- 1Department of Medical Entomology, National Institute of Infectious Diseases, Tokyo, Japan
- 2Antimicrobial Resistance Research Center, National Institute of Infectious Diseases, Tokyo, Japan
- 3Graduate School of Agricultural and Life Sciences, The University of Tokyo, Tokyo, Japan
- 4Management Department of Biosafety, Laboratory Animal, and Pathogen Bank, National Institute of Infectious Diseases, Tokyo, Japan
- 5Department of Medical Technology, Kumamoto Health Science University, Kumamoto, Japan
Introduction: Endosymbiotic bacteria show diverse strategies to manipulate host reproduction for their survival in nature. The diversity of symbionts infecting hematophagous insects and their impact on host ecology could be crucial for developing effective control measures of disease-transmitting vectors. Sand flies are a group of small insects, with some species serving as vectors for leishmaniasis, bartonellosis, and arboviral diseases. Sergentomyia squamirostris is the only known species of sand flies found on the main islands of Japan. Although no medical implications exist for S. squamirostris, we conducted whole-genome sequencing to explore its potential relevance.
Methods: We conducted whole-genome sequencing and de novo assembly of S. squamirostris using genomic DNA isolated from a single field-collected female insect sample. During this attempt, we incidentally obtained closed genomes of two new bacteria, wSSQ and RiSSQ, belonging to Wolbachia and Candidatus Tisiphia, respectively. We then investigated infection rates of both bacteria in two natural populations of S. squamirostris in Japan.
Results: Phylogenetic analysis indicated that wSSQ and RiSSQ belonged to Wolbachia and Ca. Tisiphia, respectively. Ca. Tisiphia is also known as “Torix Rickettsia,” which is considered a large group of endosymbionts of invertebrates with no known pathogenicity to humans and animals. NGS read depths for both wSSQ and RiSSQ genomes were found to be high coverages, indicating that these bacteria are S. squamirostris endosymbionts. The infection rates of wSSQ and RiSSQ in the wild population of S. squamirostris varied in the two different localities in Japan, and co-infection with both bacteria was commonly seen. wSSQ was detected in both sexes of S. squamirostris, whereas RiSSQ was detected only in female sand flies.
Conclusion: Ca. Tisiphia has recently been recognized as an underexplored endosymbiont with a widespread presence in invertebrates, including disease vectors. RiSSQ represents the first complete genomic information resource of Ca. Tisiphia infecting sand flies. Further research is needed to understand potential interactions between its host and other endosymbionts, as well as to explore the potential implications of disease control in the future.
1 Introduction
Phlebotominae sand flies (Diptera; Psychodidae) are a family of small insects with an adult body length of ~3 mm. Adult female sand flies obligately feed on the blood of vertebrates to facilitate oviposition. Male sand flies, on the other hand, do not have a hematophagous habitat and are believed to rely on plant sugars, such as nectar, as their energy source. Some species of sand flies are known to transmit significant human and animal diseases, such as leishmaniasis, arboviruses, and Bartonella. In Japan, only one species of the Phlebotominae insect, Sergentomyia squamirostris, had been described on the main island (Sanjoba et al., 2011) until S. iriomotensis was newly described in the Ryukyu Archipelago, just recently (Sanjoba and Miyagi, 2022). Although S. squamirostris may transmit some Trypanosoma parasites to amphibians (Feng and Chao, 1943), no evidence suggests that either species has medical implications for human or domestic animal health to date.
Wolbachia and Rickettsia belong to the order Rickettsiales, which comprises a large group of obligate intracellular parasitic bacteria. Wolbachia, belonging to the Ehrlichiaceae family, is a well-known maternally transmitted symbiont found in a wide range of arthropod species and some nematodes. In arthropods, Wolbachia shows intriguing phenotypes that manipulate host reproduction, including cytoplasmic incompatibility (CI), male killing (MK), feminization, and parthenogenesis (Hurst and Frost, 2015). All those phenotypes are considered to benefit the bacteria from propagating and maintaining within the host population. Additionally, certain strains of Wolbachia are known to establish obligate mutualistic relationships with their hosts, in which the bacteria provide essential nutrients, such as biotin (vitamin B7) (Foster et al., 2005; Hosokawa et al., 2010). CI is the most commonly observed host manipulation phenotype of Wolbachia, where offspring produced by infected males and uninfected females are inviable. Primary genetic factors of CI, cifA, and cifB (cytoplasmic incompatibility factors A and B) have recently been characterized (Beckmann et al., 2017; LePage et al., 2017). In the genomes of Wolbachia, cifA and cifB usually exist in pairs, with cifA located directly upstream of cifB (Martinez et al., 2021). Transgenic experiments have indicated that the expression of both cifA and cifB in paternal testes is required to induce CI death in embryos, whereas cifA expression alone in the maternal ovary can rescue this effect (Beckmann et al., 2017; LePage et al., 2017; Shropshire et al., 2018; Shropshire and Bordenstein, 2019). There is a relatively clear-cut relationship between the presence/absence of a functional cifA/B pair in the genome and that of the CI phenotype (Lindsey et al., 2018; Martinez et al., 2021). Certain strains of Wolbachia have been shown to suppress viral replication in transinfected mosquitoes (Walker et al., 2011). Due to this ability, along with their capacity to spread into uninfected populations autonomously as reproductive parasites as described above, Wolbachia has gained significant attention as a potential tool for disease control (Hoffmann et al., 2011; Caragata et al., 2021).
Rickettsiaceae includes important arthropod-borne human pathogens, such as some Rickettsia species causing spotted fever and typhus and Orientia tsutsugamushi causing scrub typhus. Although Rickettsiaceae were initially implicated as a group of such significant pathogens, the majority of known species in this family are now considered vertically transmitted symbionts of invertebrates and some protists (Werren et al., 1994; Lawson et al., 2001; Giorgini et al., 2010; Schrallhammer et al., 2013). “Torix group of Rickettsia” is also a group of Rickettsiaceae, which was first described in a species of leech, Torix tagoi, in 2005 (Kikuchi et al., 2002). Since its discovery, 16S gene fragments phylogenetically related to this bacterium have been detected in diverse species of arthropods (regardless of hematophagous habits) and some amoebae (Dyková et al., 2003). Neither an example of infection nor pathogenicity to vertebrates of this group of bacteria is known so far. Despite being found in a wide range of invertebrate species (Pilgrim et al., 2021), this group of bacteria has gained relatively little attention thus far, as the first draft (Pilgrim et al., 2017) and complete (Davison et al., 2022) genome assemblies of this group have only recently been published. Although they have been referred to as the “Torix group of Rickettsia” or the “Torix clade of Rickettsia,” Davison et al. (2022) have recently proposed a distinct genus, Candidatus Tisiphia, for these bacteria due to their apparent phylogenetic divergence from known members of the genus Rickettsia.
Numerous reports have described Wolbachia infection in wild populations of sand fly species (Cui et al., 1999; Ono et al., 2001; Rosário et al., 2022; Wang et al., 2022; Lozano-Sardaneta et al., 2023). Although fewer in number, some studies also suggest the presence of Rickettsiaceae endosymbionts in sand flies. Reeves et al. (2008) detected a 16S rRNA sequence in Lutzomyia apache in Wyoming, USA, that was 99% identical to a Rickettsia species previously reported from leeches. Li et al. (2016) detected a bacterial 16S rRNA homologous to Rickettsia from Phelbotomus cinensis in Sichuan, China. Lozano-Sardaneta et al. (2021) recently reported the detection of “Torix group” Rickettsia in Psathyromyia aclydifera in Los Tuxtlas, Mexico. These results suggest that infection of Ca. Tisiphia is not uncommon even among sand flies.
The small size and limited number of available established laboratory colonies make genomic analysis of this important group of insects challenging (Huang et al., 2024). Although S. squamirostris has not been recognized as a vector of any pathogen or nuisance pest in humans to date, we attempted to conduct a de novo genome assembly of a single specimen of wild-caught insects to test the technical feasibility of this approach and explore the potential medical implications of this species. During this attempt, we incidentally obtained two closed assemblies of the bacterial genome, each belonging to Ca. Tisiphia and Wolbachia, along with a moderately contiguous draft of the insect genome.
2 Materials and methods
2.1 Sample collection
Adult sand flies, S. squamirostris were captured in Sado Island, Niigata prefecture (38°04′56″N 138°23′00″E) in 2018 and 2019 and Hakusan, Ishikawa prefecture, Japan (36°18′31″N 136°33′00″E) in 2021 (Figure 1) using CDC miniature light traps or sticky paper traps that were prepared using A4 paper coated with castor oil. The heads and abdomens of the collected sand flies were separated from the whole body and mounted on labeled slides containing Swan solution. The remainder of the body was preserved in 99.9% ethanol for further DNA extraction. Species were identified using published keys and descriptions based on the cibarium, pharynx, and spermathecae features in females, and genitalia, coxites, and styles in males (Lewis, 1987). The morphological characteristics were compared with previous descriptions (Newstead, 1923; Sanjoba et al., 2011) observed under BX51 and SZ61 microscopes (Olympus Co., Tokyo, Japan).
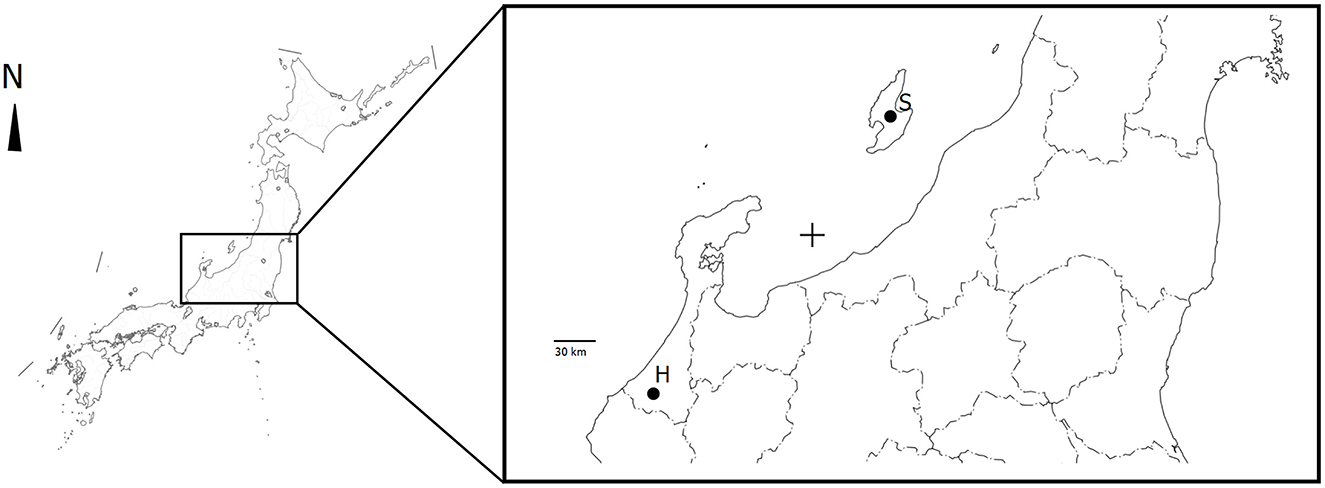
Figure 1. Map depicts the sample collection sites on the central-north coast of Japan. (S) Sado Island, Niigata and (H) Hakusan, Ishikawa Prefecture. The map was reproduced from the Geospatial Information Authority of Japan website (https://www.gsi.go.jp). This map is licensed under the Government of Japan Standard Terms of Use (Ver.2.0), which are compatible with the Creative Commons Attribution License 4.0 (CC By).
