A guide to histomorphological evaluation of intestinal inflammation in mouse models. Int. J. Clin. Exp. Pathol. 7, 4557–4576.
European Food Safety Authority, European Centre for Disease Prevention and Control (2022). The European Union summary report on antimicrobial resistance in zoonotic and indicator bacteria from humans, animals and food in 2019–2020. EFSA J. 20:e07209. doi: 10.2903/j.efsa.2022.7209
Facinelli, B., Marini, E., Magi, G., Zampini, L., Santoro, L., Catassi, C., et al. (2019). Breast milk oligosaccharides: effects of 2′-fucosyllactose and 6′-sialyllactose on the adhesion of Escherichia coli and Salmonella fyris to Caco-2 cells. J. Matern. Fetal Neonatal Med. 32, 2950–2952. doi: 10.1080/14767058.2018.1450864
Food and Drug Administration F. Food and Drug Administration-dietary supplement ingredient directory. (2023). Available at: https://www.fda.gov/food/dietary-supplements/dietary-supplement-ingredient-directory.
Foote, M. S., Du, K., Mousavi, S., Bereswill, S., and Heimesaat, M. M. (2023). Therapeutic Oral application of Carvacrol alleviates acute Campylobacteriosis in mice harboring a human gut microbiota. Biomol. Ther. 13:320. doi: 10.3390/biom13020320
Garrido, D., Ruiz-Moyano, S., Kirmiz, N., Davis, J. C., Totten, S. M., Lemay, D. G., et al. (2016). A novel gene cluster allows preferential utilization of fucosylated milk oligosaccharides in Bifidobacterium longum subsp. longum SC596. Sci. Rep. 6:35045. doi: 10.1038/srep35045
Gholami-Ahangaran, M., Ahmadi-Dastgerdi, A., Azizi, S., Basiratpour, A., Zokaei, M., and Derakhshan, M. (2022). Thymol and carvacrol supplementation in poultry health and performance. Vet. Med. Sci. 8, 267–288. doi: 10.1002/vms3.663
Gourley, C. R., Negretti, N. M., and Konkel, M. E. (2017). The food-borne pathogen Campylobacter jejuni depends on the AddAB DNA repair system to defend against bile in the intestinal environment. Sci. Rep. 7:14777. doi: 10.1038/s41598-017-14646-9
Haag, L. M., Fischer, A., Otto, B., Plickert, R., Kuhl, A. A., Gobel, U. B., et al. (2012). Campylobacter jejuni induces acute enterocolitis in gnotobiotic IL-10−/− mice via toll-like-receptor-2 and -4 signaling. PLoS One 7:e40761. doi: 10.1371/journal.pone.0040761
Heimesaat, M. M., Alutis, M., Grundmann, U., Fischer, A., Tegtmeyer, N., Böhm, M., et al. (2014). The role of serine protease HtrA in acute ulcerative enterocolitis and extra-intestinal immune responses during Campylobacter jejuni infection of gnotobiotic IL-10 deficient mice. Front. Cell. Infect. Microbiol. 4:77. doi: 10.3389/fcimb.2014.00077
Heimesaat, M. M., Bereswill, S., Fischer, A., Fuchs, D., Struck, D., Niebergall, J., et al. (2006). Gram-negative bacteria aggravate murine small intestinal Th1-type immunopathology following oral infection with toxoplasma gondii. J. Immunol. 177, 8785–8795. doi: 10.4049/jimmunol.177.12.8785
Heimesaat, M. M., Genger, C., Klove, S., Weschka, D., Mousavi, S., and Bereswill, S. (2020). The host-specific intestinal microbiota composition impacts Campylobacter coli infection in a clinical mouse model of Campylobacteriosis. Pathogens 9:804. doi: 10.3390/pathogens9100804
Heimesaat, M. M., Giladi, E., Kühl, A. A., Bereswill, S., and Gozes, I. (2018). The octapetide NAP alleviates intestinal and extra-intestinal anti-inflammatory sequelae of acute experimental colitis. Peptides 101, 1–9. doi: 10.1016/j.peptides.2017.12.023
