- 1Translational Immunology Laboratory, VIB, Leuven, Belgium
- 2Department of Microbiology and Immunology, KU Leuven, Leuven, Belgium
- 3Department of Imaging and Pathology, KU Leuven, Leuven, Belgium
Vitamin D has been proposed as a therapeutic strategy in pancreatic cancer, yet evidence for an effect of dietary vitamin D on pancreatic cancer is ambiguous, with conflicting data from human epidemiological and intervention studies. Here, we tested the role of dietary vitamin D in the in vivo context of the well-characterized Ela1-TAg transgenic mouse model of pancreatic acinar cell carcinoma. Through longitudinal magnetic resonance imaging of mice under conditions of either dietary vitamin D deficiency (<5 IU/kg vitamin D) or excess (76,500 IU/kg vitamin D), compared to control diet (1,500 IU/kg vitamin D), we measured the effect of variation of dietary vitamin D on tumor kinetics. No measurable impact of dietary vitamin D was found on pancreatic acinar cell carcinoma development, growth or mortality, casting further doubt on the already equivocal data supporting potential therapeutic use in humans. The lack of any detectable effect of vitamin D, within the physiological range of dietary deficiency or supplementation, in this model further erodes confidence in vitamin D as an effective antitumor therapeutic in pancreatic acinar cell carcinoma.
Introduction
Vitamin D has been proposed as a potential therapeutic for pancreatic cancer, a disease for which efficacious treatments are currently lacking; however, the evidence for an impact of vitamin D on pancreatic cancer is ambiguous. The most consistent evidence for vitamin D as an antitumor drug comes from cell line work. Vitamin D has been shown to be effective at inhibiting the proliferation of pancreatic cancer cell lines in vitro, including the active form 1α,25-dihydroxyvitamin D3 (1, 2), the prohormone 25-hydroxyvitamin D(3) (3), and the analogs calcipotriol (4, 5), 22-oxa-1,25-dihydroxyvitamin D3 (5), EB 1089 (2, 6, 7), MART-10 (8), 1,25-dihydroxyvitamin D(3)-3-bromoacetate (9), 19-nor-1 alpha,25-dihydroxyvitamin D2 (paricalcitol) (10), and 22- oxa-1,25-dihydroxyvitamin D3 (maxacalcitol) (11). The antiproliferative effect has even been observed in vivo, when pancreatic cancer cell lines have been transplanted into immunodeficiency mice (2, 5, 7, 8, 10, 11). It should, however, be noted that this effect is not universal, as when multiple cell lines have been tested, it is only a minority that respond to vitamin D in vitro (5, 10, 11). In addition, the complex relationship between tumor, stroma, and the immune system in lost under these testing conditions, so the results in cell lines may not reflect the physiological impact on patients.
Epidemiological studies on the interaction between vitamin D and pancreatic cancer have proven inconsistent. A clear correlation of pancreatic cancer risk has been associated with increasing latitude and lower ultraviolet B (UVB) radiation, known to induce vitamin D production. A protective effect of UVB dose is observed across countries (12, 13) and within individual countries (14–17), and a similar correlation shows reduced risk in individuals with fair skin color (15). A simple explanation of this phenomenon would be that vitamin D production protects against pancreatic cancer, yet the evidence for this is underwhelming. The best surrogate for vitamin D levels is circulating 25-hydroxyvitamin D. Cohort analysis for pancreatic cancer risk have variously identified either low (18, 19) or high (20, 21) plasma 25-hydroxyvitamin D as a risk factor for developing pancreatic cancer, with a meta-analysis indicating no significant association (22). Following pancreatic cancer development, analysis of a prospective cohort found reduced survival in patients who had insufficient levels of plasma 25-hydroxyvitamin D (23), yet a retrospective analysis of other cohorts found no link with survival (24, 25). Likewise studies have found either no link (26) or only a weak protective link (27) between plasma levels of vitamin D-binding protein, the primary carrier of 25-hydroxyvitamin D, and pancreatic cancer risk. A link between vitamin D receptor (VDR) polymorphisms and pancreatic cancer risk has been observed in the Chinese population (28), but other large studies have found no link with any of the genes in the vitamin D pathway (23, 29). Together, these studies place doubt over the link between endogenous vitamin D and pancreatic cancer risk, without excluding a potential benefit for exogenous administration.
In contrast to the large number of epidemiological studies investigating the link between pancreatic cancer and vitamin D, relatively few interventional trials have been performed. A phase II trial of EB 1089 found no antitumor effect (30), while a phase II trial of docetaxel gave a modest effect in three patients (31). Larger studies have been performed with dietary vitamin D supplementations, with two studies indicating an increased risk of pancreatic cancer after supplementation (32, 33), but a meta-analysis of nine studies finding overall no significant association of dietary vitamin D with pancreatic cancer (22). Overall, despite the positive results from pancreatic cancer cell lines, the limited epidemiological and intervention studies performed leave the status of vitamin D as a potential therapeutic for pancreatic cancer in doubt. Here, we have sought to formally test the role of dietary vitamin D in the in vivo context through utilization of a well-characterized animal model of pancreatic acinar cell carcinoma, the Ela1-TAg transgenic mouse. Through longitudinal magnetic resonance imaging (MRI) assessment of Ela1-TAg transgenic mice under conditions of either vitamin D deficiency or dietary excess, we measured the effect of vitamin D on spontaneous tumor development, growth rates, and mortality. The lack of any detectable effect of vitamin D deficiency or dietary supplementation in this model further erodes confidence in vitamin D as an effective antitumor therapeutic in pancreatic acinar cell carcinoma.
