- 1Department of Physics University of Rome Tor Vergata and INFN-Roma2, Rome, Italy
- 2Institute of Aerospace Medicine, German Aerospace Center (DLR), Cologne, Germany
A reliable radiation risk assessment in space is a mandatory step for the development of countermeasures and long-duration mission planning in human spaceflight. Research in radiobiology provides information about possible risks linked to radiation. In addition, for a meaningful risk evaluation, the radiation exposure has to be assessed to a sufficient level of accuracy. Consequently, both the radiation models predicting the risks and the measurements used to validate such models must have an equivalent precision. Corresponding measurements can be performed both with passive and active devices. The former is easier to handle, cheaper, lighter, and smaller but they measure neither the time dependence of the radiation environment nor some of the details useful for a comprehensive radiation risk assessment. Active detectors provide most of these details and have been extensively used in the International Space Station. To easily access such an amount of data, a single point access is becoming essential. This review presents an ongoing work on the development of a tool that allows obtaining information about all relevant measurements performed with active detectors providing reliable inputs for radiation model validation.
Introduction
Space radiation risks (on humans and instrumentation) are possibly the most severe challenge posed to human exploration of deep space (1). A reliable space radiation risk assessment requires further understanding in radiation biology and also more details about the characteristics of the radiation.
Future mission planning will be based on radiation models which will have to be able to describe to a sufficient level of accuracy the radiation environment the crew will be living in. Such models are under development and consist of a combination of source models, transport models, and computer-aided design (CAD) ability to describe the habitat (either a spacecraft or a space base). Each part as well as the entire final model will have to be properly validated by measurements, and consequently, these measurements will have to provide a similar degree of detail as the model. Passive detectors cannot provide information about, for example, temporal and spatial evolution, or the primary kinetic energy of the ions. Often not even active detectors permit to measure all these parameters.
The construction of future space vessels and space bases is expected to be of similar complexity compared to the International Space Station (ISS). This makes the ISS an important “validation habitat” for the mentioned radiation models. The radiation impinging on the ISS is, however, modulated by the Earth magnetic field, and thus it has features that are not found in deep space. In particular, primary cosmic radiation at low latitude is heavily reduced comprising only high energy ions; charged particles with lower energies are deflected by the magnetic field. At high latitudes, this shielding effect of the magnetic field is much weaker and the radiation field is more similar to the one encountered in deep space.
Furthermore, in a region above Brazil (South Atlantic Anomaly, SAA), the tilt and shift of the magnetic dipole-axis compared to the rotation-axis of Earth result in a close approach of the radiation belt to the Earth’s surface. In the radiation belt, a large amount of low charge ions (mostly protons) of relatively low energy is trapped.
Due to the latitude-dependent magnetic shielding and the presence of the SAA active detectors are advantageous for model validation for deep space; they can be used to select measurements from specific geographical regions and permit to construct a proper dataset for validation, i.e., from high latitudes.
Ideally, measurements and model developments should proceed in a process defining both the most suitable areas in the ISS to perform experiments and the required parameters that are to be measured. Although this approach is usually not realized, a high number of measurements have been and are being performed, which are useful for model validation.
Teams working on radiation models and looking for data for validation often encounter difficulties as literature searches are not always fruitful. Sometime this is due to the difficulty to extract numerical values from articles or it can be due to the delay in the publication procedure. Also, these searches are most often quite time consuming. Therefore, researchers heavily rely on personal communications from teams they have ongoing collaborations with.
It is obvious that a far better approach is having a single access point where all data can be found. In the future, this should lead to a worldwide accessible database. In order to foster the development of such a database and to provide to the scientific community a simple tool for the fast and successful identification of suitable data, a search book is being created in which all relevant information is to be collected.
This review provides a description of this search book that is a soon to be web-published compilation of basic information on active detector measurements of radiation environment for human exploration risk assessment, in particular, when, where (in the ISS or also in or on other relevant satellites/spacecraft), how (what kind of active detector, what kind of measurement), and who (a contributor for each dataset to whom any question about the corresponding data might be addressed).
The goal is to provide this information interactively available on the web. As this work is ongoing the list of active detectors in this article is not complete, and it will be a continuing task to update the data.
Time Frame and Measurements Considered
This review covers the ISS life span and includes also measurements performed during the same period at locations different than ISS, provided that they are of relevance for human space exploration. There are several reasons why the ISS is an ideal starting point:
(i) ISS is the best currently available test bed for radiation measurements aimed at model validation (see above);
(ii) detailed CAD simulations are being developed for ISS, and hopefully, these simulations will soon be able to track mass movements in the ISS; this would permit to compare radiation models and radiation measurements for different, well-defined shielding environments.
(iii) ISS is going to operate probably for another decade, providing the time frame needed for a coordinated approach of measurements and model development and validation (see above).
Concurrent measurements on Earth satellites as well as around Moon or Mars and in interplanetary space are also extremely important as they provide information about the radiation sources impinging on the ISS.
The Summary Tables
Table 1 shows the measurement locations in the past 16 years, as well as the detector performing each measurement. A filled box indicates that measurements were taken during that year. Further details about the time and duration of each measurement as well as detector characteristics and relevant operational parameters will be provided on the web site.
We give a brief account of the latter in Table 2 together with an email address as contact point for each of the considered instruments. For any further information and details, the reader is addressed to the references.
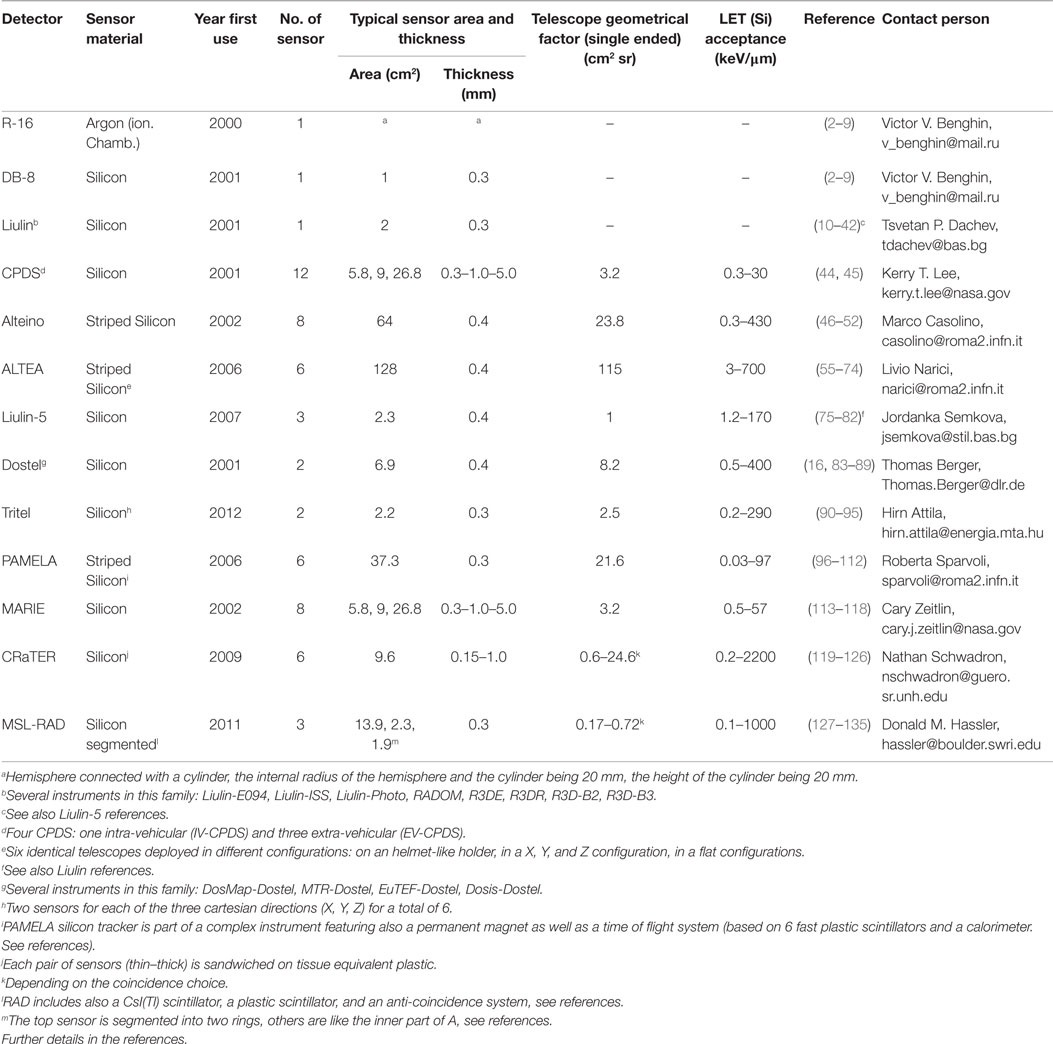
Table 2. Details of the active radiation detectors that have been active on the ISS or that have performed measurements relevant to human exploration.
