- 1Department of Clinical Laboratory, Wenzhou TCM Hospital of Zhejiang Chinese Medical University, Wenzhou, Zhejiang, China
- 2Department of TCM Science and Research Center, Wenzhou, Zhejiang, China
- 3Department of Clinical Laboratory, Wenzhou People’s Hospital, Wenzhou Women and Children’s Hospital, Wenzhou, Zhejiang, China
The enzyme AAC(6′)-Ib-cr belongs to plasmid-mediated quinolone resistance (PMQR), first reported in 2006 and now widely disseminating. Here, we developed three phenotypic methods to detect AAC(6′)-Ib-cr enzyme-producing Enterobacteriaceae (APE), two of which are proposed innovatively in this research. These tests are based on the following principles: (i) Matrix-assisted laser desorption/ionization time-of-flight (MALDI-TOF MS) can measure the mass shift of 42 Da resulting from ciprofloxacin acetylation by the AAC(6′)-Ib-cr enzyme. (ii) Co-incubation of ciprofloxacin disks with APE results in inactivation of the drug activity, making it unable to inhibit the growth of the indicator organism. We named this test the quinolone inactivation method (QIM). (iii) Based on the principles of the modified Hodge test, we designed the quinolone Hodge test (QHT). Through exploration of optimal conditions for three methods, we found that MALDI-TOF MS provides the most intuitive results after 1 h of incubation. The interpretability of the QIM and QHT results was significantly improved when the indicator organism E. coli ATCC25922 was replaced with a quinolone-slightly-resistant isolate. However, Proteus mirabilis was excluded from both QIM and QHT due to its swarming motility. Next, a validation study was conducted using a prospectively collected set of 187 clinical strains, demonstrating 100% specificity (MSM: 141/141; QIM, QHT: 135/135) and 100% sensitivity (MSM: 46/46; QIM, QHT: 33/33) compared to the genotype. In a word, this study presented three simple, efficient, and cost-effective methods for detecting APE, suitable for clinical microbiology laboratories under various conditions for the prevention and control of hospital infections.
Introduction
Quinolone antibiotics are chemically synthesized drugs with advantages of high blood concentrations, excellent broad-spectrum antibacterial activity, and strong tissue penetration (Ahmed and Daneshtalab, 2012; Ball et al., 1998), making them a preferred agent for empirical treatment of infections (Gupta et al., 2011; Pilatz et al., 2020; Taplitz et al., 2018). They were the second most frequently prescribed antimicrobial drugs after β-lactam (Mercuro et al., 2018; Sanchez et al., 2016; Shapiro et al., 2014), but followed by serious bacterial resistance (Logan et al., 2019; Pitout et al., 2022; Zhao et al., 2024). Researches have shown that bacterial resistance to quinolones is mainly mediated by chromosomal mechanisms, including alterations in drug action sites (DNA gyrase and topoisomerase IV gene mutations) and decreased drug accumulation capacity (loss of membrane porins and overexpression of efflux pumps) (Darby et al., 2023; Pham et al., 2019). Of greater concern were plasmid-mediated quinolone resistance (PMQR) due to their higher transmissibility (Ellabaan et al., 2021), including: Qnr protein families, which protect quinolone targets; the AAC(6′)-Ib-cr enzyme, which acetylates ciprofloxacin and norfloxacin; and efflux pumps mediated by qepA and oqxAB plasmid genes (Ruiz et al., 2012). Among them, the aac(6′)-Ib-cr gene is the most threatening as it provides a selective advantage in the presence of ciprofloxacin (Phan et al., 2022). Since its first report in 2006 (Robicsek et al., 2006), the prevalence of strains carrying the aac(6′)-Ib-cr gene has been increasing in both clinical and environmental settings. According to recent studies, this gene has been detected in various countries of Asia (Al-Khafaji et al., 2024; Kuo et al., 2024; Sohrabi et al., 2024; Zhan et al., 2021), Europe (Hrovat et al., 2019; Mounsey et al., 2021; Piccirilli et al., 2021), Africa (Al-Gallas et al., 2024; Masoud et al., 2021; Swedan et al., 2023), and the Americas (de Oliveira Alves et al., 2022; Golden et al., 2021; Logan et al., 2023) across multiple Gram-negative bacteria. The detection rates of the gene vary significantly by region and source, typically ranging from 10% to 50%. Furthermore, the distribution of the aac(6′)-Ib-cr gene is widespread, with its presence detected not only in clinical strains but also in animals, food, and water sources (Al-Gallas et al., 2024; Mounsey et al., 2021; Patel et al., 2024; Zahra et al., 2023).
However, unlike the qnr, qepA, and oqxAB screening, which can be accomplished by PCR amplification of the target genes, the detection of aac(6′)-Ib-cr is more complicated. This is because AAC(6′)-Ib-cr belongs to a variant of aminoglycoside acetyltransferases, and the differences between its encoding gene aac(6′)-Ib-cr and the wild-type aac(6′)-Ib exist only on just two nucleotides (Trp102Arg and Asp179Tyr) (Robicsek et al., 2006). These specific point mutations enable the acetylation of norfloxacin and ciprofloxacin at the amino nitrogen on position 7-C of the piperazine ring, thereby reducing drug activity (Vetting et al., 2008; Maurice et al., 2008). Therefore, the screening for aac(6′)-Ib-cr traditionally involves amplification of the target gene, followed by sequencing or restriction analysis (Pitout et al., 2008). Obviously, this is a time-consuming and expensive work. Although several studies have proposed alternative methods for aac(6′)-Ib-cr sequencing (Hidalgo-Grass and Strahilevitz, 2010; Wareham et al., 2010), these methods still relied on the use of large equipment not typically available in ordinary laboratories. To date, no study has proposed a method for detecting the AAC(6′)-Ib-cr enzyme based on common antimicrobial susceptibility testing consumables.
Here, we proposed three low-cost phenotypic methods of detecting the AAC(6′)-Ib-cr enzyme in Enterobacteriaceae. They each possess distinct characteristics and are suitable for clinical microbiology laboratories under different conditions. We aim to (i) improve existing phenotypic detection methods for AAC(6′)-Ib-cr, (ii) develop and validate QIM and QHT as reliable alternatives, and (iii) compare their performance with mass spectrometry and PCR. These methods are based on the following principles: (i) Matrix-assisted laser desorption/ionization time-of-flight (MALDI-TOF MS) is able to measure the mass transfer of 42 Da resulting from acetylation of ciprofloxacin by the AAC(6′)-Ib-cr enzyme. Based on this, the appearance of spectrum peaks representing acetylated ciprofloxacin and its sodium or potassium adduct reveals the presence of the AAC(6′)-Ib-cr enzyme. This method was initially reported by Pardo et al. (2016), and we followed their steps with some modifications while enriching the species of the measured strains. (ii) The quinolone inactivation method (QIM), which is introduced and named for the first time in this study, is based on a principle derived from the Carbapenem Inactivation Method (CIM) (Pierce et al., 2017; van der Zwaluw et al., 2015): after co-incubation with test strains for some time, the ciprofloxacin adhered onto the disk will be inactivated by the enzyme-producing strains, thereby unable to inhibit the growth of the indicator organism. Furthermore, replacing the indicator organism E. coli ATCC 25922 with an isolate with only slight resistance to quinolones can greatly improve the observation of results. Because AAC(6′)-Ib-cr enzyme can easily reduce the quinolone concentration to a level where slightly resistant strains can grow, but it still cannot be lowered enough to support the growth of highly sensitive wild-type strains, as this would require nearly complete depletion of the quinolone. (iii) The last approach is based on the reduction of quinolone drug activity by the AAC(6′)-Ib-cr enzyme-producing Enterobacteriaceae (APE), allowing indicator organisms to grow in a curved manner along the inoculum of the tested strain toward the disk. This principle was first proposed by Hodge et al. (1978) to detect penicillinase and has subsequently been modified multiple times for detection of β-lactamases. The excellent performance of Modified Hodge Test (MHT) for screening carbapenase has been repeatedly demonstrated (Lee et al., 2001; Pasteran et al., 2016). In this study, we attempt to apply this principle to the detection of quinolone acetyltransferases, and therefore name it the quinolone Hodge Test (QHT).
Materials and methods
Bacterial isolates
(i) Isolates genetically tested: During the first stage of this study, a total of 14 Enterobacteriaceae strains were included to explore the optimal conditions for the three methods. All isolates have been previously characterized at the molecular level to determine the various mechanisms of resistance to quinolone antibiotics, including aac(6′)-Ib-cr, qnrA, qnrB, qnrC, qnrD, qnrS, qepA, as well as the quinolone resistance-determining regions (QRDRs) of the gyrA and parC genes encoding type II topoisomerases.
(ii) Indicator organisms: We collected four E. coli strains for the screening indicator organisms in QIM and QHT, including E. coli 28B220 with a single QRDR mutation (gyrA: S83L), E. coli B2-4 with a single QRDR mutation (gyrA: D87N), E. coli B2-5 with two QRDR mutations (gyrA: S83L; parC: S80I), and E. coli ATCC 25922. All of these strains were confirmed to not carry any of the aforementioned PMQR genes.
(iii) Prospective clinical isolates: A total of 187 quinolone non-susceptible Enterobacteriaceae isolates representing 9 genera, including E. coli (n = 104), K. pneumoniae (n = 47), Klebsiella oxytoca (n = 1), P. mirabilis (n = 19), Raoultella ornithinolytica (n = 2), Serratia marcescens (n = 3), Enterobacter spp. (n = 3), Citrobacter spp. (n = 4), Morganella spp. (n = 3) and Salmonella sp. (n = 1), were isolated from clinical specimens in Wenzhou Hospital of Traditional Chinese Medicine from March to June 2023. A single isolate per patient was collected. All isolates were re-identified by Matrix-Assisted Laser Desorption/Ionization Time-of-Flight Mass Spectrometry (MALDI-TOF MS; bioMérieux, Lyons, France) and utilized for methodological validation experiments of the three methods in the second stage of the study.
