It is essential to bear in mind that the native conformation of human proteins is stabilized by intra-molecular disulfide (S–S) bonds between a single or multiple polypeptide chains. The formation of S–S bonds is catalyzed by protein disulfide isomerase (PDI) (1), the activation of which is associated with a number of human diseases, such as myocardial infarction, stroke, and cancer. Unless proper chaperone proteins are available the accidental cleavage of S–S linkages will result in the unfolding and scrambled refolding of the polypeptide chains thus producing non-native species present in many degenerative diseases (2–5). This process is the most common protein post-translational modification that, for example, in a protein with 9 disulfide bridges can theoretically form 34,459,425 different disulfide-bonded isomers, only one with a correct protein function. However, the most damaging consequence of such a modification is the formation of protein aggregates known as “inclusion bodies” that are resistant to the enzymatic degradation (6). This unusual phenomenon is the result of the formation of inter-molecular hydrophobic bonds, which in contrast to peptide links are not susceptible to the catalytic hydrolysis. It is known that the strongest and practically irreversible protein interactions involve hydrophobic bonds, which in native proteins are buried inside their tertiary structure (7).
One of the blood proteins rich in disulfides is fibrinogen (Fbg), the physiologic function of which is to provide hemostatic fibrin plug formed by the action of enzyme thrombin. This insoluble polymer, when formed at the site of vessel wall injury, is eventually removed by the action of fibrinolytic enzyme system, to give space for the growth of a connective tissue and to ensure proper wound healing. To speed up the process of fibrin elimination from the coronary or cerebral circulations, several thrombolytic therapies have been devised with the use of a variety of fibrinolytic agents. It is, however, well recognized in clinical practice that such therapies are effective only when installed 3–5 h after the onset of thrombosis (8). This enigma is now resolved by the discovery of the alternative pathway of blood coagulation induced with iron (9). Thus, in contrast to thrombin-generated fibrin the iron-induced parafibrin is totally resistant to the fibrinolytic degradation. This is due to the fact that parafibrin has different a tertiary structure than fibrin formed with thrombin. We have showed that such a dramatic modification of fibrin structure is due to the unfolding and scrambled refolding of Fbg disulfide-linked subunits leading to the exposure of hydrophobic epitopes in their polypeptide chains (9). The cleavage of disulfide bonds is induced by biologically highly reactive hydroxyl radicals (HO⋅) formed in the reaction between trivalent iron with hydroxyl groups of water according to the following formula:
As the consequence of the hydroxyl radical interaction with Fbg a huge protease resistant polymer is formed that remains in the circulation for a long time, resulting in a state of chronic inflammation due to the attraction of cytotoxic albeit ineffective T cells. The accumulating evidence indicates that there is a correlation between increased blood concentration of unbound iron and the incidence of cancer in humans (10–13), and that its reduction may prevent cancer morbidity and mortality (14). It should be noted that it is only the trivalent iron (Fe3+), and not divalent (Fe2+), which participate in the generation of hydroxyl radicals and subsequent formation of insoluble parafibrin from soluble plasma Fbg. However, when hemoglobin is released from the hemolyzed erythrocytes, the divalent ferrous ions are enzymatically converted to ferric ions. Thus, any pathologic condition in which erythrocyte membranes are damaged, e.g., in infections and/or after exposure to environmental toxins, may contribute to the excessive body storage of trivalent iron. It should be borne in mind that this form of iron accumulates with age due to the fact that there is no mechanism for its physiologic elimination, and may therefore, explain association of cancer with aging.
The unsuccessful attempts at removing parafibrin by the human body defense systems were recently suggested to contribute to Alzheimer’s disease (15) as well as to the cardiovascular disease (16). These diseases and other degenerative disorders have been known to respond well to dietary modifications, particularly to those associated with the so-called Mediterranean diet (17), which is rich in natural amphiphilic substances such as polyphenols and flavones. Relevant to the concept presented in this article is the fact that protein unfolding can be inhibited by natural products present in tea, fruits, berries, and certain grains, the consumption of which is known to lower the incidence of degenerative diseases (18). In addition, there is another important component of human diet, selenium, which is known to prevent various forms of cancer (19–23). Hence, sodium selenite, but not selenate, reacts with free sulfhydryl groups of proteins, thus preventing reductive cleavage of disulfide bonds followed by protein unfolding and abnormal refolding (24, 25).
It is proposed in this paper that the barrier formed around tumor cells composed of proteolytically resistant parafibrin can be removed by a non-enzymatic mechanism based on the interaction of hydrophobic and hydrophilic groups (Figure 1). Numerous natural substances, particularly those of amphiphilic nature such as polyphenols, when ingested with diet in sufficient quantities can prevent and/or reverse cancer formation and metastases (26–33). These findings may explain beneficial effects of the Mediterranean diet known to be associated with lower incidence of cancer and other degenerative diseases (34).
