- 1Tanz Centre for Research in Neurodegenerative Diseases, University of Toronto, Toronto, ON, Canada
- 2Memory Clinic, Toronto Western Hospital, University Health Network, Toronto, ON, Canada
- 3The Edmond J. Safra Program for Parkinson Disease, Movement Disorder Clinic, Toronto Western Hospital, University Health Network, Toronto, ON, Canada
Objective:To determine the relationship between alterations in resting state functional connectivity and social cognition dysfunction among patients with frontotemporal dementia (FTD), Alzheimer’s disease (AD), Parkinson’s disease (PD), and healthy controls (HC).
Methods: Fifty-seven participants (FTD = 10, AD = 18, PD = 19, and HC = 10) underwent structural and functional imaging and completed the Awareness of Social Inference Test-Emotion Evaluation Test (TASIT-EET), Behavioral Inhibition System/Behavioral Activation System (BIS/BAS) scale, Revised Self-Monitoring Scale (RSMS), Interpersonal Reactivity Index (IRI), and Social Norms Questionnaire (SNQ). A multi-variate pattern analysis (MVPA) was carried out to determine activation differences between the groups. The clusters from the MVPA were used as seeds for the ROI-to-voxel analysis. Relationship between social cognition deficits and uncinate integrity was also investigated.
Results: BOLD signal activation differed among the four groups of AD, PD, FTD, and HC in the left inferior temporal gyrus-anterior division [L-ITG (ant)], right central opercular cortex (R-COp), right supramarginal gyrus, posterior division (R-SMG, post), right angular gyrus (R-AG), and R-ITG. The BOLD co-activation of the L-ITG (ant) with bilateral frontal pole (FP) and paracingulate gyrus was positively associated with IRI-perspective taking (PT) (r = 0.38, p = 0.007), SNQ total (r = 0.37, p = 0.009), and TASIT-EET (r = 0.47, p < 0.001).
Conclusion: Patients with neurodegenerative diseases showed alterations in connectivity in brain regions important for social cognition compared with HCs. Functional connectivity correlated with performance on social cognition tasks and alterations could be responsible for some of the social cognition deficits observed in all neurodegenerative diseases.
Introduction
Neurodegenerative diseases consist of a heterogeneous group of conditions, including frontotemporal dementia (FTD), Alzheimer’s disease (AD), and Parkinson’s disease (PD), that present with different clinical syndromes determined by the different brain areas and circuits most often affected. The focus of research in neurodegenerative disease has been the cognitive domains of memory, language, executive, and visuospatial function. Social cognition comprises many psychological processes including perceiving and recognizing social and emotional signals, evaluating the personal emotional relevance of everyday information, maintaining and accessing common social knowledge, processing information about beliefs and intentions, and generating and selecting behavioral responses that enable an individual to participate in social interactions. There is growing awareness that social cognitive deficits, including disturbances of emotion recognition, occur in the different neurodegenerative diseases (Snowden et al., 2003; Shany-Ur and Rankin, 2011; Sollberger et al., 2014; Poveda et al., 2017).
Frontotemporal dementia comprises a number of clinical syndromes involving behavior, language, and motor dysfunction. The main syndromes encompassed by the clinical term FTD are behavioral variant (bvFTD), non-fluent variant primary progressive aphasia (nfvPPA), and semantic variant primary progressive aphasia (svPPA). The clinical expression of these syndromes is determined by the selective injury of specific areas of the brain, which leads to the diverse signs and symptoms. Dramatic personality and behavioral changes with apathy, disinhibition, prominent loss of social cognition, lack of empathy, and inability to decipher other’s emotions, are hallmarks of bvFTD (Gustafson, 1987; Neary et al., 1988, 1998). Social cognition deficits are early signs of bvFTD. There are a number of studies that have reported various social cognitive abnormalities in bvFTD patients, including abnormalities in Theory of Mind (ToM) detection of gaze direction, and recognition of facial and/or prosodic emotional expressions, in particular negative emotions such as fear and anger (Gregory et al., 2002; Keane et al., 2002; Rosen et al., 2004; Lavenu and Pasquier, 2005; Diehl-Schmid et al., 2007; Eslinger et al., 2007; Kessels et al., 2007; Werner et al., 2007; Bediou et al., 2009). There is evidence that bvFTD patients have difficulty identifying social concepts, judging appropriate actions in social dilemmas, recognizing sarcasm, and differentiating minor social transgressions from serious moral violations (Mendez et al., 2005; Lough et al., 2006; Eslinger et al., 2007; Grossman et al., 2010; Shany-Ur et al., 2012). Although svPPA and nfvPPA are primarily identified as language disorders, social cognition can also be affected (Neary et al., 1998; Hodges and Miller, 2001; Multani et al., 2017) and loss of emotion detection and decreased empathy has been reported in svPPA and nvPPA (Multani et al., 2017).
Individuals diagnosed with AD often display episodic memory dysfunction, accompanied by neuropathologic, metabolic, and functional connectivity changes within the medial temporal lobe, posterior cingulate cortex, precuneus, and lateral temporoparietal areas, suggesting impairment throughout a posterior episodic memory network (Greicius et al., 2004; Buckner et al., 2005). In AD, social cognition has received less attention but there is increasing evidence that patients with AD have impaired ToM (Koff et al., 2004) as well as decreased ability to recognize emotions (Hargrave et al., 2002; Burnham and Hogervorst, 2004; Kohler et al., 2005; Bediou et al., 2009; Martinez et al., 2018). Studies have also revealed that the social cognition deficits are not necessarily correlated with cognitive deficits (Hargrave et al., 2002; Bediou et al., 2009) although there is controversy over this (Burnham and Hogervorst, 2004; Spoletini et al., 2008). One notable study evaluated facial emotion expression recognition and noted that the worst performers were not those with the worst cognitive scores (Luzzi et al., 2007). The default mode network, a prime target in AD, has been implicated in social cognition so it shouldn’t be surprising that multiple studies are demonstrating social cognitive deficits in AD (Torralva et al., 2000; Bediou et al., 2009; Zhou et al., 2010).
Although PD is known for its motor impairments, non-motor deficits including social cognitive deficits are likely due to disruption of fronto-striatal circuits due to impaired dopamine release (Zgaljardic et al., 2006; Sawamoto et al., 2008; Skuse and Gallagher, 2009; Bodden et al., 2010). Several studies have reported that PD patients have an impaired ability to recognize facial, and to a lesser extent prosodic, expressions of emotion, particularly disgust, fear, and anger (Kan et al., 2002; Mengelberg and Siegert, 2003; Pell and Leonard, 2003, 2005; Yip et al., 2003; Dujardin et al., 2004; Mimura et al., 2006; Kawamura and Koyama, 2007; Benuzzi et al., 2008; Assogna et al., 2010; Martinez et al., 2018), but this has not been a consistent finding (Biseul et al., 2005; Pell and Leonard, 2005). Although the neural basis of these deficits is not fully understood, various neural substrates have been implicated in emotion recognition including amygdala, orbitofrontal cortex (OFC), insula, and basal ganglia (BG) (Adolphs, 2002; Adolphs et al., 2003; Hornak et al., 2003; Krolak-Salmon et al., 2003; Sprengelmeyer et al., 2003; Yoshimura et al., 2005; Fusar-Poli et al., 2009; Baggio et al., 2012). The BG, a major site of dysfunction in PD because of its loss of dopaminergic innervation, has been implicated in facial emotion recognition (Cancelliere and Kertesz, 1990; Adolphs et al., 2002) as well as in emotion recognition from prosodic cues, where it acts in conjunction with the right frontoparietal cortex and potentially the amygdala (Cancelliere and Kertesz, 1990; Starkstein et al., 1994; Adolphs et al., 2002). A few studies have demonstrated emotion recognition deficits and bilateral orbitofrontal and right amygdala atrophy in patients with PD (Ibarretxe-Bilbao et al., 2009). PD patients have also shown impaired TOM although it’s felt that cognition may be contributing to that impairment (Saltzman et al., 2000; Yu et al., 2012).
These social cognitive changes that often include changes in personality are a source of distress for caregivers (Martinez et al., 2018) and their neuroanatomical correlates require further investigation. Moreover, in addition to gray matter atrophy, there is increasing evidence for network dysfunction and functional connectivity alteration in neurodegenerative diseases (Seeley et al., 2009). FTD encompasses behavioral and language syndromes including behavioral variant FTD (bvFTD), semantic variant PPA, and non-fluent variant PPA. The most frequently studied FTD group with respect to networks is bvFTD and alterations in the saliency network is the most consistent finding (Zhou et al., 2010; Day et al., 2013; Farb et al., 2013; Filippi et al., 2013; Caminiti et al., 2015). Some studies have reported an increased default mode network connectivity in FTD (Zhou et al., 2010; Farb et al., 2013; Rytty et al., 2013), but others have reported reductions within the default mode network (Farb et al., 2013; Filippi et al., 2013). Changes in connectivity of executive control network, dorsal attention network, the auditory network (Hafkemeijer et al., 2015), and the frontoparietal and frontotemporal networks including the insular cortex (Farb et al., 2013; Rytty et al., 2013; Sedeño et al., 2016) have also been reported. Comparing patients with bvFTD and AD directly, opposite patterns emerged with increased connectivity of default mode network and disrupted saliency network in bvFTD and the opposite pattern in AD (Zhou et al., 2010).
The default mode network, encompassing the posterior cingulate, precuneus, inferior parietal cortex, OFC, medial prefrontal cortex, ventral anterior cingulate (ACC), left dorsolateral prefrontal cortex, left parahippocampus, inferior temporal cortex, nucleus accumbens, and the midbrain (Raichle et al., 2001; Greicius et al., 2003) are responsible for a baseline state of the brain that represents self-reference, emotional processing, memory, as well as spontaneous cognition and aspects of consciousness (Raichle, 2015). Altered connectivity within the DMN in particular connectivity of the precuneus, posterior cingulate cortex, and the prefrontal cortex have been implicated in AD (He et al., 2007; Sorg et al., 2007; Wang et al., 2007; Gili et al., 2011; Wu et al., 2011; Agosta et al., 2012; Binnewijzend et al., 2012; Filippi et al., 2013; Liu et al., 2013; Weiler et al., 2014; Hafkemeijer et al., 2015; Zhou et al., 2015). Based on the division into anterior and posterior default mode network, it was found that connectivity reductions in the default mode network are mainly found in the posterior default mode network (Koch et al., 2014), but with altered connectivity to the anterior default mode network (Jones et al., 2012).
Altered connectivity affecting motor regions including the supplementary motor area, the premotor area, the primary motor cortex, the cerebellum, BG, and thalamic connectivity have been reported in PD (Wu et al., 2009, 2011; Kwak et al., 2010; Hacker et al., 2012; Skidmore et al., 2013; Luo et al., 2014, 2015; Szewczyk-Krolikowski et al., 2014; Canu et al., 2015; Rolinski et al., 2015). Alterations in connectivity of amygdala to frontal, occipital, and cerebellar locations have also been reported in PD (Hu et al., 2015). Unlike in AD, no clear patterns regarding the default mode network are present with some papers reporting either no (Helmich et al., 2009; Krajcovicova et al., 2012) or only few (Tessitore et al., 2012; Disbrow et al., 2014; Canu et al., 2015) alterations of default mode network connectivity although one paper found rather substantial alterations in PD compared with healthy controls (HCs) (Chen et al., 2015). Increased default mode network connectivity was also found in some papers (Campbell et al., 2015; Gorges et al., 2015). Widespread alterations of networks in PD, within and between networks have been reported as well (Madhyastha et al., 2015; Onu et al., 2015). An investigation of the executive control network did not yield any differences in PD compared to healthy adults (Disbrow et al., 2014), but another study found impaired connectivity between the right central executive network and the salience network in PD (Putcha et al., 2015). Disrupted connectivity in frontal and parietal networks in PD with dementia (Amboni et al., 2015; Borroni et al., 2015), as well as in the dorsal attention network (Baggio et al., 2015) have been reported. Several networks have been identified to play a key role in distinct social cognitive functions. The default mode network, which is altered in AD (Greicius et al., 2004), is hypothesized to be involved in introspection. The salience network, on the other hand, disrupted in bvFTD, plays a key role in directing attention to behaviorally relevant salient information.