Antimicrobial susceptibility testing
The MICs of ciprofloxacin and levofloxacin were determined by the broth microdilution method (Nucien Pharmaceutical Co., Ltd. Guangzhou, China) and interpreted according to Clinical and Laboratory Standards Institute (CLSI)1 guidelines.
PCR and sequencing
The test strains and indicator organisms used in this study were all characterized at the molecular level by PCR and sequencing methods to identify quinolone resistance genes, including aac(6′)-Ib-cr, qnrA, qnrB, qnrC, qnrD, qnrS, qepA, as well as the quinolone resistance-determining regions of gyrA and parC. Bacterial DNA was extracted by the boiling method. The amplification reagents and primer designs were obtained from Shanghai Sangon Biotech Co., Ltd (Shanghai, China). Primers for these genes are shown in the Supplementary Table 1 (Kim et al., 2009; Lavilla et al., 2008; Nakano et al., 2019). Positive PCR products were sequenced by Sanger sequencing method in Shanghai Sangon Biotech Co., Ltd. The obtained DNA sequences were analyzed using the Blast program on the NCBI website2 to determine the genotypes.
Exploration of optimal conditions
In the first stage of the study, we aim to explore the optimal conditions for the three methods and show the experimental steps in Figure 1.
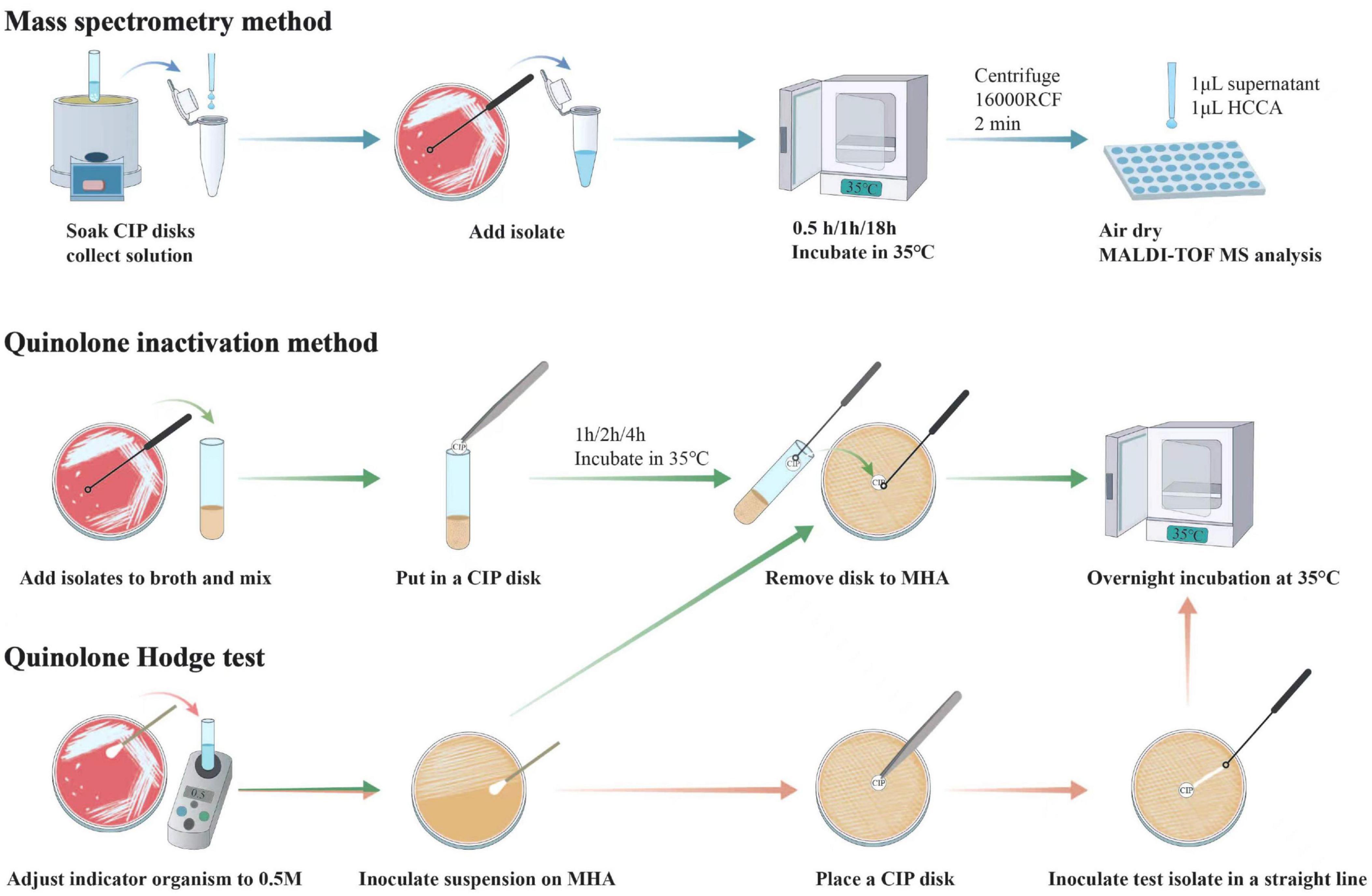
Figure 1. Workflows of mass spectrometry method, quinolone inactivation method and quinolone Hodge test. Each performance of mass spectrometry method and quinolone inactivation method required a concurrent control test which follows the same steps as the regular test, except that the test bacteria are not added. In addition, another variable for quinolone inactivation method and quinolone Hodge test is the four different indicator organisms, which are not annotated in the figure.
(i) Mass spectrometry method: We followed the method of Pardo et al. (2016) and Oviaño et al. (2017), and made some modifications. First, for reagent preparation, we diluted 100 ml:0.2 g ciprofloxacin injection (Nucien Pharmaceutical Co., Ltd. Guangzhou, China) with normal saline to obtain a concentration of 50 μg/mL. If the number of tests is small, the above solution could be obtained directly by immersing 5–7 ciprofloxacin disks (Kont Biology & Technology Co., Ltd. Wenzhou, China) in 2 mL normal saline. For analysis of bacteria, briefly, using a sterile inoculating loop, one colony was picked and suspended in 100 μL of the aforementioned CIP solution in an Eppendorf tube. Then, the mixture was vortexed for 10 s and incubated at 35°C for 30 min/1 h/18 h (with the incubation time being set as the variable of this method). At the end of incubation, 1 μL of the supernatant obtained after centrifugation was dropped onto a polished steel MALDI target plate, followed by direct overlay with 1 μL of MALDI-TOF matrix [10 mg/mL α-cyano-4-hydroxy-cinnamic acid (HCCA) in 50% acetonitrile and 0.1% trifluoroacetic acid (Bruker Daltonics GmbH, Bremen, Germany)]. The mixture was allowed to dry together. Spectra were acquired using the flexControl 3.3 software on a MALDI-TOF MS instrument (Bruker Daltonics GmbH, Bremen, Germany), with parameter settings: positive ion linear mode, mass range of 300∼600 Da, ion source 1 (IS1) at 10 kV, IS2 at 9.08 kV, lens at 3.00 kV, pulse ion extraction time of 10 ns, and laser frequency at 60 Hz. Additionally, a control experiment was conducted without the addition of any bacteria.
For data analysis, the relative molecular mass of ciprofloxacin (C17H18FN3O3) is 331, and the mass spectrometry peaks for [M+H+], [M+Na+], and [M+K+] appeared at 332, 354, and 370 Da, respectively, as shown in Figure 2. Upon acetylation by AAC(6′)-Ib-cr enzyme, N-acetylciprofloxacin (C19H20FN3O4) has gained an additional 42 Da, corresponding to peaks of 374, 396, and 412 Da for these three ionic adducts. Therefore, the presence of these three peaks indicating N-acetylated ciprofloxacin on the acquired spectral graph suggested that the tested bacteria were enzyme-producing strains.
