- 1Department of Neurosurgery, JSC “National Center for Neurosurgery”, Nur-Sultan, Kazakhstan
- 2Department of Neurosurgery and Traumatology, West Kazakhstan Marat Ospanov State Medical University, Aktobe, Kazakhstan
- 3University of Arizona College of Medicine, Phoenix, AZ, United States
- 4Department of Neurosurgery and Innovative Medicine, Irkutsk State Medical University, Irkutsk, Russia
Stereotactic brain needle biopsies are indicated for deep-seated or multiple brain lesions and for patients with poor prognosis in whom the risks of resection outweigh the potential outcome benefits. The main goal of such procedures is not to improve the resection extent but to safely acquire viable tissue representative of the lesion for further comprehensive histological, immunohistochemical, and molecular analyses. Herein, we review advanced optical techniques for improvement of safety and efficacy of stereotactic needle biopsy procedures. These technologies are aimed at three main areas of improvement: (1) avoidance of vessel injury, (2) guidance for biopsy acquisition of the viable diagnostic tissue, and (3) methods for rapid intraoperative assessment of stereotactic biopsy specimens. The recent technological developments in stereotactic biopsy probe design include the incorporation of fluorescence imaging, spectroscopy, and label-free imaging techniques. The future advancements of stereotactic biopsy procedures in neuro-oncology include the incorporation of optical probes for real-time vessel detection along and around the biopsy needle trajectory and in vivo confirmation of the diagnostic tumor tissue prior to sample acquisition.
Introduction
Brain needle biopsies are indicated for deep-seated or multiple brain lesions and for patients with poor prognosis in whom the risks of resection outweigh the potential outcome benefits. A recent systematic review and evidence-based clinical practice guideline investigating the role of stereotactic brain biopsy for low-grade gliomas provided level III evidence in support of brain biopsies and recommended that surgeons consider using advanced imaging techniques to improve diagnostic accuracy (1). In suspected low-grade tumors that are not considered for resection, biopsy location should be planned based on molecular guidance techniques, such as positron emission tomography, magnetic resonance (MR) spectroscopy, or others in order to provide a reliable molecular diagnosis (2).
The current standard-of-care method for stereotactic brain needle biopsy involves a 1.6- to 2-mm-diameter needle cannula insertion through a cranial burr-hole aligned to a predetermined trajectory. The two cannulas have overlapping side windows. When the desired position is reached, these windows are aligned, and brain tissue is lodged into cannula using suction and cut by sliding the inner cannula.
Despite the minimally invasive nature of the needle brain biopsy, the limitations and risks are still present and include the following:
1. Non-diagnostic biopsy yield. The frequency of non-diagnostic biopsies ranges in various studies
◦ Not-specified non-diagnostic biopsy rate−5.2% (3), 9% (4), 10.7% (5), 13% (6);
◦ Biopsy performed without intraoperative frozen section-−11%;
◦ Biopsy performed with frozen section on demand−1% (7);
2. Technical failure rate is 2.4–3.7% (7)
3. Complications rate:
◦ Overall complications rate−1.2% (3), 7.36% (4);
◦ Perioperative−6.3–4.8% (7);
◦ Postoperative−10.5–4.8% (7);
◦ Hemorrhagic/vessel injury complications: 2.1% (6), 3% (7), 4.35% (4), overall 8.8%, including 1% symptomatic, or >1 cm (8). One study reported the overall rate of hemorrhages on postoperative computerized tomography (CT) as 59.8%, including 41.1% of bleeds <5 mm in diameter and 8.9% of bleeds 3–4 cm in diameter (9).
4. Mortality rate was 0.6% (3), 1.34% (4), and 0.6–3.7% (7) in various studies.
Vessel injury is one of the most feared complications during minimally invasive stereotactic procedures. The rounded tip design of the biopsy needle intends to push aside any vessel encountered along the biopsy needle trajectory. However, such a design does not completely eliminate the potential for vessel injury during the forward movement of the needle. Moreover, the risk of vessel injury is believed to be higher during the side-cutting movement during biopsy acquisition. Therefore, apart from techniques for navigation on the anatomical level, like MR spectroscopy (10, 11), perfusion (12), and metabolism (13), improvements of intraoperative tools for biopsy acquisition and rapid assessment would be beneficial.