In recent years, diffusion tensor imaging (DTI) studies have revealed significant white matter tract abnormalities in addition to the previously known gray matter atrophy in neurodegenerative diseases (Zhang et al., 2009; Caso et al., 2016; Multani et al., 2016; Elahi et al., 2017). DTI allows in vivo evaluation of white matter integrity by examining distribution of water molecules within fiber tracts (Ciccarelli et al., 2008). Decreased integrity of the right uncinate fasciculus (UF) has been related to changes in emotion detection and cold-heartedness in language variants of FTD (Multani et al., 2017).
Although certain network alterations have been identified in these neurodegenerative diseases, it is unclear whether social cognition relevant regions have altered functional activity in these diseases. Previous studies highlight network-wide (connectivity between multiple neuroanatomical regions) connectivity dysfunction, which may potentially mask changes in region-to-region (ROI-to-ROI) functional connectivity (connectivity between two specific regions) and its association with social cognition function. Furthermore, ROI-to-ROI functional connectivity analysis can be used in conjunction with DTI (structural) analysis to understand whether the observed behavior or symptom is associated with functional or structural dysfunction between the two regions.
Given the vast number of neuroanatomical regions involved in social cognition, a whole-brain level functional connectivity analysis can provide insight into particular brain regions that are altered in different neurodegenerative diseases. We hypothesized that these whole-brain level disease-specific alterations in functional connectivity could help identify regions that would vary across AD, FTD, and PD, and that these changes would relate to abnormalities in social cognitive function.
Materials and Methods
Study Participants
Subjects with neurodegenerative diseases, along with their caregivers were recruited from the Memory Clinic and Movement Disorders Clinic, Toronto Western Hospital. Subjects qualified for the study if they had one of the following diagnosis: (1) AD as outlined by McKhann et al. (2011) (N = 18); (2) PD according to the PD Society Brain Bank Clinical Diagnostic Criteria (Hughes et al., 1992) (N = 19); (3) ten patients with FTD subtypes (svPPA N = 4), nfvPPA (N = 2) as outlined by Gorno-Tempini et al. (2011), or bvFTD (N = 4) as per Rascovsky et al. (2011) (Gorno-Tempini et al., 2011; Rascovsky et al., 2011); (4) age and sex matched HCs with no history of neurological or psychiatric disorders were also recruited (N = 10). Patients were excluded from the study if they had any of the following: presence of other neurological disorder, psychiatric illness, and visual or auditory deficits requiring correction beyond corrective lenses or hearing aids, respectively. The study received ethics approval of the University Health Network Research Ethics Board and all subjects provided written consent.
Measures
Participants (patients, caregivers, and HCs) completed The Awareness of Social Inference Test-Emotion Evaluation Test (TASIT-EET) to evaluate their ability to recognize emotions (McDonald et al., 2003). Cognition was evaluated using the Toronto Cognitive Assessment (TorCA) in all participants (Freedman et al., 2018) and caregivers were interviewed on the Clinical Dementia Rating (CDR) scale to assess dementia severity in patients (Morris, 1993). Social cognition measures were assessed using the following informant-based questionnaires: (1) Behavioral Inhibition System/Behavioral Activation System (BIS/BAS) scale (Carver and White, 1994) to measure behavioral inhibition (sensitivity to punishment) and behavioral activation (sensitivity to rewards) in individuals, (2) Revised Self-Monitoring Scale (RSMS) (Lennox and Wolfe, 1984) in order to measure the subjects’ awareness of their own social behavior (as assessed by the informant), we obtained informant’s perspective of the subject’s self-concern and self-focus using the Lennox and Wolfe version of the RSMS informant-based reports (Lennox and Wolfe, 1984). (3) Interpersonal Reactivity Index (IRI) (Davis, 1983) to measure empathy, which measures both the cognitive and emotional aspects of empathy, and (4) Social Norms Questionnaire (SNQ) (Rankin, 2008) to assess the subject’s ability to assess social boundaries in the mainstream culture of Canada. For participants with PD, these assessments were all performed in the on drug state. Detailed descriptions of scales are available in Supplementary Material.
Magnetic Resonance Imaging Acquisition
All structural and resting state scans were performed on a 3 Tesla Magnetic Resonance Imaging (MRI) Scanner (GE Signa HDx, Milwaukee, WI, United States) with a standard 8-channel head coil. High resolution T1-weighted images were obtained using inversion recovery fast spoiled gradient echo (IR-FSPGR), with the following parameters: 176 slices with 1.2 mm thickness; 2.8 ms echo time (TE); 7.0 ms repetition time (TR); 400 ms inversion time (TI); 11° flip angle; 26.0 cm field of view (FOV); 256 × 256 matrix size. T2∗-weighted functional data images were acquired in an interleaved order (28–32 slices for the whole brain), using the following parameters: slice thickness = 5 mm with no gap, FOV = 240 mm, TR = 2 s. For each participant, two 3-min scans were acquired. The scans were acquired in an oblique orientation and each slice was perpendicular to the long axis of the hippocampus. DTI scans were acquired in 8 min with 55 directions, using the following parameters: 2D single-shot EPI sequence, 60 contiguous slices, slice thickness = 2 mm, TR = 800 ms, TE = 100 ms, b = 1000 s/mm2, base matrix = 128 × 128, and FOV = 240 × 256 mm2.
Resting State fMRI Analysis
Resting state fMRI preprocessing and analysis was conducted using the Conn Toolbox 17e1 (RRID:SCR_009550) (Whitfield-Gabrieli and Nieto-Castanon, 2012). The preprocessing pipeline for structural and functional images consisted of the following: (1) slice-time correction in ascending order, (2) functional realignment and unwarp, (3) co-registration of functional images to structural images, (4) structural segmentation into gray matter, white matter and cerebrospinal fluid, and normalization to MNI space, (5) functional normalization to MNI space, (6) the Artifact Detection Tools (ARTs) scrubbing method for global signal outlier and motion detection, which were used as first level covariates, and (7) functional smoothing (FWHM = 8 mm).
Since the purpose of the study was to examine differences in functional connectivity and its association with social cognition function, as the first step, an exploratory voxel-to-voxel analysis was conducted to determine differences in peak blood oxygen-level dependent (BOLD) activation between the four groups (i.e., AD, PD, FTD, and HC groups). Once differences in peak activations were established, we then used MVPA at the group level to extract seeds to use for ROI-to-voxel connectivity analysis as the second step. Since there is an intricate relationship between neuroanatomical regions associated with social cognition function, this approach enabled us to only address regions whose functional activity is altered across the AD, FTD, and PD groups. Therefore, the seeds from the MVPA, which were associated with social cognition, were extracted as ROI masks and were applied for ROI-to-voxel analysis to determine differences in activation between the four groups. Lastly, ROI-to-voxel Fisher’s Z-transformation scores were extracted to conduct two-tailed partial correlations, while controlling for CDR-SoB and sentence comprehension, with social cognition measures (TASIT-EET, SNQ total, BIS, IRI-EC, IRI-PT, RSMS-EX, RSMS-SP). The ToRCA sentence comprehension score (language) was used as a covariate when conducting partial correlations for TASIT-EET and SNQ total as language comprehension may impede performance.
Diffusion Tensor Imaging and Structural Connectivity
The fMRIB Software Library (FSL) tools2 was used to conduct DTI analysis. The DTI processing, region of interest definition for the UF, and fiber tracking were performed as previously described (Galantucci et al., 2011; Taghdiri et al., 2018). Four measures were obtained for the UF: fractional anisotropy (FA), axial diffusivity (AxD), radial diffusivity (RD), and mean diffusivity (MD).
Statistical Analysis
Statistical analyses were conducted using SPSS software (SPSS Inc. v. 24). One-way ANOVA was conducted to determine group differences on age, TorCA cumulative percentage, CDR SoB, BIS, BAS-D, BAS-FS, BAS-RR, IRI-PT, IRI-EC, RSMS-EX, RSMS-SP, SNQ total score, and the DTI measures (i.e., FA, AxD, RD, and MD) of right and left UF. Dunnett T3 (Shingala and Rajyaguru, 2015) analysis was carried out as a post hoc analysis. One-way ANCOVA was carried out to examine differences on TASIT-EET performance, while controlling for TorCA sentence comprehension.
Since the frontal pole (FP) and the inferior temporal gyrus (ITG) (anterior division) are structurally connected by the UF, we carried out mediation analyses to explore the potential mediation effects of the left UF on the relationship between “Group” (coded as 1 = HC, 2 = PD, 3 = AD, and 4 = FTD) and the functional connectivity between the bilateral FP and the left ITG and post-cingulate gyrus (Model A). The right UF is also known to contribute to social cognition (Oishi et al., 2015), so a similar mediation analysis was conducted to investigate the potential mediation effects of the right UF on the relationship between the “Group” and the functional connectivity between the right ITG and FP (Model B). To do so, we used the SPSS macro PROCESS tool (Hayes, 2013) which is implemented in SPSS version 24. We estimated the total, direct, and indirect effects as well as their associated standard error (SE) and 95% confidence intervals (CIs) (while controlling for age) using the 5000 bootstrap samples (Hayes, 2009, 2013). Mediation ratio was also calculated for each model as the ratio of direct effect to the total effect (Preacher and Kelley, 2011). All mediation analyses adjusted for participants’ age. For each specific effect, if the interval did not contain zero, it was considered statistically significant.
Results
After correcting for multiple comparisons, a significant difference was found between the four groups for TorCA cumulative percentage, CDR SoB, and IRI-PT (Table 1). In addition, there was a trend toward significance in comparison of the SNQ total score and TASIT-EET between the groups.
Voxel-to-Voxel Analysis
The following regions demonstrated a difference in peak BOLD signal between the four groups (i.e., AD, PD, FTD, and HC): (a) left ITG, anterior division (L-ITG, ant), (b) right central opercular cortex (R-COp), (c) right supramarginal gyrus, posterior division (R-SMG, post), and right angular gyrus (R-AG), and (d) right ITG (R-ITG) (Supplementary Table 1 and Figure 1).