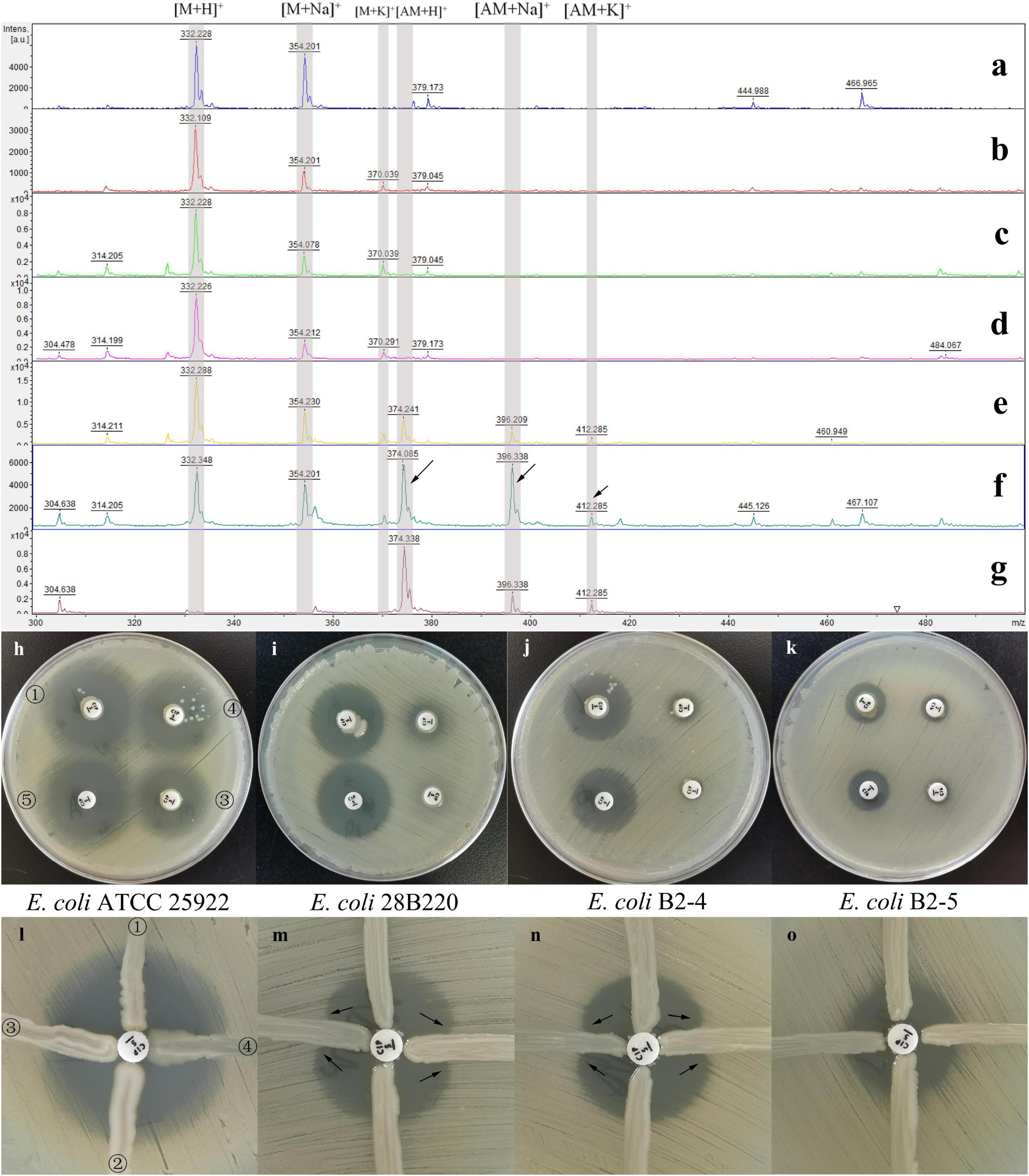
Figure 2. Results of the three methods under different conditions. Results of the mass spectrometry method (a–g), the quinolone inactivation method (h–k) and the quinolone Hodge test (l–o). MALDI-TOF MS spectra of ciprofloxacin after 0.5 h (b), 1 h (c), and 18 h (d) incubation with NAPE and after 0.5 h (e), 1 h (f), and 18 h (g) incubation with APE, and 1 h (a) incubation without bacteria. The spectral peaks at 322, 354, and 370 Da represent the three adducts of ciprofloxacin molecular ions: [M+H]+, [M+Na]+, and [M+K]+. The spectral peaks at 374, 396, and 412 Da represent the three adducts of acetylated ciprofloxacin molecular ions: [AM+H]+, [AM+Na]+, and [AM+K]+ (indicated by the arrows). E. coli ATCC 25922 (h, l), E. coli 28B220 [gyrA: S83L; (i, m)], E. coli B2-4 [gyrA: D87N; (j,n)] and E. coli B2-5 [gyrA: S83L, parC: S80I; (k,o)] were used as indicator organisms. Marked ①, ②, ③, ④, and ⑤ in the figures (h,l) are the results of NAPE, NAPE, APE, APE, and control test, respectively, and they correspond to the same positions on the other six plates. In figures (m,n), the curved growth of the indicator organisms on both sides of the test strains, indicated by the arrows, represents that the test strains are enzyme-producing strains.
(ii) Quinolone inactivation method: Briefly, using a sterile inoculation loop, 2–3 colonies of test isolate were added to a glass tube containing 1 mL of Mueller-Hinton Broth (Binhe Microbiological Reagents Co., Ltd. Hangzhou, China), followed by vortexing for 5–10 s (control test was performed without adding any bacteria). Next, a susceptibility-testing disk containing 5 μg ciprofloxacin (Kont Biology & Technology Co., Ltd. Wenzhou, China) was gently immersed at the bottom of the suspension and incubated at 35°C for 1 h/2 h/4 h (variable 1). Near the end of incubation, indicator organisms adjusted to 0.5 McFarland turbidity standard were inoculated onto Mueller-Hinton Agar (MHA; Autobio Biological Engineering Co., Ltd. Zhengzhou, China) plates by swabbing. In this study, we tested four indicator organisms (E. coli 28B220, B2-4, B2-5, and ATCC 25955, as previously described) as the second variable in the methodology. In addition, the antimicrobial susceptibility phenotypes and genetic characterizations of these organisms are detailed in Table 1. After incubation, the disk was carefully removed from the suspension using an inoculation loop and gently placed onto freshly inoculated MHA plates. The plates were then incubated overnight at 35°C. It is worth noting that when removing the disk, one should avoid dragging it along the inner wall of the glass tube, as condensate water on the wall may dilute the concentration of antibiotics adhering to the paper disks, leading to false-positive results. In addition, the control experiment followed the same steps except that no bacteria were added during incubation, as previously described.
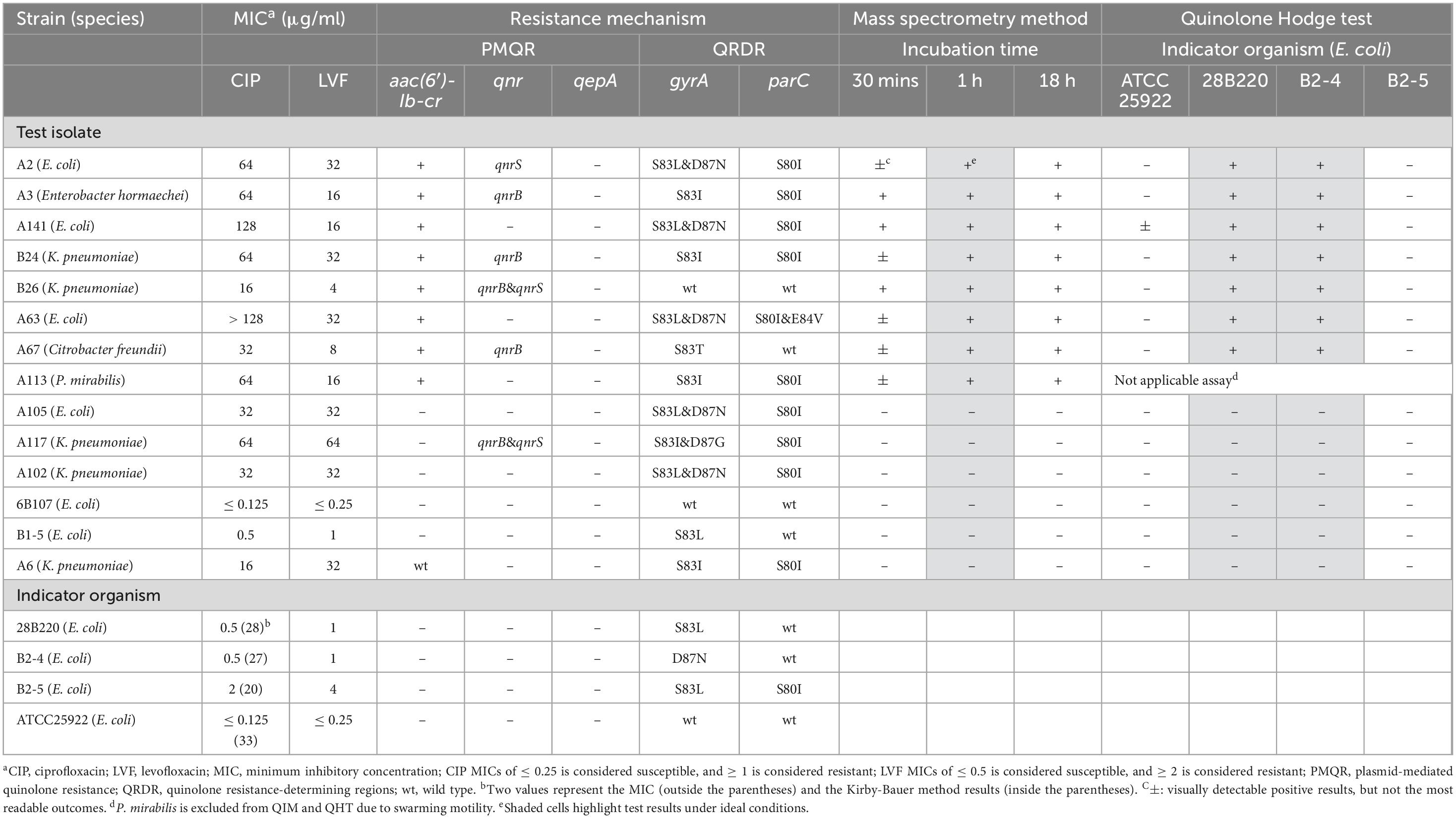
Table 1. Genotypes, quinolone susceptibility, and QHT results of strains involved in the first stage.
Next day, use a ruler to measure the diameter of the inhibition zone around each CIP disk. If the diameter of the inhibition zone in the test group was smaller than the control experiment by more than 5 mm, it was considered a positive result, i.e., the tested strain was APE. If the inhibition zone diameter was equal to or greater than the control experiment, it was considered as NAPE. If the value was within the range of the above two, it was considered an uncertain result. The selection of this cutoff value was based on the fact that, according to our repeated experimental experiences, conducting the test under optimal conditions resulted in most positive results (inhibition zone diameter) differing from the control experiment by more than 6 mm. Additionally, negative results always exhibited slightly larger diameters than the control experiment.
(iii) Quinolone Hodge test: First, the suspension of the indicator organisms (the four indicator organisms were the only variable for the method) was swabbed on the MHA plate as described previously. Next, a CIP disk was placed on the surface of the plate. Then, using a 10-μl loop, 1–2 monoclonal colonies of the tested bacteria were inoculated onto the plate in a straight line out from the edge of the disk, extending outward for a distance of 20–25 mm. Incubate overnight at 35°C. For the interpretation of the results, the production of AAC(6′)-Ib-cr was considered positive when the shape of inhibition zone on both sides of the tested strain was observed to bend toward the disk. Otherwise, the production of AAC(6′)-Ib-cr was considered negative when the shape of inhibition zone did not change.