2.2 Genomic DNA extraction
The genomic DNA (gDNA) of the insects was purified individually using a MagExtract-genome kit (TOYOBO, Japan) in an 8-striped PCR tube. Purified gDNA was eluted in 25 μl of low-TE buffer (10 mM Tris–HCl, 0.1 mM EDTA, pH 8.0). The extracted DNA was quantified using the Qubit DNA HS kit (Thermo Fisher Scientific, the US).
2.3 Sequencing gDNA
The amount of gDNA obtained varied largely among individuals. One female insect from Sado Island in 2019 (Sado-F01) with the maximum recovery of gDNA (250 ng) was selected for sequencing analysis. A DNA library for nanopore sequencing was prepared from 180 ng gDNA from Sado-F01 with the SQK-LSK109 kit (Oxford Nanopore Technology, ONT, UK). The gDNA was end-prepped using the NEBNext Ultra II End Repair/dA-Tailing Module (New England Biolabs (NEB), US). The end-prepped DNA was purified with AMPureXP (Beckman Coulter Life Sciences, US) and ligated to the 1D adapter with Quick T4 DNA Ligase (NEB). The ligated DNA was purified using AMpure XP. A short fragment wash buffer (SFB) was used in the final bead-washing step. The library was sequenced in a single R.9.4.1 flow cell for MK1B (ONT). The obtained FAST5 files (raw signals) were basecalled with guppy v6.0.1 with the “super accuracy (sup)” model. Long reads obtained from nanopore sequencing were trimmed to 50-nt at both ends to remove adapter sequences using Seqtk (https://github.com/lh3/seqtk). Reads shorter than 1.5 kb were discarded for the subsequent assembly and polishing steps.
The PCR-free TruSeq library was prepared from 24 ng gDNA of Sado-F01 using the NEBNext Ultra II FS DNA Library Prep Kit for Illumina (NEB) with TruSeq DNA and RNA combinatorial dual-indexed adapters (Illumina, US). The library was sequenced using the NextSeq500 system (Illumina) with a 150PE mid-output kit along with other libraries. The obtained reads were trimmed and filtered using FASTP (Chen et al., 2018).
2.4 De novo assembly and QC
The trimmed long reads were subjected to de novo assembly by Flye v2.9 (Kolmogorov et al., 2019) with the “–meta” option (Kolmogorov et al., 2020). The obtained assembly was polished with medaka v1.5 (https://github.com/nanoporetech/medaka) using ONT long reads. The polished assemblies were further corrected with short reads using NextPolish v1.4.0 (Hu et al., 2020). Centrifuge v1.0.4 (Kim et al., 2016) was used to check for potential contamination of bacterial genomes in this initial assembly. Contigs of suspected symbiont genomes were further polished with short reads using PolyPolish software (Wick and Holt, 2022). From the insect genome, short contigs (smaller than 3,000 bp) and redundant haplotigs were purged with Purge_dups v1.2.5 (Guan et al., 2020) using information on short read coverage. Mitochondrial DNA was assembled from a subset (~130 Mb) of short reads using MEGAHIT v1.2.9 (Li et al., 2015). The completeness of the insect genome assembly was assessed by Benchmarking Universal Single-Copy Ortholog (BUSCO) v5.4.6 (Manni et al., 2021) against the diptera_odb10 dataset. For a comparative analysis, the available genome assembly and predicted protein sequences of Lutzomyia longipalpis ASM2433408v1 (accession no. GCF_024334085.1) (Huang et al., 2024), Phlebotomus papatasi Ppap_2.1 (GCF_024763615.1) (Huang et al., 2024), P. argentipes (GCF_947086385.1), and P. perniciosus asm_v2.0 (GCA_918844115.2) were benchmarked by the same version of BUSCO. The quality of the bacterial genomes was assessed with CheckM v1.2.2 (Parks et al., 2015) using the 2015_01_16 database. The mitochondrial genome was assembled from a subset (~130 Mb) of short reads using MEGAHIT v1.2.9 (Li et al., 2015).
2.5 Coverage analysis
S. squamirostris and bacterial genome assemblies were combined into a single FASTA file. Short read data were mapped to this combined reference by minimap2 (Li, 2018) with “-x sr -a” options, and then aligned reads were sorted by Samtools (Li et al., 2009). From the resulting BAM, Samtools depth with the “-a” option was used to obtain depth at every base position. The depth data were plotted on a histogram using Matplotlib (Hunter, 2007) in python3.
2.6 Gene annotation and comparative genomics of bacteria
The AutoMLST server (Alanjary et al., 2019) was used to classify the bacterial genomes into approximate taxonomic positions. Gene prediction and functional annotation were conducted using the DNA Data Bank of Japan (DDBJ) Fast Annotation and Submission Tool (DFAST) v1.2.18 (Tanizawa et al., 2018). Circos genome plots were generated using the GenoVi pipeline v0.2.16 (Krzywinski et al., 2009; Feldbauer et al., 2021; Cumsille et al., 2023). Bacterial genome assemblies belonging to Wolbachia, Ca. Tisiphia and closely related genomes for rooting trees were downloaded from the NCBI database (Supplementary Table S1). To infer the phylogenetic placement of RiSSQ and wSSQ among other Rickettsiaceae and Ehrlichiaceae, respectively, amino acid sequences of orthologs predicted by BUSCO v5.4.6 for the bacteria_odb10 lineage dataset were used to infer the phylogenies of bacteria. Among the 124 orthologs in bacteria_odb10, 54 and 70 orthologs (Supplementary Table S2) were selected as complete orthologs found in all genomes of Rickettsiaceae and Ehrlichiaceae, respectively, for comparison. Each ortholog family was multiple aligned with MAFFT v7.520 (Katoh and Standley, 2013) and trimmed by TrimAl v1.4.1 (Capella-Gutiérrez et al., 2009) with “–nogaps” mode. The trimmed alignments were concatenated into single alignments consisting of 12,415 and 19,882 amino acid residues for Rickettsiaceae and Ehrlichiaceae, respectively. The concatenated sequence was used for tree estimation using IQ-TREE v2.2.6 (Minh et al., 2020). Partitioning was implemented based on gene boundaries, and the best partition scheme and substitution models for each alignment were searched using the “-m MFP+MERGE” option (Lanfear et al., 2012; Kalyaanamoorthy et al., 2017). To estimate the branch support of the tree, 1,000 ultrafast bootstrap (UFBoot) resamplings were conducted (Hoang et al., 2018). For RiSSQ, the 16S RNA sequences were also compared with the 16S sequences of other Ca. Tisiphia (Supplementary Table S3), using MAFFT, TrimAl, and IQ-TREE. For metabolic analysis, gene functions were annotated with “anvi-run-kegg-kofams” using the KEGG Orthology database (last updated: 24 November 2020), and the completeness of metabolic pathways was estimated with “anvi-estimate-metabolism” in Anvi'o v7.1 (Aramaki et al., 2020; Eren et al., 2021; Veseli et al., 2023).
2.7 Search for the prophage region and cifA and cifB homologs
Prophage sequences were identified using PHASTEST (Wishart et al., 2023) (accessed 2 June 2024). The dataset of Martinez et al. (2021) was used as a reference sequence for the well-characterized cifA and cifB. The BLASTP algorithm (Altschul et al., 1990) was used to search for similar sequences, using the references as targets and the predicted protein sequences of wSSQ or RiSSQ as queries. Matches to the cifB references were restricted to the first 500 amino acids from their N-terminal because this region contains highly conserved domains (AAA-ATPase-like and PD-(D/E)XK nuclease domains) among cifBs, whereas the rest of the region contains domains such as ankyrin repeats, which tend to result in spurious hits to non-cif proteins. We also generated profile hidden Markov models (hmms) from multiple sequence alignments of each reference cif protein using “hmmbuild,” and used them to search for homologs in predicted proteins of wSSQ or RiSSQ by using “hmmsearch” in the HMMER v3.1b2 package (Eddy, 2011).
2.8 Repeat masking, gene prediction, and annotation of S. squamirostris genome
Repeat elements in S. squamirostris genome assembly were predicted using RepeatModeler v2.0.5 (Flynn et al., 2020) with the “-LTRStruct” option. The assembly was then masked by RepeatMasker v4.1.5 (Tarailo-Graovac and Chen, 2009) using predicted repetitive sequence families. The BRAKER3 pipeline (Lomsadze et al., 2005; Stanke et al., 2006, 2008; Gotoh, 2008; Iwata and Gotoh, 2012; Buchfink et al., 2015; Hoff et al., 2016, 2019; Bruna et al., 2020a,b) was used to predict protein-coding sequences. The combined protein sequences of Phlebotomus papatasi (GCF_024763615.1) and Lutzomyia longipalpis (GCF_024334085.1) were used as reference databases. Orthomapper v3.0.5 was used to cluster the predicted proteins with known dipteran orthologous groups using OrthoDB v11 (Kuznetsov et al., 2023). Barrnap v0.9 (https://github.com/tseemann/barrnap) was used to predict ribosomal RNA (rRNA) genes, and tRNAscan-SE v2.0.12 (Chan et al., 2021) was used to predict transfer RNA (tRNA) genes. MitoZ v3.6 was used to predict and annotate mtDNA genes (Meng et al., 2019).