Heimesaat, M. M., Mousavi, S., Bandick, R., and Bereswill, S. (2022). Campylobacter jejuni infection induces acute enterocolitis in IL-10−/− mice pretreated with ampicillin plus sulbactam. Eur. J. Microbiol. Immunol. (Bp). 12, 73–83. doi: 10.1556/1886.2022.00014
Herzog, M. K.-M., Cazzaniga, M., Peters, A., Shayya, N., Beldi, L., Hapfelmeier, S., et al. (2023). Mouse models for bacterial enteropathogen infections: insights into the role of colonization resistance. Gut Microbes 15:2172667. doi: 10.1080/19490976.2023.2172667
Hu, J., Wang, C., Huang, X., Yi, S., Pan, S., Zhang, Y., et al. (2021). Gut microbiota-mediated secondary bile acids regulate dendritic cells to attenuate autoimmune uveitis through TGR5 signaling. Cell Rep. 36:109726. doi: 10.1016/j.celrep.2021.109726
Itoh, M., Wada, K., Tan, S., Kitano, Y., Kai, J., and Makino, I. (1999). Antibacterial action of bile acids against helicobacter pylori and changes in its ultrastructural morphology: effect of unconjugated dihydroxy bile acid. J. Gastroenterol. 34, 571–576. doi: 10.1007/s005350050374
Johny, A. K., Darre, M., Donoghue, A., Donoghue, D., and Venkitanarayanan, K. (2010). Antibacterial effect of trans-cinnamaldehyde, eugenol, carvacrol, and thymol on Salmonella Enteritidis and Campylobacter jejuni in chicken cecal contents in vitro. J. Appl. Poult. Res. 19, 237–244. doi: 10.3382/japr.2010-00181
Jurado, R. L. (1997). Iron, infections, and anemia of inflammation. Clin. Infect. Dis. 25, 888–895. doi: 10.1086/515549
Kaakoush, N. O., Castano-Rodriguez, N., Mitchell, H. M., and Man, S. M. (2015). Global epidemiology of Campylobacter infection. Clin. Microbiol. Rev. 28, 687–720. doi: 10.1128/CMR.00006-15
Kelly, C., Gundogdu, O., Pircalabioru, G., Cean, A., Scates, P., Linton, M., et al. (2017). The in vitro and in vivo effect of Carvacrol in preventing Campylobacter infection, colonization and in improving productivity of chicken broilers. Foodborne Pathog. Dis. 14, 341–349. doi: 10.1089/fpd.2016.2265
Kianmehr, M., Rezaei, A., and Boskabady, M. H. (2016). Effect of carvacrol on various cytokines genes expression in splenocytes of asthmatic mice. Iran. J. Basic Med. Sci. 19, 402–410.
Kreling, V., Falcone, F. H., Kehrenberg, C., and Hensel, A. (2020). Campylobacter sp.: pathogenicity factors and prevention methods—new molecular targets for innovative antivirulence drugs? Appl. Microbiol. Biotechnol. 104, 10409–10436. doi: 10.1007/s00253-020-10974-5
Lobo de Sá, F., Schulzke, J.-D., and Bücker, R. (2021). Diarrheal mechanisms and the role of intestinal barrier dysfunction in Campylobacter infections. Curr. Top. Microbiol. Immunol. 431, 203–231. doi: 10.1007/978-3-030-65481-8_8
Mączka, W., Twardawska, M., Grabarczyk, M., and Wińska, K. (2023). Carvacrol; a natural phenolic compound with antimicrobial properties. Antibiotics 12:824. doi: 10.3390/antibiotics12050824
Mahmoodi, M., Amiri, H., Ayoobi, F., Rahmani, M., Taghipour, Z., Ghavamabadi, R. T., et al. (2019). Carvacrol ameliorates experimental autoimmune encephalomyelitis through modulating pro-and anti-inflammatory cytokines. Life Sci. 219, 257–263. doi: 10.1016/j.lfs.2018.11.051
Manfredi, R., Nanetti, A., Ferri, M., and Chiodo, F. (1999). Fatal Campylobacter jejuni bacteraemia in patients with AIDS. J. Med. Microbiol. 48, 601–603. doi: 10.1099/00222615-48-6-601
Mansfield, L. S., Bell, J. A., Wilson, D. L., Murphy, A. J., Elsheikha, H. M., Rathinam, V. A., et al. (2007). C57BL/6 and congenic interleukin-10-deficient mice can serve as models of Campylobacter jejuni colonization and enteritis. Infect. Immun. 75, 1099–1115. doi: 10.1128/IAI.00833-06
