- 1College of Biological Sciences and Technology, Beijing Forestry University, Beijing, China
- 2National Engineering Research Center of Tree Breeding and Ecological Restoration, Beijing Forestry University, Beijing, China
Phytoremediation offers a promising strategy for addressing the global challenge of land desertification. In the Mu Us Sandy Land of China, Artemisia ordosica Krasch. has emerged as a key species for desertification control. Its root-associated microbial communities may enhance the plant’s adaptability to sandy, nutrient-poor environments. Despite their ecological significance, comprehensive investigations of these microbial communities remain limited. In this study, microbial communities in the root zone (i.e., rhizosphere soil, non-rhizosphere soil, and root endosphere) of A. ordosica were analyzed via high-throughput sequencing and different isolation approaches across successional stages (moving dunes, semi-fixed dunes, and fixed dunes) in the Mu Us Sandy Land of northern China. Metagenomic analysis revealed that microbial diversity was significantly higher in the rhizosphere and non-rhizosphere soils than in the root endosphere; moving dunes exhibited lower diversity than semi-fixed and fixed dunes. Meanwhile, distinct microbial community structures across successional stages were revealed by principal coordinates analysis (PCoA), demonstrating substantial differences between the root endosphere and other zones. Environmental factors, including nitrate nitrogen (NO3−-N), organic matter (OM), available potassium (AK), and total potassium (TK), significantly influenced microbial community composition. Moreover, dominant genera such as Arthrobacter and Paraphoma were identified, potentially contributing to A. ordosica growth. From a culturomics perspective, 93 bacterial isolates were obtained using conventional streak plate and colony pick methods, with Firmicutes (37.63%) and Bacillus (23.66%) identified as the dominant taxa. In parallel, 14 fungal strains were isolated, primarily belonging to Penicillium (35.71%) and Aspergillus (21.43%), both of which are well-documented for their stress tolerance in arid ecosystems. A high-throughput cultivation and identification method, tailored to recover rare and slow-growing bacteria, was employed and successfully broadened the cultured diversity to include Proteobacteria (46.43%) and representatives of the rarely cultivated Deinococcus-Thermus phylum. This study provides metagenomic with culturomics insights into the microbial communities associated with A. ordosica, enhancing the understanding of plant–microbe interactions in sandy land ecosystems.
1 Introduction
Land desertification is among the most pressing global environmental challenges, with reports indicating increasing negative ecological and socioeconomic impacts worldwide (Huang et al., 2016). China is one of the countries most severely affected, with desertified land comprising 17.93% of its total area. However, its aggressive anti-desertification policies are designed to achieve a reversal of desertification by 2030 (Xu and Zhang, 2021). Phytoremediation is considered an environmentally sustainable approach to restoring land affected by desertification (Poria et al., 2022). Artemisia ordosica Krasch., a psammophytic shrub species, is widely utilized in desertification control, particularly through aerial seed dispersal (Qi, 1998). As the dominant species of the most widely distributed psammophytic plant community in the Mu Us Sandy Land (MUSL) of northern China, it effectively reduces wind erosion and contributes to sand stabilization, thereby supporting desertification restoration efforts in China (Zhang, 1994; Pang et al., 2022). Artemisia ordosica thrives in nutrient-poor sandy soils and may recruit specific microbial communities that promote growth and enhance stress resistance (Brisbin et al., 2022; Schmitz et al., 2022; Acuna et al., 2023). However, most research concerning plant-associated microbial recruitment has focused on cash crops, food crops, and model plants; no studies have been conducted on A. ordosica (Wang et al., 2020; Biget et al., 2021; Upadhyay et al., 2022). Consequently, the composition of microbial communities recruited by A. ordosica at different successional stages in MUSL remains unknown.
High-throughput sequencing technology has enabled the identification of uncultured microorganisms and assessment of microbial diversity in environmental samples (Bartram et al., 2011; Caporaso et al., 2012). Briefly, these methods involve the isolation and amplification of DNA from samples, the generation of large numbers of base sequences through chemical catalysis and other processes, and the subsequent analysis and processing of sequence data using computational tools to obtain biomolecular sequence information (Reuter et al., 2015). Due to its high efficiency and accuracy, high-throughput sequencing has become an essential research tool in molecular ecology (Hu et al., 2021; Liu et al., 2021). In this study, the root endosphere, rhizosphere, and non-rhizosphere microbiomes of A. ordosica growing in sandy soils at different successional stages in MUSL were systematically investigated via high-throughput sequencing technology. First, the structure and composition of microbial communities in the A. ordosica endosphere, rhizosphere, and non-rhizosphere were examined. Second, differences in these microbial communities across sandy soils at various successional stages in MUSL and the key drivers of such variations were analyzed. The findings provided a detailed understanding of the complex bacterial communities associated with sandy land plants, which may be critical for the continued application of beneficial bacteria in sandy land phytoremediation. However, these sequencing methods generate only statistical information regarding sample microbial communities and do not facilitate the cultivation of microorganisms, making in-depth research challenging. To address this limitation, culturable microorganisms from A. ordosica root zone samples were isolated.
The conventional streak plate method is widely used to culture microorganisms by artificially supplying the nutrients required for growth (Felsenfeld et al., 1951; Wesley et al., 1983). This method is easy to perform and remains indispensable in microbiological research. However, it has several limitations, including an inability to fully replicate actual environmental conditions during cultivation and the challenge of preventing microbial competition, which impedes the growth of slow-growing or oligotrophic species (Lewis et al., 2021). Accordingly, only a small percentage of microbes can be cultivated (Hofer, 2018). Additionally, the streak plate method is time-consuming, requires substantial material resources, and has low efficiency. In 2021, a high-throughput cultivation and identification method was introduced, combining high-throughput sequencing with microbial culture (Zhang et al., 2021). This method involves the even distribution of limiting dilution cultures across the wells of 96-well cell culture plates, maximizing the probability of obtaining single-well pure cultures High-throughput sequencing is then used to identify the resulting cultures, preventing microbial competition during cultivation and yielding a more diverse collection of microbial isolates and particularly facilitating the isolation of rare and slow-growing bacterial strains. This approach previously facilitated the isolation of 276 bacterial strains, including 62 strains of bacilli (Xia et al., 2024). To maximize culture recovery and retain diverse microbial resources, two methods were utilized in this study to isolate culturable microorganisms from the A. ordosica root zone: the high-throughput cultivation and identification method and the streak plate method.
High-throughput sequencing combined with microbial culture was used to analyze microbial community structure in the root zone of A. ordosica at different successional stages in MUSL. Both macro-level community composition and the taxonomic distribution of culturable microbial resources were examined. Culture resources were separated and preserved, establishing a foundation for future research on microbial functions and molecular mechanisms of action. The advantages of the high-throughput cultivation and identification method were also assessed, providing support for the broader application of this advanced culture technology.
2 Methods
2.1 Collection and storage of root zone samples
Samples were collected from a site in the Mu Us Sandy Land (MUSL) in Yanchi, Ningxia, China (37°44′N; 107°24′E), which covers approximately 39,385 km2 and constitutes 3.6% of China’s total sand region. The site has a continental monsoon climate, with an average annual temperature of 6°C–10°C, annual precipitation of 530 mm, and an average altitude of 2,754 m. Sampling was conducted at the end of October 2022 across three different vegetation successional stages in MUSL (Supplementary Figure 1): moving dunes (C), semi-fixed dunes (SS), and fixed dunes (FS). In each sampling area, three quadrats measuring 10 m × 10 m were established. Artemisia ordosica was uniformly distributed within each quadrat, with a minimum spacing of 10 m between individual plants. A five-point sampling strategy was utilized to collect root endosphere (O) samples (diameter < 2 mm), rhizosphere soil (R), and non-rhizosphere soil (F) from A. ordosica plants at a depth of 30 cm. Collection was performed via previously described methods (Beckers et al., 2017; Xiao et al., 2017). In total, 54 samples (3 succession types × 3 root location types × 6 biological replicates) were obtained (Table 1). Soil samples were passed through a 2-mm sieve and divided into two subsamples: one stored at −20°C for microbial analysis and the other used for physical and chemical property assessments.
2.2 Determination of soil physical and chemical properties
Soil pH was measured using a pH meter. Total nitrogen (TN), total phosphorus (TP), ammonium nitrogen (NH4+-N), and available phosphorus (AP) were quantified using a fully automated chemical analyzer (Smartchem 200; AMS Alliance, Rome, Italy). Nitrate nitrogen (NO3−-N) content was determined by UV–Vis spectrophotometry (Navone, 1964). Soil organic matter (OM) content was measured using the volumetric method (Walkley and Black, 1934). Total potassium (TK) was quantified by atomic absorption spectrophotometry, and available potassium (AK) was determined by flame photometry (Isaac and Kerber, 1971).
2.3 DNA extraction and sequencing
Genomic DNA was extracted from a total of nine root samples, nine rhizosphere soil samples, and nine non-rhizosphere soil samples using the cetyltrimethylammonium bromide method and stored at −80°C. The bacterial 16S rRNA V3–V4 region was amplified by polymerase chain reaction (PCR) using the forward primer 341F (5′-ACTCCTACGGGAGGCAGCAG-3′) and the reverse primer 806R (5′-GACTACHVGGGTATCTAATCC-3′) (Yergeau et al., 2015). The fungal internal transcribed spacer 1 (ITS1) region was amplified by PCR using the forward primer ITS5-1737F (5′-GGAAGTAAAAGTCGTAACAAGG-3′) and the reverse primer ITS2-2043R (5′-GCTGCGTTCTTCATCGATGC-3′) (Liu et al., 2017). PCR amplification and sequencing were performed by Shanghai Majorbio Bio-pharm Technology Co., Ltd. using the Illumina MiSeq PE300 platform for high-throughput sequencing (Illumina, San Diego, CA, United States).
2.4 Molecular biological analysis and statistical analysis
Paired-end reads from Illumina sequencing were first processed through quality control steps: raw sequences were trimmed using a sliding window approach (10 bp window size, truncating reads from window start positions if average Q-score < 20) and filtered to discard reads shorter than 50 bp. Subsequently, reads were assembled via overlapping regions using FLASH software (Magoc and Salzberg, 2011) with a minimum overlap of 10 bp and maximum mismatch tolerance rate of 0.2. The QIIME system (Caporaso et al., 2010) was then employed to demultiplex sequences based on exact barcode matches (zero mismatches) and primers (≤2 mismatches), followed by chimera removal using UCHIME (Haas et al., 2011). Clean reads were clustered into operational taxonomic units (OTUs) at 97% sequence similarity using UPARSE, based on the SILVA132 (Quast et al., 2013) and UNITE (Abarenkov et al., 2010) databases. The OTU table was randomly resampled to match the sequencing depth of the sample with the lowest read count, ensuring uniform sequencing depth across all samples. The resampled OTU table was then used for further analysis. Data were organized using Excel 2010 (Microsoft, Redmond, WA, United States). Soil physicochemical parameters were analyzed by one-way analysis of variance (ANOVA) with Tukey’s test in SPSS (version 19.0; SPSS, Chicago, IL, United States). Alpha diversity (Shannon index) was calculated using R1 with vegan package; the relative abundances of OTUs at the phylum and genus levels were examined using R with dplyr package. Plots were generated using GraphPad Prism 9.0.0 (GraphPad Software, San Diego, CA, USA). The Bray-Curtis distance was selected as the metric to explore variations in microbial community structure using principal coordinates analysis (PCoA). The online TUTU analysis platform2 was used to visualize raw data tables or abundance data tables using its built-in algorithms. Based on the user’s selected analysis method, it processes and visualizes the data, such as performing PCoA analysis using the Bray-Curtis test. While visualizing the data, the TUTU platform can also provide data tables related to the result graphs for some analyses. For example, during heatmap analysis, it can provide tables of relative abundance and average abundance for users to analyze. A Venn diagram was generated to illustrate genera shared between groups, regardless of their relative abundances. Relationships between environmental conditions and microbial community structure were examined using the Mantel test in R using linkET package, ggplot2 package and dplyr package. Co-occurrence network analysis was performed in R using the igraph package; data were filtered using a cutoff value of 0.65 and an absolute r value of 0.65 (p < 0.05). Gephi v. 0.9.7 was used to visualize networks (Bastian et al., 2009). Relationships between soil environmental parameters and microbial community composition were assessed using Spearman correlation heat maps and redundancy analysis (RDA) via the TUTU analysis platform (see Footnote 2).