2.9 Insect phylogenomics
Protein sequences of single-copy orthologs extracted from the results of the BUSCO analysis (insecta_odb10) were used for the phylogenomic analysis of insects. The compared taxons included three mosquito genomes, Aedes aegypti AaegL5.0 (GCA_002204515.1), Anopheles gambiae AgamP3 (GCA_000005575.1), and Culex quinquefasciatus CulPip1.0 (GCA_000209185.1); one moth fly genome, Clogmia albipunctata ASM101494v1 (GCA_001014945.1); and four sand fly genomes, L. longipalpis ASM2433408v1 (GCF_024334085.1), P. argentipes (GCF_947086385.1), P. papatasi Ppap_2.1 (GCF_024763615.1), and P. perniciosus (GCA_918844115.2). Among the 1,367 insecta_odb10 ortholog groups, 1,004 were selected as complete genes among all species. Each ortholog group was multi-aligned by MAFFT v7.520 (Katoh and Standley, 2013), and the alignments were trimmed by TrimAl v1.4.1 (Capella-Gutiérrez et al., 2009) with “–nogaps” mode. The trimmed alignments were concatenated using SeqKit v2.6.1 (Shen et al., 2016), which included 355,011 amino acid residues per species. The concatenated sequence was used for tree estimation using IQ-TREE v2.2.6 (Minh et al., 2020) with 1,000 UFBoot resampling (Hoang et al., 2018). Partitioning was implemented based on gene boundaries, and the best partition scheme and substitution model search were performed with the “-m MFP+MERGE” option. Individual gene trees were also constructed from each trimmed alignment using IQ-TREE with the “-m MFP” option. Internal branches with low support values (UFBoot < 10%) in each tree were contracted to give multifurcation. The coalescent phylogenetic tree was then estimated by Astral v5.7.8 (Zhang et al., 2018) using contracted individual gene trees.
2.10 Population infection rate analysis
The presence of RiSSQ and wSSQ in other fields collected S. squamirostris samples was checked by PCR using specific primers (with the M13F tail on the forward primers) targeting the genes OmpA (RiSSQ chromosome), DnaA (RiSSQ plasmid), and wsp (wSSQ chromosome; Table 1). As a positive control to ensure the quality of the extracted gDNA, a fragment of the NAD5 gene in S. squamirostris mitochondria was also amplified. The PCR mixture (10 μl) consisted of 1x PCR Buffer for KOD FX (TOYOBO), 0.4 mM dNTPs, 0.25 μM of each primer, 0.02 U/μl of KOD-FX polymerase (TOYOBO), and 0.5–2 ng of gDNA, and the thermal condition was 95°C for 15 s followed by 40 cycles of 95°C for 15 s, 57°C for 15 s, and 68°C for 45 s. Each PCR product was visualized on 1.5% agarose gel containing GelRed dye (Biotium, US). DNA was purified from positive samples using AMPureXP (× 1.0) and subjected to capillary sequencing (GeneWiz, Japan) using the M13F primer.
2.11 Statistical analysis
Fisher's exact test was conducted in R version 4.2.2 (R Development Core Team, 2014) using the fisher.test() function.
3 Results
3.1 Two bacterial genomes assembled from an individual insect
Genomic DNA (gDNA) was extracted individually from S. squamirostris collected from Sado Island, Japan, in 2018 and 2019 and from Ishikawa Prefecture in 2021. We obtained 5.2 G base of long reads (N50 16.6 kb) by ONT and 15 Gbase of 150 bp paired-end short reads by Illumina. After de novo assembly, polishing, and correction, bacterial contamination in the resulting assembly was checked by centrifuge (Kim et al., 2016). This quality check indicated that the initial assembly contained two large contigs of potential bacterial genomes in addition to the lambda phage DNA sequence, which was used for quality control (DNA CS) in the Nanopore library preparation kit. AutoMLST (Alanjary et al., 2019) phylogenomic analysis placed one large bacterial contig (RiSSQ) close to the genome of RiCNE (GenBank accession number GCF_002259525.1) (Pilgrim et al., 2017), a member of Ca. Tisiphia in a biting midge, Culicoides newsteadi. Another large bacterial contig (wSSQ) was assigned to the Wolbachia clade.
3.2 Genome of RiSSQ
The closed assembly of the RiSSQ chromosome (1,627,389 bp, 32.8 GC%; accession no. GCA_042848025.1) contained 1,541 coding sequences (CDSs), 35 tRNA genes, and 3 rRNA genes (Figure 2). In addition to the chromosome, we found a circular small contig (25,235 bp, 31.04 GC%) of a putative RiSSQ plasmid containing 31 CDSs, one of which has homology to chromosomal replication initiator proteins (DnaA), LF885_07200 (UCM86407, 59% amino acid identity) and LF885_07390 (UCM86444, 57% amino acid identity), encoded in the two plasmids in RiCimp, a strain of Ca. Tisiphia found in Culicoides impunctatus (Davison et al., 2022). The CheckM analysis scored the quality of genome assembly with 99.05% completeness and 1.66% contamination. The metabolic pathways predicted by the RiSSQ gene set included the complete pentose phosphate pathway (PPP) (Supplementary Figure S1), which is consistent with other members of Ca. Tisiphia (Pilgrim et al., 2017; Davison et al., 2022). On the other hand, the gene set for the biotin synthesis pathway, which is often associated with mutualistic symbionts (Nikoh et al., 2014), was not present in the RiSSQ genome (Supplementary Figure S1). Furthermore, no homologs of cifA/B were found. Phylogenetic analysis using 54 complete orthologous protein sequences placed RiSSQ within Ca. Tisiphia clade (Figure 3). Among Ca. Tisiphia and RiSSQ genomes clustered with the RiClec and RiTbt genomes found in Cimex lectularius and Bemisia tabaci, respectively, which has been described as “Leech subclade” in Davison et al. (2022). Some of the Ca. Tisiphia 16S rRNA sequences were retrieved from the NCBI nucleotide collection (accessed in January 2024). Although 16S rRNA sequences did not have enough power to resolve detailed phylogenetic structures, the 16S gene sequences of “Rickettsia” in other sand fly species, Phlebotomus chinensis in Henan, China, 2015 (Li et al., 2016) (KX363666.1 and KX363668.1), Lutzomyia apache collected in Wyoming, USA (Reeves et al., 2008) (EU223247.1), and Psathyromyia aclydifera collected in Los Tuxtlas, Mexico (Lozano-Sardaneta et al., 2021) (MT158807.1) all belong to the Ca. Tisiphia clade (Supplementary Figure S2). The coverage of mapped NGS short reads was calculated to estimate the relative abundance of each bacterial chromosome and plasmid within a female S. squamirostris (Sado-F01) whole body. A single peak in the read depth distribution was observed for each haplotig-purged insect chromosome, mitochondria, bacterial chromosome, and plasmid (Supplementary Figure S3). The RiSSQ chromosome and plasmid had ~10.6- and 41.8-fold more read depths, respectively, than the insect chromosomes.
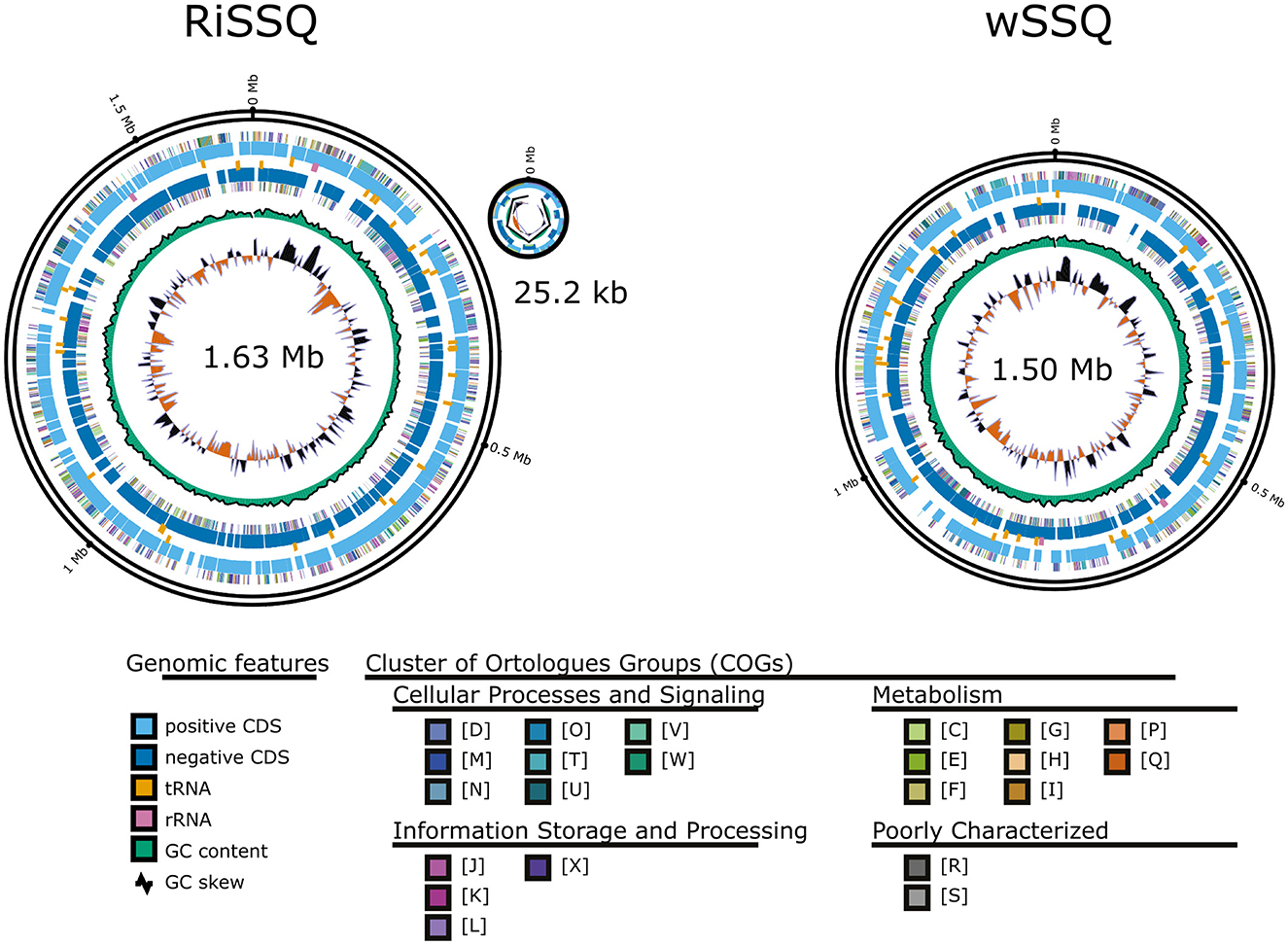
Figure 2. GenoVi circular representation of RiSSQ (+plasmid) and wSSQ. Genome size and CheckM score are described for each genome. The alphabet symbols for each COG category correspond to the following; D: cell cycle control, division, chromosome partitioning; M: cell wall/membrane/envelope biogenesis; N: cell motility; O: post-translational modification, protein turnover, chaperones; T: signal transduction mechanism; U: intracellular trafficking, secretion, and vesicular transport; V: defense mechanism; W: extracellular structures; Y: nuclear structure; Z: cytoskeleton; A: RNA processing and modification; B: chromatin structure and dynamics; J: translation, ribosomal structure, and biogenesis; K: transcription; L: replication, recombination, and repair; X: mobilome: prophages, transposons; C: energy production and conversion; E: amino acid transport and metabolism; F: nucleotide transport and metabolism; G: carbohydrate transport and metabolism; H: coenzyme transport and metabolism; I: lipid transport and metabolism; P: inorganic ion transport and metabolism; Q: secondary metabolites biosynthesis, transport, and metabolism; R: general function prediction only; and S: function unknown.
