- 1Department of Neurosurgery, Medical College of Georgia, Augusta University, Augusta, GA, United States
- 2Russell H. Morgan Department of Radiology and Radiological Science, Johns Hopkins School of Medicine, Johns Hopkins University, Baltimore, MD, United States
- 3Laboratory of Tumor Angiogenesis, Georgia Cancer Center, Department of Biochemistry and Molecular Biology, Augusta University, Augusta, GA, United States
Intracerebral hemorrhage (ICH) is a fatal stroke subtype with significant public health impact. Although neuroinflammation is a leading cause of neurological deficits after ICH, no imaging tool is currently available to monitor brain inflammation in ICH patients. Given the role of TSPO in neuroinflammation, herein we investigate whether a second-generation TSPO ligand, [125 I]IodoDPA-713 can be used to monitor the changes in TSPO expression in a preclinical model of intracerebral hemorrhage. Male CD1 mice were subjected to ICH/Sham. The brain sections, collected at different time points were incubated with [125 I]IodoDPA-713 and the brain uptake of [125 I]IodoDPA-713 was estimated using autoradiography. The specificity of [125 I]IodoDPA-713 binding was confirmed by a competitive displacement study with an unlabeled TSPO ligand, PK11195. [125 I]IodoDPA-713 binding was higher in the ipsilateral striatum with an enhanced binding observed in the peri-hematomal brain region after ICH, whereas the brain sections from sham as well as contralateral brain areas of ICH exhibited marginal binding of [125 I]IodoDPA-713. PK11195 completely reversed the [125 I] IodoDPA-713 binding to brain sections suggesting a specific TSPO-dependent binding of [125 I]IodoDPA-713 after ICH. This was further confirmed with immunohistochemistry analysis of adjacent sections, which revealed a remarkable expression of TSPO in the areas of high [125 I]IodoDPA-713 binding after ICH. The specific as well as enhanced binding of [125 I]IodoDPA-713 to the ipsilateral brain areas after ICH as assessed by autoradiography analysis provides a strong rationale for testing the applicability of [125 I]IodoDPA-713 for non-invasive neuroimaging in preclinical models of ICH.
Introduction
Intracerebral hemorrhage (ICH) is a detrimental subtype of stroke caused by bleeding within the brain tissue itself. ICH accounts for 10–20% of strokes and has a fatality rate of 40 and 54% at 30 days and 1 year, respectively (An et al., 2017). Notably, there is no substantial change in fatality rate over the last 40 years (An et al., 2017). Moreover, the survivors of ICH often exhibit neurological deficits partly because of the secondary brain insults caused by the released blood components in the brain parenchyma (Elliott and Smith, 2010; Babu et al., 2012).
The pathophysiology of ICH includes both primary as well as secondary brain damage. The primary damage, occurring within minutes to hours after the initial brain hemorrhage is mostly caused by the mass effect of the hematoma. In contrast, the secondary brain damage develops from hours to days after the initial brain insult and can lead to severe neurological disability(Aronowski and Zhao, 2011; Belur et al., 2013). The secondary brain damage is mainly attributed to the inflammatory and oxidative responses to released blood components and associated neurotoxicity (Aronowski and Zhao, 2011; Belur et al., 2013). Neuroinflammation, often characterized by microglial activation, plays a critical role in the pathophysiology of ICH, and the brain inflammatory response correlates with the expansion of hematoma, neurodegeneration, and poor functional outcomes (Platt et al., 1998; Hickenbottom et al., 1999; Leira et al., 2004; Zhao et al., 2007). Though neuroinflammation is a leading cause of neurological deficits (Yang et al., 1994; Wagner et al., 1996; Xi et al., 2006; Aronowski and Zhao, 2011; Belur et al., 2013; Zheng et al., 2016), no imaging tool is currently available to monitor brain inflammation in ICH patients. Currently, the brain inflammatory response after ICH can only be ascertained by histological examination of brain tissue sections obtained from invasive procedures like biopsy. Therefore, the development and validation of an in vivo biomarker of microglial activation is a major advancement to monitor brain pathology and thereby to assess the effectiveness of therapeutic interventions after ICH. To this end, we employed autoradiography studies with a second-generation TSPO ligand, [125 I]IodoDPA-713 as it could lay a strong platform for non-invasive neuroimaging studies after ICH.
Can TSPO be Targeted for Neuroimaging After ICH?