2.5 Bacterial and fungal conventional isolation
Root samples (1 g per A. ordosica specimen) were soaked in 75% ethanol for 3 min and rinsed five times with sterile water. Next, each sample was sequentially treated with a 3% sodium hypochlorite solution for 7, 5, and 3 min, followed by five additional rinses with sterile water. The sterilized roots were then ground in 1.5 mL of sterile water using a mortar. Samples were shaken at 175 rpm at 30°C for approximately 30 min, then incubated at room temperature for 30 s. The resulting plant tissue stock solution was serially diluted to 10−1, 10−2, 10−3, 10−4, and 10−5 of the original concentration, producing five dilutions. Blank control 1 was prepared by spreading sterile water from the final rinse, which refers to the last step of the sterilization process, onto the plate to confirm that any observed growth did not result from residual substances. Blank control 2 was prepared by rolling an A. ordosica root over the plate surface several times to ensure that untreated roots did not introduce microbial contaminants. The absence of growth on both blank control plates confirmed that all bacteria cultured from root tissue fluid had originated from the root endosphere. Rhizosphere and non-rhizosphere soil samples were prepared by mixing 10 g of soil with 90 mL of sterile water, adding a few glass beads, and shaking the mixture for 30 min. The resulting soil suspension was serially diluted to 10−2, 10−3, 10−4, and 10−5, producing five dilutions.
For the isolation of bacterial strains, 200 μL of each dilution were evenly spread onto 90-mm tryptic soy broth (TSB) agar plates, with three replicates per treatment. The plates were incubated at 37°C for 48 h, after which colony growth was examined. Single colonies were selected from plates with uniform colony distribution and inoculated onto fresh medium based on morphological characteristics such as shape, size, and color. The three-line streak method was used to obtain pure bacterial colonies.
The fungal strains were isolated from the same environmental samples. The sample pretreatment procedure was carried out according to the protocol described in bacterial isolation. To put it simply, a 200 μL continuously diluted sample is evenly coated on a 90 mm potato glucose agar (PDA) plate, in three times. All petri dishes were incubated at 27°C for 5 days to monitor mycelium development. Then, different fungal colonies were selected according to the macro characteristics (colony morphology, pigmentation and edge characteristics), and one kind was picked by sterile inoculation needle and placed on fresh PDA medium for aseptic culture.
2.6 16S rRNA gene extraction and sequencing from purified strains
Bacterial DNA was extracted from purified bacterial strains using a TIANamp Bacteria DNA Kit (TIANGEN BIOTECH, CO., LTD, BEIJING) in accordance with the manufacturer’s instructions. For most bacterial strains, DNA extraction was performed using the boiling method: Scrape the mycelium from 1 to 2 inoculation loops and add it to 150 μL of triple-distilled water, mix well. Boil the mixed mycelium solution at 100°C for 10 min, then centrifuge at 12,000 rpm for 10 min. Collect the supernatant, which is the crude DNA solution. PCR amplification was performed under the following conditions: 95°C for 5 min, followed by 30 cycles of 94°C for 30 s, 50°C for 30 s, and 72°C for 30 s, with a final extension at 72°C for 10 min using the universal bacterial primers 27F (5′-AGRGTTYGATYMTGGCTCAG-3′) and 1492R (5′-RGYTACCTTGTTACGACTT-3′) (Stackebrandt and Goodfellow, 1991). Fungal DNA was extracted from purified bacterial strains using a E.Z.N.A. SP Fungal DNA Kit (Shanghai solarbio Bioscience & Technology Co., LTD, Shanghai) in accordance with the manufacturer’s instructions. PCR amplification was performed under the following conditions: 94°C for 10 min, followed by 35 cycles of 94°C for 15 s, 55°C for 30 s, and 72°C for 40 s, with a final extension at 72°C for 7 min using the universal ITS1 (5′-TCCGTAGGTGAACCTGCGG-3′) and ITS4 (5′-TCCTCCGCTTATTGATATGC-3′).
The PCR products of bacteria and fungi were subjected to 1% agarose gel electrophoresis, with D2000 DNA Marker (Sangon, Shanghai, China) as the size marker. Aliquots of 4 μL of each sample were loaded onto the gels, and nucleic acids were stained with GelRed (Biotium, Fremont, CA, United States). Electrophoresis was performed at 120 V for 20 min (Sub-Cell GT System, Bio-Rad, Hercules, CA, United States). Electrophoresis results were visualized using a gel imaging system (Tanon Science & Technology Co., Ltd., Shanghai, China). PCR products with positive electrophoresis results were sequenced by Beijing Ruibo Biotechnology Co., Ltd. (Beijing, China) using the sequencing primers 27F and 1492R for bacteria and ITS1 for fungi. Sequence alignment was performed using the online tool EzBioCloud3 (Yoon et al., 2017). All 301 obtained bacterial sequences were deposited in GenBank under accession numbers PV428570-PV428870 and fungal sequences were deposited in GenBank under accession numbers PV426714-PV426727 (Supplementary Table 1).
2.7 High-throughput cultivation and identification of root zone bacteria
Root-associated bacteria from A. ordosica across successional stages were isolated by high-throughput cultivation using a modified version of a previously described protocol (Zhang et al., 2021). The key modifications were as follows:
2.7.1 Sample preparation
Root tissues were surface sterilized with 75% ethanol (30 s) and 8% sodium hypochlorite (5 min), then homogenized in 10% MgCl2. Soil suspensions were prepared from non-rhizosphere samples.
2.7.2 Gradient dilution strategy
Suspensions were serially diluted in 10% TSB medium (100 × to 25,000×) and inoculated into 96-well plates (160 μL/well). Three technical replicates per dilution were performed across 48 plates, with sterile medium serving as the control.
2.7.3 Cultivation and selection
After sealing with Parafilm, plates were incubated at 25°C for 14 days. Optimal dilution concentrations (ODCs) were defined as those at which approximately 30% of wells exhibited turbidity. Subsequent dilutions (1/3 ODC, 1 ODC, and 3 ODC) were used to minimize cultivation bias.
2.7.4 DNA extraction and PCR amplification
Bacterial lysates were prepared by alkaline lysis (95°C, 30 min) and neutralized. Neutralization buffer containing 40 mM Tris–HCl at pH 7.5. The 16S rRNA gene was amplified using a two-round PCR approach:
First PCR: A 400-bp fragment was amplified using primers 799F/1193R, with Escherichia coli DNA as a positive control.
Second PCR: Barcoding primers were used to generate a 500-bp amplicon for multiplex sequencing.
2.7.5 Bioinformatics and strain preservation
Amplicon sequence variants (ASVs) were analyzed as previously described (Zhang et al., 2021). Strain saturation was confirmed using rarefaction curves. All bacterial strains in 96-well cell culture plates were preserved in 80% glycerol at -80°C for long-term storage.
3 Results
3.1 Physicochemical properties of soil
Measurements of the physical and chemical characteristics of the soil revealed significant variations across successional stages in MUSL. Notably, NO3−-N, NH4+-N, AK, TK, and OM contents significantly differed (p < 0.05) (Table 2). The NO3−-N content significantly decreased during succession from moving dunes to fixed dunes (p < 0.05), particularly between moving dunes and semi-fixed dunes, where a 91.17% reduction was observed. Conversely, NH4+-N exhibited a continuous increase along the same successional gradient. The NH4+-N content was 6.13% higher in fixed dunes than in moving dunes (p < 0.05). Both AK and OM significantly varied across the successional stages (p < 0.05), initially increasing and then declining. AK increased by 40.34% from moving dunes to semi-fixed dunes but decreased by 21.5% from semi-fixed dunes to fixed dunes. OM exhibited a more gradual change compared with AK, increasing by 5.23% before decreasing by 26.39%. In the succession from moving dunes to semi-fixed dunes, TK decreased by 9.34% (p < 0.05). However, no significant differences were observed in TP, AP, available nitrogen, or pH across the successional stages from moving dunes to fixed dunes. In conclusion, soil properties differed across successional stages in MUSL. Furthermore, a significant positive correlation was identified between OM and NO3−-N (p < 0.01) (Supplementary Figure 2). Significant negative correlations were found among NH4+-N, pH, and TP (p < 0.05) and between TK and AK (p < 0.01).
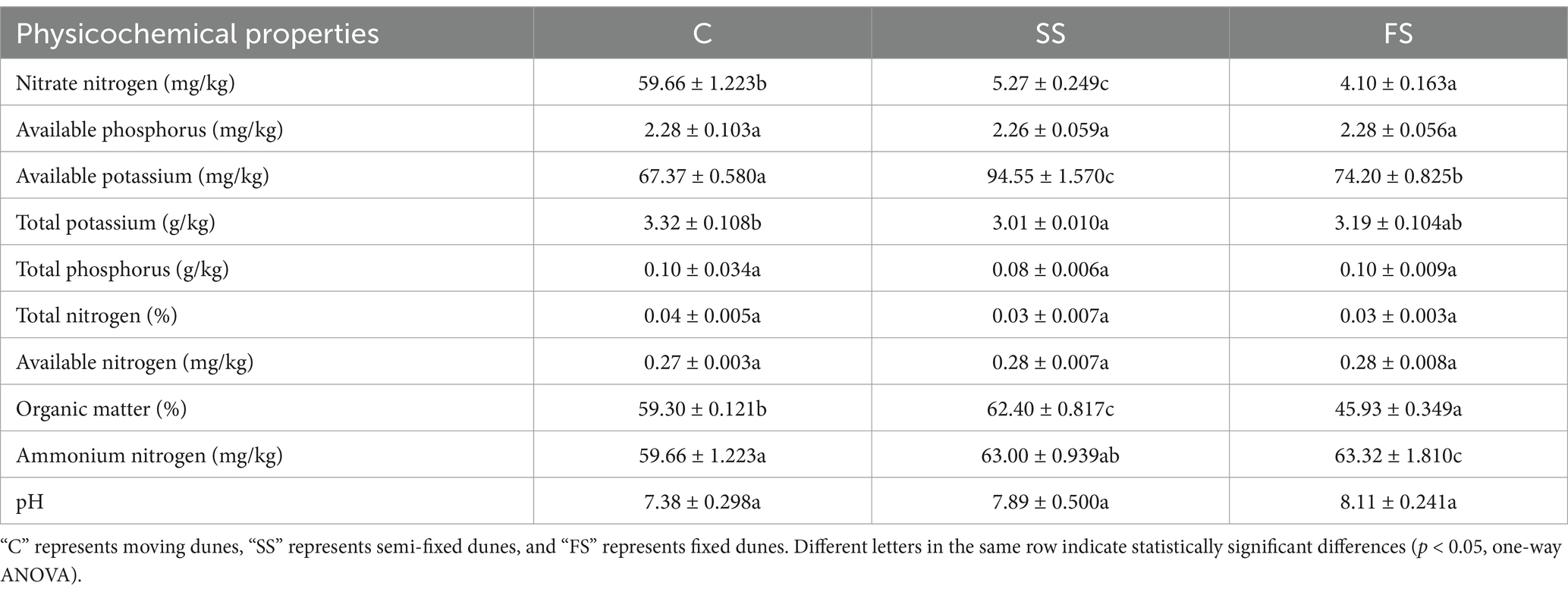
Table 2. Physicochemical characteristics of the soil at various successional stages in Mu Us Sandy Land.
