- 1Department of Pharmacy, Henan Provincial People's Hospital, Zhengzhou, China
- 2XNA Platform, School of Pharmaceutical Sciences, Zhengzhou University, Zhengzhou, China
- 3Key Laboratory of Advanced Drug Preparation Technologies, Ministry of Education, Zhengzhou University, Zhengzhou, China
Carbapenem-resistant Pseudomonas aeruginosa (CRPA) poses a global threat; however, the epidemiological characteristics and clinical significance of blaAFM-positive CRPA strains in China remain unclear. In this study, continuous surveillance was conducted from 2018 to 2022 in a hospital in Henan Province, China, and the genomic characteristics of blaAFM-positive CRPA were elucidated. We characterised the genetic features of blaAFM-positive CRPA isolates by antimicrobial susceptibility testing, conjugation assays, whole-genome sequencing, large-scale comparative genomics, and bioinformatic analyses. Among 628 CRPA isolates, one blaAFM-positive multidrug-resistant (MDR) strain, PA19-3158 (ST1123), was identified, with the blaAFM-1 gene located on a novel 518,222 bp megaplasmid. Additionally, big data analysis revealed the genomic characteristics of blaAFM-positive CRPA across China. A total of three different blaAFM gene variants were identified among these isolates, namely blaAFM-1 (44.12%), blaAFM-2 (52.94%), and blaAFM-4 (2.94%). Our findings identified ST463 as the dominant clone among blaAFM-positive CRPA in different regions of China, with some blaAFM-positive CRPA isolates from these regions exhibiting high genetic similarity. Notably, all blaAFM-positive CRPA isolates carried multiple antibiotic resistance genes (ARGs), with approximately 38% co-harboring the carbapenem-resistant gene blaKPC-2 and approximately 47% co-harboring the tigecycline-resistant gene tmexCD-toprJ. Correlation analysis underscored the significant role of mobile genetic elements in facilitating blaAFM gene transfer. These results highlight the critical need for continuous surveillance of blaAFM-positive CRPA in clinical settings to mitigate potential risks.
Introduction
Carbapenem-resistant Pseudomonas aeruginosa (CRPA) exhibits extensive drug resistance, poses significant challenges in clinical treatment, and is associated with high mortality rates in infected patients (Zhu et al., 2023; Saravanan et al., 2023). As such, CRPA has been classified as a “critical priority” pathogen for antibiotic development by the World Health Organization (WHO). The resistance of P. aeruginosa to carbapenem antibiotics is primarily mediated by several mechanisms: overexpression of the MexAB-OprM efflux pump, hyperproduction of AmpC β-lactamase, inactivation of OprD porins, and production of carbapenemases (Gray et al., 2023; Livermore, 2002). Although carbapenemase-producing P. aeruginosa constitutes a smaller proportion of CRPA isolates, this proportion has been steadily increasing in recent years (Reyes et al., 2023). Among these carbapenemases, metallo-β-lactamases (MBLs) are the most prevalent in CRPA (Yoon and Jeong, 2021). The treatment of infections caused by MBL-producing CRPA is particularly challenging, as MBLs confer resistance to most β-lactam antibiotics (Jean et al., 2019).
Historically, NDM was considered the sole member of the subclass B1b MBL. However, in 2018, a novel subclass B1b MBL, Alcaligenes faecalis metallo-β-lactamase-1 (AFM-1), was first identified in Acinetobacter faecalis isolates in China (Li et al., 2022). Since then, AFM-1 has been detected in various bacterial species, including P. aeruginosa, P. putida, and Bordetella trematum (Li et al., 2022; Zhang et al., 2021). Furthermore, as AFM-1 continues to evolve, multiple variants such as AFM-2, AFM-3, and AFM-4 have been identified in P. aeruginosa (Chen et al., 2022). However, despite its emergence, reports on bacteria producing this newly discovered MBL remain scarce, and comprehensive studies on AFM-producing pathogens are still lacking.
To further enhance our understanding of blaAFM-positive CRPA isolates, this study conducted a five-year surveillance of blaAFM-positive CRPA isolates from hospitals in Henan Province, China, systematically analyzing their characteristics. Additionally, through large-scale data analysis, the genomic features of blaAFM-positive CRPA isolates in China were further elucidated.
Methods
Characterization of blaAFM-positive CRPA isolates in clinical settings
To investigate the presence of blaAFM-positive CRPA among hospitalized patients, continuous surveillance was conducted from 2018 to 2022 in a hospital in Henan Province, China, with over 10,000 beds. The presence of the blaAFM gene was confirmed via PCR, and blaAFM-positive isolates were subjected to further analysis. This study was approved by the Ethics Committee of Zhengzhou University.
Antimicrobial susceptibility testing of blaAFM-positive CRPA isolates
Minimal inhibitory concentrations (MICs) were determined using the broth microdilution technique, and E. coli ATCC 25922 was used as the quality control. The panel of antibiotics tested included meropenem, gentamicin, ciprofloxacin, amikacin, aztreonam, colistin, ceftazidime, piperacillin/tazobactam, fosfomycin, ceftazidime/avibactam, and aztreonam/avibactam. Resistance breakpoints were interpreted according to EUCAST v.14.0 criteria for tigecycline and CLSI guidelines for the remaining antibiotics (Marchaim et al., 2014; CLSI, 2018).
Conjugation experiments
To assess the transferability of the blaAFM-positive plasmid, a conjugation assay was performed using rifampicin-resistant Escherichia coli EC600 and hygromycin-resistant Klebsiella pneumoniae YZ6 as recipients. Donor and recipient cultures were mixed at a 1:4 ratio and incubated on LB agar plates at 37°C overnight. Transconjugants were selected on LB agar plates supplemented with meropenem (2 mg/L) and rifampin (300 mg/L) or meropenem (2 mg/L) and hygromycin (200 mg/L). PCR was subsequently employed to confirm the presence of blaAFM in the transconjugants.