Emerging evidences indicate a critical role of an evolutionarily well-conserved mitochondrial outer membrane protein, TSPO (18 kDa translocator protein) in neuroinflammation (Soustiel et al., 2008, 2011; Barron et al., 2013; Daugherty et al., 2013). Notably, TSPO has gained immense interest as a therapeutic target for neurologic disorders and small-molecule TSPO ligands improved functional recovery in a variety of the neurologic disorders (Soustiel et al., 2008, 2011; Barron et al., 2013; Daugherty et al., 2013). One of the key mechanisms underlying the neuroprotective effects has been highlighted as the stimulation of mitochondrial steroid synthesis with a concomitant reduction in inflammatory response (Serra et al., 1999; Verleye et al., 2005; Mitro et al., 2012; Barron et al., 2013; Zhang et al., 2014; do Rego et al., 2015). However, recent studies with transgenic mouse models demonstrate that TSPO is not essential for steroidogenesis (Banati et al., 2014; Morohaku et al., 2014; Tu et al., 2014), suggesting an elusive role of TSPO in normal physiology and neuropathology despite its augmented expression in brain inflammatory cells.
We recently demonstrated for the first time the profound induction of TSPO after ICH in comparison to sham (Bonsack et al., 2016). Further, TSPO induction after ICH was mostly confined to the peri-hematomal brain region and was mainly observed in Iba1 positive microglia/macrophage, the inflammatory cells of the central nervous system (CNS) (Bonsack et al., 2016). Notably, a profound up regulation of TSPO was observed on day 3 and day 5-post injury and the induction of TSPO after ICH mirrored the microglial activation profile after ICH (Bonsack et al., 2016). Further, the induction of TSPO paralleled and co-localized with the expression of proinflammatory and anti-inflammatory microglial markers, CD16/32 and CD206, respectively further emphasizing a possible functional role of TSPO in brain inflammatory responses after ICH (Bonsack et al., 2016). Though the precise role of TSPO in microglial/macrophage functions after brain pathology remains largely unknown, the radio labeled ligands of TSPO are widely being tested for its ability to assess brain inflammation (Callaghan et al., 2015; Damont et al., 2015; Liu et al., 2015; Loth et al., 2016; Alam et al., 2017; Crawshaw and Robertson, 2017; Fujita et al., 2017; Ishikawa et al., 2018). However, until very recently no such effort has been made after ICH. To this end, a study comprising of five ICH patients documented for the first time the feasibility of employing [11C] labeled first generation TSPO ligand, [11C]-(R)-PK11195 in monitoring microglial activation after ICH (Abid et al., 2017). However, given the small sample size of the aforementioned study (Abid et al., 2017), future work is highly warranted establishing the applicability of TSPO ligands for neuroimaging applications after ICH.
Does [125 I]IodoDPA-713 Confer a Promising Tool for Tracking Neuroinflammatory Responses After ICH?
DPA-713 (N,N-diethyl-2-[2-(4-[methoxyphenyl)-5,7-dimethyl -pyrazolo-[1,5-α]pyrimidin-3-yl]-acetamide), a pyrazolo-pyrimidine, is a second generation TSPO ligand and less lipophilic in comparison to its previous generation counterpart, PK11195 (Endres et al., 2009). Furthermore, DPA-713 has twice the affinity for TSPO (Wang et al., 2009) in comparison to PK11195. Thereby, the use of radio labeled-DPA-713 may confer reduced non-specific binding. Consistently, PET (Positron emission tomography) imaging performed with [11C]DPA-713 in humans resulted in higher signal-to-noise ratio in comparison to [11C]-PK11195 (Doorduin et al., 2009; Endres et al., 2009). [125 I]IodoDPA-713, a radio ligand of TSPO, has been previously used to detect the expression of TSPO in an in vivo mouse model of tuberculosis and [125 I]IodoDPA-713 SPECT activity correlated with lung inflammation after tuberculosis (Wang et al., 2009). [125 I] labeled radio ligands have relatively longer half-lives (half-life of [125 I] is ≈ 2 months) permitting prolonged dynamic functional studies. In contrast, [11C] labeled radio ligands are often difficult to handle due to the short half-life of the radio nucleotide (20 min) and limited to centers having particle accelerators like, cyclotron for its synthesis. Though, a very recent study demonstrated the use of [125 I]IodoDPA-713 in a neuropathological condition, Sandhoff disease(Loth et al., 2016), it is largely unknown whether [125 I] IodoDPA-713 can be used to detect the brain expression of TSPO after ICH.