Validation experiment
In the second part of the study, we evaluated the optimized procedure among the 187 blinded clinical isolates previously described. Specifically, under the optimal conditions of the three methods, 187 clinical isolates were tested by MSM, and 168 clinical isolates were tested by QIM and QHT. The prospectively collected strains were directly used for validation tests conducted every three days. Each experiment required one negative control and one positive control, which were selected from the APE and NAPE identified in the first phase of this study. It is worth mentioning that the 19 strains of Proteus mirabilis we collected were excluded from both QIM and QHT due to their swarming motility, which caused the entire plate to be covered and prevent observation of indicator results. Subsequently, PCR and sequencing of amplification products were performed to determine the presence of the aac(6′)-Ib-cr gene in each strain. Molecular characterization served as the gold standard to calculate the specificity and sensitivity of the three methods, evaluating their performance [Specificity = number of samples with negative results in both the method being evaluated and aac(6′)-Ib-cr gene detection / number of samples with negative results in gene detection; Sensitivity = number of samples with positive results in both the method being evaluated and gene detection / number of samples with positive results in gene detection].
Results
Antimicrobial susceptibility testing and molecular characterization of quinolone resistance genes
Antimicrobial Susceptibility Testing (AST) and the detection of quinolone resistance genes were conducted on 14 Enterobacteriaceae isolates and 3 indicator organisms. Among these, 8 isolates carried the aac(6′)-Ib-cr gene, 1 isolate carried wild-type aac(6′)-Ib, and the remaining 5 isolates did not carry this gene. Additionally, the 14 isolates were found to carry various combinations of quinolone resistance genes. The molecular characterization of the 3 indicator organisms was also re-validated. The AST results and resistance determinants for the above isolates are detailed in Table 1.
Mass spectrometry method
MS spectra showed the ion peaks corresponding to the ciprofloxacin molecular ions: [M+H]+ at 332 Da, its sodium adduct [M+Na]+ at 354 Da, and potassium adduct [M+K]+ at 370 Da. The acetylation of ciprofloxacin by AAC(6′)-Ib-cr resulted in a 42 Da shift, with the addition of three acetylation molecule peaks: [AM+H]+ at 374Da, [AM+Na]+ at 396.35 Da and [AM+K]+ at 412 Da (Figure 2).
For the evaluation of experimental variables, the incubation time significantly affects result readability. While a 30-min incubation suffices for visually detectable positive results, we found that a 1-h incubation yields the most readable outcomes, as briefly illustrated in Figures 2e–f. Upon 18 h of incubation, some positive results showed a complete disappearance of spectral peaks representing ciprofloxacin, as depicted in Figure 2g. Additionally, as expected, NAPE consistently lacked spectral peaks of the acetylated form even after 18 h of incubation. Furthermore, in the result of the control test (Figure 2a), the peak at 370 Da representing the ciprofloxacin potassium adduct was absent, whereas it appeared in the experimental groups. We believe that ciprofloxacin was dissolved in NaCl solution lacking potassium ions, which explains the absence of the 370 Da peak. Upon adding bacteria to the solution, potassium ions were partially extruded from bacterial cells into the solution via ion pumps, thereby resulting in weak spectral peaks representing potassium adducts (370 Da and 412 Da).
Quinolone inactivation method
To explore the optimal conditions for QIM, two variables influencing the outcomes were identified: the use of indicator organism E. coli 28B220 (gyrA: S83L) or E. coli B2-4 (gyrA: D87N), and the selection of a 2-h incubation time under which all AAC(6′)-Ib-cr enzyme-producing and non-producing isolates were correctly categorized based on the maximum difference in diameter of inhibition zone, as shown in Figure 3.
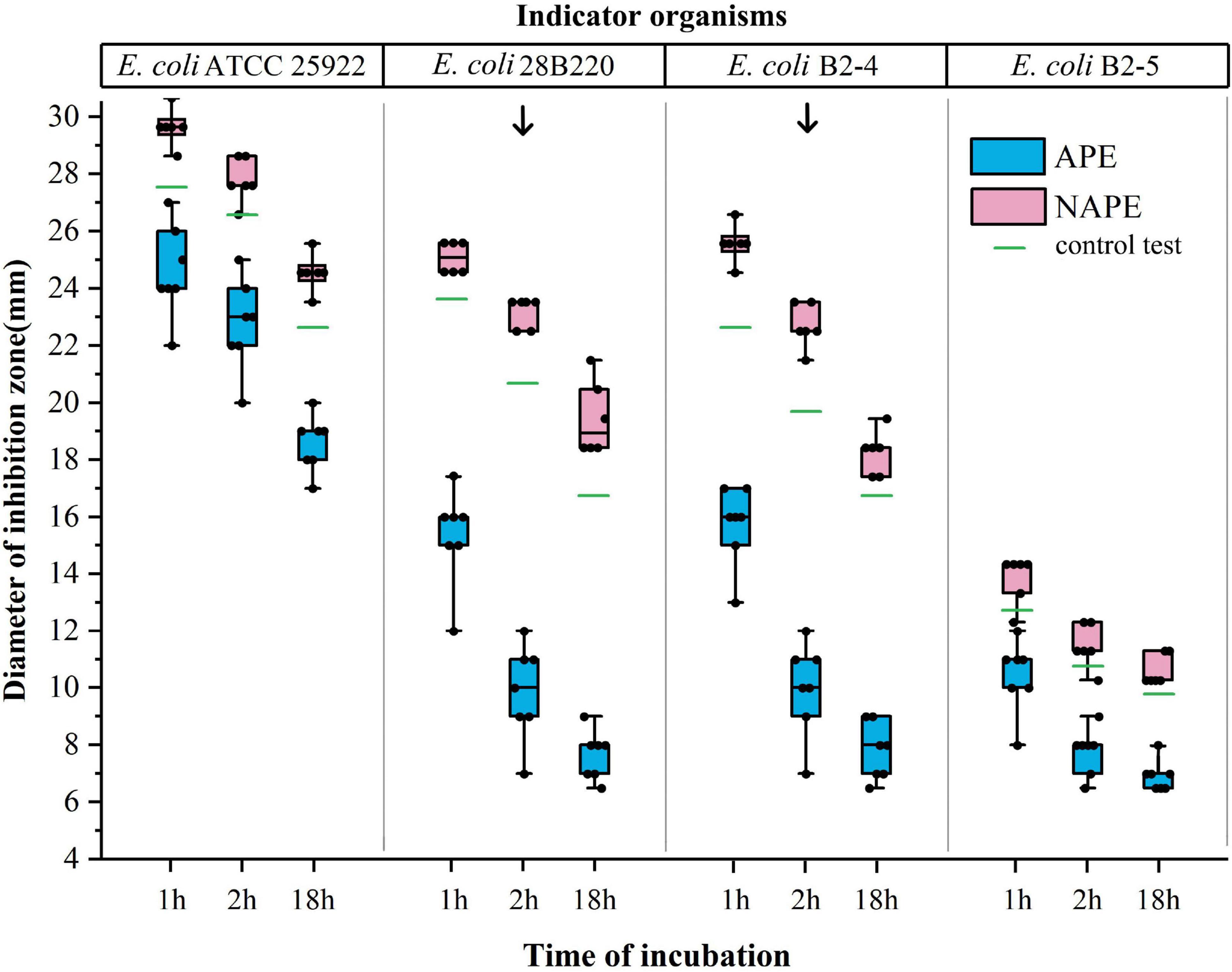
Figure 3. Effect of indicator organisms and incubation time on the inhibition zone diameter in the quinolone inactivation method. The x-axis represents the incubation time of the test bacterium with CIP disks, and the y-axis represents the inhibition zone diameter of four indicator organisms. Data of the blue boxes are from the results of APE, while data of the red boxes are from the results of NAPE. The green lines represent the results of the control tests. Arrows point to the test results under ideal conditions.
While studying the feasibility and accuracy of QIM, we observed in preliminary experiments that the indicator organism E. coli ATCC25922 was unsuitable for this method. Subsequently, we redesigned three indicator organisms and conducted comparative experiments involving the above four indicator strains in further studies. Through testing 14 isolates, we found that the difference in the inhibition zone diameters of APE and NAPE could not be effectively distinguished when either E. coli ATCC 25922 or E. coli B2-5 (gyrA: S83L, parC: S80I) was used as indicator organism, due to the inhibition zones being either too large or too small. However, when E. coli 28B220 or E. coli B2-4 was used as indicator organism, this difference became significantly pronounced. Moreover, the maximum difference occurred with a 2-h incubation time, as shown in Figures 2h–k.
Considering that the diversity of equipment and consumable specifications may affect the outcomes of this method, we did not set specific inhibition zone diameter values for positive and negative results. Instead, differentiation between positive and negative results was determined through concurrent control testing conducted during each trial. Values were set to ≤ 5 mm compared to the control test for positive results and ≥ the control test for negative results, as previously described. This difference was sufficient to distinguish between positive and negative results. In fact, this difference was above 6 mm for all tests under optimal conditions, as shown in Figure 3. Interestingly, the inhibition zone diameters of all NAPEs were slightly larger than those of the control test. Our explanation for it was that bacterial suspension adhered to the lifted disk, which increased the fluid’s surface tension due to bacterial particulates, resulting in greater absorption of residual antibiotics, a phenomenon that did not occur in the control test (Miller et al., 2017).
Quinolone Hodge test
We also tested the performance of the four indicator organisms in QHT. The results were similar to those in QIM. When E. coli ATCC 25922 was used as the indicator organism, the bending of the inhibition zone was too faint to distinguish between positive and negative results. In contrast, when using E. coli 28b220 or E. coli B2-4 instead, significantly enhanced growth of the indicator organism was observed on both sides of the APE streak, which was easily recognized by the naked eye. No such phenomenon occurred with NAPE (Figures 2l–o). Additionally, E. coli B2-5 was eliminated once again due to its initially small zone of inhibition.