This paper aims to provide a concise review of novel fluorescence-based and other optical techniques for improvement of safety and efficacy of minimally invasive stereotactic needle biopsy procedures in neuro-oncology. The main goal of such procedures is not to improve the resection extent but to safely acquire viable tissue representative of the lesion for further comprehensive histological, immunohistochemical, and molecular analyses. Here, we do omit discussion of MR, computed tomography, and ultrasound Doppler (14, 15)-based navigation techniques. Although the potential value of these techniques is undeniable, we focus this review on recently proposed optical methods. The basics of optical techniques are found in recently published reviews of this subject that describe the basics of fluorescence guidance in neuro-oncology (16–21).
Methods
We performed a literature search in the PubMed database using the terms “biopsy,” “stereotactic,” “fluorescence,” “Raman,” “spectroscopy,” “brain,” “optical,” and “optical probe” in various combinations. Our search included papers published up to November 2018. We did not set a lower bound for this search. The titles were scanned and relevant articles were selected for full-text review, resulting in relevant articles for analysis as presented in the Supplementary Data 1. Additional articles were added from reference lists if deemed relevant. After reviewing the articles, the three key areas for analysis and discussion were selected: (1) avoidance of vessel injury during stereotactic biopsies, (2) probe-based guidance methods for biopsy acquisition, and (3) methods for rapid intraoperative assessment of stereotactic biopsy specimens. The latter two areas were united in one section for discussion due to the similarity in the used optical principles toward the common goal for differentiation of tumor and normal tissues, which can be done in vivo (using the miniaturized probes) or ex vivo after the biopsy has been acquired (using either miniaturized or benchtop systems).
Results and Discussion
Avoidance of Vessel Injury During Stereotactic Biopsy (Table 1)
Intravascular Contrast Detection
Göbel et al. described a small contact forward-viewing endoscopic probe that can fit into a standard biopsy needle and visualize fluorescence signals of protoporphyrin IX (PpIX) and indocyanine green (ICG) (Figure 1A) (22). PpIX is a fluorophore (excitation maximum wavelength is 405 nm; emission maximum, 630 nm) commonly used as the basis of fluorescent-guided neuro-oncology (31), while ICG is a near-infrared fluorophore (excitation maximum wavelength is 780–800 nm; emission maximum, 830 nm) commonly used for vascular flow visualization (32). The multifiber probe includes two excitation diode lasers (405 and 785 nm for PpIX autofluorescence and ICG, respectively) and a charge-coupled device camera for signal detection. This endoscope allowed ICG detection through the brain tissue (about 1 mm thickness) on a phantom model. It also showed reliable detection of a red PpIX fluorescence from the tumor in a mouse glioma model. Subsequently, in a pilot clinical trial (n = 1), this needle endoscope was used instead of the standard brain biopsy needle mandarin to visualize fluorescence during probe advancement. The actual biopsy acquisition was performed with a small forward biting biopsy forceps. Although bright PpIX fluorescence from the viable tumor core was visible, no fluorescence in the surrounding needle positions was evident. For vessel visualization, a 200 mg/kg dose of ICG was used in the mouse model. With this, near-infrared ICG fluorescence appeared inside the blood vessels at an excitation of 785 nm (Figure 1A, top panel). With autofluorescence at 405-nm excitation, the vessels appear clearer and darker (Figure 1A, middle panel). With a combination of autofluorescence and ICG visualization simultaneously, the vessels also display clear visualization (Figure 1A, bottom panel). Vessel visualization in a human tissue was not performed.