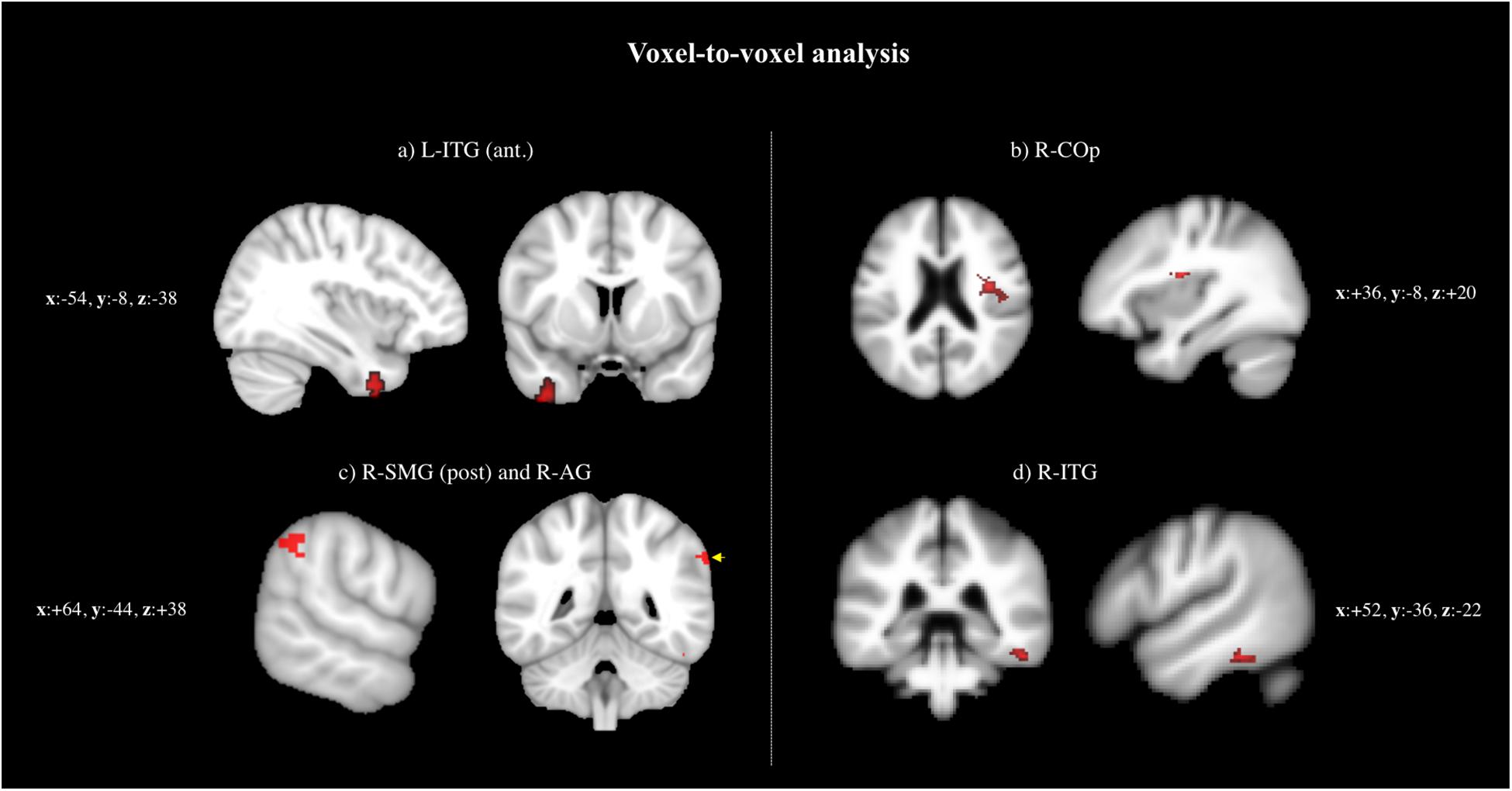
Figure 1. Voxel-to-voxel analysis: regions demonstrated a difference in peak BOLD signal between the four groups (i.e., AD, PD, FTD, and HC): (a) L-ITG (ant.), (b) RCOp, (c) R-SMG (post.) and R-AG, and (d) R-ITG.
ROI-to-Voxel Analysis
The seed-to-voxel analysis exhibited group differences for each seed extracted for voxel-to-voxel analysis (Supplementary Tables 2A–D, 3A–D). The Fisher Z-transformation score for each group is reported in Supplementary Tables 3A–D.
(a) L-ITG (ant) ROI (Supplementary Table 3A and Figure 2A): In the AD, PD, and FTD groups, a decreased functional connectivity was found between the L-ITG (ant) and the right and left lateral occipital cortex (R-LOC and L-LOC) compared to HC. Upon further examining this relationship, the PD and AD groups exhibited almost no functional connectivity, whereas the FTD group demonstrated negative connectivity between the two regions compared with positive connectivity in HC. Furthermore, the FTD group demonstrated decreased (negative) connectivity of L-ITG (ant) with the bilateral FP and paracingulate gyrus (PCG) cluster, and precuneus cortex cluster (Pc), compared to HC. The AD and the PD groups displayed almost no connectivity between the L-ITG (ant), and bilateral FP and Pc; however, this is not significantly different from HC.
(b) R-COp ROI (Supplementary Table 3B and Figure 2B): The functional connectivity of the R-COp was significantly different between HC and the three neurodegenerative groups for several GM regions. Firstly, one of the clusters, which consisted of the right insular cortex (R-IC), right frontal operculum cortex (R-FO), and R-COp, showed positive functional connectivity for PD and FTD, whereas this relationship was absent in AD and HC. Next, three significant clusters (1) R-AG and R-SMG (post), (2) L-IC and L-Cop, and (3) L-SMG were negatively functionally connected with the R-COp in HC, whereas this relationship was not observed in the three neurodegenerative groups.
(c) R-SMG (post) and R-AG ROI (Supplementary Table 3C and Figure 2C): The R-SMG (post) and R-AG also showed distinct patterns of functional connectivity between the four groups. The functional connectivity with the R-FP was positive in the HC, AD, and PD group, whereas this association was absent in the FTD group. In addition, the FTD group displayed negative functional connectivity with the right temporal fusiform cortex, posterior division (R-TFC, post), and R-ITG, whereas there was positive functional connectivity in HC, and no connectivity in AD and PD. Lastly, a significant difference in connectivity was observed between FTD, and AD and PD, with the right cerebellum (R-Cer).
(d) R-ITG (post) ROI (Supplementary Table 3D and Figure 2D): For the R-ITG (post) ROI, the FTD group displayed significantly increased connectivity with the R-Cer, compared to AD. The PD group, on the other hand, displayed no functional connectivity with the L-FP, compared to HC.
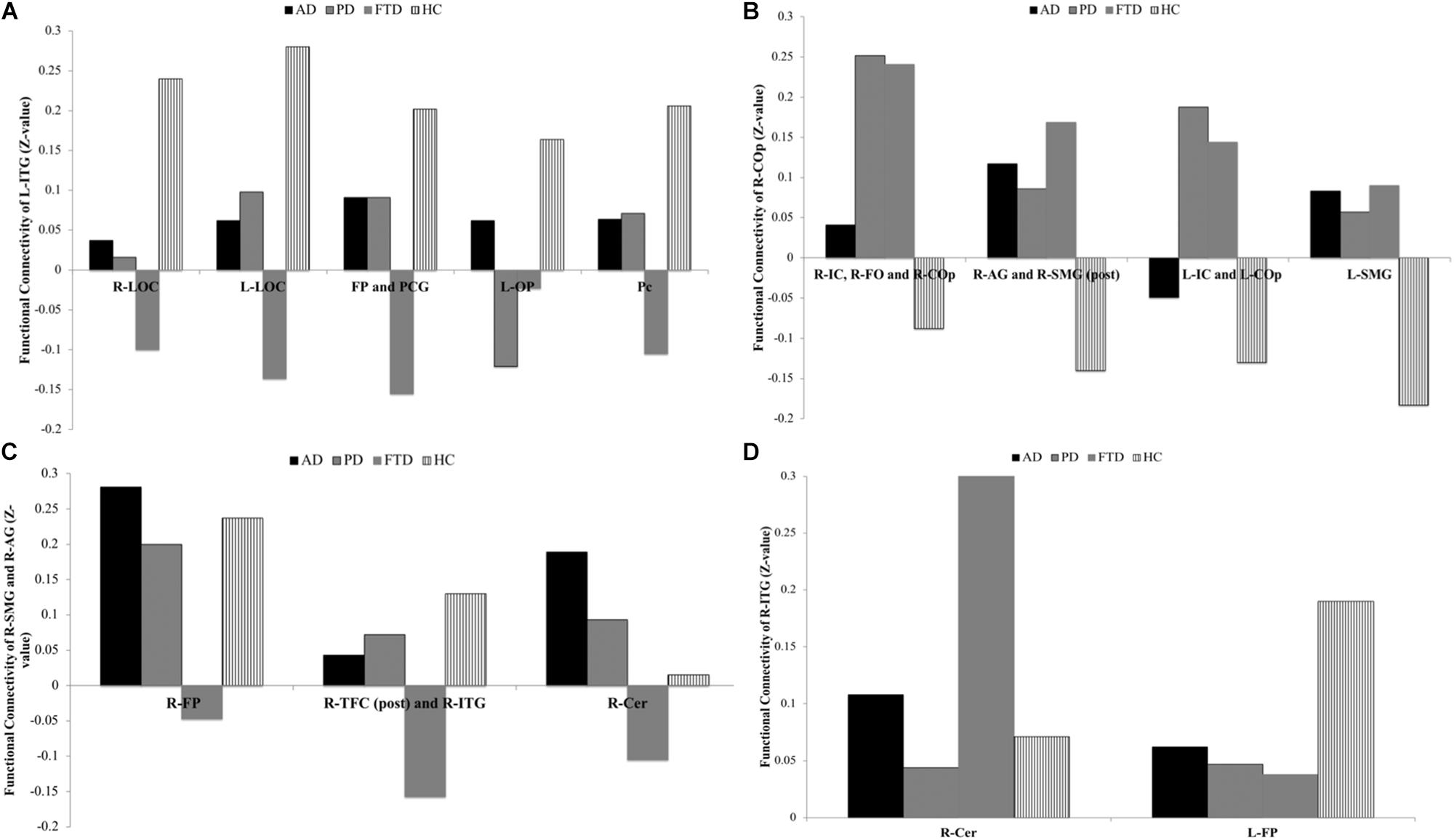
Figure 2. ROI-to-voxel analysis, group differences for each seed extracted for voxel-to-voxel analysis: (A) Group differences for functional connectivity of L-ITG, (B) group differences for functional connectivity of R-COp, (C) group differences for functional connectivity of R-SMG and R-AG, and (D) group differences for functional connectivity of R-ITG.
Association Between Functional Connectivity and Social Cognition Measures
In order to determine whether there was a relationship between differences in functional connectivity in the four groups and the social cognition measures, we only examined regions relevant to social cognition. The following regions are known to be involved in social cognition: (a) FP, (b) temporal lobe, and (c) insular cortex (Lamm and Singer, 2010; Elamin et al., 2012). Therefore, the following functionally connected regions from the ROI-to-voxel analysis were examined in all participants combined: (a) L-ITG (ant) with bilateral FP, PCG, (b) R-COp with R-IC, R-FO, R-COp, (c) R-COp with L-IC and L-COp, (d) R-SMG (post), R-AG with R-FP, (e) R-SMG (post), R-AG with R-TFC (post), R-ITG, and (f) R-ITG (post) and L-FP.
The BOLD co-activation of the L-ITG (ant) with bilateral FP, PCG was positively associated with IRI-PT (r = 0.38, p = 0.007), SNQ total (r = 0.37, p = 0.009), and TASIT-EET (r = 0.47, p < 0.001). There was also a positive correlation of functional connectivity of the R-SMG (post), R-AG, and R-FP with IRI-EC (r = 0.32, p = 0.029), IRI-PT (r = 0.43, p = 0.002), RSMS-EX (r = 0.42, p = 0.003), RSMS-SP (r = 0.35, p = 0.014), and a trend for TASIT-EET (r = 0.27 p = 0.06). Lastly, the IRI-PT (r = 0.34, p = 0.017) and TASIT-EET (r = 0.42, p = 0.003) were also positively associated with the functional connectivity of R-SMG (post), R-AG with R-TFC, and R-ITG. However, only the TASIT-EET association with connectivity between L-ITG (ant) and bilateral FP, PCG withstood correction for multiple comparisons at p < 0.0012 (Figure 3).