Materials and Methods
Mice
Ela1-TAg mice, expressing the SV40 large T Antigen under the control of the Elastase-1 acinar cell promoter, were purchased from Jackson on the C57BL/6 background (34). Mice were bred under specific pathogen-free conditions and from the time of breeder setup were exclusively fed on either ssniff® EF R/M Control chow (1,500 IU/kg vitamin D, 0.9% Calcium; “control diet”), ssniff® EF R/M Vitamin D3-deficient chow (<5 IU/kg vitamin D; “Vitamin D deficient diet,” 0.9% Calcium), or ssniff® EF R/M Vitamin D3 excess chow (76,500 IU/kg vitamin D, 1.59% Calcium; “Vitamin D excess diet”). Mice were moved to conventional conditions at 7 weeks of age (maintaining special diets) for longitudinal MRI. All experimental protocols were approved by the University of Leuven Animal Ethics Committee, and all experiments were performed in accordance with the guidelines and regulations from the University of Leuven Animal Ethics Committee. Mouse weight and blood glucose were monitored throughout.
Imaging
Mice were scanned under isoflurane anesthesia using a Bruker Biospin 9.4 T Biospec small animal MR scanner (Bruker Biospin, Ettlingen, Germany). The scanner was equipped with an actively shielded gradient set of 600 mT/m using a respiration triggered spin echo sequence (RARE) with 50 continuous slices of 0.5 mm thickness in interlaced mode (acquisition parameters: repetition time = 6,000 ms, echo time = 15.9 ms, field of view = 4.0 cm × 6.0 cm, a matrix of 200 × 400, two dummy scans, and two averages). For radio-frequency irradiation and detection, a 7.2-cm quadrature resonator was used.
Data and Statistical Analysis
Magnetic resonance imaging scans were analyzed with ImageJ (National Institute of Health, Bethesda, MD, USA) and the mean area at maximum radius was used to infer volume using the formula: 4/3*area*√(area/π). Statistical analysis was performed in R (https://www.r-project.org/ version 3.1.2). Cumulative incidence curves were generated using the R package “survplot” with the fun = function (x) {1 − x} argument (35). Survival curves were generated using the Kaplan–Meier method implemented in the R “survplot” package, and statistical analysis was performed using log-rank test implemented in the R “survdiff” package (36).
Results
In order to systematically test the role of dietary vitamin D in pancreatic acinar cell carcinoma development, growth, and mortality, we utilized the well-characterized Ela1-TAg transgenic mouse model, where expression of the SV40 large T Antigen results in the spontaneous formation of pancreatic tumors from acinar cells (34). To maximize the differences in vitamin D exposure, breeder cages were setup on either control diet (1,500 IU/kg vitamin D3), vitamin D-deficient diet (<5 IU/kg vitamin D3), or vitamin D excess diet (76,500 IU/kg vitamin D3). This study design allows alteration of dietary vitamin D levels from the in utero condition onward, with mice being weaned onto the corresponding diet. From 7 weeks of age, each mouse underwent MRI scanning for tumor detection and volume estimation, revealing exponential growth of tumors after first detection (Figure 1).
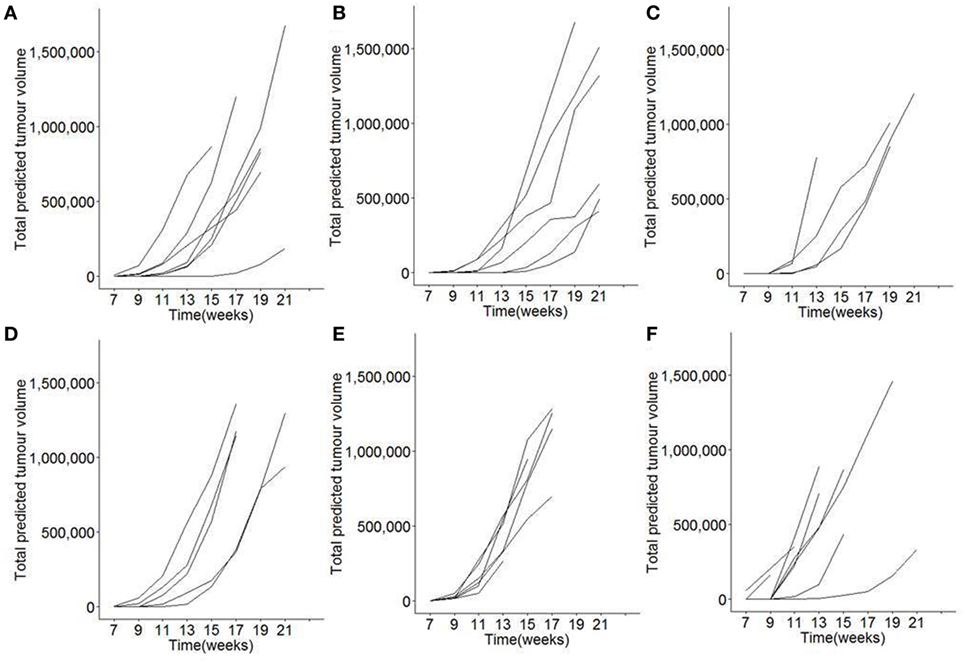
Figure 1. Longitudinal monitoring of tumor growth following modification of dietary vitamin D in mice. TAg+ mice were placed on either control, vitamin D deficient, or vitamin D excess diets in utero and aged on the same diets to 21 weeks of age. From 7 weeks onward, mice were assessed through Magnetic Resonance Imaging for tumor size. Individual total predicted tumor volume curves for (A) female mice on control diet (n = 7), (B) female mice on vitamin D-deficient diet (n = 6), (C) female mice on vitamin D excess diet (n = 4), (D) male mice on control diet (n = 5), (E) male mice on vitamin D-deficient diet (n = 6), and (F) male mice on vitamin D excess diet (n = 9).
To determine the effect of dietary vitamin D on initial tumor development, the age of first tumor detection was assessed. Reliable tumor detection was achieved for tumors >3 mm in size (data not shown). For female mice, no significant difference was observed in the cumulative incidence of pancreatic acinar cell carcinoma (Figure 2A). For male mice, marginal significance was observed for mice fed a vitamin D-deficient diet having earlier tumor onset (Figure 2B); however, this result was not significant when age of first tumor detection was directly tested (Figure 2C). It is also notable that the data from female mice did not even follow the same trend, indicating that there is no consistent effect of dietary vitamin D, either in deficiency or in supplementation, on tumor onset.