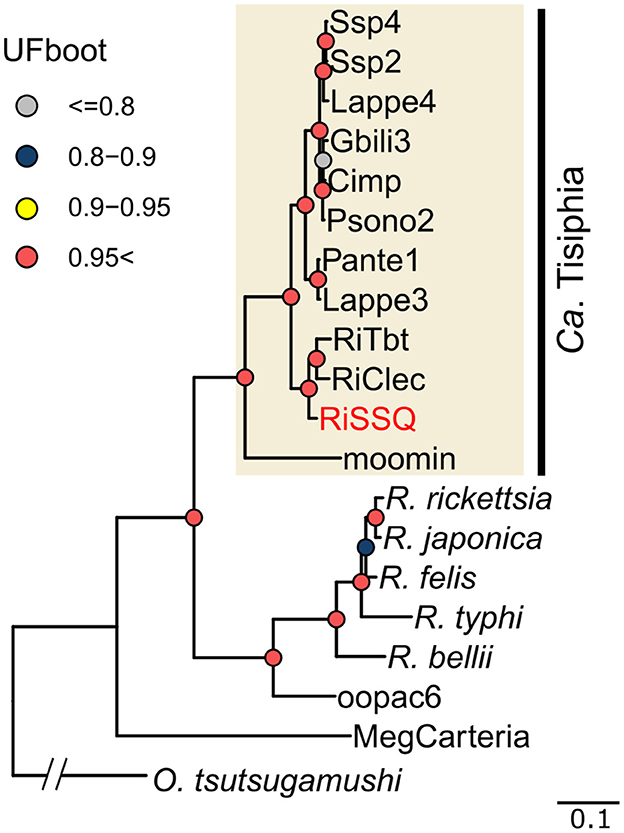
Figure 3. Phylogenetic placement of RiSSQ in Ca. Tisiphia and other Rickettsiaceae based on concatenated protein sequences of 54 orthologs. The details of each taxon written in the alias, and genes used for phylogenetic analysis are described in Supplementary Tables S1, S2, respectively.
3.3 Genome of wSSQ
The chromosome of wSSQ (1,495,460 bp, 34.9 GC%, accession no. GCA_042853495.1) contained 1,376 CDSs, 35 tRNA genes, and three rRNA genes (Figure 2). CheckM analysis scored the quality of genome assembly with 99.79% completeness and 0.21% contamination. Phylogenomic analysis of other Wolbachia genomes, outgrouped by Anaplasma marginale, using 70 orthologous protein sequences, indicated that wSSQ belongs to the Supergroup A clade of Wolbachia (Figure 4A). The relative depth of mapped NGS short reads on the wSSQ chromosome was 3.9-fold higher than that on insect chromosomes and approximately three-fold lower than that of RiSSQ (Supplementary Figure S3). The gene set for the biotin synthesis pathway is absent in the wSSQ genome (Supplementary Figure S1).
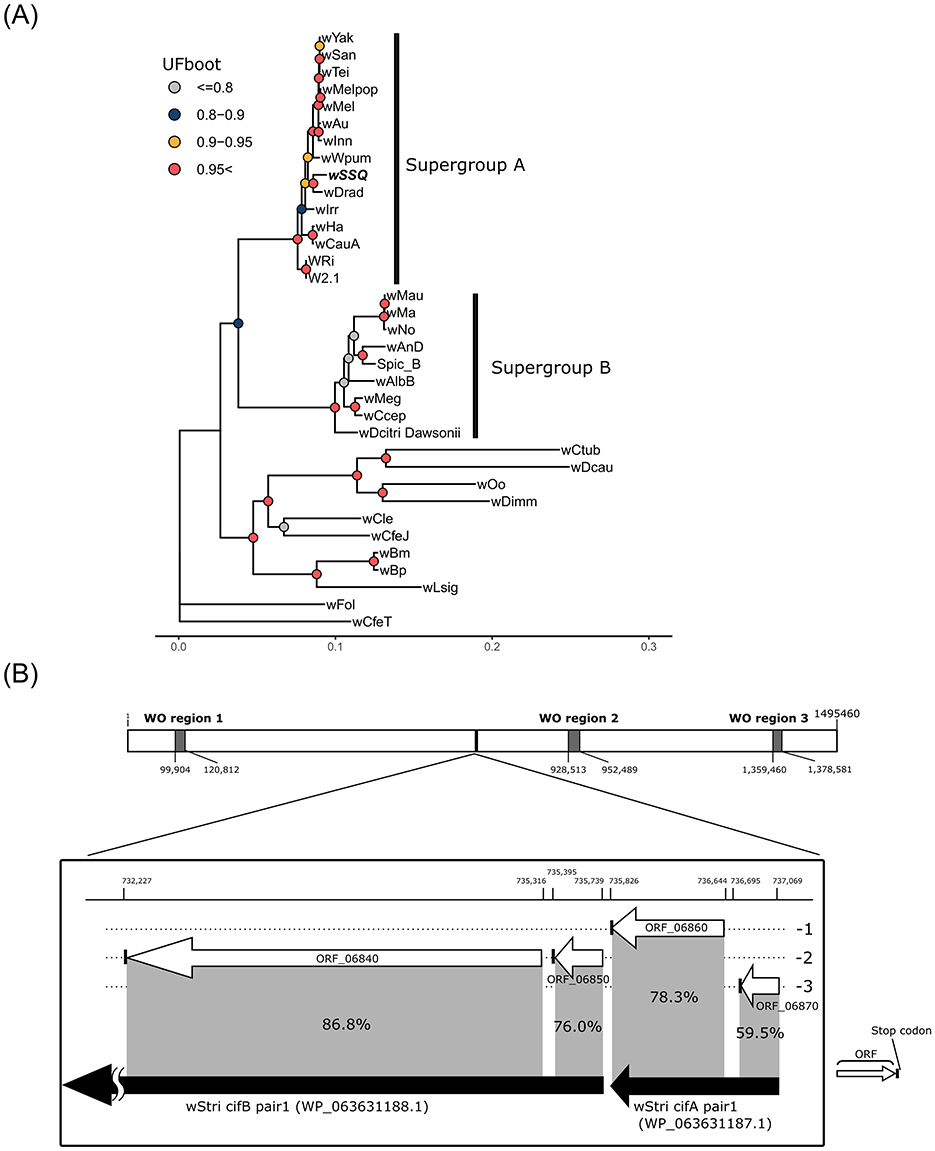
Figure 4. (A) Phylogenetic placement of the wSSQ genome among Wolbachia genomes based on concatenated protein sequences of 54 orthologs. The Anaplasma marginale (ASM2030v1) genome was used to root the tree. The details of each taxon written in the alias and genes used for phylogenetic analysis are described in Supplementary Tables S1, S3, respectively. (B) Structure of ORF_06840-70 in the wSSQ genome. Levels of the dotted line indicate the translational frame in both strands. White box arrows indicate open reading frames (ORFs), and vertical lines at the tip of ORFs indicate stop codons. Black box arrows show the wStri cifA and cifB protein sequences aligned to each wSSQ ORF with BLASTP hit %identity in the gray shade.
Four open reading frames (ORFs; ORF_06840, ORF_06850, ORF_06860, and ORF_06870) were homologous to known cifA or cifB (Martinez et al., 2021) detected by both BLASTP and HMMER searches. These ORFs were located consecutively and directly (Figure 4B) outside the predicted WO prophage regions of the wSSQ genome. The results of BLASTP alignment indicated that ORF_06840 and ORF_06850 represented fragments of a single cifB gene that had been split by a premature stop codon. This mutation separated the “AAA-ATPase-like domain” on the N-terminal from other parts of the original cifB protein. ORF_06860 and ORF_06870 were also considered to represent fragments of a single cifA gene, which has been separated due to a potential frameshift mutation (Figure 4B). The tentative wSSQ cifA (ignoring the stop codon) and cifB (joining two fragmented ORFs) amino acid sequences have high homology to cifA pair1 in wStri and cifB single 2 in Wolbachia in Acromyrmex echinatior, both of which belong to the type V group, according to Martinez et al. (2021) (Supplementary Figure S4).
3.4 Genome of S. squamirostris
The obtained assembly for the haploid genome of S. squamirostris (Ssqu_1.0, accession no. GCA_042850755.1) consisted of 2,379 contigs for chromosomal DNA (total = 195,863,774 bp, N50 = 215 kb, GC% = 35.58) and one contig for the complete mitochondrial DNA sequence (total = 15,381 bp, GC% = 23.1). BUSCO v5.4.6 (diptera_odb10, 3,285 ortholog groups) analysis found that 91.0% were completely conserved orthologs with 2.8% duplication, which was comparable to the available assemblies for other sand fly species (Figure 5A). BRAKER3 pipeline using the reference protein sequences of P. papatasi and L. longipalpis predicted 15,013 protein-coding genes. The predicted set of proteins captured 93.9% of diptera_odb10 orthologs (Figure 5A). Phylogenomic analysis was conducted using 1,004 concatenated protein orthologs. The inferred tree, rooted in the mosquito and moth fly (Psychodidae) genomes, placed S. squamirostris in the basal clade of the Phlebotominae family (Figure 5B). Although this tree topology was supported in the coalescent species tree estimated by ASTRAL using individual gene trees, there was a relatively high discordance of topologies among the individual gene trees, as seen in low quartet support scores (46.0–94.41) for internal branches within Phlebotominae (Supplementary Figure S5).