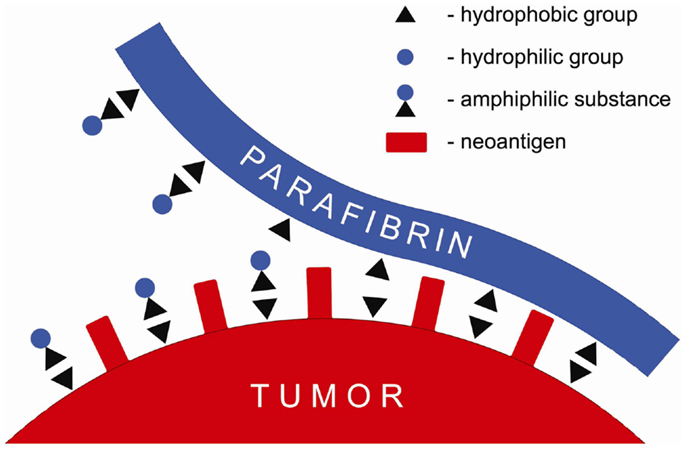
Figure 1. Schematic presentation of a putative mechanism of the anticancer effect of amphiphilic substances that displace parafibrin from surface of the cancer cell membrane.
According to the mechanism shown in Figure 1 amphiphilic substances taken up with food interfere with and/or displace the hydrophobic coat on cancer cells membranes thus exposing neoantigens and making tumors vulnerable to the natural killer cells attack and lysis.
In conclusion, the concept delineated in this article supports the original notion expressed by Basmadijan et al. (35) according to which natural products have a potential to be developed into novel anticancer medicines.
Conflict of Interest Statement
The author declares that the research was conducted in the absence of any commercial or financial relationships that could be construed as a potential conflict of interest.
References
1. Gilbert HF. Protein disulfide isomerase and assisted protein folding. J Biol Chem (1997) 272:29399–402. doi: 10.1074/jbc.272.14.8845
2. Anfinsen CB. Principles that govern the folding of protein chains. Science (1973) 181:223–30. doi:10.1126/science.181.4096.223
3. Cook KM, Hogg PJ. Post-translational control of protein function by disulfide bond cleavage. Antioxid Redox Signal (2013) 18:1987–2015. doi:10.1089/ars.2012.4807
4. Gregersen N, Bross P, Vang S, Christensen JH. Protein misfolding and human disease. Annu Rev Genomics Hum Genet (2006) 7:103–24. doi:10.1146/annurev.genom.7.080505.115737
5. Wang S, Kaufman RJ. The impact of the unfolded protein response on human disease. J Cell Biol (2012) 197:857–67. doi:10.1083/jcb.201110131
6. Fahnert B, Lilie H, Neubauer P. Inclusion bodies: formation and utilisation. Adv Biochem Eng Biotechnol (2004) 89:93–142. doi:10.1007/b93995
8. Lipinski B. Modification of fibrin structure as a possible cause of thrombolytic resistance. J Thromb Thrombolysis (2010) 29:296–8. doi:10.1007/s11239-009-0367-6
9. Lipinski B, Pretorius E. Novel pathway of iron-induced blood coagulation: implications for diabetes mellitus and its complications. Pol Arch Med Wewn (2012) 122:115–22.
10. Nelson RL. Dietary iron and colorectal cancer. Free Radic Biol Med (1992) 12:161. doi:10.1016/0891-5849(92)90010-E
11. Norat T, Lukanova A, Ferrari P, Riboli E. Meat consumption and colorectal cancer risk; dose-response meta-analysis of epidemiological studies. Int J Cancer (2002) 98:241–6. doi:10.1002/ijc.10126
12. Steegmann-Olmedillas JL. The role of iron in tumor cell proliferation. Clin Transl Oncol (2011) 13:71–6. doi:10.1007/s12094-011-0621-1
13. Toyokuni S. Role of iron in carcinogenesis: cancer as a ferrotoxic disease. Cancer Sci (2009) 100:9–16. doi:10.1111/j.1349-7006.2008.01001.x
14. Kalinowski DS, Richardson DR. The evolution of iron chelators for the treatment of iron overload disease and cancer. Pharmacol Rev (2005) 57:547–83. doi:10.1124/pr.57.4.2
15. Lipinski B, Pretorius E. The role of iron-induced fibrin in the pathogenesis of Alzheimer’s disease and the protective role of magnesium. Front Hum Neurosci (2013) 7:735. doi:10.3389/fnhum.2013.00735
16. Lipinski B, Pretorius E. Iron-induced fibrin in cardiovascular disease. Curr Neurovasc Res (2013) 10:269–74. doi:10.2174/15672026113109990016
17. Schwingshacki L, Hoffmann G. Adherence to Mediterranean diet and risk of cancer: a systematic review and meta-analysis of observational studies. Int J Cancer (2014). doi:10.1002/ijc.28824
18. Lipinski B. Hydroxyl radical and its scavengers in health and disease. Oxid Med Cell Longev (2011) 2011:809696. doi:10.1155/2011/8099696
19. Lipinski B. Prostate cancer vaccines, fibrin and selenium: a conceptual review. Open Prost Cancer J (2010) 3:69–73. doi:10.2174/1876822901003010069