Herein, we investigate whether [125 I]IodoDPA-713 can be used to monitor the changes in TSPO expression in a preclinical model of intracerebral hemorrhage. To evaluate the uptake of brain sections with [125 I]IodoDPA-713, sham or ICH was induced in CD1 male mice as described previously (Bonsack et al., 2016) and on day 3 and day 5-post surgery, the animals were euthanized and the fresh frozen sections were used for autoradiography studies as detailed in Supplementary Methods. [125 I]IodoDPA-713 binding was found to be higher in the striatum after ICH in comparison to sham and the binding of [125 I]IodoDPA-713 was mostly confined to the peri-hematomal brain areas (Figures 1A,B). Notably, consistent with very low expression of TSPO in uninjured or intact brain (Bonsack et al., 2016), sham as well as contralateral brain areas of ICH exhibited marginal binding of [125 I]IodoDPA-713 (Figure 1A). Quantitative analysis further confirmed significant induction in [125 I]IodoDPA-713 uptake in the peri-hematomal brain areas after ICH in comparison to sham (Figure 1C). More importantly, TSPO ligand, PK11195 completely inhibited [125 I]IodoDPA-713 binding to brain sections suggesting a specific TSPO-dependent binding of [125 I]IodoDPA-713 after ICH (Figure 1A). This was further confirmed with immunohistochemistry analysis of adjacent sections, which revealed a remarkable expression of TSPO in the areas of high [125 I]IodoDPA-713 binding after ICH (Figures 1D,E). Notably, brain sections from post injury days, 3 and 5 exhibited maximal microglial/macrophage activation and/or TSPO expression after ICH (Bonsack et al., 2016). Further, in contrast to other brain pathologies, TSPO induction after ICH is found predominantly in Iba1 positive activated microglia/macrophages (Bonsack et al., 2016) making it an ideal molecular candidate to track microglial/macrophage associated changes after ICH.
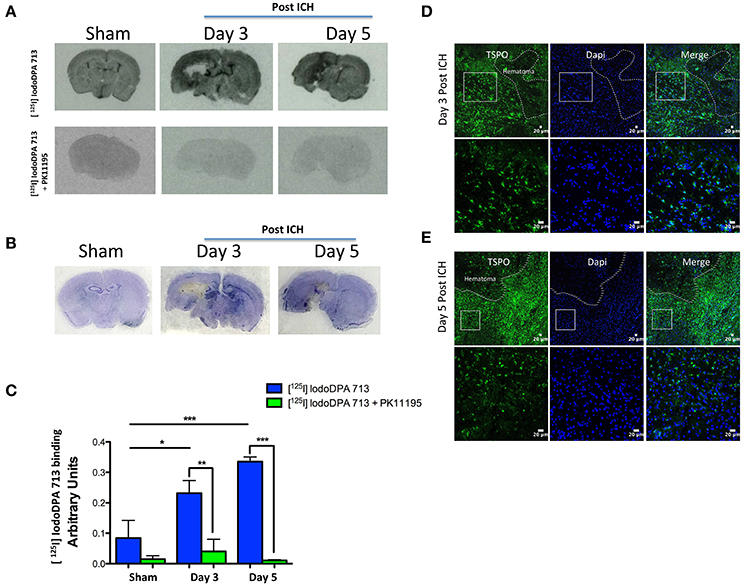
Figure 1. (A) Representative autoradiography images demonstrating the binding of [125 I]IodoDPA-713 to the brain sections from sham or ICH (top panel). PK11195 inhibited the binding of [125 I]IodoDPA-713 to the brain sections (bottom panel) (n = 3 mice/group). (B) Brain sections adjacent to the ones as depicted in (A) were subjected to cresyl violet staining and it demonstrates that the [125 I]IodoDPA-713 uptake was observed mostly in the ipsilateral striatum after ICH (n = 3 mice/group). (C) The quantification of [125 I]IodoDPA-713 binding to brain sections as assessed by estimating the optical density using image J (NIH, USA). *p < 0.05, **p < 0.01, ***p < 0.001 vs. control (n = 3 mice/group). Brain sections (n = 3 mice/group) were subjected to immunostaining further illustrates that the [125 I] IodoDPA-713 binding was observed in brain regions with enhanced TSPO expression after 3 days post-ICH (D) and 5 days post-ICH (E) and the dotted line demarcates the hematomal and peri- hematomal brain regions.
