- 1Engineering Research Center for Horticultural Crop Germplasm Creation and New Variety Breeding, Ministry of Education, Key Laboratory of Vegetable Biology of Hunan Province, College of Horticulture, Hunan Agricultural University, Changsha, Hunan, China
- 2State Key Laboratory for Biology of Plant Diseases and Insect Pests, Institute of Plant Protection, Chinese Academy of Agricultural Sciences, Beijing, China
Introduction: Allyl isothiocyanate (AITC) has demonstrated efficacy as a soil fumigant, effectively controlling soil-borne pathogens and nematodes. Although AITC has a significant effect on soil microbial communities, whether fumigation affects the production of crop endophytes is unclear.
Methods: In this study, AITC was used to fumigate the soil, and the response of endophytic bacteria (in roots, stems, and leaves) in different pepper genotypes (Xiangla359, La Xuan, Shuang Jiao) was investigated.
Results: Fumigation with AITC significantly increased soil microbial diversity, stimulated the growth of Actinomycetota, and inhibited Pseudomonadota. However, the effects on endophytic bacteria varied among pepper varieties. Specifically, fumigation significantly reduced microbial diversity in the roots and leaves of Xiangla359, but had no significant effect on La Xuan and Shuang Jiao. Furthermore, the growth-promoting effect of AITC was most pronounced in Xiangla359.
Conclusion: Our results suggest that while AITC fumigation significantly alters soil microbial diversity and composition, its effects on crop endophytes are genotype-dependent. These findings provide insight into the complex interactions between soil microbial communities and crop endophytes in response to soil fumigation.
1 Introduction
Peppers (Capsicum annuum L.), esteemed for their piquant flavor and robust nutritional profile, belong to the Solanaceae family and are renowned for their abundant polyphenols, capsaicinoids, ascorbic acid, and other phytochemicals (Barboza et al., 2022). In China, peppers are among the most extensively cultivated vegetables, with the 2021 cultivated area exceeding 213 ha, representing approximately 10% of the total vegetable cultivation acreage. The expansion of protected cultivation has driven a remarkable surge in vegetable yields; however, this progress has been paralleled by the accumulation of soil pathogens and exacerbation of soil-borne diseases, thereby impeding agricultural advancement (Ren et al., 2018). Consequently, addressing the persistent crop disorders in peppers and enhancing their yield constitute pivotal concerns in contemporary agricultural practices.
Soil fumigation represents the most direct and effective strategy for addressing the challenges associated with crop recolonization (Mao et al., 2014). Among the various fumigants, allyl isothiocyanate (AITC) stands out as a high-quality, plant-derived agent known for its efficacy in preventing and eradicating a range of soil-borne pathogenic nematodes (Dahlin and Hallmann, 2020; Ren et al., 2018), pathogenic fungi (Yu et al., 2019) and weeds (Zhang Y. et al., 2023). Additionally, AITC effectively inhibits pests during storage (Wu et al., 2009) and mitigates postharvest diseases (Otoni et al., 2014; Ugolini et al., 2014) all while maintaining a high safety profile and leaving no pesticide residues.
Recent studies have demonstrated that AITC significantly inhibits the development of gray mold in tomatoes, thereby extending their storage life (Barea-Ramos et al., 2024). Furthermore, AITC has been shown to effectively mitigate postharvest quality deterioration in edible mushrooms and raspberries (Park et al., 2023; Zou et al., 2024). Yu et al.’s (2022) research revealed that the inhibitory effect of AITC on C. heterostrophus was linked to the downregulation of genes associated with energy metabolism, oxidoreductase activity, melanin biosynthesis, and cell wall-degrading enzymes, resulting in a significant reduction in melanin production in C. heterostrophus. Moreover, AITC has been proven to effectively inhibit various pathogenic bacteria, including Rhizoctonia solani, Fusarium graminearum, Ralstonia solanacearum, P. aphanidermatum, Fusarium spp., and Phytophthora spp (Ashiq et al., 2022; Handiseni et al., 2017; Ren et al., 2018).
Soil microorganisms play a crucial role in environmental processes and are pivotal in maintaining the stability of soil ecosystems (Wu et al., 2024). The non-targeted nature of the fumigant led to a notable reduction in the population of detrimental soil microorganisms, such as Fusarium oxysporum and Mycobacterium avium. The application of AITC fumigation resulted in a transient decline in the overall microbial abundance in the soil, which gradually recovered over a one-month period (Castellano-Hinojosa et al., 2022). Furthermore, a significant increase in beneficial soil bacteria was observed following AITC fumigation (Tagele et al., 2021). AITC soil fumigation was also found to enhance the population of aerobic denitrifying bacteria (Li et al., 2023). Moreover, AITC fumigation and its degradation products demonstrated effective inhibition of soil manganese oxidation, leading to an increase in the bioavailable manganese content in the soil (Wang et al., 2024).
The diversity of inter-root microorganisms is intricately modulated by factors such as pH, effective phosphorus levels, and organic matter content (Zhou et al., 2021). Additionally, the species composition and abundance of the rhizosphere microbiome are influenced by the developmental stages of plants and the specific plant species present (Chen et al., 2022). Disparities in the distribution and abundance of inter-root and endophytic microorganisms significantly impact various physiological processes in plants, including growth regulation, disease resistance, and hormonal signaling pathways (Hartmann and Six, 2023; Yang et al., 2023). Endophytic microorganisms represent a category of microbes that engage in mutually beneficial symbiotic relationships with their host plants. Due to their prolonged coexistence within the unique plant environment, these microorganisms are recognized as valuable microbial resources that significantly contribute to the growth, development, and stress resilience of crops (Compant et al., 2019). Endophytes facilitate plant growth by providing essential nutrients or conferring protection against pathogenic challenges. The potential of plant endophytic microorganisms to enhance crop resistance and stimulate yield gains has attracted considerable research attention (Afzal et al., 2019; Sessitsch et al., 2012). Among these microorganisms, endophytic fungi have emerged as a focal point of investigation. Several studies have demonstrated that endophytic fungi play a significant role in enhancing crop nitrogen utilization (Yang et al., 2014). Notably, the presence of the endophytic bacterium Paenibacillus exerts a profound influence on the metabolic profile of isolated cultivated poplar plants (Scherling et al., 2009). Several bacterial and fungal genomes, such as those of Penicillium, Fusarium, Phytophthora, Dendrobacter, and Streptomyces, contain genes associated with nitrogen metabolism, hormone biosynthesis, phosphate utilization, and root colonization mechanisms (Huang et al., 2022; Shah et al., 2022). These Microorganisms play a crucial role in providing essential nutrients to plants, thereby facilitating growth promotion through mechanisms such as nitrogen fixation and enhanced access to phosphorus and iron (Liu et al., 2022; Phurailatpam et al., 2022).
Although the interactions between soil microbiota and crop endophytes are acknowledged to be crucial for plant health, the specific mechanisms underlying these interactions remain unclear. It has been demonstrated that soil microbial diversity and composition significantly impact plant growth and health, thereby influencing the dynamics of endophytic flora. However, changes in soil microbial communities do not invariably lead to shifts in crop endophytes, as the distinct habitats of endophytes are primarily shaped by the plant’s internal environment. Soil management practices, such as fertilization, microbial inoculation, and fumigation treatments, can modulate microbial diversity and abundance in the soil, subsequently influencing the microbial communities within the plant’s root system and above-ground structures, including endophyte populations. In this investigation, we employed HiSeq2500 high-throughput sequencing technology to explore variations in microbial community diversity and the structural composition of endophytes in different pepper varieties following AITC fumigation. Our study aims to elucidate the potential impact of changes in soil microbial communities on crop endophyte communities.
2 Materials and methods
2.1 Indoor soil fumigation experiment
The experimental soil used in this study was sourced from Yanqing, Beijing (115°44’–116°34’E, 40°16’–40°47’N) and is classified as alkaline tidal soil. Prior to use, the soil was sieved through a 2 mm mesh. A total of 12 kg of the test soil was weighed and placed into a 20 L plastic bucket. A 20% water emulsion of propylene isothiocyanate (AITC), obtained from Beijing Agronon Bio-Pharmaceutical Co., Ltd., was thoroughly mixed with the soil. Immediately after mixing, the bucket was sealed tightly with a transparent plastic cover and securely wrapped with transparent tape. The mixture was then incubated at 28°C in a light-free environment for a duration of 10 days. A control group without the addition of AITC was also included. Following the fumigation period, the soil was left uncovered in a well-ventilated area for one week to ensure the complete dispersion of any remaining fumigant. Subsequently, 30 g of the soil was collected and stored at −80°C for macrogenomic sequencing.
2.2 Pepper planting
The pepper seeds were pre-soaked and allowed to germinate for 3 days prior to sowing. Subsequently, the seeds were evenly distributed in the soil, covered with approximately 1 cm of loose soil, watered once, and maintained at a constant temperature of around 28°C. Regular watering was carried out to ensure soil moisture levels were optimal. Each experimental group consisted of 42 plants. The incubation room was set to a temperature of 28°C, with a relative humidity of 60% and a photoperiod of L//D = 12H//12H.
2.3 Sample collection
Sampling was conducted on the 55th day after pepper transplantation to assess plant height, stem thickness, and chlorophyll content. Chlorophyll levels were measured using a handheld chlorophyll meter. On the same day, rhizosphere soil samples were collected. During the sampling process, the peppers were carefully uprooted, and any loose soil attached to the root system was gently shaken off. Tightly adhered soil was then collected using a brush. A portion of the soil was stored at 4°C for the evaluation of physicochemical indices, while another portion was stored at −80°C for high-throughput sequencing.
The preparation of pepper samples involved washing the roots, stems, and leaves with distilled water to remove impurities. After washing, the roots, stems, and leaves were cut and soaked in 75% ethanol for 2 min, followed by a 5-min wash with a 2% sodium hypochlorite solution. Subsequently, the samples were transferred to 75% ethanol for 1 min and rinsed three times with sterile water, repeating this process three times. The samples were then dried, placed in self-sealing bags, and stored at −80°C for high-throughput sequencing (Ren et al., 2019). Each set of trials was conducted in triplicate.
2.4 Physicochemical properties of soil
Soil physicochemical parameters were analyzed following the method established by Li Sheik Kai. Nitrate nitrogen and ammonium nitrogen were extracted using KCl and quantified using a flow analyzer. Effective phosphorus was extracted with NaHCO3 and measured using a flow analyzer, while fast-acting potassium was extracted with CH3COONH4 and quantified using a flame spectrophotometer. pH was determined using a 2.5:1 water-to-soil ratio and potentiometric method, and electrical conductivity was measured using a 2.5:1 water-to-soil ratio and conductivity probe. Organic matter content was assessed through potassium dichromate titration.
2.5 Metagenomic sequencing
0.2 g of soil material was used to extract total genomic DNA with the E.Z.N.A.® soil DNA Kit (Omega Bio-tek, Norcross, GA, USA) according to manufacturer’s instructions. Concentration and purity of extracted DNA was determined with SynergyHTX and NanoDrop2000, respectively. DNA quality was checked on 1% agarose gel.
