- 1Academy of Life Science, Liaoning University, Shenyang, China
- 2Department of Dermatology, University of Hong Kong Shenzhen Hospital, Shenzhen, China
- 3Department of Dermatology, The Eighth Affiliated Hospital, Sun Yat-sen University, Shenzhen, China
Introduction: Sporotrichosis is a fungal disease caused by a complex of Sporothrix schenckii, leading to chronic infections of the epidermis and subcutaneous tissue in both humans and animals.
Methods: Through virtual screening targeting the key gene abaA to screen out the small-molecule drugs to treat Sporotrichosis. To further validate the antifungal activity of small-molecule drugs, growth curves, minimum bactericidal concentration (MBC), and minimum inhibitory concentration (MIC) for Sporothrix globosa (S. globosa) and Sporothrix schenckii (S. schenckii) were measured. In addition, we have done animal experiments to explore the function of the drugs. At the same time, qRT-PCR and transcriptome were used to verify the important role of abaA gene in Sporothrix.
Results: Azelastine and Mefloquine effectively inhibit S. globosa and S. schenckii. MBC, and MIC for S. globosa and S. schenckii confirmed that both Azelastine and Mefloquine inhibited the growth of S. globosa and S. schenckii. Additionally, animal experiments demonstrated that Azelastine and Mefloquine reduced skin lesions in mice; post-treatment observations revealed improvements in inflammatory infiltration and granuloma formation. Through transcriptome analysis and qRT-PCR for validation, our findings demonstrate that the abaA gene plays a crucial role in regulating the attachment of the Sporothrix cell wall to the host matrix and in melanin regulation. Notably, when the abaA gene was inhibited, there was a marked increase in the expression of repair genes. These results emphasize the significance of the abaA gene in the biology of Sporothrix.
Discussion: Two small-molecule drugs exhibit the ability to inhibit Sporothrix and treat sporotrichosis both in vitro and in murine models, suggesting their potential for development as therapeutic agents for sporotrichosis. And qRT-PCR and transcriptome results underscore the significance of the abaA gene in Sporothrix. Our results lay the foundation for the search for new treatments for other mycosis.
1 Introduction
Sporotrichosis is a fungal disease that leads to chronic fungal infections of the epidermis and subcutaneous tissue in both humans and animals. The pathogenic fungi involved are primarily a complex of S. schenckii (Chakrabarti et al., 2014; Hu et al., 2024). In the classical infection pathway, conidia initiate the fungal infection through the interaction between an implanted wound and decaying plant tissue, which results in the classification of sporotrichosis as a type of rot (Lopes-Bezerra et al., 2018; Lv et al., 2022; Liu et al., 2024). The main pathogens responsible for sporotrichosis include Sporothrix schenckii, Sporothrix globosa, and Sporothrix brasiliensis (Zu et al., 2020). In the northeastern region of our country, the predominant pathogenic strain is Sporothrix globose, however, this species remains underappreciated and poorly researched. To date, there is a limited amount of literature addressing its fundamental research and clinical implications (Nava-Pérez et al., 2022; Höft et al., 2022).
Sporothrix globosa is a dimorphic fungus to which immunocompromised individuals are particularly susceptible. This organism exists as mycelium in the environment at 25°C, from which conidia are released into the air, accompanied by fragments of the mycelium through wind and soil dispersion. When a wound comes into contact with soil or inhaled spores, the pathogen can enter the host at 37°C. Temperature changes can induce the transition to a new form-the yeast phase. Yeast forms are less readily recognized by the body’s immune cells compared to mycelial forms, rendering them less likely to be targeted by the immune response. Consequently, the dimorphic fungus S. globosa is not pathogenic in its mycelial phase but becomes pathogenic in its yeast phase. The variation in symptoms following infection is influenced by the individual’s immune status and differing genotypes. Overall, S. globosa exhibits weak virulence and typically presents with mild symptoms (Balkrishna et al., 2022).
Gene regulatory networks (GRN) control developmental events and play an important role in species evolution (Carroll, 2008; Levine, 2010; Smith et al., 2018), three DNA transcription factors, BrlA, AbaA, and WetA, regulate the developmental program of asexual fruiting bodies. BrlA activation program. AbaA regulates the development of conidiophore. In the ΔabaA mutant, the developmental program halts at the formation of the peduncle base. Consequently, the mutant conidiophores deform into globular structures that are dispersed across the rod-shaped peduncle base, resembling the appearance of an abacus (Clutterbuck, 1969). WetA controls the maturation of asexual spores (Etxebeste et al., 2019; Yu, 2010). Regarding research on the DNA-binding domain of the AbaA transcription factor, Borneman et al. cloned a homolog of the Aspergillus nidulans abaA gene, which encodes the ATTS/TEA DNA-binding domain transcriptional regulator, and transformed it into Penicillium marneffei (Borneman et al. 2000). Their findings indicate that the abaA gene plays a crucial role in the developmental process of transitioning from the mycelial phase to the yeast phase. Additionally, Alex et al. also demonstrated that the amino acid sequence of the AbaA transcription factor contains an ATTS/TEA DNA binding motif (Andrianopoulos and Timberlake, 1994). Targeted deletion of AbaA blocks asexual development at 25°C prior to spore production, resulting in abnormal conidia with repeat terminal cells. Furthermore, the abaA deletion strain fails to properly switch from multinucleated filamentous forms to mononucleated yeast cells at 37°C. Many studies have found the DNA-binding domain of abaA gene is conserved and plays an important role in dimorphic switching (Andrianopoulos and Timberlake, 1994).
In recent years, the emergence of multi-resistant pathogens has made fungal infections increasingly difficult to treat. Consequently, strategies for managing hospital infections and opportunistic infections have garnered significant attention within the scientific community (Perlroth et al., 2007; Badiee and Hashemizadeh, 2014). Currently, treatment options for sporotrichosis are limited, and emerging resistance is a concern (Zhang et al., 2024; Brown-Elliott et al., 2001), there was no improvement observed after 16 months of treatment with terbinafine, fluconazole, and itraconazole. Consequently, there is an urgent need for new treatments for sporotrichosis.
Molecular docking is an important technology in computer-aided drug design, which is widely used in new drug development (Farha and Brown, 2019). The process of researching and developing new drugs is lengthy and requires substantial financial resources. Currently, the issue of fungal drug resistance is becoming increasingly severe. In recent years, a notable trend has emerged in the development of new drugs that involves the integration of various disciplines, particularly the combination of biology, computer science, and chemistry. With advancements in computer science, computer-aided drug design has become a prominent focus in the development of new drugs, especially through the use of molecular docking techniques (Swamidass, 2011; Jadamba and Shin, 2016). The development of new drugs is a lengthy and inefficient process, primarily due to challenges related to identifying new targets, ensuring safety, and managing significant associated costs. Consequently, repurposing drugs that have already been approved for other human conditions may offer a more expedient approach to discovering new antifungal agents. In various areas of clinical research, drug repurposing has emerged as a strategy to accelerate the development of new therapies, often utilizing drug-based phenotypic screening methods or high-throughput screening of FDA-approved drug libraries (Park, 2019). In this context, target recognition can be used to target new diseases for drug repurpose (Ma et al., 2022). So this study will look for new ways to treat sporotrichosis through a combination of bioinformatics and traditional experiments. The abaA gene plays a crucial role in the dimorphic switch of Sporothrix, and the downstream virulence factors, along with other related genes regulated by the abaA gene, were also investigated using bioinformatics and transcriptomic methods.
2 Materials and methods
2.1 Screening of small molecule drugs targeting AbaA protein
The target was AbaA protein, a key dimorphic switch protein of S. schenckii, used the Robetta1 to predict the three-dimensional structure of AbaA, the DNA binding domain of AbaA protein was found by bioinformatics analysis. Then, the DNA-binding domain portion of the model’s highest-quality three-dimensional structure was truncated for binding pocket prediction. The grid box was determined by aligning the structure of the AbaA DNA domain, and the x, y, and z-coordinates of the grid box (x = 67.154, y = 85.033, z = −92.830; x = 58.693, y = 85.350, z = −82.023) were determined. AutoDock Vina was then used to perform bulk molecular docking in the FDA-approved small molecule database, the docking results were comprehensively analyzed in terms of binding energy, price, pharmacodynamics and side effects (Moreira et al., 2021; Haozhen et al., 2023).
2.2 Fungal strain and culture conditions
The strain of S. globosa, S. schenckii used was maintained at the Research Center for Pathogenic Fungi, Liaoning University, China. To obtain a mycelial culture, the S. globosa, S. schenckii was inoculated onto Sabouraud dextrose agar (SDA) solid medium (10 g/L tryptone, 40 g/L glucose, 15 g/L agar) and incubated at 25°C. To induce the switch of S. globosa and S. schenckii from the mycelial phase to the yeast phase, mycelial culture was enriched and transferred to brain-heart infusion (BHI) liquid medium, which was incubated at 37°C.
2.3 In vitro antifungal susceptibility
Antifungal susceptibility testing was performed using the proto-cols described in the CLSI document. Sporothrix was cultured at 25°C for 4 days, followed by filtration and centrifugation. The spores were resuspended in autoclaved BHI liquid medium, adjusting the spore concentration to 1 × 105 CFU/mL. In a 96-well plate, 200 μL of the test drug working solution was added to Well 1, while 100 μL of BHI liquid medium was added to Wells 2–11. Well 12 received 200 μL of BHI liquid medium as a negative control. A 100 μL aliquot from Well 1 was transferred to Well 2 and gently mixed by pipetting up and down. This serial dilution process was repeated for Wells 3–10. After mixing Well 10, 100 μL of the supernatant was discarded. Finally, 100 μL of the prepared spore suspension (from Step 2) was added to each well. MIC and MBC results were read by visual inspection and from the readings of the cell optical density at an absorbance of 625 nm (OD625) (Joao et al., 2020; Van Cutsem et al., 1994).
2.4 Measurement of growth curve
The Sporothrix cultured at 25°C with shaking at 150 rpm for 4 days were transferred to 50-mL sterile centrifuge tubes and centrifuged at 8,000 rpm for 5 min. The supernatant was then discarded. The mycelium was diluted in BHI medium, in groups, the final concentration of the drug was 50 μg/mL by adding quantitative Sporothrix suspension and DMSO-dissolved small molecule drug in groups. 37°C for 96 h, OD625 was measured and photographed under bright-field microscopy at 40 × magnification every 12 h, the experiment was repeated three times for each group.
2.5 Murine model of sporotrichosis
Male 8-week-old KM mice were purchased from Liaoning Changsheng Biotechnology Co. Ltd., permit No. SCXK (Liao)2020–0001. The mice in all groups were injected intraperitoneally with cortisol solution every other day for 1 week before inoculation with sporotrichosis suspension. 20 mg/kg, after 1 week it was changed to every 2 days, gavage administration was started after successful modeling (Feng et al., 2010; Boyce et al., 2011). All groups of mice had their abdominal skin shaved with a razor before being injected with Sporothrix suspension, 0.1 mL Sporothrix suspension was injected intradermically into one of the hair-removal sites with a 1 mL syringe, it contains about 1 × 107 spores.
2.6 Small molecule drugs treatment
After modeling, Daily Gavage was started. Mice were randomly assigned to two treatment cohorts: the Azelastine treatment group and Mefloquine treatment group. These cohorts were further stratified into high-dose (Azelastine: 6 mg/kg/day, Mefloquine: 20 mg/kg/day), low-dose (Azelastine: 3 mg/kg/day, Mefloquine: 3.8 mg/kg/day), and a control group receiving 0.5% carboxymethylcellulose sodium salt.
2.7 Histological examination of the skin
Following drug administration, the mice were euthanized through cervical dislocation and immersed for 5 min in a 5% phenol solution for disinfection purposes. The specimens were then rinsed three times with autoclaved sterile distilled water and placed on a sterile dissecting board under aseptic conditions. Skin lesions were excised and immediately fixed in a 4% paraformaldehyde solution for histopathological processing. Skin lesion samples were sent to Jijia for HE staining, the infiltration of inflammatory cells and the formation of granuloma were observed and analyzed.