3.2 General features of the sequencing data
After quality control, a total of 3,516,828 optimized bacterial sequences were obtained, distributed across the three root zone samples. Specifically, the three successional stages in MUSL contained 2,867,563 optimized sequences in the R samples, 2,535,205 in the F samples, and 2,535,281 in the O samples. The length of the optimized bacterial sequences was primarily 360–380 bp, with an average length of 376 bp. After quality control, a total of 3,936,363 optimized fungal sequences were obtained, which were distributed across the three root zone samples. Specifically, 1,534,082 optimized sequences were identified in the R samples, 1,224,167 in the F samples, and 1,178,114 in the O samples across the three successional stages in MUSL. The length of the optimized fungal sequences was primarily 241–260 bp, with an average length of 245 bp.
The optimized sequences were clustered into OTUs for species classification based on 97% sequence similarity. A total of 7,252 OTUs were generated for bacterial samples, and 1,454 OTUs were generated for fungal samples. The sparse curve of the coverage index approached a saturation plateau as the number of reads increased, indicating that sequencing depth was sufficient for comprehensive data analysis (Supplementary Figure 3).
3.3 Diversity and structure of the microbial community
Microbial diversity in each sample was assessed using alpha diversity, measured by the Shannon index (Figure 1). The bacterial Shannon indices were 10.79-fold and 11.29-fold higher in the R and F samples, respectively, than in the O samples (Figure 1A). Similarly, the Shannon index for fungi was 1.42-fold higher in the R samples and 1.33-fold higher in the F samples compared with the O samples (Figure 1B). C samples exhibited the lowest bacterial and fungal diversities across all root zone types (Figure 1).
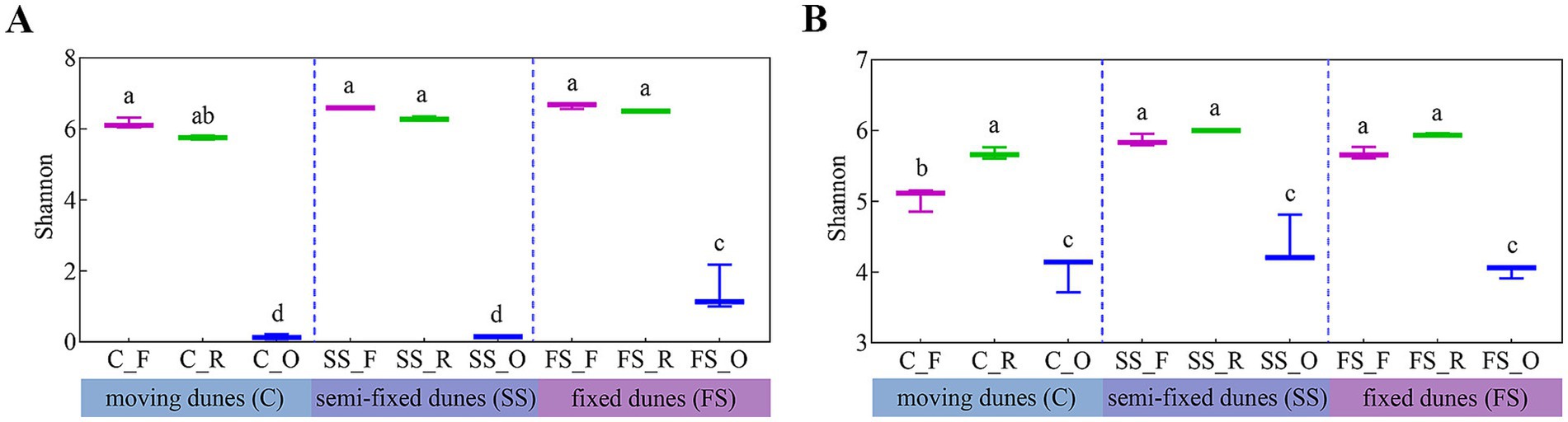
Figure 1. Alpha diversity of microbial communities in the root zone of Artemisia ordosica across three successional stages in Mu Us Sandy Land. Shannon indices of (A) bacterial and (B) fungal communities. Whiskers indicate the lowest and highest values. One-way ANOVAs are used to examine the data, and significant differences at p < 0.05 are indicated through different small letters on the bars.
The influence of soil physical and chemical factors on alpha diversity was analyzed in the root zone of A. ordosica. A negative correlation was observed between the Chao1 index and OM content in the bacterial community (r = −0.76, p = 0.018, linear regression) (Supplementary Figure 4). In the fungal community, the Chao1 index showed significant positive correlations with AK content (r = 0.8, p = 0.009) and NH4+-N (r = 0.69, p = 0.04), as well as a significant negative correlation with TK content (r = −0.79, p = 0.011) (Supplementary Figure 5).
All samples were clearly divided into two groups: endosphere and non-endosphere (Figure 2A). The results of Bray–Curtis dissimilarity-based ADONIS (R2 = 0.927, p = 0.001) and ANOSIM (R2 = 0.896, p = 0.001) tests confirmed significant differences in bacterial composition across the root zone of A. ordosica at different successional stages in MUSL. Additionally, close clustering was observed among root endosphere bacterial communities from C, SS, and FS, indicating high similarity among these communities. However, bacterial communities in C samples were distinct from those in other root zone samples of A. ordosica.
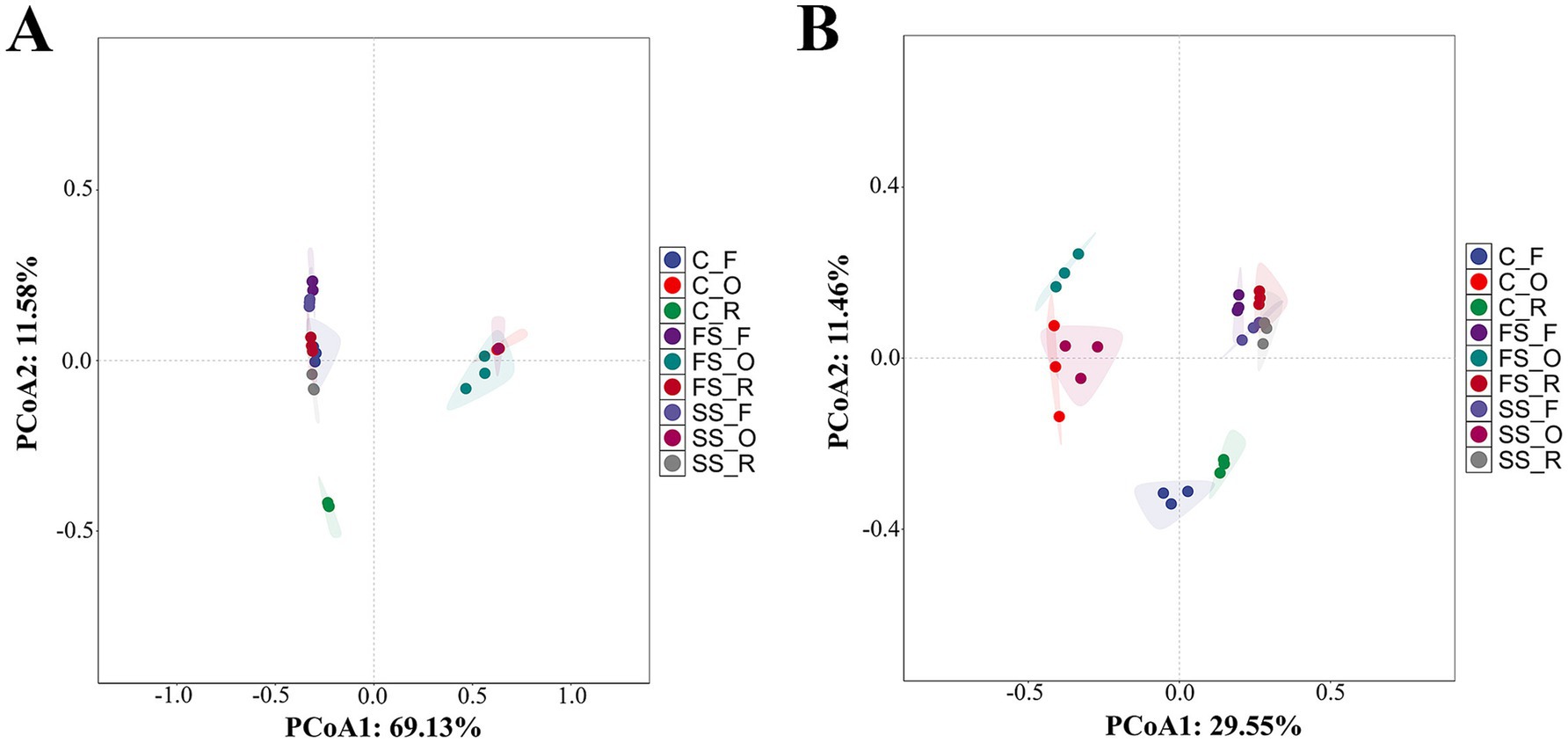
Figure 2. Principal coordinates analysis (PCoA) of microbial communities across the root zones of Artemisia ordosica Krasch. at different successional stages in Mu Us Sandy Land [(A) bacteria (B) fungi]. FS, SS, and C represent fixed dunes, semi-fixed dunes, and moving dunes, respectively. O, F, and R represent root endosphere, non-rhizosphere soil, and rhizosphere soil.
Similar to bacterial composition, fungal composition was clearly grouped into endosphere and non-endosphere groups (Figure 2B). The results of Bray–Curtis dissimilarity-based ADONIS (R2 = 0.66, p = 0.001) and ANOSIM (R2 = 0.737, p = 0.001) tests confirmed significant differences in fungal composition across the root zone of A. ordosica at different successional stages in MUSL. Furthermore, clustering was observed among root endosphere fungal communities from C, SS, and FS, particularly between C and SS, suggesting that these fungal communities were highly similar. However, rhizosphere and non-rhizosphere fungal communities of A. ordosica in C samples differed from those in the SS and FS samples.
3.4 Taxonomic distribution of microbial communities
This study identified 3, 9, and 12 major bacterial phyla (i.e., phyla with > 1% relative abundance in at least one group) in the endosphere, rhizosphere, and non-rhizosphere, respectively, of A. ordosica. The three most prevalent endosphere bacterial phyla in FS samples were unclassified_k__norank_d__Bacteria (78.89%), Proteobacteria (11.80%), and Actinobacteria (7.57%) (Figure 3A). However, in SS and C samples, nearly all endosphere bacterial sequences (98.87% and 98.45%, respectively) belonged to unclassified_k__norank_d__Bacteria. In the rhizosphere bacterial community, Proteobacteria (32.32%–43.11%) and Actinobacteria (18.58%–36.17%) were the most prevalent phyla, followed by Bacteroidetes (6.38%–15.11%) and Acidobacteria (4.68%–10.06%). Notably, rhizosphere communities in C samples had greater abundances of Proteobacteria, Bacteroidetes, and Firmicutes compared with the other two sampling sites (Figure 3A). The most abundant non-rhizosphere bacterial phyla were Actinobacteria (29.47%–31.56%) and Proteobacteria (25.35%–32.73%), followed by Acidobacteria (8.24%–15.01%) and Chloroflexi (4.98%–7.50%). Compared with the other two sampling sites, Acidobacteria were less common within rhizosphere communities in C samples.