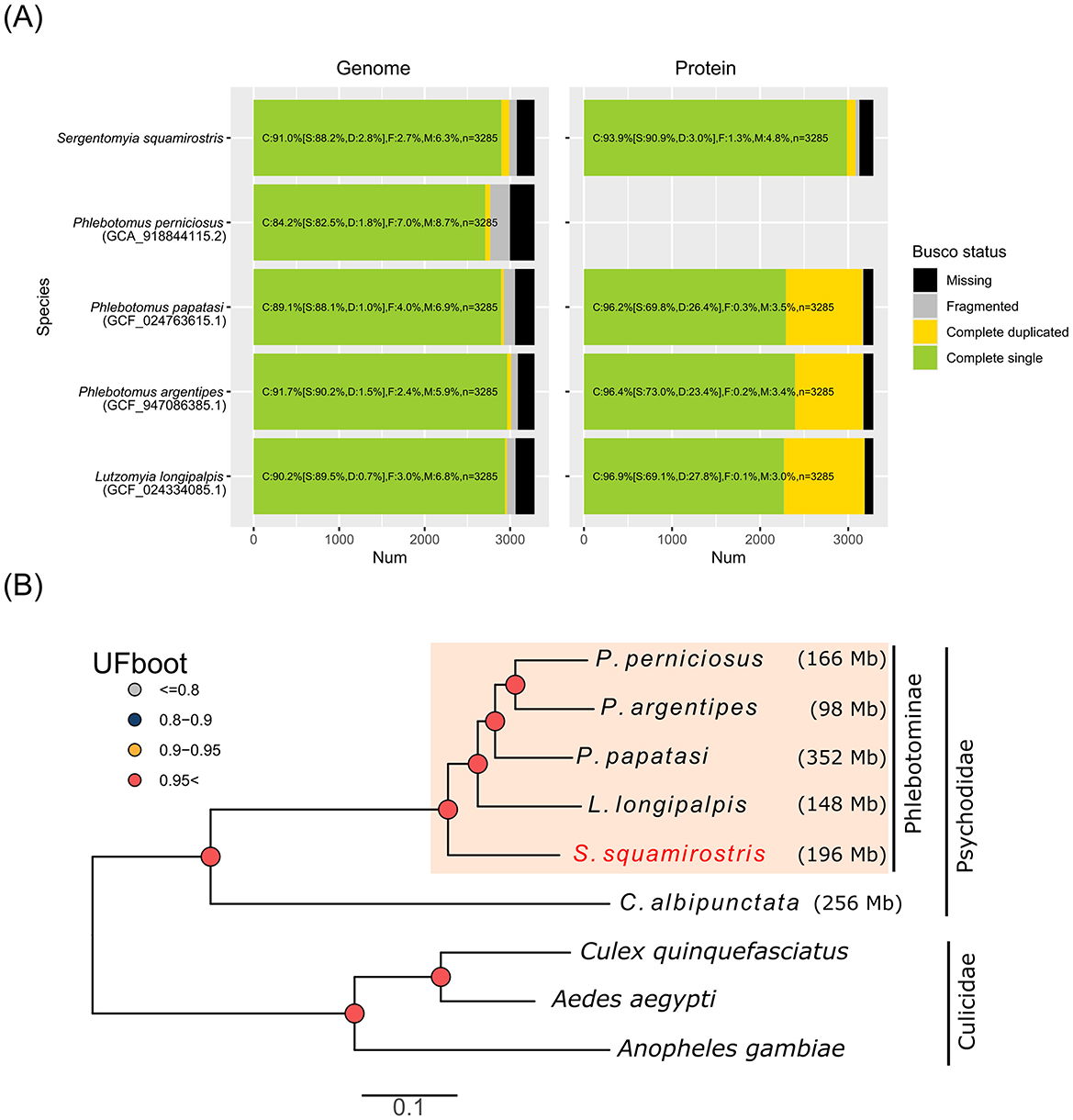
Figure 5. (A) Comparison of BUSCO scores for assembly and protein prediction of S. squamirostris to those in the reference genomes of sand fly species. Protein data for P. perniciosus were not registered as of January 2024. (B) ML phylogenetic tree for sand fly genomes based on concatenated protein sequences of 1,004 orthologs (Supplementary Table S4). Genomes of the drain fly (Clogmia albipunctata) and three mosquito (Culicidae) species are included in the root of the tree.
3.5 Population analysis
The genomic DNA of an additional 31 females and 13 males collected during 2018–2019 from Sado Island was tested for infection with RiSSQ and wSSQ using PCR (Figure 6 and Table 2). S. squamirostris mitochondrial NAD5 gene was used as a positive control to assess the integrity of the extracted DNA. Among the 32 female S. squamirostris (including Sado19-F01) samples during this period, 23 (72%) flies were PCR positive for RiSSQ gene markers, and 24 (75%) were positive for wSSQ chromosome gene markers. In contrast, among the 13 males investigated, 5 (38%) flies were positive for the wSSQ marker, suggesting a lower infection rate than females (p = 0.016 in Fisher's exact test for female-vs.-male positive rate), and were all negative for RiSSQ (p = 6.8 × 10−8 in Fisher's exact test for female-vs.-male positive rate). The RiSSQ plasmid marker (DnaA) was completely associated with the RiSSQ chromosome marker. Among the 32 females, 20 individuals were co-infected with both RiSSQ and wSSQ (p = 0.076 in Fisher's exact test under the “independent infection” null hypothesis). We also analyzed 16 females and six males of S. squamirostris in Ishikawa Prefecture, ~200 km across the strait from Sado Island (Figure 6 and Table 2). One male specimen was excluded from the analysis because it tested negative for NAD5 (positive control). In the Ishikawa population, only one female was positive for RiSSQ, and two females were positive for wSSQ out of the 15 NAD5 positive specimens. This RiSSQ-infected female was also infected with wSSQ. The infection rates of RiSSQ and wSSQ among the female population were significantly different between Sado and Ishikawa (p = 2.8 × 10−5 and 1.0 × 10−4, respectively, in the Fisher's exact test). Although the amplified gene markers (NAD5, OmpA, DnaA, and wsp genes) of the symbionts were directly sequenced and compared to each assembled reference of RiSSQ and wSSQ from Sado-F01 individuals, no variable nucleotide site was detected.
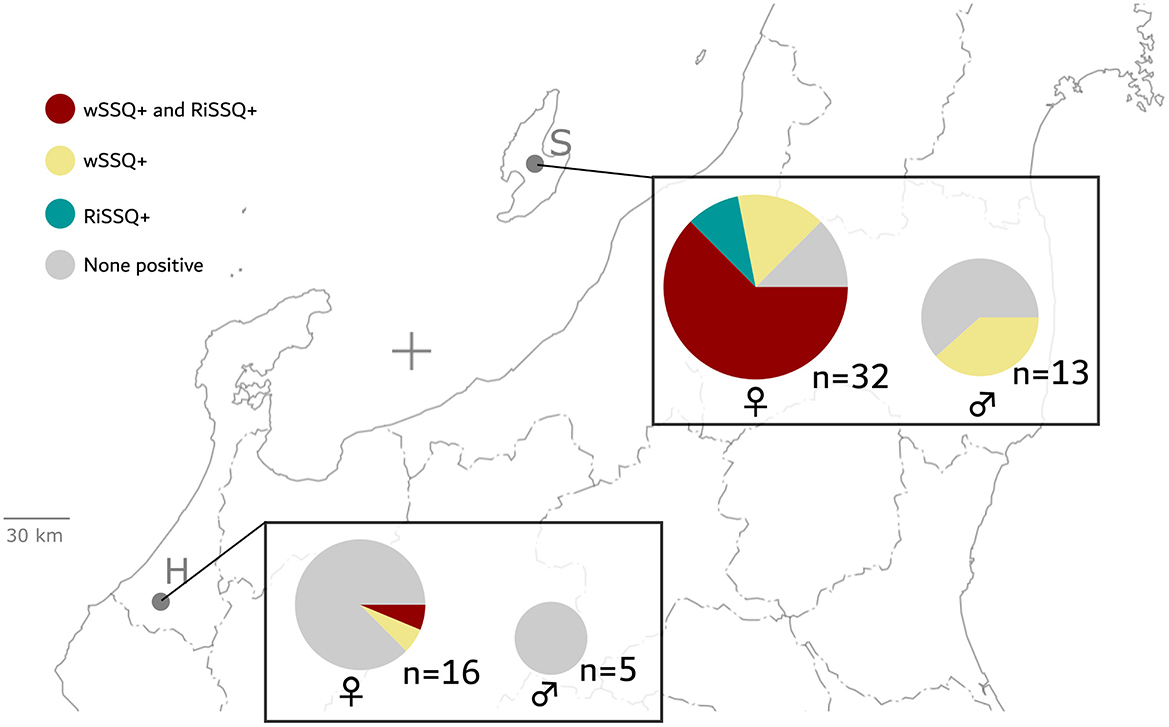
Figure 6. Frequencies of RiSSQ and/or wSSQ infection statuses by sex and population (S: Sado Island, Niigata prefecture or H: Hakusan, Ishikawa Prefecture) in S. squamirostris, based on the results described in Table 2. The background map corresponds to the one in Figure 1.
4 Discussion
In this study, we assembled the closed genomes of two bacteria, RiSSQ and wSSQ, from gDNA obtained from wild-caught S. squamirostris. Both bacteria belong to large taxonomic clades consisting of numerous known endosymbionts of arthropods. RiSSQ is a member of an uncultured group of Rickettsiaceae known as the “Torix group of Rickettsia.” Based on a recent phylogenomic analysis, Davison et al. (2022) admitted apparent distinctness from members of the genus Rickettsia and proposed a new genus “Candidatus Tisiphia,” for this group. The high coverage of the NGS reads of the RiSSQ chromosome and the plasmid, which has helped to assemble the complete genome, indicates a high abundance of this bacteria within an adult female of S. squamirostris. A complete gene set of PPP was found in the RiSSQ genome, which is a distinct metabolic feature of Ca. Tisiphia and Ca. Megaira from the other members of Rickettsiaceae (Pilgrim et al., 2017). Davison et al. (2022) hypothesized that the PPP is an ancestral feature of the main Rickettsia clade, which has been lost in several lineages. RiSSQ has at least one plasmid that is found in all RiSSQ-positive insects in the wild population of S. squamirostris. Ca. Tisiphia in Culicoides impunctatus (RiCimp) contains two plasmids (Davison et al., 2022). Lehman et al. (2024) recently reported that the plasmid pRiCimp001 in RiCimp carries a unique RalF effector of the T4SS. However, this characteristic may not be present in RiSSQ, as no RalF homolog was found in either its plasmid or chromosome. wSSQ belongs to Wolbachia supergroup A, which includes strains associated with CI, MK, and parthenogenesis induction. Although the molecular basis of these reproductive phenotypes has not been fully elucidated, cifA and cifB are considered essential factors for CI in Wolbachia. It is assumed that wSSQ may have lost its CI-inducing ability due to functionally disruptive mutations in the cifA and cifB homologs. Horizontal acquisition and pseudogenization of cif genes are frequently seen in Wolbachia (Martinez et al., 2021). However, it should be noted that Owashi et al. (2024) have recently reported that a Rickettsia strain with only split cifB induces CI in Hemipteran insect, Nesidiocoris tenuis. Thus, crossing experiments are needed to determine the presence or absence of CI in S. squamirostris with wSSQ.