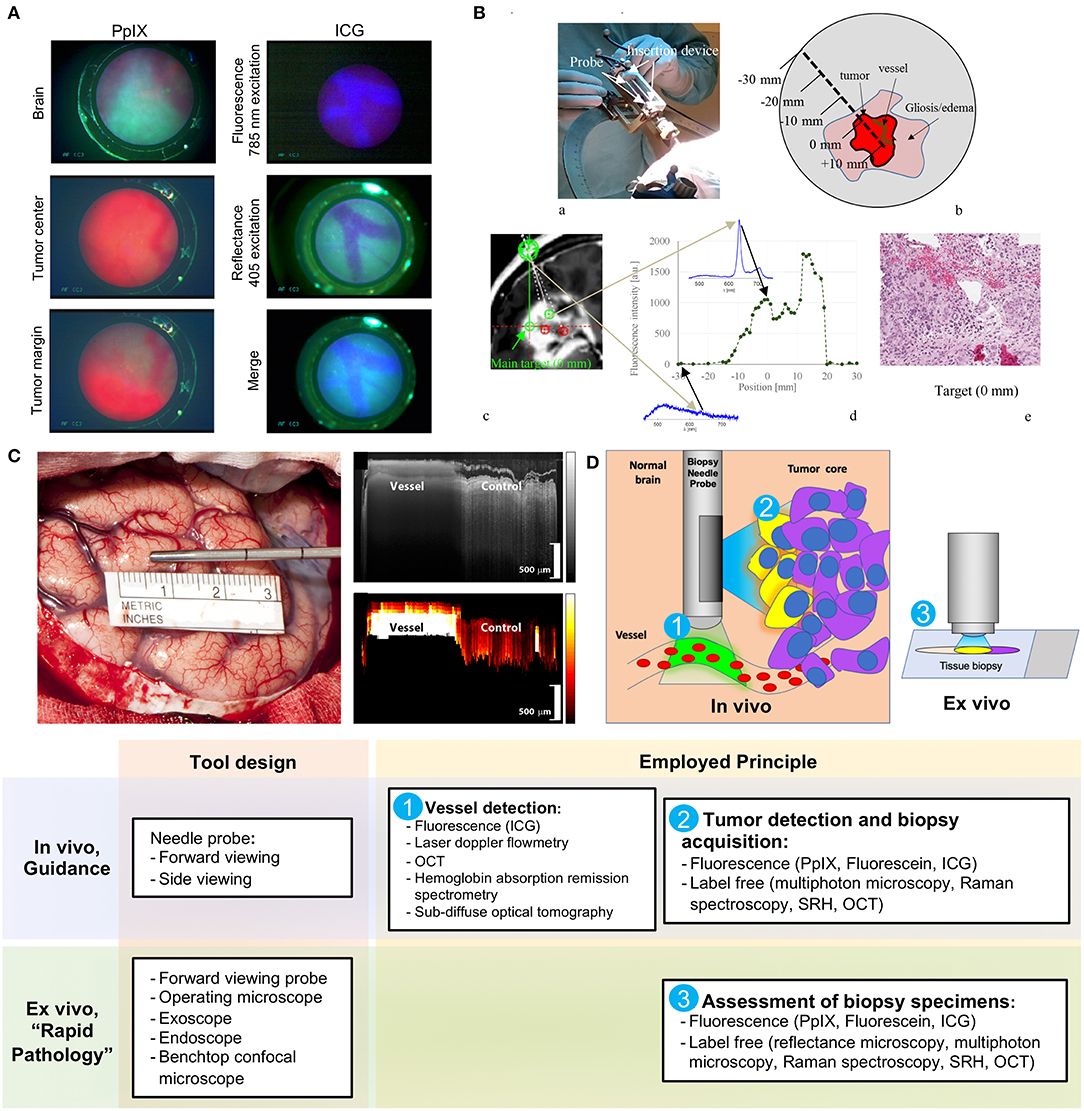
Figure 1. Examples of the optical technologies for brain needle biopsies. (A) Images from the fluorescence optical needle endoscope described by Göbel et al. (22) for PpIX visualization in the tumor (left column) and vessel visualization using ICG (right column) in a mouse model. Adapted with permission from Göbel et al. (22)© The Optical Society. (B) Illustration of PpIX spectroscopy method for tumor detection during stereotactic biopsy described by Haj-Hosseini et al. (28). The top two panels show the probe positioned in the stereotactic frame and the concept of measurements along the trajectory. The bottom panels show an MR image with calculated targets, spectral data of PpIX along the injection trajectory, and the histopathology slide of the target. Adapted with permission from Haj-Hosseini et al. (28)© The Optical Society. (C) Stain-less reflectance imaging method from Ramakonar et al. (27). Left panel shows a photo of an imaging needle rolled over a vessel of 650 μm. The imaging window of the probe is not visible and is facing toward the tissue. The upper right panel displays OCT B-scan consisting of A-scans. The tissue surface corresponds to the top of the image. Depth increases going down the image. The bottom right panel displays a speckle decorrelation image calculated form the OCT scan with high decorrelation as white and low decorrelation as dark red. Adapted from Ramakonar et al. (27) under Creative Commons Attribution license. (D) Schematic summary of advanced optical methods and tool designs, for increasing safety of stereotactic brain biopsies. OCT, optical coherence tomography; PpIX, protoporphyrin IX; ICG, indocyanine green; SRH, Stimulated Raman Histology.