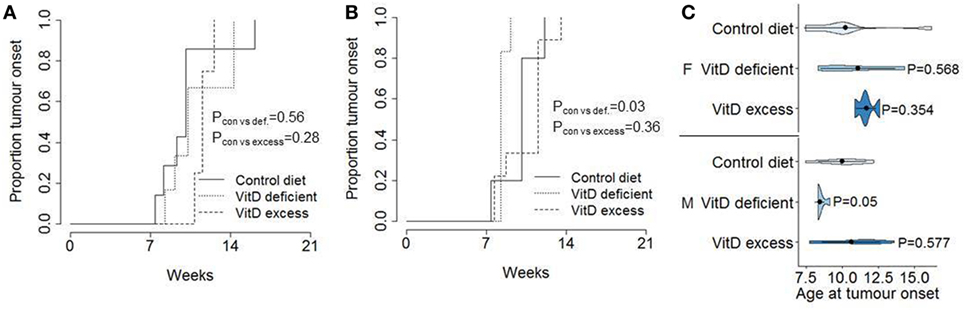
Figure 2. No consistent effect of dietary vitamin D on tumor onset in a pancreatic cancer model. TAg+ mice were placed on either control, vitamin D-deficient, or vitamin D excess diets in utero and aged on the same diets to 21 weeks of age. Cumulative incidence of pancreatic cancer was recorded as a function of age at tumor detection, in (A) female (n = 7, 6, 4) and (B) male (n = 5, 6, 9) mice. The P values were calculated using the log-rank test. (C) Violin plots showing the mean, SD, and kernel probability density of the age at tumor onset under each condition. The P values were calculated using two-tailed unpaired t test.
We next assessed the growth rate of the established tumor burden. Due to the variable onset and exponential growth rates (Figure 1), total tumor volume estimates from MRI were square root transformed and plotted from time of first detection (Figures 3A–F). Linear plots on the transformed graphs indicate that once established the tumors exhibited a consistent growth rate until experimental end-point. This analysis allows tumor growth to be calculated as the percentage increase in total tumor volume every 14 days (i.e., time between MRI assessment), averaged over the entire period of monitoring. Using this criteria, no difference was observed in tumor growth rates in either males or females, under conditions of either vitamin D dietary deficiency or supplementation (Figure 3G).
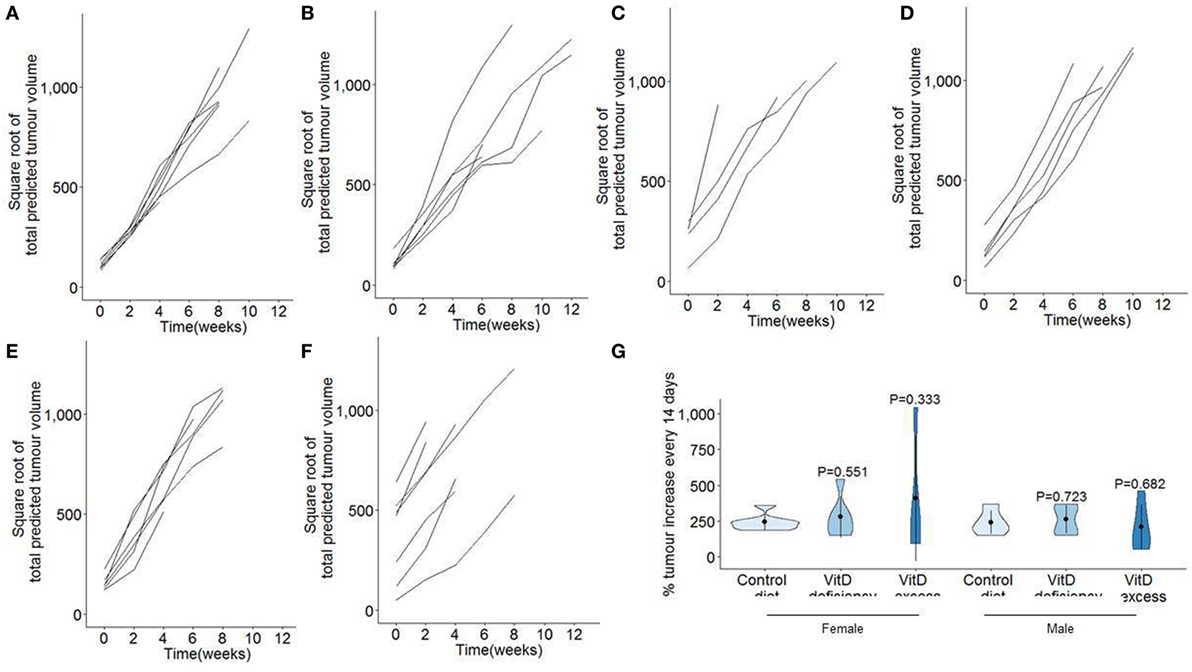
Figure 3. No effect of dietary vitamin D on tumor growth in a pancreatic cancer model. TAg+ mice were placed on either control, vitamin D-deficient or vitamin D excess diets in utero and aged on the same diets to 21 weeks of age. From 7 weeks onward, mice were assessed through Magnetic Resonance Imaging for tumor size. Total predicted tumor volumes were square root transformed and normalized to age of first detection for (A) female mice on control diet (n = 7), (B) female mice on vitamin D-deficient diet (n = 6), (C) female mice on vitamin D excess diet (n = 4), (D) male mice on control diet (n = 5), (E) male mice on vitamin D-deficient diet (n = 6), and (F) male mice on vitamin D excess diet (n = 9). (G) Violin plots showing the mean, SD, and kernel probability density of the percentage of tumor volume increase every 2 weeks. The P values were calculated using two-tailed unpaired t test.