Whole-genome sequencing and genome assembly
To characterize the genetic features of the identified blaAFM-positive CRPA isolates, genomic DNA was extracted using the FastPure bacterial DNA isolation mini kit and quantified with a Qubit 4 Fluorometer. Whole-genome sequencing (WGS) was performed on the Illumina HiSeq 2500 platform with 2 × 150 bp paired-end libraries. De novo genome assembly was carried out using SPAdes v.3.14.0 (Bankevich et al., 2012). Additionally, blaAFM-positive plasmids were extracted using the Qiagen Plasmid Midi Kit and subjected to Nanopore sequencing. The plasmid sequences were assembled using Unicycler v.0.4.8 (Wick et al., 2017). Genome annotation was performed with Prokka v.1.11 (Seemann, 2014).
Screening of blaAFM-positive CRPA isolates in retrospective data from China
All P. aeruginosa genome sequences available in the National Center for Biotechnology Information (NCBI) genome database as of 2 August 2024 were retrieved (Li et al., 2024). Subsequently, blaAFM-positive P. aeruginosa isolates originating from China were selected for further analysis. Basic information regarding the blaAFM-positive P. aeruginosa isolates, including the region, year of isolation, and accession number, was organized into an Excel spreadsheet for downstream analysis.
Bioinformatics analysis
ARGs and plasmid replicons were identified using online tools with default parameters (threshold value: 80% identity and coverage) (Bortolaia et al., 2020; Carattoli and Hasman, 2020). Virulence genes were annotated using the Virulence Factor Database (VFDB) (Kleinheinz et al., 2014). Multi-locus sequence typing (MLST) of the isolates was determined using the mlst tool. SNPs in the genomes of blaAFM-positive CRPAs were detected using snp-dists v.0.7.0.1 Phylogenetic trees were constructed using Roary and FastTree based on core single-nucleotide polymorphism (SNP) alignments and further refined with iTOL (Price et al., 2009; Letunic and Bork, 2021). Plasmid comparison maps were generated using BRIG v.0.95 and Easyfig v.2.2.3 (Alikhan et al., 2011; Sullivan et al., 2011).
Statistical analysis
Statistical analyses and visualizations were conducted using R v4.2.2 (R Foundation for Statistical Computing, Vienna, Austria). Spearman correlation analysis was employed to assess relationships among ARGs, insertion sequences, virulence factors, and plasmid replicons. Clinical data were organized using Microsoft Excel 2013.
Data availability
Genome sequence data reported in this study have been deposited in the National Center for Biotechnology Information under the accession number NZ_CP140112.1 (pAFM-PA19-3158). All study data are included in the article and/or supporting information.
Results
Overview of CRPA isolates
In this study, a single blaAFM-1-positive CRPA strain, designated PA19-3158, was isolated from a hospital in Henan Province, China. This strain was isolated from the blood sample of a 29-year-old male patient in the ICU. MLST analysis revealed that PA19-3158 belongs to ST1123. MIC testing demonstrated resistance to carbapenems as well as aztreonam, ceftazidime, piperacillin/tazobactam, ceftazidime/avibactam, and aztreonam/avibactam, while retaining susceptibility to ciprofloxacin and colistin (Supplementary Table S1). Additionally, conjugation experiments indicated that the blaAFM-1 gene in PA19-3158 was non-transferable to E. coli EC600 and K. pneumoniae YZ6.
Characterization of blaAFM-positive plasmids
To investigate the characteristics of blaAFM-positive plasmids, the plasmids carrying blaAFM-1 from PA19-3158 were extracted and subjected to long-read sequencing using the Nanopore platform. Through the combined analysis of short-read and long-read sequencing data, we identified that the blaAFM-1 gene is located on a 518,222 bp megaplasmid, with an average GC content of 56.5% and 647 predicted open reading frames (Figure 1). Interestingly, plasmid typing revealed that this plasmid does not belong to any known replicon type. The plasmid harbors a wide array of resistance genes, including β-lactam resistance genes (blaAFM-1, blaOXA-246, and blaCARB-4), sulfonamide resistance gene (sul1), quinolone resistance gene (qnrVC1), aminoglycoside resistance genes (aac(6′)-II and ant(2″)), trimethoprim resistance gene (dfrA27), chloramphenicol resistance genes (catB11 and cmlA8), rifampin resistance gene (arr-3), macrolide resistance genes (msr(E), mph(E), and mph(F)), and bleomycin resistance gene (bleMBL).