Ruhm et al. investigated forward-viewing microfiber probe for ICG detection using a computer simulation of ICG fluorescence excited and detected through the same fiber-optic system in homogeneous human brain tissue (23). Although detection of intravascular ICG is widely used in open vascular neurosurgery (33), its application for detection of deep vessels might not be optimal due to the fast redistribution and ICG fluorescence decay. Leakage of blood or trace amounts of blood containing ICG in the vicinity of the probe would result in false-positive measurements. Furthermore, the dosage and timing of ICG administration would need to be optimized, and the necessity for additional drug injection should be considered, especially when comparing to other methods for vessel detection.
Laser Doppler Flowmetry
Another recently reported tool is a 2.2-mm-diameter forward-viewing needle probe that combines fluorescence spectral detection and laser Doppler flowmetry (28). The probe was designed to fit the Leksell® Stereotactic System. By measuring the frequency shift in the 780-nm backscattered laser light caused by the cell movements in the capillaries, the device assesses brain perfusion and blood flow. The pilot study on three patients demonstrated reliable detection of PpIX spectra (405 excitation laser) along the trajectory of the device (Figure 1B). Although increased perfusion was detected in two biopsy locations, no information regarding the changes in the surgical plan or needle trajectory was reported (28). A similar stand-alone forward-viewing laser Doppler flowmetry needle probe had been investigated by the same group during the electrode placement for deep brain stimulation in patients (29, 30). Although the main goal of these studies was to establish the link between the measured blood flow and anatomy along trajectories, the authors did encounter one bleeding episode evidenced by significantly increased blood flow measures, which was later confirmed by CT (29). Subsequently, the authors suggested that this method could detect small vessels and thus decrease the risk of bleeding complications in stereotactic procedures (30). Obtaining measurements every 0.5 mm along the injection trajectory was proposed; however, it would result in a significant increase of surgical time (20 s per measurement resulting in at least 33.3 min for 5-cm trajectory) (28). Additionally, this probe has not been integrated into a brain biopsy needle for side view assessment. Future studies are necessary to assess if vascular imaging is helpful to guide intraoperative adjustments of the surgical plan and prevent vessel injury and bleeding.
Stain-Less Reflectance Imaging Approaches
Ramakonar et al. reported the performance of a side-viewing probe that fit a standard side-cutting brain biopsy needle for optical coherence tomography (OCT) imaging and differentiation of solid tissue and vessels (Figure 1C) (27). This imaging method is based on the detection and reconstruction of backscattered light from the 1,300-nm near-infrared illumination light, allowing 1–1.5 mm penetration depth and 5–20 μm resolution. OCT imaging was presented in an earlier paper by Kut et al. that demonstrated the modality's efficacy as a label-free technique for differentiating cancer from non-cancer in human brain tissues at a 1- to 1.5-mm penetration depth (34). To overcome the challenge of OCT's shallow depth, Ramakonar et al. was able to develop an optically guided biopsy needle capable of OCT imaging in order to visualize blood vessels at greater tissue depths. The information is then displayed on a monitor as a B-scan acquired during needle movements. Initial analysis of surface cortical vessels in patients that underwent craniotomy showed that the device detected blood vessels with a diameter of >500 μm with a sensitivity of 91.2% and a specificity of 97.7% (27). Furthermore, the authors validated these findings by demonstrating deep brain vessel detection capability (vessels were preselected on MRI) during brain needle biopsies in three patients (27).