Validation experiment
The results of PCR and sequencing of 187 clinical isolates used for method performance validation showed that a total of 46 APEs were screened in this prospective study, accounting for 24.6% (46/187), including E. coli (12/104), K. pneumoniae (18/47), P. mirabilis (13/19), Enterobacter spp. (1/3), Citrobacter spp. (2/4), and no APE was detected in the remaining genera (0/10). All APEs (including strains studied in the first phase) carried the aac(6′)-Ib-cr gene with both Trp102Arg and Asp179Tyr mutations, which means that no single-point mutation was found across all strains. This finding was consistent with earlier reports (Robicsek et al., 2006). Additionally, 7 isolates carrying the wild-type aac(6′)-Ib gene were detected. They exhibited identical assay results to non-AAC(6′)-Ib-cr enzyme producers in the validation study. Thus, the proportion of -cr variant among the total number of the gene was calculated as 86.8% (46/53) in this study.
Under optimal conditions, validation experiments were conducted for MSM, QIM, and QHT. The results indicated that MSM correctly identified all APEs and NAPEs with 100% specificity and sensitivity (141/141; 46/46). However, despite QIM and QHT also demonstrating 100% specificity (135/135) and sensitivity (33/33) compared to the genotype, Proteus mirabilis was excluded from these data. The data are detailed in Table 2.
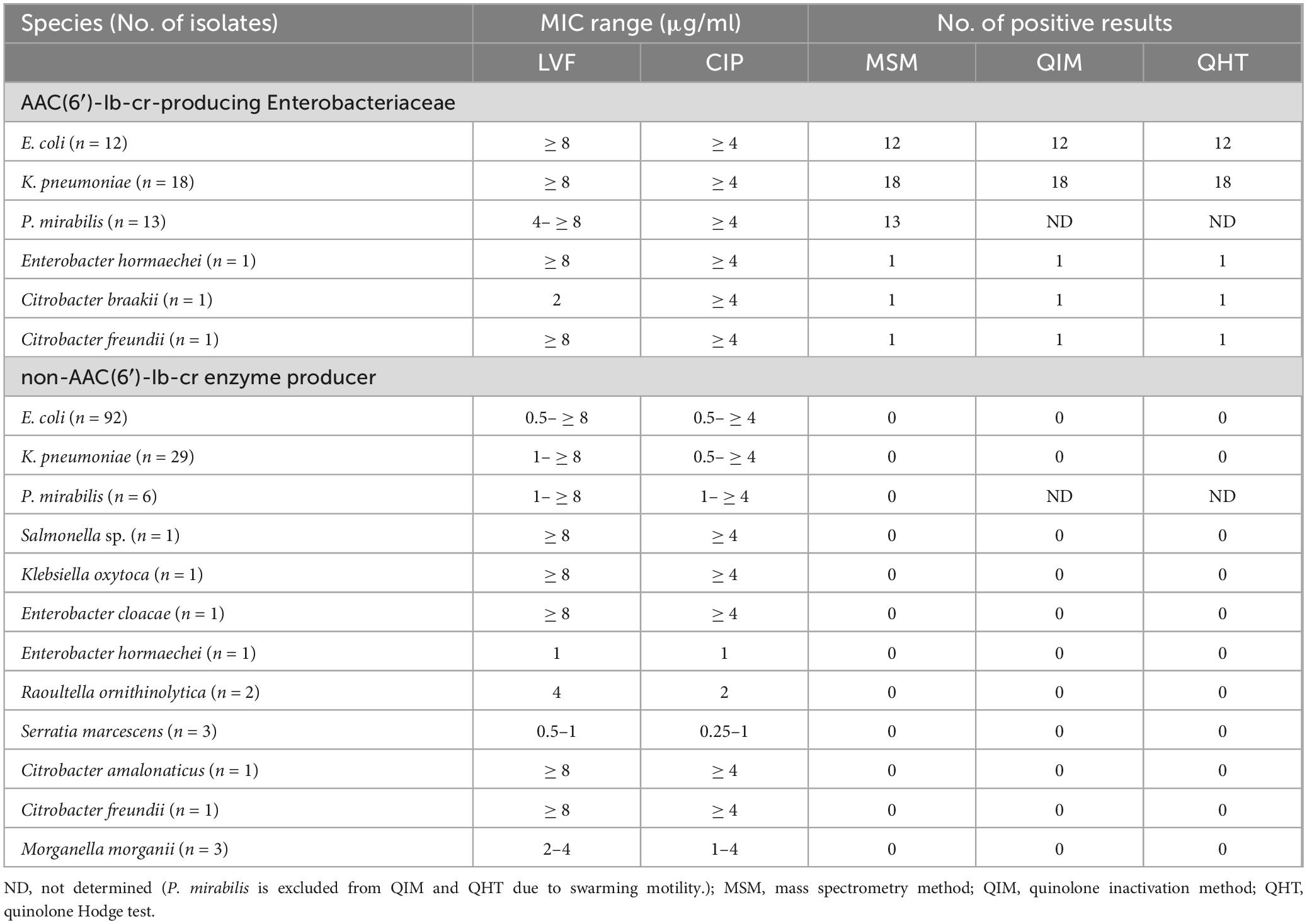
Table 2. Detection of AAC(6′)-Ib-cr enzyme producing clinical isolates using the three methods in this study.
Performance evaluation and selection guide of methods
We described the specificity, sensitivity, cost, required equipment, turnaround time, and test execution time of four APE detection methods in Table 3. And based on the experience gained from this work, we provide a step-by-step recommendation for selecting genotypic testing, MSM, QIM, or QHT based on existing resources. (i) If MALDI-TOF MS is available, MSM should be prioritized over genotypic testing. This is because the aac(6′)-Ib-cr gene cannot be detected by a PCR device alone without sequencing equipment. In fact, even in most hospitals of developed countries, only PCR devices are commonly available. More importantly, Phenotypic testing provides a more accurate assessment of clinical resistance compared to genotypic testing, which only detects the presence of aac(6′)-Ib-cr gene without accounting for the functional expression of it. (ii) In less developed areas, QHT can be chosen as an initial screening method, followed by QIM to recheck samples with subjective controversy in the screening results.
Discussion
The resistance phenotype to quinolones is typically insufficient to distinguish between PMQR and other resistance mechanisms (Rodríguez-Martínez et al., 2016; Strahilevitz et al., 2009). As the most prevalent PMQR (Guillard et al., 2014; Jomehzadeh et al., 2022; Shrestha et al., 2023; Tayh et al., 2024), AAC(6′)-Ib-cr confers a low level of quinolone resistance in host organisms, generally below the resistance breakpoints established by CLSI (Machuca et al., 2016; Robicsek et al., 2006; Ruiz, 2019), yet it poses a significantly grave clinical threat (Redgrave et al., 2014; Tacconelli et al., 2018). Several factors substantiate its significance in this process, including its increasing prevalence (Kuo et al., 2024; Zhan et al., 2021), association with other resistance elements (Dulyayangkul et al., 2024; Raherison et al., 2017), as well as the accelerated induction of mutations in QRDRs (Liang et al., 2023; Ortiz-Padilla et al., 2020; Recacha et al., 2019). Of concern is that clinical microbiology laboratories have not yet considered these genes in antimicrobial resistance screening, which may be attributed to the current lack of reliable screening assays for AAC(6′)-Ib-cr (Strahilevitz et al., 2009). In this study, we proposed three methods for detecting the AAC(6′)-Ib-cr enzyme in Enterobacteriaceae, all of which demonstrated an overall performance with 100% specificity and sensitivity. Additionally, all three methods cost less than $1 per test, and QIM and QHT did not require complex equipment and professional personnel to perform, as they were easy to operate and yield simple results. Furthermore, our experiments clearly showed that neither mutations in quinolone action target genes nor plasmid-mediated Qnr proteins would interfere with the determination of AAC(6′)-Ib-cr in these new methods. This is not difficult to explain. All tests are based on the acetylation modification activity of the AAC(6′)-Ib-cr enzyme toward ciprofloxacin, which is specific and independent among all mechanisms of resistance to quinolone(Robicsek et al., 2006; Ruiz et al., 2012).
In fact, the concept of using mass spectrometry for the detection of resistant enzymes was already commonplace and dated back to the early 2000s (Burckhardt and Zimmermann, 2011). Currently, it is mainly applied in the detection of carbapenemases (Alvarez-Buylla et al., 2013; Zhang et al., 2023). In contrast to carbapenemases, the detection of the AAC(6′)-Ib-cr enzyme by MS has an additional advantage, because the former result is explained by the complete disappearance of the spectral peaks representing ertapenem (Burckhardt and Zimmermann, 2011), which requires sufficient reaction time, while the latter result is explained by the appearance of new peaks representing the modified products, which are produced early in the reaction (Oviaño et al., 2017; Pardo et al., 2016). Therefore, although the activity of modifying enzymes is much lower than that of hydrolyzing enzymes, the incubation time required for AAC(6′)-Ib-cr detection is still shorter than that needed for carbapenemase detection. In addition, we found that MS was extremely sensitive to CIP detection, as clear ion peaks could be obtained with a 10 μg/ml CIP solution. This was why we believed that CIP reagents could be directly obtained by immersing CIP disks in normal saline. Such adjustments offered numerous benefits: simplifying reagent preparation, reducing costs, and the fact that antibiotic disks are often easy to store for a long time. Furthermore, preliminary research experience showed us that adding the matrix solution directly before the sample droplet dries did not affect the spectral peaks (allowing co-drying). Such a research was necessary because the drying time for sample droplets without surfactants was extremely long at room temperature. Adding the matrix solution could reduce the surface tension of the liquid, similar to the effect of surfactants, and greatly shorten the waiting time for drying (Miller et al., 2017; Wu et al., 2019).
The QIM and QHT methods were proposed for the first time in this study, and our objective was to design a low-cost, as minimally instrument-free method for the detection of the plasmid-mediated quinolone resistance determinant AAC(6′)-Ib-cr in Enterobacteriaceae. However, in our previous study (Yunxiang et al., 2015), we found that when E. coli ATCC 25922 was used as an indicator organism, it was only suitable for enzymes with strong hydrolytic activity, such as carbapenemases. Its performance was unsatisfactory in detecting aac(6′)-Ib-cr-mediated acetyltransferase. Therefore, through the comparative experiments involving four strains of E. coli with different degrees of QRDR mutations, we attempted to propose new insights into indicator organisms that played a critical role in the test.