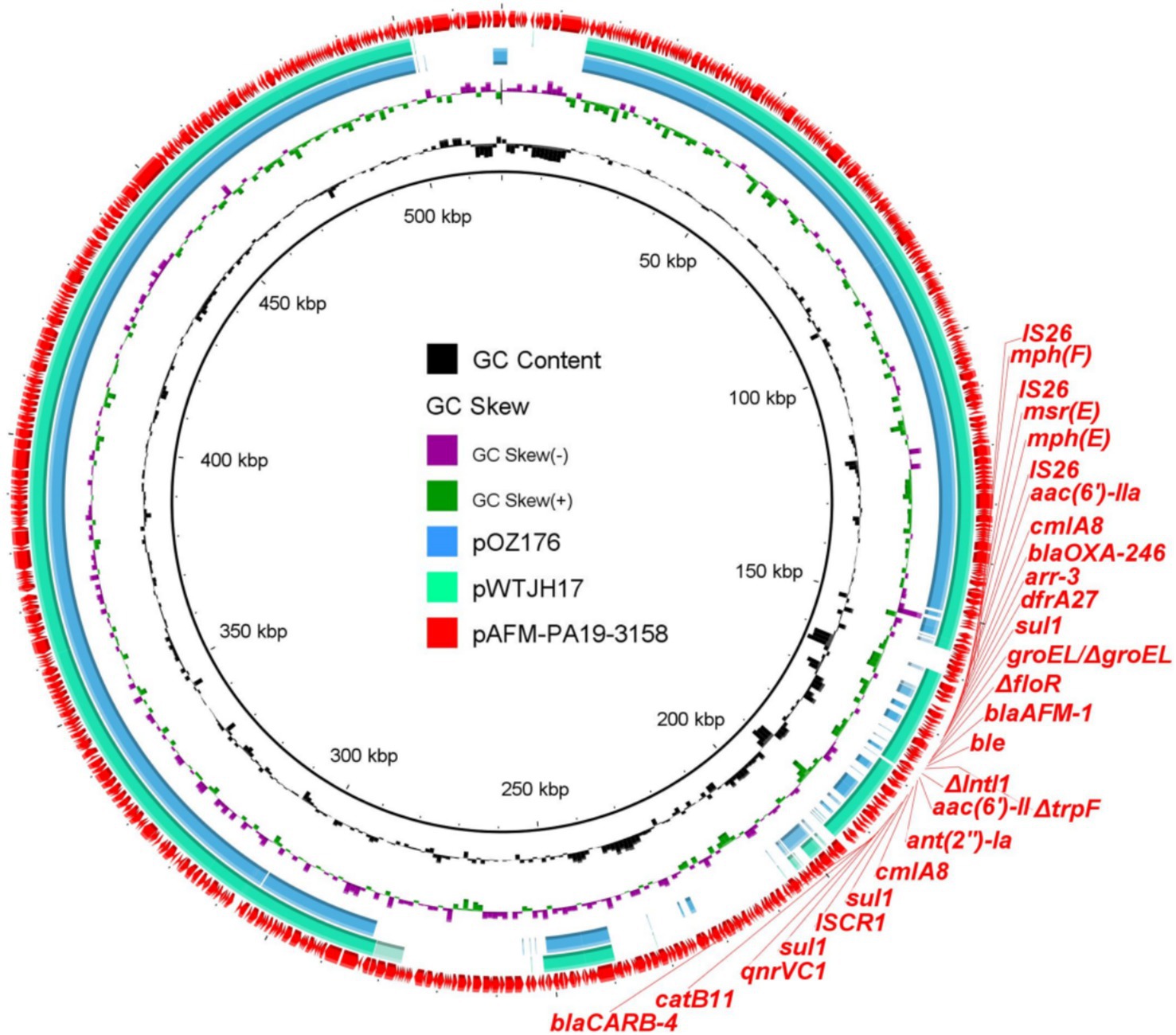
Figure 1. Circular comparison between blaAFM-bearing plasmids found in this study and the NCBI database. The blaAFM-bearing plasmid pAFM-PA19-3158 was used as the reference in the outermost ring.
BLAST analysis revealed that no plasmids identical to the blaAFM-1-positive plasmid (pAFM-PA19-3158) were found in the NCBI database. However, the plasmids pOZ176 (74% coverage, 99.95% identity) and pWTJH17 (79% coverage, 99.99% identity) exhibited the highest similarity to pAFM-PA19-3158. Both of these plasmids were derived from human-associated P. aeruginosa. Compared with the other two plasmids, plasmid pAFM-PA19-3158 carries additional resistance genes (ant(2″)-Ia and mph(F)), suggesting its capacity to continuously acquire resistance determinants and evolve. This poses a further challenge to clinical treatment options by limiting the effectiveness of available antibiotics.
Phylogenetic evolution of blaAFM-positive genomics in the NCBI database from China
To further investigate the genomic epidemiological distribution of blaAFM-positive P. aeruginosa isolates in China, we retrieved all P. aeruginosa genome data from the NCBI database and selected blaAFM-positive P. aeruginosa isolates of Chinese origin for further analysis in combination with data from this study (Supplementary Table S2). A total of 33 eligible isolates were identified from the database. Except for two isolates with unknown sources, the remaining isolates were all derived from human samples. These isolates were distributed across five different regions of China, with the earliest detected in 2017. The blaAFM-positive P. aeruginosa isolates have been consistently reported between 2017 and 2023, indicating their persistent presence in clinical settings over time.
To investigate the evolutionary relationships of blaAFM-positive CRPA in China, we constructed a phylogenetic tree combining blaAFM-positive CRPA isolates from this study with those retrieved from the NCBI database (Figure 2). We observed a high genetic similarity (SNP number < 100) among blaAFM-1-positive strains from different regions, indicating a potential risk of clonal transmission (Supplementary Table S3). Additionally, the blaAFM genes in these isolates were predominantly blaAFM-1 (n = 15) and blaAFM-2 (n = 18), with only one strain carrying blaAFM-4. MLST analysis classified these isolates into eight known STs, with ST463 being the most prevalent (n = 14), followed by ST275 (n = 8). Notably, blaAFM-positive ST463 strains were detected in all years except 2017 and 2023, suggesting its sustained presence in clinical settings over time.
