- 1Department of Radiation Medicine, Georgetown University Hospital, Washington, DC, USA
- 2George Washington University, Washington, DC, USA
- 3University of South Carolina School of Medicine, Columbia, SC, USA
- 4Department of Urology, Georgetown University Hospital, Washington, DC, USA
- 5Department of Radiation Oncology, The Valley Hospital, Ridgewood, NJ, USA
Background: Recent data suggest that intensity-modulated radiation therapy (IMRT) plus brachytherapy boost for unfavorable prostate cancer provides improved biochemical relapse-free survival over IMRT alone. Stereotactic body radiation therapy (SBRT) may be a less invasive alternative to brachytherapy boost. Here, we report the 3-year gastrointestinal (GI) and genitourinary (GU) toxicities of IMRT plus SBRT boost.
Materials and methods: Between March 2008 and September 2012, patients with prostate cancer were treated with robotic SBRT (19.5 Gy in three fractions) followed by fiducial-guided IMRT (45–50.4 Gy) on an institutional protocol. Toxicity was prospectively graded using the common terminology criteria for adverse events version 4.0 (CTCAEv.4) at the start of and at 1- to 6-month intervals after therapy. Rectal telangiectasias were graded using the Vienna Rectoscopy Score (VRS).
Results: At a median follow-up of 4.2 years (2.4–7.5), 108 patients (4 low-, 45 intermediate-, and 59 high-risk) with a median age of 74 years (55–92) were treated with SBRT plus IMRT, with 8% on anticoagulation and an additional 48% on antiplatelet therapy at the start of therapy. The cumulative incidence of late ≥grade 2 GI toxicity was 12%. Of these, 7% were due to late rectal bleeding, with six patients requiring up to two coagulation procedures. One patient with rectal telangiectasias was treated with hyperbaric oxygen (grade 3 toxicity). No rectal fistulas or stenoses were observed. Ten patients had multiple non-confluent telangiectasias (VRS grade 2), and three patients had multiple confluent telangiectasias (VRS grade 3). The cumulative incidence of late grade 3 GU toxicity was 6%. Most late toxicities were due to hematuria requiring bladder fulguration. There were no late ≥grade 4 GU toxicities.
Conclusion: Rates of clinically significant GI and GU toxicities are modest following IMRT plus SBRT boost. Future studies should compare cancer control, quality of life, and toxicity with other treatment modalities for patients with high-risk prostate cancer.
Introduction
Prostate cancer is the most common malignancy in men in the United States, with an estimated 220,800 men diagnosed in 2015 (1). Of these patients, approximately 15% present with high-risk disease (2). Radiotherapy is the mainstay for treatment of such patients, and several randomized prospective trials have demonstrated that dose-escalated radiotherapy results in improved biochemical-free survival (3–5). Further improvements have also been achieved with the advent of image-guided radiation therapy (IGRT) (6) and low dose rate (LDR) brachytherapy boost (7, 8).
Recent clinical data have demonstrated that large radiation fraction sizes likely confer a radiobiologic advantage in the setting of prostate adenocarcinoma (9), thus providing the rationale for high dose rate (HDR) brachytherapy as a boost to external beam radiation therapy (EBRT) for intermediate- and high-risk patients. Several institutional series have reported favorable outcomes, with biochemical control rates of 87–88 and 69% at 5–7 years for intermediate- and high-risk disease, respectively (10–13). These results have subsequently been confirmed in randomized trials (14, 15). Not surprisingly, such cancer control outcomes present with an increased risk of clinically significant long-term genitourinary (GU) toxicities such as urethral stricture (16–18).
In an effort to maximize the benefit of administering high doses per fraction and patient acceptance, we have examined the use of stereotactic body radiation therapy (SBRT) as a prostatic boost to image-guided intensity-modulated radiation therapy (IMRT) for the treatment of patients with unfavorable clinically localized prostate cancer. Previously, we reported early outcomes of this treatment modality, with a 3-year biochemical-free survival rate of 100% for intermediate-risk and 89.8% for high-risk disease (19). Similarly, we reported that such a therapy conferred minimal impact on long-term quality of life (QOL) (19). Several other studies have supported our early results (20–23). Here, we report the 3-year gastrointestinal (GI) and GU toxicity from this therapy.
Materials and Methods
Patient Selection
Patients with histologically confirmed adenocarcinoma of the prostate were included in the study. Exclusion criteria included clinically involved lymph nodes, bone metastases, or prior pelvic radiotherapy. Androgen deprivation therapy (ADT) was considered for all intermediate- and high-risk patients and ultimately was administered at the discretion of the treating physicians. The MedStar Health Research Institute-Georgetown University Oncology Institutional Review Board approved this study. This research study was carried out under a continuing review approved by this Institutional Review Board (IRB#2009-510). Continuing review is in accordance with institutional guidelines and was approved though expedited review by the IRB Chair or designee on 1/8/2016. The informed consent requirement was waived by the Committee that approved the study, and all data used in this study were anonymized.
SBRT Treatment Planning and Delivery
All patients had four or more gold fiducials placed in the prostate prior to treatment planning. To allow for fiducial stabilization, planning images were obtained a minimum of 7 days after fiducial placement. Patients underwent magnetic resonance imaging (MRI) followed shortly thereafter by a thin cut (1.25 mm) CT scan. For the few patients with contraindications to MRI, CT-urethrogram was employed as an alternative imaging approach to identify the location of the prostatic apex (24). Both scans were performed with an empty bladder. Patients were advised to adhere to a low-gas, low-motility diet starting at least 5 days prior to all treatment planning imaging and treatment delivery. They were also instructed to remain nothing by mouth (Non-Per Os) for at least 4 hours prior to imaging as well as SBRT treatment. An enema was administered 1–2 hours prior to imaging and SBRT treatment.