Moon, J.-H., Herr, Y., Kim, S.-W., and Lee, J.-Y. (2011). In vitro activity of deferoxamine against Porphyromonas gingivalis. FEMS Microbiol. Lett. 323, 61–67. doi: 10.1111/j.1574-6968.2011.02357.x
Moore, J. E., Corcoran, D., Dooley, J. S., Fanning, S., Lucey, B., Matsuda, M., et al. (2005). Campylobacter. Vet. Res. 36, 351–382. doi: 10.1051/vetres:2005012
Mooyottu, S., Flock, G., Upadhyay, A., Upadhyaya, I., Maas, K., and Venkitanarayanan, K. (2017). Protective effect of carvacrol against gut dysbiosis and Clostridium difficile associated disease in a mouse model. Front. Microbiol. 8:625. doi: 10.3389/fmicb.2017.00625
Mortensen, N. P., Kuijf, M. L., Ang, C. W., Schiellerup, P., Krogfelt, K. A., Jacobs, B. C., et al. (2009). Sialylation of Campylobacter jejuni lipo-oligosaccharides is associated with severe gastro-enteritis and reactive arthritis. Microbes Infect. 11, 988–994. doi: 10.1016/j.micinf.2009.07.004
Mouftah, S. F., Cobo-Díaz, J. F., Álvarez-Ordóñez, A., Elserafy, M., Saif, N. A., Sadat, A., et al. (2021). High-throughput sequencing reveals genetic determinants associated with antibiotic resistance in Campylobacter spp. from farm-to-fork. PLoS One 16:e0253797. doi: 10.1371/journal.pone.0253797
Mousavi, S., Busmann, L. V., Bandick, R., Shayya, N. W., Bereswill, S., and Heimesaat, M. M. (2023). Oral application of carvacrol, butyrate, ellagic acid, and 2′-fucosyl-lactose to mice suffering from acute campylobacteriosis – results from a preclinical placebo-controlled intervention study. Eur. J. Microbiol. Immunol. (Bp). 13, 88–105. doi: 10.1556/1886.2023.00037
Mousavi, S., Schmidt, A.-M., Escher, U., Kittler, S., Kehrenberg, C., Thunhorst, E., et al. (2020). Carvacrol ameliorates acute campylobacteriosis in a clinical murine infection model. Gut Pathog. 12:2. doi: 10.1186/s13099-019-0343-4
Mullineaux-Sanders, C., Suez, J., Elinav, E., and Frankel, G. (2018). Sieving through gut models of colonization resistance. Nat. Microbiol. 3, 132–140. doi: 10.1038/s41564-017-0095-1
Nachamkin, I., Allos, B. M., and Ho, T. (1998). Campylobacter species and Guillain-Barre syndrome. Clin. Microbiol. Rev. 11, 555–567. doi: 10.1128/CMR.11.3.555
Negretti, N. M., Gourley, C. R., Clair, G., Adkins, J. N., and Konkel, M. E. (2017). The food-borne pathogen Campylobacter jejuni responds to the bile salt deoxycholate with countermeasures to reactive oxygen species. Sci. Rep. 7:15455. doi: 10.1038/s41598-017-15379-5
Newburg, D. S. (2005). Innate immunity and human milk. J. Nutr. 135, 1308–1312. doi: 10.1093/jn/135.5.1308
Obaidat, M. M., and Frank, J. F. (2009). Inactivation of Salmonella and Escherichia coli O157: H7 on sliced and whole tomatoes by allyl isothiocyanate, carvacrol, and cinnamaldehyde in vapor phase. J. Food Prot. 72, 315–324. doi: 10.4315/0362-028X-72.2.315
Omarova, S., Awad, K., Moos, V., Püning, C., Gölz, G., Schulzke, J.-D., et al. (2023). Intestinal barrier in post-Campylobacter jejuni irritable bowel syndrome. Biomol. Ther. 13:449. doi: 10.3390/biom13030449
Price, A., Jewkes, J., and Sanderson, P. (1979). Acute diarrhoea: Campylobacter colitis and the role of rectal biopsy. J. Clin. Pathol. 32, 990–997. doi: 10.1136/jcp.32.10.990
Robinson, T. P., Bu, D. P., Carrique-Mas, J., Fevre, E. M., Gilbert, M., Grace, D., et al. (2016). Antibiotic resistance is the quintessential one health issue. Trans. R. Soc. Trop. Med. Hyg. 110, 377–380. doi: 10.1093/trstmh/trw048