2.8 Statistical analysis
The study groups were compared statistically using the SPSS 23.0. Significance for all statistical tests is shown in the figures for p < 0.05, p < 0.01, and p < 0.001.
2.9 Ethics statement
The experiment was conducted in strict accordance with the Guide for the Care and Use of Laboratory Animals.
2.10 cDNA library construction and sequencing
Total RNA was extracted from the mycelial phase of a 48-h culture, the yeast phase of 48-h induction and the liquid of 48-h culture following the addition of Azelastine. The total amount and purity of the extracted RNA were assessed. After qualification, eukaryotic mRNA was enriched using magnetic beads with Oligo (DT). Fragmentation buffer was subsequently added to cleave the mRNA into shorter fragments. Using the mRNA as a template, single-stranded cDNA was synthesized with random primers, followed by the addition of RNase H to produce double-stranded cDNA. The resulting double-stranded cDNA was purified, its ends were repaired, a tail was added, and sequencing linkers were connected. Fragments were selected using AMPure XP beads. Finally, PCR amplification and purification were performed to obtain the final library. The libraries were quality-checked, and those meeting the criteria were subjected to PE150 sequencing using the Illumina HiSeq 2,500 high-throughput sequencing platform.
2.11 Transcriptome analysis
First, the quality of sequencing data was evaluated using FASTP (Chen et al., 2018) software. Following quality control, the sequencing data were aligned with ribosome sequences from the NCBI RefSeq (Pruitt et al., 2007) and RFAM (Kalvari et al., 2021) databases utilizing Bowtie 2 (Langmead et al., 2019) software. The alignment results were statistically processed using samtools (Danecek et al., 2021) and subsequently compared to the reference genome with Bowtie 2 (Langmead et al., 2019). Transcript assembly was performed with StringTie (Pertea et al., 2015) software, leveraging available reference information, followed by quantitative analysis of gene expression levels. Once the transcript read counts were obtained, they were converted to gene read counts using the R package maximport (Soneson et al., 2015). After acquiring read counts for all samples, we employed the differential analysis software DESEQ2 (Love et al., 2014) to conduct differential expression analysis of genes. For this analysis, we utilized the GO, KEGG, and EggNOG databases for functional annotation, functional enrichment, and GSEA analyses.
2.12 Real-time quantitative PCR
Approximately 100 mg of S. globosa mycelial-phase cells and yeast-phase cells were collected and rapidly frozen in liquid nitrogen. The samples were subsequently ground under continuous liquid nitrogen cooling using a mortar and pestle. Total RNA was extracted from both phases using the TRIzol Reagent Kit (Vazyme Biotech Co., Ltd., China) in accordance with the manufacturer’s protocol. Quantitative real-time PCR was conducted on the StepOnePlus system. Following normalization with the 18S rDNA reference gene, the relative expression levels of the target gene between the yeast-phase and mycelial-phase were compared and analyzed using the 2^(-ΔΔCt) method.
3 Results
3.1 Discovery of anti—S. globosa entry inhibitors among candidates in the FDA approved drug library
The abaA gene sequence of S. globosa utilized in this study was obtained through sequencing conducted in our laboratory. The three-dimensional structure of this gene is currently unknown, prompting a search for similar proteins to facilitate further investigation. In order to search for similar proteins of AbaA, we first searched for similar genes of abaA (Table 1), HMPREF1624, which exhibited the highest total score and sequence similarity, was selected and subsequently searched in the UniProt database (Supplementary Table S1). This protein contains a DNA-binding domain known as TEA (Supplementary Table S2), which is identical to that of the AbaA protein. We used the RoseTTAFold module in the Robetta server to predict the three-dimensional structure of the AbaA protein (Figures 1A–E), the five predicted models are evaluated in SAVES v6.0 (Table 2), we finally chose the highest score Result-1 for follow-up processing (Moreira et al., 2021; Cho et al., 2009; Ma et al., 2022). We utilized PyMOL to truncate the AbaA protein’s DNA-binding domain from the model Result-1 (Figure 1A). The binding pockets of this domain were predicted using DoGSiteScorer (Table 3, Figure 1F). We then employed AutoDock Tools to preprocess the top two pockets, setting the size and coordinates of the docking box. Subsequently, we conducted batch molecular docking using AutoDock Vina (Supplementary Table S3). We compared the binding energies and analyzed the primary efficacy and side effects of the selected small molecule drugs. Ultimately, we chose four small molecule drugs, Avodart, Eltrombopag, Azelastine, and Mefloquine, for further study (Figures 1G,H).
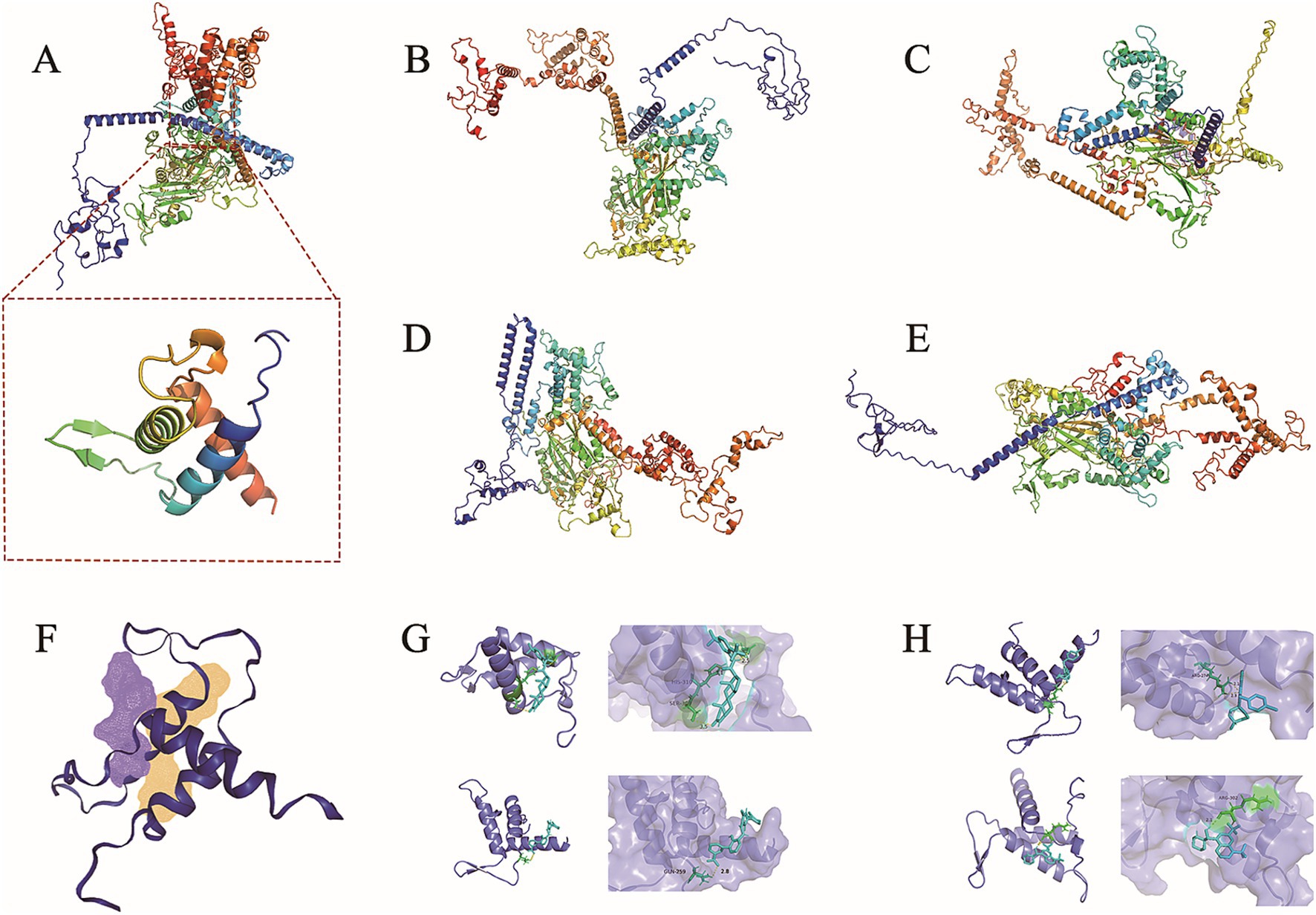
Figure 1. AbaA three-dimensional structure prediction and docking results display. (A–E) The three-dimensional structure of AbaA protein was predicted using Robetta, the three-dimensional structure of AbaA DNA-binding domain is shown under (A). (F) Use DoGSiteScorer to predict the protein-binding pocket. (G,H) The four graphs are Avodart, Eltrombopag, Azelastine, Mefloquine and AbaA DNA binding domain docking results.
3.2 Azelastine and Mefloquine have in vitro antifungal activity against the S. globosa and S. schenckii
In our previous studies, abaA gene expression was upregulated during the dimorphic switch of the S. schenckii from the mycelial phase to the yeast phase, and the deletion of this gene causes S. schenckii to become less resistant to all kinds of stress, these results suggest that the gene is critical for S. schenckii dimorphic switch (Zheng et al., 2021). Therefore, it is reasonable to speculate that this gene is also important for the dimorphic switch of S. globosa. Consequently, we suspect that AbaA is also upregulated during the yeast phase of S. globosa. To explore the antifungal activity of Azelastine and Mefloquine, the selected drugs were administered to S. globosa and the S. schenckii phase reversal process respectively, in order to observe their effects on the dimorphic switch of S. globosa and S. schenckii. The results indicate that Avodart and Eltrombopag have no effect on either fungus (Figures 2A,B). In contrast, Azelastine and Mefloquine showed inhibitory effects on both S. globosa and S. schenckii when compared to the control group and DMSO solvent control group (Figures 2C–F). When Azelastine and Mefloquine were added, the conidia of S. globosa dropped off and did not grow. Both Azelastine and Mefloquine significantly inhibited the growth and spore production of S. globosa and S. schenckii. In the control and solvent control groups, dimorphic switch of S. globosa and S. schenckii occurred, there was no phase inversion in the Azelastine and Mefloquine groups. Thus, both small-molecule drugs inhibited the growth and dimorphic switch of S. globosa and S. schenckii.