20. Freitas M, Alves V, Sarmento-Ribeiro AB, Mota-Pinto MA. Combined effect of sodium selenite and docetaxel on PC3 metastatic prostate cancer cell line. Biochem Biophys Res Commun (2011) 408:713–9. doi:10.1016/j.bbrc.2011.04.109
21. Micke O, Schomburg L, Buentzel J, Kisters K, Muecke R. Selenium in oncology: from chemistry to clinics. Molecules (2009) 14:3975–88. doi:10.3390/molecules14103975
22. Rayman MP. Selenium in cancer prevention: a review of the evidence and mechanism of action. Proc Nutr Soc (2005) 64:527–42. doi:10.1079/PNS2005467
23. Kralova V, Brigulova K, Cervinka M, Rudolf E. Antiproliferative and cytotoxic effects of sodium selenite in human colon cancer. Toxicol In vitro (2009) 23:1497–503. doi:10.1016/j.tiv.2009.07.012
24. Frenkel GG, Falvey D, MacVicar C. Products of the reaction of selenite with intracellular sulfhydryl groups. Biol Trace Elem Res (1991) 30:9–18. doi:10.1007/BF02990338
25. Spallholtz JE. On the nature of selenium toxicity and carcinostatic activity. Free Radic Biol Med (1994) 17:45–64. doi:10.1016/0891-5849(94)90007-8
26. Adhami VM, Mukhtar H. Polyphenols from green tea and pomegranate for prevention of prostate cancer. Free Radic Res (2006) 40:1095–104. doi:10.1080/10715760600796498
27. Fresco P, Borges F, Diniz C, Marques MP. New insight on the anticancer properties of dietary polyphenols. Med Res Rev (2006) 26:747–66. doi:10.1002/med.20060
28. Heger M, van Golen RF, Broekgaarden M, Michel MC. The molecular basis for the pharmacokinetics and pharmacodynamics of curcumin and its metabolites in relation to cancer. Pharmacol Rev (2014) 66:222–307. doi:10.1124/pr.110.004044
29. Lambert JD, Elias RJ. The antioxidant and pro-oxidant activities of green tea polyphenols: a role in cancer prevention. Arch Biochem Biophys (2010) 501:65–72. doi:10.1016/j.abb.2010.06.013
30. Radin NS. Meta-analysis of anticancer drug structures – significance of their polar allylic moieties. Anticancer Agents Med Chem (2007) 7:209–22. doi:10.2174/187152007780058696
31. Schramm L. Going green: the role of the green tea component EGCG in chemoprevention. J Carcinog Mutagen (2013) 4(142):1000142. doi:10.4172/2157-2518.1000142
32. Shukla Y, Singh R. Resveratrol and cellular mechanisms of cancer prevention. Ann N Y Acad Sci (2011) 1251:1–8. doi:10.1111/j.1749-6632.2010.05870.x
33. Takemura H, Sakakibara H, Yamazaki S, Shimoi K. Breast cancer and flavonoids – a role in prevention. Curr Pharm Des (2013) 19:6125–32. doi:10.2174/1381612811319340006
34. Bosetti C, Turati F, Dal Pont A, Ferraroni M, Polesel J, Negri E, et al. The role of Mediterranean diet on the risk of pancreatic cancer. Br J Cancer (2013) 109:1360–6. doi:10.1038/bjc.2013.345
Keywords: cancer, fibrinogen, iron, polyphenols, protein disulfide bonds, selenium
Citation: Lipinski B (2014) Cancer wars: significance of protein unfolding in cancer and its inhibition with natural amphiphilic substances. Front. Oncol. 4:183. doi: 10.3389/fonc.2014.00183
Received: 27 May 2014; Paper pending published: 24 June 2014;
Accepted: 27 June 2014; Published online: 11 July 2014.
Edited by:
Silvia Giordano, University of Turin, ItalyReviewed by:
Xiaoping Zhang, Mount Sinai School of Medicine, USALaurent G. Désaubry, CNRS and University of Strasbourg, France
Copyright: © 2014 Lipinski. This is an open-access article distributed under the terms of the Creative Commons Attribution License (CC BY). The use, distribution or reproduction in other forums is permitted, provided the original author(s) or licensor are credited and that the original publication in this journal is cited, in accordance with accepted academic practice. No use, distribution or reproduction is permitted which does not comply with these terms.
*Correspondence: b.lipinski2006@rcn.com