A Brief Description of the Detectors
The R-16 detector (2–9) is a combination of two Argon filled ionization chamber with two different shielding. It has been also the first active detector in use in the ISS.
The DB-8 (2–9) detector is similar to the Liulin (see below). Four DB-8 units are a part of the ISS radiation monitoring system (RMS). All the DB-8 units are identical and two independent sensors with different shielding operate in each of the DB-8 units. The DB-8 units were located in different locations in the ZVEDA module to provide measurements in different shielding environments.
Liulin (10–42) labels a set of small detectors of very similar dimensions and operational principles but differing in read out, storing, and other characteristics that tailored each detector to the experiments it was built for. Details on these instruments can be found in the references, e.g., Ref. (40). All Liulin-type instruments use silicon detectors and measure the deposited energy and the number of charged particles hitting the device, which can be converted to dose rate and particle flux. The first Liulin was developed in the late 80s (43) for use in the MIR Station. The ISS instruments are Liulin-E094 (April to August 2001), Liulin-ISS (September 2005 to June 2014), Liulin-5 (May 2007 to present), R3DE (February 2008 to September 2009), and R3DR (March 2009 to August 2010), the latter two have been deployed outside the ISS. Several Liulins (R3D-B2, R3D-B3, Liulin-Photo) have also been used in Foton and Bion satellites flying in Low Earth Orbit (LEO) and in the Chandrayaan-1 satellite around the moon (RADOM). The Liulin-5 (75–82) is the first of a new Liulin type: it is a telescope using three silicon detectors providing both a coincidence (telescope) and a non-coincidence read out (for direct dose measurements).
The charged particle directional spectrometer (CPDS) (44, 45) is a detector used by NASA to monitor ISS radiation environment. It is a telescope with a 12 element silicon stack with different thickness (6 thick, 5 mm and 6 thinner, 0.3 and 1 mm) and a Cerenkov detector at the bottom. Thick detectors are for particle identification. The individual sensor cards are identical in design to those used on the MARIE instrument (see below). Three CPDS have been deployed externally (EV-CPDS). EV1-CPDS and EV3-CPDS were aligned with the +X and −X axis in the ISS coordinate system, while EV2-CPSDS with the −Z axis. Another detector (IV-CPDS) was deployed inside the USLab initially pointing to the +X direction and since 8 August 2006 to the +Z direction.
Alteino (46–52), often referred to as SilEye3, is an upgraded SilEye (53), which was successfully deployed in the MIR station. As all the ALTEA systems it originates from investigations of the radiation potentially causing the “Light Flash” anomalous perception to the astronauts [Ref. (54) and references therein]. Alteino features a stack of eight silicon striped sensors (each 80 mm × 80 mm × 0.38 mm) and two plastic scintillators: one in front of and one behind the silicon stack. Sensors are segmented in alternating directions (X and Y). The direction and the height in the stack of eight provide a set of three coordinates for each sensor traversal and for each particle, and permit to track its direction. Alteino is a pure telescope recording radiation only when both scintillators are hit. The detector has the size of a shoebox, and it is able to measure the LET in silicon and the flux and the trajectory of each ion, using the ability to know which of the sensor strip has been hit. Alteino was deployed in the Russian segment of the ISS, in the Russian space program and then in an ESA sponsored experiments under the ALTCRISS project. Alteino data were downloaded periodically via PCMCIA cards. Alteino provided detailed particle spectra in the Russian modules.
ALTEA (55–74) is a system of six identical silicon telescopes. Each telescope is similar to Alteino (also in size) but lacking the scintillators and featuring six striped silicon sensors with twice the area of the sensors used in Alteino (each 160 mm × 80 mm × 0.38 mm). The bi-directional geometrical factor is 230 cm2 sr and the system is auto-triggered by traversing ions. The six telescopes are identical and in the periods of interest have been deployed either on a helmet shaped holder in different locations in the USLab, on a three axis (X, Y, and Z) holder in other locations in the USLab or in a flat configuration for measuring effectiveness of shielding materials in one location in Columbus. ALTEA data are downlinked via real time telemetry. A software package on ground provides flux, dose, dose equivalent, and spectra in real time. ALTEA ISS surveys allowed for the 3D characterization of the radiation environment in the USLab (relevant for model validation). Also of relevance is the study of the iron abundance (ISS – USLab), which is apparently lower than expected.
DosTel (16, 83–89) is a detector family based on two silicon detectors, each with an area of 6.94 cm2 arranged in telescope geometry. With this setup, the DosTel applied for various experiments onboard space stations and shuttle missions can measure energy deposition of radiation hitting a detector (“dose mode”) or coincidental hits in the two detectors (“telescope” or “LET” mode). From 2009 onward, two identical DosTel units have been deployed in Columbus looking in two directions (X and Y). The long duration of the DosTel measurements in Columbus permits to study the variation of the radiation during a long solar modulation period.
TRITEL (90–95) is a set of three small two elements silicon telescopes, mounted in a 3D configuration (X, Y, and Z). Each telescope is made of two sensors, 220 mm × 0.3 mm, and has a geometrical factor of 5.1 cm2 sr. All sensors are identical, fully depleted, passivated implanted planar silicon (PIPS) detectors. TRITEL has been deployed in the ISS since 2012. TRITEL has been deployed in the Columbus modulus in the EPM rack (TRITEL-SURE, close to DosTel) and in the Zvezda modulus (TRITEL-RS).