One notable feature of RiSSQ is its high abundance in adult female sand flies. This raises an intriguing question of whether Ca. Tisiphia could interfere with other symbionts, such as Wolbachia, or pathogens co-infecting the same insect. Such potential interaction could have important medical implications for arthropod-borne diseases. However, further investigation is required because our knowledge of this group of bacteria is quite limited. In particular, the modes of transmission and reproductive phenotypes are still unknown for the majority of Ca. Tisiphia members, including RiSSQ. The maternal transmission of Ca. Tisiphia in arthropod hosts has been confirmed, either directly or indirectly, in several cases (Watanabe et al., 2014; Pilgrim et al., 2020; Thongprem et al., 2020). However, maternal transmission alone does not fully account for the maintenance and propagation of symbionts within the host population (Fine, 1975). For this reason, Wolbachia and other endosymbionts that mainly rely on maternal transmission must exhibit their unique phenotypes (either mutualism or reproductive parasitism) to survive in nature. Although Ca. Tisiphia exhibits both paternal and maternal transmission in leafhoppers (Watanabe et al., 2014), the transmission dynamics in S. squamirostris remain unknown. The absence of RiSSQ in adult males also suggests that CI may not be occurring, while other reproductive manipulations such as MK, thelytokous parthenogenesis, or feminization are possible. On the other hand, wSSQ was detected in both male and female hosts. Interestingly, the wSSQ strain also showed that the infection rate was moderately biased to females in the Sado population. It was reported that a Wolbachia strain in Aedes albopictus, wAlbA, showed different density dynamics between females and males, where the infection rapidly becomes undetectable in male adults (Tortosa et al., 2010). Alternatively, such a pattern of moderate bias in infection rate between the sexes could have resulted from a conflict between a sex-distortion (MK, parthenogenesis, or feminization) effect of symbionts and a suppressor gene evolving in the hosts (Hornett et al., 2022). Nevertheless, we will need reliable sex markers or an established colony under laboratory conditions to test these hypotheses.
The draft genome of S. squamirostris showed BUSCO completeness comparable to that of other available genome assemblies of sand fly species. This suggests that obtaining reference-quality assemblies using a wild-caught single insect and a portable nanopore sequencer is a feasible objective. Phylogenetic analysis using >1,000 protein sequences of single-copy orthologs placed S. squamirostris in the basal lineage of Phlebotominae. This result aligns with a recent study by Charamis et al. (2024), which placed Sergentomyia schwetzi as the basal lineage of Phlebotominae based on alignments with 575 single-copy orthologs obtained from transcriptomic analysis. On the other hand, studies using a few gene markers, such as those by Grace-Lema et al. (2015), supported different phylogenetic placements. Expanding the availability of reference genomes for more species within this group of insects will significantly enhance our understanding of their evolution.
5 Conclusion
S. squamirostris is naturally co-infected with Ca. Tisiphia and Wolbachia, offering insights into host–endosymbiont interactions. However, the potential influence of these bacteria on pathogen transmission in sand flies still remains unclear. Further research is required to understand their impact on host ecology and potential implications for disease control.
Data availability statement
Genome assemblies, predicted genes, and annotations of S. squamirostris (GCA042850755.1), RiSSQ (GCA042848025.1), and wSSQ (GCA_042853495.1) were registered in DDBJ and are available through the International Nucleotide Sequence Database Collaboration (INSDC) network under BioProject PRJDB17301. Raw NGS read sequences from the MinION and NextSeq500 analyses are also available under accession no. DRX508768 and DRX508769, respectively.
Ethics statement
The manuscript presents research on animals that do not require ethical approval for their study.
Author contributions
KI: Conceptualization, Investigation, Methodology, Visualization, Writing – original draft, Writing – review & editing. AK: Investigation, Resources, Writing – original draft, Writing – review & editing. DK: Formal analysis, Writing – original draft, Writing – review & editing. MK: Conceptualization, Writing – original draft, Writing – review & editing. KS: Conceptualization, Funding acquisition, Supervision, Writing – original draft, Writing – review & editing. HI: Formal analysis, Writing – original draft, Writing – review & editing. CS: Conceptualization, Funding acquisition, Project administration, Supervision, Writing – original draft, Writing – review & editing.
Funding
The author(s) declare that financial support was received for the research and/or publication of this article. This study was supported by AMED under Grant Number JP21jm0110024h0001 and JSPS KAKENHI under Grant Numbers 19KK0274 and 23K05254.
Acknowledgments
We thank Kanako Minagawa from the University of Tokyo for technical assistance.
Conflict of interest
The authors declare that the research was conducted in the absence of any commercial or financial relationships that could be construed as a potential conflict of interest.
Generative AI statement
The author(s) declare that no Gen AI was used in the creation of this manuscript.
Publisher's note
All claims expressed in this article are solely those of the authors and do not necessarily represent those of their affiliated organizations, or those of the publisher, the editors and the reviewers. Any product that may be evaluated in this article, or claim that may be made by its manufacturer, is not guaranteed or endorsed by the publisher.
Supplementary material
The Supplementary Material for this article can be found online at: https://www.frontiersin.org/articles/10.3389/fmicb.2025.1577636/full#supplementary-material
Abbreviations
CI, cytoplasmic incompatibility; MK, male killing; gDNA, genomic DNA; BUSCO, Benchmarking Universal Single-Copy Ortholog; PPP, pentose phosphate pathway; BAM, binary alignment map.
References
Alanjary, M., Steinke, K., and Ziemert, N. (2019). AutoMLST: an automated web server for generating multi-locus species trees highlighting natural product potential. Nucleic Acids Res. 47, W276–W282. doi: 10.1093/nar/gkz282
Altschul, S. F., Gish, W., Miller, W., Myers, E. W., and Lipman, D. J. (1990). Basic local alignment search tool. J. Mol. Biol. 215, 403–410. doi: 10.1016/S0022-2836(05)80360-2
Aramaki, T., Blanc-Mathieu, R., Endo, H., Ohkubo, K., Kanehisa, M., Goto, S., et al. (2020). KofamKOALA: KEGG Ortholog assignment based on profile HMM and adaptive score threshold. Bioinformatics 36, 2251–2252. doi: 10.1093/bioinformatics/btz859
Baldo, L., Hotopp, J. C. D., Jolley, K. A., Bordenstein, S. R., Biber, S. A., Choudhury, R. R., et al. (2006). Multilocus sequence typing system for the endosymbiont Wolbachia pipientis. Appl. Environ. Microbiol. 72, 7098–7110. doi: 10.1128/AEM.00731-06
Beckmann, J. F., Ronau, J. A., and Hochstrasser, M. (2017). A Wolbachia deubiquitylating enzyme induces cytoplasmic incompatibility. Nat. Microbiol. 2:17007. doi: 10.1038/nmicrobiol.2017.7
Bruna, T., Hoff, K., Lomsadze, A., Stanke, M., and Borodovsky, M. (2020a). BRAKER2: automatic eukaryotic genome annotation with GeneMark-EP+ and AUGUSTUS supported by a protein database. NAR Genom. Bioinform. 3:lqaa108. doi: 10.1093/nargab/lqaa108
Bruna, T., Lomsadze, A., and Borodovsky, M. (2020b). GeneMark-EP+: eukaryotic gene prediction with self-training in the space of genes and proteins. NAR Genom. Bioinform. 2. doi: 10.1093/nargab/lqaa026
Buchfink, B., Xie, C., and Huson, D. H. (2015). Fast and sensitive protein alignment using DIAMOND. Nat. Methods 12, 59–60. doi: 10.1038/nmeth.3176
Capella-Gutiérrez, S., Silla-Martínez, J. M., and Gabaldón, T. (2009). trimAl: a tool for automated alignment trimming in large-scale phylogenetic analyses. Bioinformatics 25, 1972–1973. doi: 10.1093/bioinformatics/btp348
Caragata, E. P., Dutra, H. L. C., Sucupira, P. H. F., Ferreira, A. G. A., and Moreira, L. A. (2021). Wolbachia as translational science: controlling mosquito-borne pathogens. Trends Parasitol. 37, 1050–1067. doi: 10.1016/j.pt.2021.06.007
Chan, P. P., Lin, B. Y., Mak, A. J., and Lowe, T. M. (2021). tRNAscan-SE 2.0: improved detection and functional classification of transfer RNA genes. Nucleic Acids Res. 49, 9077–9096. doi: 10.1093/nar/gkab688
Charamis, J., Balaska, S., Ioannidis, P., Dvorák, V., Mavridis, K., McDowell, M. A., et al. (2024). Comparative genomics uncovers the evolutionary dynamics of detoxification and insecticide target genes across 11 phlebotomine sand flies. Genome Biol. Evol. 16:evae186. doi: 10.1093/gbe/evae186
Chen, S., Zhou, Y., Chen, Y., and Gu, J. (2018). fastp: an ultra-fast all-in-one FASTQ preprocessor. Bioinformatics 34, i884–i890. doi: 10.1093/bioinformatics/bty560
Cui, L., Chang, S. H., Strickman, D., and Rowton, E. (1999). Frequency of Wolbachia infection in laboratory and field sand fly (Diptera: Psychodidae) populations. J. Am. Mosq. Control Assoc. 15, 571–572.
Cumsille, A., Durán, R. E., Rodríguez-Delherbe, A., Saona-Urmeneta, V., Cámara, B., Seeger, M., et al. (2023). GenoVi, an open-source automated circular genome visualizer for bacteria and archaea. PLoS Comput. Biol. 19:e1010998. doi: 10.1371/journal.pcbi.1010998
Davison, H. R., Pilgrim, J., Wybouw, N., Parker, J., Pirro, S., Hunter-Barnett, S., et al. (2022). Genomic diversity across the Rickettsia and “Candidatus Megaira” genera and proposal of genus status for the Torix group. Nat. Commun. 13:2630. doi: 10.1038/s41467-022-30385-6
Dyková, I., Veverková, M., Fiala, I., Machácková, B., and Pecková, H. (2003). Nuclearia pattersoni sp. n. (Filosea), a new species of amphizoic amoeba isolated from gills of roach (Rutilus rutilus), and its rickettsial endosymbiont. Folia Parasitol. 50, 161–170. doi: 10.14411/fp.2003.030
Eddy, S. R. (2011). Accelerated profile HMM Searches. PLoS Comput. Biol. 7:e1002195. doi: 10.1371/journal.pcbi.1002195
Eren, A. M., Kiefl, E., Shaiber, A., Veseli, I., Miller, S. E., Schechter, M. S., et al. (2021). Community-led, integrated, reproducible multi-omics with anvi'o. Nat Microbiol 6, 3–6. doi: 10.1038/s41564-020-00834-3
Feldbauer, R., Gosch, L., Lüftinger, L., Hyden, P., Flexer, A., and Rattei, T. (2021). DeepNOG: fast and accurate protein orthologous group assignment. Bioinformatics 36, 5304–5312. doi: 10.1093/bioinformatics/btaa1051
Feng, L. C., and Chao, C. S. (1943). The development of Trypanosoma bocagei in phlebotomus squamirostris. Chin. Med. J. 62, 210–217.