CT and MR images were fused for treatment planning. The clinical target volume (CTV) included the prostate, areas of radiographic extracapsular extension (ECE), and the proximal seminal vesicles to the point of separation. Pelvic lymph nodes were not included in the CTV. The SBRT planning target volume 1 (PTV1) equaled the CTV expanded 3 mm posteriorly and 5 mm in all other dimensions. The prescription dose was 19.5 Gy to the PTV1 delivered in three fractions of 6.5 Gy over 3–5 days. The prescription isodose line was limited to ≥75%, which limited the maximum prostatic urethra dose to 133% of the prescription dose. The rectum, bladder, penile bulb, and membranous urethra were contoured and evaluated with dose–volume histogram (DVH) analysis during treatment planning using Multiplan (Accuray Inc., Sunnyvale, CA, USA) inverse treatment planning. Less than 1 cc of the rectum and less than 10 cc of the bladder were to receive 19.5 Gy. Less than 50% of the membranous urethra was to receive 18 Gy. Further details on dose and volume constraints to the critical structures have been previously described (19, 25).
Intensity-Modulated Radiation Therapy
Patients initiated IMRT treatment the week following SBRT. The more generous planning target volume 2 (PTV2) included a margin of 1.0 cm around the CTV except at the rectal interface where a margin of 0.5 cm was added. Daily doses of 1.8 Gy were delivered to the PTV2 5 days a week to a total dose of 45–50.4 Gy in 25–28 fractions. One-hundred percent of the PTV2 was to receive at least 95% of the prescription dose, and 5% of the volume was to receive no more than 105% of the prescription dose. For the bladder and rectum, the maximum dose constraint limit was 50 Gy, the full-volume dose constraint limit was 30 Gy, and no part of either volume received more than 55.5 Gy. Dose to the femoral heads was limited to 45 Gy.
Linear-Quadratic Transformation of a Sample Combined Physical IMRT Plus SBRT Boost DVH to a Radiobiologically Equivalent DVH
A radiobiologically equivalent dose DVH was generated by adding doses in 2 Gy equivalents for IMRT and SBRT plans from a sample patient (26). Cumulative DVHs were extracted from the treatment planning software and converted to radiobiologically equivalent DVHs using MIM software (MIMvista Corporation, Cleveland, OH, USA). An α/β ratio of 1.5 was utilized to transform target volume doses (CTV and PTV), and an α/β ratio of 3 was used to transform doses for all other organs at risk. Combination of radiobiologically equivalent DVH for an example patient is shown in Figure 1.
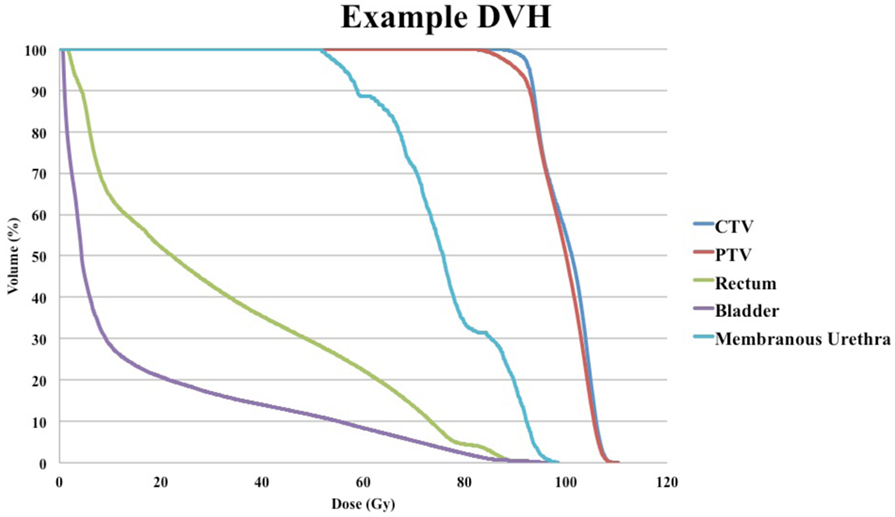
Figure 1. Example of radiobiologically equivalent dose–volume histogram (DVH) of a patient with late grade 2 rectal bleeding treated with two argon plasma coagulations and no genitourinary toxicity.
Follow-up and Toxicity Assessment
Patients were assessed at the start of and at 1 month after therapy, every 3 months for the first year, and every 6 months thereafter. The utilization of alpha-antagonists, oral corticosteroids, anticoagulation or antiplatelet therapy, and anti-diarrheal therapy was documented at each visit. GI and GU toxicities were prospectively documented at follow-up visits using the National Cancer Institute Common Terminology Criteria (CTC) version 4.0 as previously described (27). Specifically, acute toxicity was defined as occurring up to the first 6 months after therapy, and late toxicity was defined as occurring at 6 months or thereafter.
Grade 1 rectal bleeding was defined as transient and not requiring medications for symptomatic management. Grade 2 rectal bleeding represented bleeding which required a new medication (i.e., steroid suppository) or up to two argon plasma coagulations (APCs). More than two APC procedures, a blood transfusion, or use of hyperbaric oxygen (HBO) was defined as grade 3 rectal bleeding. Grade 1 diarrhea was defined as transient diarrhea not requiring medical management. Grade 2 diarrhea was defined as increased stool frequency requiring management with anti-diarrheal medication. The development of rectal strictures or fistulas was defined as grade 4 GI toxicity. Furthermore, because all patients were treated on an institutional protocol, all rectal bleeding events were assessed by endoscopy. Radiation-induced rectal telangiectasias were graded using the Vienna Rectoscopy Score (VRS), with grade 1 defined as a single telangiectasia, grade 2 as multiple non-confluent telangiectasias, and grade 3 as multiple confluent telangiectasias (28).