PAMELA (96–112) was developed from an instrument that flew aboard the balloon missions MASS, TS93, and CAPRICE, with a design optimized for the study of antimatter in the cosmic radiation. For this type of investigation, it is necessary to have information about the particle charge, energy, and type of interaction from several redundant sub-detectors, in order to uniquely identify rare particles from background. It is composed of a Time-of-Flight (ToF) system, a magnetic spectrometer (MS), a sampling electromagnetic calorimeter (SeC), and a neutron detector (ND).
The ToF comprises six layers of fast plastic scintillators arranged in three double planes (S1, S2, and S3), with alternate layers placed orthogonal to each other. ToF information for charged particles is combined with track length information from the MS to determine particle velocities; particle charge (Z) can be determined up to Z = 8.
The MS consists of a permanent magnet and a silicon tracker (six equidistant 300 μm thick silicon detector planes inserted inside the magnetic cavity). Ionization loss measurements are also made in the silicon planes.
The SeC is made of 44 single-sided silicon sensor layers (380 μm thick) interleaved with 22 plates of tungsten absorber. The main task of the calorimeter is to select positrons and antiprotons from like-charged backgrounds, and it is therefore not of interest in our case; however, it also provides a measurement of the energy of the incident electrons independent from the MS, thus allowing a cross-calibration of the two energy determinations.
The ND is placed below the calorimeter with the aim to increase the electromagnetic and hadronic discrimination capability of the Pamela instrument.
PAMELA’s most important scientific results are related to the anomalous positron abundance. Here, however, the highest interest is in the extraordinary spectrometric ability in the low energy regime (below few GeV/n) for small Z (Z < 8) ions.
MARIE (113–118) is a telescope with an eight element silicon stack with Cerenkov detector at the bottom with different thickness (4 thick, 5 mm and 4 thinner, 0.3 and 1 mm). Thick detectors are for particle identification. The individual sensor cards are identical in design to those used on the CPDS instrument (see above). MARIE has been mounted on Odyssey’s equipment deck, pointing opposite to the spacecraft’s velocity vector. Odyssey is in a circular 2-h polar orbit around Mars. Forward field of view (FOV) points into deep space, rear FOV is partially blocked by Mars. MARIE performed the first characterization of the Martian radiation environment aimed at the risk assessment for human exploration.
CRaTER (119–126) is a telescope with three silicon detector pairs sandwiching pieces of tissue-equivalent plastic. Each pair has one thin detector (150 mm) for measuring high-LET particles and one thick detector (1 mm) for low-LET particles. CRaTER is part of LRO in orbit around the Moon. In nominal orbit, one end of telescope points zenith, the other nadir (toward lunar surface) with the rear FOV entirely filled by the lunar disk. LRO was in a circular 2-h polar orbit above the Moon for the prime mission and is now in an elliptical orbit. The overall dimensions are 24 cm × 23 cm × 16 cm. Of important is the long period of observations that permits to investigate the evolution of the deep space radiation environment during the solar cycle.
RAD (127–135) is a three-element silicon stack, a CsI(Tl) scintillator with p-i-n diode readout and a plastic scintillator at the bottom of the stack; an anti-coincidence system surrounds the CsI(Tl) scintillator and the plastic scintillator and enables their use as neutral particle detectors. It is mounted on the top deck of Curiosity rover pointing to the zenith. The rover is controlled so that tilt does not exceed 10°. During cruise phase, RAD was shielded from above by the Descent Vehicle and from below by the heat shield. On Mars, RAD was shielded by Martian atmosphere (~20 g cm−2 CO2). The detector is small (mass 1.56 kg, total volume 240 cm3) and has several coincidence and anti-coincidence capabilities. RAD allowed for the first detailed measurements of the Mars surface radiation environment.
Active Detectors in Space: The Future
With the advances of the field, active detectors may partly replace passive detectors. Power requirements as well as required sensor dimensions are slowly entering the region of acceptability for a widespread use in space, both as area detectors (for which constraints on dimensions and power are not so strong and therefore requirements for detailed measurement of the radiation field can be fulfilled) and as personal detectors. In the latter case, the miniaturization would not allow for a complete characterization of the radiation field. Nevertheless, active personal dosimeters certainly would provide a more comprehensive picture than passive detectors, and they would permit real time monitoring and alarming capabilities [see, for example, Ref. (136)]. It would be desirable that the data of all future active devices flow into a network of databases in order to make it available in quasi real time for all interested teams.
Conclusion
The development of a search book comprising information about radiation measurements relevant for the validation of models for human exploration has been reported. While not yet complete the extent of measurements of active detectors is already impressive and constitutes a valuable tool for anyone developing or validating radiation models for deep space spacecraft or habitats.
Complementing information about other measurements and detectors will be added in the future, and a similar approach for passive detectors should be started. A final output on a web page is foreseen.
Author Contributions
All authors (LN, TB, DM, and GR) have participated to the idea and to the writing of the Search Book and of this first mini review.
Conflict of Interest Statement
The authors declare that the research was conducted in the absence of any commercial or financial relationships that could be construed as a potential conflict of interest.
Acknowledgments
The authors acknowledge the very important help given by all the researchers that collaborated in providing information about the devices they are or have been working with. Specifically, V. V. Benghin, M. Casolino, T. P. Dachev, A. Hirn, K. T. Lee, V. A. Shurshakov, J. V. Semkova, R. Sparvoli, and C. Zeitlin.
References
1. Durante M, Cucinotta FA. Physical basis of radiation protection in space travel. Rev Mod Phys (2011) 83:1245–81. doi: 10.1103/RevModPhys.83.1245
2. Kuznetsov NV, Nymmik RA, Panasyuk MI, Sosnovets EN, Teltsov MV. Detection and prediction of absorbed radiation doses from solar proton fluxes onboard orbital stations. Cosmic Res (2004) 42:203–9. doi:10.1023/B:COSM.0000033295.49916.83
3. Benghin VV, Petrov VM, Kireeva SA, Markov AV, Volkov AN, Aleksandrin AP, et al. Analysis of radiation dose increases caused by solar cosmic ray events observed by the radiation monitoring system on the Russian segment of the International Space Station. Adv Space Res (2005) 36:1749–52. doi:10.1016/j.asr.2005.08.008
4. Petrov VM, Benghin VV, Shurshakov VA, Panasyuk MI, Kutuzov YV, Morozov OV, et al. Absorbed doses in October-November 2003 onboard the Russian segment of the International Space Station according to the data of radiation control system. Cosmic Res (2006) 44:106–10. doi:10.1134/S0010952506020031
5. Benghin VV. On-board predicting algorithm of radiation exposure for the International Space Station radiation monitoring system. J Atmos Sol Terr Phys (2008) 70:675–9. doi:10.1016/j.jastp.2007.08.045
6. Lishnevskii A, Panasyuk M, Benghin V, Petrov VM, Volkov AN, Nechayev OY. Variations of radiation environment onboard the ISS in the year 2008. Cosmic Research (2010) 48:206–10. doi:10.1134/S0010952510030020
7. Lishnevskii A, Panasyuk M, Benghin V, Petrov VM, Volkov AN, Nechaev OY. Variations of radiation environment on the International Space Station in 2005-2009. Cosmic Research (2012) 50:319–23. doi:10.1134/S0010952512040028
8. Lishnevskii A, Panasyuk M, Nechaev O, Benghin VV, Petrov VM, Volkov AN. Results of monitoring variations of absorbed dose rate onboard the International Space Station during the period 2005-2011. Cosmic Research (2012) 50:391–6. doi:10.1134/S0010952512050036
9. Lishnevskii A, Benghin VV. A method for a short-term forecast of the absorbed dose accumulation dynamics on the International Space Station based on radiation monitoring system data. Sol Syst Res (2014) 48:568–73. doi:10.1134/S0038094614070144
10. Dachev T, Tomov B, Matviichuk Y, Dimitrov P, Lemaire J, Gregoire G. Calibration results obtained with Liulin-4 type dosimeters. Adv Space Res (2002) 30:917–25. doi:10.1016/S0273-1177(02)00411-8
11. Uchihori Y, Kitamura H, Fujitaka K, Dachev TP, Tomov BT, Dimitrov PG. Analysis of the calibration results obtained with Liulin-4J spectrometer-dosimeter on protons and heavy ions. Radiat Meas (2002) 35:127–34. doi:10.1016/S1350-4487(01)00286-4
12. Dachev TP, Stassinopoulos EG, Tomov BT, Dimitrov PG, Matviichuk YN, Shurshakov VA. Analysis of the cyclotron facility calibration and aircraft results obtained by LIULIN-3M instrument. Adv Space Res (2003) 32:67–71. doi:10.1016/S0273-1177(03)90372-3
13. Spurný F, Gschwind R, Dačev T. Response of a semiconductor spectrometer to photons-comparison between experimental data and MC-calculations. Nucl Energy Saf (2003) 11:114–7.