DNA extract was fragmented to an average size of about 350 bp using Covaris M220 (Gene Company Limited, China) for paired-end library construction. Paired-end library was constructed using NEXTFLEX Rapid DNA-Seq (Bioo Scientific, Austin, TX, USA). Paired-end sequencing was performed on Illumina NovaSeq™ X Plus (Illumina Inc., San Diego, CA, USA) at Majorbio Bio-Pharm Technology Co., Ltd (Shanghai, China) using NovaSeq X Series 25B Reagent Kit according to the manufacturer’s instructions.1
2.6 DNA extraction, PCR amplification, and high-throughput sequencing
DNA extraction from treated plant samples was performed utilizing the Qiagen magnetic bead method, while total DNA from soil samples was isolated using the E.Z.N.A.® Soil DNA Kit. Following extraction, the purity and concentration of DNA were evaluated using the Scandrop200 and confirmed by 1% agarose gel electrophoresis, respectively. Primers were tailed with PacBio barcode sequences to distinguish each sample. Amplification reactions (20-μL volume) consisted of 5 × FastPfu buffer 4 μL, 2.5 mM dNTPs 2 μL, forward primer (5 μM) 0.8 μL, reverse primer (5 μM) 0.8 μL, FastPfu DNA Polymerase 0.4 μL, template DNA 10 ng and DNase-free water. The PCR amplification was performed as follows: initial denaturation at 95°C for 3 min, followed by 27 cycles of denaturing at 95°C for 30 s, annealing at 60°C for 30 s and extension at 72°C for 45 s, and single extension at 72°C for 10 min, and end at 4°C (T100 Thermal Cycler PCR thermocycler, BIO-RAD, USA). After electrophoresis, The PCR products were purified using the AMPure® PB beads (Pacifc Biosciences, CA, USA) and quantified with Qubit 4.0 (Thermo Fisher Scientific, USA). For amplification of the 16S rRNA gene in pepper root, stem, and leaf samples, primers 779F (AACMGGATTAGATACCCKG) and 1193R (ACGTCATCCCCACCTTCC) were employed, whereas primers 338F (ACTCCTACGGGAGGCAGCA) and 806R (GGACTACHVGGGTWTCTAAT) were utilized for soil samples. Subsequent high-throughput and macro-genome sequencing were conducted by Shanghai Meiji Biotechnology Co., Ltd.
2.7 Statistical analyses
The purified amplicons were pooled in equimolar and paired-end sequenced (2 × 300) on an Illumina MiSeq platform (Illumina, San Diego, USA). The original sequences were sequenced, spliced, quality controlled, and filtered to obtain optimized sequences (Magoc and Salzberg, 2011), clustered into operable taxonomic units (OTUs) according to similarity levels. The optimized sequences were compared with the Silva (SSU123)16S rRNA Database. A confidence threshold of 0.7 was chosen to combine with the RDP classifier Bayesian algorithm v2.21 to taxonomically analyze (Magoc and Salzberg, 2011), OTU sequences at 97% similarity level to obtain species annotation information for OTUs. The best-hit taxonomy of non-redundant genes was obtained by aligning them against the NCBI NR database by DIAMOND[6] (version 2.0.13)2 with an e-value cutoff of 1e-5. Similarly, the functional annotation (GO, KEGG, eggNOG, CAZy, CARD, PHI) of non-redundant genes was obtained. Based on the taxonomic and functional annotation and the abundance profile of non-redundant genes, the differential analysis was carried out at each taxonomic, functional, or gene-wise level by Kruskal–Wallis test. The experimental data was organized using Microsoft Excel 2010 software. The comparison of means between groups was conducted using SPSS 26 for independent samples t-test and one-way ANOVA. The significance level was set at α = 0.05. PcoA was generated using the Bray Curtis algorithm, and community composition and variability analyses were performed using the Meguiar’s Cloud platform. Graphing was carried out using Origin 2017. The Welch t-test method was used to calculate the confidence interval. The stats package (version 3.3.1) of R and the scipy package (version 1.0.0) of Python were used. The linear discriminant analysis (LDA) effect size (LEfSe)3 was performed to identify the significantly abundant taxa (phylum to genera) of bacteria among the different groups (LDA score > 3.8, P < 0.05).
3 Results
3.1 Effects of allyl isothiocyanate on soil microorganisms and functions
Gene-based species taxonomic annotations were compared with the NR database to extract species and abundance information across various taxonomic levels, including domain, kingdom, phylum, order, family, genus, and species for each sample. A total of 244 phyla, 501 orders, 1,003 families, 1,966 genera, and 30,841 species were identified through NR species annotation. The results of the bacterial community alpha diversity index (Table 1) demonstrate that AITC fumigation significantly enhanced the richness of the soil microbial community.
A comparative analysis of soil microorganisms before and after allyl isothiocyanate (AITC) fumigation revealed shifts in the top ten microorganisms at the phylum level within the soil microbial community (Figure 1A). The predominant phyla identified were Actinomycetota, Pseudomonadota, Acidobacteriota, Chloroflexota, Gemmatimonadota, Nitrososphaerota, Verrucomicrobiota, Planctomycetota, Candidatus Dormibacteraeota, and unclassified d_Bacteria. Notably, the relative abundance of Actinomycetota showed a significant increase (P < 0.01) post-fumigation. Conversely, there was a marked decrease in the relative abundance of Pseudomonadota and Bacteroidota (P < 0.01), indicating that Actinomycetota exhibited a significant increase (P < 0.01) (Figures 2A–C).
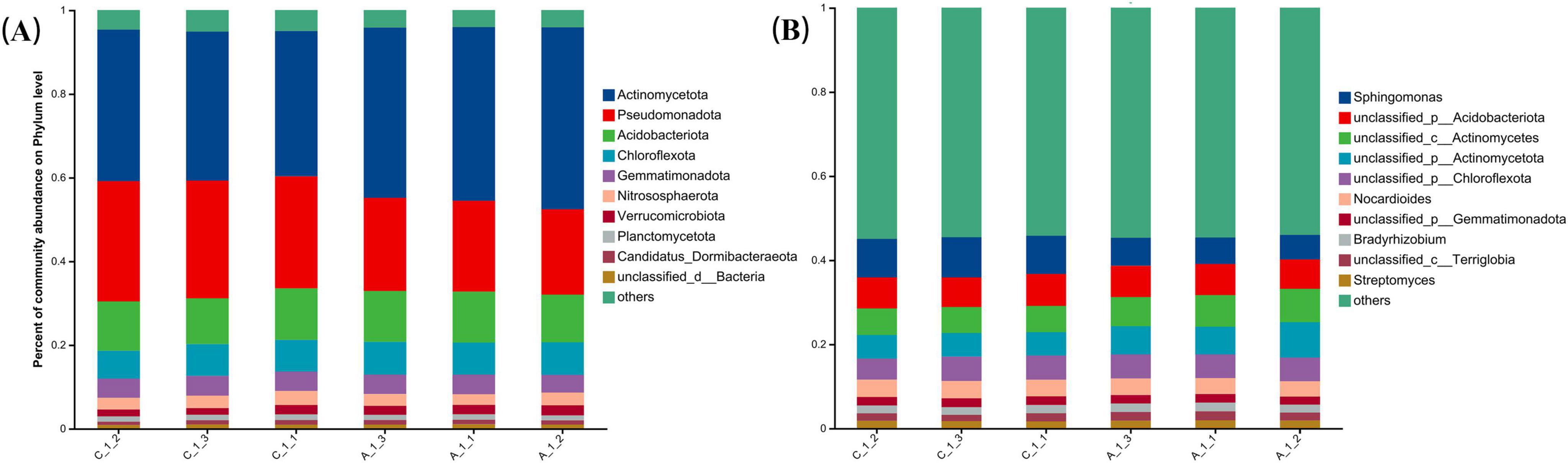
Figure 1. Bar chart showing changes in the relative abundance of the top ten bacterial communities at the phylum level (A) and genus level (B) in soil samples.
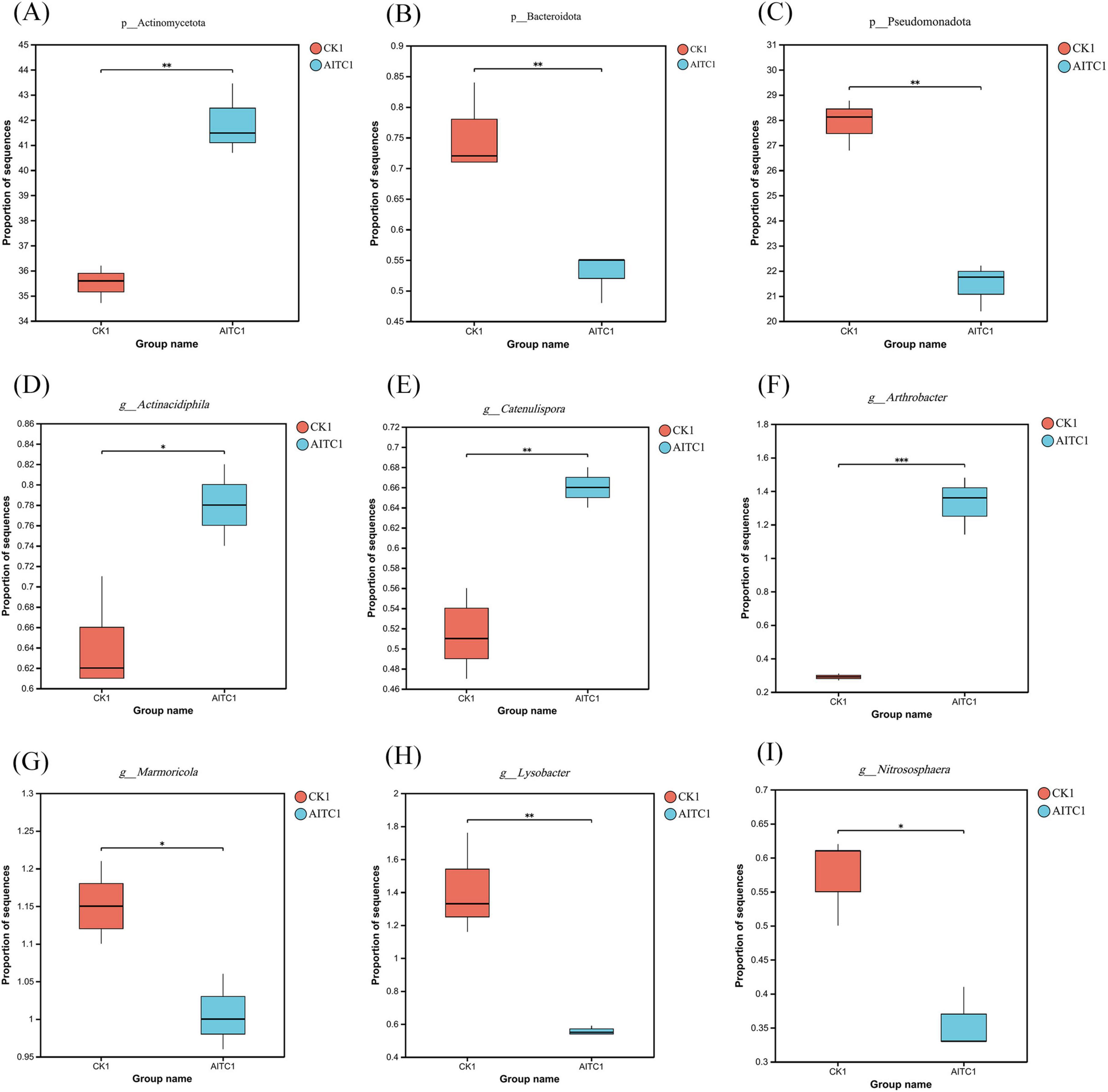
Figure 2. Microorganisms with significant differences in soil microorganisms at the phylum level (A–C) and genus level (D–I) after AITC fumigation. *0.01 < p ≤ 0.05, **0.001 < p ≤ 0.01.