Markwardt et al. in a phantom model and in ex vivo porcine brains demonstrated that a side-viewing double fiber probe for remission spectrometry inserted in a side-cutting biopsy needle can determine the proximity of the vessel to the needle (26). The method is based on the illumination of the tissue with a light from a broad wavelength light emitting diode (LED) light source (400–700 nm) and spectroscopic analysis of the remitted light. The remitted light is assessed at wavelengths that are characteristic of hemoglobin absorption (578 and 650 nm for oxygenated and deoxygenated, respectively), allowing the estimation of the proximity of the vessels to the probe. The method results are displayed as ratio values that represent the proximity, size, and orientation of the hemoglobin-containing vessels to the probe. Although there is no visual information regarding the appearance of the tissue, the technique is relatively simple and inexpensive.
Pichette et al. in phantom experiments demonstrated that interstitial sub-diffuse optical tomography technology can detect vessels with diameters of more than 300 μm for up to 2 mm from the biopsy needle (24). The 1.7-mm-diameter probe consists of 24 side-viewing fibers that provide circumferential scanning and detection of the spectrally resolved remitted light from the tissue around the needle guide. The main advantage of this method is that the probe scans the whole volume of tissues surrounding the needle, creating a two-dimensional visual signal map across the depth and circumference position. An additional advantage is higher tissue penetration depth when compared to optical coherence tomography (2 vs. ~1 mm) (24). Remission spectrometry and sub-diffuse optical tomography both rely on the hemoglobin absorption spectra and do not require additional contrast agents (24, 26).
Methods for Biopsy Acquisition Guidance and for Rapid Intraoperative Assessment of Obtained Specimens
Identification of Viable Diagnostic Tissue
Decreasing the number of stereotactic biopsies represents another strategy to minimize the risk of brain and vascular damage. Therefore, technologies that allow for the detection of viable tumor tissue along the biopsy needle trajectory are valuable. Such techniques allow not only to increase accuracy for diagnostic sample acquisition for proper histopathological diagnostics but also to avoid unnecessary repetitive needle biopsies.
Methods Based on the Detection of Molecular Labels in the Tumor Tissue
The presence of 5-aminolevulinic (5-ALA)-induced PpIX fluorescence is diagnostic for malignant tumors and can be used to assess stereotactic biopsy samples before submitting them to pathology, while the absence of fluorescence can filter out necrotic areas and inflammatory reactive tissue without malignant cells. This method was confirmed in multiple studies (35–40). Detection of PpIX fluorescence was highly diagnostic for viable tumor tissue in patients with intracranial lymphomas (41), high-grade gliomas, and anaplastic foci in patients with low-grade gliomas (42). Fluorescence positive samples might even not require intraoperative frozen section analysis and could be sent directly for a permanent section because of the high positive predictive value of PpIX fluorescence (39, 43, 44). In cases of low or negative fluorescence, the intraoperative frozen section analysis is recommended with subsequent biopsies upon the results of the intraoperative histopathological analysis (43). Excitation of PpIX at 633 nm can further increase the diagnostic utility of such a method, as it allows for deeper imaging through a small layer of blood or tissue, which was previously impossible with 405-nm excitation (45, 46).
Similarly to 5-ALA, fluorescein sodium can be used to assess stereotactic biopsy specimens under the special fluorescence mode of the surgical microscope. High-grade glioma tissue shows strong yellow fluorescence when excited at about 488 nm (47). Being a relatively inexpensive drug, fluorescein sodium could also be visualized using a low-cost miniature device, Fluoropen, which is essentially a LED torch with a blue filter mounted at the light source for excitation and a yellow filter attached around the torch as a cone collar (48). The device is positioned close to the specimen and yellow fluorescence is observed through the yellow filter (48). Observation of fluorescein fluorescence from the specimens had a 100% positive predictive value and a 25% negative predictive value of a lesional tissue (49). Overall, the fluorescein-based method was shown to be as effective as frozen section analysis for the diagnostic biopsy screening (49).