14. Atwell W, Reddell B, Dachev T, Tomov B. International Space Station mobile dosimetry unit: a comparison of flight measurements with model calculations. J Aerosp (2004) 113:350–8. doi:10.4271/2004-01-2277
15. Dachev TP, Spurny F, Reitz G, Tomov BT, Dimitrov PG, Matviichuk YN. Simultaneous investigation of galactic cosmic rays on aircrafts and on International Space Station. Adv Space Res (2005) 36:1665–70. doi:10.1016/j.asr.2005.05.073
16. Reitz G, Beaujean R, Benton E, Burmeister S, Dachev T, Deme S, et al. Space radiation measurements on-board ISS – the DOSMAP experiment. Radiat Prot Dosimetry (2005) 116:374–9. doi:10.1093/rpd/nci262
17. Dachev T, Atwell W, Semones E, Tomov B, Reddell B. ISS observations of SAA radiation distribution by Liulin-E094 instrument on ISS. Adv Space Res (2006) 37:1672–7. doi:10.1016/j.asr.2006.01.001
18. Spurny F, Ploc O, Jadrnıckova I, Turek K, Dachev T, Gelev M. Monitoring of onboard aircraft exposure to cosmic radiation: May-December 2005. Adv Space Res (2007) 40:1551–7. doi:10.1016/j.asr.2006.10.006
19. Spurný F, Ploc O, Dachev T. On the neutron contribution to the exposure level onboard space vehicles. Radiat Prot Dosimetry (2007) 126:519–23. doi:10.1093/rpd/ncm104
20. Wilson JW, Nealy JE, Dachev TP, Tomov BT, Cucinotta FA, Badavi FF, et al. Time serial analysis of the induced LEO environment within the ISS 6A. Adv Space Res (2007) 40:1562–70. doi:10.1016/j.asr.2006.12.030
21. Semkova J. Radiation detection and dosimetry for estimating the space weather radiation impact on crewmembers during long space missions. C R Acad Bulg Sci (2007) 60:957–66.
22. Häder DP, Richter P, Schuster M, Dachev T, Tomov B, Georgiev P, et al. R3D-B2 – measurement of ionizing and solar radiation in open space in the BIOPAN 5 facility outside the FOTON M2 satellite. Adv Space Res (2009) 43:1200–11. doi:10.1016/j.asr.2009.01.021
23. Damasso M, Dachev T, Falzetta G, Giardi MT, Rea G, Zanini A. The radiation environment observed by Liulin-photo and R3D-B3 spectrum-dosimeters inside and outside Foton-M3 spacecraft. Radiat Meas (2009) 44:263–72. doi:10.1016/j.radmeas.2009.03.007
24. Dachev TP, Tomov BT, Matviichuk YN, Dimitrov PG, Spurny F. Monitoring lunar radiation environment: RADOM instrument on Chandrayaan-1. Curr Sci (2009) 96:544–6.
25. Dachev TP, Tomov BT, Matviichuk YN, Dimitrov PG, Bankov NG. Relativistic electrons high doses at International Space Station and Foton M2/M3 satellites. Adv Space Res (2009) 44:1433–40. doi:10.1016/j.asr.2009.09.023
26. Dachev TP. Characterization of near Earth radiation environment by Liulin type instruments. Adv Space Res (2009) 44:1441–9. doi:10.1016/j.asr.2009.08.007
27. Slaba TC, Blattnig SR, Badavi FF, Stoffle NN, Rutledge RD, Lee KT, et al. Statistical validation of HZETRN as a function of vertical cutoff rigidity using ISS measurements. Adv Space Res (2011) 47:600–10. doi:10.1016/j.asr.2010.10.021
28. Dachev TP, Tomov BT, Matviichuk YN, Dimitrov PG, Spurny F, Ploc O. Liulin type spectrometry-dosemetri instruments. Radiat Prot Dosimetry (2011) 144:675–9. doi:10.1093/rpd/ncq506
29. Dachev TP, Spurny F, Ploc O. Characterization of radiation environment by Liulin type spectrometers. Radiat Prot Dosimetry (2011) 144:680–3. doi:10.1093/rpd/ncq534
30. Vadawale SV, Goswami JN, Dachev TP, Tomov BN, Girish V. Monitoring of the earth and moon radiation environment with RADOM experiment onboard Chandrayaan-1. Adv Geosci (2011) 25:121–33.