At the genus level (Figure 1B), the top ten microorganisms in relative abundance in the soil microbial community were Sphingomonas, unclassified_ p_Acidobacteriota, unclassified_c_Actinomycetes, unclassified_p_ Actinomycetota, unclassified_p_Chloroflexota, Nocardioides, unclassified_p_Gemmatimonadota, Bradyrhizobium, unclassified_ c_Terriglobia, Streptomyces. Of which, Sphingomonas, Lysobacter, Nitrososphaera, Marmoricola, Candidatus_Nitrosocosmicus, Phycicoccus, Nitrosopumilus showed significant reduction in relative abundance after fumigation, Arthrobacter, Blastococcus, Pseudarthrobacter, Actinacidiphila, Catenulispora, Mycobacterium, Pseudonocardia, and Trebonia increased significantly in relative abundance (Figures 2D–I).
By comparing the results with the KEGG database, the top three annotations at Level 1 (Figure 3A) in soil samples were identified as Metabolism, Environmental Information Processing, and Genetic Information Processing. The known gene functions with the top 10 abundances were analyzed using ANOVA. The analysis of variance revealed that the Metabolism function was significantly higher in fumigated soil compared to unfumigated soil (P < 0.05), while Genetic Information Processing, Cellular Processes, and Human Diseases were significantly lower in the fumigated soil (P < 0.05). At Level 2 (Figure 3B), metabolic pathways were also significantly higher in the soil samples than in the unfumigated soil, with significant increases observed in the following pathways: Biosynthesis of Secondary Metabolites, Microbial Metabolism in Diverse Environments, Carbon Metabolism, Quorum Sensing, ABC Transporters, and Pyruvate Metabolism (P < 0.05). The top 10 pathways annotated at the Pathway classification level that exhibited significant differences (Figure 3C) included Biosynthesis of Secondary Metabolites, Microbial Metabolism in Diverse Environments, Carbon Metabolism, Biosynthesis of Cofactors, Two-Component System, Quorum Sensing, ABC Transporters, Pyruvate Metabolism, and Purine Metabolism.
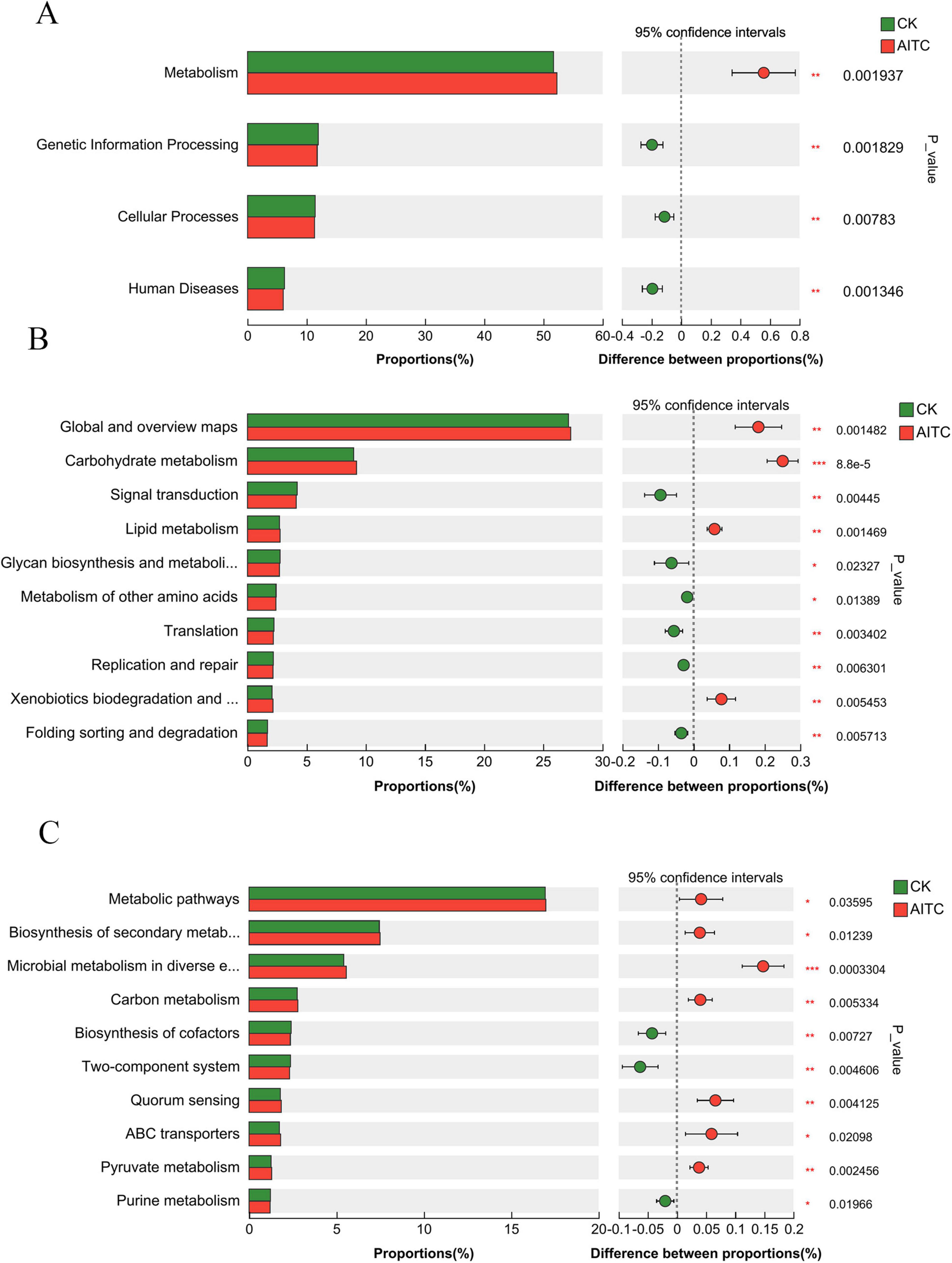
Figure 3. The KEGG functional levels of soil microorganisms after AITC fumigation were significantly different. (A) Pathway level 1. (B) Pathway level 2. (C) Pathway level 3.
3.2 Effects of allyl isothiocyanate on the growth of peppers and soil physicochemical indexes
Allyl isothiocyanate (AITC) fumigation exerts a positive effect on the growth and development of peppers, although varietal discrepancies are evident (Figure 4). By the 55th day post-planting, fumigation significantly enhanced the plant height of Xiangla359 and Shuang Jiao, as well as the stem thickness of Shuang Jiao. Specifically, in the treatment group, the plant height of Xiangla359 exhibited a significant increase of 28.2%, accompanied by a notable 10.4% increase in stem thickness. Moreover, La Xuan demonstrated a growth of 12.5% in plant height, while Shuang Jiao exhibited a substantial rise of 25.9%. As depicted in Table 2, the impacts of soil fumigation with AITC on the physicochemical indicators of the rhizosphere soil for various chili pepper cultivars varied; however, these variations were not deemed statistically significant.
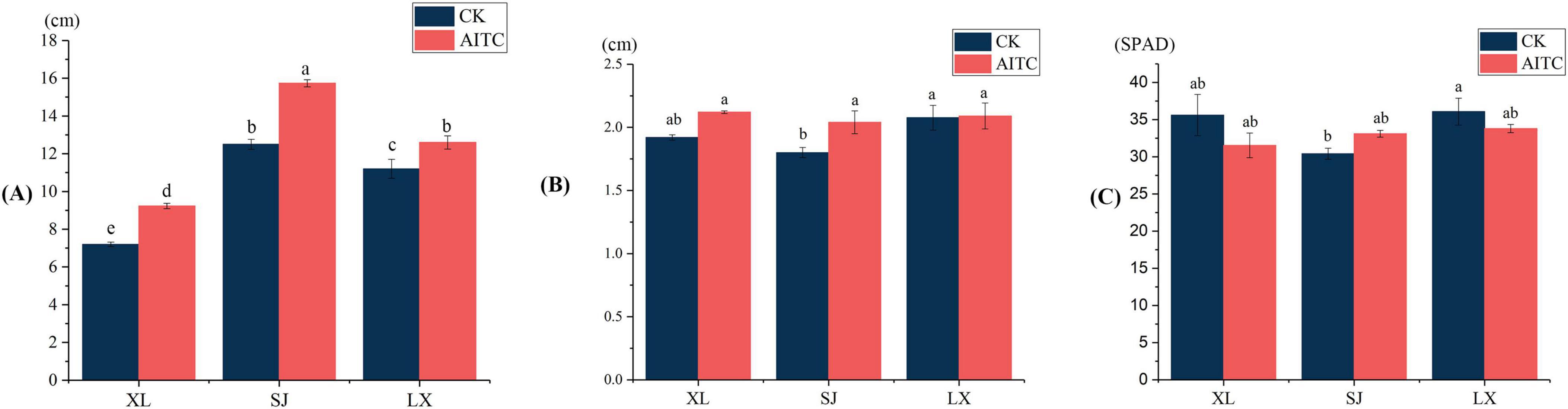
Figure 4. The impact of AITC fumigation on the growth of pepper plants. (A) Height of pepper plants under different treatments. (B) Stem diameter of chili pepper plants under different treatments. (C) Chlorophyll content of chili pepper leaves under different treatments. All results are expressed as mean ± standard deviation (SD), and the same letters indicate no statistical difference (P = 0.05) according to Duncan’s new multiple range test.