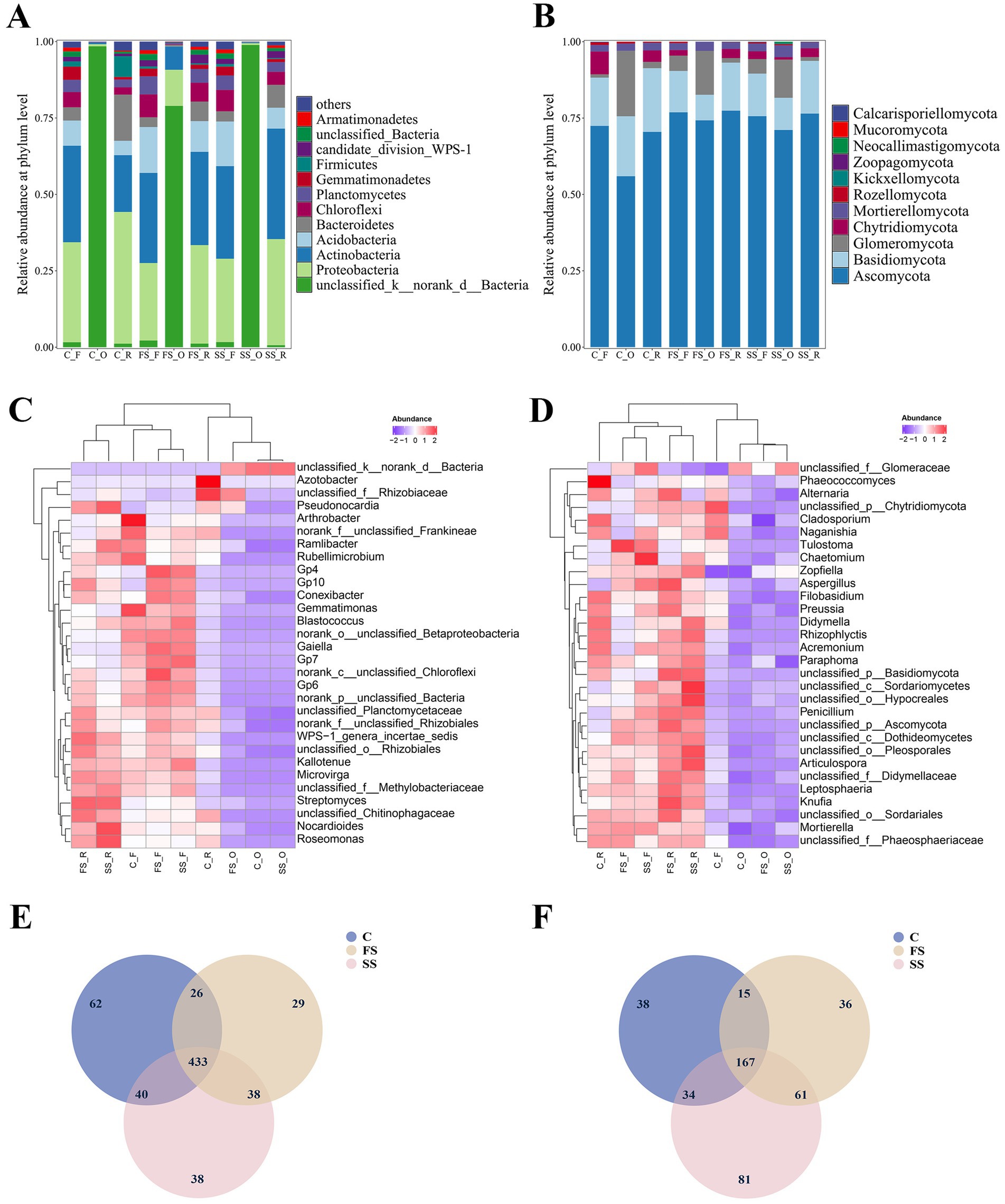
Figure 3. Compositions of the (A) bacterial and (B) fungal communities at the phylum level in the root zone of Artemisia ordosica under various successional stages in Mu Us Sandy Land (MUSL). Heat maps displaying the 30 most abundant (C) bacterial and (D) fungal genera in the root zone of A. ordosica. Color intensity represents the relative abundance of each genus as a percentage of total effective sequences. (E) Venn diagram illustrating exclusive and shared bacterial genera across the three successional stages in MUSL. (F) Venn diagram illustrating exclusive and shared fungal genera across the three successional stages in MUSL. FS, SS, and C represent fixed dunes, semi-fixed dunes, and moving dunes, respectively. O, F, and R represent root endosphere, non-rhizosphere soil, and rhizosphere soil.
In the fungal community, A. ordosica endosphere, rhizosphere, and non-rhizosphere contained four, five, and five dominant phyla (i.e., phyla with >1% relative abundance in at least one group), respectively. As shown in Figure 3B, the four predominant fungal phyla in the endosphere were Ascomycota (55.95%–74.25%), Glomeromycota (12.50%–21.43%), Basidiomycota (8.38%–19.64%), and Mortierellomycota (2.38%–3.91%). The SS samples contained a higher abundance of Mortierellomycota sequences than the other two sampling sites, whereas the FS samples contained a higher abundance of Glomeromycota sequences. In the rhizosphere fungal community, the most abundant phyla were Ascomycota (70.47%–77.42%) and Basidiomycota (15.73%–20.87%), followed by Chytridiomycota (2.89%–3.76%) and Mortierellomycota (1.98%–2.51%). The most abundant phyla in the non-rhizosphere fungal community were Ascomycota (72.44%–76.88%) and Basidiomycota (13.55%–15.81%), followed by Chytridiomycota (1.82%–7.49%) and Glomeromycota (1.07%–5.01%). Notably, non-rhizosphere communities from C samples contained a higher abundance of Chytridiomycota compared with those from the other two sampling sites (Figure 3B).
Hierarchically clustered heat map analysis was performed to identify and evaluate the 30 dominant bacterial genera (Figure 3C). At the genus level, unclassified_k__norank_d__Bacteria (92.09%) was the most abundant bacterial taxon in the endosphere of A. ordosica. In contrast to the endosphere, Azotobacter (3.46%) and Nocardioides (3.22%) were dominant bacteria in the rhizosphere of A. ordosica, followed by Microvirga (2.75%), Arthrobacter (2.66%), and Streptomyces (2.59%). Gp6 (4.68%) and Arthrobacter (5.16%) were dominant bacteria in the non-endosphere. The bacterial communities of A. ordosica were primarily clustered into three categories based on R, F, and O sample types. However, R samples from the moving dunes (C_R) did not cluster with R samples from the other two sampling sites (Figure 3C). Additionally, rhizosphere bacterial communities in SS and FS samples were more closely compared to those in the C samples, similar to findings regarding non-rhizosphere samples (Figure 3C). In contrast, endosphere bacterial communities exhibited greater similarity between C samples and SS samples (Figure 3C).
Moreover, hierarchically clustered heat map analysis was conducted to evaluate the 30 most abundant fungal genera (Figure 3D). Among the endosphere samples, the most abundant fungal genera in A. ordosica were unclassified_f__Glomeraceae (8.80%), Paraphoma (7.61%), and unclassified_c__Sordariomycetes (3.21%), followed by Mortierella (3.05%) and Aspergillus (2.88%). In contrast, fungal communities in the rhizosphere and non-rhizosphere of A. ordosica were dominated by unclassified_p__Ascomycota (6.48 and 6.58%, respectively) and unclassified_c__Sordariomycetes (3.97 and 3.46%, respectively). Compared with the endosphere, these taxa were more abundant in the rhizosphere and non-rhizosphere. Furthermore, heat map analysis indicated that, except for C samples, the fungal communities of A. ordosica were primarily clustered into three distinct groups based on R, F, and O sample types (Figure 3D). Fungal communities in SS and FS samples exhibited greater similarity, clustering more closely relative to C samples (Figure 3D).
3.5 Venn diagram of microbial communities
In total, 666 bacterial genera were identified in the root zone of A. ordosica. Among these, 433 genera were detected in MUSL samples at different successional stages (Figure 3E). C samples contained the greatest number of annotated bacterial genera (126 genera), whereas FS samples contained the fewest (93 genera). Additionally, 62, 38, and 29 genera were unique to the C, SS, and FS samples, respectively (Figure 3E).
In the fungal community, 432 genera were annotated, with 167 genera shared across the three successional stages in MUSL, comprising 38.66% of the total fungal genera. This proportion was lower than that observed for bacteria, suggesting that fungal-specific genera were more characteristic of different successional stages in MUSL. The numbers of unique fungal genera identified in C, SS, and FS samples were 38, 81, and 36, respectively (Figure 3F). The SS plot contained the highest numbers of fungal genera (254) and unique fungal genera (81, corresponding to 18.75%) (Figure 3F).
3.6 Relationships between microbial community structure and environmental factors
Correlations between the microbial community and environmental factors in the root zone of A. ordosica were analyzed by Mantel test heat map analysis, as shown in Figure 4. The bacterial community exhibited a strong positive correlation with AK content (p < 0.01) and positive correlations with TK and TN contents (both p < 0.05). The fungal community also showed positive correlations with TK content and pH (both p < 0.05). Additionally, the analysis revealed a negative correlation between TK and AK contents, negative correlations between TN content and both NH4+-N content and pH, and a positive correlation between OM and NO3−-N contents. These findings suggest that AK significantly increased the richness of bacterial communities in the root zone of A. ordosica, whereas TK promoted the richness of both fungal and bacterial communities.
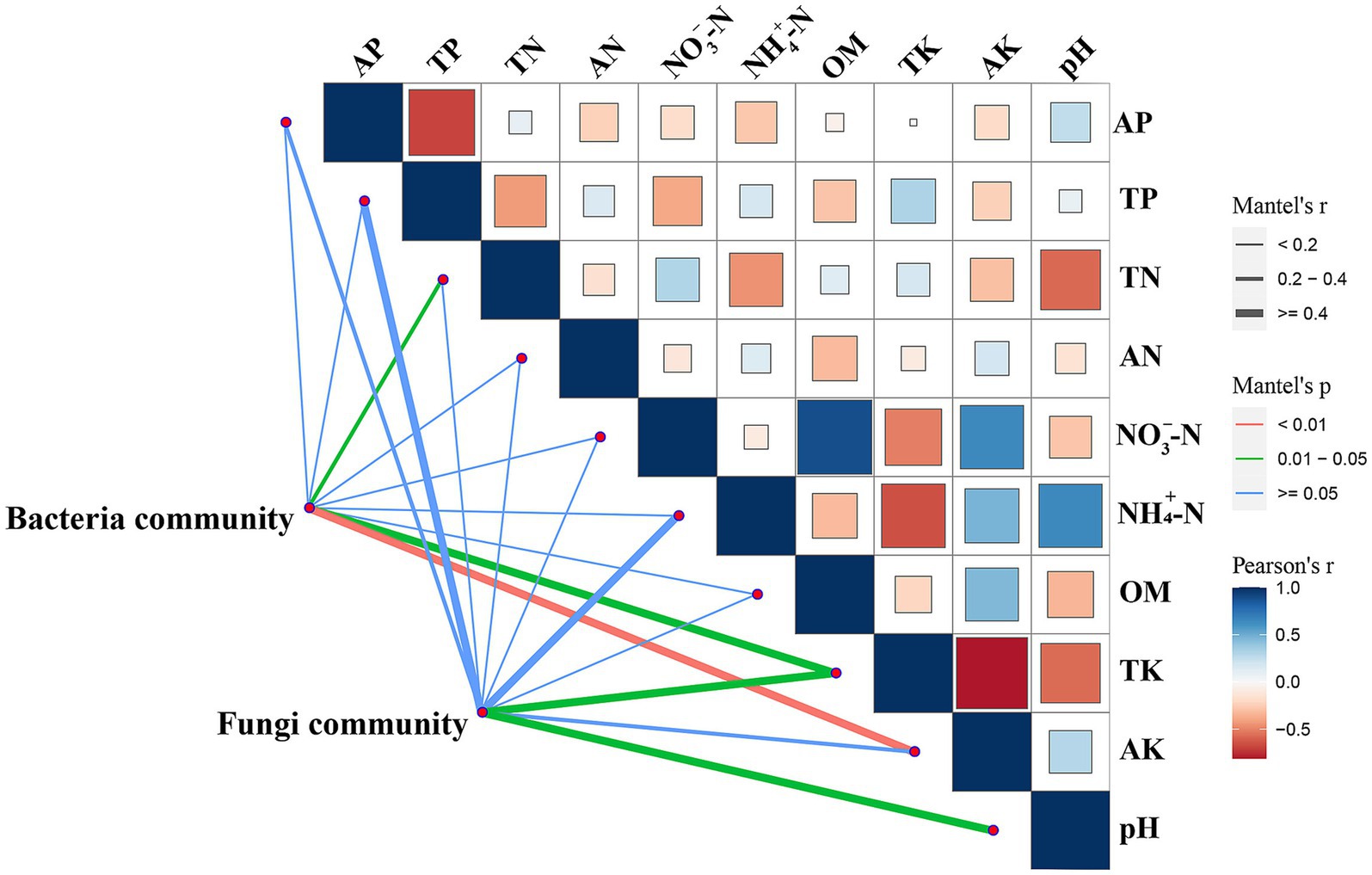
Figure 4. Mantel test heat map analysis of microorganisms in the root zone of Artemisia ordosica. The thickness of the lines connecting the microbial community and edaphic physicochemical factors represents Mantel’s r, whereas line color indicates Mantel’s p. Correlations between two edaphic physicochemical factors are depicted by square size and color; larger squares indicate stronger associations. Pearson’s r is reflected by the square color gradient. AP, available phosphorus; TN, total nitrogen; TP, total phosphorus; TK, total potassium; NO3—N, nitrate nitrogen; AK, available potassium; AN, available nitrogen; NH4+-N, ammonium nitrogen; OM, organic matter.