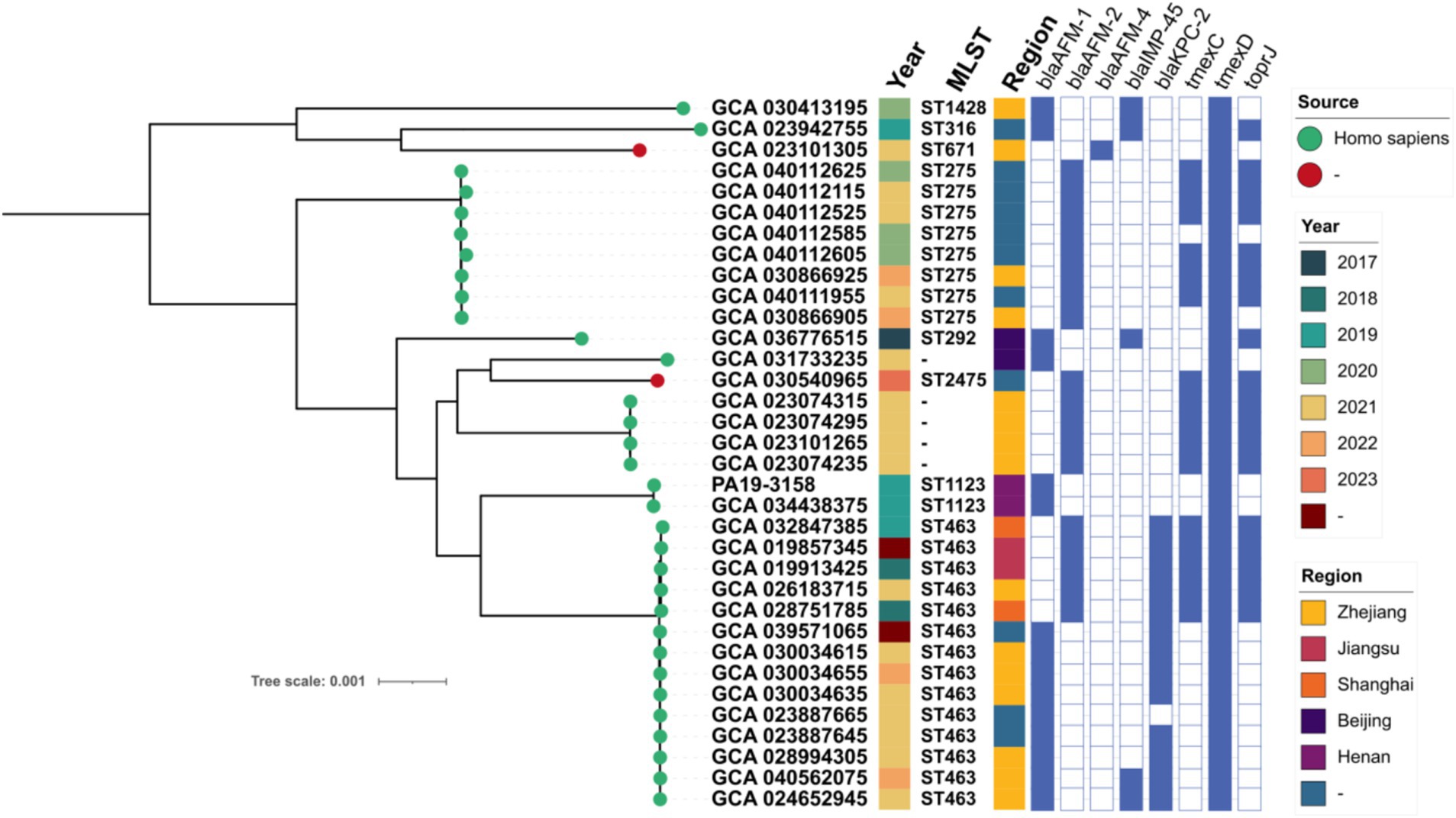
Figure 2. Phylogenetic tree of 34 blaAFM-positive CRPA strains from this study and the NCBI database. Resistance genes are indicated by squares: solid square indicates positive; hollow square indicates negative.
Distribution of ARGs in blaAFM-positive strains
Resistance gene analysis revealed that blaAFM-positive P. aeruginosa isolates harbored a wide range of ARGs. Across different years, 10 shared ARGs were identified among the isolates, with those from 2021 carrying the highest number of unique ARGs (n = 9), followed by 2019 (n = 5). In addition to the blaAFM gene, other carbapenem resistance genes such as blaIMP-45 (n = 5) and blaKPC-2 (n = 13) were also detected in several isolates. Notably, ARGs including aph(3′)-IIb (34/34), fosA (34/34), sul1 (34/34), tmexD (34/34), bleMBL (34/34), catB7 (34/34), and blaPDC-374 (32/34) were identified in the majority of isolates. In contrast, genes such as blaTEM-1, blaOXA-494, and blaPDC-172 were detected in only a single isolate. This highlights both the conserved and variable resistance profiles of blaAFM-positive P. aeruginosa across different years.
Correlation analysis of ARGs, virulence factors, insertion sequences and plasmid replicons
In total, 68 ARGs, 3 plasmid replicons, 67 insertion sequences, and 333 virulence factors were identified in the blaAFM-positive P. aeruginosa isolates. To investigate the relationship between ARGs, insertion sequences, plasmid replicons, and virulence factors in these isolates, a correlation analysis was performed (Figures 3A–C). The linear association analysis indicated that the number of ARGs in blaAFM-positive isolates showed a positive correlation with insertion sequences (rSpearman = 0.31, p < 0.001). However, no significant correlations were observed with plasmid replicons or virulence factors (|rSpearman| < 0.3).