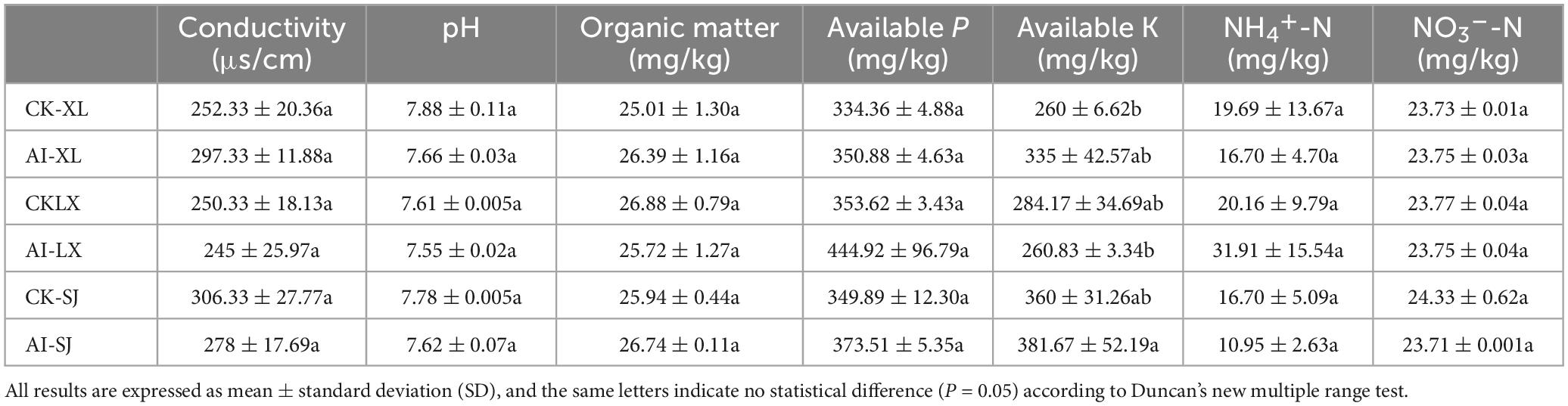
Table 2. Effect of soil fumigation with allyl isothiocyanate on physicochemical indexes of rhizosphere soil of different varieties of capsicum.
3.3 Effect of soil fumigation with allyl isothiocyanate on the diversity index of the bacterial community of pepper
Significant variations in the bacterial community diversity index (ACE) were observed among different parts of the pepper plant, including inter-root, intra-root, intra-stem, and intra-leaf regions, regardless of the fumigation treatment (Figure 5A). These findings suggest that tissue variability remained consistent even after fumigation. However, 80 days post-fumigation, the ACE values for intra-root and intra-leaf bacterial diversity were significantly reduced by allyl isothiocyanate (AITC), while no significant impact was observed on inter-root and intra-stem bacterial diversity. This indicates that the stimulating effect of AITC on soil microbial community diversity diminished over time but continued to significantly influence microbial diversity within the roots and leaves of the crop.
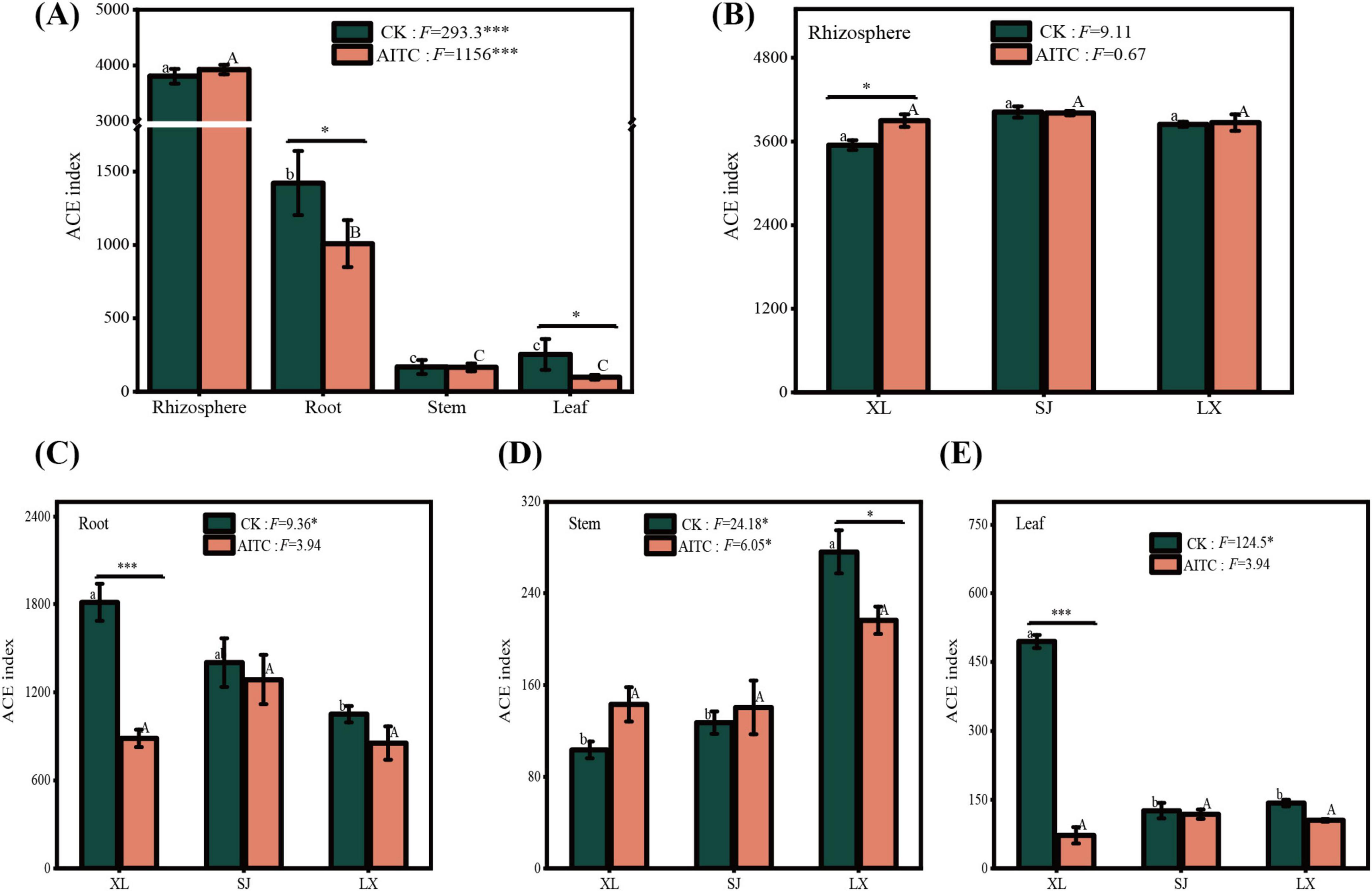
Figure 5. The impact of AITC fumigation on the microbial diversity index of chili plants: (A) overall effect on different tissues; (B) response of rhizosphere bacteria; (C) response of endophytic fungi in roots; (D) response of endophytic bacteria in stems; (E) response of endophytic bacteria in leaves. *0.01 < p ≤ 0.05, ***p ≤ 0.001.
Further analysis of the responses of bacterial communities in the root, stem, and leaf tissues of three pepper varieties revealed distinct spatial characteristics and significant differences in the effects of allyl isothiocyanate (AITC) fumigation on endophytic bacteria. Inter-root bacterial diversity showed no significant differences among Xiangla359 (XL), Shuang Jiao (SJ), and La Xuan (LX). However, AITC fumigation significantly increased the inter-root bacterial diversity index (ACE) in Xiangla359 (Figure 5B). Within the roots, both Xiangla359 and Shuang Jiao exhibited significantly higher bacterial diversity compared to La Xuan. Nevertheless, AITC fumigation resulted in a significant decrease in bacterial diversity within the roots of Xiangla359, with no significant differences observed among the three varieties after fumigation (Figure 5C). Conversely, La Xuan displayed significantly higher bacterial diversity in the stems compared to Xiangla359 and Shuang Jiao. However, AITC fumigation led to a significant reduction in bacterial diversity in the stems of La Xuan, with no significant differences among the three varieties after fumigation (Figure 5D). In terms of leaf tissues, Xiangla359 exhibited significantly higher bacterial diversity compared to Shuang Jiao and La Xuan. AITC fumigation significantly reduced bacterial diversity in the leaves of Xiangla359 but had no significant effect on Shuang Jiao and La Xuan (Figure 5E). Overall, the impact of AITC fumigation on endophytes in pepper roots and leaves was more pronounced than in stems, resulting in a significant reduction in microbial diversity in the roots and leaves of Xiangla359, while showing no significant effects on Shuang Jiao and La Xuan.
3.4 Effect of allyl isothiocyanate on the structure of the bacterial community of chili peppers
In the unfumigated treatment, both tissue site and genotype significantly influenced the structure of the endophytic bacterial community in pepper, accounting for 22% (p < 0.01) and 19% (p < 0.01) of the variation, respectively (Figure 6A). Conversely, allyl isothiocyanate (AITC) fumigation markedly enhanced the contribution of genotype to the endophytic bacterial community structure in pepper, increasing it to 58% (p < 0.001) while concurrently reducing the contribution of tissue site (Figure 6B).
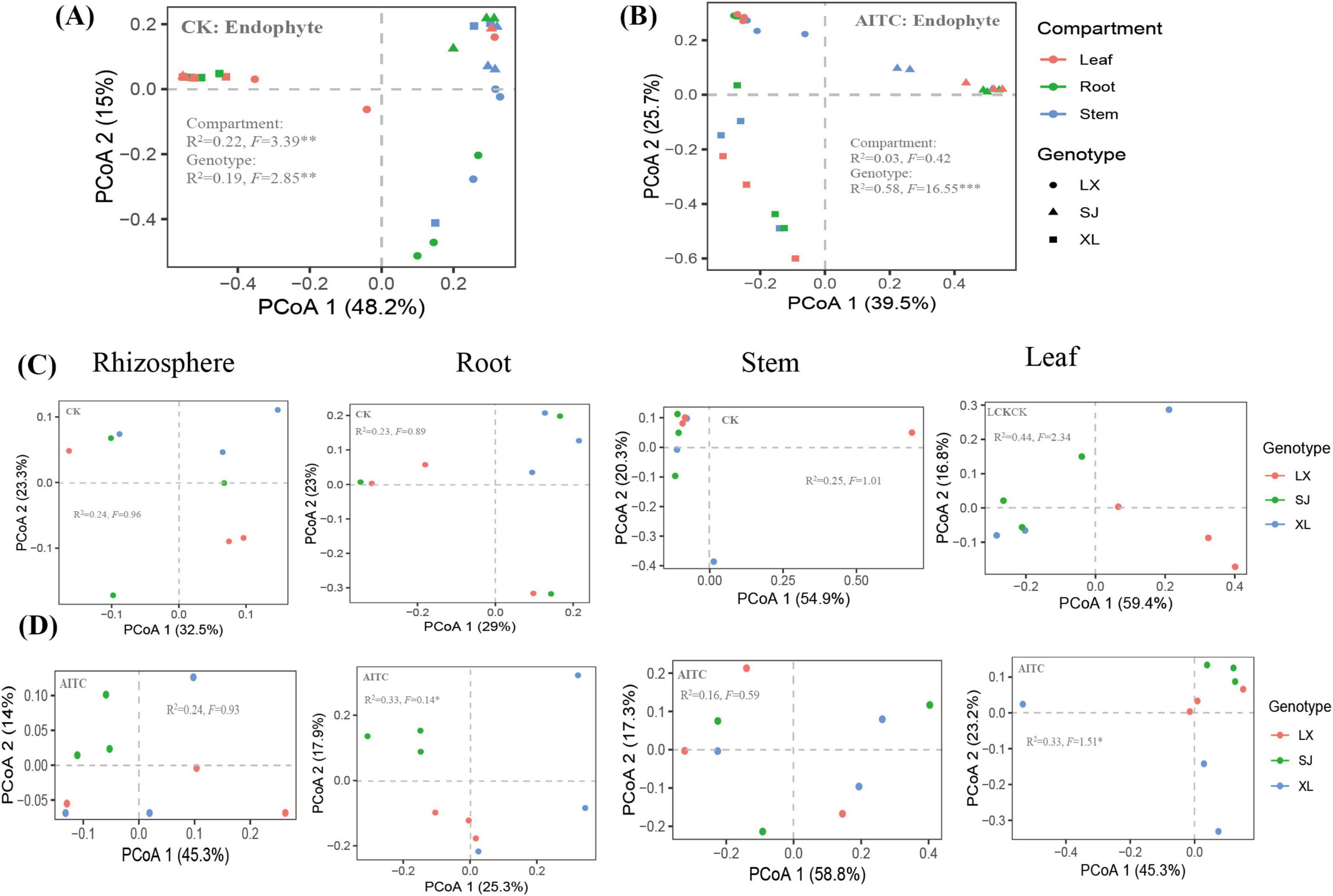
Figure 6. Based on the Bray Curtis algorithm PCoA (Principal Co-ordinates Analysis), (A) PCoA of endophytic bacteria in untreated samples; (B) PCoA of endophytic bacteria treated with AITC (Allyl isothiocyanate); (C) PCoA of rhizosphere, endophytic, stem, and leaf bacteria in untreated samples; (D) PCoA of rhizosphere, endophytic, stem, and leaf bacteria in treated samples. PERMANOVA method was used to calculate statistical differences.