RDA was performed to assess potential relationships between microbial community composition and environmental parameters. As shown in Figure 5A, the primary soil environmental factors with significant impacts on the composition and assembly of the bacterial community of A. ordosica were NO3−-N (R2 = 0.8763740, p = 0.001), OM (R2 = 0.9850710, p = 0.003), AK (R2 = 0.9605907, p = 0.004) and TK (R2 = 0.6475219, p = 0.042). The analysis also indicated that the bacterial community structure of the FS samples was positively correlated with pH but negatively correlated with OM and NO3−-N contents. The bacterial community structure of SS samples showed a strong positive correlation with AK but was negatively correlated with TK content. However, the bacterial community composition in C samples was negatively correlated with NH4+-N content and pH.
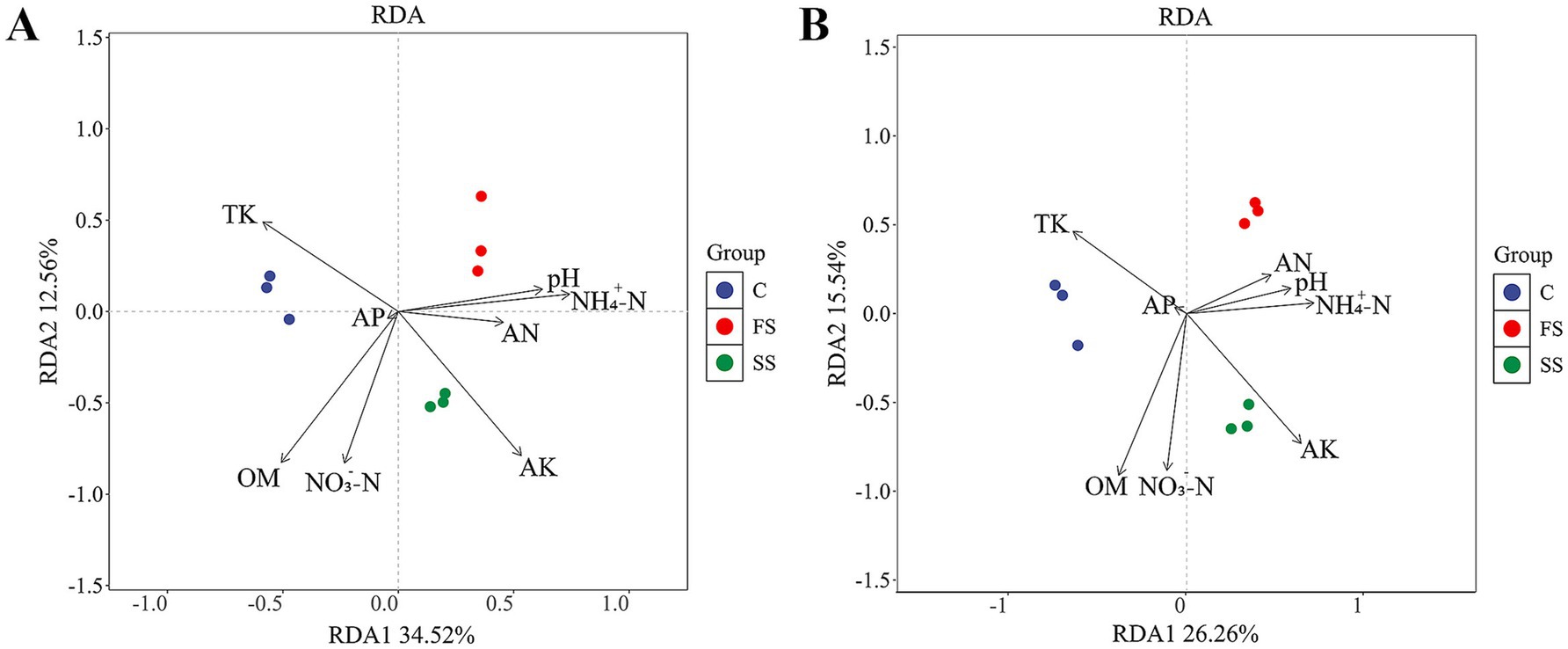
Figure 5. Redundancy analysis (RDA) of correlations between microbial communities and soil physicochemical factors [(A) bacteria (B) fungi]. Arrows indicate the magnitude and direction of environmental factors influencing bacterial community structure. NH4+-N, ammonium nitrogen; OM, organic matter; AP, available phosphorus; TP, total phosphorus; TK, total potassium; NO3−-N, nitrate nitrogen; AK, available potassium; AN, available nitrogen. FS, SS, and C represent fixed dunes, semi-fixed dunes, and moving dunes, respectively.
The primary soil environmental parameters that significantly influenced the composition and assembly of the fungal community of A. ordosica were OM (R2 = 0.98765748, p = 0.002), NO3−-N (R2 = 0.78070967, p = 0.008), AK (R2 = 0.93774130, p = 0.009), and TK (R2 = 0.62823881, p = 0.049), as shown in Figure 5B. Additionally, AK content was positively correlated with the fungal community structure of SS samples (Figure 5B).
3.7 Molecular ecological network of microbial communities
To examine correlations among root zone microorganisms in A. ordosica at different successional stages in MUSL, a network diagram was constructed depicting microbial co-occurrence patterns at each stage. In the bacterial co-occurrence network analysis, most nodes exhibited positive interactions, whereas in the fungal network, nearly all nodes demonstrated positive associations, suggesting that microbial communities in the root zone of A. ordosica formed a highly interconnected network through synergistic relationships during succession (Figure 6). For bacterial communities, the number of connections varied across sites: 4,001 connections in C, 3,497 in SS, and 1,739 in FS. These findings suggest that moving dunes (C) had a more complex bacterial network than the other sites. The modularity of bacterial networks in C and SS samples was higher than in FS samples, indicating that C and SS exhibited greater network complexity and a more defined functional organization. The average degree of the fungal community was relatively high, suggesting strong species interactions. Additionally, the modularities of fungal communities in C and FS were > 0.4, indicating that these sites maintained modular structures.
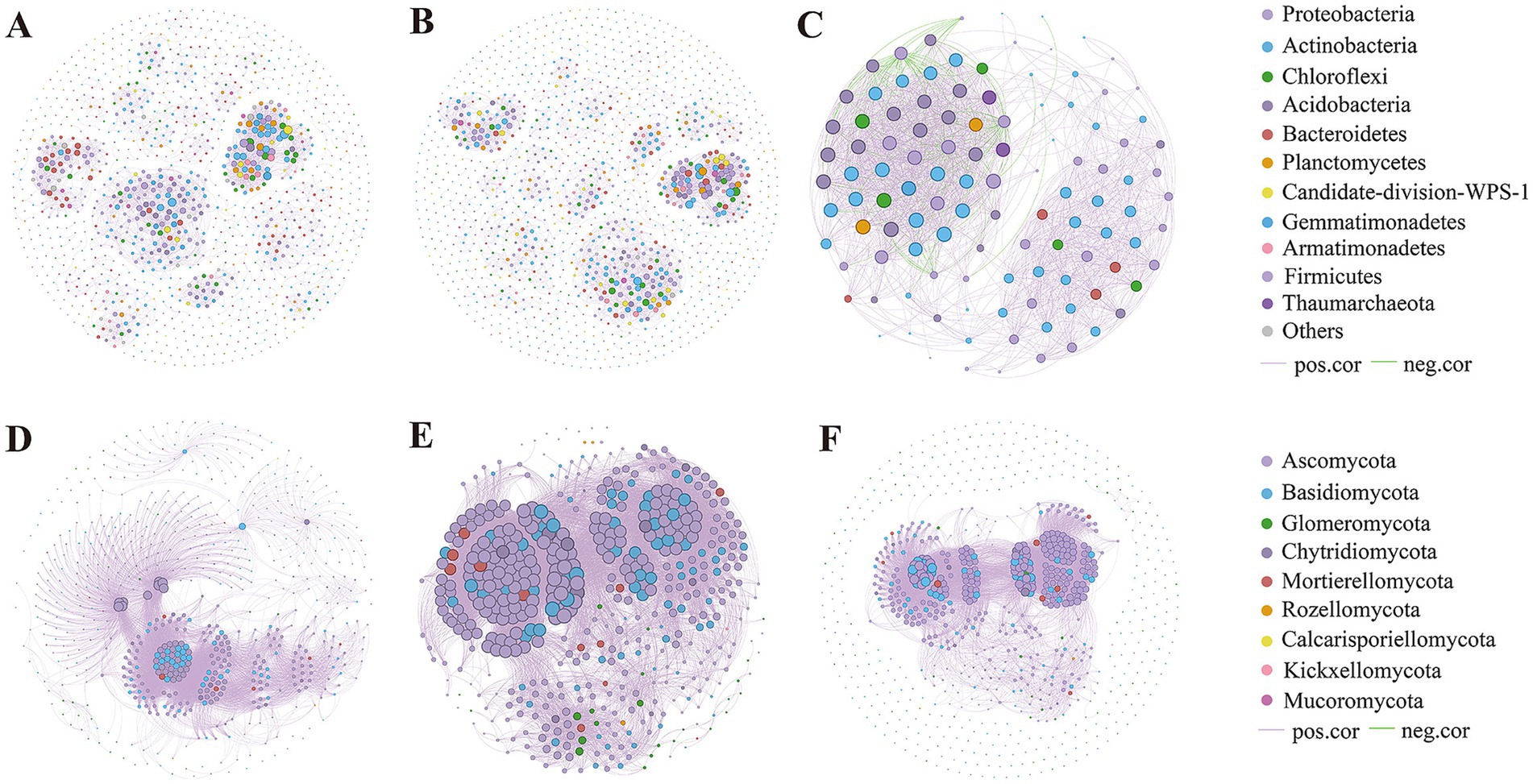
Figure 6. Bacterial (A,B,C) and fungal (D,E,F) networks of dominant microbiota at the phylum level in moving dunes (C) (A,D), semi-fixed dunes (SS) (B,E), and fixed dunes (FS) (C,F). OTU abundance is represented by node size, and the phylum-level taxonomic classification is indicated by node color. Positive correlations (purple) and negative correlations (green) are represented by the edge color.
3.8 Culturomics insight of microbial community with different isolation methods
To characterize the culturable microbiota associated with A. ordosica, conventional streak plate and colony pick methods were employed for isolation of microorganisms across all samples. In parallel, a high-throughput cultivation method specifically designed for bacterial isolation was implemented to expand the taxonomic coverage of culturable bacteria.