Uncinate Fasciculus (UF) Structural Integrity Analysis
Since the FP and the ITG (anterior division) are structurally connected by the UF (Von Der Heide et al., 2013), we used one-way ANOVA, followed by Dunnett’s T3 post hoc analysis to examine the structural integrity of this white matter tract across the four groups (Table 2).
We also carried out mediation analyses to explore the potential mediation effect of the UF on the observed effects of “group” on the functional connectivity between the L-ITG (ant), and bilateral FP and PCG. These analyses revealed that the effect of “group” on functional connectivity was partially mediated through the structural connectivity of the left UF [Table 3 (Model A) and Figure 4]. However, the mediation ratio (i.e., ratio of indirect to total effect) was only 26.3% (Table 3), which showed that the effect of “group” on the functional connectivity between the L-ITG and bilateral FP and PCG was beyond the effects of structural connectivity of the left UF alone. The right UF MD showed no significant mediating effect on the relationship between the “group” and functional connectivity of right ITG and FP (Table 3, Model B).
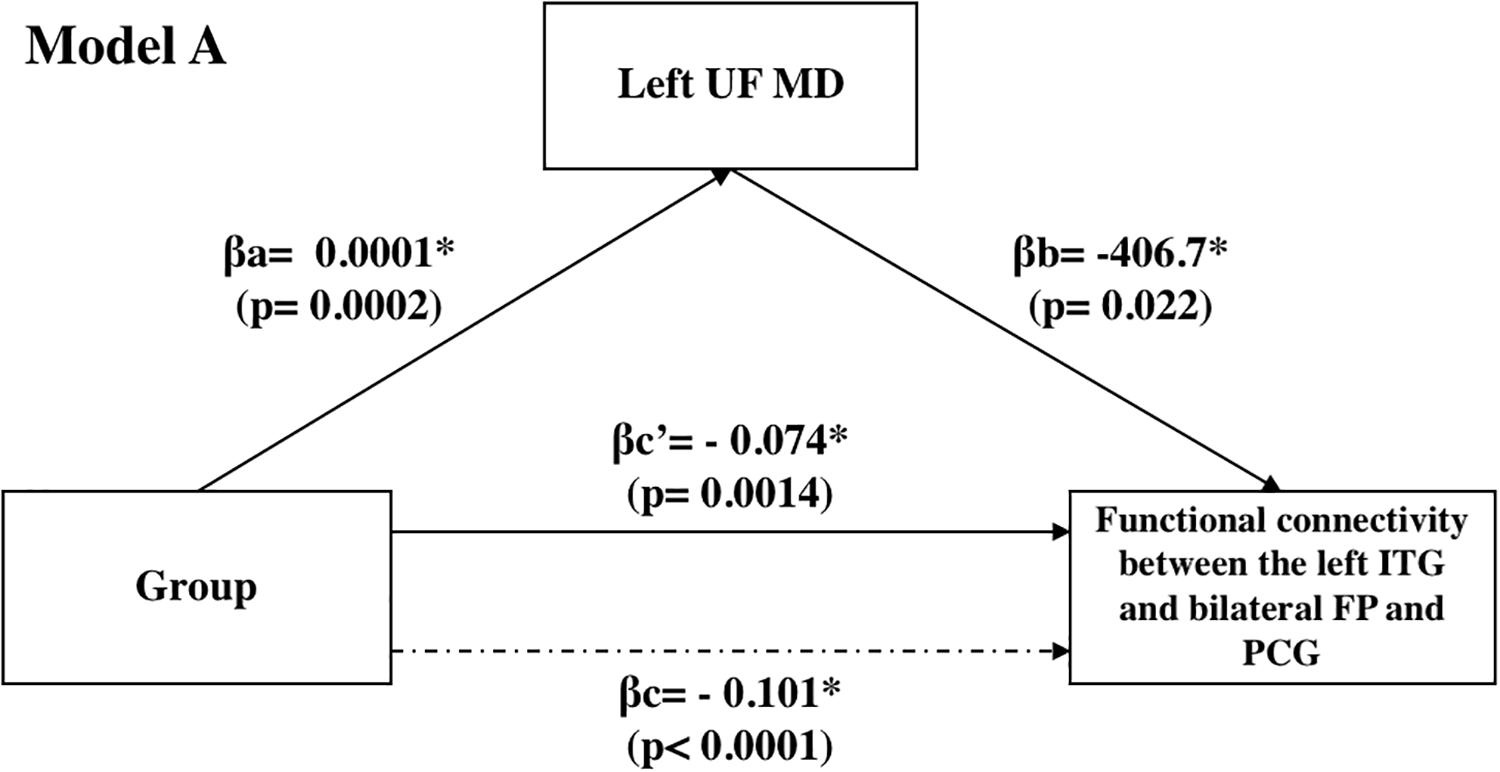
Figure 4. Mediation analyses: effect of the UF on the observed effects of “group” on the functional connectivity between the L-ITG (ant), and bilateral FP and PCG.
Discussion
Our results reveal alterations in functional connectivity in patients with AD, PD, and FTD compared to HC. There was a difference in BOLD signal activation in the L-ITG (ant), R-COp, R-SMG and R-AG, and R-ITG (post) among the four groups. These areas displayed altered connectivity with other regions in the neurodegenerative disease groups. While the HC group displayed positive functional connectivity between the L-ITG (ant) and all its clusters, the AD group exhibited no functional connectivity between this area and most of the regions and decreased functional connectivity between the R-COp, and the R-AG and R-SMG (post) in the AD group. The functional connectivity between the R-ITG and bilateral LOC was also decreased in PD but in a distinct pattern from the AD group. In the PD group, there was increased connectivity between the R-COp, and (a) the R-IC, R-FO, and R-COp, as well as (b) the L-IC and L-COp, compared to the HC and the AD group. Furthermore, there was no connectivity between the R-ITG (post) and the L-FP, in contrast to the HC and AD groups.
Most of the previous studies identified neural substrates underlying emotional empathy by comparing stimuli with different emotional intensities (Breiter et al., 1996; Morris et al., 1996; Phillips et al., 1997; Sprengelmeyer et al., 1998; Blair et al., 1999), by comparing the perception of emotions and the observation of others experiencing the same emotions (Wicker et al., 2003; Jabbi et al., 2007; Jabbi and Keysers, 2008), or by comparing the perception of emotions with the imitation of the same emotions (Carr et al., 2003). While these studies found neural activity in brain areas such as the ACC, anterior insula, superior temporal cortex, amygdala, and inferior frontal cortex (Breiter et al., 1996; Morris et al., 1996; Phillips et al., 1997; Sprengelmeyer et al., 1998; Blair et al., 1999; Carr et al., 2003; Wicker et al., 2003; Hofmann, 2006; Jabbi et al., 2007; Shdo et al., 2018), the designs employed in the previous work did not allow to isolate intentionally controlled processes from automatically generated processes of empathy. In addition, although a number of studies investigated the modulation of “empathy for pain” by cognitive mechanisms (Lamm et al., 2007; Hein and Singer, 2008) or experience to painful practices (Cheng et al., 2007), the neuronal basis underlying the cognitive modulation of “emotional empathy” has, to our knowledge, not been investigated so far.
Social Cognition in Neurodegenerative Groups
All three neurodegenerative groups scored significantly lower on perspective-taking, a measure of empathy, compared to HC (Davis, 1983) and this was positively associated with the functional connectivity of the L-ITG (ant) with bilateral FP and PCG, as well as the connectivity between R-SMG, R-AG cluster with the (a) R-FP, and (b) R-TFC (post), R-ITG. These areas have been previously implicated in empathy in functional MRI studies (Breiter et al., 1996; Morris et al., 1996; Phillips et al., 1997; Sprengelmeyer et al., 1998; Blair et al., 1999; Carr et al., 2003; Hofmann, 2006; Jabbi et al., 2007; Shdo et al., 2018). Compared to the HC, the FTD group performed worse on the SNQ total, a measure of social norm knowledge. The SNQ total showed a positive association with functional connectivity between the L-ITG (ant), and bilateral FP and PCG. The anterior temporal lobe volume has been related to social norms performance on SNQ in bvFTD (Panchal et al., 2016) but our data show that the functional connectivity of the ITG with FP and PCG is related to the SNQ across all the neurodegenerative diseases so although the anterior temporal lobe is not usually atrophied in AD or PD, altered functional connectivity in temporal and frontal lobes can also contribute to social norms deficits.
The TASIT-EET score also exhibited a positive correlation with the functional connectivity between the R-SMG (post) and R-AG cluster with the R-TFC (post) and ITG. Lastly, although the IRI-EC, RSMS-EX, and RSMS-SP did not withstand correction for multiple comparisons, they were positively associated with the functional connectivity of R-SMG, R-AG with the R-FP. The alterations observed in the functional connectivity of these areas in the patients can explain some of the impairments in these social cognition tasks.
Overall, the findings of this study suggest positive functional connectivity between the L-ITG and bilateral FP and PCG is related to increased ability to take on others’ point of view. Perspective-taking requires one to deliberately suppress self-perception in order to reflect on others’ point of view and represents cognitive empathy (Ruby and Decety, 2003). Loss of cognitive and affective empathy is prominent in bvFTD and cognitive empathy deficits are also reported in individuals with AD (Dermody et al., 2016). In both groups, distinct patterns of atrophy are associated with cognitive empathy impairment. In the AD group, this is primarily related to the left temporoparietal atrophy, whereas bilateral frontoinsular, temporal, parietal, and occipital atrophy is associated with loss of cognitive empathy in the bvFTD group (Dermody et al., 2016). Furthermore, the FP plays a key role in inhibiting self-perception when assessing situations from a third person’s viewpoint (Ruby and Decety, 2003). Therefore, the loss of cognitive empathy in the neurodegenerative groups in the current study can be attributed to loss of functional connectivity between the L-ITG and bilateral FP and PCG.
Distinct Pattern of Functional Connectivity in FTD
The most distinct pattern of functional connectivity was observed in the FTD group. Our results reveal that the FTD group showed negative connectivity between the R-ITG (ant), and (a) R-LOC; (b) L-LOC; (c) bilateral FP and PCG; and (d) Pc cortex, compared to HC and was also significantly different from the AD and PD groups. Functional connectivity between the R-COp cortex and the R-IC, R-FO, and R-COp cortex was present in FTD, whereas this association was absent in the HC and AD group. The R-COp cortex functional connectivity was negative with the (a) R-AG and R-SMG (post) and (b) L-IC and L-COp in HC, while it was positive in the FTD group. Moreover, R-COp cortex connectivity with L-SMG was absent in FTD but positive in HC. The R-SMG (post) and R-AG seed exhibited no connectivity with the R-FP in the FTD group, compared to AD, PD, and HC. Moreover, the R-SMG (post) and AG displayed negative connectivity with the R-TFC (post) and R-ITG, whereas the HC group showed positive connectivity. There was also negative functional connectivity of the R-SMG (post) and R-AG with the R-Cer, and this association was absent in the HC group. Lastly, the R-ITG (post) was functionally connected to the R-Cer. This pattern was not observed in the HC group and was also significantly different from the AD and PD groups.