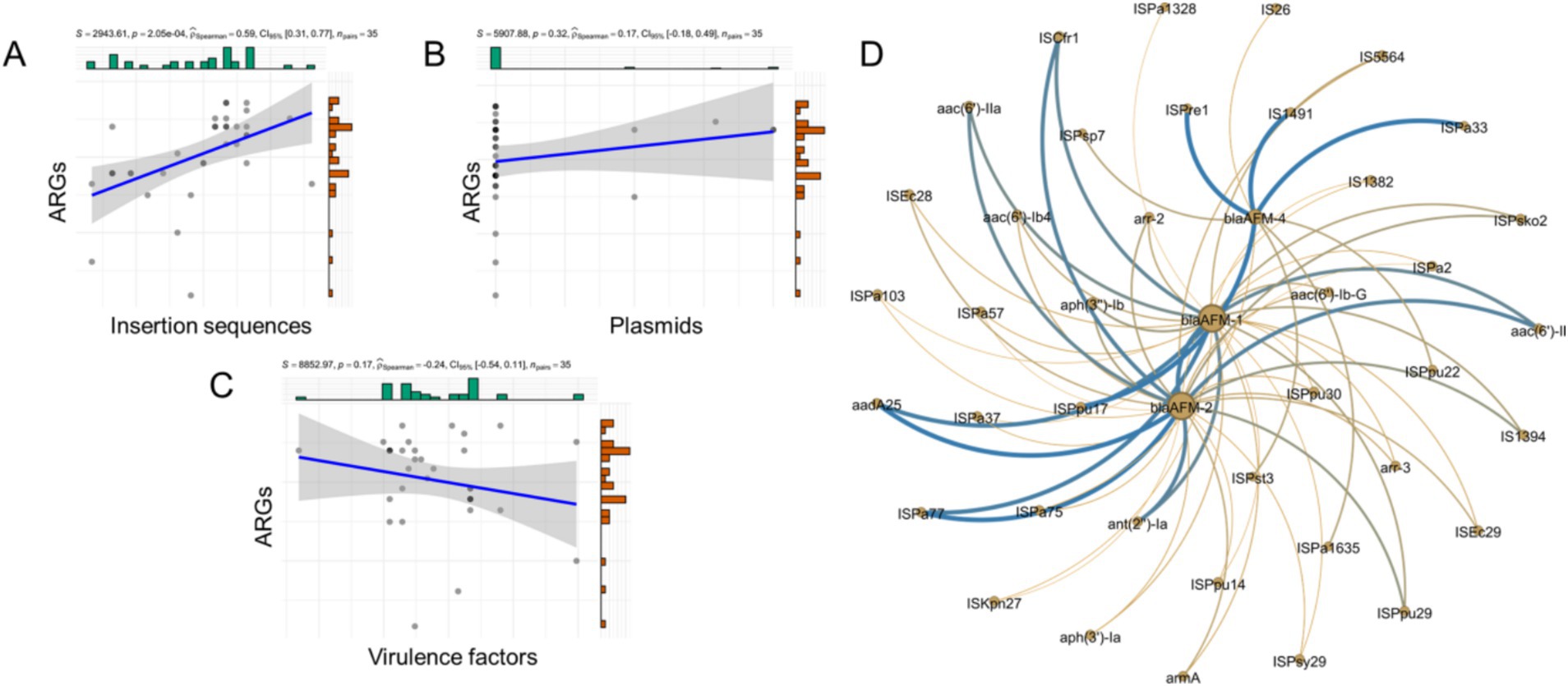
Figure 3. Correlation between ARGs, plasmid replicons, insertion sequences and Virulence genes. (A–C) The correlation of counts of ARGs, plasmid replicons, insertion sequences and VFs detected from genomes of CRPA. (D) The correlation of blaAFM with other ARGs and insertion sequences. The nodes represent ARGs or insertion sequences. Connections between nodes indicate that they are related. Bluer colors and thicker lines represent stronger positive correlations. The yellower the color and the thicker the lines represent the stronger the negative correlation. All associated genes in the figure had p values less than 0.05.
To further examine the relationship between ARGs and insertion sequences, a network graph was constructed (Figure 3D). The results revealed that blaAFM-2 exhibited strong positive correlations with ISCfr1, ant(2″)-Ia, and aac(6′)-IIa (R > 0.8, p < 0.05). Similarly, blaAFM-1 was positively correlated with aph(3″)-Ib, IS5564, and aac(6′)-Ib-G (R > 0.5, p < 0.05). In contrast, no ARGs showed significant correlations with blaAFM-4, although it displayed positive correlations with insertion sequences ISPa1635, ISPpu22, and ISPsp7 (R > 0.5, p < 0.05).
Discussion
In 2021, Lin et al. identified AFM-1 (Alcaligenes faecalis metallo-β-lactamase-1), a novel metallo-β-lactamase (MBL), as a new variant of NDM-1 through molecular and functional characterization (Zhang et al., 2021). Phylogenetic analysis demonstrated that AFM-1 shares 86% amino acid sequence homology with NDM-1. However, due to the low detection rate of the blaAFM gene in P. aeruginosa, systematic studies on blaAFM-positive strains remain scarce (Chen et al., 2022; Zhang et al., 2023). To address this knowledge gap, this study conducted long-term surveillance of blaAFM-positive strains in hospitals in Henan Province, China. Using genomic approaches, we characterized the features of a blaAFM-positive strain isolated from Henan. Besides, we further analyzed the genomic characteristics of all blaAFM-positive CRPA isolates reported in China.
Previous studies have indicated that blaKPC-producing CRPA accounts for approximately 40% of clinical CRPA infections in certain regions, with ST463 identified as the predominant blaKPC-positive clone (Hu et al., 2021). Importantly, ST463 has emerged as a major CRPA lineage responsible for bloodstream infections and is associated with significantly higher mortality rates compared to non-ST463 CRPA infections (Zhang et al., 2023). Our findings revealed that ST463 is the dominant clone among blaAFM-positive CRPA in China, with approximately 38% of these isolates co-harboring the blaKPC-2 gene. The coexistence of both enzymes may confer resistance to nearly all β-lactam antibiotics severely limiting treatment options, increasing healthcare costs, and elevating patient mortality rates. The potential spread of these multidrug-resistant and highly pathogenic strains poses a significant risk of clinical treatment failure, underscoring the urgent need for continuous surveillance of ST463 blaAFM-positive CRPA strains.