The conventional streak plate and colony pick methods yielded 93 purified bacterial strains which belonged to six phyla, 30 genera. The core bacterial phyla captured by this method were Firmicutes (relative abundance: 37.63%), Proteobacteria (relative abundance: 30.11%), Actinobacteria (27.96%), and Bacteroidetes (2.15%) (Figure 7A). In addition, Bacillus (23.66%) was the most predominant bacterial genus, followed by Streptomyces (16.13%) and Pseudomonas (11.83%) (Figure 7B). Meanwhile, a total of 14 fungal strains were successfully isolated by colony picking. Taxonomic classification based on ITS-rDNA sequencing revealed that the isolates were predominantly composed of the genus Penicillium (relative abundance: 35.71%), followed by Aspergillus (21.43%) and Cladosporium (14.29%).
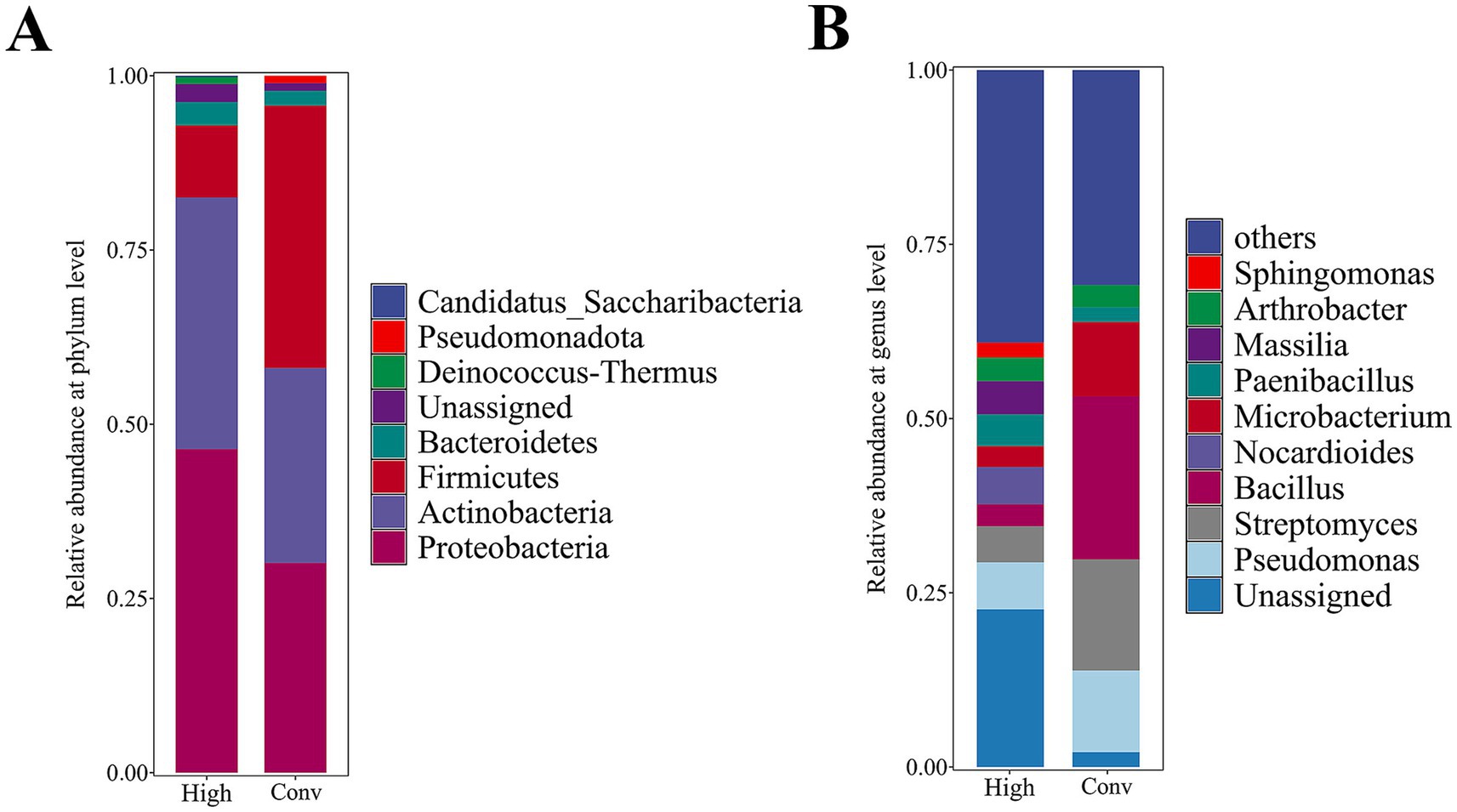
Figure 7. Taxonomic composition of bacteria in the root zone of Artemisia ordosica analyzed using the conventional streak plate and colony pick methods (Conv) and the high-throughput cultivation and identification method (High). (A) Composition of bacterial communities at the phylum level obtained using the two culture methods. (B) Composition of bacterial communities at the genus level obtained using the two culture methods. The 10 genera with the highest relative abundance are shown; the remaining genera are combined into the “Other” category.
The high-throughput cultivation and identification method, coupled with amplicon sequence variant (ASV) analysis, which identified 504 unique ASVs. Saturation analysis of sequencing-derived strains demonstrated gradual stabilization of diversity metrics (Supplementary Figure 6), confirming that our 48 × 96-well plate cultivation system provided sufficient strain recovery. Colony purity assessment revealed that 17.7% of wells contained >95% pure cultures (Supplementary Figure 7). The high-throughput method captured seven phyla, 102 genera bacterial strains. The most abundant phyla isolated using this method were Proteobacteria (relative abundance: 46.43%), Actinobacteria (relative abundance: 36.11%), Firmicutes (10.32%), and Bacteroidetes (3.37%) (Figure 7A). At the genus level, Unassigned (22.62%) and Pseudomonas (6.75%) were dominant bacteria, followed by Nocardioides (5.36%), Streptomyces (5.16%) (Figure 7B). Intriguingly, four strains belonging to the Deinococcus genera (phylum Deinococcus-Thermus) were isolated via this approach, potentially linked to the adaptation of A. ordosica to arid sandy environments.
4 Discussion
4.1 Differences in microbial community structure and diversity across root zones and successional stages in sandy land
Microorganisms inhabiting the plant endosphere and rhizosphere serve as bridges among plants, microbial communities, and the surrounding environment (Niu et al., 2017). Our findings demonstrated that microbial diversity was lowest in moving dunes (C), consistent with their sparse vegetation coverage (Supplementary Figure 1). Considering both microbial diversity patterns and geomorphic characteristics of moving dunes, it is likely that scarce vegetation resources contributed to low microbial diversity. This finding is consistent with previous research indicating that plants promote the growth of soil bacteria and fungi during community succession (Li et al., 2013).
Notably, beta diversity patterns revealed divergent community structures across successional stages, with C samples exhibiting the most pronounced differences in rhizosphere and non-rhizosphere compartments (Figure 2). However, root endosphere communities maintained high structural similarity regardless of site, reinforcing their documented stability against environmental variations (Edwards et al., 2015; Wagner et al., 2016). Environmental differences among various plant compartments likely contribute to the stability and limited diversity of the root endosphere. Additionally, plant immunity serves as a selective barrier, limiting microbial entry and colonization, thereby maintaining the root endosphere as a relatively stable and less diverse environment (de Lamo et al., 2021; Oukala et al., 2021). Compared with the simpler environment of the endosphere, the rhizosphere receives nutrients and signaling molecules secreted by plants, which recruit specific microorganisms and promote their colonization (Jin et al., 2019; Dhungana et al., 2023). Complex physical and chemical interactions occur in the rhizosphere during plant–microbe interactions (Liu et al., 2020). These interactions require support from multiple functional microorganisms, leading to the formation of a complex and diverse rhizosphere microbial community (Kuppe et al., 2022). In contrast, the non-rhizosphere soil environment lacks plant-derived secretions, resulting in a microbial community structure distinct from that of the rhizosphere.
4.2 Factors influencing microbial community diversity and composition
The diversity and composition of microbial communities are strongly influenced by soil physicochemical properties, including nutrient availability and pH (Wang et al., 2024; Eduah et al., 2024). Consistent with previous research, our findings highlight nitrogen and phosphorus—essential for bacterial metabolism—as major determinants of microbial community dynamics (Liao et al., 2015). Soil pH is also a key determinant of the soil microbiome (Zhang et al., 2022; Liu et al., 2023). Interestingly, bacterial communities in moving dunes exhibited a negative correlation with pH, suggesting niche-specific adaptations to alkaline conditions. Microorganisms acquire nutrients by decomposing OM, and microbial community composition can be strongly influenced by altered organic material availability (Fagervold et al., 2014; Yang et al., 2024). This mechanistic link explains the strong association observed between OM content and both bacterial and fungal communities in our redundancy analysis.
Co-occurrence networks provide insights into microbial interactions and community formation (Williams et al., 2014). The higher connectivity in moving dunes (C) implies that early successional stages foster more intricate microbial networks, possibly due to greater environmental stress or niche opportunities. The pronounced modularity in bacterial networks at C and SS sites represents a higher degree of network complexity and suggests that network modules exhibited clearer structural organization and functional differentiation. This modular structure likely enhanced the functional efficiency of microbial interactions (Supplementary Table 2). Notably, most nodes exhibited positive interactions, particularly among fungal communities, which indicates that microbial communities in the root zone of A. ordosica formed a highly interconnected network, facilitated by synergistic interactions among species during succession in MUSL (Figure 6).
4.3 Dominant genera of microorganisms in the root zone
At the genus level, several functionally important taxa emerged as key components of the A. ordosica root zone microbiome. The rhizosphere was particularly enriched with Arthrobacter, Nocardioides and Streptomyces, bacterial genera with plant growth-promoting capabilities and stress tolerance traits. Genome sequence analysis of Arthrobacter koreensis 5J12A revealed that Arthrobacter spp. can tolerate desiccation and promote plant growth (Manzanera et al., 2015). The novel desert-adapted strain KC13(T), isolated from the Karakum Desert, was identified as Nocardioides spp. (Saygin et al., 2024). Streptomyces spp. also exhibits growth-promoting effects on plants. Strain Z38, isolated from contaminated root tissue, was confirmed to enhance plant growth (Costa et al., 2020). In greenhouse experiments, seed treatments with Streptomyces TKSC3 and Streptomyces SS8 led to increases of 20%, 23%, and 33% in germination rate, root length, and dry weight, respectively. Additionally, single and combined Streptomyces treatments successfully inhibited rice leaf blight (Hata et al., 2021). These findings suggest that representative strains of these genera, which are enriched in the root zone of A. ordosica, contribute to plant growth promotion and drought resistance. Similarly, fungal communities featured Paraphoma as a dominant endophytic genus, which has been demonstrated to possess the ability to enhance plant growth and secondary metabolite production under abiotic stress conditions (Li et al., 2024). These findings highlight the roles of dominant genera, such as Paraphoma, in plant growth promotion and their potential applications in phytoremediation strategies.
4.4 Analysis of microbial taxonomic composition based on different isolation methods
The cultivation approaches employed in this study successfully captured diverse bacterial and fungal communities associated with A. ordosica roots, revealing distinct taxonomic profiles through different isolation methods. The conventional streak plate and colony pick methods predominantly recovered Firmicutes, particularly Bacillus species, which may reflect their fast-growing nature and spore-forming capabilities under standard culture conditions. The fungal isolates were dominated by Penicillium and Aspergillus, genera well-known for their stress tolerance and ecological versatility in harsh environments (Yovchevska et al., 2025; Ortega-Villaizán et al., 2025; Haseena et al., 2024). These findings collectively expand our understanding of the culturable microbiome associated with A. ordosica, providing a valuable repository of microbial resources for further investigation.