Microglia, the resident neuroimmune cells are broadly distributed throughout the brain (Lawson et al., 1990). Microglia comprise ≈5–20% of the total glial population of the CNS and are the first non-neuronal cells to respond to a brain injury via activation (Wang and Dore, 2007; Xiong and Yang, 2015). While some microglial functions are beneficial, activated microglia also play a detrimental role after ICH (Wang and Dore, 2007; Xiong and Yang, 2015). Notably, the activated microglia are regarded as the key cellular regulators of brain inflammation after ICH based on their local release of cytokines, chemokines, prostaglandins, and reactive oxygen species (Melton et al., 2003; Nakanishi, 2003; Aronowski and Hall, 2005; Wang and Dore, 2007; Zhang et al., 2009) and the neuroinflammatory response correlates with blood brain barrier damage, cerebral edema, hematoma expansion, neurological deterioration, and poor functional outcomes (Platt et al., 1998; Hickenbottom et al., 1999; Leira et al., 2004; Zhao et al., 2007). Furthermore, neuroimmune response after ICH also regulates the brain recruitment of blood-derived monocytes/macrophages (Tessier et al., 1997; Shiratori et al., 2010; Starossom et al., 2012) and a massive infiltration of macrophages in the peri-hematomal region occurs after ICH (Min et al., 2016; Chang et al., 2017). Though the precise functional role of microglia and infiltrating macrophages after a brain injury is largely controversial, it is postulated that a key role of activated microglia and macrophages after ICH is to phagocytose the cellular debris and blood components left in the brain after hemorrhage, a process called hematoma resolution, which is vital for the functional recovery. Consistently, it is reported that brain-infiltrating macrophages after ICH are polarized to the anti-inflammatory M2 phenotype and contribute to neurological recovery after ICH (Min et al., 2016; Chang et al., 2017). Further, a human ICH study employing microarray analysis demonstrated significant up-regulation of both pro- and anti-inflammatory genes in the peri-hematomal brain region (Carmichael et al., 2008). Of note, TSPO expression is observed in both activated microglia and brain infiltrating macrophages after ICH (Li et al., 2017), and the induction of TSPO temporarily correlated with microglia activation, which persists for a long time after ICH (Wang, 2010; Yabluchanskiy et al., 2010; Bonsack et al., 2016; Li et al., 2017). In addition, our in vitro studies revealed a negative regulatory role of TSPO in the release of proinflammatory cytokines from murine macrophages(Bonsack et al., 2016), together making it an ideal candidate to possibly track the functional changes associated with microglia/macrophage and thereby neuroinflammatory responses after ICH. Along these lines, the autoradiography study results as outlined above provide a strong rationale for testing the applicability of [125 I] labeled DPA-713 for non-invasive neuroimaging studies after ICH.
Conclusions
ICH is a fatal stroke subtype with significant public health impact. Though neuroinflammation plays a critical role in ICH pathophysiology, no imaging tool is currently available to track activation-associated microglial/macrophage changes after ICH. Along these lines, TSPO expression is observed in both activated microglia and brain infiltrating macrophages after ICH (Li et al., 2017), and the induction of TSPO temporarily correlated with microglia activation, which persists for a long time after ICH (Wang, 2010; Yabluchanskiy et al., 2010; Bonsack et al., 2016; Li et al., 2017). Given the clinical applicability of [125 I] labeled DPA-713 coupled with its increased binding to the peri-hematomal region in a TSPO-dependent manner, in comparison to other brain regions after ICH as demonstrated herein, future studies need to be conducted testing its potential to detect the microglial/macrophage activation after ICH. Further, given the complex pathophysiology of ICH, the applicability of [125 I] IodoDPA-713 for non- invasive neuroimaging (SPECT) both in the acute as well as sub acute phases of ICH needs detailed evaluation employing preclinical animal models of ICH as it would lay a strong foundation for future clinical applications.
Ethics Statement
Animal studies (protocol #2012-0459) were reviewed and approved by the Committee on Biosafety, Animal Care and Use and Radiation safety for Research and Education at Augusta University, in compliance with NIH and USDA guidelines. The protocols with regard to the synthesis of [125 I]IodoDPA-713 was approved by the Johns Hopkins University, Biosafety and Radiation Safety Committees.
Author Contributions
FB carried out the immunohistochemical and autoradiography studies and participated in the data analysis. MP and CF provided [125 I]IodoDPA-713 for the studies. CA and AA participated in timely project discussions. SS-R conceived and designed the experiments. SS-R also conducted the animal surgeries, autoradiography studies and data analysis and drafted the manuscript. All authors read and approved the final manuscript.
Conflict of Interest Statement
The authors declare that the research was conducted in the absence of any commercial or financial relationships that could be construed as a potential conflict of interest.
Acknowledgments
This work was supported by a grant from the American Heart Association (14SDG18730034) to SS-R.
Supplementary Material
The Supplementary Material for this article can be found online at: https://www.frontiersin.org/articles/10.3389/fnins.2018.00066/full#supplementary-material
Abbreviations
TSPO, 18 kDa translocator protein; ICH, Intracerebral Hemorrhage; PBS, Phosphate-buffered Saline; Iba1, Ionized calcium binding adaptor molecule 1; CD 16/32, Cluster of Differentiation 16/32; Dapi,4′,6-diamidino-2-phenylindole; SE, Standard Error; CNS, Central Nervous System; DPA-713, N,N-diethyl-2-[2-(4[methoxy-phenyl)-5,7-dimethyl- pyrazolo[1,5-a]pyrimidin-3-yl]-acetamide.
References
Abid, K. A., Sobowale, O. A., Parkes, L. M., Naish, J., Parker, G. J. M., du Plessis, D., et al. (2017). Assessing inflammation in acute intracerebral hemorrhage with PK11195 PET and dynamic contrast-enhanced MRI. J. Neuroimaging. doi: 10.1111/jon.12477. [Epub ahead of print].