Fine, P. E. (1975). Vectors and vertical transmission: an epidemiologic perspective. Ann. N. Y. Acad. Sci. 266, 173–194. doi: 10.1111/j.1749-6632.1975.tb35099.x
Flynn, J. M., Hubley, R., Goubert, C., Rosen, J., Clark, A. G., Feschotte, C., et al. (2020). RepeatModeler2 for automated genomic discovery of transposable element families. Proc. Natl. Acad. Sci. U. S. A. 117, 9451–9457. doi: 10.1073/pnas.1921046117
Foster, J., Ganatra, M., Kamal, I., Ware, J., Makarova, K., Ivanova, N., et al. (2005). The Wolbachia genome of Brugia malayi: endosymbiont evolution within a human pathogenic nematode. PLoS Biol. 3:e121. doi: 10.1371/journal.pbio.0030121
Giorgini, M., Bernardo, U., Monti, M. M., Nappo, A. G., and Gebiola, M. (2010). Rickettsia symbionts cause parthenogenetic reproduction in the parasitoid wasp Pnigalio soemius (Hymenoptera: Eulophidae). Appl. Environ. Microbiol. 76, 2589–2599. doi: 10.1128/AEM.03154-09
Gotoh, O. (2008). A space-efficient and accurate method for mapping and aligning cDNA sequences onto genomic sequence. Nucleic Acids Res. 36, 2630–2638. doi: 10.1093/nar/gkn105
Grace-Lema, D. M., Yared, S., Quitadamo, A., Janies, D. A., Wheeler, W. C., Balkew, M., et al. (2015). A phylogeny of sand flies (Diptera: Psychodidae: Phlebotominae), using recent Ethiopian collections and a broad selection of publicly available DNA sequence data. Syst. Entomol. 40, 733–744. doi: 10.1111/syen.12135
Guan, D., McCarthy, S. A., Wood, J., Howe, K., Wang, Y., and Durbin, R. (2020). Identifying and removing haplotypic duplication in primary genome assemblies. Bioinformatics 36, 2896–2898. doi: 10.1093/bioinformatics/btaa025
Hoang, D. T., Chernomor, O., von Haeseler, A., Minh, B. Q., and Vinh, L. S. (2018). UFBoot2: improving the ultrafast bootstrap approximation. Mol. Biol. Evol. 35, 518–522. doi: 10.1093/molbev/msx281
Hoff, K. J., Lange, S., Lomsadze, A., Borodovsky, M., and Stanke, M. (2016). BRAKER1: unsupervised RNA-Seq-based genome annotation with GeneMark-ET and AUGUSTUS. Bioinformatics 32, 767–769. doi: 10.1093/bioinformatics/btv661
Hoff, K. J., Lomsadze, A., Borodovsky, M., and Stanke, M. (2019). Whole-genome annotation with BRAKER. Methods Mol. Biol. 1962, 65–95. doi: 10.1007/978-1-4939-9173-0_5
Hoffmann, A. A., Montgomery, B. L., Popovici, J., Iturbe-Ormaetxe, I., Johnson, P. H., Muzzi, F., et al. (2011). Successful establishment of Wolbachia in Aedes populations to suppress dengue transmission. Nature 476, 454–457. doi: 10.1038/nature10356
Hornett, E. A., Kageyama, D., and Hurst, G. D. D. (2022). Sex determination systems as the interface between male-killing bacteria and their hosts. Proc. Biol. Sci. 289:20212781. doi: 10.1098/rspb.2021.2781
Hosokawa, T., Koga, R., Kikuchi, Y., Meng, X. Y., and Fukatsu, T. (2010). Wolbachia as a bacteriocyte-associated nutritional mutualist. Proc. Natl. Acad. Sci. U. S. A. 107, 769–774. doi: 10.1073/pnas.0911476107
Hu, J., Fan, J., Sun, Z., and Liu, S. (2020). NextPolish: a fast and efficient genome polishing tool for long-read assembly. Bioinformatics 36, 2253–2255. doi: 10.1093/bioinformatics/btz891
Huang, M., Kingan, S., Shoue, D., Nguyen, O., Froenicke, L., Galvin, B., et al. (2024). Improved high quality sand fly assemblies enabled by ultra low input long read sequencing. Sci. Data 11:918. doi: 10.1038/s41597-024-03628-y
Hurst, G. D. D., and Frost, C. L. (2015). Reproductive parasitism: maternally inherited symbionts in a biparental world. Cold Spring Harb. Perspect. Biol. 7:a017699. doi: 10.1101/cshperspect.a017699
Iwata, H., and Gotoh, O. (2012). Benchmarking spliced alignment programs including Spaln2, an extended version of Spaln that incorporates additional species-specific features. Nucleic Acids Res. 40:e161. doi: 10.1093/nar/gks708
Kalyaanamoorthy, S., Minh, B. Q., Wong, T. K. F., von Haeseler, A., and Jermiin, L. S. (2017). ModelFinder: fast model selection for accurate phylogenetic estimates. Nat. Methods 14, 587–589. doi: 10.1038/nmeth.4285
Katoh, K., and Standley, D. M. (2013). MAFFT multiple sequence alignment software version 7: improvements in performance and usability. Mol. Biol. Evol. 30, 772–780. doi: 10.1093/molbev/mst010
Kikuchi, Y., Sameshima, S., Kitade, O., Kojima, J., and Fukatsu, T. (2002). Novel clade of Rickettsia spp. from leeches. Appl. Environ. Microbiol. 68, 999–1004. doi: 10.1128/AEM.68.2.999-1004.2002
Kim, D., Song, L., Breitwieser, F. P., and Salzberg, S. L. (2016). Centrifuge: rapid and sensitive classification of metagenomic sequences. Genome Res. 26, 1721–1729. doi: 10.1101/gr.210641.116
Kolmogorov, M., Bickhart, D. M., Behsaz, B., Gurevich, A., Rayko, M., Shin, S. B., et al. (2020). metaFlye: scalable long-read metagenome assembly using repeat graphs. Nat. Methods 17, 1103–1110. doi: 10.1038/s41592-020-00971-x
Kolmogorov, M., Yuan, J., Lin, Y., and Pevzner, P. A. (2019). Assembly of long, error-prone reads using repeat graphs. Nat. Biotechnol. 37, 540–546. doi: 10.1038/s41587-019-0072-8
Krzywinski, M., Schein, J., Birol, I., Connors, J., Gascoyne, R., Horsman, D., et al. (2009). Circos: an information aesthetic for comparative genomics. Genome Res. 19, 1639–1645. doi: 10.1101/gr.092759.109
Kuznetsov, D., Tegenfeldt, F., Manni, M., Seppey, M., Berkeley, M., Kriventseva, E. V., et al. (2023). OrthoDB v11: annotation of orthologs in the widest sampling of organismal diversity. Nucleic Acids Res. 51, D445–D451. doi: 10.1093/nar/gkac998
Lanfear, R., Calcott, B., Ho, S. Y. W., and Guindon, S. (2012). Partitionfinder: combined selection of partitioning schemes and substitution models for phylogenetic analyses. Mol. Biol. Evol. 29, 1695–1701. doi: 10.1093/molbev/mss020
Lawson, E. T., Mousseau, T. A., Klaper, R., Hunter, M. D., and Werren, J. H. (2001). Rickettsia associated with male-killing in a buprestid beetle. Heredity 86, 497–505. doi: 10.1046/j.1365-2540.2001.00848.x
Lehman, S. S., Verhoeve, V. I., Driscoll, T. P., Beckmann, J. F., and Gillespie, J. J. (2024). Metagenome diversity illuminates the origins of pathogen effectors. MBio 15:e0075923. doi: 10.1128/mbio.00759-23
LePage, D. P., Metcalf, J. A., Bordenstein, S. R., On, J., Perlmutter, J. I., Shropshire, J. D., et al. (2017). Prophage WO genes recapitulate and enhance Wolbachia-induced cytoplasmic incompatibility. Nature 543, 243–247. doi: 10.1038/nature21391
Lewis, D. J. (1987). Phlebotomine sandflies (Diptera: Psychodidae) from the Oriental Region. Syst. Entomol. 12, 163–180. doi: 10.1111/j.1365-3113.1987.tb00194.x
Li, D., Liu, C. M., Luo, R., Sadakane, K., and Lam, T. W. (2015). MEGAHIT: an ultra-fast single-node solution for large and complex metagenomics assembly via succinct de Bruijn graph. Bioinformatics 31, 1674–1676. doi: 10.1093/bioinformatics/btv033
Li, H. (2018). Minimap2: pairwise alignment for nucleotide sequences. Bioinformatics 34, 3094–3100. doi: 10.1093/bioinformatics/bty191
Li, H., Handsaker, B., Wysoker, A., Fennell, T., Ruan, J., Homer, N., et al. (2009). The sequence alignment/map format and SAMtools. Bioinformatics 25, 2078–2079. doi: 10.1093/bioinformatics/btp352
Li, K., Chen, H., Jiang, J., Li, X., Xu, J., and Ma, Y. (2016). Diversity of bacteriome associated with Phlebotomus chinensis (Diptera: Psychodidae) sand flies in two wild populations from China. Sci. Rep. 6:36406. doi: 10.1038/srep36406
Lindsey, A. R. I., Rice, D. W., Bordenstein, S. R., Brooks, A. W., Bordenstein, S. R., and Newton, I. L. G. (2018). Evolutionary genetics of cytoplasmic incompatibility genes cifA and cifB in prophage WO of Wolbachia. Genome Biol. Evol. 10, 434–451. doi: 10.1093/gbe/evy012
Lomsadze, A., Ter-Hovhannisyan, V., Chernoff, Y. O., and Borodovsky, M. (2005). Gene identification in novel eukaryotic genomes by self-training algorithm. Nucleic Acids Res. 33, 6494–6506. doi: 10.1093/nar/gki937
Lozano-Sardaneta, Y. N., Marina, C. F., Torres-Monzón, J. A., Sánchez-Cordero, V., and Becker, I. (2023). Molecular detection of Wolbachia and Bartonella as part of the microbiome of phlebotomine sand flies from Chiapas, Mexico. Parasitol. Res. 122, 1293–1301. doi: 10.1007/s00436-023-07829-z
Lozano-Sardaneta, Y. N., Valderrama, A., Sánchez-Montes, S., Grostieta, E., Colunga-Salas, P., Sánchez-Cordero, V., et al. (2021). Rickettsial agents detected in the genus Psathyromyia (Diptera: Phlebotominae) from a biosphere reserve of Veracruz, Mexico. Parasitol. Int. 82, 102286. doi: 10.1016/j.parint.2021.102286
Manni, M., Berkeley, M. R., Seppey, M., Simão, F. A., and Zdobnov, E. M. (2021). BUSCO update: novel and streamlined workflows along with broader and deeper phylogenetic coverage for scoring of eukaryotic, prokaryotic, and viral genomes. Mol. Biol. Evol. 38, 4647–4654. doi: 10.1093/molbev/msab199
Martinez, J., Klasson, L., Welch, J. J., and Jiggins, F. M. (2021). Life and death of selfish genes: comparative genomics reveals the dynamic evolution of cytoplasmic incompatibility. Mol. Biol. Evol. 38, 2–15. doi: 10.1093/molbev/msaa209