The high-throughput cultivation method demonstrated enhanced capacity to isolate rare and slow-growing taxa, as evidenced by the recovery of Deinococcus species—radiation-resistant extremophiles particularly suited to arid environments (Rainey et al., 2005). The limitations of laboratory-based microbial culture are primarily due to difficulties in replicating environmental conditions and microbial competition. Cultivation in 96-well plates helps mitigate these challenges by diluting the medium, thus facilitating the growth of rare and slow-growing bacteria (Lewis et al., 2021).
The isolated strains, particularly those belonging to drought-adapted genera like Deinococcus and Streptomyces, represent promising candidates for developing microbial inoculants to enhance plant stress resistance. Future studies should first validate their phytobeneficial effects through controlled pot experiments under water-deficit conditions. Subsequently, integrated transcriptomic and metabolomic analyses could elucidate the molecular mechanisms underlying their plant growth promotion and drought mitigation capabilities. Finally, the potential of these candidate strains to serve as microbial inoculants for sustainable agriculture in arid regions warrants exploration.
5 Conclusion
This study characterized the microbial community profiles of the root zone (i.e., rhizosphere soil, non-rhizosphere soil, and root endosphere) of A. ordosica at different successional stages (i.e., moving dunes, semi-fixed dunes, and fixed dunes) in MUSL, China, using integrated metagenomic and culturomic approaches. Notably, the Shannon indices for bacteria in the rhizosphere soil and non-rhizosphere soil were 10.79-fold and 11.29-fold higher, respectively, compared with the root endosphere. Furthermore, across all root zone types, bacterial and fungal diversities were lowest in samples from moving dunes. PCoA analysis was implemented to reveal distinct microbial community structures across successional stages, such that the root endosphere substantially differed from other zones. Environmental factors, including NO3−-N, OM, AK, and TK contents, significantly influenced microbial community composition. Among the dominant taxa, genera such as Arthrobacter and Paraphoma were identified, suggesting potential roles in supporting A. ordosica growth. Meanwhile, in the bacterial and fungal co-occurrence network analysis, most nodes exhibited positive interactions, suggesting that microbial communities in the root zone of A. ordosica formed a highly interconnected network through synergistic relationships during succession.
Conventional streak plate and colony pick methods yielded 93 bacterial isolates (six phyla, 30 genera) dominated by Firmicutes (37.63%) and Bacillus (23.66%), alongside 14 fungal strains primarily comprising Penicillium (35.71%) and Aspergillus (21.43%)—taxa with documented stress-tolerance in arid ecosystems. Besides, the high-throughput method captured a broader culturable bacterial diversity, isolating 504 unique ASVs across seven phyla and 102 genera, including Proteobacteria assemblages (46.43%) and rare extremophiles (Deinococcus-Thermus). The high-throughput cultivation protocol substantially expanded the recoverable microbial diversity, addressing the “great plate count anomaly” in sandy land soils.
These findings provide metagenomic with culturomics insights into microbial communities in the root zone of A. ordosica at different successional stages in Mu Us Sandy Land that may contribute to desertification mitigation. Characterization of the root-associated microbial community, particularly the enrichment of Arthrobacter species, identified candidate taxa for bioinoculant development aimed at enhancing pioneer plant establishment through rhizosphere microbiome engineering. This study effectively bridges microbial ecological theory and practical bioremediation, offering a pathway for the targeted use of sandy land-adapted microbial resources.
Data availability statement
The original contributions presented in the study are publicly available. This data can be found at: https://www.ncbi.nlm.nih.gov/, accession numbers PRJNA1244809, PRJNA1246532, and PRJNA1244737.
Author contributions
WZ: Data curation, Investigation, Software, Writing – original draft. SH: Data curation, Formal analysis, Methodology, Writing – review & editing. YC: Data curation, Software, Writing – review & editing. ZY: Data curation, Writing – review & editing. GZ: Data curation, Writing – review & editing. XH: Data curation, Formal analysis, Funding acquisition, Methodology, Supervision, Writing – review & editing.
Funding
The author(s) declare that financial support was received for the research and/or publication of this article. This research was supported by the National Natural Science Foundation of China (nos. 32171869 and 31770110).
Acknowledgments
We sincerely thank Wei Feng and Yuqing Zhang for their invaluable help in sample collection and experiments.
Conflict of interest
The authors declare that the research was conducted in the absence of any commercial or financial relationships that could be construed as a potential conflict of interest.
Generative AI statement
The authors declare that no Gen AI was used in the creation of this manuscript.
Publisher’s note
All claims expressed in this article are solely those of the authors and do not necessarily represent those of their affiliated organizations, or those of the publisher, the editors and the reviewers. Any product that may be evaluated in this article, or claim that may be made by its manufacturer, is not guaranteed or endorsed by the publisher.
Supplementary material
The Supplementary material for this article can be found online at: https://www.frontiersin.org/articles/10.3389/fmicb.2025.1585700/full#supplementary-material
Footnotes
References
Abarenkov, K., Nilsson, R. H., Larsson, K.-H., Alexander, I. J., Eberhardt, U., Erland, S., et al. (2010). The UNITE database for molecular identification of fungi—recent updates and future perspectives. New Phytol. 186, 281–285. doi: 10.1111/j.1469-8137.2009.03160.x
Acuna, J. J., Rilling, J. I., Inostroza, N. G., Manquian, J., Zhang, Q., Gupta, V. V. S. R., et al. (2023). Diversity, community structure, and potential functions of root-associated bacterial communities of different wheat (Triticum aestivum) cultivars under field conditions. Agronomy 13:1392. doi: 10.3390/agronomy13051392
Bartram, A. K., Lynch, M. D. J., Stearns, J. C., Moreno-Hagelsieb, G., and Neufeld, J. D. (2011). Generation of multimillion-sequence 16S rRNA gene libraries from complex microbial communities by assembling paired-end illumina reads. Appl. Environ. Microbiol. 77, 3846–3852. doi: 10.1128/aem.02772-10
Bastian, M., Heymann, S., and Jacomy, M. (2009). Gephi: an open source software for exploring and manipulating networks. Proc. Int. AAAI Conf. Web Soc. Media 3, 361–362. doi: 10.1609/icwsm.v3i1.13937
Beckers, B., De Beeck, M. O., Weyens, N., Boerjan, W., and Vangronsveld, J. (2017). Structural variability and niche differentiation in the rhizosphere and endosphere bacterial microbiome of field-grown poplar trees. Microbiome 5:25. doi: 10.1186/s40168-017-0241-2
Biget, M., Mony, C., Aubry, M., Jambon, O., Quaiser, A., Chable, V., et al. (2021). The drivers of vine-plant root microbiota endosphere composition include both abiotic and plant-specific factors. Oeno One 55, 299–315. doi: 10.20870/oeno-one.2021.55.3.4616
Brisbin, M. M., Mitarai, S., Saito, M. A., and Alexander, H. (2022). Microbiomes of bloom-forming Phaeocystis algae are stable and consistently recruited, with both symbiotic and opportunistic modes. ISME J. 16, 2255–2264. doi: 10.1038/s41396-022-01263-2
Caporaso, J. G., Kuczynski, J., Stombaugh, J., Bittinger, K., Bushman, F. D., Costello, E. K., et al. (2010). QIIME allows analysis of high-throughput community sequencing data. Nat. Methods 7, 335–336. doi: 10.1038/nmeth.f.303
Caporaso, J. G., Lauber, C. L., Walters, W. A., Berg-Lyons, D., Huntley, J., Fierer, N., et al. (2012). Ultra-high-throughput microbial community analysis on the Illumina HiSeq and MiSeq platforms. ISME J. 6, 1621–1624. doi: 10.1038/ismej.2012.8
Costa, J. S. D., Hoskisson, P. A., Paterlini, P., Romero, C. M., and Alvarez, A. (2020). Whole genome sequence of the multi-resistant plant growth-promoting bacteria Streptomyces sp. Z38 with potential application in agroindustry and bio-nanotechnology. Genomics 112, 4684–4689. doi: 10.1016/j.ygeno.2020.08.022
de Lamo, F. J., Simkovicová, M., Fresno, D. H., de Groot, T., Tintor, N., Rep, M., et al. (2021). Pattern-triggered immunity restricts host colonization by endophytic fusaria, but does not affect endophyte-mediated resistance. Mol. Plant Pathol. 22, 204–215. doi: 10.1111/mpp.13018
Dhungana, I., Kantar, M. B., and Nguyen, N. H. (2023). Root exudate composition from different plant species influences the growth of rhizosphere bacteria. Rhizosphere 25:100645. doi: 10.1016/j.rhisph.2022.100645
Eduah, J. O., Arthur, A., Dogbatse, J. A., Amoako-Attah, I., and Afful, E. A. (2024). Ecological effects of soil physicochemical properties and copper speciation on the microbial properties associated with land use management in cacao production. Environ. Technol. Innovation 33:103538. doi: 10.1016/j.eti.2024.103538
Edwards, J., Johnson, C., Santos-Medellin, C., Lurie, E., Podishetty, N. K., Bhatnagar, S., et al. (2015). Structure, variation, and assembly of the root-associated microbiomes of rice. PNAS 112, E911–E920. doi: 10.1073/pnas.1414592112
Fagervold, S. K., Bourgeois, S., Pruski, A. M., Charles, F., Kerhervé, P., Vétion, G., et al. (2014). River organic matter shapes microbial communities in the sediment of the Rhone prodelta. ISME J. 8, 2327–2338. doi: 10.1038/ismej.2014.86
Felsenfeld, O., Soman, D. W., Waters, T., and Ishihara, S. J. (1951). Studies on recently isolated cholera vibrios; re-evaluation of culture methods. J. Bacteriol. 62, 175–180. doi: 10.1128/jb.62.2.175-180.1951
Haas, B. J., Gevers, D., Earl, A. M., Feldgarden, M., Ward, D. V., Giannoukos, G., et al. (2011). Chimeric 16S rRNA sequence formation and detection in sanger and 454-pyrosequenced PCR amplicons. Genome Res. 21, 494–504. doi: 10.1101/gr.112730.110
Haseena, M., Arumugam, S., Gopal, S., Salmen, S. H., Aljawdah, H. M., and Munusamy, M. A. (2024). Stress tolerance activity and diversification of endophytic fungi from the medicinal plant Abutilon indicum. Environ. Res. Commun. 6:ad61c5. doi: 10.1088/2515-7620/ad61c5
Hata, E. M., Yusof, M. T., and Zulperi, D. (2021). Induction of systemic resistance against bacterial leaf streak disease and growth promotion in Rice Plant by Streptomyces shenzhenesis TKSC3 and Streptomyces sp. SS8. Plant Pathol. J. 37, 173–181. doi: 10.5423/ppj.Oa.05.2020.0083
Hofer, U. (2018). The majority is uncultured. Nat. Rev. Microbiol. 16, 716–717. doi: 10.1038/s41579-018-0097-x
Hu, T. S., Chitnis, N., Monos, D., and Dinh, A. (2021). Next-generation sequencing technologies: an overview. Hum. Immunol. 82, 801–811. doi: 10.1016/j.humimm.2021.02.012
Huang, J., Yu, H., Guan, X., Wang, G., and Guo, R. (2016). Accelerated dryland expansion under climate change. Nat. Clim. Chang. 6:166. doi: 10.1038/nclimate2837
Isaac, R. A., and Kerber, J. D. (1971). Atomic absorption and flame photometry: techniques and uses in soil, plant, and water analysis. SSSAJ 35, 757–760. doi: 10.2136/1971.instrumentalmethods.c2
Jin, X., Shi, Y. J., Tan, S. C., Ma, C. L., Wu, F. Z., Pan, K., et al. (2019). Effects of cucumber root exudates components on soil bacterial community structure and abundance. Allelopath. J. 48, 167–174. doi: 10.26651/allelo.j/2019-48-2-1252
Kuppe, C. W., Schnepf, A., von Lieres, E., Watt, M., and Postma, J. A. (2022). Rhizosphere models: their concepts and application to plant-soil ecosystems. Plant Soil 474, 17–55. doi: 10.1007/s11104-021-05201-7
Lewis, W. H., Tahon, G., Geesink, P., Sousa, D. Z., and Ettema, T. J. G. (2021). Innovations to culturing the uncultured microbial majority. Nat. Rev. Microbiol. 19, 225–240. doi: 10.1038/s41579-020-00458-8
Li, M., Han, L., He, C., Li, X., and He, X. L. (2024). The promotion of dark septate endophytes on the performance and active ingredients accumulation of Astragalus mongholicus under cadmium stress. Agronomy 14:1801. doi: 10.3390/agronomy14081801
Li, J. J., Zheng, Y. M., Yan, J. X., Li, H. J., and He, J. Z. (2013). Succession of plant and soil microbial communities with restoration of abandoned land in the loess plateau. China. J. Soils Sediments 13, 760–769. doi: 10.1007/s11368-013-0652-z
Liao, J. Q., Wang, J., and Huang, Y. (2015). Bacterial community features are shaped by geographic location, physicochemical properties, and oil contamination of soil in main oil fields of China. Microb. Ecol. 70, 380–389. doi: 10.1007/s00248-015-0572-0
Liu, L. L., Chen, Z. Y., Su, Z., Li, S., Ali, A., Cai, Z. C., et al. (2023). Soil pH indirectly determines Ralstonia solanacearum colonization through its impacts on microbial networks and specific microbial groups. Plant Soil 482, 73–88. doi: 10.1007/s11104-022-05671-3
Liu, S., Lin, Y. H., Murphy, A., Anderson, J., Walker, N., Lynn, D. G., et al. (2020). Mapping reaction-diffusion networks at the plant wound site with pathogens. Front. Plant Sci. 11:1074. doi: 10.3389/fpls.2020.01074
Liu, Y. X., Qin, Y., Chen, T., Lu, M. P., Qian, X. B., Guo, X. X., et al. (2021). A practical guide to amplicon and metagenomic analysis of microbiome data. Protein Cell 12, 315–330. doi: 10.1007/s13238-020-00724-8
Liu, Z., Wang, Y., Pan, X., Ge, Q., Ma, Q., Li, Q., et al. (2017). Identification of fungal communities associated with the biodeterioration of waterlogged archeological wood in a Han dynasty tomb in China. Front. Microbiol. 8:1633. doi: 10.3389/fmicb.2017.01633
Magoc, T., and Salzberg, S. L. (2011). FLASH: fast length adjustment of short reads to improve genome assemblies. Bioinformatics 27, 2957–2963. doi: 10.1093/bioinformatics/btr507
Manzanera, M., Narváez-Reinaldo, J. J., García-Fontana, C., Vílchez, J. I., and González-López, J. (2015). Genome sequence of Arthrobacter koreensis 5J12A, a plant growth-promoting and desiccation-tolerant strain. Genome Announce. 3, e00648–e00615. doi: 10.1128/genomeA.00648-15
Navone, R. (1964). Direct ultraviolet spectrophotometric determination of nitrate in water. Anal. Chem. 36, 629–632.