Alam, M. M., Lee, J., and Lee, S. Y. (2017). Recent progress in the development of TSPO PET ligands for neuroinflammation imaging in neurological diseases. Nucl. Med. Mol. Imaging 51, 283–296. doi: 10.1007/s13139-017-0475-8
An, S. J., Kim, T. J., and Yoon, B. W. (2017). Epidemiology, risk factors, and clinical features of intracerebral hemorrhage: an update. J. Stroke 19, 3–10. doi: 10.5853/jos.2016.00864
Aronowski, J., and Hall, C. E. (2005). New horizons for primary intracerebral hemorrhage treatment: experience from preclinical studies. Neurol. Res. 27, 268–279. doi: 10.1179/016164105X25225
Aronowski, J., and Zhao, X. (2011). Molecular pathophysiology of cerebral hemorrhage: secondary brain injury. Stroke 42, 1781–1786. doi: 10.1161/STROKEAHA.110.596718
Babu, R., Bagley, J. H., Di, C., Friedman, A. H., and Adamson, C. (2012). Thrombin and hemin as central factors in the mechanisms of intracerebral hemorrhage-induced secondary brain injury and as potential targets for intervention. Neurosurg. Focus 32, E8. doi: 10.3171/2012.1.FOCUS11366
Banati, R. B., Middleton, R. J., Chan, R., Hatty, C. R., Kam, W. W., Quin, C., et al. (2014). Positron emission tomography and functional characterization of a complete PBR/TSPO knockout. Nat. Commun. 5:5452. doi: 10.1038/ncomms6452
Barron, A. M., Garcia-Segura, L. M., Caruso, D., Jayaraman, A., Lee, J. W., Melcangi, R. C., et al. (2013). Ligand for translocator protein reverses pathology in a mouse model of Alzheimer's disease. J. Neurosci. 33, 8891–8897. doi: 10.1523/JNEUROSCI.1350-13.2013
Belur, P. K., Chang, J. J., He, S., Emanuel, B. A., and Mack, W. J. (2013). Emerging experimental therapies for intracerebral hemorrhage: targeting mechanisms of secondary brain injury. Neurosurg. Focus 34, E9. doi: 10.3171/2013.2.FOCUS1317
Bonsack, F., Alleyne, C. H. Jr., and Sukumari-Ramesh, S. (2016). Augmented expression of TSPO after intracerebral hemorrhage: a role in inflammation?. J. Neuroinflammation 13, 151. doi: 10.1186/s12974-016-0619-2
Callaghan, P. D., Wimberley, C. A., Rahardjo, G. L., Berghofer, P. J., Pham, T. Q., Jackson, T., et al. (2015). Comparison of in vivo binding properties of the 18-kDa translocator protein (TSPO) ligands [(18)F]PBR102 and [(18)F]PBR111 in a model of excitotoxin-induced neuroinflammation. Eur. J. Nucl. Med. Mol. Imaging 42, 138–151. doi: 10.1007/s00259-014-2895-3
Carmichael, S. T., Vespa, P. M., Saver, J. L., Coppola, G., Geschwind, D. H., Starkman, S., et al. (2008). Genomic profiles of damage and protection in human intracerebral hemorrhage. J. Cereb. Blood Flow Metab. 28, 1860–1875. doi: 10.1038/jcbfm.2008.77
Chang, C. F., Wan, J., Li, Q., Renfroe, S. C., Heller, N. M., and Wang, J. (2017). Alternative activation-skewed microglia/macrophages promote hematoma resolution in experimental intracerebral hemorrhage. Neurobiol. Dis. 103, 54–69. doi: 10.1016/j.nbd.2017.03.016
Crawshaw, A. A., and Robertson, N. P. (2017). The role of TSPO PET in assessing neuroinflammation. J. Neurol. 264, 1825–1827. doi: 10.1007/s00415-017-8565-1
Damont, A., Medran-Navarrete, V., Cacheux, F., Kuhnast, B., Pottier, G., Bernards, N., et al. (2015). Novel Pyrazolo[1,5-a]pyrimidines as translocator protein 18 kDa (TSPO) ligands: synthesis, in vitro biological evaluation, [(18)F]-labeling, and in vivo neuroinflammation PET images. J. Med. Chem. 58, 7449–7464. doi: 10.1021/acs.jmedchem.5b00932
Daugherty, D. J., Selvaraj, V., Chechneva, O. V., Liu, X. B., Pleasure, D. E., and Deng, W. (2013). A TSPO ligand is protective in a mouse model of multiple sclerosis. EMBO Mol. Med. 5, 891–903. doi: 10.1002/emmm.201202124