Finally, we assessed the impact that changes to dietary vitamin D had on tumor-induced mortality (Figure 4). On the control diet, 50% of mice had reached end-point (condition deteriorating to end-point guidelines) by 20 weeks in female mice (Figure 4A) and 18 weeks in male mice (Figure 4B). No significant alteration was observed in female mice by changing the vitamin D content of the diet (Figure 4A). In male mice, an increase in mortality was observed in mice fed a vitamin D-deficient diet (Figure 4B); however, the significance was marginal compared to control diet and there was no difference between vitamin D deficiency and vitamin D excess. Overall, these results do not indicate a convincing impact of vitamin D on mortality in this murine model of pancreatic acinar cell carcinoma.
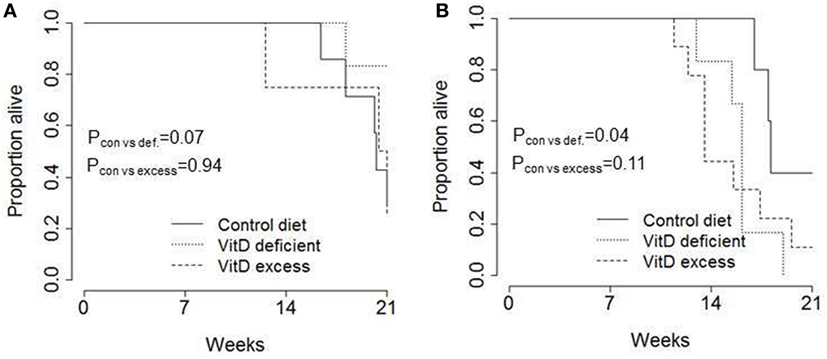
Figure 4. No consistent impact of dietary vitamin D on survival in a transgenic pancreatic cancer model. TAg+ mice were placed on either control, vitamin D-deficient or vitamin D excess diets in utero and aged on the same diets to 21 weeks of age. Kaplan–Meier plots showing the overall pancreatic cancer survival in (A) female (n = 7, 6, 4) and (B) male (n = 5, 6, 9) mice on control, vitamin D-deficient and vitamin D excess diets. The P values were calculated using the log-rank test.
Discussion
Direct comparisons between the dietary doses used here and the recommended dietary intakes in humans are complicated by species differences. The European Food Safety Authority recommends 200 IU/day intake for adults, with an upper intake of 4,000 IU/day. The diets used here result in consumption of 0.02 IU/day (Vitamin D deficient), 5 IU/day (control), and 250 IU/day (Vitamin D excess). These values, however, do not take into account the body weight difference between mice and humans; normalized to average human body weight, the daily intakes range from far below recommended intake (30 IU/day/70 kg for Vitamin D-deficient diet) to far above the upper intake limit (9,000 IU/day/70 kg for control diet and 460,000 IU/day/70 kg). The most relevant comparison, however, is probably the resulting circulating serum 1α,25-dihydroxyvitamin D3 level. In adult humans, the desirable level of 1α,25-dihydroxyvitamin D3 is 20 ng/ml, while ostemolacia or rickets develops at levels lower than 10 ng/ml, adverse effects are reported at levels above 80 ng/ml and toxicity at 150 ng/ml and greater (37–39). In mice, diets with vitamin D levels below 125 IU/kg result in serum levels of 1α,25-dihydroxyvitamin D3 in the sub-20 ng/ml range, diets 1,000–5,000 IU/kg result in serum 1α,25-dihydroxyvitamin D3 in the 20–40 ng/ml range, 125,000 IU/kg causes 150–200 ng/ml serum 1α,25-dihydroxyvitamin D3, 250,000–500,000 IU/kg causes >600 ng/ml serum 1α,25-dihydroxyvitamin D3 and hypercalcemia, while >1,000,000 IU/kg results in toxicity (40–42). An approximate cross-species comparison would, therefore, have the “Vitamin D deficient” diet paralleling Vitamin D deficiency in humans, the “control” diet resulting in serum 1α,25-dihydroxyvitamin D3 in the recommended range for humans, and the “Vitamin D excess” diet resulting in the serum 1α,25-dihydroxyvitamin D3 above the recommended range for humans and approaching the threshold for adverse effects.
The lack of any measurable impact of vitamin D on pancreatic acinar cell carcinoma development, growth, or mortality in the TAg mouse model casts further doubt on the already equivocal data supporting potential therapeutic use in humans. There are, however, several pertinent caveats to be made, including the use of a single mouse model [as the low-throughput nature of mouse models prohibits parallel screening (43)], potential differences between acinar cell carcinomas and other pancreatic tumors, and the species barrier. Nevertheless, while there are distinct limitations to mouse models for pancreatic cancer, they also have distinct advantages over analysis of cell lines. The generation of pancreatic cancer cell lines necessitates a selection for independence from micro-environmental cues and stromal interactions. In effect, any molecule which inhibits proliferation will exhibit antitumor impacts in these systems, regardless of additional more complex pro-tumor effects which may negate the antitumor properties. Vitamin D and its analogs are thought to directly inhibit proliferation by increasing p21 and p27 (11, 44) and inhibiting FOXM1 (2) and the Wnt/β-catenin signaling pathway (4). In vivo, other, opposing, cues may come into consideration, such as the effect of vitamin D in countering retinoic acid-induced apoptosis (45) and the tolerogenic impact vitamin D has on the immune system (46). This latter effect may have profound pro-tumor consequences, and yet the interaction will be absent not only in in vitro models but also in the xenograft transplantations, due to the use of immunocompromised mice. The most parsimonious reconciliation of the cell line and mouse model work is that the antiproliferative impact of vitamin D is negated in vivo by these additional pro-tumor effects.