Functional connectivity between the R-FP, and R-SMG and R-AG was absent in the FTD group, and this also correlates with decreased cognitive empathy and impairment in emotion detection. The R-SMG is involved in egocentricity bias, where one projects one’s own beliefs onto others in social scenarios (Silani et al., 2013). Empathy also requires social perception, which entails non-verbal cues such as body language, facial expression, and eye-gazing, as well as higher mental processes (ToM) (Pelphrey et al., 2004). Social perception has been attributed to the R-SMG, R-AG, superior parietal lobe, and parahippocampal gyri (Lawrence et al., 2006). As the FP inhibits self-perception and the R-SMG is a key component of egocentricity bias and social perception (including R-AG), we hypothesize that this functional synchronization between the three regions may allow individuals to inhibit (FP) their egocentricity bias (R-SMG) and allow them to take on others’ perspective by mediating social perception cues. Lastly, the positive functional association between R-SMG and R-AG with R-TFC (post) and R-ITG observed in the HC group was absent in the AD and PD groups and was negative in FTD. Its association with cognitive empathy suggests that this loss of connectivity in individuals with FTD may be responsible for their inability to appreciate social perception cues and the negative association with the R-TFC (post) and R-ITG may be responsible for FTD patient’s inability to process emotional facial cues (right posterior fusiform cortex) (Geday et al., 2003) and therefore they are impaired in detecting others’ emotions. Hence, we postulate that individuals who display altered functional connectivity pattern in these regions may have trouble dissociating from self-referential thinking. As a result, they are unable to empathize and are inaccurate at detecting emotions in others.
Overall, the findings of this study suggest that alteration in functional connectivity of the L-ITG, and R-SMG and R-AG with social cognition-relevant regions (i.e., FP, temporal lobe, and insular cortex) is associated with decreased cognitive empathy and emotion detection impairment in neurodegenerative diseases, particularly FTD but also in AD and PD. Changes in social cognition have been associated with caregiver burden and depression (Martinez et al., 2018) especially when unrecognized. Our findings could provide a basis for using resting state functional connectivity as a biomarker of deficits in social cognition. It may also be an early sign of disease and so should be evaluated in the early stages. This altered connectivity may be amenable to interventions so functional connectivity may also prove useful as an outcome measure in interventional studies.
Data Availability Statement
The datasets generated for this study are available on request to the corresponding author.
Ethics Statement
The studies involving human participants were reviewed and approved by the University Health Network Research Ethics Board. The patients/participants provided their written informed consent to participate in this study.
Author Contributions
NM and FT carried out the acquisition of data, analyzed and interpreted the data, and drafted the manuscript for intellectual content. CA, BV, and KM performed a major role in the acquisition of data. DT-W, RK, SF, AL, AV, and CM interpreted the data and revised the manuscript for intellectual content. MT performed a major role in the acquisition of data, interpreted the data, drafted the manuscript for intellectual content, and revised the manuscript for intellectual content.
Funding
This work was supported by the Alzheimer’s Society of Canada.
Conflict of Interest
The authors declare that the research was conducted in the absence of any commercial or financial relationships that could be construed as a potential conflict of interest.
Acknowledgments
The authors would like to thank Dr. AV for helping with recruiting participants.
Supplementary Material
The Supplementary Material for this article can be found online at: https://www.frontiersin.org/articles/10.3389/fnins.2019.01259/full#supplementary-material
Footnotes
References
Adolphs, R. (2002). Neural systems for recognizing emotion. Curr. Opin. Neurobiol. 12, 169–177. doi: 10.1016/s0959-4388(02)00301-x
Adolphs, R., Baron-Cohen, S., and Tranel, D. (2002). Impaired recognition of social emotions following amygdala damage. J. Cogn. Neurosci. 14, 1264–1274. doi: 10.1162/089892902760807258
Adolphs, R., Tranel, D., and Damasio, A. R. (2003). Dissociable neural systems for recognizing emotions. Brain Cogn. 52, 61–69. doi: 10.1016/s0278-2626(03)00009-5
Agosta, F., Pievani, M., Geroldi, C., Copetti, M., Frisoni, G. B., and Filippi, M. (2012). Resting state fMRI in Alzheimer’s disease: beyond the default mode network. Neurobiol. Aging 33, 1564–1578. doi: 10.1016/j.neurobiolaging.2011.06.007
Amboni, M., Tessitore, A., Esposito, F., Santangelo, G., Picillo, M., Vitale, C., et al. (2015). Resting-state functional connectivity associated with mild cognitive impairment in Parkinson’s disease. J. Neurol. 262, 425–434.
Assogna, F., Pontieri, F. E., Cravello, L., Peppe, A., Pierantozzi, M., Stefani, A., et al. (2010). Intensity-dependent facial emotion recognition and cognitive functions in Parkinson’s disease. J. Int. Neuropsychol. Soc. 16, 867–876. doi: 10.1017/s1355617710000755
Baggio, H., Segura, B., Ibarretxe-Bilbao, N., Valldeoriola, F., Marti, M., Compta, Y., et al. (2012). Structural correlates of facial emotion recognition deficits in Parkinson’s disease patients. Neuropsychologia 50, 2121–2128. doi: 10.1016/j.neuropsychologia.2012.05.020
Baggio, H. C., Segura, B., Garrido−Millan, J. L., Marti, M., Compta, Y., Valldeoriola, F., et al. (2015). Resting−state frontostriatal functional connectivity in Parkinson’s disease–related apathy. Mov. Disord. 30, 671–679. doi: 10.1002/mds.26137
Bediou, B., Ryff, I., Mercier, B., Milliery, M., Hénaff, M.-A., D’Amato, T., et al. (2009). Impaired social cognition in mild Alzheimer Disease. J. Geriatr. Psychiatry Neurol 22, 130–140. doi: 10.1177/0891988709332939
Benuzzi, F., Nichelli, P. F., and Ariatti, A. (2008). Recognition of emotions from visual and prosodic cues in Parkinson’s disease. Neurol. Sci. 29, 219–227. doi: 10.1007/s10072-008-0971-9
Binnewijzend, M. A., Schoonheim, M. M., Sanz-Arigita, E., Wink, A. M., van der Flier, W. M., Tolboom, N., et al. (2012). Resting-state fMRI changes in Alzheimer’s disease and mild cognitive impairment. Neurobiol. Aging 33, 2018–2028. doi: 10.1093/cercor/bhu259
Biseul, I., Sauleau, P., Haegelen, C., Trebon, P., Drapier, D., Raoul, S., et al. (2005). Fear recognition is impaired by subthalamic nucleus stimulation in Parkinson’s disease. Neuropsychologia 43, 1054–1059. doi: 10.1016/j.neuropsychologia.2004.10.006
Blair, R., Morris, J. S., Frith, C. D., Perrett, D. I., and Dolan, R. J. (1999). Dissociable neural responses to facial expressions of sadness and anger. Brain 122, 883–893. doi: 10.1093/brain/122.5.883
Bodden, M. E., Dodel, R., and Kalbe, E. (2010). Theory of mind in Parkinson’s disease and related basal ganglia disorders: a systematic review. Mov. Disord. 25, 13–27. doi: 10.1002/mds.22818
Borroni, B., Premi, E., Formenti, A., Turrone, R., Alberici, A., Cottini, E., et al. (2015). Structural and functional imaging study in dementia with Lewy bodies and Parkinson’s disease dementia. Parkinsonism Relat. Disord. 21, 1049–1055. doi: 10.1016/j.parkreldis.2015.06.013
Breiter, H. C., Etcoff, N. L., Whalen, P. J., Kennedy, W. A., Rauch, S. L., Buckner, R. L., et al. (1996). Response and habituation of the human amygdala during visual processing of facial expression. Neuron 17, 875–887. doi: 10.1016/s0896-6273(00)80219-6
Buckner, R. L., Snyder, A. Z., Shannon, B. J., LaRossa, G., Sachs, R., Fotenos, A. F., et al. (2005). Molecular, structural, and functional characterization of Alzheimer’s disease: evidence for a relationship between default activity, amyloid, and memory. J. Neurosci. 25, 7709–7717. doi: 10.1523/jneurosci.2177-05.2005
Burnham, H., and Hogervorst, E. (2004). Recognition of facial expressions of emotion by patients with dementia of the Alzheimer type. Dementi. $geriatr. Cogn. Disord. 18, 75–79. doi: 10.1159/000077813
Caminiti, S. P., Canessa, N., Cerami, C., Dodich, A., Crespi, C., Iannaccone, S., et al. (2015). Affective mentalizing and brain activity at rest in the behavioral variant of frontotemporal dementia. NeuroImage: Clin. 9, 484–497. doi: 10.1016/j.nicl.2015.08.012
Campbell, M. C., Koller, J. M., Snyder, A. Z., Buddhala, C., Kotzbauer, P. T., and Perlmutter, J. S. (2015). CSF proteins and resting-state functional connectivity in Parkinson disease. Neurology 84, 2413–2421. doi: 10.1212/WNL.0000000000001681
Cancelliere, A. E., and Kertesz, A. (1990). Lesion localization in acquired deficits of emotional expression and comprehension. Brain Cogn. 13, 133–147. doi: 10.1016/0278-2626(90)90046-q
Canu, E., Agosta, F., Sarasso, E., Volontè, M. A., Basaia, S., Stojkovic, T., et al. (2015). Brain structural and functional connectivity in P arkinson’s disease with freezing of gait. Hum. Brain Mapp. 36, 5064–5078. doi: 10.1002/hbm.22994
Carr, L., Iacoboni, M., Dubeau, M.-C., Mazziotta, J. C., and Lenzi, G. L. (2003). Neural mechanisms of empathy in humans: a relay from neural systems for imitation to limbic areas. Proc. Natl. Acad. Sci. U.S.A. 100, 2.
Carver, C. S., and White, T. L. (1994). Behavioral inhibition, behavioral activation, and affective responses to impending reward and punishment: the BIS/BAS scales. J. Personal. Soc. Psychol. 67, 319–333. doi: 10.1037/0022-3514.67.2.319
Caso, F., Agosta, F., and Filippi, M. (2016). Insights into white matter damage in Alzheimer’s disease: from postmortem to in vivo diffusion tensor MRI studies. Neurodegener. Dis. 16, 26–33. doi: 10.1159/000441422
Chen, Y., Yang, W., Long, J., Zhang, Y., Feng, J., Li, Y., et al. (2015). Discriminative analysis of Parkinson’s disease based on whole-brain functional connectivity. PloS One 10:e0124153. doi: 10.1371/journal.pone.0124153
Cheng, Y., Lin, C.-P., Liu, H.-L., Hsu, Y.-Y., Lim, K.-E., Hung, D., et al. (2007). Expertise modulates the perception of pain in others. Curr. Biol. 17, 1708–1713. doi: 10.1016/j.cub.2007.09.020
Ciccarelli, O., Catani, M., Johansen-Berg, H., Clark, C., and Thompson, A. (2008). Diffusion-based tractography in neurological disorders: concepts, applications, and future developments. Lancet Neurol. 7, 715–727. doi: 10.1016/S1474-4422(08)70163-7
Davis, M. H. (1983). Measuring individual differences in empathy: Evidence for a multidimensional approach. J. Personal. Soc. Psychol. 44, 113–126. doi: 10.1037//0022-3514.44.1.113
Day, G. S., Farb, N. A., Tang-Wai, D. F., Masellis, M., Black, S. E., Freedman, M., et al. (2013). Salience network resting-state activity: prediction of frontotemporal dementia progression. JAMA Neurol. 0, 1249–1253.
Dermody, N., Wong, S., Ahmed, R., Piguet, O., Hodges, J. R., and Irish, M. (2016). Uncovering the neural bases of cognitive and affective empathy deficits in Alzheimer’s disease and the behavioral-variant of frontotemporal dementia. J. Alzheimer’s Dis. 53, 801–816. doi: 10.3233/jad-160175
Diehl-Schmid, J., Pohl, C., Ruprecht, C., Wagenpfeil, S., Foerstl, H., and Kurz, A. (2007). The Ekman 60 Faces Test as a diagnostic instrument in frontotemporal dementia. Arch. Clin. Neuropsychol. 22, 459–464. doi: 10.1016/j.acn.2007.01.024
Disbrow, E., Carmichael, O., He, J., Lanni, K., Dressler, E., Zhang, L., et al. (2014). Resting state functional connectivity is associated with cognitive dysfunction in non-demented people with Parkinson’s disease. J. Parkinson’s Dis. 4, 453–465.