do Rego, J. L., Vaudry, D., and Vaudry, H. (2015). The non-benzodiazepine anxiolytic drug etifoxine causes a rapid, receptor-independent stimulation of neurosteroid biosynthesis. PLoS ONE 10:e0120473. doi: 10.1371/journal.pone.0120473
Doorduin, J., Klein, H. C., Dierckx, R. A., James, M., Kassiou, M., and de Vries, E. F. (2009). [11C]-DPA-713 and [18F]-DPA-714 as new PET tracers for TSPO: a comparison with [11C]-(R)-PK11195 in a rat model of herpes encephalitis. Mol. Imaging Biol. 11, 386–398. doi: 10.1007/s11307-009-0211-6
Elliott, J., and Smith, M. (2010). The acute management of intracerebral hemorrhage: a clinical review. Anesth. Analg. 110, 1419–1427. doi: 10.1213/ANE.0b013e3181d568c8
Endres, C. J., Pomper, M. G., James, M., Uzuner, O., Hammoud, D. A., Watkins, C. C., et al. (2009). Initial evaluation of 11C-DPA-713, a novel TSPO PET ligand, in humans. J. Nucl. Med. 50, 1276–1282. doi: 10.2967/jnumed.109.062265
Fujita, M., Kobayashi, M., Ikawa, M., Gunn, R. N., Rabiner, E. A., Owen, D. R., et al. (2017). Comparison of four (11)C-labeled PET ligands to quantify translocator protein 18 kDa (TSPO) in human brain: (R)-PK11195, PBR28, DPA-713, and ER176-based on recent publications that measured specific-to-non-displaceable ratios. EJNMMI Res. 7, 84. doi: 10.1186/s13550-017-0334-8
Hickenbottom, S. L., Grotta, J. C., Strong, R., Denner, L. A., and Aronowski, J. (1999). Nuclear factor-kappaB and cell death after experimental intracerebral hemorrhage in rats. Stroke 30, 2472–2477. discussion: 2477–2478. doi: 10.1161/01.STR.30.11.2472
Ishikawa, A., Tokunaga, M., Maeda, J., Minamihisamatsu, T., Shimojo, M., Takuwa, H., et al. (2018). In vivo visualization of tau accumulation, microglial activation, and brain atrophy in a mouse model of tauopathy rTg4510. J. Alzheimers Dis. 61, 1037–1052. doi: 10.3233/JAD-170509
Lawson, L. J., Perry, V. H., Dri, P., and Gordon, S. (1990). Heterogeneity in the distribution and morphology of microglia in the normal adult mouse brain. Neuroscience 39, 151–170. doi: 10.1016/0306-4522(90)90229-W
Leira, R., Davalos, A., Silva, Y., Gil-Peralta, A., Tejada, J., Garcia, M., et al. (2004). Early neurologic deterioration in intracerebral hemorrhage: predictors and associated factors, Neurology 63, 461–467. doi: 10.1212/01.WNL.0000133204.81153.AC
Li, M., Ren, H., Sheth, K. N., Shi, F. D., and Liu, Q. (2017). A TSPO ligand attenuates brain injury after intracerebral hemorrhage. FASEB J. 31, 3278–3287. doi: 10.1096/fj.201601377RR
Liu, B., Le, K. X., Park, M. A., Wang, S., Belanger, A. P., Dubey, S., et al. (2015). In vivo detection of age- and disease-related increases in neuroinflammation by 18F-GE180 TSPO MicroPET imaging in wild-type and Alzheimer's Transgenic mice. J. Neurosci. 35, 15716–15730. doi: 10.1523/JNEUROSCI.0996-15.2015
Loth, M. K., Choi, J., McGlothan, J. L., Pletnikov, M. V., Pomper, M. G., and Guilarte, T. R. (2016). TSPO in a murine model of Sandhoff disease: presymptomatic marker of neurodegeneration and disease pathophysiology. Neurobiol. Dis. 85, 174–186. doi: 10.1016/j.nbd.2015.11.001
Melton, L. M., Keith, A. B., Davis, S., Oakley, A. E., Edwardson, J. A., and Morris, C. M. (2003). Chronic glial activation, neurodegeneration, and APP immunoreactive deposits following acute administration of double-stranded RNA. Glia 44, 1–12. doi: 10.1002/glia.10276
Min, H., Jang, Y. H., Cho, I. H., Yu, S. W., and Lee, S. J. (2016). Alternatively activated brain-infiltrating macrophages facilitate recovery from collagenase-induced intracerebral hemorrhage. Mol. Brain 9, 42. doi: 10.1186/s13041-016-0225-3