How then to account for the inconsistent associations found between serum vitamin D and pancreatic cancer? One plausible explanation lies in the known risks factors of pancreatic cancer, which include smoking, lack of physical activity, obesity, type 2 diabetes, and chronic pancreatitis (47). In several studies, smoking has been demonstrated to reduce serum 1α,25-dihydroxyvitamin D3 levels (48–50). Likewise, increased body mass index (BMI), an (imperfect) measure of obesity, is consistently associated with reduced serum 1α,25-dihydroxyvitamin D3 (51). Lower 1α,25-dihydroxyvitamin D3 is also found in patients with either type 2 diabetes (52) or pancreatitis from various sources (53). Increased 1α,25-dihydroxyvitamin D3 is also associated with increased exercise, although the effect is difficult to entangle from increased exposure to UVB and reduced BMI (54). In any case, lower vitamin D levels are directly associated with many of the major risk factors for pancreatic cancer. Thus, it is possible that the inconsistent association of vitamin D with pancreatic cancer is actually a reflection of these indirect (and non-causative) associations.
The lack of support for an anti-oncogenic role of vitamin D in pancreatic cancer stands in contrast to that observed in several other solid tumors. In colorectal cancer, for example, vitamin D (55, 56) and various vitamin D analogs (57, 58) have proven efficacious as antitumorigenic agents in multiple mouse models. Supporting this function, genetic ablation of VDR in mice increases development of colorectal cancers (59, 60). Beyond pancreatic cancer the evidence from humans is also stronger. Epidemiological data from human studies support a protective role for vitamin D in colorectal cancer and bladder cancer, with elevated circulating 1α,25-dihydroxyvitamin D3 levels reducing cancer risk (61). For other cancer types, the epidemiological data remains equivocal, or, in the case of prostate cancer, even identifies a negative association (61), although there are suggestive protective associations with several. The effect of vitamin D on cancer may also be broader than risk of development; in breast cancer, for example, circulating 1α,25-dihydroxyvitamin D3 is only weakly associated with risk, but is strongly associated with survival (62). At a genetic level, polymorphisms in VDR are associated with total risk of developing cancer (63), with the strongest effects being on skin cancer and gynecological cancers (63, 64), while several other cancers show no consistent association (although stratification by ethnicity and sex can identify specific risk groups). Our negative results in pancreatic cancer should not, therefore, be taken as evidence against the protective nature of vitamin D in other cancers, although it does caution against excessively broad characterization of vitamin D as a generic panacea for cancer.
Finally, we would note that even direct extrapolation of our study to the human context, which would not be advisable without the study of additional models, would not completely preclude therapeutic potential of vitamin D. First, our study was performed on acinar cell carcinoma, and it is plausible that other, more common, forms of pancreatic cancer may respond differently to vitamin D. Second, we used dietary modification with classical vitamin D, while therapeutic approaches in patients may include the use of alternative delivery systems and modified vitamin D analogs capable of more potent biological effects. Third, VDR was recently identified as a gemcitabine sensitizer in pancreatic cancer cells in an in vitro screen (65), and in a study of transplanted murine tumor cells into immuno-competent mice the vitamin D analog calcipotriol enhanced the antitumor effect of gemcitabine (66). Thus even in the absence of a monotherapeutic in vivo antitumor effect, there is potential for vitamin D to exhibit utility as a component of a combination therapy.
Ethics Statement
All experimental protocols were approved by the University of Leuven Animal Ethics Committee, and all experiments were performed in accordance with the guidelines and regulations from the University of Leuven Animal Ethics Committee.
Author Contributions
JD and AL designed the study. JD, NH, TD, and UH performed experiments. VL analyzed the data. AL wrote the manuscript, which was reviewed and approved by all authors.
Conflict of Interest Statement
The authors declare that the research was conducted in the absence of any commercial or financial relationships that could be construed as a potential conflict of interest.
Acknowledgments
This work was supported by the VIB and IUAP (T-TIME). VL was supported by an FWO post-doctoral fellowship. The authors thank Yulia Lampi and Umberto Papa for technical assistance and all staff at the molecular Small Animal Imaging Center (moSAIC) for MRI training and assistance.
References
1. Kanemaru M, Maehara N, Chijiiwa K. Antiproliferative effect of 1alpha,25-dihydroxyvitamin D3 involves upregulation of cyclin-dependent kinase inhibitor p21 in human pancreatic cancer cells. Hepatogastroenterology (2013) 60:1199–205. doi:10.5754/hge11073
2. Li Z, Jia Z, Gao Y, Xie D, Wei D, Cui J, et al. Activation of vitamin D receptor signaling downregulates the expression of nuclear FOXM1 protein and suppresses pancreatic cancer cell stemness. Clin Cancer Res (2015) 21:844–53. doi:10.1158/1078-0432.CCR-14-2437
3. Schwartz GG, Eads D, Rao A, Cramer SD, Willingham MC, Chen TC, et al. Pancreatic cancer cells express 25-hydroxyvitamin D-1 alpha-hydroxylase and their proliferation is inhibited by the prohormone 25-hydroxyvitamin D3. Carcinogenesis (2004) 25:1015–26. doi:10.1093/carcin/bgh086
4. Arensman MD, Nguyen P, Kershaw KM, Lay AR, Ostertag-Hill CA, Sherman MH, et al. Calcipotriol targets LRP6 to inhibit Wnt signaling in pancreatic cancer. Mol Cancer Res (2015) 13:1509–19. doi:10.1158/1541-7786.MCR-15-0204
5. Kawa S, Yoshizawa K, Tokoo M, Imai H, Oguchi H, Kiyosawa K, et al. Inhibitory effect of 220-oxa-1,25-dihydroxyvitamin D3 on the proliferation of pancreatic cancer cell lines. Gastroenterology (1996) 110:1605–13. doi:10.1053/gast.1996.v110.pm8613068
6. Zugmaier G, Jäger R, Grage B, Gottardis MM, Havemann K, Knabbe C. Growth-inhibitory effects of vitamin D analogues and retinoids on human pancreatic cancer cells. Br J Cancer (1996) 73:1341–6. doi:10.1038/bjc.1996.256
7. Colston KW, James SY, Ofori-Kuragu EA, Binderup L, Grant AG. Vitamin D receptors and anti-proliferative effects of vitamin D derivatives in human pancreatic carcinoma cells in vivo and in vitro. Br J Cancer (1997) 76:1017–20. doi:10.1038/bjc.1997.501
8. Chiang KC, Yeh CN, Hsu JT, Yeh TS, Jan YY, Wu CT, et al. Evaluation of the potential therapeutic role of a new generation of vitamin D analog, MART-10, in human pancreatic cancer cells in vitro and in vivo. Cell Cycle (2013) 12:1316–25. doi:10.4161/cc.24445
9. Persons KS, Eddy VJ, Chadid S, Deoliveira R, Saha AK, Ray R. Anti-growth effect of 1,25-dihydroxyvitamin D3-3-bromoacetate alone or in combination with 5-amino-imidazole-4-carboxamide-1-beta-4-ribofuranoside in pancreatic cancer cells. Anticancer Res (2010) 30:1875–80.