The blaAFM gene has been detected in various bacterial genera, but it is relatively rare in P. aeruginosa (Li et al., 2022; Fang et al., 2023). However, due to its ability to spread via plasmids and other mobile genetic elements, it warrants close attention (Chen et al., 2022; Zhou et al., 2023). This study identified a novel, large plasmid carrying the blaAFM gene, which harbors a greater number of resistance genes compared to other similar plasmids. This suggests that plasmids carrying the blaAFM gene may continuously acquire additional resistance genes from external sources, driving their evolution. However, the plasmid is relatively large and lacks an oriT site, which may explain its inability to transfer. Moreover, we observed that these blaAFM-positive CRPA strains not only harbor the blaAFM gene but also carry multiple important resistance genes. Notably, over time, the variety of resistance genes carried by blaAFM-positive CRPA strains has steadily increased.
In studies of human and animal microbiomes, a considerable proportion of plasmids have been found to be untypeable using existing replicon-based classification systems. For instance, a study involving donors from China and the United States identified 5,372 plasmid-like clusters (PLCs), of which only 18.9% (155 clusters) could be assigned to one of the 37 known replicon types; the remaining 81.1% were untypeable (Yang et al., 2023). These untypeable plasmids may possess novel replication mechanisms or exhibit highly divergent replication origin sequences. Importantly, they often carry multiple antimicrobial resistance genes and evade detection by current replicon-based surveillance frameworks (Lassalle et al., 2023). As a result, conventional plasmid epidemiology may fail to capture their distribution and contribution to resistance dissemination. The prevalence of untypeable plasmids highlights the remarkable genomic plasticity of bacteria and underscores their relevance in tracking resistance spread, improving monitoring strategies, and designing novel genetic vectors.
Despite the geographic diversity of the blaAFM-positive CRPA isolates, evolutionary analysis revealed only minimal SNP diffe rences between isolates from different regions, indicating a potential risk for the transmission of resistance genes due to population movement. Notably, we observed that the blaAFM gene often coexists with the blaKPC and tmexCD-toprJ genes. Previous studies have shown that the tmexCD-toprJ efflux pump system can mediate resistance to tigecycline. As tigecycline is considered a last-resort antibiotic for treating infections caused by carbapenem-resistant bacteria, the emergence of tmexCD-toprJ-positive carbapenem-resistant strains compromises the efficacy of tigecycline, severely limiting therapeutic options and increasing the risk of clinical treatment failure (Zhang et al., 2022; He et al., 2019).
Insertion sequences and plasmids are crucial in mediating the spread of resistance genes (Li et al., 2024). To further investigate their roles in blaAFM-positive CRPA isolates, we conducted a correlation analysis. We found that, unlike other carbapenem-resistant bacteria, plasmids play a limited role in the acquisition of foreign resistance genes in blaAFM-positive CRPA (Li et al., 2024). The acquisition and spread of resistance genes appear to be primarily associated with insertion sequences, such as ISCfr1, ISPre1, and IS1491 (Li et al., 2025). Additionally, we observed significant positive correlations between the blaAFM gene and multiple other resistance genes, which may result in a multidrug-resistant phenotype. This highlights the potential risk and warrants increased vigilance in managing these strains.
We acknowledge several limitations in the current study. First, only a single blaAFM-positive CRPA isolate was recovered, limiting the sample size and broader representativeness. Second, our analysis focused solely on blaAFM-positive CRPA strains from China, without including genomic comparisons with globally reported blaAFM-positive CRPA isolates.
Conclusion
This study systematically analyzed the genomic epidemiological characteristics of blaAFM-positive CRPA in China, revealing the resistance gene profiles and evolutionary traits associated with these isolates. blaAFM-1 and blaAFM-2 were identified as the predominant blaAFM variants, and blaAFM-positive CRPA strains from China were frequently found to harbor multiple critical resistance genes and exhibit diverse ST types. The resistance genes in blaAFM-positive CRPA strains from different regions demonstrated potential for interregional dissemination. The emergence of blaAFM-positive CRPA across multiple provinces in China highlights the urgent need for stringent monitoring and the implementation of appropriate measures to mitigate the future threats posed by these strains.
Data availability statement
The datasets presented in this study can be found in online repositories. The names of the repository/repositories and accession number(s) can be found in the article/Supplementary material.
Ethics statement
The studies involving humans were approved by this study was approved by the Ethics Committee of Zhengzhou University. The studies were conducted in accordance with the local legislation and institutional requirements. Written informed consent for participation was not required from the participants or the participants’ legal guardians/next of kin in accordance with the national legislation and institutional requirements.
Author contributions
HL: Investigation, Writing – original draft, Resources. CZ: Methodology, Software, Writing – original draft. BZ: Data curation, Formal analysis, Writing – original draft. YL: Project administration, Visualization, Writing – review & editing. SQ: Funding acquisition, Project administration, Resources, Writing – review & editing.
Funding
The author(s) declare that financial support was received for the research and/or publication of this article. This work was supported by National Natural Science Foundation of China (U20041257), Training Plan for Young Backbone Teachers in Colleges and Universities in Henan (2021GGJS016), Key project of Natural Science Foundation of Henan province (252300421272).
Acknowledgments
This work was supported by National Supercomputing Center in Zhengzhou.
Conflict of interest
The authors declare that the research was conducted in the absence of any commercial or financial relationships that could be construed as a potential conflict of interest.
Generative AI statement
The authors declare that no Gen AI was used in the creation of this manuscript.
Publisher’s note
All claims expressed in this article are solely those of the authors and do not necessarily represent those of their affiliated organizations, or those of the publisher, the editors and the reviewers. Any product that may be evaluated in this article, or claim that may be made by its manufacturer, is not guaranteed or endorsed by the publisher.