Mitro, N., Cermenati, G., Giatti, S., Abbiati, F., Pesaresi, M., Calabrese, D., et al. (2012). LXR and TSPO as new therapeutic targets to increase the levels of neuroactive steroids in the central nervous system of diabetic animals. Neurochem. Int. 60, 616–621. doi: 10.1016/j.neuint.2012.02.025
Morohaku, K., Pelton, S. H., Daugherty, D. J., Butler, W. R., Deng, W., and Selvaraj, V. (2014). Translocator protein/peripheral benzodiazepine receptor is not required for steroid hormone biosynthesis. Endocrinology 155, 89–97. doi: 10.1210/en.2013-1556
Nakanishi, H. (2003). Microglial functions and proteases. Mol. Neurobiol. 27, 163–176. doi: 10.1385/MN:27:2:163
Platt, N., da Silva, R. P., and Gordon, S. (1998). Recognizing death: the phagocytosis of apoptotic cells. Trends Cell Biol. 8, 365–372. doi: 10.1016/S0962-8924(98)01329-4
Serra, M., Madau, P., Chessa, M. F., Caddeo, M., Sanna, E., Trapani, G., et al. (1999). 2-Phenyl-imidazo[1,2-a]pyridine derivatives as ligands for peripheral benzodiazepine receptors: stimulation of neurosteroid synthesis and anticonflict action in rats. Br. J. Pharmacol. 127, 177–187. doi: 10.1038/sj.bjp.0702530
Shiratori, M., Tozaki-Saitoh, H., Yoshitake, M., Tsuda, M., and Inoue, K. (2010). P2X7 receptor activation induces CXCL2 production in microglia through NFAT and PKC/MAPK pathways. J. Neurochem. 114, 810–819. doi: 10.1111/j.1471-4159.2010.06809.x
Soustiel, J. F., Vlodavsky, E., Milman, F., Gavish, M., and Zaaroor, M. (2011). Improvement of cerebral metabolism mediated by Ro5-4864 is associated with relief of intracranial pressure and mitochondrial protective effect in experimental brain injury. Pharm Res. 28, 2945–2953. doi: 10.1007/s11095-011-0463-0
Soustiel, J. F., Zaaroor, M., Vlodavsky, E., Veenman, L., Weizman, A., and Gavish, M. (2008). Neuroprotective effect of Ro5-4864 following brain injury. Exp. Neurol. 214, 201–208. doi: 10.1016/j.expneurol.2008.08.008
Starossom, S. C., Mascanfroni, I. D., Imitola, J., Cao, L., Raddassi, K., Hernandez, S. F., et al. (2012). Galectin-1 deactivates classically activated microglia and protects from inflammation-induced neurodegeneration. Immunity 37, 249–263. doi: 10.1016/j.immuni.2012.05.023
Sukumari-Ramesh, S., Alleyne, C. H. Jr., and Dhandapani, K. M. (2012a). Astrogliosis: a target for intervention in intracerebral hemorrhage? Transl. Stroke Res. 3, 80–87. doi: 10.1007/s12975-012-0165-x
Sukumari-Ramesh, S., Alleyne, C. H. Jr, and Dhandapani, K. M. (2012b). Astrocyte-specific expression of survivin after intracerebral hemorrhage in mice: a possible role in reactive gliosis? J. Neurotrauma 29, 2798–2804. doi: 10.1089/neu.2011.2243
Sukumari-Ramesh, S., Alleyne, C. H. Jr., and Dhandapani, K. M. (2015). The histone deacetylase inhibitor suberoylanilide hydroxamic acid (SAHA) confers acute neuroprotection after intracerebral hemorrhage in mice. Transl. Stroke Res. 7, 141–148. doi: 10.1007/s12975-015-0421-y
Sukumari-Ramesh, S., and Alleyne, C. H. Jr. (2016). Post-injury administration of tert-butylhydroquinone attenuates acute neurological injury after intracerebral hemorrhage in mice. J. Mol. Neurosci. 58, 525–531. doi: 10.1007/s12031-016-0722-y
Tessier, P. A., Naccache, P. H., Clark-Lewis, I., Gladue, R. P., Neote, K. S., and McColl, S. R. (1997). Chemokine networks in vivo: involvement of C-X-C and C-C chemokines in neutrophil extravasation in vivo in response to TNF-alpha. J. Immunol. 159, 3595–3602.