For GU toxicities, a transient toxicity requiring no new medications for symptomatic management was considered grade 1. The use of a new medication or an increase in the dosage of an already-used medication for symptomatic management was considered grade 2. Grade 3 hematuria, urethral stricture, and urinary retention were defined as requiring an outpatient procedure such as fulguration, urethral dilation, or transurethral resection of the prostate (TURP), respectively. Any toxicity requiring initiation of more invasive therapy was defined as grade 4.
Statistical Analysis
Logistic regression analysis was performed to identify patient characteristics associated with an increased risk of late ≥grade 2 GI toxicity, late ≥grade 2 rectal bleeding, and late grade 3 GU toxicity. Statistical analysis was performed using MedCalc software (Ostend, Belgium).
Results
From March 2008 to September 2012, 108 prostate cancer patients were treated on an institutional IMRT plus SBRT boost protocol. The median follow-up was 4.2 years (range 2.4–7.5). Patient characteristics are shown in Table 1. The median age was 74 years (range 55–91). Similar numbers of Caucasians (47%) and African-Americans (42%) were treated. Patients were generally healthy, with a Charlson comorbidity index of 0–1 in 75%. Eight percent were on anticoagulation therapy, and an additional 48% were on antiplatelet therapy at the start of radiation therapy. The median pre-treatment prostate-specific antigen was 9.1 ng/ml (range 0.86–39.8 ng/ml). By D’Amico classification, 4% were diagnosed with low-, 42% with intermediate-, and 55% with high-risk disease. Seventy-eight percent of patients were treated with an IMRT dose of 45 Gy in 25 fractions. Sixty-three percent received ADT for a median of 6 months (range 3–36 months).
The prevalence of GI and GU toxicities following treatment are shown in Tables 2 and 3. The majority of toxicities were observed at one specific follow-up appointment and resolved on subsequent follow-ups. The most common acute grade 2 GI toxicity was diarrhea, with a peak in 7% of patients at 1 month (Table 2). There were no acute ≥grade 2 rectal bleeding events. The cumulative rate of late ≥grade 2 GI toxicity was 12% (Figure 2), 7% of which was due to rectal bleeding and 5% due to diarrhea. Rectal bleeding occurred most commonly at 12 months following radiation therapy. Of the seven patients who developed ≥grade 2 bleeding, one was on both anticoagulation and antiplatelet therapy, three on antiplatelet therapy only, and three on neither therapy. Six of these patients were assigned as grade 2 bleeding for requiring one or two coagulation procedures, and one was assigned as grade 3 for undergoing HBO. Further details for patients who developed rectal bleeding are shown in Table 4. Of note, logistic regression analysis identified no patient characteristics associated with an increased risk of late ≥grade 2 GI toxicity or late ≥grade 2 rectal bleeding, including use of anticoagulation or antiplatelet therapy (data not shown).
A total of 42 patients underwent a colonoscopy during the follow-up period either to assess the etiology of rectal bleeding or for routine cancer screening. Radiation-induced telangiectasias were noted in 16 cases and were graded as VRS grade 1 in 3 patients, grade 2 in 10 patients, and grade 3 in 3 patients. One patient was noted to have an incidental grade 1 ulcer, which spontaneously resolved on subsequent colonoscopy. Importantly, no rectal strictures or fistulas (grade 4 toxicity) were noted.
The most common acute grade 2 GU toxicity was urinary retention relieved by medical management, peaking at 1 month (Table 3). One patient with acute urinary retention underwent a TURP and was classified as grade 3. There were no ≥grade 2 hematuria events acutely. The cumulative rate of late ≥grade 2 GU toxicity was 40%. The majority of these toxicities were due to obstructive or irritative symptoms requiring medical management with alpha-antagonists and/or anti-muscarinics. In fact, the cumulative rate of late ≥grade 3 GU toxicity was much lower at 6% (Figure 3), 4% due to hematuria and 2% due to retention. The most common areas of radiation cystitis noted on cystoscopy were the bladder neck, trigone, or lateral walls. Of the four patients who experienced bleeding, two were on antiplatelet therapy and none were on anticoagulation therapy. One of these patients with recurrent bleeding secondary to vigorous physical activity also elected to proceed with HBO. Details for patients with hematuria are provided in Table 5. Of the two patients who underwent a TURP for late urinary retention, one had a long history of benign prostatic hypertrophy and prostatitis with two prior TURP procedures. This patient also elected to undergo HBO. No patient developed a urethral stricture or any grade 4 or 5 GU toxicity. Logistic regression also identified no patient characteristics associated with an increased risk of late grade 3 GU toxicity.
Discussion
This study aimed to assess the safety of performing IMRT with SBRT boost for unfavorable clinically localized prostate cancer. SBRT boost was chosen for this study due to the potential radiobiologic benefits of hypofractionation (29) as well as the ease of the treatment modality, especially for the elderly prostate cancer patient population.
Intensity-modulated radiation therapy plus SBRT boost was generally well tolerated with minimal acute toxicity (Tables 2 and 3). Cumulative late ≥grade 2 and ≥grade 3 GU toxicities were observed in 40 and 6% of patients, respectively. It should be noted that the seemingly high rate of grade 2 GU toxicity was due to use of alpha-antagonists or corticosteroids for transient irritative or obstructive symptoms. In fact, the prevalence of any grade 2 GU toxicity was 10–16% after the 3-month time period (Table 3). It is encouraging that only 4% of patients developed hematuria requiring fulguration, and only 2% developed retention requiring a TURP. In comparison, published brachytherapy boost studies have reported late GU toxicity rates of 8–31% (≥grade 2) and 3–18% (≥grade 3) (Table 6) (14–16, 30). Importantly, the ASCENDE-RT trial reported a cumulative late ≥grade 3 GU toxicity rate of 18% at 6 years for patients undergoing a LDR boost, most commonly due to urethral strictures, urinary retention, or incontinence (30). Our 6% cumulative rate of clinically significant late GU toxicity is lower than that reported in this trial, though longer follow-up will be necessary to confirm our results. Other institutions using the SBRT boost technique have reported a 0–2.3% rate of late ≥grade 3 GU toxicities, which are comparable to our results reported here (20–23).