10. Schwartz GG, Eads D, Naczki C, Northrup S, Chen T, Koumenis C. 19-nor-1 alpha,25-dihydroxyvitamin D2 (paricalcitol) inhibits the proliferation of human pancreatic cancer cells in vitro and in vivo. Cancer Biol Ther (2008) 7:430–6. doi:10.4161/cbt.7.3.5418
11. Kawa S, Yoshizawa K, Nikaido T, Kiyosawa K. Inhibitory effect of 22-oxa-1,25-dihydroxyvitamin D3, maxacalcitol, on the proliferation of pancreatic cancer cell lines. J Steroid Biochem Mol Biol (2005) 97:173–7. doi:10.1016/j.jsbmb.2005.06.021
12. Garland CF, Cuomo RE, Gorham ED, Zeng K, Mohr SB. Cloud cover-adjusted ultraviolet B irradiance and pancreatic cancer incidence in 172 countries. J Steroid Biochem Mol Biol (2016) 155:257–63. doi:10.1016/j.jsbmb.2015.04.004
13. Mohr SB, Garland CF, Gorham ED, Grant WB, Garland FC. Ultraviolet B irradiance and vitamin D status are inversely associated with incidence rates of pancreatic cancer worldwide. Pancreas (2010) 39:669–74. doi:10.1097/MPA.0b013e3181ce654d
14. Grant WB. Role of solar UVB irradiance and smoking in cancer as inferred from cancer incidence rates by occupation in Nordic countries. Dermatoendocrinol (2012) 4:203–11. doi:10.4161/derm.20965
15. Tran B, Whiteman DC, Webb PM, Fritschi L, Fawcett J, Risch HA, et al. Association between ultraviolet radiation, skin sun sensitivity and risk of pancreatic cancer. Cancer Epidemiol (2013) 37:886–92. doi:10.1016/j.canep.2013.08.013
16. Neale RE, Youlden DR, Krnjacki L, Kimlin MG, van der Pols JC. Latitude variation in pancreatic cancer mortality in Australia. Pancreas (2009) 38:387–90. doi:10.1097/MPA.0b013e31819975f4
17. Kinoshita S, Wagatsuma Y, Okada M. Geographical distribution for malignant neoplasm of the pancreas in relation to selected climatic factors in Japan. Int J Health Geogr (2007) 6:34. doi:10.1186/1476-072X-6-34
18. Wolpin BM, Ng K, Bao Y, Kraft P, Stampfer MJ, Michaud DS, et al. Plasma 25-hydroxyvitamin D and risk of pancreatic cancer. Cancer Epidemiol Biomarkers Prev (2012) 21:82–91. doi:10.1158/1055-9965.EPI-11-0836
19. Bao Y, Ng K, Wolpin BM, Michaud DS, Giovannucci E, Fuchs CS. Predicted vitamin D status and pancreatic cancer risk in two prospective cohort studies. Br J Cancer (2010) 102:1422–7. doi:10.1038/sj.bjc.6605658
20. Stolzenberg-Solomon RZ, Vieth R, Azad A, Pietinen P, Taylor PR, Virtamo J, et al. A prospective nested case-control study of vitamin D status and pancreatic cancer risk in male smokers. Cancer Res (2006) 66:10213–9. doi:10.1158/0008-5472.CAN-06-1876
21. Stolzenberg-Solomon RZ, Jacobs EJ, Arslan AA, Qi D, Patel AV, Helzlsouer KJ, et al. Circulating 25-hydroxyvitamin D and risk of pancreatic cancer: cohort consortium vitamin D pooling project of rarer cancers. Am J Epidemiol (2010) 172:81–93. doi:10.1093/aje/kwq120
22. Liu SL, Zhao YP, Dai MH, You L, Wen Z, Xu JW. Vitamin D status and the risk of pancreatic cancer: a meta-analysis. Chin Med J (Engl) (2013) 126:3356–9.