Consequently, we conducted a detailed analysis of the bacterial community structure across various tissues (roots, stems, and leaves) of different pepper cultivars. Following to AITC fumigation, the impact of genotypes on inter-root and intra-stem bacterial community structures was found to be statistically insignificant. However, there was a notable increase in the explanatory power of the bacterial community structure in roots (23 vs. 33%, p < 0.05), accompanied by a corresponding decrease in leaves (44 vs. 33%, p < 0.05) (Figures 6C, D). It is evident that allyl isothiocyanate (AITC) fumigation primarily influences the bacterial community structure in roots and leaves of pepper and is closely associated with the genotype of the plant.
Linear discriminant analysis effect size (LEfSe) was employed to identify species with significant differences across various parts of pepper plants. The LEfSe species hierarchy chart (LDA > 3.8) was used to identify groups exhibiting significant variations. As shown in the distribution plots (Figures 7A–C) with LDA scores exceeding 3.8, significant differences were observed in the microbial communities enriched in different parts of the pepper plant under various treatments.
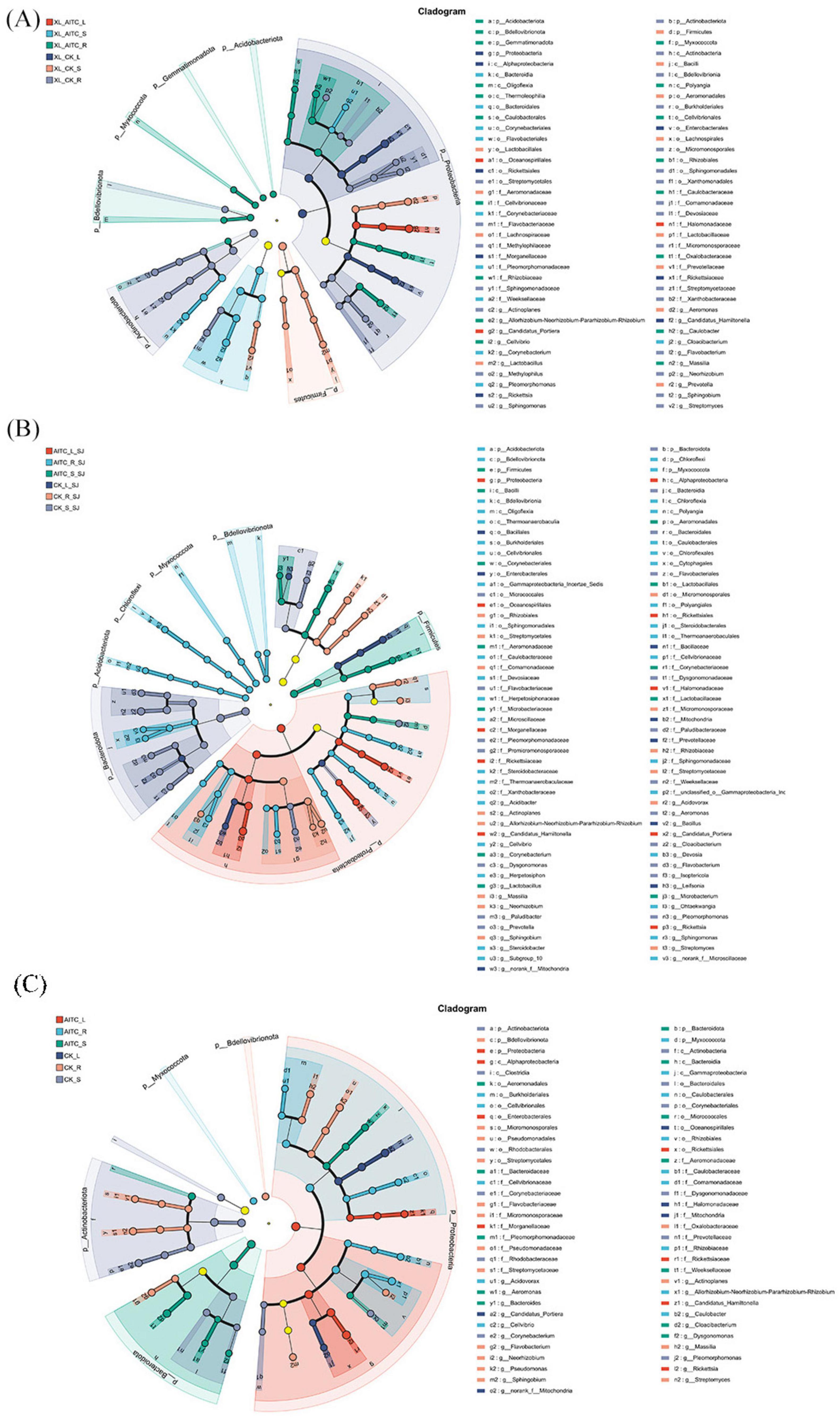
Figure 7. LEfSe cladogram analysis of endophytic microbial community (LDA = 3.8) in pepper after soil fumigation (P = 0.05). The five rings in the cladogram represent the phylum, class, order, family and genus, respectively, when read from the inside. The different color nodes (except yellow) on the ring represent significant changes in taxonomic composition due to the treatments. (A) Xiangla359; (B) Shuangjiao; (C) Laxuan.
At the genus level, untreated Xiangla359 roots exhibited a marked predominance of Streptomyces, Sphingomonas, Sphingobium, Methylophilus, Neorhizobium, and Flavobacterium. In contrast, the stems showed a significant increase in Prevotella, Lactobacillus, and Aeromonas, while the leaves were predominantly dominated by Rickettsia. Following AITC treatment, the roots displayed a significant abundance of Cellvibrio, Massilia, Caulobacter, and Allorhizobium-Neorhizobium-Pararhizobium-Rhizobium. In the stems, Pleomorphomonas, Corynebacterium, and Cloacibacterium were notably abundant.
Untreated Shuang Jiao roots demonstrated substantial concentrations of Streptomyces, Sphingobium, Neorhizobium, Massilia, Acidovorax, and Allorhizobium-Neorhizobium-Pararhizobium-Rhizobium. The stems were notably enriched with Actinoplanes, Prevotella, Paludibacter, Pleomorphomonas, Aeromonas, Cloacibacterium, Dysgonomonas, and Flavobacterium, while Bacillus and Leifsonia were primarily concentrated in the leaves. After AITC treatment, the roots of Shuang Jiao displayed a notable rise in Subgroup_10, Steroidobacter, Sphingomonas, Ohtaekwangia, Herpetosiphon, Cellvibrio, Acetobacter, and Devosia. In the stems, Corynebacterium, Lactobacillus, and Microbacterium were significantly more abundant.
In untreated La Xuan roots, Streptomyces, Sphingomonas, Sphingobium, Pseudomonas, Neorhizobium, Flavobacterium, Massilia, and Actinoplanes were notably abundant. The stems exhibited significant accumulation of Corynebacterium and Pleomorphomonas. Following AITC treatment, the roots displayed a marked increase in Cellvibrio, Acidovorax, Caulobacter, and Allorhizobium-Neorhizobium-Pararhizobium-Rhizobium. In the stems, Dysgonomonas, Aeromonas, Cloacibacterium, and Bacteroidota were significantly more prevalent, while Rickettsia was predominantly concentrated in the leaves.
In the rhizosphere of pepper plants, the predominant bacterial phyla included Actinobacteriota, Proteobacteria, Acidobacteriota, Chloroflexi, Firmicutes, Gemmatimonadota, Bacteroidota, and Myxococcota (Supplementary Figure 1A). Fumigation with allyl isothiocyanate (AITC) significantly increased the relative abundance of Armatimonadota and enhanced the presence of Acidobacteriota in the inter-root region of Shuang Jiao. At the genus level, the primary taxa identified included Streptomyces, Allorhizobium-Neorhizobium-Pararhizobium-Rhizobium, Pseudomonas, Massilia, Flavobacterium, Actinoplanes, Methylophilus, and Sphingobium (Supplementary Figure 1B). Moreover, fumigation led to a substantial rise in the relative abundance of Pseudarthrobacter (34.21%) and RB41 (62.21%), while reducing the abundance of Gaiella (41.09%) in the inter-root soil of Shuang Jiao.
In the root systems of pepper plants, the predominant bacterial phyla identified were Proteobacteria, Actinobacteriota, Firmicutes, Bacteroidota, Myxococcota, Bdellovibrionota, Chloroflexi, Acidobacteriota, Gemmatimonadota, and Verrucomicrobiota (Supplementary Figure 2A). Fumigation with AITC significantly increased the relative abundance of Myxococcota, while simultaneously decreasing Actinobacteriota in the roots of Shuang Jiao. At the genus level, the major taxa present in pepper roots included Streptomyces, Allorhizobium-Neorhizobium-Pararhizobium-Rhizobium, Pseudomonas, unclassified_f_Alcaligenaceae, Massilia, Flavobacterium, Actinoplanes, Methylophilus, Sphingobium, and unclassified_f_Oxalobacteraceae (Supplementary Figure 2B). AITC fumigation notably enhanced the relative abundance of Cloacibacterium (356.38%) and led to significant increases in Allorhizobium-Neorhizobium-Pararhizobium-Rhizobium (221.94%), Bacillus (141.51%), Pseudomonas (191.47%), and Corynebacterium (209.91%) within the roots of Xiangla359. In contrast, fumigation reduced the abundance of Cloacibacterium (61.78%), Streptomyces (63.76%), Pseudomonas (88.80%), and Massilia (70.26%) in Shuang Jiao roots, while increasing that of Corynebacterium (148.74%) and Bacillus (13.88%). Similarly, in La Xuan roots, fumigation led to an increase in the relative abundance of Cloacibacterium (75.37%), Allorhizobium-Neorhizobium-Pararhizobium-Rhizobium (80.11%), and Corynebacterium (23.56%), while reducing Streptomyces (59.55%) and Massilia (40.36%) (Figure 8).