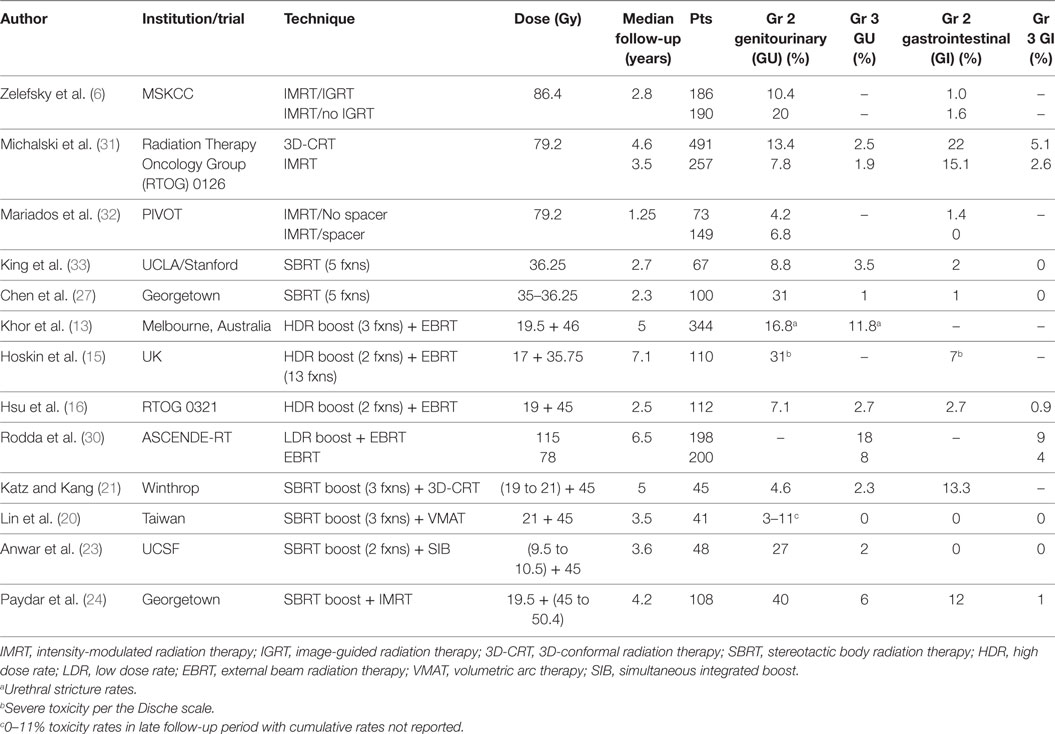
Table 6. Summary of late ≥grade 2 or 3 toxicities reported for various techniques, including IMRT + SBRT boost.
Our study reported overall modest rates of GI toxicity, with a 12% cumulative incidence of late ≥grade 2 GI toxicity, 7% late ≥grade 2 rectal bleeding, and 1% late grade 3 bleeding. In comparison, we have previously reported a 1.5% rate of late ≥grade 2 rectal bleeding with SBRT alone (34). Other studies have reported a somewhat lower 1–3% rate of late ≥grade 2 rectal bleeding with brachytherapy boost (16, 30). Moreover, 16 (15%) of our patients were noted to have telangiectasias, 3 of which were multiple confluent telangiectasias (VRS grade 3). No VRS grade 3 telangiectasias were previously noted with SBRT alone (34).
Despite overall higher rates of GI and GU toxicity compared to SBRT alone, a sample DVH of the combined SBRT and IMRT plans for one patient (Figure 1) demonstrates that the bladder volume receiving 55 and 70 Gy and the rectal volumes receiving 50 and 70 Gy in 2 Gy equivalents are well below the Radiation Therapy Oncology Group recommendations (35). This suggests that the wide IMRT margins and the resulting near-maximal dose at the bladder neck and anterior rectal wall likely contribute to bleeding events, and future dosimetric studies will need to define the appropriate dose-constraints for patients treated with this approach.
Previously, we reported that IMRT with SBRT boost resulted in minimal impact on long-term bowel QOL (19), a finding which is seemingly discordant with late toxicity results shown here. A similar phenomenon has been seen following IMRT monotherapy (31, 36). Several explanations are possible. For example, the most common toxicities—rectal bleeding, hematuria, and urinary obstruction—were transient and resolved by the following time point (37). Also, bleeding likely renders a less bothersome impact on QOL than frequency and urgency (38). Lastly, effects on QOL are reported as temporal changes in mean scores derived from the expanded prostate cancer index composite-26 questionnaire while the CTCAE toxicity scoring system focuses on individual uncommon events (39).
Analysis of such uncommon events is still necessary, since telangiectasias or ulcers may be a precursor lesion for a fistula. While a small percentage of telangiectasias progress to fistulas and often do so after multiple invasive procedures (40, 41), such a late toxicity can nonetheless be devastating for a patient. Thus, it is encouraging that only three of our patients required two APC procedures, with no reportable fistulas to date. Though fistulas most often develop within the first 3 years (40), longer follow-up is still necessary to detect a potential late occurrence.
What is the minimum follow-up time to assess late toxicity after prostate SBRT such that clinically meaningful events are captured fully without undue delay in reporting these important outcomes? For the majority of patients, rectal bleeding occurred at the 1- to 1.5-year time point and resolved after one to two APC procedures, suggesting that a 3-year median follow-up likely captures most rectal bleeding events. However, hematuria occurred starting at 18 months post-treatment and continued to present as late as 36 months, emphasizing the necessity of long-term follow-up for such patients. While no fistulas or strictures of the urethra have been observed, longer follow-up may be necessary to reveal such toxicity as well.