Supplementary material
The Supplementary material for this article can be found online at: https://www.frontiersin.org/articles/10.3389/fmicb.2025.1546662/full#supplementary-material
Footnotes
References
Alikhan, N. F., Petty, N. K., Ben Zakour, N. L., and Beatson, S. A. (2011). BLAST ring image generator (BRIG): simple prokaryote genome comparisons. BMC Genomics 12:402. doi: 10.1186/1471-2164-12-402
Bankevich, A., Nurk, S., Antipov, D., Gurevich, A. A., Dvorkin, M., Kulikov, A. S., et al. (2012). SPAdes: a new genome assembly algorithm and its applications to single-cell sequencing. J. Comput. Biol. 19, 455–477. doi: 10.1089/cmb.2012.0021
Bortolaia, V., Kaas, R. S., Ruppe, E., Roberts, M. C., Schwarz, S., Cattoir, V., et al. (2020). ResFinder 4.0 for predictions of phenotypes from genotypes. J. Antimicrob. Chemother. 75, 3491–3500. doi: 10.1093/jac/dkaa345
Carattoli, A., and Hasman, H. (2020). PlasmidFinder and in silico pMLST: identification and typing of plasmid replicons in whole-genome sequencing (WGS). Methods Mol. Biol. 2075, 285–294. doi: 10.1007/978-1-4939-9877-7_20
Chen, M., Cai, H., Li, Y., Wang, N., Zhang, P., Hua, X., et al. (2022). Plasmid-borne AFM alleles in Pseudomonas aeruginosa clinical isolates from China. Microbiol. Spectr. 10:e0203522. doi: 10.1128/spectrum.02035-22
CLSI (2018). Performance standards for antimicrobial susceptibility testing. 28th Edn. CLSI supplement M100. Wayne, PA: Clinical and Laboratory Standards Institute.
Fang, Y., Wang, N., Wu, Z., Zhu, Y., Ma, Y., Li, Y., et al. (2023). An XDR Pseudomonas aeruginosa ST463 strain with an IncP-2 plasmid containing a novel transposon Tn6485f encoding Bla(IMP-45) and Bla(AFM-1) and a second plasmid with two copies of Bla(KPC-2). Microbiol. Spectr. 11:e0446222. doi: 10.1128/spectrum.04462-22
Gray, H. K., Beaird, O. E., Smith, E. A., Schaenman, J. M., and Yang, S. (2023). Domestically acquired NDM-1-producing Pseudomonas aeruginosa, Southern California, USA, 2023. Emerg. Infect. Dis. 29, 2382–2385. doi: 10.3201/eid2911.230646
He, T., Wang, R., Liu, D. J., Walsh, T. R., Zhang, R., Lv, Y., et al. (2019). Emergence of plasmid-mediated high-level tigecycline resistance genes in animals and humans. Nat. Microbiol. 4, 1450–1456. doi: 10.1038/s41564-019-0445-2
Hu, Y., Liu, C., Wang, Q., Zeng, Y., Sun, Q., Shu, L., et al. (2021). Emergence and expansion of a Carbapenem-resistant Pseudomonas aeruginosa clone are associated with plasmid-borne Bla (KPC-2) and virulence-related genes. mSystems 6:6. doi: 10.1128/mSystems.00154-21
Jean, S. S., Gould, I. M., Lee, W. S., and Hsueh, P. R. (2019). International society of antimicrobial C. New drugs for multidrug-resistant gram-negative organisms: time for stewardship. Drugs 79, 705–714. doi: 10.1007/s40265-019-01112-1
Kleinheinz, K. A., Joensen, K. G., and Larsen, M. V. (2014). Applying the ResFinder and virulence finder web-services for easy identification of acquired antibiotic resistance and E. coli virulence genes in bacteriophage and prophage nucleotide sequences. Bacteriophage 4:e27943:e27943. doi: 10.4161/bact.27943
Lassalle, F., Al-Shalali, S., Al-Hakimi, M., Njamkepo, E., Bashir, I. M., Dorman, M. J., et al. (2023). Genomic epidemiology reveals multidrug resistant plasmid spread between Vibrio cholerae lineages in Yemen. Nat. Microbiol. 8, 1787–1798. doi: 10.1038/s41564-023-01472-1
Letunic, I., and Bork, P. (2021). Interactive tree of life (iTOL) v5: an online tool for phylogenetic tree display and annotation. Nucleic Acids Res. 49, W293–W296. doi: 10.1093/nar/gkab301
Li, Y., Liu, X., Yao, H., Zhao, X., Chi, L., Jin, C. Y., et al. (2025). The evolution of carbapenem-resistant Pseudomonas aeruginosa in the COVID-19 era: a global perspective and regional insights. Int. J. Antimicrob. Agents 65:107466. doi: 10.1016/j.ijantimicag.2025.107466
Li, Y., Sun, X., Dong, N., Wang, Z., and Li, R. (2024). Global distribution and genomic characteristics of carbapenemase-producing Escherichia coli among humans, 2005-2023. Drug Resist. Updat. 72:101031. doi: 10.1016/j.drup.2023.101031
Li, Y., Zhang, Y., Sun, X., Wu, Y., Yan, Z., Ju, X., et al. (2024). National genomic epidemiology investigation revealed the spread of carbapenem-resistant Escherichia coli in healthy populations and the impact on public health. Genome Med. 16:57. doi: 10.1186/s13073-024-01310-x
Li, Y., Zhu, Y., Zhou, W., Chen, Z., Moran, R. A., Ke, H., et al. (2022). Alcaligenes faecalis metallo-beta-lactamase in extensively drug-resistant Pseudomonas aeruginosa isolates. Clin. Microbiol. Infect. 28, 880.e1–e8. doi: 10.1016/j.cmi.2021.11.012