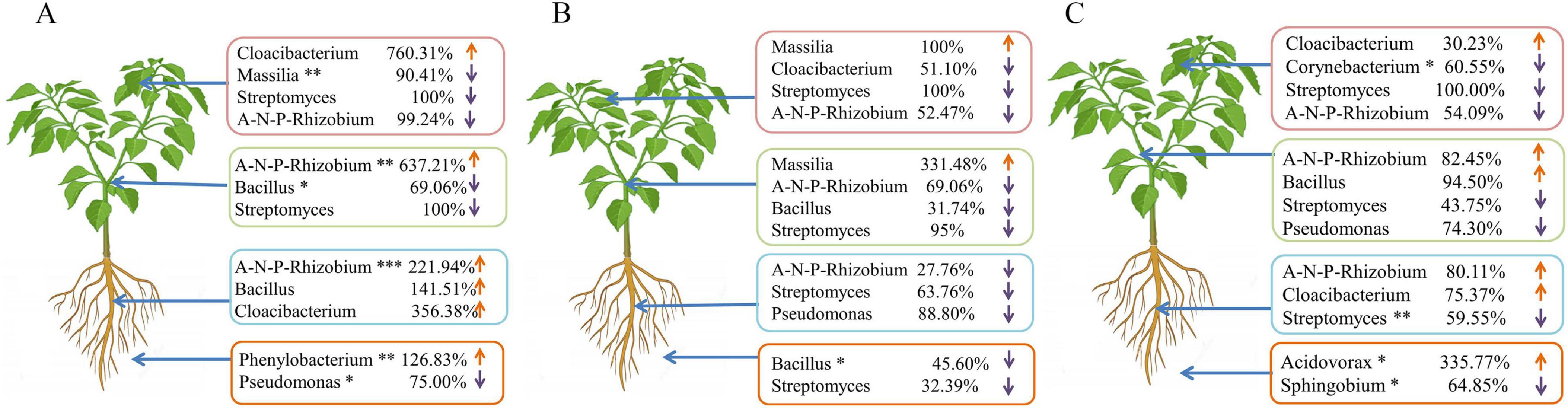
Figure 8. Changes in microorganisms in the roots, stems, leaves, and rhizosphere soil of different varieties of pepper plants. (A) Xiangla359; (B) Shuang Jiao; (C) La Xuan; A-N-P-Rhizobium: Allorhizobium-Neorhizobium-Pararhizobium-Rhizobium (*p < 0.05, ** < 0.01, *** < 0.001).
In pepper stems, the predominant bacterial phyla identified included Proteobacteria, Firmicutes, Bacteroidota, Actinobacteriota, Deinococcota, Cyanobacteria, Gemmatimonadota, Myxococcota, Bdellovibrionota, and Verrucomicrobiota (Supplementary Figure 3A). At the genus level, the dominant taxa were Cloacibacterium, Corynebacterium, Bacillus, Aeromonas, Lactobacillus, Flavobacterium, Paludibacter, Prevotella, and the Allorhizobium-Neorhizobium-Pararhizobium-Rhizobium group (Supplementary Figure 3B). Following fumigation, the Allorhizobium-Neorhizobium-Pararhizobium-Rhizobium group in Xiangla359 stems showed a significant increase in relative abundance (637.21%), accompanied by a 50.10% increase in Corynebacterium. In contrast, Massilia and Pseudomonas saw a decline in relative abundance by 63.85% and 24.61%, respectively. In the La Xuan stem microbiome, fumigation resulted in increased abundances of Cloacibacterium (153.46%), Corynebacterium (60.77%), Bacillus (94.50%), and Allorhizobium-Neorhizobium-Pararhizobium-Rhizobium (82.45%). Meanwhile, Massilia, Pseudomonas, and Streptomyces decreased by 94.50, 74.30, and 43.75%, respectively. In another cultivar, fumigation led to a 331.48% increase in Massilia, while relative abundances of Cloacibacterium, Allorhizobium-Neorhizobium-Pararhizobium-Rhizobium, and Streptomyces decreased by 38.02, 69.06, and 95%, respectively, within the stem microbiome (Figure 8).
In pepper leaves, the predominant bacterial phyla identified included Proteobacteria, Firmicutes, Bacteroidota, Actinobacteriota, Deinococcota, Cyanobacteria, Gemmatimonadota, Myxococcota, Bdellovibrionota, and Verrucomicrobiota (Supplementary Figure 4A). At the genus level, Bacillus, Cloacibacterium, Corynebacterium, Prevotella, and Lactobacillus were the most abundant genera (Supplementary Figure 4B). Fumigation with allyl isothiocyanate (AITC) induced notable shifts in these microbial communities, particularly increasing the relative abundance of Cloacibacterium (760.31%) in Xiangla359 leaves, while significantly decreasing Massilia (90.41%), Pseudomonas (48.43%), Streptomyces (100%), and Allorhizobium-Neorhizobium-Pararhizobium-Rhizobium (99.24%). In Shuang Jiao leaves, AITC treatment led to a 100% increase in Massilia, while Corynebacterium (52.47%), Streptomyces (100%), Bacillus (73.76%), and the Allorhizobium-Neorhizobium-Pararhizobium-Rhizobium group (52.47%) were reduced. Similarly, in La Xuan leaves, AITC exposure resulted in substantial increases in Massilia (1,209.09%) and Pseudomonas (144.73%), alongside reductions in Corynebacterium (60.55%), Streptomyces (100%), Bacillus (55.90%), and the Allorhizobium-Neorhizobium-Pararhizobium-Rhizobium group (54.09%) (Figure 8).
The traceability analysis results revealed that AITC fumigation significantly altered the distribution of the source and sink of the pepper microbiome across sub-compartments (soil, roots, stems, and leaves), particularly in Xiangla359 varieties. Compared to the non-fumigated group, the fumigation treatment promoted the accumulation of microorganisms in the roots, stems, and leaves of Xiangla359 peppers, while enhancing the enrichment of endophytic bacteria in the stems and roots of Laxuan pepper and Shuangjiao pepper respectively. This shift indicated that fumigation not only influenced the overall structure of the microbial community but also regulated the dynamic balance between source and sink by altering the migration and distribution of microorganisms. However, the extent of this regulation varied across genotypes (Figure 9).
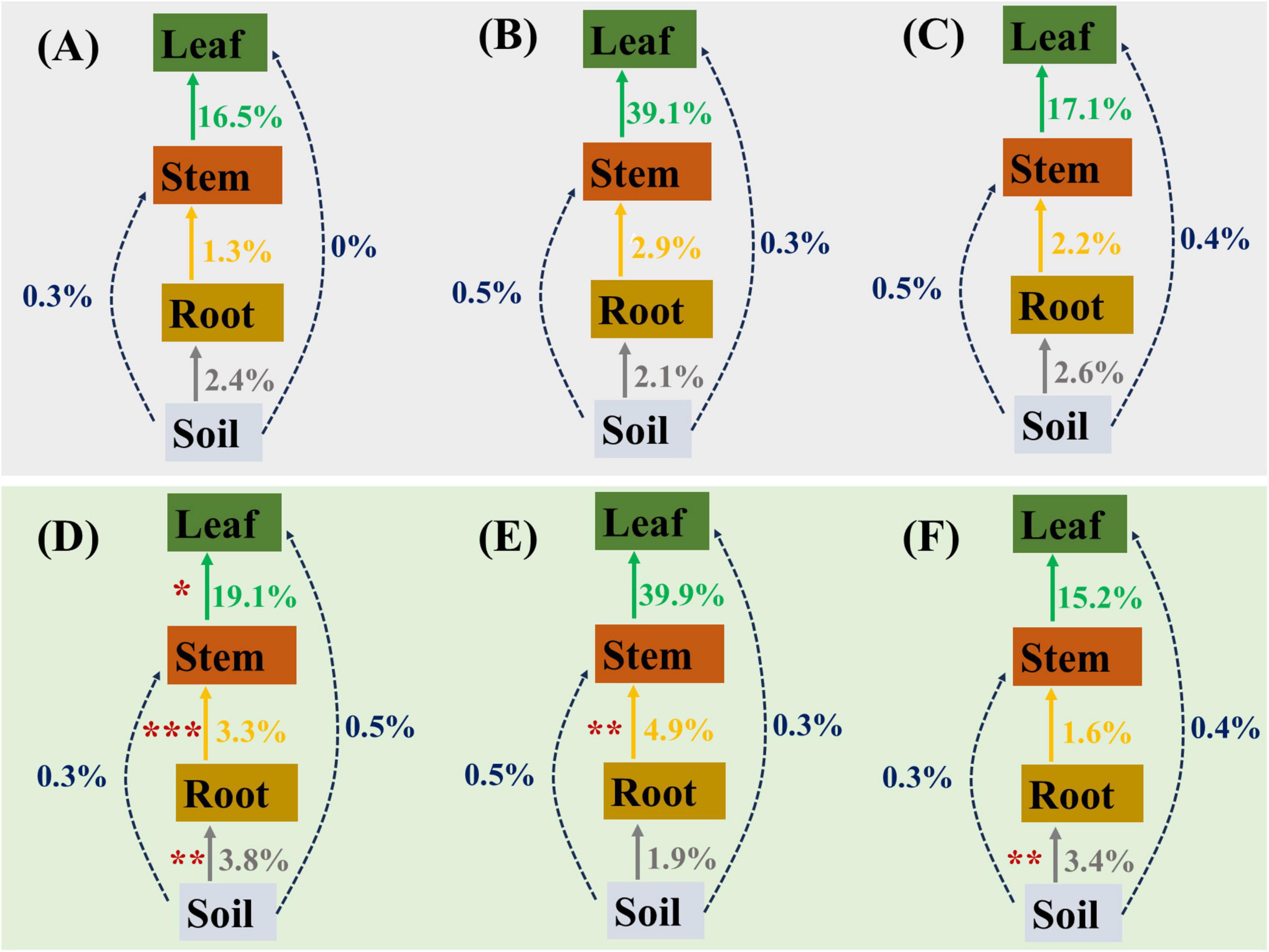
Figure 9. Traceability analysis of microorganisms in different genotypes of pepper across various sub-chambers (soil, root, stem, leaf) in the unfumigated (A,B) and AITC fumigated (D–F) groups. (A,D): Xiangla359; (B,E): Laxuan; (C,F): Shuang Jiao. The values to the right of the arrows represent the percentage from source to sink, and the asterisk to the left of the arrows indicates a significant difference between the fumigated and control groups.