Our encouraging rates of GI and GU toxicity are consistent with results from other institutions using this modality (20–23), though minor differences in technique do exist. For instance, the three largest published series included pelvic lymph nodes in the external beam radiotherapy portion (20–23) and utilized a 3D-conformal (21, 22), volumetric arc therapy (20), or simultaneous integrated boost (23) technique for dose delivery. It should be emphasized that no overall survival benefit has been demonstrated thus far for the treatment of pelvic nodes in high-risk disease (42, 43), and inclusion of pelvic nodes remains a matter of controversy. Furthermore, different dose-fractionation schemas as well as PTV margins were used for the SBRT portion in each study. For instance, Anwar et al. utilized PTV margins of 0 mm posteriorly and 2 mm elsewhere (23). Such smaller margins may have contributed to the lower rates of overall toxicity, with only one late grade 3 GU and no grade 3 GI toxicities reported.
These low toxicity rates question the use of larger PTV margins or IMRT at all. Even with dose-escalated external beam radiotherapy for clinically localized prostate cancer, most failures occur locally within the prostate or adjacent seminal vesicles (44). However, surgical pathology studies report a median ECE of 0.5–2.4 mm and a 4–5 mm margin necessary to cover ≥90% of the ECE (45–48). Our treatment planning studies have furthermore demonstrated potential under-dosing of the posterior prostate without adequate PTV margin (49). Thus, the appropriate planning technique remains an ongoing debate, and long-term outcomes will identify the optimal combinatorial approach as well as target volume.
One approach to maintaining adequate posterior margins while reducing the risk of rectal bleeding is to place a tissue equivalent spacer in the perirectal space prior to treatment. This approach can increase percent target coverage of the PTV while simultaneously reducing the rectal volume receiving near-maximal dose (50). In fact, a recent prospective trial demonstrated a statistically significant reduction in late rectal toxicity from 7 to 2% with the use of rectal spacers in the setting of IMRT (32). An improvement in long-term bowel and 6-month urinary QOL decline was also shown with the use of spacers (32). At our institution, we have recently initiated rectal spacer use for high-risk patients receiving IMRT with SBRT boost for a potential reduction in late rectal bleeding. If rectal spacers effectively prevent rectal bleeding in all patients, 14 patients would require spacer placement to prevent one cauterization event for bleeding, assuming a 7% bleeding rate. While the impact on QOL would undoubtedly be positive, the cost-effectiveness of this therapy remains a matter of debate (51).
Conclusion
Fiducial-guided IMRT with SBRT boost is a promising new treatment option for men with unfavorable prostate cancer. Early results suggest encouraging biochemical response, minimal impact on long-term QOL, and low toxicity. These data provide a basis for the design of a phase III clinical trial.
Author Contributions
IP is the lead author, who participated in data collection, data analysis, manuscript drafting, table/figure creation, and manuscript revision. AP participated in data collection, manuscript drafting, and table/figure creation. RC and JK participated in data collection. TY aided in clinical data collection. EB is a dosimetrist who developed the majority of patients’ IMRT treatment plans and contributed to the dosimetric data analysis and interpretation. SL is the dosimetrist who developed the majority of patients’ SBRT treatment plans and contributed to the dosimetric data analysis and interpretation. AS and KH aided in patient enrollment and collection of clinical data. SS is a senior author who collected the dosimetric data, participated in its analysis, and helped draft the manuscript. AD, JL, and TK are senior authors who aided in drafting the manuscript. SC was the principal investigator who initially developed the concept of the study and the design, aided in data collection, and drafted and revised the manuscript. All the authors read and approved the final manuscript.
Conflict of Interest Statement
SC serves as a clinical consultant to Accuray Inc. The Department of Radiation Medicine at Georgetown University Hospital receives a grant from Accuray to support a research coordinator. The other authors declare that they have no competing interests.
Abbreviations
3D-CRT, 3D-conformal radiation therapy; ADT, androgen deprivation therapy; APC, argon plasma coagulation; CCI, Charlson comorbidity index; CTC, common terminology criteria; CTCAEv.4, common terminology criteria for adverse events version 4.0; CTV, clinical target volume; DVH, dose–volume histogram; ECE, extracapsular extension; EBRT, external beam radiation therapy; EPIC-26, expanded prostate cancer index composite-26; GI, gastrointestinal; GU, genitourinary; HBO, hyperbaric oxygen; HDR, high dose rate; IGRT, image-guided radiation therapy; IMRT, intensity-modulated radiation therapy; LDR, low dose rate; MRI, magnetic resonance imaging; NCI, National Cancer Institute; NPO, Non-Per Os; OAR, organs at risk; PSA, prostate-specific antigen; PTV, planning target volume; QOL, quality of life; RTOG, Radiation Therapy Oncology Group; SBRT, stereotactic body radiation therapy; SIB, simultaneous integrated boost; TURP, transurethral resection of the prostate; VMAT, volumetric arc therapy; VRS, Vienna Rectoscopy Score.