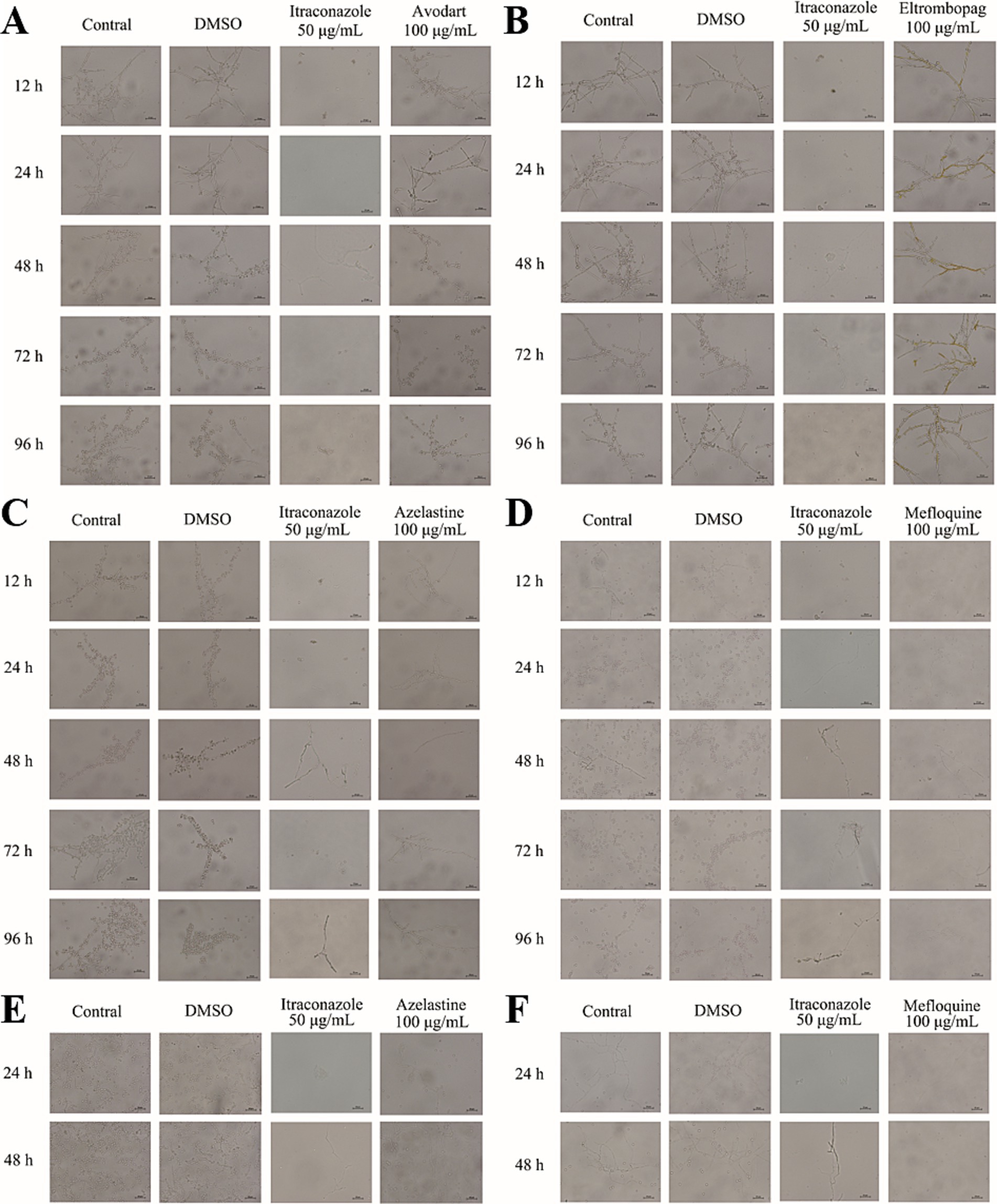
Figure 2. Antifungal activity of the candidate against Sporothrix globose and Sporothrix schenckii under bright-field microscopy at 40 × magnification. (A) In vitro antifungal activity of Avodart against Sporothrix globose. (B) In vitro antifungal activity of Eltrombopag against Sporothrix globose. (C) In vitro antifungal activity of Azelastine against Sporothrix globose. (D) In vitro antifungal activity of Mefloquine against Sporothrix globose. (E) In vitro antifungal activity of Azelastine against Sporothrix schenckii. (F) In vitro antifungal activity of Mefloquine against Sporothrix schenckii. The test concentration was 50 μg/mL, 100 μg/mL, and the selected concentration was 100 μg/mL. The amount of DMSO is the amount of candidate drugs.
3.3 Determination of fungal growth curves, MIC and MBC of Azelastine and Mefloquine
Given the subjective nature of morphological observations, we aimed to further investigate the inhibitory effects of Azelastine and Mefloquine on S. globosa and S. schenckii. To achieve this, we determined the growth curves for these small molecule drugs in relation to the inhibition of both S. globosa and S. schenckii, and we determined the MIC and MBC of the two small molecule drugs. The addition of Azelastine and Mefloquine significantly inhibited the growth of S. globosa and S. schenckii compared with the control group and DMSO solvent control group (Figure 3). Although the measured MIC and MBC values do not show a small-dose advantage over other fungicide (Table 4), these agents are considered safer than antibiotics and are administered at lower doses compared to Itraconazole alone. Both drug stents can also be optimised for the development of new small-molecule drugs to treat sporotrichosis if the clinical dose is higher than the safe range.
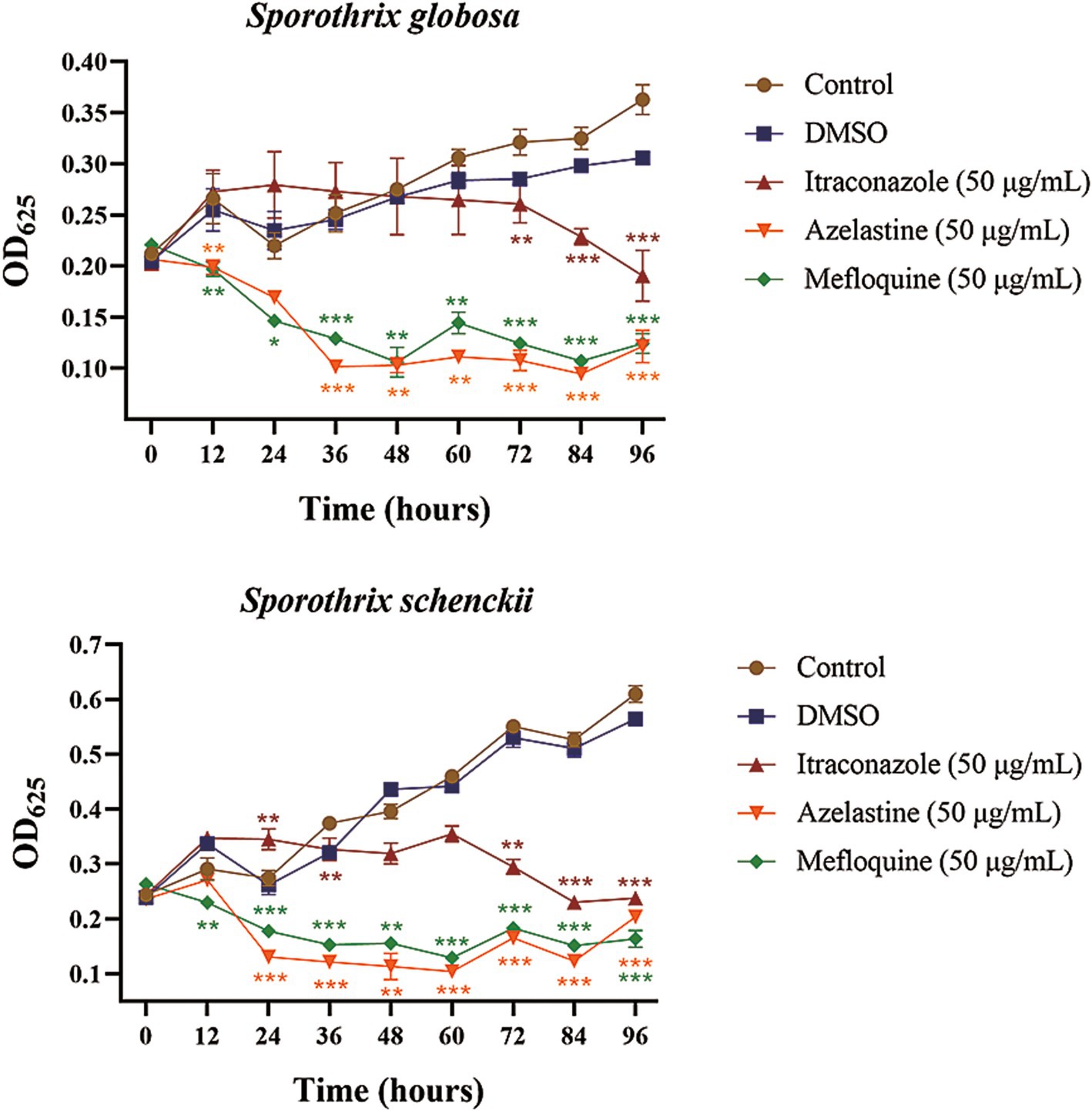
Figure 3. The growth curves of Sporothrix globose and Sporothrix schenckii were inhibited by small molecule drugs. (A) The growth curves of the small molecule drugs for the inhibition of Sporothrix globosa. (B) The growth curves of the small molecule drugs for the inhibition of and Sporothrix schenckii. DMSO group was solvent control group and Itraconazole group was positive control group. *, p < 0.05; **, p < 0.01; ***, p < 0.001. Data processing used SPSS 23.0 for Ducan’s multiple comparison test to analyze its significance.
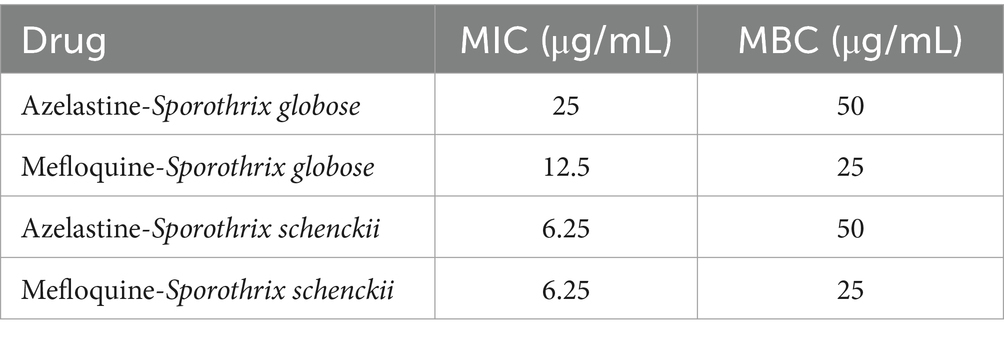
Table 4. Azelastine and Mefloquine inhibited MIC and MBC of Sporothrix globose, Sporothrix schenckii.
3.4 Azelastine and Mefloquine are effective for sporotrichosis
We verify the efficacy of Azelastine and Mefloquine in the treatment of sporotrichosis at the animal level. The model was established by intradermal injection of Sporothrix spore suspension in mice (Figure 4) Intradermal injection of spore into the abdomen of mice produced varying degrees of skin lesions, some grow nodules, some ulcers, or cysts. For some nodules, the pus was collected by gastric perfusion. The pus was dipped into a high-temperature sterilized cotton swab and diluted with sterile distilled water. 40 μL of pus was spread evenly on SDA medium and cultured at 25°C for 4 days, microscopy confirmed S. schenckii (Supplementary Figure S1). Inflammatory ulcers and nodules began to appear in the skin of mice 1 week after intradermal injection, the morphology of the lesions was analyzed by reviewing the literature, and the pus was purified and cultured, microscopic observation showed that the lesions were Sporotrichosis. The Sporotrichosis was most severe in the third week, when the drug was given intragastrically for 10 days, the nodules in the Itraconazole positive control group and the Azelastine and Mefloquine groups became smaller and the crusts were improved (Figure 5). In comparison to the mock group, the control group exhibited larger granulomas and a greater infiltration of inflammatory cells. Notably, the granulomas in the positive control group were smaller than those in the control group. The granulomas observed in both the low and high dose Azelastine groups were wider than those in the positive control group, yet smaller than those in the control group; they were dispersed and did not form a cohesive mass, with milder inflammatory cell infiltration compared to the control group. In contrast, the inflammatory infiltration in both the low and high dose Mefloquine groups did not show improvement relative to the control group; however, the granulomas were significantly smaller, with the inflammatory cells remaining dispersed and not coalescing (Figure 6). The granuloma width of the control group was significantly different from that in both the positive control group and the treatment group (Table 5). Statistical analysis of inflammatory cell statistics showed that there were significant differences between the control group and the positive control group (Table 6).