Sela, D. A., and Mills, D. A. (2010). Nursing our microbiota: molecular linkages between bifidobacteria and milk oligosaccharides. Trends Microbiol. 18, 298–307. doi: 10.1016/j.tim.2010.03.008
Sharifi-Rad, M., Varoni, E. M., Iriti, M., Martorell, M., Setzer, W. N., del Mar, C. M., et al. (2018). Carvacrol and human health: a comprehensive review. Phytother. Res. 32, 1675–1687. doi: 10.1002/ptr.6103
Shayya, N. W., Foote, M. S., Langfeld, L. Q., Du, K., Bandick, R., Mousavi, S., et al. (2023). Human microbiota associated IL-10−/− mice: a valuable enterocolitis model to dissect the interactions of Campylobacter jejuni with host immunity and gut microbiota. Eur. J. Microbiol. Immunol. (Bp). 12, 107–122. doi: 10.1556/1886.2022.00024
Su, X., Gao, Y., and Yang, R. (2023). Gut microbiota derived bile acid metabolites maintain the homeostasis of gut and systemic immunity. Front. Immunol. 14:1127743. doi: 10.3389/fimmu.2023.1127743
Sun, X., Liu, B., Sartor, R. B., and Jobin, C. (2013). Phosphatidylinositol 3-kinase-gamma signaling promotes Campylobacter jejuni-induced colitis through neutrophil recruitment in mice. J. Immunol. 190, 357–365. doi: 10.4049/jimmunol.1201825
Sun, X., Threadgill, D., and Jobin, C. (2012). Campylobacter jejuni induces colitis through activation of mammalian target of rapamycin signaling. Gastroenterology 142, 86–95.e5. doi: 10.1053/j.gastro.2011.09.042
Sun, X., Winglee, K., Gharaibeh, R. Z., Gauthier, J., He, Z., Tripathi, P., et al. (2018). Microbiota-derived metabolic factors reduce campylobacteriosis in mice. Gastroenterology 154, 1751–1763.e2. e2. doi: 10.1053/j.gastro.2018.01.042
Szott, V., Reichelt, B., Alter, T., Friese, A., and Roesler, U. (2020). In vivo efficacy of carvacrol on Campylobacter jejuni prevalence in broiler chickens during an entire fattening period. Eur. J. Microbiol. Immunol. (Bp). 10, 131–138. doi: 10.1556/1886.2020.00011
Ultee, A., Bennik, M. H. J., and Moezelaar, R. (2002). The phenolic hydroxyl Group of Carvacrol is Essential for action against the food-borne pathogen Bacillus cereus. Appl. Environ. Microbiol. 68, 1561–1568. doi: 10.1128/AEM.68.4.1561-1568.2002
Upadhyay, A., Arsi, K., Wagle, B. R., Upadhyaya, I., Shrestha, S., Donoghue, A. M., et al. (2017). Trans-cinnamaldehyde, carvacrol, and eugenol reduce Campylobacter jejuni colonization factors and expression of virulence genes in vitro. Front. Microbiol. 8:713. doi: 10.3389/fmicb.2017.00713
van Alphen, L. B., Burt, S. A., Veenendaal, A. K., Bleumink-Pluym, N. M., and van Putten, J. P. (2012). The natural antimicrobial carvacrol inhibits Campylobacter jejuni motility and infection of epithelial cells. PLoS One 7:e45343. doi: 10.1371/journal.pone.0045343
van Asbeck, B. S., Marcelis, J. H., Marx, J. J., Struyvenberg, A., van Kats, J. H., and Verhoef, J. (1983). Inhibition of bacterial multiplication by the iron chelator deferoxamine: potentiating effect of ascorbic acid. Eur. J. Clin. Microbiol. 2, 426–431. doi: 10.1007/BF02013899
Van Asbeck, B., Marcelis, J., Van Kats, J., Jaarsma, E., and Verhoef, J. (1983). Synergy between the iron chelator deferoxamine and the antimicrobial agents gentamicin, chloramphenicol, cefalothin, cefotiam and cefsulodin. Eur. J. Clin. Microbiol. 2, 432–438. doi: 10.1007/BF02013900
Wagenaar, J. A., Newell, D. G., Kalupahana, R. S., and Mughini-Gras, L. (2023). “Campylobacter: animal reservoirs, human infections, and options for control” in Zoonoses: infections affecting humans and animals, ed. A. Sing (Cham: Springer), 267–293.