References
1. SEER. Cancer Statistics Factsheets: Prostate Cancer. Bethesda, MD: National Cancer Institute (2016). Available from: http://seer.cancer.gov/statfacts/html/prost.html
2. Cooperberg MR, Lubeck DP, Mehta SS, Carroll PR; CaPSURE. Time trends in clinical risk stratification for prostate cancer: implications for outcomes (data from CaPSURE). J Urol (2003) 170(6 Pt 2):S21–5. doi: 10.1097/01.ju.0000095025.03331.c6
3. Zietman AL, DeSilvio ML, Slater JD, Rossi CJ Jr, Miller DW, Adams JA, et al. Comparison of conventional-dose vs high-dose conformal radiation therapy in clinically localized adenocarcinoma of the prostate: a randomized controlled trial. JAMA (2005) 294(10):1233–9. doi:10.1001/jama.294.10.1233
4. Kuban DA, Tucker SL, Dong L, Starkschall G, Huang EH, Cheung MR, et al. Long-term results of the M. D. Anderson randomized dose-escalation trial for prostate cancer. Int J Radiat Oncol Biol Phys (2008) 70(1):67–74. doi:10.1016/j.ijrobp.2007.06.054
5. Peeters ST, Heemsbergen WD, Koper PC, van Putten WL, Slot A, Dielwart MF, et al. Dose response in radiotherapy for localized prostate cancer: results of the Dutch multicenter randomized phase III trial comparing 68 Gy of radiotherapy with 78 Gy. J Clin Oncol (2006) 24(13):1990–6. doi:10.1200/JCO.2005.05.2530
6. Zelefsky MJ, Kollmeier M, Cox B, Fidaleo A, Sperling D, Pei X, et al. Improved clinical outcomes with high-dose image guided radiotherapy compared with non-IGRT for the treatment of clinically localized prostate cancer. Int J Radiat Oncol Biol Phys (2012) 84(1):125–9. doi:10.1016/j.ijrobp.2011.11.047
7. Shilkrut M, Merrick GS, McLaughlin PW, Stenmark MH, Abu-Isa E, Vance SM, et al. The addition of low-dose-rate brachytherapy and androgen-deprivation therapy decreases biochemical failure and prostate cancer death compared with dose-escalated external-beam radiation therapy for high-risk prostate cancer. Cancer (2013) 119(3):681–90. doi:10.1002/cncr.27784
8. Morris WJ, Tyldesley S, Pai HH, Halperin R, McKenzie MR, Duncan G, et al. ASCENDE-RT*: a multicenter, randomized trial of dose-escalated external beam radiation therapy (EBRT-B) versus low-dose-rate brachytherapy (LDR-B) for men with unfavorable-risk localized prostate cancer. J Clin Oncol (2015) 33(Suppl 7):abstr3.
9. Phan TP, Syed AM, Puthawala A, Sharma A, Khan F. High dose rate brachytherapy as a boost for the treatment of localized prostate cancer. J Urol (2007) 177:123–127; discussion 127. doi:10.1016/j.juro.2006.08.109
10. Demanes DJ, Rodriguez RR, Schour L, Brandt D, Altieri G. High-dose-rate intensity-modulated brachytherapy with external beam radiotherapy for prostate cancer: California endocurietherapy’s 10-year results. Int J Radiat Oncol Biol Phys (2005) 61:1306–16. doi:10.1016/j.ijrobp.2004.08.014
11. Galalae RM, Martinez A, Mate T, Mitchell C, Edmundson G, Nuernberg N, et al. Long-term outcome by risk factors using conformal high-dose-rate brachytherapy (HDR-BT) boost with or without neoadjuvant androgen suppression for localized prostate cancer. Int J Radiat Oncol Biol Phys (2004) 58:1048–55. doi:10.1016/j.ijrobp.2003.08.003
12. Martinez AA, Gustafson G, Gonzalez J, Armour E, Mitchell C, Edmundson G, et al. Dose escalation using conformal high-dose-rate brachytherapy improves outcome in unfavorable prostate cancer. Int J Radiat Oncol Biol Phys (2002) 53:316–27. doi:10.1016/S0360-3016(02)02733-5
13. Khor R, Duchesne G, Tai KH, Foroudi F, Chander S, Van Dyk S, et al. Direct 2-arm comparison shows benefit of high-dose-rate brachytherapy boost vs external beam radiation therapy alone for prostate cancer. Int J Radiat Oncol Biol Phys (2013) 85(3):679–85. doi:10.1016/j.ijrobp.2012.07.006
14. Sathya JR, Davis IR, Julian JA, Guo Q, Daya D, Dayes IS, et al. Randomized trial comparing iridium implant plus external-beam radiation therapy with external-beam radiation therapy alone in node-negative locally advanced cancer of the prostate. J Clin Oncol (2005) 23(6):1192–9. doi:10.1200/JCO.2005.06.154
15. Hoskin PJ, Rojas AM, Bownes PJ, Lowe GJ, Ostler PJ, Bryant L. Randomised trial of external beam radiotherapy alone or combined with high-dose-rate brachytherapy boost for localised prostate cancer. Radiother Oncol (2012) 103(2):217–22. doi:10.1016/j.radonc.2012.01.007
16. Hsu IC, Bae K, Shinohara K, Pouliot J, Purdy J, Ibbott G, et al. Phase II trial of combined high-dose-rate brachytherapy and external beam radiotherapy for adenocarcinoma of the prostate: preliminary results of RTOG 0321. Int J Radiat Oncol Biol Phys (2010) 78(3):751–8. doi:10.1016/j.ijrobp.2009.08.048
17. Sullivan L, Williams SG, Tai KH, Foroudi F, Cleeve L, Duchesne GM. Urethral stricture following high dose rate brachytherapy for prostate cancer. Radiother Oncol (2009) 91(2):232–6. doi:10.1016/j.radonc.2008.11.013
18. Tward JD, Jarosek S, Chu H, Thorpe C, Shrieve DC, Elliott S. Time course and accumulated risk of severe urinary adverse events after high- versus low-dose-rate prostate brachytherapy with or without external beam radiation therapy. Int J Radiat Oncol Biol Phys (2016) 95(5):1443–53. doi:10.1016/j.ijrobp.2016.03.047