31. Dachev TP, Tomov BT, Matviichuk YN, Dimitrov PG, Vadawale SV, Goswami JN, et al. An overview of RADOM results for earth and moon radiation environment on Chandrayyan-1 satellite. Adv Space Res (2011) 48:779–91. doi:10.1016/j.asr.2011.05.009
32. Dachev TP, Semkova J, Tomov B, Matviichuk Y, Dimitrov P, Koleva R, et al. Space Shuttle drops down the SAA doses on ISS. Adv Space Res (2011) 47:2030–8. doi:10.1016/j.asr.2011.01.034
33. Dachev TP, Tomov BT, Matviichuk YN, Dimitrov PG, Bankov NG, Reitz G. Relativistic electron fluxes and dose rate variations during April-May 2010 geomagnetic disturbances in the R3DR data on ISS. Adv Space Res (2012) 50:282–92. doi:10.1016/j.asr.2012.03.028
34. Schuster M, Dachev T, Richter P, Häder D-P, Lebert M. R3DE, radiation risk radiometer-dosimeter on the International Space Station (ISS) – radiation data recorded during 18 month of EXPOSE-E exposure to open space climate. J Astrobiol (2012) 12:393–402. doi:10.1089/ast.2011.0743
35. Dachev T, Horneck G, Häder D-P, Lebert M, Richter P, Schuster M, et al. Time profile of cosmic radiation exposure during the EXPOSE-E mission: the R3D instrument. J Astrobiol (2012) 12:403–11. doi:10.1089/ast.2011.0759
36. Semkova J, Dachev T, Koleva R, Maltchev S, Bankov N, Benghin V, et al. Radiation environment on the International Space Station during the solar particle events in March 2012. Astrobiol Outreach (2013) 1:102. doi:10.4172/2332-2519.1000102
37. Dachev TP, Tomov BT, Matviichuk YN, Dimitrov PG, Bankov NG, Reitz G. Relativistic electron fluxes and dose rate variations observed on the International Space Station. J Atmos Sol Terr Phys (2013) 99:150–6. doi:10.1016/j.jastp.2012.07.007
38. Dachev T. Analysis of the space radiation doses obtained simultaneously at 2 different locations outside ISS. Adv Space Res (2013) 52:1902–10. doi:10.1016/j.asr.2013.08.011
39. Dachev TP. Profile of the ionizing radiation exposure between the earth surface and free space. J Atmos Sol Terr Phys (2013) 102:148–56. doi:10.1016/j.jastp.2013.05.015
40. Dachev TP, Semkova JV, Tomov BT, Matviichuk YN, Maltchev PGS, Koleva R, et al. Overview of the Liulin type instruments for space radiation measurement and their scientific results. Life Sci Space Res (Amst) (2015) 4:92–114. doi:10.1016/j.lssr.2015.01.005
41. Dachev T, Horneck G, Häder D-P, Schuster M, Lebert M. EXPOSE-R cosmic radiation time profile. J Astrobiol (2015) 14:17–25. doi:10.1017/S1473550414000093
42. Dachev TP, Tomov BT, Matviichuk YN, Dimitrov PG, Bankov NG, Shurshakov VV, et al. “BION-M” No. 1 spacecraft radiation environment as observed by the RD3-B3 radiometer-dosimeter in April-May 2013. J Atmos Sol Terr Phys (2015) 123:82–91. doi:10.1016/j.jastp.2014.12.011
43. Dachev TP, Matviichuk YN, Semkova JV, Koleva RT, Boichev B, Baynov P, et al. Space radiation dosimetry with active detections for the scientific program of the second Bulgarian cosmonaut on board the Mir space station. Adv Space Res (1989) 9:247–51. doi:10.1016/0273-1177(89)90445-6
44. Badhwar GD. Radiation measurements on the International Space Station. Phys Med (2001) 17:287–91.
45. Lee K, Flanders J, Semones E, Shelfer T, Riman F. Simultaneous observation of the radiation environment inside and outside the ISS. Adv Space Res (2007) 40:1558–61. doi:10.1016/j.asr.2007.02.083
46. Bidoli V, Casolino M, De Pascale MP, Furano G, Minori M, Morselli A, et al. The Sileye-3/Alteino experiment for the study of light flashes, radiation environment and astronaut brain activity on board the International Space Station. J Radiat Res (2002) 43:s47–52. doi:10.1269/jrr.43.S47
47. Casolino M, Bidoli V, Furano G, Minori M, Morselli A, Narici L, et al. The Sileye-3/Alteino experiment on board the International Space Station. Nucl Phys B Proc Suppl (2002) 113:71–8.
48. Casolino M, Bidoli V, Minori M, Narici L, De Pascale MP, Picozza P, et al. Relative nuclear abundances inside ISS with Sileye-3/Alteino experiment. Adv Space Res (2006) 37:1686–90. doi:10.1016/j.asr.2006.02.050
49. Casolino M, Bidoli V, Minori M, Narici L, De Pascale MP, Picozza P, et al. Detector response and calibration of the cosmic-ray detector of the Sileye-3/Alteino experiment. Adv Space Res (2006) 37:1691–6. doi:10.1016/j.asr.2005.03.136
50. Scrimaglio R, Rantucci E, Segreto E, Nurzia G, Finetti N, Di Gaetano A, et al. Analysis of Sileye-3/Alteino data with a neural network technique: particle discrimination and energy reconstruction. Adv Space Res (2006) 37:1697–703. doi:10.1016/j.asr.2005.12.004
51. Larsson O, Benghin VV, Casolino M, Chernikch IV, Di Fino L, Fuglesang C, et al. Relative nuclear abundances from C to Fe and integrated flux inside the Russian part of the ISS with the Sileye3/Alteino experiment. J Phys G Nucl Part Phys (2014) 41:015202. doi:10.1088/0954-3899/41/1/015202
52. Larsson O, Benghin VV, Berger T, Casolino M, Di Fino L, Fuglesang C, et al. Measurements of heavy-ion anisotropy and dose rates in the Russian section of the International Space Station with the Sileye3/Alteino detector. J Phys G Nucl Part Phys (2015) 42:025002. doi:10.1088/0954-3899/42/2/025002
53. Bidoli V, Casolino M, De Grandis E, De Pascale MP, Furano G, Morselli A, et al. In-flight performances of SilEye-2 experiment and cosmic ray abundances inside space station MIR. J Phys G Nucl Part Phys (2001) 27:2051–64. doi:10.1088/0954-3899/27/10/307
54. Narici L. Heavy ions light flashes and brain functions: recent observations at accelerators and in spaceflight. New J Phys (2008) 10:075010. doi:10.1088/1367-2630/10/7/075010
55. Casolino M, De Pascale MP, Morselli A, Narici L, Picozza P, Prigiobbe V, et al. Light flash observation in space: experiment ELFO. Nuovo Cimento Soc Ital Fis D (1997) 19:1601–23.
56. Narici L, Bidoli V, Casolino M, De Pascale MP, Furano G, Modena I, et al. The ALTEA facility on the International Space Station. Phys Med (2001) 17s:255–7.