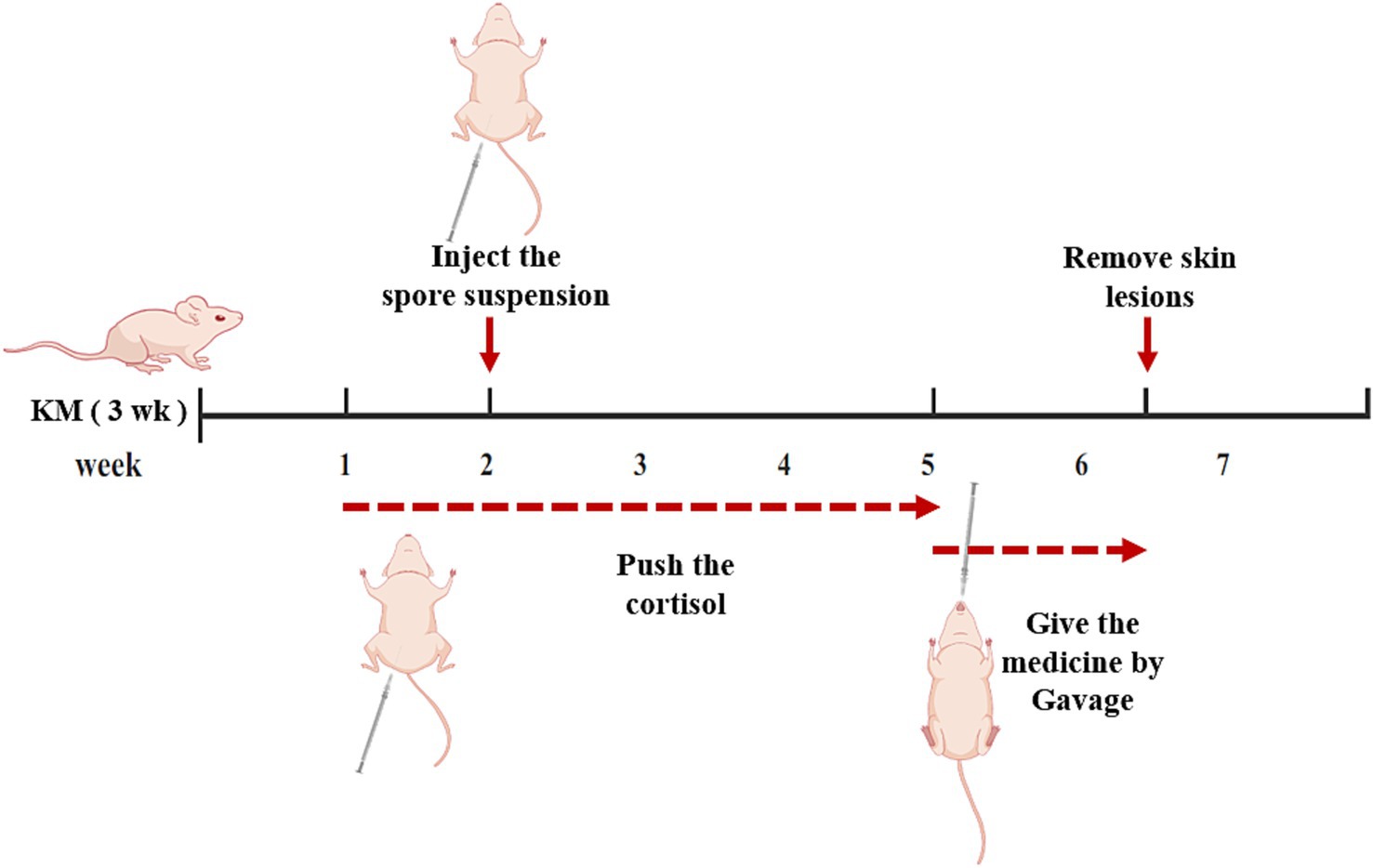
Figure 4. Mouse model and treatment process. After a week of acclimation, the mice were injected with a cortisol solution for immunosuppression, after 1 week of inhibition, the mold was made by injection Sporothrix suspension, meanwhile, immunosuppression was continued. Three weeks after successful modeling, the rats were treated with medicine. After 10 days of administration, mice were killed and skin lesions were examined.
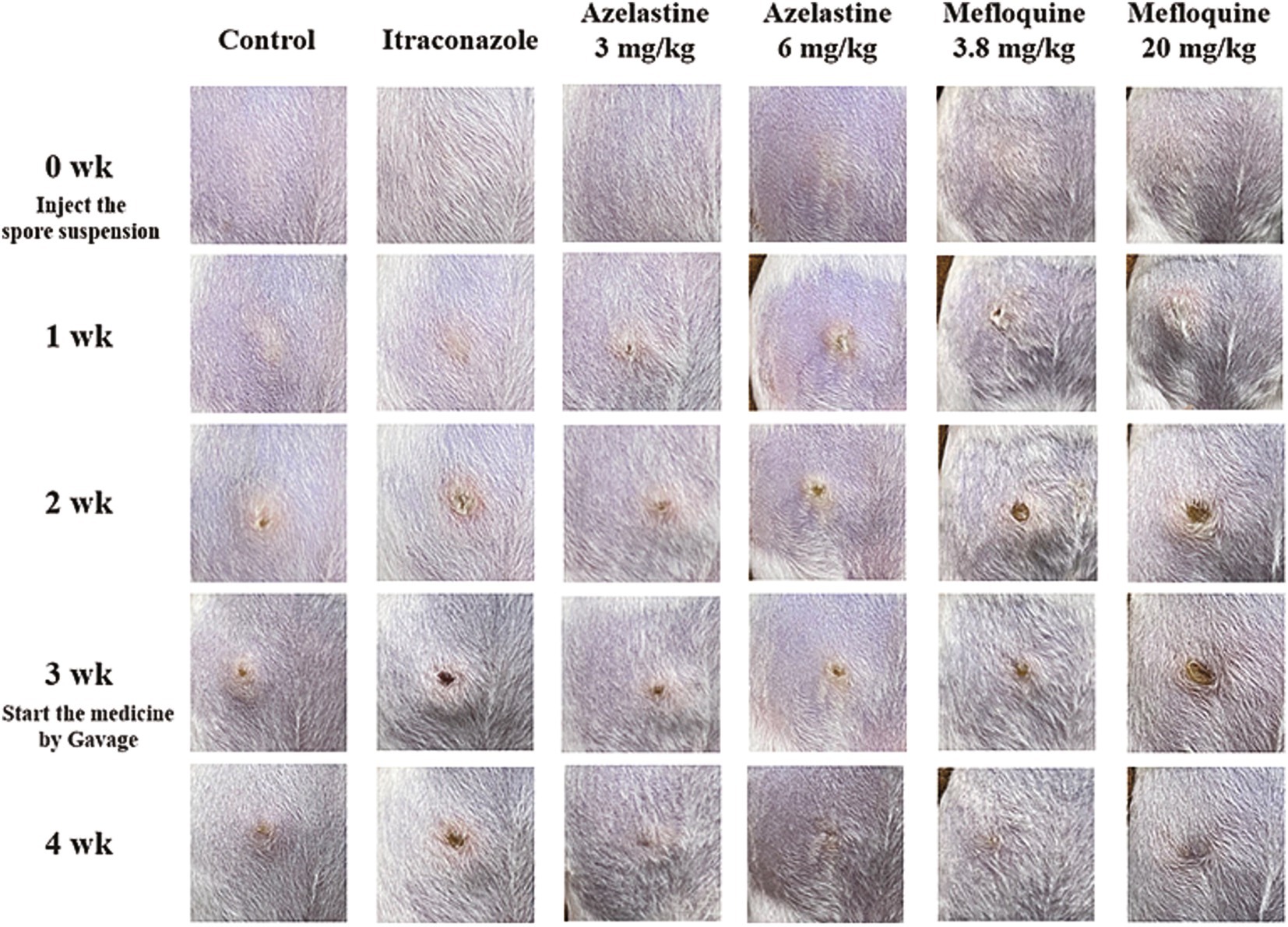
Figure 5. Pictures of mouse model and treatment process. Intradermal injection of Sporothrix suspension into the abdomen of mice produced varying degrees of skin lesions, some grow nodules, some ulcers, or cysts.
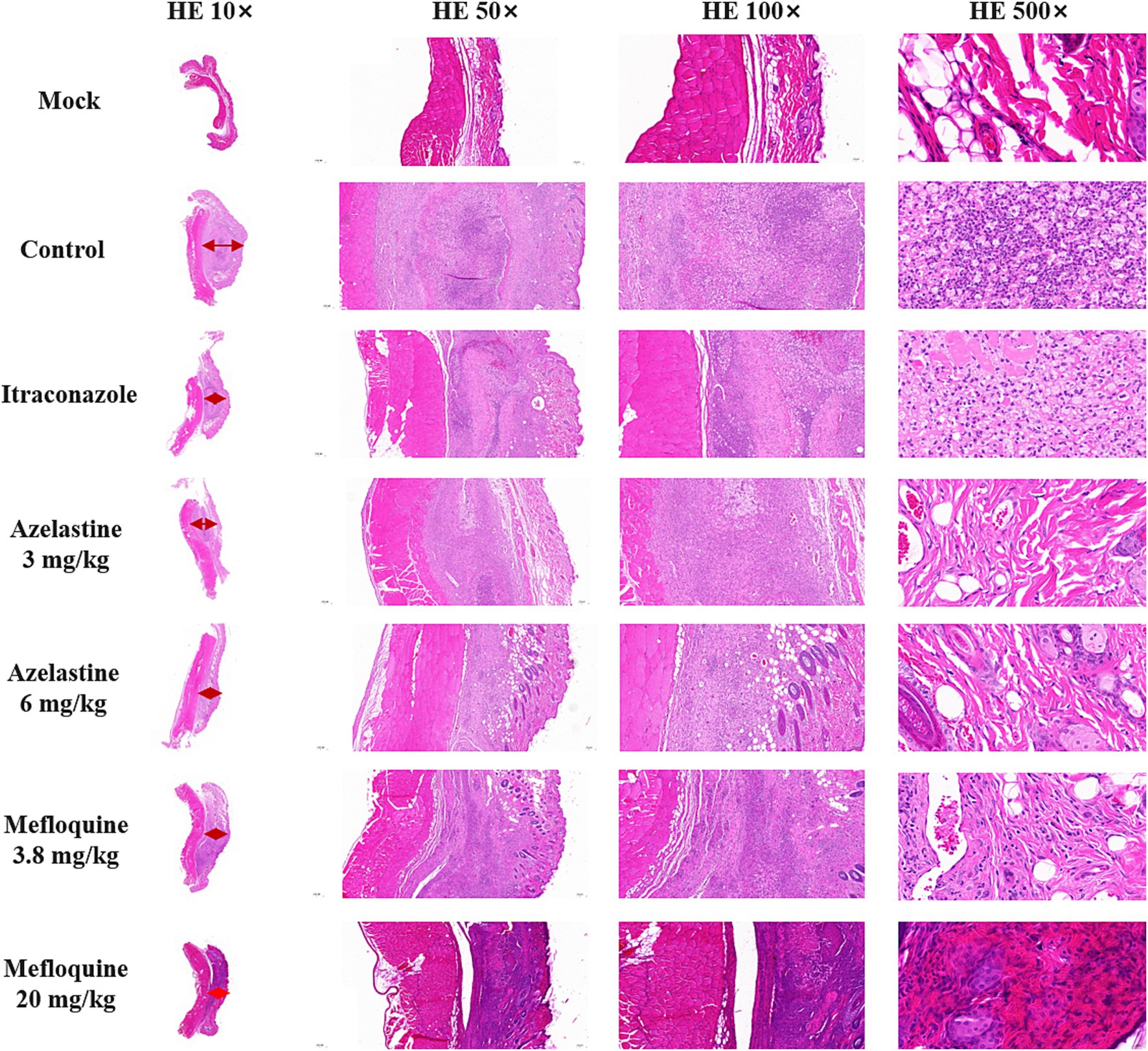
Figure 6. HE staining results of skin lesions in mice. The Mock group was the result of HE staining of healthy skin, the red arrow was granuloma, control group was not given medicine after successful modeling. The width of granuloma in control group was 3,320 μm; the width of granuloma in Itraconazole group was 1,460 μm; the width of granuloma in Azelastine 3 mg/kg group was 1,880 μm; the width of granuloma in Azelastine 6 mg/kg group was 1,480 μm; the width of granuloma in Mefloquine 3.8 mg/kg group was 1,940 μm; the width of granuloma in Mefloquine 20 mg/kg group was 1,251 μm. (Granuloma width was measured by software CaseViewer).