Niu, B., Paulson, J. N., Zheng, X., and Kolter, R. (2017). Simplified and representative bacterial community of maize roots. PNAS 114, E2450–E2459. doi: 10.1073/pnas.1616148114
Ortega-Villaizán, A. G., King, E., Patel, M. K., Rodríguez-Dobreva, E., González-Teuber, M., Ramos, P., et al. (2025). Identification of a drought stress response module in tomato plants commonly induced by fungal endophytes that confer increased drought tolerance. Plant Mol. Biol. 115:7. doi: 10.1007/s11103-024-01532-y
Oukala, N., Aissat, K., and Pastor, V. (2021). Bacterial endophytes: the hidden actor in plant immune responses against biotic stress. Plants 10:1012. doi: 10.3390/plants10051012
Pang, Y., Wu, B., Jia, X., and Xie, S. (2022). Wind-proof and sand-fixing effects of Artemisia ordosica with different coverages in the mu us Sandy land, northern China. J. Arid. Land 14, 877–893. doi: 10.1007/s40333-022-0070-4
Poria, V., Debiec-Andrzejewska, K., Fiodor, A., Lyzohub, M., Ajijah, N., Singh, S., et al. (2022). Plant growth-promoting bacteria (PGPB) integrated phytotechnology: a sustainable approach for remediation of marginal lands. Front. Plant Sci. 13:866. doi: 10.3389/fpls.2022.999866
Quast, C., Pruesse, E., Yilmaz, P., Gerken, J., Schweer, T., Yarza, P., et al. (2013). The SILVA ribosomal RNA gene database project: improved data processing and web-based tools. Nucleic Acids Res. 41, D590–D596. doi: 10.1093/nar/gks1219
Rainey, F. A., Ray, K., Ferreira, M., Gatz, B. Z., Nobre, M. F., Bagaley, D., et al. (2005). Extensive diversity of ionizing-radiation-resistant bacteria recovered from Sonoran Desert soil and description of nine new species of the genus Deinococcus obtained from a single soil sample. Appl. Environ. Microbiol. 71, 5225–5235. doi: 10.1128/aem.71.9.5225-5235.2005
Reuter, J. A., Spacek, D. V., and Snyder, M. P. (2015). High-throughput sequencing technologies. Mol. Cell 58, 586–597. doi: 10.1016/j.molcel.2015.05.004
Saygin, H., Guven, K., Cetin, D., and Sahin, N. (2024). Polyphasic characterization and genomic insights into Nocardioides turkmenicus sp. nov. isolated from a desert soil. Anton Leeuw. Int. J. G. 117:25. doi: 10.1007/s10482-023-01920-2
Schmitz, L., Yan, Z., Schneijderberg, M., de Roij, M., Pijnenburg, R., Zheng, Q., et al. (2022). Synthetic bacterial community derived from a desert rhizosphere confers salt stress resilience to tomato in the presence of a soil microbiome. ISME J. 16, 1907–1920. doi: 10.1038/s41396-022-01238-3
Stackebrandt, E., and Goodfellow, M. (1991). “Nucleic acid techniques in bacterial systematics” in 16S rRNA sequencing primers. eds. E. Stackebrandt and M. Goodfellow (New York, NY, USA: Wiley), 132–136.
Upadhyay, S. K., Srivastava, A. K., Rajput, V. D., Chauhan, P. K., Bhojiya, A. A., Jain, D., et al. (2022). Root exudates: mechanistic insight of plant growth promoting rhizobacteria for sustainable crop production. Front. Microbiol. 13:916488. doi: 10.3389/fmicb.2022.916488
Wagner, M. R., Lundberg, D. S., del Rio, T. G., Tringe, S. G., Dangl, J. L., and Mitchell-Olds, T. (2016). Host genotype and age shape the leaf and root microbiomes of a wild perennial plant. Nat. Commun. 7:12151. doi: 10.1038/ncomms12151
Walkley, A., and Black, I. A. (1934). An examination of the Degtjareff method for determining soil organic matter, and a proposed modification of the chromic acid titration method. Soil Sci. 37, 29–38. doi: 10.1097/00010694-193401000-00003
Wang, X. L., Wang, M. X., Xie, X. G., Guo, S. Y., Zhou, Y., Zhang, X. B., et al. (2020). An amplification-selection model for quantified rhizosphere microbiota assembly. Sci. Bull. 65, 983–986. doi: 10.1016/j.scib.2020.03.005
Wang, W. B., Yang, Y. Y., Li, J. G., Bu, P. T., Lu, A. J., Wang, H., et al. (2024). Consecutive fertilization-promoted soil nutrient availability and altered rhizosphere bacterial and bulk fungal community composition. Forests 15:514. doi: 10.3390/f15030514
Wesley, R. D., Swaminathan, B., and Stadelman, W. J. (1983). Isolation and enumeration of Campylobacter jejuni from poultry products by a selective enrichment method. Appl. Environ. Microbiol. 46, 1097–1102. doi: 10.1128/aem.46.5.1097-1102.1983
Williams, R. J., Howe, A., and Hofmockel, K. S. (2014). Demonstrating microbial co-occurrence pattern analyses within and between ecosystems. Front. Microbiol. 5:358. doi: 10.3389/fmicb.2014.00358
Xia, X., Wei, Q., Wu, H., Chen, X., Xiao, C., Ye, Y., et al. (2024). Bacillus species are core microbiota of resistant maize cultivars that induce host metabolic defense against corn stalk rot. Microbiome 12:156. doi: 10.1186/s40168-024-01887-w
Xiao, X., Chen, W., Zong, L., Yang, J., Jiao, S., Lin, Y., et al. (2017). Two cultivated legume plants reveal the enrichment process of the microbiome in the rhizocompartments. Mol. Ecol. 26, 1641–1651. doi: 10.1111/mec.14027
Xu, D., and Zhang, X. (2021). Multi-scenario simulation of desertification in North China for 2030. Land Degrad. Dev. 32, 1060–1074. doi: 10.1002/ldr.3746
Yang, N., Li, Y., Lin, L., Niu, L. H., Zhang, W. L., and Wang, L. F. (2024). Transition of organic matter from allochthonous to autochthonous alters benthic nutrient dynamics via microbial food webs. Sci. Total Environ. 916:170186. doi: 10.1016/j.scitotenv.2024.170186
Yergeau, E., Bell, T. H., Champagne, J., Maynard, C., Tardif, S., Tremblay, J., et al. (2015). Transplanting soil microbiomes leads to lasting effects on willow growth, but not on the rhizosphere microbiome. Front. Microbiol. 6:1436. doi: 10.3389/fmicb.2015.01436
Yoon, S.-H., Ha, S.-M., Kwon, S., Lim, J., Kim, Y., Seo, H., et al. (2017). Introducing EzBioCloud: a taxonomically united database of 16S rRNA gene sequences and whole-genome assemblies. Int. J. Syst. Evol. Microbiol. 67, 1613–1617. doi: 10.1099/ijsem.0.001755
Yovchevska, L., Miteva-Staleva, J., Dishliyska, V., Stoyancheva, G., Gocheva, Y., Abrashev, R., et al. (2025). Response to salt stress of the halotolerant filamentous fungus Penicillium chrysogenum P 13. Molecules 30:1196. doi: 10.3390/molecules30061196
Zhang, X. (1994). The ecological background of mu us Sandy land and the principle and optimization model of grassland construction. J. Plant Ecol., 1–16.
Zhang, J., Liu, Y. X., Guo, X., Qin, Y., Garrido-Oter, R., Schulze-Lefert, P., et al. (2021). High-throughput cultivation and identification of bacteria from the plant root microbiota. Nat. Protoc. 16, 988–1012. doi: 10.1038/s41596-020-00444-7
Keywords: Artemisia ordosica Krasch., root zone microbiota, high-throughput sequencing, successional stages, land phytoremediation
Citation: Zhu W, Han S, Cheng Y, Yu Z, Zhao G and He X (2025) Root zone microbial communities of Artemisia ordosica Krasch. at different successional stages in Mu US Sandy Land: a metagenomic perspective with culturomics insights. Front. Microbiol. 16:1585700. doi: 10.3389/fmicb.2025.1585700
Edited by:
Wen-Jun Li, Sun Yat-sen University, ChinaReviewed by:
Xiaowei Zheng, Chinese Academy of Sciences (CAS), ChinaLing Hao, George Washington University, United States
Copyright © 2025 Zhu, Han, Cheng, Yu, Zhao and He. This is an open-access article distributed under the terms of the Creative Commons Attribution License (CC BY). The use, distribution or reproduction in other forums is permitted, provided the original author(s) and the copyright owner(s) are credited and that the original publication in this journal is cited, in accordance with accepted academic practice. No use, distribution or reproduction is permitted which does not comply with these terms.
*Correspondence: Xiangwei He, aGV4aWFuZ3dlaUBiamZ1LmVkdS5jbg==
†These authors have contributed equally to this work and share first authorship