57. Narici L, Bidoli V, Casolino M, De Pascale MP, Furano G, Morselli A, et al. ALTEA: anomalous long term effects in astronauts. A probe on the influence of cosmic radiation and microgravity on the central nervous system during long flights. Adv Space Res (2003) 31:141–6. doi:10.1016/S0273-1177(02)00881-5
58. Narici L, Belli F, Bidoli V, Casolino M, De Pascale MP, Di Fino L, et al. The ALTEA/Alteino projects: studying functional effects of microgravity and cosmic radiation. Adv Space Res (2004) 33:1352–7. doi:10.1016/j.asr.2003.09.052
59. Scrimaglio R, Nurzia G, Rantucci E, Segreto E, Finetti N, Di Gaetano A, et al. Simulation of the ALTEA experiment on the International Space Station with the Geant 3.21 program. Adv Space Res (2006) 37:1770–6. doi:10.1016/j.asr.2004.11.029
60. Di Fino L, Belli F, Bidoli V, Casolino M, Narici L, Picozza P, et al. ALTEA data handling. Adv Space Res (2006) 37:1710–5. doi:10.1016/j.asr.2005.01.105
61. Zaconte V, Belli F, Bidoli V, Casolino M, Di Fino L, Narici L, et al. ALTEA: flight model calibration at GSI. Adv Space Res (2006) 37:1704–9. doi:10.1016/j.asr.2005.02.028
62. Zaconte V, Belli F, Bidoli V, Casolino M, Di Fino L, Narici L, et al. ALTEA: the instrument calibration. Nucl Instrum Methods Phys Res B (2008) 266:2070–8. doi:10.1016/j.nimb.2008.02.072
63. La Tessa C, Di Fino L, Larosa M, Lee K, Mancusi D, Matthia D, et al. Simulation of ALTEA calibration data with PHITS, FLUKA and GEANT4. Nucl Instrum Methods Phys Res B (2009) 267:3549–57. doi:10.1016/j.nimb.2009.06.086
64. La Tessa C, Di Fino L, Larosa M, Narici L, Picozza P, Zaconte V. Estimate of the space station thickness at a USLab site using ALTEA measurements and fragmentation cross sections. Nucl Instrum Methods Phys Res B (2009) 267:3383–7. doi:10.1016/j.nimb.2009.06.107
65. Zaconte V, Casolino M, De Santis C, Di Fino L, La Tessa C, Larosa M, et al. The radiation environment in the ISS-USLab measured by ALTEA: spectra and relative nuclear abundances in the polar, equatorial and SAA regions. Adv Space Res (2010) 46:797–9. doi:10.1016/j.asr.2010.02.032
66. Zaconte V, Casolino M, Di Fino L, La Tessa C, Larosa M, Narici L, et al. High energy radiation influences in the ISS-USLab: ion discrimination and particle abundances. Radiat Meas (2010) 45:168–72. doi:10.1016/j.radmeas.2010.01.020
67. Di Fino L, Casolino M, De Santis C, Larosa M, La Tessa C, Narici L, et al. Heavy ions anisotropy measured by ALTEA in the International Space Station. Radiat Res (2011) 176:397–406. doi:10.1667/RR2179.1
68. Larosa M, Agostini F, Casolino M, De Santis C, Di Fino L, La Tessa C, et al. Ion rates in the International Space Station during the December 2006 solar particle event. J Phys G Nucl Part Phys (2011) 38:095102. doi:10.1088/0954-3899/38/9/095102
69. Narici L, Casolino M, Di Fino L, Larosa M, Larsson O, Picozza P, et al. Iron flux inside the International Space Station is measured to be lower than predicted. Radiat Meas (2012) 47:1030–4. doi:10.1016/j.radmeas.2012.07.006
70. Di Fino L, Zaconte V, Ciccotelli A, Larosa M, Narici L. Fast probabilistic particle identification algorithm using silicon strip detectors. Adv Space Res (2012) 50:408–14. doi:10.1016/j.asr.2012.04.015
71. Di Fino L, Zaconte V, Stangalini M, Sparvoli R, Picozza P, Piazzesi R, et al. Solar particle event detected by ALTEA on board the International Space Station. The March 7th, 2012 X5.4 flare. J Space Weather Space Clim (2014) 4:A19. doi:10.1051/swsc/2014015
72. Berrilli F, Casolino M, Del Moro D, Di Fino L, Larosa M, Narici L, et al. The relativistic solar particle event of May 17th, 2012 observed on board the International Space Station. J Space Weather Space Clim (2014) 4:A16. doi:10.1051/swsc/2014014
73. Di Fino L, Larosa M, Zaconte V, Casolino M, Picozza P, Narici L. Measurements on radiation shielding efficacy of polyethylene and Kevlar in the ISS (Columbus). J Radiat Res (2014) 55:i64–5. doi:10.1093/jrr/rrt198
74. Narici L, Casolino M, Di Fino L, Larosa M, Picozza P, Zaconte V. Radiation survey in the International Space Station. J Space Weather Space Clim (2015). doi:10.1051/swsc/2015037
75. Semkova J, Koleva R, Shurshakov V, Benghin V, Maltchev St, Kanchev N, et al. Status and calibration results of Liulin-5 charged particle telescope designed for radiation measurements in a human phantom onboard the ISS. Adv Space Res (2007) 40:1586–92. doi:10.1016/j.asr.2007.01.008
76. Semkova J, Koleva R, Maltchev S, Benghin V, Shurshakov V, Chernykh I, et al. Preliminary results of Liulin-5 experiment for investigation of the dynamics of radiation doses distribution in a human phantom aboard the International Space Station. C R Acad Bulg Sci (2008) 61:787–94.
77. Semkova J, Koleva R, Maltchev St, Kanchev N, Benghin V, Chernykh I, et al. Radiation measurements inside a human phantom aboard the International Space Station using Liulin-5 charged particle telescope. Adv Space Research (2010) 45:858–65. doi:10.1016/j.asr.2009.08.027
78. Semkova J, Koleva R. Overview on radiation quantities observed by Liulin-5 instrument in a human phantom on the International Space Station during the minimum of the 23rd solar cycle. C R Acad Bulg Sci (2010) 63:1533–42.
79. Semkova J, Koleva R, Maltchev St, Bankov N, Benghin V, Chernykh I, et al. Depth dose measurements with the Liulin-5 experiment inside the spherical phantom of the Matroshka-R project onboard the International Space Station. Adv Space Res (2012) 49:471–8. doi:10.1016/j.asr.2011.10.005
80. Semkova J, Koleva R, Bankov N, Malchev St, Petrov VM, Shurshakov VA, et al. Study of radiation conditions onboard the International Space Station by means of the Liulin-5 dosimeter. Cosmic Res (2013) 51:124–32. doi:10.1134/S0010952512060068
81. Semkova J, Koleva R, Maltchev St, Bankov N, Benghin V, Chernykh I, et al. Radiation characteristics in the spherical tissue-equivalent phantom on the ISS during solar activity minimum according to the data from Liulin-5 experiment. J Atmos Sol Terr Phys (2013) 99:157–63. doi:10.1016/j.jastp.2012.07.006
82. Semkova J, Dachev T, Koleva R, Bankov N, Maltchev S, Benghin V, et al. Observation of radiation environment in the International Space Station in 2012-March 2013 by Liulin-5 particle telescope. J Space Weather Space Clim (2014) 4:A32. doi:10.1051/swsc/2014029
83. Reitz G, Beaujean R, Heilmann C, Kopp J, Leicher M, Strauch K. Results of dosimetric measurements in space missions. Adv Space Res (1998) 22(4):495–500.
84. Beaujean R, Kopp J, Reitz G. Active dosimetry on recent space flights. Radiat Prot Dosimetry (1999) 85:223–6.
85. Beaujean R, Reitz G, Kopp J. Recent European measurements inside Biorack. Mutat Res (1999) 430:183–9.
86. Reitz G, Strauch K, Beaujean R, Kopp J, Luszik-Bhadra M, Heinrich W. Dosimetric mapping inside BIORACK on shuttle missions STS76, STS81 and STS1984. In: Brinckmann E, Varajão C, Perry M, editors. SP1222 Biorack on Spacehab. Noordwijk, Netherlands: ESA (1999). p. 161–9.