3.5 The regulatory effect of the abaA gene on downstream virulence factors was analyzed using bioinformatics
To further investigate the regulation of the abaA gene on these virulence factors, we reviewed the literature to identify the downstream virulence factors associated with Sporothrix (García-Carnero and Martínez-Álvarez, 2022; Teixeira et al., 2014; Félix-Contreras et al., 2020). We downloaded the gene sequences of these virulence factors from the NCBI and utilized the JASPAR database2 to identify virulence factors containing AbaA binding sites (Andrianopoulos and Timberlake, 1994). The sequences of the abaA binding sites were then compared using DANMAN sequence alignment software, allowing us to screen for virulence factors that may be regulated by the abaA gene. These genes are GPI-anchored cell wall beta-1,3-endoglucanase EglC, scytalone dehydratase, CFEM domain protein, laccase precursor, molecular chaperone HTPG, DNA mismatch repair protein. This screening was subsequently validated through transcriptome analysis. To validate the reliability of the bioinformatics analysis, we subsequently conducted transcriptome analysis and qRT-PCR for verification.
3.6 Transcriptome sequencing data processing and assembly result statistics
RNA-Seq sequencing yielded between 41,934,980 and 47,331,900 raw reads from nine libraries, with raw bases ranging from 6.29 G to 7.02 G. The number of clean reads varied from 43,514,694 to 8,516,234. The GC content ranged from 54.26 to 56.27%. The Q30(%), which represents the percentage of bases with an accuracy exceeding 99.9%, the Q30 value was greater than 92% in this experiment, indicating that the sequencing quality was reliable (Supplementary Table S5).
Gene expression was analyzed using DESeq, with the criteria for screening differentially expressed genes set at |log2foldchange| > 1 and significance p < 0.05. A total of 2,681 genes were found to be differentially expressed between the Mycelial Phase (MP) and Yeast Phase (YP), of which 1,500 genes were up-regulated and 1,181 genes were down-regulated. Additionally, 5,256 genes were differentially expressed between Yeast Phase + Azelastine (YPA) and Yeast Phase (YP), with 2,100 genes up-regulated and 3,156 genes down-regulated (Figure 7E).
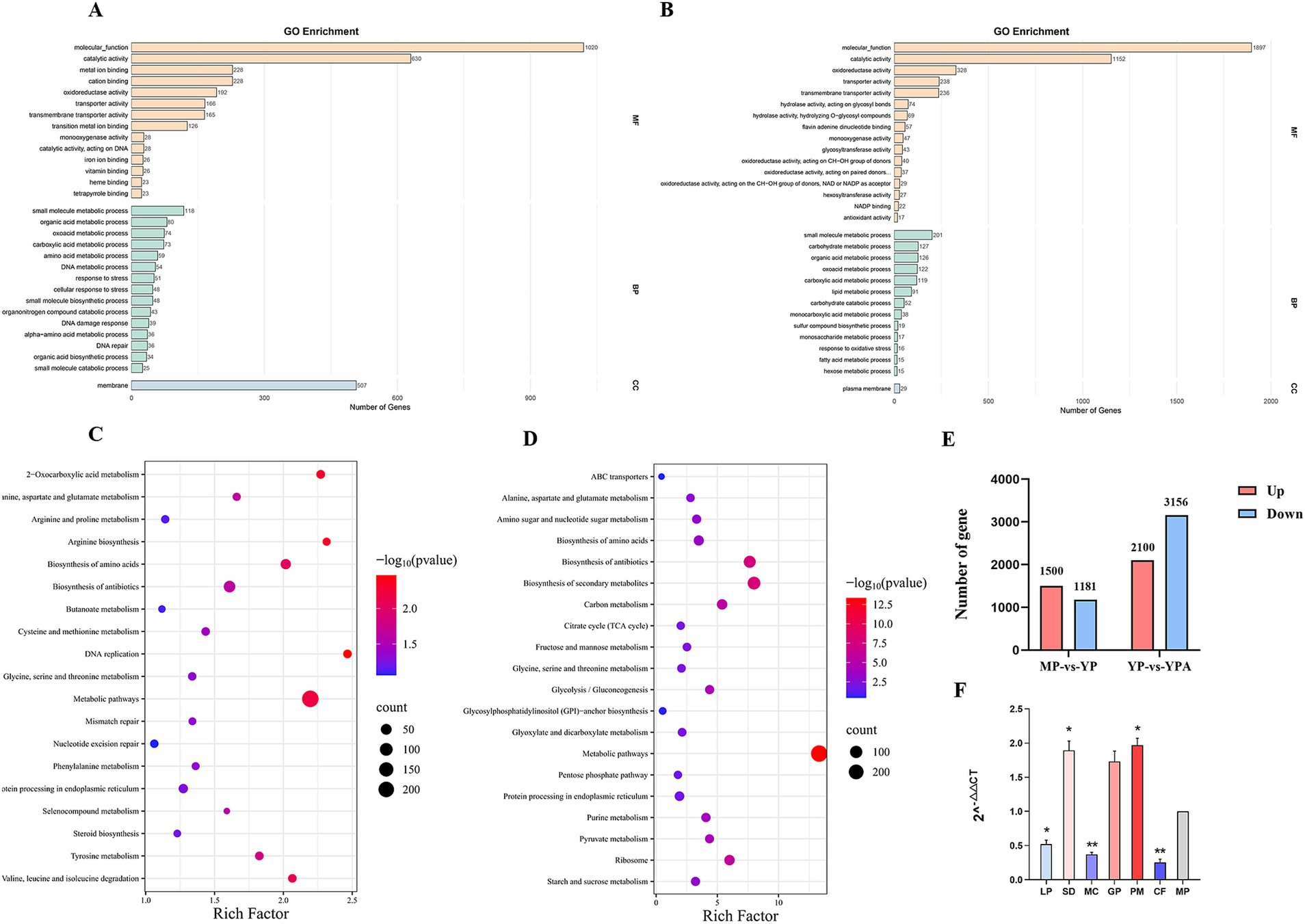
Figure 7. Transcriptome data analysis. (A) Gene Ontology Enrichment Analysis of MP and YP differentially expressed genes. (B) Gene Ontology Enrichment Analysis of YP and YPA differentially expressed genes. (C) Histogram of the Kyoto Encyclopedia of Genes and Genomes pathway classification of differentially-expressed genes of MP and YP. (D) Histogram of the Kyoto Encyclopedia of Genes and Genomes pathway classification of differentially-expressed genes of YP and YPA. (E) Histograms of differentially expressed genes. There were 2,681 genes differing between MP and YP, and 5,256 genes differing between YP and YPA. (F) qRT-PCR showed that abaA regulated virulence factor gene was expressed in yeast phase and mycelial phase with other genes. The expression of each gene was expressed as 2^-△△ CT value (mean ± SEM), and the expression of each gene in the mycelial phase was constant as 1, which served as a baseline comparison with other genes in the yeast phase. Gene: LP: laccase precursor; SD: scytalone dehydratase; MC: molecular chaperone HtpG; GP: GPI-anchored cell wall beta-1,3-endoglucanase EglC; PM: DNA mismatch repair protein. CF: CFEM domain protein. The significance was analyzed by independent sample t test (*, p < 0.05; **, p < 0.01). MP: mycelial phase baseline.
We utilized the Gene Ontology (GO) and Kyoto Encyclopedia of Genes and Genomes (KEGG) databases to analyze significantly differentially expressed genes between the MP and YP, as well as between the YP and YPA. The GO functional enrichment analysis for the comparison of the MP versus YP (Figure 7A) revealed that 1,020 differentially expressed genes were enriched in molecular functions, with 630 genes enriched in catalytic activity and 507 genes enriched in membrane functions. The KEGG pathway enrichment analysis (Figure 7C) indicated that the differentially expressed genes in the treatment group were significantly enriched in metabolic and biosynthetic pathways, such as valine, leucine, and isoleucine degradation (KEGG: ko00280) and amino acid biosynthesis (KEGG: ko01230). The enrichment of these differential genes in the aforementioned functions and pathways suggests potential changes in cell membrane structure and the production of virulence factors during the dimorphic transformation of Sporothrix. Notably, serine metabolism can generate precursors for glutathione, enhancing the fungus’s ability to cope with reactive oxygen species (ROS) produced by the host’s immune response, thereby aiding its survival following macrophage phagocytosis (Cheng et al., 2019).
For the YP and YPA, GO functional enrichment analysis (Figure 7B) revealed that 1,897 differentially expressed genes were enriched in molecular functions, with 1,152 genes enriched in catalytic activity and 328 genes enriched in oxidative activity. Additionally, KEGG pathway enrichment analysis (Figure 7D) demonstrated that the differentially expressed genes in this treatment group were enriched in the biosynthesis of secondary metabolites (KEGG: ko01110) and metabolic pathways. The significant enrichment of differentially expressed genes in molecular functions, catalytic activity, and metabolic pathways indicates that the drug had a substantial impact on the original metabolic activities of the strain. Furthermore, the functional enrichment of differentially expressed genes in oxidative activity and the biosynthesis of secondary metabolites pathway suggests that the drug may have exerted specific effects on the synthesis of secondary metabolites and the oxidative stress system in the fungal. This further implies potential disturbances in the synthesis of virulence factors and the mechanisms of host immune evasion in the fungal. Transcriptome results indicated that several virulence factors regulated by abaA, including GPI-anchored cell wall beta-1,3-endoglucanase (EGLC), dehydratase, and CFEM domain protein, were significantly down-regulated. Conversely, genes associated with fungal growth and repair, such as laccase precursor, molecular chaperone HTPG, and DNA mismatch repair protein, exhibited significant up-regulation. Further validation and functional analyses of these candidate genes will be performed.
3.7 The results of qRT-PCR were consistent with those of transcriptome
To verify the reliability of the transcriptome results, we performed qRT-PCR validation. (Figure 7F). The primers used are shown in Supplementary Table S4. The results of qRT-PCR were consistent with those of transcriptome analysis (Figure 8). Interestingly, among the screened genes, genes related to the cell wall structure of Sporothrix (GPI-anchored cell wall beta-1,3-endoglucanase EglC, CFEM domain protein) were significantly down-regulated; and genes related to melanin (scytalone dehydratase) were significantly down-regulated. Other genes such as DNA repair-related genes (molecular chaperone HtpG, DNA mismatch repair protein) and fungal adaptability-related genes (laccase precursor) were significantly up-regulated. This suggests that the abaA gene plays a crucial role in regulating the anchoring of the Sporothrix cell wall to the host matrix and in the regulation of melanin. Furthermore, inhibition of the abaA gene results in a significant expression of repair genes, highlighting its importance to Sporothrix. We analyzed the transcriptome data of the virulence factors regulated by the abaA gene and observed that the expression levels of these virulence factors were inversely correlated with those of yeast following the addition of Azelastine (Figure 8). This finding further confirms that the expression level of abaA is down-regulated upon the introduction of small molecule drugs, indicating that the abaA gene serves a regulatory function in these virulence factors.