The selection of an ideal indicator organism must meet two simple criteria: (i) its minimum inhibitory concentration (MIC) of ciprofloxacin should range between 0.25 to 1 μg/ml. (ii) in the Kirby-Bauer disk diffusion susceptibility test, the indicator organism which must be non-mucoid, should exhibit a clear boundary with an inhibition zone diameter of 28–30 mm. Such organisms, which are readily obtainable from clinical samples, share a common feature of a single-point mutation (S83L or D87N) in the gyrA gene, resulting in low-level resistance to quinolones. However, they still exhibit good susceptibility to ciprofloxacin in the Kirby-Bauer test (Machuca et al., 2016; Pham et al., 2019). Our approaches are based on the fact that the enzyme AAC(6′)-Ib-cr produced by the test strains modifies ciprofloxacin, reducing the drug’s effective concentration to such a level that the indicator organism with weak quinolone resistance is sufficient to protect itself, while the highly sensitive wild-type strain (E. coli ATCC 25922) remains difficult to survive (Robicsek et al., 2006). This principle is similar to that when a bacterium possesses both a gyrA mutation and plasmid-mediated AAC(6′)-Ib-cr enzyme, it always exhibits a higher level of resistance to quinolones (Machuca et al., 2016). We redesigned the indicator organism aiming to combine these two low-level resistance mechanisms to exhibit enhanced drug resistance effects in both QIM and QHT methods. Indeed, The combination of resistance mechanisms is also common to other classes of antibiotics. For example, the resistance of Pseudomonas aeruginosa to imipenem is due to a decrease in antibiotic uptake (via downregulation of OMPD2) and the production of AmpC-type β-lactamase (Livermore, 1992). Either mechanism alone is not sufficient to cause clinically significant levels of resistance, but only their combination results in high-level resistance. Therefore, as our experimental results showed, when E. coli ATCC25922 was used as the indicator organism, its indication effect for AAC(6′)-Ib-cr production was not significant. However, when it was replaced by strain E. coli 28B220, there would be an “illusion” of high level of resistance at the junction of the two bacteria (quinolone Hodge test), similar to that shown when the two resistance mechanisms worked together. When another resistance mechanisms is added, the inherently small inhibition zone may dilute the changes caused by the AAC(6′)-Ib-cr enzyme.
In addition, it was worth noting that the aac(6′)-Ib-cr genes carried by all 46 strains of APE in this study exhibited identical mutations at codons 102 (Trp-Arg) and 179 (Asp-Tyr). The remaining codons showed high similarity to the wild-type aac(6′)-Ib gene, and no independent occurrences of the W102R or D179Y mutations were observed. This was consistent with previous studies (Robicsek et al., 2006). We believed that the high sequence homology ensures relatively consistent enzyme activities of the encoded products (Vetting et al., 2008), which was the primary reason for the high performance of these enzyme activity methods. Therefore, compared to labor-intensive, time-consuming and expensive genotype testing, direct detection of AAC(6′)-Ib-cr enzyme activity represents a more cost-effective laboratory strategy. On the other hand, we observed that CIP was completely modified after incubation with APE for up to 18 h (no 322 Da peak was shown; Figure 2g). This compelled us to reassess the AAC(6′)-Ib-cr enzyme. To our knowledge, the concept of AAC(6′)-Ib-cr as a low-level resistance mechanism is based on in vitro experiments (Robicsek et al., 2006), which are inherently short-term studies. Thus, its prolonged presence in the human body and its sustained impact on antibiotic efficacy warrant re-evaluation.
There are some limitations to our study. Although all three methods demonstrated 100% specificity and sensitivity, real-world variability should be acknowledged. For example, we are still unable to explain whether a single mutation of the aac(6′)-Ib gene (W102R or D179Y) in strains might challenge these methods, whether our methods are applicable to non-fermenting Gram-negative bacilli, whether severe porin protein loss that prevents ciprofloxacin from entering the periplasmic space could lead to false negatives, and the variability between different laboratories.
In summary, this study presents three simple, efficient, and cost-effective methods for the detection of AAC(6′)-Ib-cr in Enterobacteriaceae. Each method has its own advantages: MSM is a rapid and highly accurate method for AAC(6′)-Ib-cr detection, but it relies on MALDI-TOF MS. QIM and QHT offer cost-effective alternatives but are unsuitable for P. mirabilis. Overall, these methods can enhance routine clinical screening for PMQR mechanisms.
Data availability statement
The original contributions presented in this study are included in this article/Supplementary material, further inquiries can be directed to the corresponding authors.
Ethics statement
This study was approved by the Ethics Committee of Wenzhou Hospital of Traditional Chinese Medicine, Zhejiang, China. The research was conducted in accordance with local legislation and institutional requirements. Written informed consent was obtained from all participants.
Author contributions
SL: Conceptualization, Data curation, Formal Analysis, Funding acquisition, Investigation, Methodology, Project administration, Resources, Software, Supervision, Validation, Visualization, Writing – original draft, Writing – review and editing. WC: Data curation, Investigation, Methodology, Project administration, Writing – review and editing. RM: Formal Analysis, Investigation, Methodology, Writing – review and editing. MC: Data curation, Investigation, Software, Supervision, Validation, Writing – review and editing. XD: Data curation, Formal Analysis, Writing – review and editing. XJ: Resources, Writing – review and editing. WK: Conceptualization, Data curation, Funding acquisition, Investigation, Methodology, Resources, Writing – review and editing.
Funding
The authors declare that financial support was received for the research and/or publication of this article. This work was supported by the Science and Technology Planning Project of Wenzhou (Grant Nos. Y2023770 and Y20210744 to SL).
Conflict of interest
The authors declare that the research was conducted in the absence of any commercial or financial relationships that could be construed as a potential conflict of interest.
Generative AI statement
The authors declare that no Generative AI was used in the creation of this manuscript.
Publisher’s note
All claims expressed in this article are solely those of the authors and do not necessarily represent those of their affiliated organizations, or those of the publisher, the editors and the reviewers. Any product that may be evaluated in this article, or claim that may be made by its manufacturer, is not guaranteed or endorsed by the publisher.
Supplementary material
The Supplementary Material for this article can be found online at: https://www.frontiersin.org/articles/10.3389/fmicb.2025.1513425/full#supplementary-material
Footnotes
References
Ahmed, A., and Daneshtalab, M. (2012). Nonclassical biological activities of quinolone derivatives. J. Pharm. Pharm. Sci. 15, 52–72. doi: 10.18433/j3302n
Al-Gallas, N., Fadel, M., Altammar, K., Awadi, Y., and Aissa, R. (2024). Pathovars, occurrence, and characterization of plasmid-mediated quinolone resistance in diarrheal Escherichia coli isolated from farmers and farmed chickens in Tunisia and Nigeria. Lett. Appl. Microbiol. 77:ovae043. doi: 10.1093/lambio/ovae043
Al-Khafaji, N., Almjalawi, B., Ewadh, R., Al-Dahmoshi, H., Abed, S., Nasrolahi, A., et al. (2024). Prevalence of plasmid-mediated quinolone resistance genes and biofilm formation in different species of quinolone-resistant clinical Shigella isolates: A cross-sectional study. Eur. J. Med. Res. 29:419. doi: 10.1186/s40001-024-02007-y
Alvarez-Buylla, A., Picazo, J. J., and Culebras, E. (2013). Optimized method for Acinetobacter species carbapenemase detection and identification by matrix-assisted laser desorption ionization-time of flight mass spectrometry. J. Clin. Microbiol. 51, 1589–1592. doi: 10.1128/JCM.00181-13
Ball, P., Fernald, A., and Tillotson, G. (1998). Therapeutic advances of new fluoroquinolones. Expert. Opin. Investig. Drugs 7, 761–783. doi: 10.1517/13543784.7.5.761
Burckhardt, I., and Zimmermann, S. (2011). Using matrix-assisted laser desorption ionization-time of flight mass spectrometry to detect carbapenem resistance within 1 to 2.5 hours. J. Clin. Microbiol. 49, 3321–3324. doi: 10.1128/JCM.00287-11