87. Beaujean R, Kopp J, Burmeister S, Petersen F, Reitz G. Dosimetry inside Mir station using a silicon detector telescope (DOSTEL). Radiat Meas (2002) 35:433–8. doi:10.1016/S1350-4487(02)00074-4
88. Badhwar GD, Atwell W, Reitz G, Beaujean R, Heinrich W. Radiation measurements on the Mir orbital station. Radiat Meas (2002) 35(5):393–422. doi:10.1016/S1350-4487(02)00072-0
89. Labrenz J, Burmeister S, Berger T, Heber B, Reitz G. Matroshka DOSTEL measurements onboard the International Space Station (ISS). J Space Weather Space Clim (2015). doi:10.1051/swsc/2015039
90. Pázmándi T, Deme S, Láng E. Space dosimetry with the application of a 3D silicon detector telescope: response function and inverse algorithm. Radiat Prot Dosimetry (2006) 120:401–4. doi:10.1093/rpd/nci539
91. Hirn A, Apáthy I, Bodnár L, Csőke A, Deme S, Pázmándi T. Development of a complex instrument measuring dose in the Van Allen belts. Acta Astronaut (2008) 63:878–85. doi:10.1016/j.actaastro.2008.04.001
92. Hirn A. Models of performances of dosimetric telescopes in the anisotropic radiation field in Low Earth Orbit. Acta Astronaut (2010) 66:1368–72. doi:10.1016/j.actaastro.2009.11.016
93. Zábori B, Hirn A, Bencze P. The relationship between plasma effects and cosmic radiation studied with TriTel-LMP measurements during the ESEO mission. Adv Space Res (2011) 48:240–53. doi:10.1016/j.asr.2011.03.020
94. Zábori B, Hirn A. TRITEL 3 dimensional space dosimetric telescope in the European student earth orbiter project of ESA. Acta Astronaut (2012) 71:20–31. doi:10.1016/j.actaastro.2011.08.010
95. Kuwahara T, Hirn A, Apáthy I, Bodnár L, Csőke A, Deme S, et al. TRITEL-JMS: a three-dimensional silicon detector telescope dedicated for operation on board microsatellite RISESAT. Trans Jpn Soc Aeronaut Space Sci (2014) 12:Tr_13–Tr_17. doi:10.2322/tastj.12.Tr_13
96. Adriani O, Barbarino GC, Bazilevskaya GA, Bellotti R, Boezio M, Bogomolov EA, et al. Pamela’s measurements of magnetospheric effects on high-energy solar particles. Astrophys J Lett (2015) 801:L3. doi:10.1088/2041-8205/801/1/L3
97. Adriani O, Barbarino GC, Bazilevskaya GA, Bellotti R, Boezio M, Bogomolov EA, et al. TRAPPED proton fluxes at Low Earth Orbits measured by the Pamela experiment. Astrophys J Lett (2015) 799:L4. doi:10.1088/2041-8205/799/1/L4
98. Adriani O, Barbarino GC, Bazilevskaya GA, Bellotti R, Boezio M, Bogomolov EA, et al. Measurement of boron and carbon fluxes in cosmic rays with the Pamela experiment. Astrophys J (2014) 791:93. doi:10.1088/0004-637X/791/2/93
99. Adriani O, Barbarino GC, Bazilevskaya GA, Bellotti R, Boezio M, Bogomolov EA, et al. The PAMELA mission: heralding a new era in precision cosmic ray physics. Phys Rep (2014) 544:323–70. doi:10.1016/j.physrep.2014.06.003
100. Adriani O, Barbarino GC, Bazilevskaya GA, Bellotti R, Bianco A, Boezio M, et al. Cosmic-ray positron energy spectrum measured by PAMELA. Phys Rev Lett (2013) 111:081102. doi:10.1103/PhysRevLett.111.081102
101. Adriani O, Barbarino GC, Bazilevskaya GA, Bellotti R, Boezio M, Bogomolov EA, et al. Measurement of the isotopic composition of hydrogen and helium nuclei in cosmic rays with the PAMELA experiment. Astrophys J (2013) 770:2. doi:10.1088/0004-637X/770/1/2
102. Adriani O, Barbarino GC, Bazilevskaya GA, Bellotti R, Boezio M, Bogomolov EA, et al. Time dependence of the proton flux measured by Pamela during the 2006 July-2009 December solar minimum. Astrophys J (2013) 765:91. doi:10.1088/0004-637X/765/2/91
103. Adriani O, Barbarino GC, Bazilevskaya GA, Bellotti R, Boezio M, Bogomolov EA, et al. Observations of the 2006 December 13 and 14 solar particle events in the 80MeVn-1-3 GeV n-1 range from space with the PAMELA detector. Astrophys J (2011) 742:102. doi:10.1088/0004-637X/742/2/102
104. Adriani O, Barbarino GC, Bazilevskaya GA, Bellotti R, Boezio M, Bogomolov EA, et al. The discovery of geomagnetically trapped cosmic-ray antiprotons. Astrophys J Lett (2011) 737:L29. doi:10.1088/2041-8205/737/2/L29
105. Adriani O, Barbarino GC, Bazilevskaya GA, Bellotti R, Boezio M, Bogomolov EA, et al. Cosmic-ray electron flux measured by the PAMELA experiment between 1 and 625 GeV. Phys Rev Lett (2011) 106(20):201101. doi:10.1103/PhysRevLett.106.201101
106. Adriani O, Barbarino GC, Bazilevskaya GA, Bellotti R, Boezio M, Bogomolov EA, et al. PAMELA measurements of cosmic-ray proton and helium spectra. Science (2011) 332:69–72. doi:10.1126/science.1199172
107. Adriani O, Barbarino GC, Bazilevskaya GA, Bellotti R, Boezio M, Bogomolov EA, et al. PAMELA results on the cosmic-ray antiproton flux from 60 MeV to 180 GeV in kinetic energy. Phys Rev Lett (2010) 105:121101. doi:10.1103/PhysRevLett.105.121101
108. Adriani O, Barbarino GC, Bazilevskaya GA, Bellotti R, Boezio M, Bogomolov EA, et al. A statistical procedure for the identification of positrons in the PAMELA experiment. Astropart Phys (2010) 34:1–11. doi:10.1016/j.astropartphys.2010.04.007
109. Adriani O, Barbarino GC, Bazilevskaya GA, Bellotti R, Boezio M, Bogomolov EA, et al. An anomalous positron abundance in cosmic rays with energies 1.5-100 GeV. Nature (2009) 458:607–9. doi:10.1038/nature07942
110. Adriani O, Barbarino GC, Bazilevskaya GA, Bellotti R, Boezio M, Bogomolov EA, et al. New measurement of the antiproton-to-proton flux ratio up to 100 GeV in the cosmic radiation. Phys Rev Lett (2009) 102:051101. doi:10.1103/PhysRevLett.102.051101
111. Adriani O, Barbarino G, Bazilevskaya GA, Bellotti R, Boezio M, Bogomolov EA, et al. Measurements of quasi-trapped electron and positron fluxes with PAMELA. J Geophys Res Space Phys (2009) 114:A12218. doi:10.1029/2009JA014660
112. Picozza P, Galper AM, Castellini G, Adriani O, Altamura F, Ambriola M, et al. PAMELA – a payload for antimatter matter exploration and light-nuclei astrophysics. Astropart Phys (2007) 27:296–315. doi:10.1016/j.astropartphys.2006.12.002
113. Badhwar GD. Martian radiation environment experiment (MARIE). Space Sci Rev (2004) 110:131–42. doi:10.1023/B:SPAC.0000021009.68228.a8