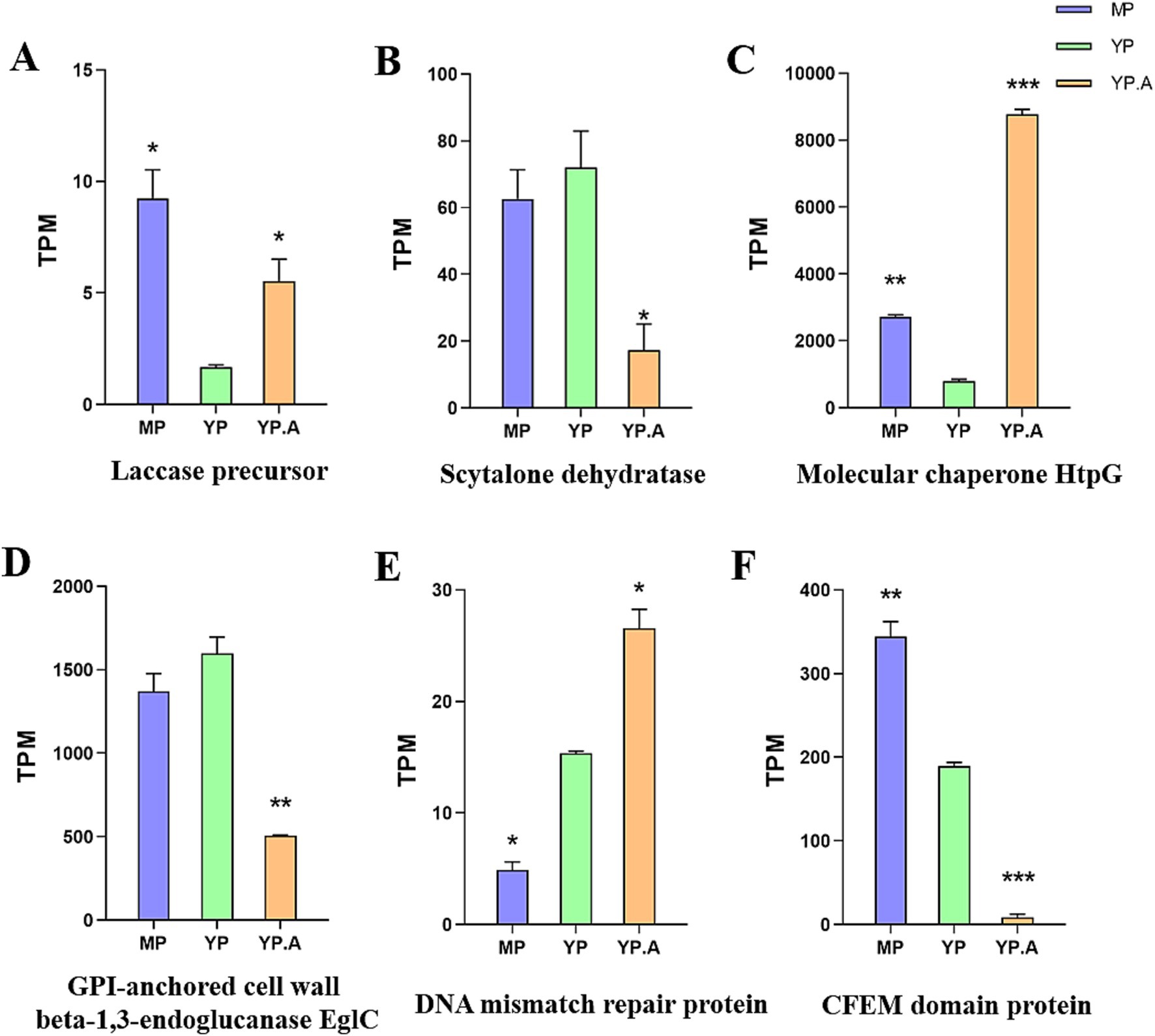
Figure 8. (A-F) The transcriptome shows the ratio of abaA-regulated virulence factors to other genes in mycelial phase, yeast phase, and yeast phase + Azelastine, with results presented as TPM values (mean ± SEM), significance was tested by independent-sample T-test with yeast phase as baseline (*, p < 0.05; **, p < 0.01; ***, p < 0.001).
4 Discussion
Currently, the issue of drug resistance in invasive fungal diseases is becoming increasingly serious, with emerging resistance also observed in sporotrichosis. Therefore, there is an urgent need to search for new small-molecule drugs to treat sporotrichosis and to explore innovative methods for drug discovery. If we want to find new small molecule drugs, we have to find new targets (Ortiz-Ramírez et al., 2022; Cortés Juan Carlos et al., 2019). S. globosa is a dimorphic fungus that exists in the mycelial form in the environment at 25°C, where it is non-pathogenic. Upon entering the body at 37°C, S. globosa transforms into its yeast phase, at which point it becomes pathogenic. The AbaA gene, which is involved in the dimorphic switching process, can influence the virulence of S. globosa, making it a key target for selection. The abaA gene, which plays a crucial role in the dimorphic switch process, can influence the virulence of S. globosa, making it a focal point for target selection. The abaA gene is essential for dimorphic switch; in Talaromyces marneffei, deletion of the abaA gene impacts dimorphic switch (Borneman et al., 2000), while in S. schenckii, deletion of the abaA gene affects virulence. Consequently, we chose abaA gene as a novel drug target.
We employed molecular docking techniques to screen for small molecules that could inhibit S. globosa and S. schenckii. The literature review revealed that almost all invasive mycoses can lead to central nervous system diseases when they are severe (Krysan, 2016). Consequently, it is essential for fungicides to penetrate the blood–brain barrier and achieve elevated serum and tissue concentrations (Bing et al., 2012; Karbwang and Harinasuta, 1992). Through the analysis and screening of molecular docking results, we eliminated the side effects associated with drugs used for treating cancer and psychiatric diseases. Subsequently, we combined considerations of price, efficacy, and in vitro antifungal tests to identify two small-molecule drugs.
Two small-molecule drugs demonstrated a tendency to inhibit the growth of Sporothrix in antifungal experiments. The proposed mechanism involves the regulation of conidiophore development by AbaA. In ΔabaA mutants, the formation of the peduncle is associated with inhibited growth of Sporothrix, leading to the cessation of the developmental program. Furthermore, the molecular structures of the drugs Azelastine and Mefloquine interact with AbaA, resulting in the inhibition of both mycelial and spore development of Sporothrix, as evidenced by a decrease in OD625.Azelastine is a histamine 1 receptor blocker, its role is not an antagonist, but a reverse agonist, reduce the H1 receptor component activity (Watts et al., 2019). Azelastine has few side effects, is low-cost, can be taken for long periods of time and is safe for children over 6 years of age (Konrat et al., 2022). The drug has also been shown to have in vitro antiviral activity against the coronavirus (Lythgoe and Middleton, 2020). In addition, Azelastine also has anti-inflammatory effects, mainly by stabilizing mast cells and inhibiting the production of leukotrienes and proinflammatory cytokine (Watts et al., 2019). It can also down-regulate the expression of intercellular adhesion molecule-1 and reduce the migration of inflammatory cells. However, the effects of Azelastine on fungi have been poorly studied. Azelastine, a cationic amphiphilic drug belonging to a pharmacologically diverse class of compounds with distinct target molecules (Tummino et al., 2021), has been shown to induce phospholipidosis at the submicron scale. This property suggests that its antifungal activity against Sporothrix may result from a combination of direct fungal growth inhibition and host-mediated mechanisms. Furthermore, Azelastine remains effective when administered as a nasal spray for the treatment of rhinitis, indicating its significant potential as a nasal spray for the treatment of sporotrichosis (Konrat et al., 2022).
The results of pharmacological studies of Mefloquine show that Mefloquine has many useful features in the treatment of fungal infections. First, when taken orally, it can be well absorbed and establishes high serum and tissue levels, second, it can penetrate the blood–brain barrier to high nerve concentrations relative to plasma, Mefloquine has a long half-life, so it can be used as a prophylactic regimen (Montoya et al., 2020). In addition, some studies have shown that Mefloquine has certain antifungal activity against Candida, Cryptococcus and Aspergillus (Montoya et al., 2020). Mefloquine has been shown to inhibit the formation of egg granulomas in Schistosoma japonicum, suggesting that it may play a role in modulating the host immune system in vivo (Huang et al., 2011). Furthermore Mefloquine may inhibits Sporothrix through two mechanisms, in addition to binding to key target proteins of Sporothrix, it may also influence the host immune response. The underlying mechanisms of this interaction will be explored in further studies. The selection of dosing concentration was informed by a review of pertinent literature, indicating that both Azelastine and Mefloquine are capable of penetrating the blood–brain barrier and possess potential therapeutic effects against fungal infections. With regard to Azelastine, The pharmacokinetic results of the drug demonstrated Azelastine 16 mg did not increase side effects, and the maximum dose for adults was 40 mg, through the above pharmacokinetics analysis, combined with mouse and human drug delivery conversion formula, we selected two dosing concentrations of 3 mg/kg and 6 mg/kg, to conduct animal experiments (McTavish and Sorkin, 1989). About Mefloquine, adults were given 18–20 mg/kg, or (750–1,250 mg) a day, the clinical pharmacokinetics of Mefloquine showed that the peak time of Mefloquine administration was 6–24 h in healthy subjects, the maximum blood concentration of the drug was 1,000 μg/mL after taking 1,000 mg. Based on the above pharmacokinetics analysis, we selected a dosing concentration of 3.8 mg/kg; we also found that many studies treated neuropathic pain by intraperitoneal administration of Mefloquine at a dose of 20 mg/kg, so we chose a larger concentration of 20 mg/kg to study the effect of Mefloquine on sporotrichosis. As for the choice of the positive itraconazole concentration, the final choice was 60 mg/kg according to the conversion of doses between human and mice (Bonifaz and Vázquez-González, 2010; Saraiva et al., 2012; Moreira et al., 2015).
The abaA gene is crucial for the dimorphic switch of Sporothrix and influences the virulence of certain fungi. In this study, several genes regulated by abaA, including various virulence factors and functional genes, were identified through a combination of bioinformatics predictions and experimental approaches. GPI-anchored cell wall beta-1,3-endoglucanase EGLC functions as a GPI-anchored protein. Its biosynthesis is crucial for maintaining the integrity of fungal cell walls and is recognized as a significant virulence factor in various pathogenic fungi, including Candida albicans, where it plays an adhesive role in the process of host cell infection (Martinez-Lopez et al., 2004; Cormack et al., 1999). It is also an important target for many fungicide. The CFEM domain protein, characterized by its unique cell wall structure in fungi, is associated with glycosylphosphatidylinositol (GPI)-anchored proteins. This protein can bind to virulence factor effectors that contain the CFEM domain, thereby playing a critical role in the formation and enhancement of adhesion and virulence (Choi and Dean, 1997; Wang et al., 2022). As a classical virulence factor of pathogenic fungi, melanin plays a significant role in the immune evasion of these organisms, particularly in their resistance to oxidative attacks from immune cells, such as reactive oxygen species (ROS) (García-Carnero and Martínez-Álvarez, 2022; Wang et al., 1995). Scytalone dehydratase has been implicated in the formation of melanin virulence factors in fungi. After Wang et al. expressed the gene for scytalone dehydratase, which encodes the sickle dehydratase, in melanin-deficient, nonpathogenic Colletotrichum lagenarium (OSD1), it restored both melanin production and pathogenicity in this species (Wang et al., 2001). Furthermore, scytalone dehydratase has been utilized as a target for antifungal drug development aimed at inhibiting melanin synthesis (Eisenman et al., 2009). Laccase precursor is a precursor of laccase (Motoyama et al., 2022), a virulence-related cell wall enzyme associated with the blackening of Cryptococcus neoformans and the acquisition of resistance to polyene and echinocandins (Eisenman et al., 2009). The DNA mismatch repair protein- PMS2 is responsible for recognizing and repairing erroneous insertions, deletions, and base misincorporations that occur during DNA replication and recombination, as well as for addressing certain types of DNA damage (Iyer et al., 2006). Its absence contributes to the evolution of fungal resistance (Legrand et al., 2007; Dos Reis et al., 2019). Molecular Chaperone HTPG, a member of the Hsp90 family, is an important chaperone whose overexpression enhances the virulence of Candida in mice (Hodgetts et al., 1996). It is also considered central to the buffering effect known as pipelization (Burnie et al., 2006). HTPG has been described as suppressing phenotypic variation under normal conditions, yet it releases such variation when its function is impaired (Bergman and Siegal, 2003). Therefore, these genes may serve as new targets for future research.