ICG may also be used as a highlighter of the tumor tissue (50–54). Although there are few reports about the ICG use for tumor detection during stereotactic biopsies (16), open surgical visualization showed high sensitivity but low specificity of the second-window ICG (52). Other targeted molecular labels that can be used for open fluorescence-guided surgery, for example, BLZ-100, an ICG-conjugated tumor-targeting peptide chlorotoxin used for imaging, also hold potential for stereotactic needle biopsy procedures (55).
Apart from the macroscopic identification of the retained fluorescent drug, the obtained stereotactic biopsy specimens could be subjected to analysis with other methods alternative to a frozen section. Such methods might be less laborious and time-consuming. Stereotactic samples could be stained with rapid fluorophores ex vivo and scanned with a confocal microscope (56, 57). Miniaturized handheld confocal laser endomicroscopy with fluorescein sodium injected intraoperatively as a contrast can visualize the histological architecture of brain biopsies ex vivo and in vivo (58–62). However, this probe has not been assessed in stereotactic procedures.
A thin forward-viewing fiber-based confocal laser endomicroscope that can fit biopsy needles is available for 488-nm and 660-nm excitable fluorophores (fluorescein sodium and ICG, respectively) (63, 64). A pilot study has demonstrated that such an endomicroscope with fluorescein as a contrast can visualize brain tumor architecture during a stereotactic brain biopsy procedure in humans (65). A similar technique was tested by Lynagh et al. in a proof-of-concept study for the detection of fluorescein and blue-fluorescence protein labeled glioma cells in a rat model using a 0.65-mm fiber microendoscope coupled with a clinical stereotactic biopsy needle (66). Microendoscopy technology is similar to a needle-based contact endoscope (22) and other fiber probes for spectral measurements that were discussed above (28, 35, 44), but is able to provide fluorescence tissue image with higher resolution down to the cellular level.
Label-Free Methods
Various microscopy imaging methods that are not dependent on the drug-induced contrast and instead rely on intrinsic optical properties of the tissues recently received clinical attention. Such methods could be used for rapid intraoperative assessment of the acquired stereotactic brain biopsies to increase diagnostic yield and improve pathology workflow.
It has been shown that measurements of reflectance and fluorescence spectra of unstained brain tissues can differentiate radiation necrosis in brain tumor tissue (67). Reflectance confocal microscopy can differentiate viable brain tumor tissue from necrotic tissue and further characterize histological appearance with high diagnostic accuracy (68, 69). Label-free multiphoton microscopy methods were successfully used for the imaging of Alzheimer's disease brain samples (70). Raman microspectroscopic microscopy imaging has been studied for histological assessment of brain tumor biopsies (71). A notably successful variant of Raman microscopy is the Stimulated Raman Histology (SRH) technique, which has been used for ex vivo histological assessment of brain tumor biopsies. SRH provided high-resolution digital images that look similar to the standard hematoxylin and eosin staining (72, 73). SRH is a stand-alone imaging system that can be positioned in the operating room for rapid intraoperative pathological assessment (72, 73). Although most of the imaging studies were performed on benchtop microscopes, some multiphoton microscopy modes (CARS and TREF) will be available in endoscopes and needle-size probes in the near future (74).
Stevens et al. described a 1.8-mm-diameter forward-viewing 830-nm Raman-based spectroscopy probe without fibers that fit a standard brain biopsy needle (75, 76). The new design of the probe resulted in significant noise reduction compared to the silica-fiber-based Raman imaging and demonstrated discrimination of various porcine brain structures including white matter, gray matter, and blood vessels based on the Raman spectra (75).
Summary
Current developments for increasing the safety and efficacy of stereotactic brain biopsy procedures are centered around the two main areas: avoidance of the vessels and detection of the viable diagnostic tissue, which could be achieved in vivo or ex vivo (Figure 1D).
Because vessel avoidance is a major component for the safety of any stereotactic needle-based procedure including needle brain biopsy, deep brain stimulation (77), or interstitial laser thermal therapy (78, 79), advancements in the surgical tools that allow for timely vessel detection is of utmost importance. Several technologies for vessel detection implemented into a biopsy needle included detection of fluorescent intravascular contrast, laser Doppler flowmetry, optical coherence and interstitial sub-diffuse tomography, and remission spectrometry. Non-fluorescence methods based on the blood flow and hemoglobin detection are thought to be the most promising as they avoid dealing with fluctuations in the intravascular contrast concentrations after injection. It should be noted that the current literature does not provide definitive evidence on the efficacy of such methods for vessel injury avoidance; therefore, more clinical studies are necessary.