Tu, L. N., Morohaku, K., Manna, P. R., Pelton, S. H., Butler, W. R., Stocco, D. M., et al. (2014). Peripheral benzodiazepine receptor/translocator protein global knock-out mice are viable with no effects on steroid hormone biosynthesis. J. Biol. Chem. 289, 27444–27454. doi: 10.1074/jbc.M114.578286
Verleye, M., Akwa, Y., Liere, P., Ladurelle, N., Pianos, A., Eychenne, B., et al. (2005). The anxiolytic etifoxine activates the peripheral benzodiazepine receptor and increases the neurosteroid levels in rat brain. Pharmacol. Biochem. Behav. 82, 712–720. doi: 10.1016/j.pbb.2005.11.013
Wagner, K. R., Xi, G., Hua, Y., Kleinholz, M., de Courten-Myers, G. M., Myers, R. E., et al. (1996). Lobar intracerebral hemorrhage model in pigs: rapid edema development in perihematomal white matter. Stroke 27, 490–497. doi: 10.1161/01.STR.27.3.490
Wang, H., Pullambhatla, M., Guilarte, T. R., Mease, R. C., and Pomper, M. G. (2009). Synthesis of [(125)I]iodoDPA-713: a new probe for imaging inflammation. Biochem. Biophys. Res. Commun. 389, 80–83. doi: 10.1016/j.bbrc.2009.08.102
Wang, J. (2010). Preclinical and clinical research on inflammation after intracerebral hemorrhage. Prog. Neurobiol. 92, 463–477. doi: 10.1016/j.pneurobio.2010.08.001
Wang, J., and Dore, S. (2007). Inflammation after intracerebral hemorrhage, Journal of cerebral blood flow and metabolism. J. Cereb. Blood Flow Metab. 27, 894–908. doi: 10.1038/sj.jcbfm.9600403
Xi, G., Keep, R. F., and Hoff, J. T. (2006). Mechanisms of brain injury after intracerebral haemorrhage. Lancet Neurol. 5, 53–63. doi: 10.1016/S1474-4422(05)70283-0
Xiong, X. Y., and Yang, Q. W. (2015). Rethinking the roles of inflammation in the intracerebral hemorrhage. Transl. Stroke Res. 6, 339–341. doi: 10.1007/s12975-015-0402-1
Yabluchanskiy, A., Sawle, P., Homer-Vanniasinkam, S., Green, C. J., and Motterlini, R. (2010). Relationship between leukocyte kinetics and behavioral tests changes in the inflammatory process of hemorrhagic stroke recovery. Int. J. Neurosci. 120, 765–773. doi: 10.3109/00207454.2010.523129
Yang, G. Y., Betz, A. L., Chenevert, T. L., Brunberg, J. A., and Hoff, J. T. (1994). Experimental intracerebral hemorrhage: relationship between brain edema, blood flow, and blood-brain barrier permeability in rats. J. Neurosurg. 81, 93–102. doi: 10.3171/jns.1994.81.1.0093
Zhang, D., Hu, X., Qian, L., Wilson, B., Lee, C., Flood, P., et al. (2009). Prostaglandin E2 released from activated microglia enhances astrocyte proliferation in vitro. Toxicol. Appl. Pharmacol. 238, 64–70. doi: 10.1016/j.taap.2009.04.015
Zhang, L. M., Qiu, Z. K., Zhao, N., Chen, H. X., Liu, Y. Q., Xu, J. P., et al. (2014). Anxiolytic-like effects of YL-IPA08, a potent ligand for the translocator protein (18 kDa) in animal models of post-traumatic stress disorder. Int. J. Neuropsychopharmacol. 17, 1659–1669. doi: 10.1017/S1461145714000479
Zhao, X., Zhang, Y., Strong, R., Zhang, J., Grotta, J. C., and Aronowski, J. (2007). Distinct patterns of intracerebral hemorrhage-induced alterations in NF-kappaB subunit, iNOS, and COX-2 expression. J. Neurochem. 101, 652–663. doi: 10.1111/j.1471-4159.2006.04414.x
Keywords: [125 I]IodoDPA-713, microglial activation, stroke, intracerebral hemorrhage, gliosis
Citation: Bonsack F, Foss CA, Arbab AS, Alleyne CH Jr., Pomper MG and Sukumari-Ramesh S (2018) [125 I]IodoDPA-713 Binding to 18 kDa Translocator Protein (TSPO) in a Mouse Model of Intracerebral Hemorrhage: Implications for Neuroimaging. Front. Neurosci. 12:66. doi: 10.3389/fnins.2018.00066
Received: 10 August 2017; Accepted: 29 January 2018;
Published: 22 February 2018.
Edited by:
Oscar Arias-Carrión, Hospital General Dr. Manuel Gea Gonzalez, MexicoReviewed by:
Braxton A. Norwood, Expesicor LLC, United StatesDong-Hoon Lee, University of Sydney, Australia
Hong Xu, University of Pennsylvania, United States
Copyright © 2018 Bonsack, Foss, Arbab, Alleyne, Pomper and Sukumari-Ramesh. This is an open-access article distributed under the terms of the Creative Commons Attribution License (CC BY). The use, distribution or reproduction in other forums is permitted, provided the original author(s) and the copyright owner are credited and that the original publication in this journal is cited, in accordance with accepted academic practice. No use, distribution or reproduction is permitted which does not comply with these terms.
*Correspondence: Sangeetha Sukumari-Ramesh, sramesh@augusta.edu