Livermore, D. M. (2002). Multiple mechanisms of antimicrobial resistance in Pseudomonas aeruginosa: our worst nightmare? Clin. Infect. Dis. 34, 634–640. doi: 10.1086/338782
Marchaim, D., Pogue, J. M., Tzuman, O., Hayakawa, K., Lephart, P. R., Salimnia, H., et al. (2014). Major variation in MICs of tigecycline in gram-negative bacilli as a function of testing method. J. Clin. Microbiol. 52, 1617–1621. doi: 10.1128/JCM.00001-14
Price, M. N., Dehal, P. S., and Arkin, A. P. (2009). FastTree: computing large minimum evolution trees with profiles instead of a distance matrix. Mol. Biol. Evol. 26, 1641–1650. doi: 10.1093/molbev/msp077
Reyes, J., Komarow, L., Chen, L., Ge, L., Hanson, B. M., Cober, E., et al. (2023). Global epidemiology and clinical outcomes of carbapenem-resistant Pseudomonas aeruginosa and associated carbapenemases (POP): a prospective cohort study. Lancet Microbe 4, e159–e170. doi: 10.1016/S2666-5247(22)00329-9
Saravanan, M., Belete, M. A., and Arockiaraj, J. (2023). Carbapenem-resistant Pseudomonas aeruginosa in intensive care units increase mortality as an emerging global threat. Int. J. Surg. 109, 1034–1036. doi: 10.1097/JS9.0000000000000184
Seemann, T. (2014). Prokka: rapid prokaryotic genome annotation. Bioinformatics 30, 2068–2069. doi: 10.1093/bioinformatics/btu153
Sullivan, M. J., Petty, N. K., and Beatson, S. A. (2011). Easyfig: a genome comparison visualizer. Bioinformatics 27, 1009–1010. doi: 10.1093/bioinformatics/btr039
Wick, R. R., Judd, L. M., Gorrie, C. L., and Holt, K. E. (2017). Unicycler: resolving bacterial genome assemblies from short and long sequencing reads. PLoS Comput. Biol. 13:e1005595. doi: 10.1371/journal.pcbi.1005595
Yang, L., Mai, G., Hu, Z., Zhou, H., Dai, L., Deng, Z., et al. (2023). Global transmission of broad-host-range plasmids derived from the human gut microbiome. Nucleic Acids Res. 51, 8005–8019. doi: 10.1093/nar/gkad498
Yoon, E. J., and Jeong, S. H. (2021). Mobile Carbapenemase Genes in Pseudomonas aeruginosa. Front. Microbiol. 12:614058. doi: 10.3389/fmicb.2021.614058
Zhang, X., Tang, M., Xu, Y., Xu, M., Qian, C., Zheng, X., et al. (2023). Characteristics of rare ST463 carbapenem-resistant Pseudomonas aeruginosa clinical isolates from blood. J. Glob. Antimicrob. Resist. 32, 122–130. doi: 10.1016/j.jgar.2023.01.011
Zhang, X., Wang, L., Li, D., Wang, C., Guo, Q., and Wang, M. (2021). Characterization of the novel plasmid-encoded MBL gene blaAFM-1, integrated into a blaIMP-45-bearing transposon Tn6485e in a carbapenem-resistant Pseudomonas aeruginosa clinical isolate. J. Antimicrob. Chemother. 77, 83–88. doi: 10.1093/jac/dkab342
Zhang, P., Wang, J., Shi, W., Wang, N., Jiang, Y., Chen, H., et al. (2022). In vivo acquisition of Bla(KPC-2) with low biological cost in Bla(AFM-1)-harboring ST463 hypervirulent Pseudomonas aeruginosa from a patient with hematologic malignancy. J. Glob. Antimicrob. Resist. 31, 189–195. doi: 10.1016/j.jgar.2022.09.004
Zhang, P., Wu, W., Wang, N., Feng, H., Wang, J., Wang, F., et al. (2023). Pseudomonas aeruginosa high-risk sequence type 463 co-producing KPC-2 and AFM-1 Carbapenemases, China, 2020-2022. Emerg. Infect. Dis. 29, 2136–2140. doi: 10.3201/eid2910.230509
Zhou, L., Yang, C., Zhang, X., Yao, J., Chen, L., Tu, Y., et al. (2023). Characterization of a novel Tn6485h transposon carrying both blaIMP-45 and blaAFM-1 integrated into the IncP-2 plasmid in a carbapenem-resistant Pseudomonas aeruginosa. J. Glob. Antimicrob. Resist. 35, 307–313. doi: 10.1016/j.jgar.2023.10.010
Keywords: patients, carbapenem resistance, Pseudomonas aeruginosa , blaAFM , genomics
Citation: Lu H, Zhang C, Zhao B, Li Y and Qin S (2025) Genomic insights into blaAFM-positive carbapenem-resistant Pseudomonas aeruginosa in China. Front. Microbiol. 16:1546662. doi: 10.3389/fmicb.2025.1546662
Edited by:
Shaolin Wang, China Agricultural University, ChinaReviewed by:
Okon Okwong Kenneth, Federal University, Wukari, NigeriaMahmuda Yasmin, University of Dhaka, Bangladesh
Muzafar Ahmad Rather, University of Minnesota Twin Cities, United States
Narjess Bostanghadiri, Tehran University of Medical Sciences, Iran
Copyright © 2025 Lu, Zhang, Zhao, Li and Qin. This is an open-access article distributed under the terms of the Creative Commons Attribution License (CC BY). The use, distribution or reproduction in other forums is permitted, provided the original author(s) and the copyright owner(s) are credited and that the original publication in this journal is cited, in accordance with accepted academic practice. No use, distribution or reproduction is permitted which does not comply with these terms.
*Correspondence: Yan Li, eWFuX2xpMThAMTYzLmNvbQ==; Shangshang Qin, cWluc2hhbmdzaGFuZ0AxMjYuY29t