Dujardin, K., Blairy, S., Defebvre, L., Duhem, S., Noël, Y., Hess, U., et al. (2004). Deficits in decoding emotional facial expressions in Parkinson’s disease. Neuropsychologia 42, 239–250. doi: 10.1016/s0028-3932(03)00154-4
Elahi, F. M., Marx, G., Cobigo, Y., Staffaroni, A. M., Kornak, J., Tosun, D., et al. (2017). Longitudinal white matter change in frontotemporal dementia subtypes and sporadic late onset Alzheimer’s disease. NeuroImage: Clin. 16, 595–603. doi: 10.1016/j.nicl.2017.09.007
Elamin, M., Pender, N., Hardiman, O., and Abrahams, S. (2012). Social cognition in neurodegenerative disorders: a systematic review. J. Neurol. Neurosurg. Psychiatry 83, 1071–1079. doi: 10.1136/jnnp-2012-302817
Eslinger, P. J., Moore, P., Troiani, V., Antani, S., Cross, K., Kwok, S., et al. (2007). Oops! resolving social dilemmas in frontotemporal dementia. J. Neurol. Neurosurg. Psychiatry 78, 457–460. doi: 10.1136/jnnp.2006.098228
Farb, N. A., Grady, C. L., Strother, S., Tang-Wai, D. F., Masellis, M., Black, S., et al. (2013). Abnormal network connectivity in frontotemporal dementia: evidence for prefrontal isolation. Cortex 49, 1856–1873. doi: 10.1016/j.cortex.2012.09.008
Filippi, M., Agosta, F., Scola, E., Canu, E., Magnani, G., Marcone, A., et al. (2013). Functional network connectivity in the behavioral variant of frontotemporal dementia. Cortex 49, 2389–2401. doi: 10.1016/j.cortex.2012.09.017
Freedman, M., Leach, L., Tartaglia, M. C., Stokes, K. A., Goldberg, Y., Spring, R., et al. (2018). The toronto cognitive assessment (TorCA): normative data and validation to detect amnestic mild cognitive impairment. Alzheimer’s Res. Ther. 10:65.
Fusar-Poli, P., Placentino, A., Carletti, F., Landi, P., Allen, P., Surguladze, S., et al. (2009). Functional atlas of emotional faces processing: a voxel-based meta-analysis of 105 functional magnetic resonance imaging studies. J. Psychiatry Neurosci. 34, 418–432.
Galantucci, S., Tartaglia, M. C., Wilson, S. M., Henry, M. L., Filippi, M., Agosta, F., et al. (2011). White matter damage in primary progressive aphasias: a diffusion tensor tractography study. Brain 134(Pt 10), 3011–3029. doi: 10.1093/brain/awr099
Geday, J., Gjedde, A., Boldsen, A.-S., and Kupers, R. (2003). Emotional valence modulates activity in the posterior fusiform gyrus and inferior medial prefrontal cortex in social perception. Neuroimage 18, 675–684. doi: 10.1016/s1053-8119(02)00038-1
Gili, T., Cercignani, M., Serra, L., Perri, R., Giove, F., Maraviglia, B., et al. (2011). Regional brain atrophy and functional disconnection across Alzheimer’s disease evolution. J. Neurology, Neurosurg. Psychiatry 82, 58–66. doi: 10.1136/jnnp.2009.199935
Gorges, M., Müller, H.-P., Lulé, D., Pinkhardt, E. H., Ludolph, A. C., Kassubek, J., et al. (2015). To rise and to fall: functional connectivity in cognitively normal and cognitively impaired patients with Parkinson’s disease. Neurobiol. Aging 36, 1727–1735. doi: 10.1016/j.neurobiolaging.2014.12.026
Gorno-Tempini, M. L., Hillis, A. E., Weintraub, S., Kertesz, A., Mendez, M., Cappa, S. F., et al. (2011). Classification of primary progressive aphasia and its variants. Neurology 76, 1006–1014. doi: 10.1212/WNL.0b013e31821103e6
Gregory, C., Lough, S., Stone, V., Erzinclioglu, S., Martin, L., Baron−Cohen, S., et al. (2002). Theory of mind in patients with frontal variant frontotemporal dementia and Alzheimer’s disease: theoretical and practical implications. Brain 125, 752–764. doi: 10.1093/brain/awf079
Greicius, M. D., Krasnow, B., Reiss, A. L., and Menon, V. (2003). Functional connectivity in the resting brain: a network analysis of the default mode hypothesis. Proc. Natl. Acad. Sci. U.S.A, 100, 253–258. doi: 10.1073/pnas.0135058100
Greicius, M. D., Srivastava, G., Reiss, A. L., and Menon, V. (2004). Default-mode network activity distinguishes Alzheimer’s disease from healthy aging: evidence from functional MRI. Proc. Natl. Acad. Sci. U.S.A. 101, 4637–4642. doi: 10.1073/pnas.0308627101
Grossman, M., Eslinger, P. J., Troiani, V., Anderson, C., Avants, B., Gee, J. C., et al. (2010). The role of ventral medial prefrontal cortex in social decisions: converging evidence from fMRI and frontotemporal lobar degeneration. Neuropsychologia 8, 3505–3512. doi: 10.1016/j.neuropsychologia.2010.07.036
Gustafson, L. (1987). Frontal lobe degeneration of non-Alzheimer type. II. Clinical picture and differential diagnosis. Arch. Gerontol. Geriat. 6, 209–223. doi: 10.1016/0167-4943(87)90022-7
Hacker, C. D., Perlmutter, J. S., Criswell, S. R., Ances, B. M., and Snyder, A. Z. (2012). Resting state functional connectivity of the striatum in Parkinson’s disease. Brain 135, 3699–3711. doi: 10.1093/brain/aws281
Hafkemeijer, A., Möller, C., Dopper, E. G., Jiskoot, L. C., Schouten, T. M., van Swieten, J. C., et al. (2015). Resting state functional connectivity differences between behavioral variant frontotemporal dementia and Alzheimer’s disease. Front. Hum. Neurosci. 9:474. doi: 10.3389/fnhum.2015.00474
Hargrave, R., Maddock, R. J., and Stone, V. (2002). Impaired recognition of facial expressions of emotion in Alzheimer’s disease. J. Neuropsychiatry Clin. Neurosci. 14, 64–71. doi: 10.1176/jnp.14.1.64
Hayes, A. F. (2009). Beyond Baron and Kenny: statistical mediation analysis in the new millennium. Commun. Monogr. 76, 408–420. doi: 10.1080/03637750903310360
Hayes, A. F. (2013). Introduction to Mediation, Moderation, and Conditional Process Analysis: A Regression-Based Approach. New York, NY: Guilford Press.
He, Y., Wang, L., Zang, Y., Tian, L., Zhang, X., Li, K., et al. (2007). Regional coherence changes in the early stages of Alzheimer’s disease: a combined structural and resting-state functional MRI study. Neuroimage 35, 488–500. doi: 10.1016/j.neuroimage.2006.11.042
Hein, G., and Singer, T. (2008). I feel how you feel but not always: the empathic brain and its modulation. Curr. Opin. Neurobiol. 18, 153–158. doi: 10.1016/j.conb.2008.07.012
Helmich, R. C., Derikx, L. C., Bakker, M., Scheeringa, R., Bloem, B. R., and Toni, I. (2009). Spatial remapping of cortico-striatal connectivity in Parkinson’s disease. Cereb. Cortex 20, 1175–1186. doi: 10.1093/cercor/bhp178
Hodges, J. R., and Miller, B. (2001). The classification, genetics and neuropathology of frontotemporal dementia. Int. Spec. Top. Pap. Part I. Neurocase 7, 31–35. doi: 10.1076/neur.7.1.31.16209
Hofmann, S. G. (2006). The emotional consequences of social pragmatism: the psychophysiological correlates of self-monitoring. Biol. Psychol. 3, 169–174. doi: 10.1016/j.biopsycho.2006.03.001
Hornak, J., Bramham, J., Rolls, E. T., Morris, R. G., O’Doherty, J., Bullock, P., et al. (2003). Changes in emotion after circumscribed surgical lesions of the orbitofrontal and cingulate cortices. Brain 26, 1691–1712. doi: 10.1093/brain/awg168
Hu, X., Song, X., Yuan, Y., Li, E., Liu, J., Liu, W., et al. (2015). Abnormal functional connectivity of the amygdala is associated with depression in Parkinson’s disease. Mov. Disord. 30, 238–244. doi: 10.1002/mds.26087
Hughes, A. J., Daniel, S. E., Kilford, L., and Lees, A. J. (1992). Accuracy of clinical diagnosis of idiopathic Parkinson’s disease: a clinico-pathological study of 100 cases. J. Neurol. Neurosurg. Psychiatry 5, 181–184. doi: 10.1136/jnnp.55.3.181
Ibarretxe-Bilbao, N., Junque, C., Tolosa, E., Marti, M.-J., Valldeoriola, F., Bargallo, N., et al. (2009). Neuroanatomical correlates of impaired decision-making and facial emotion recognition in early Parkinson’s disease. Eur. J. Neurosci. 30, 1162–1171. doi: 10.1111/j.1460-9568.2009.06892.x
Jabbi, M., and Keysers, C. (2008). Inferior frontal gyrus activity triggers anterior insula response to emotional facial expressions. Emotion 8, 775–780. doi: 10.1037/a0014194
Jabbi, M., Swart, M., and Keysers, C. (2007). Empathy for positive and negative emotions in the gustatory cortex. Neuroimage 34, 1744–1753. doi: 10.1016/j.neuroimage.2006.10.032
Jones, D. T., Vemuri, P., Murphy, M. C., Gunter, J. L., Senjem, M. L., Machulda, M. M., et al. (2012). Non-stationarity in the “resting brain’s” modular architecture. PloS One 7:e39731. doi: 10.1371/journal.pone.0039731
Kan, Y., Kawamura, M., Hasegawa, Y., Mochizuki, S., and Nakamura, K. (2002). Recognition of emotion from facial, prosodic and written verbal stimuli in Parkinson’s disease. Cortex 38, 623–630. doi: 10.1016/s0010-9452(08)70026-1
Kawamura, M., and Koyama, S. (2007). Social cognitive impairment in Parkinson’s disease. J. Neurol. 254, IV49–IV53.