19. Mercado C, Kress MA, Cyr RA, Chen LN, Yung TM, Lei S, et al. Intensity modulated radiation therapy with stereotactic body radiation therapy for unfavorable prostate cancer: the Georgetown University experience. Front Oncol (2016) 6:114. doi:10.3389/fonc.2016.00114
20. Lin YW, Lin LC, Lin KL. The early result of whole pelvic radiotherapy and stereotactic body radiotherapy boost for high-risk localized prostate cancer. Front Oncol (2014) 4:278. doi:10.3389/fonc.2014.00278
21. Katz AJ, Kang J. Stereotactic body radiotherapy with or without external beam radiation as treatment for organ confined high-risk prostate carcinoma: a six year study. Radiat Oncol (2014) 9:1. doi:10.1186/1748-717X-9-1
22. Katz AJ, Santoro M, Ashley R, Diblasio F, Witten M. Stereotactic body radiotherapy as boost for organ-confined prostate cancer. Technol Cancer Res Treat (2010) 9(6):575–82. doi:10.1177/153303461000900605
23. Anwar M, Weinberg V, Seymour Z, Hsu IJ, Roach M III, Gottschalk AR. Outcomes of hypofractionated stereotactic body radiotherapy boost for intermediate and high-risk prostate cancer. Radiat Oncol (2016) 11(1):8. doi:10.1186/s13014-016-0585-y
24. Paydar I, Kim BS, Cyr RA, Rashid H, Anjum A, Yung TM, et al. Urethrogram-directed stereotactic body radiation therapy for clinically localized prostate cancer in patients with contraindications to magnetic resonance imaging. Front Oncol (2015) 5:194. doi:10.3389/fonc.2015.00194
25. Oermann EK, Slack RS, Hanscom HN, Lei S, Suy S, Park HU, et al. A pilot study of intensity modulated radiation therapy with hypofractionated stereotactic body radiation therapy (SBRT) boost in the treatment of intermediate- to high-risk prostate cancer. Technol Cancer Res Treat (2010) 9:453–62. doi:10.1177/153303461000900503
26. Wheldon TE, Deehan C, Wheldon EG, Barrett A. The linear-quadratic transformation of dose-volume histograms in fractionated radiotherapy. Radiother Oncol (1998) 46(3):285–95. doi:10.1016/S0167-8140(97)00162-X
27. Chen LN, Suy S, Uhm S, Oermann EK, Ju AW, Chen V, et al. Stereotactic body radiation therapy (SBRT) for clinically localized prostate cancer: the Georgetown University experience. Radiat Oncol (2013) 8:58. doi:10.1186/1748-717X-8-58
28. Wachter S, Gerstner N, Goldner G, Potzi R, Wambersie A, Potter R. Endoscopic scoring of late rectal mucosal damage after conformal radiotherapy for prostatic carcinoma. Radiother Oncol (2000) 54:11–9. doi:10.1016/S0167-8140(99)00173-5
29. Fowler JF. The radiobiology of prostate cancer including new aspects of fractionated radiotherapy. Acta Oncol (2005) 44:265–76. doi:10.1080/02841860410002824
30. Rodda S, Tyldesley S, Morris W. GU and GI toxicity in ASCENDE-RT*: a multicentre randomized trial of dose-escalated radiation for prostate cancer. Radiother Oncol (2015) 115(Suppl 1):S22–3. doi:10.1016/S0167-8140(15)40047-7
31. Michalski JM, Yan Y, Watkins-Bruner D, Bosch WR, Winter K, Galvin JM, et al. Preliminary toxicity analysis of 3-dimensional conformal radiation therapy versus intensity modulated radiation therapy on the high-dose arm of the Radiation Therapy Oncology Group 0126 prostate cancer trial. Int J Radiat Oncol Biol Phys (2013) 87(5):932–8. doi:10.1016/j.ijrobp.2013.07.041
32. Mariados N, Sylvester J, Shah D, Karsh L, Hudes R, Beyer D, et al. Hydrogel spacer prospective multicenter randomized controlled pivotal trial: dosimetric and clinical effects of perirectal spacer application in men undergoing prostate image guided intensity modulated radiation therapy. Int J Radiat Oncol Biol Phys (2015) 92(5):971–7. doi:10.1016/j.ijrobp.2015.04.030
33. King CR, Brooks JD, Gill H, Presti JC Jr. Long-term outcomes from a prospective trial of stereotactic body radiotherapy for low-risk prostate cancer. Int J Radiat Oncol Biol Phys (2012) 82(2):877–82. doi:10.1016/j.ijrobp.2010.11.054
34. Joh DY, Chen LN, Porter G, Bhagat A, Sood S, Kim JS, et al. Proctitis following stereotactic body radiation therapy for prostate cancer. Radiat Oncol (2014) 9:277. doi:10.1186/s13014-014-0277-4
35. Lawton CA, Michalski J, El-Naqa I, Buyyounouski MK, Lee WR, Menard C, et al. RTOG GU Radiation oncology specialists reach consensus on pelvic lymph node volumes for high-risk prostate cancer. Int J Radiat Oncol Biol Phys (2009) 74(2):383–7. doi:10.1016/j.ijrobp.2008.08.002
36. Bruner DW, Hunt D, Michalski JM, Bosch WR, Galvin JM, Amin M, et al. Preliminary patient-reported outcomes analysis of 3-dimensional radiation therapy versus intensity-modulated radiation therapy on the high-dose arm of the Radiation Therapy Oncology Group (RTOG) 0126 prostate cancer trial. Cancer (2015) 121(14):2422–30. doi:10.1002/cncr.29362
37. Schmid MP, Pötter R, Bombosch V, Sljivic S, Kirisits C, Dörr W, et al. Late gastrointestinal and urogenital side-effects after radiotherapy – incidence and prevalence. Subgroup-analysis within the prospective Austrian–German phase II multicenter trial for localized prostate cancer. Radiother Oncol (2012) 104(1):114–8. doi:10.1016/j.radonc.2012.05.007