4 Discussion
The microbial community structure in the rhizosphere of peppers shows considerable variability, with a notable degree of specificity in the interaction between plant genotypes and microbial composition (Weinert et al., 2011). Certain plant genotypes can selectively accumulate beneficial microorganisms, thereby enhancing plant defenses against pathogens (Berendsen et al., 2012). In this study, we observed significant differences in bacterial community diversity across different regions of the pepper plant, including inter-root, intra-root, intra-stem, and intra-leaf areas, independent of fumigation treatments. Furthermore, the variability in microbial composition within these tissues remained stable, irrespective of fumigation. Distinct plant genotypes appear to promote specific microbial enrichments, likely influenced by root-secreted compounds, inter-root environmental conditions, and additional biochemical factors (Cordovez et al., 2019). Moreover, AITC fumigation significantly altered soil metabolic processes, leading to changes in root distribution and inter-root exudation patterns.
The modification of soil microbial community structure by AITC fumigation influences microbial composition within the roots, stems, and leaves of peppers through various mechanisms: (1) Nutrient availability: AITC fumigation alters soil nutrient cycling, particularly by enhancing nitrogen and phosphorus mineralization, which significantly increases the availability of these nutrients for plant uptake (Fang et al., 2022; Huang et al., 2020; Li et al., 2023). Additionally, nutrient release from organic matter following fumigation—likely due to the suppression of specific microbial taxa—supports root nourishment. Compounds generated through these processes act as nutrient sources for soil microorganisms, selectively promoting the growth of particular microbial populations in the rhizosphere. This selective enrichment subsequently affects microbial community composition within the plant’s stems and leaves. (2) Microbial interaction: Interactions among soil microorganisms are intricate, involving both competitive and symbiotic relationships. Beneficial microorganisms are essential for enhancing plant resistance to pathogens, while harmful microorganisms can detrimentally impact plant health. The balance of these interactions strongly influences the microbial community composition within plant tissues. For example, allyl isothiocyanate (AITC) fumigation has been shown to increase soil microbial diversity and promote the growth of beneficial bacteria, such as Bacillus species. These beneficial microorganisms can colonize plant roots from the soil and subsequently move through the vascular system to the stems and leaves. This translocation process introduces novel microbial species and reshapes the existing microbial communities across different plant tissues. (3) Soil health: Prior to fumigation, soils generally exhibit low quality, often characterized by nutrient imbalances, degraded soil structure, and an unbalanced microbial community. Fumigation can significantly improve soil health by enhancing nutrient cycling and restructuring the microbial community (Cordovez et al., 2019). Healthy soils support high microbial diversity, which promotes vigorous plant growth. This robust plant growth stimulates the development of an extensive root system, thereby sustaining a diverse array of microorganisms within the roots and influencing microbial communities in the stems and leaves.
Fumigation with allyl isothiocyanate (AITC) induces significant shifts in soil microbial communities and functions, subsequently affecting the populations of beneficial bacteria in the roots, stems, and leaves of various pepper cultivars. AITC fumigation promotes the proliferation of diverse beneficial microorganisms, each with specialized biological functions. For example, Allorhizobium-Neorhizobium-Pararhizobium-Rhizobium species and nitrogen-fixing (Guo et al., 2024; Zhang W. et al., 2023), and Streptomyces strains play crucial roles in enhancing plant nutrient uptake (Olenska et al., 2020). Additionally, plant growth-promoting rhizobacteria (PGPR) such as Pseudomonas and the inter-root probiotic Massilia contribute to increased plant vigor and resilience (He et al., 2024). Cellulolytic activities are associated with Cellvibrio and Sphingomonas, while Devosia species are linked to inter-root exudates (Chen et al., 2019).
5 Conclusion
In this study, we investigated the effects of allyl isothiocyanate (AITC) soil fumigation on the endophytic bacterial communities associated with different pepper genotypes (Xiangla359, La Xuan, and Shuang Jiao). Our findings revealed that AITC fumigation predominantly influenced bacterial community structure in both roots and leaves, with a strong correlation to the genetic characteristics of the pepper cultivars. Notably, AITC fumigation led to a significant reduction in microbial diversity within the roots and leaves of Xiangla359, while having no substantial impact on La Xuan and Shuang Jiao.
Moreover, the growth-promoting effects of AITC fumigation varied among the three pepper varieties, with Xiangla359 exhibiting the most pronounced enhancement. This variation appears to be attributable to differential fumigation effects on beneficial bacterial populations across the roots, stems, and leaves of each cultivar. For instance, the Allorhizobium-Neorhizobium-Pararhizobium-Rhizobium increased in the roots and stems of Xiangla359 and La Xuan but decreased in Shuang Jiao. Similarly, Bacillus populations increased in the roots of Xiangla359 but declined in La Xuan and Shuang Jiao, while Streptomyces consistently decreased in the roots across all pepper varieties.
These results indicate that AITC fumigation significantly alters the diversity and structural composition of soil microbial communities; however, its effects on endophytic communities in pepper plants are highly dependent on the plant genotype.
Data availability statement
The raw sequence data reported in this paper have been deposited in the Genome Sequence Archive (Genomics, Proteomics & Bioinformatics 2021) in National Genomics Data Center (Nucleic Acids Res 2022), China National Center for Bioinformation / Beijing Institute of Genomics, Chinese Academy of Sciences (GSA: CRA025577) that are publicly accessible at https://ngdc.cncb.ac.cn/gsa.
Author contributions
WT: Writing – original draft, Writing – review and editing. QW: Writing – original draft. AC: Writing – original draft. DY: Writing – original draft. YL: Writing – original draft. ZL: Writing – original draft. BY: Writing – original draft. WF: Writing – original draft.
Funding
The authors declare that financial support was received for the research and/or publication of this article. This work was supported by the National Key Research and Development Program of China (2021YFD1600300-4, 2023YFD1701200), Special Project of Biological Seed Industry and Fine and Deep Processing of Agricultural Products (202202AE090031), and Innovation Program of Chinese Academy of Agricultural Sciences (CAAS-CSAL-202401).
Conflict of interest
The authors declare that the research was conducted in the absence of any commercial or financial relationships that could be construed as a potential conflict of interest.
Generative AI statement
The authors declare that no Generative AI was used in the creation of this manuscript.
Publisher’s note
All claims expressed in this article are solely those of the authors and do not necessarily represent those of their affiliated organizations, or those of the publisher, the editors and the reviewers. Any product that may be evaluated in this article, or claim that may be made by its manufacturer, is not guaranteed or endorsed by the publisher.
Supplementary material
The Supplementary Material for this article can be found online at: https://www.frontiersin.org/articles/10.3389/fmicb.2025.1520443/full#supplementary-material
Footnotes
- ^ www.illumina.com
- ^ http://ab.inf.uni-tuebingen.de/software/diamond/
- ^ http://huttenhower.sph.harvard.edu/lefse
References
Afzal, I., Shinwari, Z. K., Sikandar, S., and Shahzad, S. (2019). Plant beneficial endophytic bacteria: Mechanisms, diversity, host range and genetic determinants. Microbiol. Res. 221, 36–49. doi: 10.1016/j.micres.2019.02.001
Ashiq, S., Edwards, S. G., Fatukasi, O., Watson, A., and Back, M. A. (2022). In vitro activity of isothiocyanates against Fusarium graminearum. Plant Pathol. 71, 594–601. doi: 10.1111/ppa.13505
Barboza, G. E., Garcia, C. C., de Bem Bianchetti, L., Romero, M., and Scaldaferro, M. (2022). Monograph of wild and cultivated chili peppers (Capsicum L., Solanaceae). PhytoKeys 200, 1–423. doi: 10.3897/phytokeys.200.71667
Barea-Ramos, J. D., Rodriguez, M. J., Calvo, P., Melendez, F., Lozano, J., and Martin-Vertedor, D. (2024). Inhibition of Botrytis cinerea in tomatoes by allyl-isothiocyanate release from black mustard (Brassica nigra) seeds and detection by E-nose. Food Chem. 432:137222. doi: 10.1016/j.foodchem.2023.137222
Berendsen, R. L., Pieterse, C. M. J., and Bakker, P. A. H. M. (2012). The rhizosphere microbiome and plant health. Trends Plant Sci. 17, 478–486. doi: 10.1016/j.tplants.2012.04.001
Castellano-Hinojosa, A., Boyd, N. S., and Strauss, S. L. (2022). Impact of fumigants on non-target soil microorganisms: A review. J. Hazard. Mater. 427:128149. doi: 10.1016/j.jhazmat.2021.128149
Chen, J., Feng, W., Yan, H., Liu, P., Zhou, G., Guo, S., et al. (2022). Explore the interaction between root metabolism and rhizosphere microbiota during the growth of Angelica sinensis. Front. Plant Sci. 13:1005711. doi: 10.3389/fpls.2022.1005711
Chen, S., Waghmode, T. R., Sun, R., Kuramae, E. E., Hu, C., and Liu, B. (2019). Root-associated microbiomes of wheat under the combined effect of plant development and nitrogen fertilization. Microbiome 7:136. doi: 10.1186/s40168-019-0750-2
Compant, S., Samad, A., Faist, H., and Sessitsch, A. (2019). A review on the plant microbiome: Ecology, functions, and emerging trends in microbial application. J. Adv. Res. 19, 29–37. doi: 10.1016/j.jare.2019.03.004