Keane, J., Calder, A. J., Hodges, J. R., and Young, A. W. (2002). Face and emotion processing in frontal variant frontotemporal dementia. Neuropsychologia 40, 655–665. doi: 10.1016/s0028-3932(01)00156-7
Kessels, R. P., Gerritsen, L., Montagne, B., Ackl, N., Diehl, J., and Danek, A. (2007). Recognition of facial expressions of different emotional intensities in patients with frontotemporal lobar degeneration. Behav. Neurol. 18, 31–36. doi: 10.1155/2007/868431
Koch, K., Myers, N. E., Göttler, J., Pasquini, L., Grimmer, T., Förster, S., et al. (2014). Disrupted intrinsic networks link amyloid-β pathology and impaired cognition in prodromal Alzheimer’s disease. Cereb. Cortex 25, 4678–4688. doi: 10.1093/cercor/bhu151
Koff, E., Brownell, H., Winner, E., Albert, M., and Zaitchik, D. (2004). Inference of mental states in patients with Alzheimer’s disease. Cogn. Neuropsyc. 9, 301–313. doi: 10.1080/13546800344000246
Kohler, C. G., Anselmo-Gallagher, G., Bilker, W., Karlawish, J., Gur, R. E., and Clark, C. M. (2005). Emotion-discrimination deficits in mild Alzheimer disease. Am. J. Geriatr. Psychiatry 13, 926–933. doi: 10.1176/appi.ajgp.13.11.926
Krajcovicova, L., Mikl, M., Marecek, R., and Rektorova, I. (2012). The default mode network integrity in patients with Parkinson’s disease is levodopa equivalent dose-dependent. J. Neural Transm. 119, 443–454. doi: 10.1007/s00702-011-0723-5
Krolak-Salmon, P., Hénaff, M., Isnard, J., Tallon-Baudry, C., Guénot, M., Vighetto, A., et al. (2003). An attention modulated response to disgust in human ventral anterior insula. Ann. Neurol. 53, 446–453. doi: 10.1002/ana.10502
Kwak, Y., Peltier, S., Bohnen, N., Müller, M., Dayalu, P., and Seidler, R. D. (2010). Altered resting state cortico-striatal connectivity in mild to moderate stage Parkinson’s disease. Front. Syst. Neurosci. 4:143. doi: 10.3389/fnsys.2010.00143
Lamm, C., Batson, C. D., and Decety, J. (2007). The neural substrate of human empathy: effects of perspective-taking and cognitive appraisal. J. Cogn. Neurosci. 19, 42–58. doi: 10.1162/jocn.2007.19.1.42
Lamm, C., and Singer, T. (2010). The role of anterior insular cortex in social emotions. Brain Struct. Funct. 214, 579–591. doi: 10.1007/s00429-010-0251-3
Lavenu, I., and Pasquier, F. (2005). Perception of emotion on faces in frontotemporal dementia and Alzheimer’s disease: a longitudinal study. Dement. Geriatr. Cogn. Disord. 19, 37–41. doi: 10.1159/000080969
Lawrence, E., Shaw, P., Giampietro, V., Surguladze, S., Brammer, M., and David, A. (2006). The role of ‘shared representations’ in social perception and empathy: an fMRI study. Neuroimage 9, 1173–1184. doi: 10.1016/j.neuroimage.2005.09.001
Lennox, R. D., and Wolfe, R. N. (1984). Revision of the self-monitoring scale. J. Personal. Soc. Psychol. 46, 1349–1364. doi: 10.1037/0022-3514.46.6.1349
Liu, Y., Yu, C., Zhang, X., Liu, J., Duan, Y., Alexander-Bloch, A. F., et al. (2013). Impaired long distance functional connectivity and weighted network architecture in Alzheimer’s disease. Cereb. Cortex 4, 1422–1435. doi: 10.1093/cercor/bhs410
Lough, S., Kipps, C. M., Treise, C., Watson, P., Blair, J. R., and Hodges, J. R. (2006). Social reasoning, emotion and empathy in frontotemporal dementia. Neuropsychologia 4, 950–958. doi: 10.1016/j.neuropsychologia.2005.08.009
Luo, C., Guo, X., Song, W., Zhao, B., Cao, B., Yang, J., et al. (2015). Decreased resting-state interhemispheric functional connectivity in Parkinson’s disease. BioMed Res. Int. 2015:692684.
Luo, C., Song, W., Chen, Q., Zheng, Z., Chen, K., Cao, B., et al. (2014). Reduced functional connectivity in early-stage drug-naive Parkinson’s disease: a resting-state fMRI study. Neurobiol. Aging 35, 431–441. doi: 10.1016/j.neurobiolaging.2013.08.018
Luzzi, S., Piccirilli, M., and Provinciali, L. (2007). Perception of emotions on happy/sad chimeric faces in Alzheimer disease: relationship with cognitive functions. Alzheimer Dis. Assoc. Disord. 21, 130–135. doi: 10.1097/wad.0b013e318064f445
Madhyastha, T. M., Askren, M. K., Zhang, J., Leverenz, J. B., Montine, T. J., and Grabowski, T. J. (2015). Group comparison of spatiotemporal dynamics of intrinsic networks in Parkinson’s disease. Brain 138, 2672–2686. doi: 10.1093/brain/awv189
Martinez, M., Multani, N., Anor, C. J., Misquitta, K., Tang-Wai, D. F., Keren, R., et al. (2018). Emotion detection deficits and decreased empathy in patients with alzheimer’s disease and Parkinson’s Disease affect caregiver mood and burden. Front. Aging Neurosci. 10:120. doi: 10.3389/fnagi.2018.00120
McDonald, S., Flanagan, S., Rollins, J., and Kinch, J. (2003). TASIT: a new clinical tool for assessing social perception after traumatic brain injury. J. Head Trauma Rehabil. 18, 219–238. doi: 10.1097/00001199-200305000-00001
McKhann, G. M., Knopman, D. S., Chertkow, H., Hyman, B. T., Jack, C. R., Kawas, C. H., et al. (2011). The diagnosis of dementia due to Alzheimer’s disease: recommendations from the national institute on Aging-Alzheimer’s association workgroups on diagnostic guidelines for Alzheimer’s disease. Alzheimer’s Dement. 7, 263–269.
Mendez, M. F., Anderson, E., and Shapira, J. S. (2005). An investigation of moral judgement in frontotemporal dementia. Cogn. Behav. Neurol. 18, 193–197. doi: 10.1097/01.wnn.0000191292.17964.bb
Mengelberg, A., and Siegert, R. (2003). Is theory-of-mind impaired in Parkinson’s disease? Cogn. Neuropsyc. 8, 191–209. doi: 10.1080/13546800244000292
Mimura, M., Oeda, R., and Kawamura, M. (2006). Impaired decision-making in Parkinson’s disease. Parkinsonism Relat. Disord. 12, 169–175. doi: 10.1016/j.parkreldis.2005.12.003
Morris, J. C. (1993). The clinical dementia rating (CDR): current version and scoring rules. Neurology 43, 2412–2414.
Morris, J. S., Frith, C. D., Perrett, D. I., Rowland, D., Young, A. W., Calder, A. J., et al. (1996). A differential neural response in the human amygdala to fearful and happy facial expressions. Nature 383, 812–815. doi: 10.1038/383812a0
Multani, N., Anor, C. J., Tang-Wai, D. F., Keren, R., Fox, S., Lang, A. E., et al. (2016). Functional connectivity of the anterior cingulate cortex in Alzheimer’s disease. J. Alzheimer’s Assoc. 12:922. doi: 10.1016/j.jpsychires.2013.03.018
Multani, N., Galantucci, S., Wilson, S. M., Shany-Ur, T., Poorzand, P., Growdon, M. E., et al. (2017). Emotion detection deficits and changes in personality traits linked to loss of white matter integrity in primary progressive aphasia. NeuroImage Clin. 16, 447–454. doi: 10.1016/j.nicl.2017.08.020
Neary, D., Snowden, J. S., Gustafson, L., Passant, U., Stuss, D., Black, S., et al. (1998). Frontotemporal lobar degeneration A consensus on clinical diagnostic criteria. Neurology 51, 1546–1554. doi: 10.1212/wnl.51.6.1546
Neary, D., Snowden, J. S., Northen, B., and Goulding, P. (1988). Dementia of frontal lobe type. J. Neurol. Neurosurg. Psychiatry 51, 353–361.
Oishi, K., Faria, A. V., Hsu, J., Tippett, D., Mori, S., and Hillis, A. E. (2015). Critical role of the right uncinate fasciculus in emotional empathy. Ann. Neurol. 77, 68–74. doi: 10.1002/ana.24300
Onu, M., Badea, L., Roceanu, A., Tivarus, M., and Bajenaru, O. (2015). Increased connectivity between sensorimotor and attentional areas in Parkinson’s disease. Neuroradiology 57, 957–968. doi: 10.1007/s00234-015-1556-y
Panchal, H., Paholpak, P., Lee, G., Carr, A., Barsuglia, J. P., Mather, M., et al. (2016). Neuropsychological and neuroanatomical correlates of the social norms questionnaire in frontotemporal dementia versus alzheimer’s disease. Am. J. Alzheimer’s Dis. Other Dementias 31, 326–332. doi: 10.1177/1533317515617722
Pell, M. D., and Leonard, C. L. (2003). Processing emotional tone from speech in Parkinson’s disease: a role for the basal ganglia. Cogn. Affect. Behav. Neurosci. 3, 275–288. doi: 10.3758/cabn.3.4.275
Pell, M. D., and Leonard, C. L. (2005). Facial expression decoding in early Parkinson’s disease. Cogn. Brain Res. 23, 327–340. doi: 10.1016/j.cogbrainres.2004.11.004
Pelphrey, K. A., Morris, J. P., and Mccarthy, G. (2004). Grasping the intentions of others: the perceived intentionality of an action influences activity in the superior temporal sulcus during social perception. J. Cogn. Neurosci. 16, 1706–1716. doi: 10.1162/0898929042947900
Phillips, M. L., Young, A. W., Senior, C., Brammer, M., Andrew, C., Calder, A. J., et al. (1997). A specific neural substrate for perceiving facial expressions of disgust. Nature 389, 495–498. doi: 10.1038/39051
Poveda, B., Osborne-Crowley, K., Laidlaw, K., Macleod, F., and Power, K. (2017). Social cognition, behaviour and relationship continuity in dementia of the Alzheimer type. Brain Impairment 18, 175–187. doi: 10.1016/j.cortex.2018.02.012
Preacher, K. J., and Kelley, K. (2011). Effect size measures for mediation models: quantitative strategies for communicating indirect effects. Psychol. Methods 16, 93–115. doi: 10.1037/a0022658
Putcha, D., Ross, R. S., Cronin-Golomb, A., Janes, A. C., and Stern, C. E. (2015). Altered intrinsic functional coupling between core neurocognitive networks in Parkinson’s disease. NeuroImage Clin. 7, 449–455. doi: 10.1016/j.nicl.2015.01.012
Raichle, M. E., MacLeod, A. M., Snyder, A. Z., Powers, W. J., Gusnard, D. A., and Shulman, G. L. (2001). A default mode of brain function. Proc. Nat. Acad. Sci. U.S.A. 98, 676–682.
Rankin, K. (2008). Social Norms Questionaire. Domain Specific Tasks of Executive Function Bethesda. MA: NINDS.