38. Lee JY, Scarlett S, Daignault-Newton S, Sanda MG, Michalski JM, Sandler HM, et al. Impact of rectal bleeding on quality of life measures after prostate radiation therapy: comparison of the EPIC-26 and EPIC-CP instruments. Int J Radiat Oncol Biol Phys (2015) 93(3):S201. doi:10.1016/j.ijrobp.2015.07.481
39. Wei JT, Dunn RL, Litwin MS, Sandler HM, Sanda MG. Development and validation of the expanded prostate cancer index composite (EPIC) for comprehensive assessment of health-related quality of life in men with prostate cancer. Urology (2000) 56:899–905. doi:10.1016/S0090-4295(00)00858-X
40. Leong N, Pai HH, Morris WJ, Keyes M, Pickles T, Tyldesley S, et al. Rectal ulcers and rectoprostatic fistulas after (125)I low dose rate prostate brachytherapy. J Urol (2016) 195(6):1811–6. doi:10.1016/j.juro.2015.12.095
41. Tran A, Wallner K, Merrick G, Seeberger J, Armstrong J, Mueller A, et al. Rectal fistulas after prostate brachytherapy. Int J Radiat Oncol Biol Phys (2005) 63(1):150–4. doi:10.1016/j.ijrobp.2005.01.021
42. Pommier P, Chabaud S, Lagrange JL, Richaud P, Lesaunier F, Le Prise E, et al. Is there a role for pelvic irradiation in localized prostate adenocarcinoma? Preliminary results of GETUG-01. J Clin Oncol (2007) 25(34):5366–73. doi:10.1200/JCO.2006.10.5171
43. Lawton CA, DeSilvio M, Roach M III, Uhl V, Kirsch R, Seider M, et al. An update of the phase III trial comparing whole pelvic to prostate only radiotherapy and neoadjuvant to adjuvant total androgen suppression: updated analysis of RTOG 94-13, with emphasis on unexpected hormone/radiation interactions. Int J Radiat Oncol Biol Phys (2007) 69(3):646–55. doi:10.1016/j.ijrobp.2007.04.003
44. Zumsteg ZS, Spratt DE, Romesser PB, Pei X, Zhang Z, Kollmeier M, et al. Anatomical patterns of recurrence following biochemical relapse in the dose escalation era of external beam radiotherapy for prostate cancer. J Urol (2015) 194(6):1624–30. doi:10.1016/j.juro.2015.06.100
45. Chao KK, Goldstein NS, Yan D, Vargas CE, Ghilezan MI, Korman HJ, et al. Clinicopathologic analysis of extracapsular extension in prostate cancer: should the clinical target volume be expanded posterolaterally to account for microscopic extension? Int J Radiat Oncol Biol Phys (2006) 65(4):999–1007. doi:10.1016/j.ijrobp.2006.02.039
46. Davis BJ, Pisansky TM, Wilson TM, Rothenberg HJ, Pacelli A, Hillman DW, et al. The radial distance of extraprostatic extension of prostate carcinoma: implications for prostate brachytherapy. Cancer (1999) 85(12):2630–7. doi:10.1002/(SICI)1097-0142(19990615)85:12<2630::AID-CNCR20>3.3.CO;2-C
47. Sohayda C, Kupelian PA, Levin HS, Klein EA. Extent of extracapsular extension in localized prostate cancer. Urology (2000) 55(3):382–6. doi:10.1016/S0090-4295(99)00458-6
48. Teh BS, Bastasch MD, Wheeler TM, Mai WY, Frolov A, Uhl BM, et al. IMRT for prostate cancer: defining target volume based on correlated pathologic volume of disease. Int J Radiat Oncol Biol Phys (2003) 56(1):184–91. doi:10.1016/S0360-3016(03)00085-3
49. Ju AW, Wang H, Oermann EK, Sherer BA, Uhm S, Chen VJ, et al. Hypofractionated stereotactic body radiation therapy as monotherapy for intermediate-risk prostate cancer. Radiat Oncol (2013) 8:30. doi:10.1186/1748-717X-8-30
50. Ruggieri R, Naccarato S, Stavrev P, Stavreva N, Fersino S, Giaj Levra N, et al. Volumetric-modulated arc stereotactic body radiotherapy for prostate cancer: dosimetric impact of an increased near-maximum target dose and of a rectal spacer. Br J Radiol (2015) 88(1054):20140736. doi:10.1259/bjr.20140736
Keywords: prostate cancer, SBRT, IMRT, CyberKnife, common terminology criteria
Citation: Paydar I, Pepin A, Cyr RA, King J, Yung TM, Bullock EG, Lei S, Satinsky A, Harter KW, Suy S, Dritschilo A, Lynch JH, Kole TP and Collins SP (2017) Intensity-Modulated Radiation Therapy with Stereotactic Body Radiation Therapy Boost for Unfavorable Prostate Cancer: A Report on 3-Year Toxicity. Front. Oncol. 7:5. doi: 10.3389/fonc.2017.00005
Received: 11 November 2016; Accepted: 05 January 2017;
Published: 07 February 2017
Edited by:
William Small Jr., Stritch School of Medicine, USAReviewed by:
John Austin Vargo, University of Pittsburgh, USAWilliam F. Hartsell, Northwestern Medicine Chicago Proton Center, USA
Copyright: © 2017 Paydar, Pepin, Cyr, King, Yung, Bullock, Lei, Satinsky, Harter, Suy, Dritschilo, Lynch, Kole and Collins. This is an open-access article distributed under the terms of the Creative Commons Attribution License (CC BY). The use, distribution or reproduction in other forums is permitted, provided the original author(s) or licensor are credited and that the original publication in this journal is cited, in accordance with accepted academic practice. No use, distribution or reproduction is permitted which does not comply with these terms.
*Correspondence: Sean P. Collins, spc9@gunet.georgetown.edu