Ex vivo confirmation of PpIX or fluorescein fluorescence from biopsy samples has been established to have a high positive predictive value of a diagnostic biopsy in multiple studies and can be recommended as a routine method for stereotactic biopsy at this current stage. However, the possibility for identification of the correct biopsy needle position in vivo prior to actual biopsy acquisition with a small optical tool is even more exciting. Most of such reports are based on the detection of the intra-tumoral fluorescent molecules (PpIX, fluorescein, and ICG); however, the development of reflectance and multiphoton microscopy techniques and militarization of microscopes would allow label-free tumor tissue identification through a biopsy needle in the future. There is a marked diversity in qualitative and quantitative optical methods for brain tumor tissue identification, and each of them requires a significant learning curve and dedication. So far, the most progress has been achieved with 5-ALA-induced PpIX, which is highly specific for malignant tissues and can be detected spectroscopically with a single optical fiber inserted in the biopsy needle.
Another limitation is that most of the discussed methods require timely interpretation and adjustments of multiple parameters during the procedure to ensure optimal performance, which is not always practical and may lead to information overload. For example, results of quantitative spectroscopy are usually displayed on the screen in a graph form. This information could be converted into an auditory format, which would be easy to understand and non-disruptive during the surgery (80). Finally, the possibility of light-induced tissue damage should be carefully considered when developing novel optical and fluorescent tools for in vivo diagnostics.
When considering the design of an ideal probe, it should incorporate vessel detection as well as tumor detection modules, most likely in forward and side views simultaneously for the safest possible probe insertion and biopsy acquisition. Machine learning, coupled with optical visualization technologies, would be a basis for computer-aided, real-time tissue image analysis for the selection of the best probe trajectory or location for biopsy acquisition. Besides these technologies that are used for vessel avoidance and tumor detection, future development of probes could also include augmented or virtual reality for clinicians to perform more accurate probe trajectory planning.
Author Contributions
All authors listed have made a substantial, direct and intellectual contribution to the work, and approved it for publication.
Conflict of Interest
The authors declare that the research was conducted in the absence of any commercial or financial relationships that could be construed as a potential conflict of interest.
Supplementary Material
The Supplementary Material for this article can be found online at: https://www.frontiersin.org/articles/10.3389/fonc.2019.00947/full#supplementary-material
Abbreviations
PpIX, protoporphyrin IX; ICG, indocyanine green.
References
1. Ragel BT, Ryken TC, Kalkanis SN, Ziu M, Cahill D, Olson JJ. The role of biopsy in the management of patients with presumed diffuse low grade glioma. J Neuro Oncol. (2015) 125:481–501. doi: 10.1007/s11060-015-1866-2
2. Schwartz C, Kreth FW. Comment on: the role of biopsy in the management of patients with presumed diffuse low grade glioma: a systematic review and evidence-based clinical practice guideline. J Neuro Oncol. (2016) 128:173. doi: 10.1007/s11060-016-2086-0
3. Teixeira MJ, Fonoff ET, Mandel M, Alves HL, Rosemberg S. Stereotactic biopsies of brain lesions. Arq Neuropsiquiatr. (2009) 67:74–7. doi: 10.1590/S0004-282X2009000100018