Rascovsky, K., Grossman, M., Hillis, A. E., Kertesz, A., Johnson, J. K., Kipps, C. M., et al. (2011). Sensitivity of revised diagnostic criteria for the behavioural variant of frontotemporal dementia. Brain 134, 2456–2477. doi: 10.1093/brain/awr179
Rolinski, M., Griffanti, L., Szewczyk-Krolikowski, K., Menke, R. A., Wilcock, G. K., Filippini, N., et al. (2015). Aberrant functional connectivity within the basal ganglia of patients with Parkinson’s disease. NeuroImage Clin. 8, 126–132. doi: 10.1016/j.nicl.2015.04.003
Rosen, H. J., Pace-Savitsky, K., Perry, R. J., Kramer, J. H., Miller, B. L., and Levenson, R. W. (2004). Recognition of emotion in the frontal and temporal variants of frontotemporal dementia. Dementia Geriatr. Cogn. Disord. 17, 277–281. doi: 10.1159/000077154
Ruby, P., and Decety, J. (2003). What you believe versus what you think they believe: a neuroimaging study of conceptual perspective−taking. Eur. J. Neurosci. 17, 2475–2480. doi: 10.1046/j.1460-9568.2003.02673.x
Rytty, R., Nikkinen, J., Paavola, L., Abou Elseoud, A., Moilanen, V., Visuri, A., et al. (2013). GroupICA dual regression analysis of resting state networks in a behavioral variant of frontotemporal dementia. Front. Hum. Neurosci. 7:461. doi: 10.3389/fnhum.2013.00461
Saltzman, J., Strauss, E., Hunter, M., and Archibald, S. (2000). Theory of mind and executive functions in normal human aging and Parkinson’s disease. J. Int. Neuropsychol. Soc. 6, 781–788. doi: 10.1017/s1355617700677056
Sawamoto, N., Piccini, P., Hotton, G., Pavese, N., Thielemans, K., and Brooks, D. J. (2008). Cognitive deficits and striato-frontal dopamine release in Parkinson’s disease. Brain 131, 1294–1302. doi: 10.1093/brain/awn054
Sedeño, L., Couto, B., Garcia-Cordero, I., Melloni, M., Baez, S., Sepúlveda, J. P. M., et al. (2016). Brain network organization and social executive performance in frontotemporal dementia. J. Int. Neuropsychol. Soc. 22, 250–262. doi: 10.1017/s1355617715000703
Seeley, W. W., Crawford, R. K., Zhou, J., Miller, B. L., and Greicius, M. D. (2009). Neurodegenerative diseases target large-scale human brain networks. Neuron 62, 42–52. doi: 10.1016/j.neuron.2009.03.024
Shany-Ur, T., Poorzand, P., Grossman, S. N., Growdon, M. E., Jang, J. Y., Ketelle, R. S., et al. (2012). Comprehension of insincere communication in neurodegenerative disease: lies, sarcasm, and theory of mind. Cortex 48, 1329–1341. doi: 10.1016/j.cortex.2011.08.003
Shany-Ur, T., and Rankin, K. P. (2011). Personality and social cognition in neurodegenerative disease. Curr. Opin. Neurol. 24, 550–555. doi: 10.1097/WCO.0b013e32834cd42a
Shdo, S. M., Ranasinghe, K. G., Gola, K. A., Mielke, C. J., Sukhanov, P. V., Miller, B. L., et al. (2018). Deconstructing empathy: neuroanatomical dissociations between affect sharing and prosocial motivation using a patient lesion model. Neuropsychologia 116, 126–135. doi: 10.1016/j.neuropsychologia.2017.02.010
Shingala, M. C., and Rajyaguru, A. (2015). Comparison of post hoc tests for unequal variance. Int. J. N. Technol. Sci. Eng. 2, 22–33.
Silani, G., Lamm, C., Ruff, C. C., and Singer, T. (2013). Right supramarginal gyrus is crucial to overcome emotional egocentricity bias in social judgments. J. Neurosci. 33, 15466–15476. doi: 10.1523/JNEUROSCI.1488-13.2013
Skidmore, F., Yang, M., Baxter, L., Von Deneen, K., Collingwood, J., He, G., et al. (2013). Reliability analysis of the resting state can sensitively and specifically identify the presence of Parkinson disease. Neuroimage 75, 249–261. doi: 10.1016/j.neuroimage.2011.06.056
Skuse, D. H., and Gallagher, L. (2009). Dopaminergic-neuropeptide interactions in the social brain. Trends Cogn. Sci. 13, 27–35. doi: 10.1016/j.tics.2008.09.007
Snowden, J., Gibbons, Z., Blackshaw, A., Doubleday, E., Thompson, J., Craufurd, D., et al. (2003). Social cognition in frontotemporal dementia and Huntington’s disease. Neuropsychologia 41, 688–701. doi: 10.1016/s0028-3932(02)00221-x
Sollberger, M., Rosen, H. J., Shany-Ur, T., Ullah, J., Stanley, C. M., Laluz, V., et al. (2014). Neural substrates of socioemotional self-awareness in neurodegenerative disease. Brain Behav. 4, 201–214. doi: 10.1002/brb3.211
Sorg, C., Riedl, V., Mühlau, M., Calhoun, V. D., Eichele, T., Läer, L., et al. (2007). Selective changes of resting-state networks in individuals at risk for Alzheimer’s disease. Proc. Natl. Acad. Sci. 04, 18760–18765. doi: 10.1073/pnas.0708803104
Spoletini, I., Marra, C., Di Iulio, F., Gianni, W., Sancesario, G., Giubilei, F., et al. (2008). Facial emotion recognition deficit in amnestic mild cognitive impairment and Alzheimer disease. Am. J. Geriat. Psychiatry 6, 389–398. doi: 10.1097/JGP.0b013e318165dbce
Sprengelmeyer, R., Rausch, M., Eysel, U. T., and Przuntek, H. (1998). Neural structures associated with recognition of facial expressions of basic emotions. Proc. R. Soc. Lon. Ser. B Biol. Sci. 265, 1927–1931. doi: 10.1098/rspb.1998.0522
Sprengelmeyer, R., Young, A., Mahn, K., Schroeder, U., Woitalla, D., Büttner, T., et al. (2003). Facial expression recognition in people with medicated and unmedicated Parkinson’s disease. Neuropsychologia 41, 1047–1057. doi: 10.1016/s0028-3932(02)00295-6
Starkstein, S. E., Federoff, J. P., Price, T. R., Leiguarda, R. C., and Robinson, R. G. (1994). Neuropsychological and neuroradiologic correlates of emotional prosody comprehension. Neurology 44, 515–522.
Szewczyk-Krolikowski, K., Menke, R. A., Rolinski, M., Duff, E., Salimi-Khorshidi, G., Filippini, N., et al. (2014). Functional connectivity in the basal ganglia network differentiates PD patients from controls. Neurology 83, 208–214. doi: 10.1212/WNL.0000000000000592
Taghdiri, F., Chung, J., Irwin, S., Multani, N., Tarazi, A., Ebraheem, A., et al. (2018). Decreased number of self-paced saccades in post-concussion syndrome associated with higher symptom burden and reduced white matter integrity. J. Neurotrauma 35, 719–729. doi: 10.1089/neu.2017.5274
Tessitore, A., Esposito, F., Vitale, C., Santangelo, G., Amboni, M., Russo, A., et al. (2012). Default-mode network connectivity in cognitively unimpaired patients with Parkinson disease. Neurology 79, 2226–2232. doi: 10.1212/WNL.0b013e31827689d6
Torralva, T., Dorrego, F., Sabe, L., Chemerinski, E., and Starkstein, S. E. (2000). Impairments of social cognition and decision making in Alzheimer’s disease. Int. Psychogeriat. 12, 359–368. doi: 10.1017/s1041610200006463
Von Der Heide, R. J., Skipper, L. M., Klobusicky, E., and Olson, I. R. (2013). Dissecting the uncinate fasciculus: disorders, controversies and a hypothesis. Brain 136, 1692–1707. doi: 10.1093/brain/awt094
Wang, K., Liang, M., Wang, L., Tian, L., Zhang, X., Li, K., et al. (2007). Altered functional connectivity in early Alzheimer’s disease: a resting−state fMRI study. Hum. Brain Mapp. 28, 967–978.
Weiler, M., Fukuda, A. H. P., Massabki, L. M., Lopes, T. R., Franco, A. P., Damasceno, B., et al. (2014). default mode, executive function, and language functional connectivity networks are compromised in mild Alzheimer’ s Disease. Curr. Alzheimer Res. 11, 274–282. doi: 10.2174/1567205011666140131114716
Werner, K., Roberts, N., Rosen, H., Dean, D., Kramer, J., Weiner, M., et al. (2007). Emotional reactivity and emotion recognition in frontotemporal lobar degeneration. Neurology 69, 148–155. doi: 10.1212/01.wnl.0000265589.32060.d3
Whitfield-Gabrieli, S., and Nieto-Castanon, A. (2012). Conn: a functional connectivity toolbox for correlated and anticorrelated brain networks. Brain Connect. 2, 125–141. doi: 10.1089/brain.2012.0073
Wicker, B., Keysers, C., Plailly, J., Royet, J.-P., Gallese, V., and Rizzolatti, G. (2003). Both of us disgusted in My insula: the common neural basis of seeing and feeling disgust. Neuron 40, 655–664. doi: 10.1016/s0896-6273(03)00679-2
Wu, T., Wang, L., Chen, Y., Zhao, C., Li, K., and Chan, P. (2009). Changes of functional connectivity of the motor network in the resting state in Parkinson’s disease. Neurosci. Lett. 460, 6–10. doi: 10.1016/j.neulet.2009.05.046
Wu, X., Li, R., Fleisher, A. S., Reiman, E. M., Guan, X., Zhang, Y., et al. (2011). Altered default mode network connectivity in Alzheimer’s disease—a resting functional MRI and bayesian network study. Hum. Brain Mapp. 2, 1868–1881. doi: 10.1002/hbm.21153
Yip, J. T., Lee, T. M., Ho, S., Tsang, K., and Li, L. S. (2003). Emotion recognition in patients with idiopathic Parkinson’s disease. Mov. Disord. 18, 1115–1122. doi: 10.1002/mds.10497
Yoshimura, N., Kawamura, M., Masaoka, Y., and Homma, I. (2005). The amygdala of patients with Parkinson’s disease is silent in response to fearful facial expressions. Neuroscience 131, 523–534. doi: 10.1016/j.neuroscience.2004.09.054
Yu, R.-L., Wu, R.-M., Chiu, M.-J., Tai, C.-H., Lin, C.-H., and Hua, M.-S. (2012). Advanced Theory of Mind in patients at early stage of Parkinson’s disease. Parkinsonism Relat. Disord. 18, 21–24. doi: 10.1016/j.parkreldis.2011.08.003
Zgaljardic, D. J., Borod, J. C., Foldi, N. S., Mattis, P. J., Gordon, M. F., Feigin, A., et al. (2006). An examination of executive dysfunction associated with frontostriatal circuitry in Parkinson’s disease. J. Clin. Exp. Neuropsychol. 28, 1127–1144. doi: 10.1080/13803390500246910
Zhang, Y., Schuff, N., Du, A.-T., Rosen, H. J., Kramer, J. H., Gorno-Tempini, M. L., et al. (2009). White matter damage in frontotemporal dementia and Alzheimer’s disease measured by diffusion MRI. Brain 132, 2579–2592. doi: 10.1093/brain/awp071
Zhou, B., Yao, H., Wang, P., Zhang, Z., Zhan, Y., Ma, J., et al. (2015). Aberrant functional connectivity architecture in Alzheimer’s disease and mild cognitive impairment: a whole-brain, data-driven analysis. BioMed Res. Int. 2015:495375.
Keywords: neurodegeneration, social cognition, resting-state fMRI, neuroimage analysis, functional connectivity
Citation: Multani N, Taghdiri F, Anor CJ, Varriano B, Misquitta K, Tang-Wai DF, Keren R, Fox S, Lang AE, Vijverman AC, Marras C and Tartaglia MC (2019) Association Between Social Cognition Changes and Resting State Functional Connectivity in Frontotemporal Dementia, Alzheimer’s Disease, Parkinson’s Disease, and Healthy Controls. Front. Neurosci. 13:1259. doi: 10.3389/fnins.2019.01259
Received: 11 June 2019; Accepted: 06 November 2019;
Published: 22 November 2019.
Edited by:
Gianfranco Spalletta, Santa Lucia Foundation (IRCCS), ItalyReviewed by:
Jiu Chen, Nanjing Medical University, ChinaFabrizio Piras, Santa Lucia Foundation (IRCCS), Italy
Copyright © 2019 Multani, Taghdiri, Anor, Varriano, Misquitta, Tang-Wai, Keren, Fox, Lang, Vijverman, Marras and Tartaglia. This is an open-access article distributed under the terms of the Creative Commons Attribution License (CC BY). The use, distribution or reproduction in other forums is permitted, provided the original author(s) and the copyright owner(s) are credited and that the original publication in this journal is cited, in accordance with accepted academic practice. No use, distribution or reproduction is permitted which does not comply with these terms.
*Correspondence: Maria Carmela Tartaglia, Y2FybWVsYS50YXJ0YWdsaWFAdWhuLmNh