5 Conclusion
In this study, we employ bioinformatics to identify new therapeutic targets for Sporotrichosis. To further predict these targets, selected small molecule drugs were tested in vitro and in vivo. The results concluded that Azelastine and Mefloquine are effective in treating Sporotrichosis. Furthermore, our study indicates that these small molecule drugs may possess a broad-spectrum antifungal effect, potentially providing new insights for the treatment of other invasive fungal diseases. At the same time, we investigated the virulence factors and growth repair-related genes regulated by abaA through transcriptomics and qRT-PCR. The results indicated that abaA is crucial for the toxicity, growth, and development of Sporothrix. The methodology employed in this research establishes a foundation for the investigation of drug resistance in other fungi, and the regulation of downstream related factors by abaA offers a robust basis for future studies.
Data availability statement
The datasets presented in this study can be found in online repositories. The names of the repository/repositories and accession number(s) can be found at: NCBI PRJNA1163991.
Ethics statement
The animal study was approved by Liaoning University Research Integrity and Academic Ethics Committee. The study was conducted in accordance with the local legislation and institutional requirements.
Author contributions
YW: Data curation, Writing – original draft. XW: Investigation, Writing – review & editing. XF: Validation, Writing – original draft. CH: Investigation, Writing – review & editing. FZ: Methodology, Writing – review & editing. ZZ: Funding acquisition, Writing – review & editing.
Funding
The author(s) declare that financial support was received for the research and/or publication of this article. This study was supported by Shenzhen Science and Technology Innovation Commission Project (grant no. JCYJ20210324114612034) which were distributed to Hong Kong University Shenzhen Hospital.
Conflict of interest
The authors declare that the research was conducted in the absence of any commercial or financial relationships that could be construed as a potential conflict of interest.
Generative AI statement
The authors declare that no Gen AI was used in the creation of this manuscript.
Publisher’s note
All claims expressed in this article are solely those of the authors and do not necessarily represent those of their affiliated organizations, or those of the publisher, the editors and the reviewers. Any product that may be evaluated in this article, or claim that may be made by its manufacturer, is not guaranteed or endorsed by the publisher.
Supplementary material
The Supplementary material for this article can be found online at: https://www.frontiersin.org/articles/10.3389/fmicb.2025.1546020/full#supplementary-material
Footnotes
References
Andrianopoulos, A., and Timberlake, W. E. (1994). The aspergillus Nidulans Abaa gene encodes a transcriptional activator that acts as a genetic switch to control development. Mol. Cell. Biol. 14, 2503–2515. doi: 10.1128/MCB.14.4.2503
Badiee, P., and Hashemizadeh, Z. (2014). Opportunistic invasive fungal infections: diagnosis and clinical management. Indian J. Med. Res. 139, 195–204
Balkrishna, A., Verma, S., Mulay, V. P., Gupta, A. K., Haldar, S., and Varshney, A. (2022). Withania somnifera (L.) Dunal whole-plant extracts exhibited anti-Sporotrichotic effects by destabilizing peripheral integrity of Sporothrix Globosa yeast cells. PLoS Negl. Trop. Dis. 16:e0010484. doi: 10.1371/journal.pntd.0010484
Bergman, A., and Siegal, M. L. (2003). Evolutionary capacitance as a general feature of complex gene networks. Nature 424, 549–552. doi: 10.1038/nature01765
Bing, Z., Cheng, W., Linqi, W., Sachs Matthew, S., and Xiaorong, L. (2012). The antidepressant sertraline provides a promising therapeutic option for neurotropic Cryptococcal infections. Antimicrob. Agents Chemother. 56:212. doi: 10.1128/aac.00212-12
Bonifaz, A., and Vázquez-González, D. (2010). Sporotrichosis: an update. Giornale Italiano Dermatol. Venereol. 145, 659–673. doi: 10.1016/j.fgb.2012.03.005
Borneman, A. R., Hynes, M. J., and Andrianopoulos, A. (2000). The Abaa homologue of Penicillium Marneffei participates in two developmental Programmes: Conidiation and dimorphic growth. Mol. Microbiol. 38, 1034–1047. doi: 10.1046/j.1365-2958.2000.02202.x
Boyce, K. J., Schreider, L., Kirszenblat, L., and Andrianopoulos, A. (2011). The two-component histidine kinases Drka and Slna are required for in vivo growth in the human pathogen Penicillium Marneffei. Mol. Microbiol. 82, 1164–1184. doi: 10.1111/j.1365-2958.2011.07878.x
Brown-Elliott, B. A., Wallace, R. J., Blinkhorn, R., Crist, C. J., and Mann, L. B. (2001). Successful treatment of disseminated Mycobacterium chelonae infection with linezolid. Clin. Infect. Dis. 33, 1433–1434. doi: 10.1086/322523
Burnie, J. P., Carter, T. L., Hodgetts, S. J., and Matthews, R. C. (2006). Fungal heat-shock proteins in human disease. FEMS Microbiol. Rev. 30, 53–88. doi: 10.1111/j.1574-6976.2005.00001.x
Carroll, S. B. (2008). Evo-Devo and an expanding evolutionary synthesis: a genetic theory of morphological evolution. Cell 134, 25–36. doi: 10.1016/j.cell.2008.06.030
Chakrabarti, A., Bonifaz, A., Gutierrez-Galhardo, M. C., Mochizuki, T., and Li, S. (2014). Global epidemiology of Sporotrichosis. Sabouraudia 53, 3–14. doi: 10.1093/mmy/myu062
Chen, S., Zhou, Y., Chen, Y., and Jia, G. (2018). Fastp: an ultra-fast all-in-one Fastq preprocessor. Bioinformatics 34, i884–i890. doi: 10.1093/bioinformatics/bty560
Cheng, Z. X., Guo, C., Chen, Z. G., Yang, T. C., Zhang, J. Y., Wang, J., et al. (2019). Glycine, serine and threonine metabolism confounds efficacy of complement-mediated killing. Nat. Commun. 10:3325. doi: 10.1038/s41467-019-11129-5
Cho, K. I., Kim, D., and Lee, D. (2009). A feature-based approach to modeling protein-protein interaction hot spots. Nucleic Acids Res. 37, 2672–2687. doi: 10.1093/nar/gkp132
Choi, W., and Dean, R. A. (1997). The adenylate cyclase gene Mac1 of Magnaporthe Grisea controls Appressorium formation and other aspects of growth and development. Plant Cell 9, 1973–1983. doi: 10.1105/tpc.9.11.1973
Clutterbuck, A. J. (1969). A mutational analysis of conidial development in aspergillus Nidulans. Genetics 63:317. doi: 10.1093/genetics/63.2.317
Cormack, B. P., Ghori, N., and Falkow, S. (1999). An Adhesin of the yeast pathogen Candida Glabrata mediating adherence to human epithelial cells. Science 285, 578–582. doi: 10.1126/science.285.5427.578
Cortés Juan Carlos, G., Curto, M. Á., Carvalho, V. S. D., Pérez, P., and Ribas, J. C. (2019). The fungal Cell Wall as a target for the development of new antifungal therapies. Biotechnol. Adv. 37:107352. doi: 10.1016/j.biotechadv.2019.02.008
Danecek, P., Bonfield, J. K., Liddle, J., Marshall, J., Ohan, V., Pollard, M. O., et al. (2021). Twelve years of Samtools and Bcftools. Gigascience 10:giab008. doi: 10.1093/gigascience/giab008
Dos Reis, T. F., Silva, L. P., de Castro, P. A., et al. (2019). The Aspergillus fumigatus mismatch repair Msh2 homolog is important for virulence and azole resistance. Msphere 4, E00416–E00419. doi: 10.1128/mSphere.00416-19
Eisenman, H. C., Frases, S., Nicola, A. M., Rodrigues, M. L., and Casadevall, A. (2009). Vesicle-associated Melanization in Cryptococcus neoformans. Microbiology 155, 3860–3867. doi: 10.1099/mic.0.032854-0
Etxebeste, O., Otamendi, A., Garzia, A., Espeso, E. A., and Cortese, M. S. (2019). Rewiring of transcriptional networks as a major event leading to the diversity of asexual multicellularity in Fungi. Crit. Rev. Microbiol. 45, 548–563. doi: 10.1080/1040841X.2019.1630359
Farha, M. A., and Brown, E. D. (2019). Drug repurposing for antimicrobial discovery. Nat. Microbiol. 4, 565–577. doi: 10.1038/s41564-019-0357-1
Félix-Contreras, C., Alba-Fierro, C. A., Ríos-Castro, E., Luna-Martínez, F., Cuéllar-Cruz, M., and Ruiz-Baca, E. (2020). Proteomic analysis of Sporothrix schenckii Cell Wall reveals proteins involved in oxidative stress response induced by Menadione. Microb. Pathog. 141:103987. doi: 10.1016/j.micpath.2020.103987
Feng, P., Xie, Z., Sun, J., Zhang, J., Li, X., Lu, C., et al. (2010). Molecular cloning, characterization and expression of Pmrsr1, a Ras-related gene from yeast form of Penicillium Marneffei. Mol. Biol. Rep. 37, 3533–3540. doi: 10.1007/s11033-009-9947-y
García-Carnero, L. C., and Martínez-Álvarez, J. A. (2022). Virulence factors of Sporothrix schenckii. J. Fungi 8:318. doi: 10.3390/jof8030318
Haozhen, W., Ziyin, L., Yang, L., Zhao, L., Gao, T., Lu, X., et al. (2023). Virtual screening of novel 24-Dehydroxysterol reductase (Dhcr24) inhibitors and the biological evaluation of Irbesartan in cholesterol-lowering effect. Molecules 28:643. doi: 10.3390/molecules28062643
Hodgetts, S., Matthews, R., Morrissey, G., Mitsutake, K., Piper, P., and Burnie, J. (1996). Over-expression of Saccharomyces Cerevisiae Hsp90 enhances the virulence of this yeast in mice. FEMS Immunol. Med. Microbiol. 16, 229–234. doi: 10.1111/j.1574-695X.1996.tb00140.x
Höft, M. A., Duvenage, L., and Claire, J. H. (2022). Key thermally dimorphic fungal pathogens: shaping host immunity. Open Biol. 12:210219. doi: 10.1098/rsob.210219
Hu, L., Deng, B., Wu, R., Zhan, M., Hu, X., and Huang, H. (2024). Optimized expression of peptidyl-prolyl Cis/Transisomerase Cyclophilinb with prokaryotic toxicity from Sporothrix Globosa. J. Ind. Microbiol. Biotechnol. 51:17. doi: 10.1093/jimb/kuae017
Huang, Y. X., Xu, Y. L., Yu, C. X., Li, H. J., Yin, X. R., Wang, T. S., et al. (2011). Effect of Praziquantel prolonged administration on granuloma formation around Schistosoma Japonicum eggs in lung of sensitized mice. Parasitol. Res. 109, 1453–1459. doi: 10.1007/s00436-011-2485-2
Iyer, R. R., Pluciennik, A., Burdett, V., and Modrich, P. L. (2006). DNA mismatch repair: functions and mechanisms. Chem. Rev. 106, 302–323. doi: 10.1002/chin.200620268
Jadamba, E., and Shin, M. (2016). A systematic framework for drug repositioning from integrated omics and drug phenotype profiles using pathway-drug network. Biomed. Res. Int. 2016:7147039. doi: 10.1155/2016/7147039