Walker, R. I., Caldwell, M. B., Lee, E. C., Guerry, P., Trust, T. J., and Ruiz-Palacios, G. (1986). Pathophysiology of Campylobacter enteritis. Microbiol. Rev. 50, 81–94. doi: 10.1128/mr.50.1.81-94.1986
WHO. World Health Organisation. Campylobacter. (2020). Available at: https://www.who.int/news-room/fact-sheets/detail/campylobacter.
Wu, Y., Ran, L., Yang, Y., Gao, X., Peng, M., Liu, S., et al. (2023). Deferasirox alleviates DSS-induced ulcerative colitis in mice by inhibiting ferroptosis and improving intestinal microbiota. Life Sci. 314:121312. doi: 10.1016/j.lfs.2022.121312
Young, K. T., Davis, L. M., and Dirita, V. J. (2007). Campylobacter jejuni: molecular biology and pathogenesis. Nat. Rev. Microbiol. 5, 665–679. doi: 10.1038/nrmicro1718
Yu, Z.-T., Nanthakumar, N. N., and Newburg, D. S. (2016). The human milk oligosaccharide 2′-fucosyllactose quenches Campylobacter jejuni–induced inflammation in human epithelial cells HEp-2 and HT-29 and in mouse intestinal mucosa. J. Nutr. 146, 1980–1990. doi: 10.3945/jn.116.230706
Zhang, Q., Beyi, A. F., and Yin, Y. (2023). Zoonotic and antibiotic-resistant Campylobacter: a view through the one health lens. One Health Adv. 1:4. doi: 10.1186/s44280-023-00003-1
Zhao, G., Williams, J., Washington, M. K., Yang, Y., Long, J., Townsend, S. D., et al. (2022). 2’-Fucosyllactose ameliorates chemotherapy-induced intestinal mucositis by protecting intestinal epithelial cells against apoptosis. Cell. Mol. Gastroenterol. Hepatol. 13, 441–457. doi: 10.1016/j.jcmgh.2021.09.015
Keywords: natural compounds, Campylobacter jejuni , immune-modulatory effects, secondary abiotic IL-10−/− mice, human gut microbiota associated mice, campylobacteriosis model, host-pathogen interaction, placebo-controlled preclinical intervention study
Citation: Mousavi S, Foote MS, Du K, Bandick R, Bereswill S and Heimesaat MM (2024) Oral treatment of human gut microbiota associated IL-10−/− mice suffering from acute campylobacteriosis with carvacrol, deferoxamine, deoxycholic acid, and 2-fucosyl-lactose. Front. Microbiol. 15:1290490. doi: 10.3389/fmicb.2024.1290490
Edited by:
Ozan Gundogdu, University of London, United KingdomReviewed by:
Abhinav Upadhyay, University of Connecticut, United StatesPei Shang, Mayo Clinic, United States
Ulrich Steinhoff, University of Marburg, Germany
Igori Balta, University of Life Sciences "King Mihai I", Romania
Copyright © 2024 Mousavi, Foote, Du, Bandick, Bereswill and Heimesaat. This is an open-access article distributed under the terms of the Creative Commons Attribution License (CC BY). The use, distribution or reproduction in other forums is permitted, provided the original author(s) and the copyright owner(s) are credited and that the original publication in this journal is cited, in accordance with accepted academic practice. No use, distribution or reproduction is permitted which does not comply with these terms.
*Correspondence: Markus M. Heimesaat, bWFya3VzLmhlaW1lc2FhdEBjaGFyaXRlLmRl
†These authors have contributed equally to this work and share last authorship