Darby, E., Trampari, E., Siasat, P., Gaya, M., Alav, I., Webber, M., et al. (2023). Molecular mechanisms of antibiotic resistance revisited. Nat. Rev. Microbiol. 21, 280–295. doi: 10.1038/s41579-022-00820-y
de Oliveira Alves, W., Scavuzzi, A., Beltrão, E., de Oliveira, ÉM., Dos Santos Vasconcelos, C. R., and Rezende, A. M. (2022). Occurrence of blaNDM-7 and association with blaKPC-2, blaCTX-M15, aac, aph, mph(A), catB3 and virulence genes in a clinical isolate of Klebsiella pneumoniae with different plasmids in Brazil. 204:459. doi: 10.1007/s00203-022-03051-0
Dulyayangkul, P., Beavis, T., Lee, W., Ardagh, R., Edwards, F., Hamilton, F., et al. (2024). Harvesting and amplifying gene cassettes confers cross-resistance to critically important antibiotics. PLoS Pathog. 20:e1012235. doi: 10.1371/journal.ppat.1012235
Ellabaan, M., Munck, C., Porse, A., Imamovic, L., and Sommer, M. (2021). Forecasting the dissemination of antibiotic resistance genes across bacterial genomes. Nat. Commun. 12:2435. doi: 10.1038/s41467-021-22757-1
Golden, A., Karlowsky, J., Walkty, A., Baxter, M., Denisuik, A., McCracken, M., et al. (2021). Comparison of phenotypic antimicrobial susceptibility testing results and WGS-derived genotypic resistance profiles for a cohort of ESBL-producing Escherichia coli collected from Canadian hospitals: Canward 2007-18. J. Antimicrob. Chemother. 76, 2825–2832. doi: 10.1093/jac/dkab268
Guillard, T., Bertrand, X., de Champs, C., Cholley, P., Bajolet, O., and Gbaguidi-Haore, H. (2014). aac(6′)-Ib-cr is the major plasmid-mediated quinolone resistance determinant in extended-spectrum β-lactamase-producing Escherichia coli in eastern France. J. Glob. Antimicrob. Resist. 2, 111–113. doi: 10.1016/j.jgar.2014.01.006
Gupta, K., Hooton, T., Naber, K., Wullt, B., Colgan, R., Miller, L., et al. (2011). International clinical practice guidelines for the treatment of acute uncomplicated cystitis and pyelonephritis in women: A 2010 update by the infectious diseases society of america and the European society for microbiology and infectious diseases. Clin. Infect. Dis. 52, e103–e120. doi: 10.1093/cid/ciq257
Hidalgo-Grass, C., and Strahilevitz, J. (2010). High-resolution melt curve analysis for identification of single nucleotide mutations in the quinolone resistance gene aac(6′)-Ib-cr. Antimicrob. Agents Chemother. 54, 3509–3511. doi: 10.1128/AAC.00485-10
Hodge, W., Ciak, J., and Tramont, E. C. (1978). Simple method for detection of penicillinase-producing Neisseria gonorrhoeae. J. Clin. Microbiol. 7, 102–103. doi: 10.1128/jcm.7.1.102-103.1978
Hrovat, K., Molan, K., Seme, K., and Ambrožič Avguštin, J. (2019). Molecular characterization of extended-spectrum β-lactamase-producing Escherichia coli isolated from lower respiratory tract samples between 2002 and 2019 in the Central Slovenia region. Ann. Clin. Microbiol. Antimicrob. 23:6. doi: 10.1186/s12941-023-00664-1
Jomehzadeh, N., Saki, M., Ahmadi, K., and Zandi, G. (2022). The prevalence of plasmid-mediated quinolone resistance genes among Escherichia coli strains isolated from urinary tract infections in southwest Iran. Mol. Biol. Rep. 49, 3757–3763. doi: 10.1007/s11033-022-07215-5
Kim, H., Park, C., Kim, C., Kim, E., Jacoby, G., and Hooper, D. (2009). Prevalence of plasmid-mediated quinolone resistance determinants over a 9-year period. Antimicrob. Agents Chemother. 53, 639–645. doi: 10.1128/AAC.01051-08
Kuo, P., Lin, W., Tang, S., Cheng, Y., Bregente, C., Thi Thuy Duong, T., et al. (2024). A longitudinal epidemiology study of fluoroquinolone-nonsusceptible Klebsiella pneumoniae reveals an increasing prevalence of qnrB and qnrS in Taiwan. J. Infect. Public Health 17, 457–463. doi: 10.1016/j.jiph.2024.01.005
Lavilla, S., González-López, J., Sabaté, M., García-Fernández, A., Larrosa, M., Bartolomé, R., et al. (2008). Prevalence of qnr genes among extended-spectrum beta-lactamase-producing enterobacterial isolates in Barcelona, Spain. J. Antimicrob. Chemother. 61, 291–295. doi: 10.1093/jac/dkm448
Lee, K., Chong, Y., Shin, H., Kim, Y., Yong, D., and Yum, J. (2001). Modified Hodge and EDTA-disk synergy tests to screen metallo-beta-lactamase-producing strains of Pseudomonas and Acinetobacter species. Clin. Microbiol. Infect. 7, 88–91. doi: 10.1046/j.1469-0691.2001.00204.x
Liang, H., Zhang, J., Hu, J., Li, X., and Li, B. (2023). Fluoroquinolone residues in the environment rapidly induce heritable fluoroquinolone resistance in Escherichia coli. Environ. Sci. Technol. 57, 4784–4795. doi: 10.1021/acs.est.2c04999
Livermore, D. (1992). Interplay of impermeability and chromosomal beta-lactamase activity in imipenem-resistant Pseudomonas aeruginosa. Antimicrob. Agents Chemother. 36, 2046–2048. doi: 10.1128/AAC.36.9.2046
Logan, L., Coy, L., Pitstick, C., Marshall, S., Medernach, R., Domitrovic, T., et al. (2023). The role of the plasmid-mediated fluoroquinolone resistance genes as resistance mechanisms in pediatric infections due to Enterobacterales. Front. Cell. Infect. Microbiol. 13:1249505. doi: 10.3389/fcimb.2023.1249505
Logan, L., Medernach, R., Rispens, J., Marshall, S., Hujer, A., Domitrovic, T., et al. (2019). Community origins and regional differences highlight risk of plasmid-mediated fluoroquinolone resistant Enterobacteriaceae infections in children. Pediatr. Infect. Dis. J. 38, 595–599. doi: 10.1097/INF.0000000000002205
Machuca, J., Ortiz, M., Recacha, E., Díaz-De-Alba, P., Docobo-Perez, F., Rodríguez-Martínez, J., et al. (2016). Impact of AAC(6′)-Ib-cr in combination with chromosomal-mediated mechanisms on clinical quinolone resistance in Escherichia coli. J. Antimicrob. Chemother. 71, 3066–3071. doi: 10.1093/jac/dkw258
Masoud, S. M., Abd, E. R., Aly, S. A., and Ibrahem, R. A. (2021). Co-Existence of certain ESBLs, MBLs and plasmid mediated quinolone resistance genes among MDR E. coli isolated from different clinical specimens in Egypt. Antibiotics 10:835. doi: 10.3390/antibiotics10070835
Maurice, F., Broutin, I., Podglajen, I., Benas, P., Collatz, E., and Dardel, F. (2008). Enzyme structural plasticity and the emergence of broad-spectrum antibiotic resistance. EMBO Rep. 9, 344–349. doi: 10.1038/embor.2008.9
Mercuro, N., Kenney, R., Abreu-Lanfranco, O., and Davis, S. (2018). Ambulatory quinolone prescribing: Moving from opportunity to implementation. Clin. Infect. Dis. 67, 1306–1307. doi: 10.1093/cid/ciy315
Miller, R., Aksenenko, E. V., and Fainerman, V. B. (2017). Dynamic interfacial tension of surfactant solutions. Adv Colloid Interface Sci. 247, 115–129. doi: 10.1016/j.cis.2016.12.007
Mounsey, O., Schubert, H., Findlay, J., Morley, K., Puddy, E., Gould, V., et al. (2021). Limited phylogenetic overlap between fluoroquinolone-resistant Escherichia coli isolated on dairy farms and those causing bacteriuria in humans living in the same geographical region. J. Antimicrob. Chemother. 76, 3144–3150. doi: 10.1093/jac/dkab310
Nakano, R., Nakano, A., Abe, M., Nagano, N., Asahara, M., Furukawa, T., et al. (2019). Prevalence and mechanism of fluoroquinolone resistance in clinical isolates of Proteus mirabilis in Japan. Heliyon 5:e01291. doi: 10.1016/j.heliyon.2019.e01291
Ortiz-Padilla, M., Diaz-Diaz, S., Machuca, J., Tejada-Gonzalez, A., Recacha, E., Docobo-Pérez, F., et al. (2020). Role of low-level quinolone resistance in generating tolerance in Escherichia coli under therapeutic concentrations of ciprofloxacin. J. Antimicrob. Chemother. 75, 2124–2132. doi: 10.1093/jac/dkaa151
Oviaño, M., Rodríguez-Martínez, J., Pascual, Á, and Bou, G. (2017). Rapid detection of the plasmid-mediated quinolone resistance determinant AAC(6′)-Ib-cr in Enterobacteriaceae by MALDI-TOF MS analysis. J. Antimicrob. Chemother. 72, 1074–1080. doi: 10.1093/jac/dkw552
Pardo, C., Tan, R., Hennequin, C., Beyrouthy, R., Bonnet, R., and Robin, F. (2016). Rapid detection of AAC(6′)-Ib-cr production using a MALDI-TOF MS strategy. Eur. J. Clin. Microbiol. Infect. Dis. 35, 2047–2051. doi: 10.1007/s10096-016-2762-1
Pasteran, F., Gonzalez, L., Albornoz, E., Bahr, G., Vila, A., and Corso, A. (2016). Triton hodge test: Improved protocol for modified hodge test for enhanced detection of NDM and other Carbapenemase producers. J. Clin. Microbiol. 54, 640–649. doi: 10.1128/JCM.01298-15
Patel, V., Patil, K., Patel, D., Kikani, B., Madamwar, D., and Desai, C. (2024). Distribution of bacterial community structures and spread of antibiotic resistome at industrially polluted sites of Mini river, Vadodara, Gujarat, India. Environ. Monit. Assess. 196:208. doi: 10.1007/s10661-024-12380-0
Pham, T., Ziora, Z., and Blaskovich, M. (2019). Quinolone antibiotics. Medchemcomm 10, 1719–1739. doi: 10.1039/c9md00120d
Phan, M., Peters, K., Alvarez Fraga, L., Wallis, S., Hancock, S., Nhu, N., et al. (2022). Plasmid-mediated ciprofloxacin resistance imparts a selective advantage on Escherichia coli ST131. Antimicrob. Agents Chemother. 66:e0214621. doi: 10.1128/AAC.02146-21