Cordovez, V., Dini-Andreote, F., Carrion, V. J., and Raaijmakers, J. M. (2019). Ecology and evolution of plant microbiomes. Annu. Rev. Microbiol. 73:69. doi: 10.1146/annurev-micro-090817-062524
Dahlin, P., and Hallmann, J. (2020). New insights on the role of Allyl isothiocyanate in controlling the root knot nematode meloidogyne hapla. Plants 9:603. doi: 10.3390/plants9050603
Fang, W., Wang, Q., Li, Y., Hua, J., Jin, X., Yan, D., et al. (2022). Microbial regulation of nitrous oxide emissions from chloropicrin-fumigated soil amended with biochar. J. Hazard. Mater. 429:128060. doi: 10.1016/j.jhazmat.2021.128060
Guo, T., Yao, X., Wu, K., Guo, A., and Yao, Y. (2024). Response of the rhizosphere soil microbial diversity to different nitrogen and phosphorus application rates in a hulless barley and pea mixed-cropping system. Appl. Soil Ecol. 195:105262. doi: 10.1016/j.apsoil.2023.105262
Handiseni, M., Zhou, X., and Jo, Y. (2017). Soil amended with Brassica juncea plant tissue reduces sclerotia formation, viability and aggressiveness of Rhizoctonia solani AG1-IA towards rice. Crop Prot. 100, 77–80. doi: 10.1016/j.cropro.2017.06.009
Hartmann, M., and Six, J. (2023). Soil structure and microbiome functions in agroecosystems. Nat. Rev. Earth Environ. 4, 4–18. doi: 10.1038/s43017-022-00366-w
He, X., Wang, D., Jiang, Y., Li, M., Delgado-Baquerizo, M., Mclaughlin, C., et al. (2024). Heritable microbiome variation is correlated with source environment in locally adapted maize varieties. Nat. Plants 10, 598–617. doi: 10.1038/s41477-024-01654-7
Huang, B., Yan, D., Wang, Q., Fang, W., Song, Z., Cheng, H., et al. (2020). Effects of dazomet fumigation on soil phosphorus and the composition of phoD -harboring microbial communities. J. Agric. Food. Chem. 68, 5049–5058. doi: 10.1021/acs.jafc.9b08033
Huang, X., Zeng, Z., Chen, Z., Tong, X., Jiang, J., He, C., et al. (2022). Deciphering the potential of a plant growth promoting endophyte Rhizobium sp. WYJ-E13, and functional annotation of the genes involved in the metabolic pathway. Front. Microbiol. 13:1035167. doi: 10.3389/fmicb.2022.1035167
Li, Y., Lu, D., Xia, Y., Xu, X., Huang, H., Mei, X., et al. (2023). Effects of allyl isothiocyanate fumigation on medicinal plant root knot disease control, plant survival, and the soil bacterial community. BMC Microbiol. 23:278. doi: 10.1186/s12866-023-02992-w
Liu, T., Liao, Q. H., Yu, F. Q., Zi, S. H., Tian, S. H., and Fan, L. Y. (2022). Plant growth - promoting activities of bacterial endophytes isolated from the medicinal plant Pairs polyphylla var. Yunnanensis. World J. Microbiol. Biotechnol. 38:15. doi: 10.1007/s11274-021-03194-0
Magoc, T., and Salzberg, S. L. (2011). Flash: Fast length adjustment of short reads to improve genome assemblies. Bioinformatics 27, 2957–2963. doi: 10.1093/bioinformatics/btr507
Mao, L., Wang, Q., Yan, D., Ma, T., Liu, P., Shen, J., et al. (2014). Evaluation of chloropicrin as a soil fumigant against Ralstonia solanacarum in ginger (Zingiber officinale Rosc.) production in China. PLoS One 9:e91767. doi: 10.1371/journal.pone.0091767
Olenska, E., Malek, W., Wojcik, I., Swiecicka, I., Thijs, S., and Vangronsveld, J. (2020). Beneficial features of plant growth-promoting rhizobacteria for improving plant growth and health in challenging conditions: A methodical review. Sci. Total Environ. 743:140682. doi: 10.1016/j.scitotenv.2020.140682
Otoni, C. G., de Fatima Ferreira, Soares, N., Silva, W. A. D., Medeiros, E. A. A., and Junior, J. C. B. (2014). Use of Allyl isothiocyanate-containing sachets to reduce aspergillus flavus sporulation in peanuts. Packag. Technol. Sci. 27, 549–558. doi: 10.1002/pts.2063
Park, D., Park, S., Liu, K., and Ku, K. (2023). Optimal allyl isothiocyanate concentration on Botrytis cinerea during the postharvest storage of blackberries and mechanism of blackberry color changes at high concentration of allyl isothiocyanate. Postharvest Biol. Technol. 199:112292. doi: 10.1016/j.postharvbio.2023.112292
Phurailatpam, L., Gupta, A., Sahu, P. K., and Mishra, S. (2022). Insights into the functional potential of bacterial endophytes from the ethnomedicinal plant, Piper longum L. Symbiosis 87, 165–174. doi: 10.1007/s13199-022-00864-x
Ren, F., Dong, W., and Yan, D. (2019). Organs, cultivars, soil, and fruit properties affect structure of endophytic mycobiota of pinggu peach trees. Microorganisms 7:322. doi: 10.3390/microorganisms7090322
Ren, Z., Li, Y., Fang, W., Yan, D., Huang, B., Zhu, J., et al. (2018). Evaluation of allyl isothiocyanate as a soil fumigant against soil-borne diseases in commercial tomato (Lycopersicon esculentum Mill.) Production in China. Pest Manag. Sci. doi: 10.1002/ps.4911 Online ahead of print.
Scherling, C., Ulrich, K., Ewald, D., and Weckwerth, W. (2009). A metabolic signature of the beneficial interaction of the endophyte paenibacillus sp. Isolate and in vitro-grown poplar plants revealed by metabolomics. Mol. Plant. Microbe. Interact. 22, 1032–1037. doi: 10.1094/MPMI-22-8-1032
Sessitsch, A., Hardoim, P., Doring, J., Weilharter, A., Krause, A., Woyke, T., et al. (2012). Functional characteristics of an endophyte community colonizing rice roots as revealed by metagenomic analysis. Mol. Plant. Microbe. Interact. 25, 28–36. doi: 10.1094/MPMI-08-11-0204
Shah, S., Shah, B., Sharma, R., Rekadwad, B., Shouche, Y. S., Sharma, J., et al. (2022). Colonization with non-mycorrhizal culturable endophytic fungi enhances orchid growth and indole acetic acid production. BMC Microbiol. 22:101. doi: 10.1186/s12866-022-02507-z
Tagele, S. B., Kim, R., and Shin, J. (2021). Interactions between brassica biofumigants and soil microbiota: Causes and impacts. J. Agric. Food. Chem. 69, 11538–11553. doi: 10.1021/acs.jafc.1c03776
Ugolini, L., Martini, C., Lazzeri, L., D’Avino, L., and Mari, M. (2014). Control of postharvest grey mould (Botrytis cinerea Per.: Fr.) On strawberries by glucosinolate-derived allyl-isothiocyanate treatments. Postharvest Biol. Technol. 90, 34–39. doi: 10.1016/j.postharvbio.2013.12.002
Wang, X., Wang, Q., Zhang, D., Liu, J., Fang, W., Li, Y., et al. (2024). Fumigation alters the manganese-oxidizing microbial communities to enhance soil manganese availability and increase tomato yield. Sci. Total Environ. 919:170882. doi: 10.1016/j.scitotenv.2024.170882
Weinert, N., Piceno, Y., Ding, G., Meincke, R., Heuer, H., Berg, G., et al. (2011). PhyloChip hybridization uncovered an enormous bacterial diversity in the rhizosphere of different potato cultivars: Many common and few cultivar-dependent taxa. FEMS Microbiol. Ecol. 75, 497–506. doi: 10.1111/j.1574-6941.2010.01025.x
Wu, H., Cui, H., Fu, C., Li, R., Qi, F., Liu, Z., et al. (2024). Unveiling the crucial role of soil microorganisms in carbon cycling: A review. Sci. Total Environ. 909:168627. doi: 10.1016/j.scitotenv.2023.168627
Wu, H., Zhang, G. A., Zeng, S., and Lin, K. C. (2009). Extraction of allyl isothiocyanate from horseradish (Armoracia rusticana) and its fumigant insecticidal activity on four stored-product pests of paddy. Pest Manag. Sci. 65, 1003–1008. doi: 10.1002/ps.1786
Yang, B., Wang, X., Ma, H., Jia, Y., Li, X., and Dai, C. (2014). Effects of the fungal endophyte Phomopsis liquidambari on nitrogen uptake and metabolism in rice. Plant Growth Regul. 73, 165–179. doi: 10.1007/s10725-013-9878-4
Yang, K., Fu, R., Feng, H., Jiang, G., Finkel, O., Sun, T., et al. (2023). RIN enhances plant disease resistance via root exudate-mediated assembly of disease- suppressive rhizosphere microbiota. Mol. Plant. 16, 1379–1395. doi: 10.1016/j.molp.2023.08.004
Yu, H., Jia, W., Zhao, M., Le, Li, Liu, J., et al. (2022). Antifungal mechanism of isothiocyanates against Cochliobolus heterostrophus. Pest Manag. Sci. 78, 5133–5141. doi: 10.1002/ps.7131
Yu, J., Vallad, G. E., and Boyd, N. S. (2019). Evaluation of allyl isothiocyanate as a soil fumigant for tomato (Lycopersicon esculentum Mill.) production. Plant Dis. 103, 2764–2770. doi: 10.1094/PDIS-11-18-2013-RE
Zhang, W., Mao, G., Zhuang, J., and Yang, H. (2023). The co-inoculation of Pseudomonas chlororaphis H1 and Bacillus altitudinis Y1 promoted soybean [Glycine max (L.) Merrill] growth and increased the relative abundance of beneficial microorganisms in rhizosphere and root. Front. Microbiol. 13:1079348. doi: 10.3389/fmicb.2022.1079348
Zhang, Y., Fang, W., Yan, D., Ji, Y., Chen, X., Guo, A., et al. (2023). Comparison of drip-irrigated or injected allyl isothiocyanate against key soil-borne pathogens and weeds. Pest Manag. Sci. 79, 3860–3870. doi: 10.1002/ps.7590
Zhou, N., Mu, M., Xie, H., Wu, Y., Zhou, Y., and Li, W. (2021). Rhizospheric fungal diversities and soil biochemical factors of fritillaria taipaiensis over five cultivation years. Horticulturae 7:560. doi: 10.3390/horticulturae7120560
Zou, Y., Zhang, Y., Zheng, E., Zhou, H., Qu, H., and Yang, H. (2024). Integration of transcriptomics and metabolomics to reveal the mechanism of allyl isothiocyanate delaying postharvest quality deterioration of Coprinus comatus. Postharvest Biol. Technol. 216:113075. doi: 10.1016/j.postharvbio.2024.113075
Keywords: soil fumigation, endophyte, soil microorganism, AITC, genotype, pepper
Citation: Tian W, Wang Q, Cao A, Yan D, Li Y, Liu Z, Yang B and Fang W (2025) Genotype-dependent responses of pepper endophytes to soil microbial community shifts induced by allyl isothiocyanate fumigation. Front. Microbiol. 16:1520443. doi: 10.3389/fmicb.2025.1520443
Received: 31 October 2024; Accepted: 25 March 2025;
Published: 14 May 2025.
Edited by:
Paola Grenni, National Research Council, ItalyReviewed by:
Setu Bazie Tagele, University of California, Riverside, United StatesJunli Cao, Shanxi Agricultural University, China
Copyright © 2025 Tian, Wang, Cao, Yan, Li, Liu, Yang and Fang. This is an open-access article distributed under the terms of the Creative Commons Attribution License (CC BY). The use, distribution or reproduction in other forums is permitted, provided the original author(s) and the copyright owner(s) are credited and that the original publication in this journal is cited, in accordance with accepted academic practice. No use, distribution or reproduction is permitted which does not comply with these terms.
*Correspondence: Wensheng Fang, ZmFuZ3dlbnNoZW5nQGNhYXMuY24=; Zhoubin Liu, aG5semIyMDIwQGh1bmF1LmVkdS5jbg==; Bozhi Yang, eWFuZ3lfMTIyM0AxNjMuY29t