23. Yuan C, Qian ZR, Babic A, Morales-Oyarvide V, Rubinson DA, Kraft P, et al. Prediagnostic plasma 25-hydroxyvitamin D and pancreatic cancer survival. J Clin Oncol (2016) 34:2899–905. doi:10.1200/JCO.2015.66.3005
24. McGovern EM, Lewis ME, Niesley ML, Huynh N, Hoag JB. Retrospective analysis of the influence of 25-hydroxyvitamin D on disease progression and survival in pancreatic cancer. Nutr J (2016) 15:17. doi:10.1186/s12937-016-0135-3
25. Van Loon K, Owzar K, Jiang C, Kindler HL, Mulcahy MF, Niedzwiecki D, et al. 25-Hydroxyvitamin D levels and survival in advanced pancreatic cancer: findings from CALGB 80303 (Alliance). J Natl Cancer Inst (2014) 106:dju185. doi:10.1093/jnci/dju185
26. Piper MR, Freedman DM, Robien K, Kopp W, Rager H, Horst RL, et al. Vitamin D-binding protein and pancreatic cancer: a nested case-control study. Am J Clin Nutr (2015) 101:1206–15. doi:10.3945/ajcn.114.096016
27. Weinstein SJ, Stolzenberg-Solomon RZ, Kopp W, Rager H, Virtamo J, Albanes D. Impact of circulating vitamin D binding protein levels on the association between 25-hydroxyvitamin D and pancreatic cancer risk: a nested case-control study. Cancer Res (2012) 72:1190–8. doi:10.1158/0008-5472.CAN-11-2950
28. Li L, Wu B, Yang L, Yin G, Wei W, Sui S, et al. Association of vitamin D receptor gene polymorphisms with pancreatic cancer: a pilot study in a North China population. Oncol Lett (2013) 5:1731–5. doi:10.3892/ol.2013.1215
29. Arem H, Yu K, Xiong X, Moy K, Freedman ND, Mayne ST, et al. Vitamin D metabolic pathway genes and pancreatic cancer risk. PLoS One (2015) 10:e0117574. doi:10.1371/journal.pone.0117574
30. Evans TR, Colston KW, Lofts FJ, Cunningham D, Anthoney DA, Gogas H, et al. A phase II trial of the vitamin D analogue Seocalcitol (EB1089) in patients with inoperable pancreatic cancer. Br J Cancer (2002) 86:680–5. doi:10.1038/sj.bjc.6600162
31. Blanke CD, Beer TM, Todd K, Mori M, Stone M, Lopez C. Phase II study of calcitriol-enhanced docetaxel in patients with previously untreated metastatic or locally advanced pancreatic cancer. Invest New Drugs (2009) 27:374–8. doi:10.1007/s10637-008-9184-6
32. Waterhouse M, Risch HA, Bosetti C, Anderson KE, Petersen GM, Bamlet WR, et al. Vitamin D and pancreatic cancer: a pooled analysis from the pancreatic cancer case-control consortium. Ann Oncol (2015) 26:1776–83. doi:10.1093/annonc/mdv236
33. Zablotska LB, Gong Z, Wang F, Holly EA, Bracci PM. Vitamin D, calcium, and retinol intake, and pancreatic cancer in a population-based case-control study in the San Francisco Bay area. Cancer Causes Control (2011) 22:91–100. doi:10.1007/s10552-010-9678-3
34. Ornitz DM, Hammer RE, Messing A, Palmiter RD, Brinster RL. Pancreatic neoplasia induced by SV40 T-antigen expression in acinar cells of transgenic mice. Science (1987) 238:188–93. doi:10.1126/science.2821617
35. Teno JM, Harrell FE Jr, Knaus W, Phillips RS, Wu AW, Connors A Jr, et al. Prediction of survival for older hospitalized patients: the HELP survival model. Hospitalized elderly longitudinal project. J Am Geriatr Soc (2000) 48:S16–24. doi:10.1111/j.1532-5415.2000.tb03126.x
36. Harrington DP, Fleming TR. A class of rank test procedures for censored survival-data. Biometrika (1982) 69:553–66. doi:10.1093/biomet/69.3.553
38. Dahlquist DT, Dieter BP, Koehle MS. Plausible ergogenic effects of vitamin D on athletic performance and recovery. J Int Soc Sports Nutr (2015) 12:33. doi:10.1186/s12970-015-0093-8
39. Ross AC, Manson JE, Abrams SA, Aloia JF, Brannon PM, Clinton SK, et al. The 2011 report on dietary reference intakes for calcium and vitamin D from the institute of medicine: what clinicians need to know. J Clin Endocrinol Metab (2011) 96:53–8. doi:10.1210/jc.2010-2704
40. Seldeen KL, Pang M, Rodríguez-Gonzalez M, Hernandez M, Sheridan Z, Yu P, et al. A mouse model of vitamin D insufficiency: is there a relationship between 25(OH) vitamin D levels and obesity? Nutr Metab (Lond) (2017) 14:26. doi:10.1186/s12986-017-0174-6
41. van der Meijden K, Buskermolen J, van Essen HW, Schuurman T, Steegenga WT, Brouwer-Brolsma EM, et al. Long-term vitamin D deficiency in older adult C57BL/6 mice does not affect bone structure, remodeling and mineralization. J Steroid Biochem Mol Biol (2016) 164:344–52. doi:10.1016/j.jsbmb.2015.09.004
42. Deluca HF, Prahl JM, Plum LA. 1,25-Dihydroxyvitamin D is not responsible for toxicity caused by vitamin D or 25-hydroxyvitamin D. Arch Biochem Biophys (2011) 505:226–30. doi:10.1016/j.abb.2010.10.012
43. Murtaugh LC. Pathogenesis of pancreatic cancer: lessons from animal models. Toxicol Pathol (2014) 42:217–28. doi:10.1177/0192623313508250
44. Kawa S, Nikaido T, Aoki Y, Zhai Y, Kumagai T, Furihata K, et al. Vitamin D analogues up-regulate p21 and p27 during growth inhibition of pancreatic cancer cell lines. Br J Cancer (1997) 76:884–9. doi:10.1038/bjc.1997.479
45. Mouratidis PX, Dalgleish AG, Colston KW. Investigation of the mechanisms by which EB1089 abrogates apoptosis induced by 9-cis retinoic acid in pancreatic cancer cells. Pancreas (2006) 32:93–100. doi:10.1097/01.mpa.0000191648.47667.4f
46. Adorini L, Penna G. Induction of tolerogenic dendritic cells by vitamin D receptor agonists. Handb Exp Pharmacol (2009) 188:251–73. doi:10.1007/978-3-540-71029-5_12
47. Bardeesy N, DePinho RA. Pancreatic cancer biology and genetics. Nat Rev Cancer (2002) 2:897–909. doi:10.1038/nrc949
48. Brot C, Jorgensen NR, Sorensen OH. The influence of smoking on vitamin D status and calcium metabolism. Eur J Clin Nutr (1999) 53:920–6. doi:10.1038/sj.ejcn.1600870
49. Kassi EN, Stavropoulos S, Kokkoris P, Galanos A, Moutsatsou P, Dimas C, et al. Smoking is a significant determinant of low serum vitamin D in young and middle-aged healthy males. Hormones (Athens) (2015) 14:245–50. doi:10.14310/horm.2002.1521
50. Jiang CQ, Chan YH, Xu L, Jin YL, Zhu T, Zhang WS, et al. Smoking and serum vitamin D in older Chinese people: cross-sectional analysis based on the Guangzhou Biobank Cohort study. BMJ Open (2016) 6:e010946. doi:10.1136/bmjopen-2015-010946
51. Saneei P, Salehi-Abargouei A, Esmaillzadeh A. Serum 25-hydroxy vitamin D levels in relation to body mass index: a systematic review and meta-analysis. Obes Rev (2013) 14:393–404. doi:10.1111/obr.12016
52. Van Belle TL, Gysemans C, Mathieu C. Vitamin D and diabetes: the odd couple. Trends Endocrinol Metab (2013) 24:561–8. doi:10.1016/j.tem.2013.07.002
53. Klapdor S, Richter E, Klapdor R. Vitamin D status and per-oral vitamin D supplementation in patients suffering from chronic pancreatitis and pancreatic cancer disease. Anticancer Res (2012) 32:1991–8.