Meng, G., Li, Y., Yang, C., and Liu, S. (2019). MitoZ: a toolkit for animal mitochondrial genome assembly, annotation and visualization. Nucleic Acids Res. 47:e63. doi: 10.1093/nar/gkz173
Minh, B. Q., Schmidt, H. A., Chernomor, O., Schrempf, D., Woodhams, M. D., von Haeseler, A., et al. (2020). IQ-TREE 2: new models and efficient methods for phylogenetic inference in the genomic era. Mol. Biol. Evol. 37, 1530–1534. doi: 10.1093/molbev/msaa015
Newstead, R. (1923). On a new species of Phlebotomus from Japan. Ann. Trop. Med. Parasitol. 17, 531–532. doi: 10.1080/00034983.1923.11684374
Nikoh, N., Hosokawa, T., Moriyama, M., Oshima, K., Hattori, M., and Fukatsu, T. (2014). Evolutionary origin of insect-Wolbachia nutritional mutualism. Proc. Natl. Acad. Sci. U. S. A. 111, 10257–10262. doi: 10.1073/pnas.1409284111
Ono, M., Braig, H. R., Munstermann, L. E., Ferro, C., and O'Neill, S. L. (2001). Wolbachia infections of phlebotomine sand flies (Diptera: Psychodidae). J. Med. Entomol. 38, 237–241. doi: 10.1603/0022-2585-38.2.237
Owashi, Y., Arai, H., Adachi-Hagimori, T., and Kageyama, D. (2024). Rickettsia induces strong cytoplasmic incompatibility in a predatory insect. Proc. Biol. Sci. 291:20240680. doi: 10.1098/rspb.2024.0680
Parks, D. H., Imelfort, M., Skennerton, C. T., Hugenholtz, P., and Tyson, G. W. (2015). CheckM: assessing the quality of microbial genomes recovered from isolates, single cells, and metagenomes. Genome Res. 25, 1043–1055. doi: 10.1101/gr.186072.114
Pilgrim, J., Ander, M., Garros, C., Baylis, M., Hurst, G. D. D., and Siozios, S. (2017). Torix group Rickettsia are widespread in Culicoides biting midges (Diptera: Ceratopogonidae), reach high frequency and carry unique genomic features. Environ. Microbiol. 19, 4238–4255. doi: 10.1111/1462-2920.13887
Pilgrim, J., Siozios, S., Baylis, M., and Hurst, G. D. D. (2020). Tissue tropisms and transstadial transmission of a Rickettsia endosymbiont in the highland midge, Culicoides impunctatus (Diptera: Ceratopogonidae). Appl. Environ. Microbiol. 86. doi: 10.1128/AEM.01492-20
Pilgrim, J., Thongprem, P., Davison, H. R., Siozios, S., Baylis, M., Zakharov, E. V., et al. (2021). Torix Rickettsia are widespread in arthropods and reflect a neglected symbiosis. Gigascience 10:giab021. doi: 10.1093/gigascience/giab021
R Development Core Team (2014). R: A Language and Environment for Statistical Computing, version 4.2.2. Vienna: R Foundation for Statistical Computing. Available onine at: http://www.r-project.org (accessed October 31, 2022).
Reeves, W. K., Kato, C. Y., and Gilchriest, T. (2008). Pathogen screening and bionomics of Lutzomyia apache (Diptera: Psychodidae) in Wyoming, USA. J. Am. Mosq. Control Assoc. 24, 444–447. doi: 10.2987/5745.1
Rosário, A. A. do, Dias-Lima, A. G., Lambert, S. M., Souza, B. M. P. da S., and Bravo, F. (2022). Identification and molecular characterization of Wolbachia strains and natural infection for Leishmania sp. in neotropical Phlebotominae (Diptera: Psychodidae) species, leishmaniasis vectors. Acta Trop. 235:106624. doi: 10.1016/j.actatropica.2022.106624
Sanjoba, C., and Miyagi, I. (2022). A new species of the genus Sergentomyia França and Parrot (Diptera: Psychodidae) from Iriomote Island, Ryukyu Archipelago, Japan. ???? 73, 125–129. doi: 10.7601/mez.73.125
Sanjoba, C., Zbel, Y., Asada, M., Osada, Y., Gantuya, S., and Matsumoto, Y. (2011). Recent collections of Sergentomyia squamirostris (Diptera: Psychodidae) in Japan, with descriptions and illustrations. Med. Entomol. Zool. 62, 71–77. doi: 10.7601/mez.62.71
Schrallhammer, M., Ferrantini, F., Vannini, C., Galati, S., Schweikert, M., Görtz, H.-D., et al. (2013). “Candidatus Megaira polyxenophila” gen. nov., sp. nov.: considerations on evolutionary history, host range and shift of early divergent rickettsiae. PLoS ONE 8:e72581. doi: 10.1371/journal.pone.0072581
Shen, W., Le, S., Li, Y., and Hu, F. (2016). SeqKit: a cross-platform and ultrafast toolkit for FASTA/Q file manipulation. PLoS ONE 11:e0163962. doi: 10.1371/journal.pone.0163962
Shropshire, J. D., and Bordenstein, S. R. (2019). Two-By-One model of cytoplasmic incompatibility: synthetic recapitulation by transgenic expression of cifA and cifB in Drosophila. PLoS Genet. 15:e1008221. doi: 10.1371/journal.pgen.1008221
Shropshire, J. D., On, J., Layton, E. M., Zhou, H., and Bordenstein, S. R. (2018). One prophage WO gene rescues cytoplasmic incompatibility in Drosophila melanogaster. Proc. Natl. Acad. Sci. U. S. A. 115, 4987–4991. doi: 10.1073/pnas.1800650115
Stanke, M., Diekhans, M., Baertsch, R., and Haussler, D. (2008). Using native and syntenically mapped cDNA alignments to improve de novo gene finding. Bioinformatics 24, 637–644. doi: 10.1093/bioinformatics/btn013
Stanke, M., Schöffmann, O., Morgenstern, B., and Waack, S. (2006). Gene prediction in eukaryotes with a generalized hidden Markov model that uses hints from external sources. BMC Bioinformatics 7:62. doi: 10.1186/1471-2105-7-62
Tanizawa, Y., Fujisawa, T., and Nakamura, Y. (2018). DFAST: a flexible prokaryotic genome annotation pipeline for faster genome publication. Bioinformatics 34, 1037–1039. doi: 10.1093/bioinformatics/btx713
Tarailo-Graovac, M., and Chen, N. (2009). Using RepeatMasker to identify repetitive elements in genomic sequences. Curr. Protoc. Bioinformatics Chapter 4, 4.10.1–4.10.14. doi: 10.1002/0471250953.bi0410s25
Thongprem, P., Evison, S. E. F., Hurst, G. D. D., and Otti, O. (2020). Transmission, tropism, and biological impacts of torix Rickettsia in the common bed bug Cimex lectularius (Hemiptera: Cimicidae). Front. Microbiol. 11:608763. doi: 10.3389/fmicb.2020.608763
Tortosa, P., Charlat, S., Labbé, P., Dehecq, J.-S., Barré, H., and Weill, M. (2010). Wolbachia age-sex-specific density in Aedes albopictus: a host evolutionary response to cytoplasmic incompatibility? PLoS ONE 5:e9700. doi: 10.1371/journal.pone.0009700
Veseli, I., Chen, Y. T., Schechter, M. S., Vanni, C., Fogarty, E. C., Watson, A. R., et al. (2023). Microbes with higher metabolic independence are enriched in human gut microbiomes under stress. eLife. doi: 10.7554/eLife.89862.1
Walker, T., Johnson, P. H., Moreira, L. A., Iturbe-Ormaetxe, I., Frentiu, F. D., McMeniman, C. J., et al. (2011). The wMel Wolbachia strain blocks dengue and invades caged Aedes aegypti populations. Nature 476, 450–453. doi: 10.1038/nature10355
Wang, J., Gou, Q.-Y., Luo, G.-Y., Hou, X., Liang, G., and Shi, M. (2022). Total RNA sequencing of Phlebotomus chinensis sandflies in China revealed viral, bacterial, and eukaryotic microbes potentially pathogenic to humans. Emerg Microbes Infect. 11, 2080–2092. doi: 10.1080/22221751.2022.2109516
Watanabe, K., Yukuhiro, F., Matsuura, Y., Fukatsu, T., and Noda, H. (2014). Intrasperm vertical symbiont transmission. Proc. Natl. Acad. Sci. U. S. A. 111, 7433–7437. doi: 10.1073/pnas.1402476111
Werren, J. H., Hurst, G. D., Zhang, W., Breeuwer, J. A., Stouthamer, R., and Majerus, M. E. (1994). Rickettsial relative associated with male killing in the ladybird beetle (Adalia bipunctata). J. Bacteriol. 176, 388–394. doi: 10.1128/jb.176.2.388-394.1994
Wick, R. R., and Holt, K. E. (2022). Polypolish: short-read polishing of long-read bacterial genome assemblies. PLoS Comput. Biol. 18:e1009802. doi: 10.1371/journal.pcbi.1009802
Wishart, D. S., Han, S., Saha, S., Oler, E., Peters, H., Grant, J. R., et al. (2023). PHASTEST: faster than PHASTER, better than PHAST. Nucleic Acids Res. 51, W443–W450. doi: 10.1093/nar/gkad382
Keywords: sand fly, Wolbachia, Candidatus tisiphia, symbionts, genome
Citation: Itokawa K, Kuroki A, Kobayashi D, Kuroda M, Sawabe K, Isawa H and Sanjoba C (2025) Genomic analysis of co-infection with Wolbachia and Candidatus Tisiphia in the sand fly Sergentomyia squamirostris. Front. Microbiol. 16:1577636. doi: 10.3389/fmicb.2025.1577636
Received: 21 February 2025; Accepted: 07 April 2025;
Published: 09 May 2025.
Edited by:
Takema Fukatsu, National Institute of Advanced Industrial Science and Technology (AIST), JapanReviewed by:
Xiao-Li Bing, Nanjing Agricultural University, ChinaHiroshi Arai, University of Liverpool, United Kingdom
Copyright © 2025 Itokawa, Kuroki, Kobayashi, Kuroda, Sawabe, Isawa and Sanjoba. This is an open-access article distributed under the terms of the Creative Commons Attribution License (CC BY). The use, distribution or reproduction in other forums is permitted, provided the original author(s) and the copyright owner(s) are credited and that the original publication in this journal is cited, in accordance with accepted academic practice. No use, distribution or reproduction is permitted which does not comply with these terms.
*Correspondence: Chizu Sanjoba, YXNhbmpvYmFAZy5lY2MudS10b2t5by5hYy5qcA==