Joao, I., Bujdáková, H., and Jordao, L. (2020). Opportunist coinfections by nontuberculous mycobacteria and Fungi in immunocompromised patients. Antibiotics 9:771. doi: 10.3390/antibiotics9110771
Kalvari, I., Nawrocki, E. P., Ontiveros-Palacios, N., Argasinska, J., Lamkiewicz, K., Marz, M., et al. (2021). Rfam 14: expanded coverage of metagenomic, viral and Microrna families. Nucleic Acids Res. 49, D192–D200. doi: 10.1093/nar/gkaa1047
Karbwang, J., and Harinasuta, T. (1992). Overview: clinical pharmacology of Antimalarials. Southeast Asian J. Trop. Med. Public Health 23, 95–109
Konrat, R., Papp, H., Kimpel, J., Rössler, A., Szijártó, V., Nagy, G., et al. (2022). The anti-histamine Azelastine, identified by computational drug repurposing, inhibits infection by major variants of Sars-Cov-2 in cell cultures and reconstituted human nasal tissue. Front. Pharmacol. 13:861295. doi: 10.3389/fphar.2022.861295
Krysan, D. J. (2016). Challenges in the development of novel Anticryptococcal agents. Future Med. Chem. 8, 1375–1377. doi: 10.4155/fmc-2016-0123
Langmead, B., Wilks, C., Antonescu, V., and Charles, R. (2019). Scaling read aligners to hundreds of threads on general-purpose processors. Bioinformatics 35, 421–432. doi: 10.1093/bioinformatics/bty648
Legrand, M., Chan, C. L., Jauert, P. A., and Kirkpatrick, D. T. (2007). Role of DNA mismatch repair and double-Strand break repair in genome stability and antifungal drug resistance in Candida albicans. Eukaryot. Cell 6, 2194–2205. doi: 10.1128/EC.00299-07
Levine, M. (2010). Transcriptional enhancers in animal development and evolution. Curr. Biol. 20, R754–R763. doi: 10.1016/j.cub.2010.06.070
Liu, Z., Li, S. S., Zhang, G. Y., Lv, S., Wang, S., and Li, F. Q. (2024). Whole transcriptome sequencing for revealing the pathogenesis of Sporotrichosis caused by Sporothrix Globosa. Sci. Rep. 14:359. doi: 10.1038/s41598-023-50728-7
Lopes-Bezerra, L. M., Mora-Montes, H. M., and Zhang, Y. (2018). Sporotrichosis between 1898 and 2017: the evolution of knowledge on a changeable disease and on emerging etiological agents. Med. Mycol. 56, 126–143. doi: 10.1093/mmy/myx103
Love, M. I., Huber, W., and Anders, S. (2014). Moderated estimation of fold change and dispersion for Rna-Seq data with Deseq2. Genome Biol. 15, 1–21. doi: 10.1186/s13059-014-0550-8
Lv, S., Xin, H., Liu, Z., Lin, Y., Hanfei, W., and Li, F. (2022). Clinical epidemiology of Sporotrichosis in Jilin Province, China (1990–2019): a series of 4969 cases. Infect. Drug Resist. 15, 1753–1765. doi: 10.2147/IDR.S354380
Lythgoe, M. P., and Middleton, P. (2020). Ongoing clinical trials for the Management of the Covid-19 pandemic. Trends Pharmacol. Sci. 41, 363–382. doi: 10.1016/j.tips.2020.03.006
Ma, X., Zhao, Y., Yang, T., Gong, N., Chen, X., Liu, G., et al. (2022). Integration of network pharmacology and molecular docking to explore the molecular mechanism of Cordycepin in the treatment of Alzheimer's disease. Front. Aging Neurosci. 14:1058780. doi: 10.3389/fnagi.2022.1058780
Martinez-Lopez, R., Monteoliva, L., Diez-Orejas, R., Nombela, C., and Gil, C. (2004). The Gpi-anchored protein Caecm33p is required for Cell Wall integrity, morphogenesis and virulence in Candida albicans. Microbiology 150, 3341–3354. doi: 10.1099/mic.0.27320-0
McTavish, D., and Sorkin, E. M. (1989). Azelastine. A review of its Pharmacodynamic and pharmacokinetic properties, and therapeutic potential. Drugs 38, 778–800. doi: 10.2165/00003495-198938050-00005
Montoya, M. C., Beattie, S., Alden, K. M., and Krysan, D. J. (2020). Derivatives of the antimalarial drug Mefloquine are broad-Spectrum antifungal molecules with activity against drug-resistant clinical isolates. Antimicrob. Agents Chemother. 64, e02331–e02319. doi: 10.1128/AAC.02331-19
Moreira, M. H., Almeida, F. C. L., Domitrovic, T., and Palhano, F. L. (2021). A systematic structural comparison of all solved small proteins deposited in Pdb. The effect of disulfide bonds in protein fold. Comput. Struct. Biotechnol. J. 19, 6255–6262. doi: 10.1016/j.csbj.2021.11.015
Moreira, J. A. S., Freitas, D. F. S., and Lamas, C. C. (2015). The impact of Sporotrichosis in Hiv-infected patients: a systematic review. Infection 43, 267–276. doi: 10.1007/s15010-015-0746-1
Motoyama, T., Kondoh, Y., Shimizu, T., Hayashi, T., Honda, K., Uchida, M., et al. (2022). Identification of Scytalone dehydratase inhibitors effective against melanin biosynthesis dehydratase inhibitor-resistant Pyricularia oryzae. J. Agric. Food Chem. 70, 3109–3116. doi: 10.1021/acs.jafc.1c04984
Nava-Pérez, N., Neri-García, L. G., Romero-González, O. E., Terrones-Cruz, J. A., García-Carnero, L. C., and Mora-Montes, H. M. (2022). Biological and clinical attributes of Sporothrix Globosa, a causative agent of Sporotrichosis. Infect. Drug Resist. 15:2067. doi: 10.2147/IDR.S362099
Ortiz-Ramírez, J. A., Cuéllar-Cruz, M., and López-Romero, E. (2022). Sporothrix Schenckiicell compensatory responses of Fungi to damage of the Cell Wall induced by Calcofluor white and Congo red with emphasis on and a review. Front. Cell. Infect. Microbiol. 12:976924. doi: 10.3389/fcimb.2022.976924
Park, K. (2019). A review of computational drug repurposing. Transl. Clinic. Pharmacol. 27, 59–63. doi: 10.12793/tcp.2019.27.2.59
Perlroth, J., Choi, B., and Spellberg, B. (2007). Nosocomial fungal infections: epidemiology, diagnosis, and treatment. Med. Mycol. 45, 321–346. doi: 10.1080/13693780701218689
Pertea, M., Pertea, G. M., Antonescu, C. M., Chang, T.-C., Mendell, J. T., and Salzberg, S. L. (2015). Stringtie enables improved reconstruction of a transcriptome from Rna-Seq reads. Nat. Biotechnol. 33, 290–295. doi: 10.1038/nbt.3122
Pruitt, K. D., Tatusova, T., and Maglott, D. R. (2007). Ncbi reference sequences (Refseq): a curated non-redundant sequence database of genomes, transcripts and proteins. Nucleic Acids Res. 35, D61–D65. doi: 10.1093/nar/gkl842
Saraiva, F. D. F., Hoagland, B. D. S., Do Valle, A. C. F., Fraga, B. B., de Barros, M. B., de Oliveira Schubach, A., et al. (2012). Sporotrichosis in Hiv-infected patients: report of 21 cases of endemic Sporotrichosis in Rio De Janeiro, Brazil. Sabouraudia 50, 170–178. doi: 10.3109/13693786.2011.596288
Smith, S. J., Rebeiz, M., and Davidson, L. (2018). From pattern to process: studies at the Interface of gene regulatory networks, morphogenesis, and evolution. Curr. Opin. Genet. Dev. 51, 103–110. doi: 10.1016/j.gde.2018.08.004
Soneson, C., Love, M. I., and Robinson, M. D. (2015). Differential analyses for Rna-Seq: transcript-level estimates improve gene-level inferences. F1000Research 4:1521. doi: 10.12688/f1000research.7563.2
Swamidass, S. J. (2011). Mining small-molecule screens to repurpose drugs. Brief. Bioinform. 12, 327–335. doi: 10.1093/bib/bbr028
Teixeira, M. M., de Almeida, L. G. P., Kubitschek-Barreira, P., Alves, F. L., Kioshima, E. S., Abadio, A. K., et al. (2014). Comparative genomics of the major fungal agents of human and animal Sporotrichosis: Sporothrix Schenckii and Sporothrix Brasiliensis. BMC Genomics 15:943. doi: 10.1186/1471-2164-15-943
Tummino, T. A., Rezelj, V. V., Fischer, B., Fischer, A., O'Meara, M. J., Monel, B., et al. (2021). Drug-induced Phospholipidosis confounds drug repurposing for Sars-Cov-2. Science 373, 541–547. doi: 10.1126/science.abi4708
Van Cutsem, J., Kurata, H., Matsuoka, H., Mikami, Y., Pfaller, M. A., Scalarone, G. M., et al. (1994). Antifungal drug susceptibility testing. J. Med. Vet. Mycol. 32, 267–276. doi: 10.1080/02681219480000891
Wang, Y., Aisen, P., and Casadevall, A. (1995). Cryptococcus Neoformans melanin and virulence: mechanism of action. Infect. Immun. 63, 3131–3136. doi: 10.1016/1380-2933(95)00016-X
Wang, H.-L., Kim, S., and Breuil, C. (2001). A Scytalone dehydratase gene from Ophiostoma Floccosum restores the Melanization and pathogenicity phenotypes of a melanin-deficient Colletotrichum Lagenarium mutant. Mol. Gen. Genomics. 266, 126–132. doi: 10.1007/s004380100534
Wang, D., Zhang, D.-D., Song, J., Li, J. J., Wang, J., Li, R., et al. (2022). Verticillium Dahliae Cfem proteins manipulate host immunity and differentially contribute to virulence. BMC Biol. 20:55. doi: 10.1186/s12915-022-01254-x
Watts, A. M., Cripps, A. W., West, N. P., and Cox, A. J. (2019). Modulation of allergic inflammation in the nasal mucosa of allergic rhinitis sufferers with topical pharmaceutical agents. Front. Pharmacol. 10:294. doi: 10.3389/fphar.2019.00294
Yu, J.-H. (2010). Regulation of development in aspergillus Nidulans and Aspergillus fumigatus. Mycobiology 38, 229–237. doi: 10.4489/MYCO.2010.38.4.229
Zhang, Z., Li, L., and Wang, H. (2024). Sporothrix globosadisasters pile up on the rubbing heel: as secondary infection to infection. Emerg. Microb. Infect. 13:2358073. doi: 10.1080/22221751.2024.2358073
Zheng, F., Gao, W., and Wang, Y. (2021). Sporothrix schenckii map of dimorphic switching-related signaling pathways in based on its transcriptome. Mol. Med. Rep. 24:12285. doi: 10.3892/mmr.2021.12285
Keywords: Sporothrix globosa , abaA gene, virtual screening, small molecule drugs, transcriptomics
Citation: Wang Y, Wu X, Fan X, Han C, Zheng F and Zhang Z (2025) Screening and transcriptomic analysis of anti-Sporothrix globosa targeting AbaA. Front. Microbiol. 16:1546020. doi: 10.3389/fmicb.2025.1546020
Edited by:
Israel Nissan, Ministry of Agriculture and Rural Development, IsraelReviewed by:
Patricia Ponce-Noyola, University of Guanajuato, MexicoTong-Bao Liu, Southwest University, China
Copyright © 2025 Wang, Wu, Fan, Han, Zheng and Zhang. This is an open-access article distributed under the terms of the Creative Commons Attribution License (CC BY). The use, distribution or reproduction in other forums is permitted, provided the original author(s) and the copyright owner(s) are credited and that the original publication in this journal is cited, in accordance with accepted academic practice. No use, distribution or reproduction is permitted which does not comply with these terms.
*Correspondence: Zhenying Zhang, emhhbmd6eUBoa3Utc3poLm9yZw==; Fangliang Zheng, Zmx6aGVuZzE5ODBAMTYzLmNvbQ==
†These authors have contributed equally to this work and share first authorship
Yahveth Cantero-Fortiz, orcid.org/0000-0002-7836-4723
Mercè Boada, orcid.org/0000-0003-2617-3009