54. Looker AC. Do body fat and exercise modulate vitamin D status? Nutr Rev (2007) 65:S124–6. doi:10.1301/nr.2007.aug.S124-S126
55. Newmark HL, Yang K, Kurihara N, Fan K, Augenlicht LH, Lipkin M. Western-style diet-induced colonic tumors and their modulation by calcium and vitamin D in C57Bl/6 mice: a preclinical model for human sporadic colon cancer. Carcinogenesis (2009) 30:88–92. doi:10.1093/carcin/bgn229
56. Hummel DM, Thiem U, Höbaus J, Mesteri I, Gober L, Stremnitzer C, et al. Prevention of preneoplastic lesions by dietary vitamin D in a mouse model of colorectal carcinogenesis. J Steroid Biochem Mol Biol (2013) 136:284–8. doi:10.1016/j.jsbmb.2012.09.003
57. Kikuchi H, Murakami S, Suzuki S, Kudo H, Sassa S, Sakamoto S. Chemopreventive effect of a vitamin D(3) analog, alfacalcidol, on colorectal carcinogenesis in mice with ulcerative colitis. Anticancer Drugs (2007) 18:1183–7. doi:10.1097/CAD.0b013e3282eea468
58. Fichera A, Little N, Dougherty U, Mustafi R, Cerda S, Li YC, et al. A vitamin D analogue inhibits colonic carcinogenesis in the AOM/DSS model. J Surg Res (2007) 142:239–45. doi:10.1016/j.jss.2007.02.038
59. Kallay E, Pietschmann P, Toyokuni S, Bajna E, Hahn P, Mazzucco K, et al. Characterization of a vitamin D receptor knockout mouse as a model of colorectal hyperproliferation and DNA damage. Carcinogenesis (2001) 22:1429–35. doi:10.1093/carcin/22.9.1429
60. Larriba MJ, Ordóñez-Morán P, Chicote I, Martín-Fernández G, Puig I, Muñoz A, et al. Vitamin D receptor deficiency enhances Wnt/beta-catenin signaling and tumor burden in colon cancer. PLoS One (2011) 6:e23524. doi:10.1371/journal.pone.0023524
61. Mondul AM, Weinstein SJ, Layne TM, Albanes D. Vitamin D and cancer risk and mortality: state of the science, gaps, and challenges. Epidemiol Rev (2017) 39:28–48. doi:10.1093/epirev/mxx005
62. Kim Y, Je Y. Vitamin D intake, blood 25(OH)D levels, and breast cancer risk or mortality: a meta-analysis. Br J Cancer (2014) 110:2772–84. doi:10.1038/bjc.2014.175
63. Gnagnarella P, Pasquali E, Serrano D, Raimondi S, Disalvatore D, Gandini S. Vitamin D receptor polymorphism FokI and cancer risk: a comprehensive meta-analysis. Carcinogenesis (2014) 35:1913–9. doi:10.1093/carcin/bgu150
64. Mun MJ, Kim TH, Hwang JY, Jang WC. Vitamin D receptor gene polymorphisms and the risk for female reproductive cancers: a meta-analysis. Maturitas (2015) 81:256–65. doi:10.1016/j.maturitas.2015.03.010
65. Bhattacharjee V, Zhou Y, Yen TJ. A synthetic lethal screen identifies the vitamin D receptor as a novel gemcitabine sensitizer in pancreatic cancer cells. Cell Cycle (2014) 13:3839–56. doi:10.4161/15384101.2014.967070
Keywords: pancreatic cancer, vitamin D, in vivo, pancreatic acinar carcinoma, magnetic resonance imaging
Citation: Dooley J, Lagou V, Heirman N, Dresselaers T, Himmelreich U and Liston A (2017) Murine Pancreatic Acinar Cell Carcinoma Growth Kinetics Are Independent of Dietary Vitamin D Deficiency or Supplementation. Front. Oncol. 7:133. doi: 10.3389/fonc.2017.00133
Received: 29 April 2017; Accepted: 07 June 2017;
Published: 28 June 2017
Edited by:
Michael J. Wargovich, University of Texas Health Science Center San Antonio, United StatesReviewed by:
Angela Hague, University of Bristol, United KingdomAndrzej Ptasznik, University of Pennsylvania, United States
Copyright: © 2017 Dooley, Lagou, Heirman, Dresselaers, Himmelreich and Liston. This is an open-access article distributed under the terms of the Creative Commons Attribution License (CC BY). The use, distribution or reproduction in other forums is permitted, provided the original author(s) or licensor are credited and that the original publication in this journal is cited, in accordance with accepted academic practice. No use, distribution or reproduction is permitted which does not comply with these terms.
*Correspondence: Adrian Liston, YWRyaWFuLmxpc3RvbkB2aWIta3VsZXV2ZW4uYmU=