114. Zeitlin C, Cleghorn T, Cucinotta F, Saganti P, Andersen V, Lee K, et al. Overview of the Martian radiation environment experiment. Adv Space Res (2004) 33:2204–10. doi:10.1016/S0273-1177(03)00514-3
115. Atwell W, Saganti P, Cucinotta FA, Zeitlin CJ. A space radiation shielding model of the Martian radiation environment experiment (MARIE). Adv Space Res (2004) 33:2219–21. doi:10.1016/S0273-1177(03)00527-1
116. Lee KT, Cleghorn T, Cucinotta F, Pinsky L, Zeitlin C. Heavy ion observations by MARIE in cruise phase and Mars orbit. Adv Space Res (2004) 33:2211–4. doi:10.1016/S0273-1177(03)00519-2
117. Cleghorn TF, Saganti PB, Zeitlin CJ, Cucinotta FA. Solar particle events observed at Mars: dosimetry measurements and model calculations. Adv Space Res (2004) 33:2215–8. doi:10.1016/S0273-1177(03)00518-0
118. Zeitlin C, Boynton WV, Mitrofanov I, Hassler D, Atwell W, Cleghorn TF, et al. Mars Odyssey measurements of galactic cosmic rays and solar particles in Mars orbit, 2002-2008. Space Weather (2010) 8:11. doi:10.1029/2009SW000563
119. Spence HE, Case AW, Golightly MJ, Heine T, Larsen BA, Blake JB, et al. CRaTER: the cosmic ray telescope for the effects of radiation experiment on the lunar reconnaissance orbiter mission. Space Sci Rev (2010) 150:243–84. doi:10.1007/s11214-009-9584-8
120. Schwadron NA, Baker T, Blake B, Case AW, Cooper JF, Golightly M, et al. Lunar radiation environment and space weathering from the cosmic ray telescope for the effects of radiation (CRaTER). J Geophys Res Planets (2012) 117:E00H13. doi:10.1029/2011JE003978
121. Wilson JK, Spence H, Case AW, Blake JB, Golightly MJ, Kasper J, et al. The first cosmic ray albedo proton map of the moon. J Geophys Res Planets (2012) 117:E00H23. doi:10.1029/2011JE003921
122. Case AW, Kasper JC, Spence H, Zeitlin C, Looper MD, Golightly MJ, et al. The deep space galactic cosmic ray lineal energy spectrum at solar minimum. Space Weather (2013) 11:361–8. doi:10.1002/swe.20051
123. Zeitlin C, Case AW, Spence H, Schwadron NA, Golightly M, Wilson JK, et al. Measurements of galactic cosmic ray shielding with the CRaTER instrument. Space Weather (2013) 11:284–96. doi:10.1002/swe.20043
124. Looper MD, Mazur JE, Blake JB, Spence HE, Golightly MJ, Case AW, et al. CRaTER observations and Geant4 simulations. Space Weather (2013) 11:142–52. doi:10.1002/swe.20034
125. Joyce CJ, Schwadron NA, Wilson JK, Spence HE, Kasper J, Golightly M, et al. Validation of PREDICCS using CRaTER/LRO observations during three major solar events in 2012 using CRaTER and the EMMREM model. Space Weather (2013) 11:1–11. doi:10.1002/swe.20059
126. Mazur JE, Zeitlin C, Schwadron N, Looper MD, Townsend LW, Blake JB, et al. Update on radiation dose from galactic and solar protons at the moon using the LRO/CRaTER microdosimeter. Space Weather (2015) 13:363–4. doi:10.1002/2015SW001175
127. Hassler DM, Zeitlin C, Wimmer-Schweingruber RF, Böttcher S, Martin C, Andrews J, et al. The radiation assessment detector (RAD) investigation. Space Sci Rev (2012) 170:503–58. doi:10.1007/s11214-012-9913-1
128. Zeitlin C, Hassler DM, Cucinotta FA, Ehresmann B, Wimmer-Schweingruber RF, Brinza DE, et al. Measurements of energetic particle radiation in transit to Mars on the Mars science laboratory. Science (2013) 340:1080–4. doi:10.1126/science.1235989
129. Hassler DM, Zeitlin C, Wimmer-Schweingruber RF, Ehresmann B, Rafkin S, Eigenbrode JL, et al. Mars’ surface radiation environment measured with the Mars science laboratory’s curiosity rover. Science (2014) 343:1244797. doi:10.1126/science.1244797
130. Köhler J, Ehresmann B, Martina C, Böhm E, Kharytonov A, Kortmann O, et al. Inversion of neutron/gamma spectra from scintillator measurements. Nucl Instrum Methods Phys Res B (2011) 269:2641–8. doi:10.1016/j.nimb.2011.07.021
131. Köhler J, Zeitlin C, Ehresmann B, Wimmer-Schweingruber RF, Hassler D, Reitz G, et al. Measurements of the neutron spectrum on the Martian surface with MSL/RAD. J Geophys Res Planets (2014) 119:594–603. doi:10.1002/2013JE004539
132. Ehresmann B, Zeitlin C, Hassler DM, Wimmer-Schweingruber RF, Böhm E, Böttcher S, et al. Charged particle spectra obtained with the Mars science laboratory radiation assessment detector (MSL/RAD) on the surface of Mars. J Geophys Res Planets (2014) 119:468–79. doi:10.1002/2013JE004547
133. Rafkin SCR, Zeitlin C, Ehresmann B, Köhler J, Guo J, Kahanpää H, et al. Diurnal variations of energetic particle radiation at the surface of Mars as observed by the Mars science laboratory radiation assessment detector. J Geophys Res Planets (2014) 119:1345–58. doi:10.1002/2013JE004525
134. Köhler J, Ehresmann B, Zeitlin C, Wimmer-Schweingruber RF, Hassler DM, Reitz G, et al. Measurements of the neutron spectrum in transit to Mars on the Mars science laboratory. Life Sci Space Res (Amst) (2015) 5:6–12. doi:10.1016/j.lssr.2015.03.001
135. Guo J, Zeitlin C, Wimmer-Schweingruber RF, Hassler DM, Posner A, Heber B, et al. Variations of dose rate observed by MSL/RAD in transit to Mars. Astron Astrophys (2015) 577:A58. doi:10.1051/0004-6361/201525680
Keywords: active radiation detectors, International Space Station, human space exploration, space radiation risk, database
Citation: Narici L, Berger T, Matthiä D and Reitz G (2015) Radiation Measurements Performed with Active Detectors Relevant for Human Space Exploration. Front. Oncol. 5:273. doi: 10.3389/fonc.2015.00273
Received: 30 September 2015; Accepted: 23 November 2015;
Published: 08 December 2015
Edited by:
Marco Durante, GSI, GermanyReviewed by:
Evagelia C. Laiakis, Georgetown University, USAUlrich H. Straube, European Space Agency, Germany
Copyright: © 2015 Narici, Berger, Matthiä and Reitz. This is an open-access article distributed under the terms of the Creative Commons Attribution License (CC BY). The use, distribution or reproduction in other forums is permitted, provided the original author(s) or licensor are credited and that the original publication in this journal is cited, in accordance with accepted academic practice. No use, distribution or reproduction is permitted which does not comply with these terms.
*Correspondence: Livio Narici, bmFyaWNpQHJvbWEyLmluZm4uaXQ=