Piccirilli, A., Cherubini, S., Azzini, A., Tacconelli, E., Lo Cascio, G., Maccacaro, L., et al. (2021). Whole-genome sequencing (WGS) of carbapenem-resistant K. pneumoniae Isolated in long-term care facilities in the Northern Italian region. Microorganisms 9:1985. doi: 10.3390/microorganisms9091985
Pierce, V., Simner, P., Lonsway, D., Roe-Carpenter, D., Johnson, J., Brasso, W., et al. (2017). Modified carbapenem inactivation method for phenotypic detection of carbapenemase production among Enterobacteriaceae. J. Clin. Microbiol. 55, 2321–2333. doi: 10.1128/JCM.00193-17
Pilatz, A., Dimitropoulos, K., Veeratterapillay, R., Yuan, Y., Omar, M., MacLennan, S., et al. (2020). Antibiotic prophylaxis for the prevention of infectious complications following prostate biopsy: A systematic review and meta-analysis. J. Urol. 204, 224–230. doi: 10.1097/JU.0000000000000814
Pitout, J., Peirano, G., Chen, L., DeVinney, R., and Matsumura, Y. (2022). Escherichia coli ST1193: Following in the Footsteps of E. coli ST131. Antimicrob Agents Chemother. 66:e51122. doi: 10.1128/aac.00511-22
Pitout, J., Wei, Y., Church, D., and Gregson, D. (2008). Surveillance for plasmid-mediated quinolone resistance determinants in Enterobacteriaceae within the Calgary Health Region, Canada: The emergence of aac(6′)-Ib-cr. J. Antimicrob. Chemother. 61, 999–1002. doi: 10.1093/jac/dkn068
Raherison, S., Jove, T., Gaschet, M., Pinault, E., Tabesse, A., Torres, C., et al. (2017). Expression of the aac(6′)-Ib-cr gene in class 1 integrons. Antimicrob. Agents Chemother. 61, e2704–e2716. doi: 10.1128/AAC.02704-16
Recacha, E., Machuca, J., Díaz-Díaz, S., García-Duque, A., Ramos-Guelfo, M., Docobo-Pérez, F., et al. (2019). Suppression of the SOS response modifies spatiotemporal evolution, post-antibiotic effect, bacterial fitness and biofilm formation in quinolone-resistant Escherichia coli. J. Antimicrob. Chemother. 74, 66–73. doi: 10.1093/jac/dky407
Redgrave, L., Sutton, S., Webber, M., and Piddock, L. (2014). Fluoroquinolone resistance: mechanisms, impact on bacteria, and role in evolutionary success. Trends Microbiol. 22, 438–445. doi: 10.1016/j.tim.2014.04.007
Robicsek, A., Strahilevitz, J., Jacoby, G., Macielag, M., Abbanat, D., Park, C., et al. (2006). Fluoroquinolone-modifying enzyme: A new adaptation of a common aminoglycoside acetyltransferase. Nat. Med. 12, 83–88. doi: 10.1038/nm1347
Rodríguez-Martínez, J., López-Cerero, L., Díaz-de-Alba, P., Chamizo-López, F., Polo-Padillo, J., and Pascual, Á (2016). Assessment of a phenotypic algorithm to detect plasmid-mediated quinolone resistance in Enterobacteriaceae. J. Antimicrob. Chemother. 71, 845–847. doi: 10.1093/jac/dkv392
Ruiz, J. (2019). Transferable mechanisms of quinolone resistance from 1998 onward. Clin. Microbiol. Rev. 32:e00007-19. doi: 10.1128/CMR.00007-19
Ruiz, J., Pons, M. J., and Gomes, C. (2012). Transferable mechanisms of quinolone resistance. Int. J. Antimicrob. Agents 40, 196–203. doi: 10.1016/j.ijantimicag.2012.02.011
Sanchez, G., Babiker, A., Master, R., Luu, T., Mathur, A., and Bordon, J. (2016). Antibiotic resistance among urinary isolates from female outpatients in the United States in 2003 and 2012. Antimicrob. Agents Chemother. 60, 2680–2683. doi: 10.1128/AAC.02897-15
Shapiro, D. J., Hicks, L. A., Pavia, A. T., and Hersh, A. L. (2014). Antibiotic prescribing for adults in ambulatory care in the USA, 2007-09. J. Antimicrob. Chemother. 69, 234–240. doi: 10.1093/jac/dkt301
Shrestha, R., Thapa, A., Shrestha, D., Pokhrel, S., Aryal, A., Adhikari, R., et al. (2023). Characterization of transferrable mechanisms of quinolone resistance (TMQR) among quinolone-resistant Escherichia coli and Klebsiella pneumoniae causing Urinary tract infection in nepalese children. BMC Pediatr. 23:458. doi: 10.1186/s12887-023-04279-5
Sohrabi, M., Fathi, J., Mohebi, S., Hashemizadeh, Z., Kholdi, S., Hadadi, M., et al. (2024). High prevalence of plasmid-mediated quinolone resistance in escherichia coli strains producing extended-spectrum beta-lactamases isolated from faeces and urine of pregnant women with acute cystitis. Mol. Biol. Rep. 51:566. doi: 10.1007/s11033-024-09491-9
Strahilevitz, J., Jacoby, G. A., Hooper, D. C., and Robicsek, A. (2009). Plasmid-mediated quinolone resistance: A multifaceted threat. Clin. Microbiol. Rev. 22, 664–689. doi: 10.1128/CMR.00016-09
Swedan, S., Alabdallah, E., and Ababneh, Q. (2023). Resistance to aminoglycoside and quinolone drugs among Klebsiella pneumoniae clinical isolates from northern Jordan. Heliyon 10:e23368. doi: 10.1016/j.heliyon.2023.e23368
Tacconelli, E., Carrara, E., Savoldi, A., Harbarth, S., Mendelson, M., Monnet, D., et al. (2018). Discovery, research, and development of new antibiotics: The WHO priority list of antibiotic-resistant bacteria and tuberculosis. Lancet Infect. Dis. 18, 318–327. doi: 10.1016/S1473-3099(17)30753-3
Taplitz, R., Kennedy, E., Bow, E., Crews, J., Gleason, C., Hawley, D., et al. (2018). Antimicrobial prophylaxis for adult patients with cancer-related immunosuppression: asco and IDSA clinical practice guideline update. J. Clin Oncol. 36, 3043–3054. doi: 10.1200/jco.18.00374
Tayh, G., Fhoula, I., Said, M., Boudabous, A., and Slama, K. (2024). Prevalence and characterization of quinolone resistance and integrons in clinical Gram-negative isolates from Gaza strip, Palestine. Mol. Biol. Rep. 51:855. doi: 10.1007/s11033-024-09721-0
van der Zwaluw, K., de Haan, A., Pluister, G., Bootsma, H., de Neeling, A., and Schouls, L. (2015). The carbapenem inactivation method (CIM), a simple and low-cost alternative for the Carba NP test to assess phenotypic carbapenemase activity in gram-negative rods. PLoS One 10:e0123690. doi: 10.1371/journal.pone.0123690
Vetting, M., Park, C., Hegde, S., Jacoby, G., Hooper, D., and Blanchard, J. (2008). Mechanistic and structural analysis of aminoglycoside N-acetyltransferase AAC(6′)-Ib and its bifunctional, fluoroquinolone-active AAC(6′)-Ib-cr variant. Biochemistry 47, 9825–9835. doi: 10.1021/bi800664x
Wareham, D., Umoren, I., Khanna, P., and Gordon, N. (2010). Allele-specific polymerase chain reaction (PCR) for rapid detection of the aac(6′)-Ib-cr quinolone resistance gene. Int. J. Antimicrob. Agents 36, 476–477. doi: 10.1016/j.ijantimicag.2010.07.012
Wu, M., Di, Y., Man, X., and Doi, M. (2019). Drying droplets with soluble surfactants. Langmuir 35, 14734–14741. doi: 10.1021/acs.langmuir.9b02229
Yunxiang, Z., Chen, Y., Shen, L., Jin, X., Xu, J., Lang, S., et al. (2015). The study of genotype and plasmid transfer of carbapenem-resistant Enterobacteriaceae carrying blaNDM-1 with blaIMP-4 or blaKPC-2. Chin. J. Infect. Dis. 9, 542–549. doi: 10.3760/cma.j.issn.1000-6680.2015.09.007
Zahra, Q., Gul, J., Shah, A. R., Yasir, M., and Karim, A. M. (2023). Antibiotic resistance genes prevalence prediction and interpretation in beaches affected by urban wastewater discharge. One Health 17:100642. doi: 10.1016/j.onehlt.2023.100642
Zhan, Q., Xu, Y., Wang, B., Yu, J., Shen, X., Liu, L., et al. (2021). Distribution of fluoroquinolone resistance determinants in Carbapenem-resistant Klebsiella pneumoniae clinical isolates associated with bloodstream infections in China. BMC Microbiol. 21:164. doi: 10.1186/s12866-021-02238-7
Zhang, Y., Tsao, M., Chang, C., Lin, K., Keller, J., and Lin, H. (2023). Rapid identification of carbapenem-resistant Klebsiella pneumoniae based on matrix-assisted laser desorption ionization time-of-flight mass spectrometry and an artificial neural network model. J. Biomed. Sci. 30:25. doi: 10.1186/s12929-023-00918-2
Keywords: AAC(6′)-Ib-cr, PMQR, quinolone, antimicrobial resistance, Enterobacteriaceae
Citation: Liang S, Cai W, Mao R, Chen M, Dai X, Jin X and Kong W (2025) Three simple and cost-effective assays for AAC(6′)-Ib-cr enzyme activity. Front. Microbiol. 16:1513425. doi: 10.3389/fmicb.2025.1513425
Received: 18 October 2024; Accepted: 03 April 2025;
Published: 25 April 2025.
Edited by:
Arpit Shukla, University College Cork, IrelandReviewed by:
Santi M. Mandal, Indian Institute of Technology Kharagpur, IndiaNagwan El Menofy, Al-Azhar University, Egypt
Dhara Patel, Institute of Advanced Research, India
Copyright © 2025 Liang, Cai, Mao, Chen, Dai, Jin and Kong. This is an open-access article distributed under the terms of the Creative Commons Attribution License (CC BY). The use, distribution or reproduction in other forums is permitted, provided the original author(s) and the copyright owner(s) are credited and that the original publication in this journal is cited, in accordance with accepted academic practice. No use, distribution or reproduction is permitted which does not comply with these terms.
*Correspondence: Wanzhong Kong, MjI0NTI2NDJAcXEuY29t; Shizhou Liang, NjM5NDExNjk0QHFxLmNvbQ==