4. Chen CC, Hsu PW, Erich Wu TW, Lee ST, Chang CN, Wei KC, et al. Stereotactic brain biopsy: Single center retrospective analysis of complications. Clin Neurol Neurosurg. (2009) 111:835–9. doi: 10.1016/j.clineuro.2009.08.013
5. Shastri-Hurst N, Tsegaye M, Robson DK, Lowe JS, Macarthur DC. Stereotactic brain biopsy: an audit of sampling reliability in a clinical case series. Br J Neurosurg. (2009) 20:222–6. doi: 10.1080/02688690600875507
6. Bander ED, Jones SH, Pisapia D, Magge R, Fine H, Schwartz TH, et al. Tubular brain tumor biopsy improves diagnostic yield for subcortical lesions. J Neurooncol. (2019) 141:121–9. doi: 10.1007/s11060-018-03014-w
7. Dammers R, Schouten JW, Haitsma IK, Vincent AJ, Kros JM, Dirven CM. Towards improving the safety and diagnostic yield of stereotactic biopsy in a single centre. Acta Neurochir. (2010) 152:1915–21. doi: 10.1007/s00701-010-0752-0
8. Frati A, Pichierri A, Bastianello S, Raco A, Santoro A, Esposito V, et al. Frameless stereotactic cerebral biopsy: our experience in 296 cases. Stereotact Funct Neurosurg. (2011) 89:234–45. doi: 10.1159/000325704
9. Kulkarni AV, Guha A, Lozano A, Bernstein M. Incidence of silent hemorrhage and delayed deterioration after stereotactic brain biopsy. J Neurosurg. (1998) 89:31–5. doi: 10.3171/jns.1998.89.1.0031
10. Preul MC, Caramanos Z, Collins DL, Villemure JG, Leblanc R, Olivier A, et al. Accurate, noninvasive diagnosis of human brain tumors by using proton magnetic resonance spectroscopy. Nat Med. (1996) 2:323–5. doi: 10.1038/nm0396-323
11. Chernov MF, Muragaki Y, Ochiai T, Taira T, Ono Y, Usukura M, et al. Spectroscopy-supported frame-based image-guided stereotactic biopsy of parenchymal brain lesions: comparative evaluation of diagnostic yield and diagnostic accuracy. Clin Neurol Neurosurg. (2009) 111:527–35. doi: 10.1016/j.clineuro.2009.03.006
12. Lefranc M, Monet P, Desenclos C, Peltier J, Fichten A, Toussaint P, et al. Perfusion MRI as a neurosurgical tool for improved targeting in stereotactic tumor biopsies. Stereotact Funct Neurosurg. (2012) 90:240–7. doi: 10.1159/000338092
13. Levivier M, Goldman S, Pirotte B, Brucher JM, Baleriaux D, Luxen A, et al. Diagnostic yield of stereotactic brain biopsy guided by positron emission tomography with [18F]fluorodeoxyglucose. J Neurosurg. (1995) 82:445–52. doi: 10.3171/jns.1995.82.3.0445
14. Gilsbach J, Mohadjer M, Mundinger F. A new safety device to prevent bleeding complications during stereotactic biopsy—The “stereotactic” Doppler sonography. Acta Neurochir. (1987) 89:77–9. doi: 10.1007/BF01406671
15. Virdyawan V, Y Baena FR. Vessel pose estimation for obstacle avoidance in needle steering surgery using multiple forward looking sensors. In: 2018 IEEE/RSJ International Conference on Intelligent Robots and Systems (IROS). (2018). p. 3845–52. doi: 10.1109/IROS.2018.8594198
16. Cho SS, Salinas R, Lee JYK. Indocyanine-green for fluorescence-guided surgery of brain tumors: evidence, techniques, and practical experience. Front Surg. (2019) 6:11. doi: 10.3389/fsurg.2019.00011
17. Zhang DY, Singhal S, Lee JYK. Optical principles of fluorescence-guided brain tumor surgery: a practical primer for the neurosurgeon. Neurosurgery. (2019) 85:312–4. doi: 10.1093/neuros/nyy315
18. Wei L, Roberts DW, Sanai N, Liu JTC. Visualization technologies for 5-ALA-based fluorescence-guided surgeries. J Neurooncol. (2019) 141:495–505. doi: 10.1007/s11060-018-03077-9
19. Hadjipanayis CG, Stummer W. 5-ALA and FDA approval for glioma surgery. J Neurooncol. (2019) 141:479–86. doi: 10.1007/s11060-019-03098-y
20. Valdes PA, Juvekar P, Agar NYR, Gioux S, Golby AJ. Quantitative wide-field imaging techniques for fluorescence guided neurosurgery. Front Surg. (2019) 6:31. doi: 10.3389/fsurg.2019.00031
21. Lakomkin N, Hadjipanayis CG. The use of spectroscopy handheld tools in brain tumor surgery: current evidence and techniques. Front Surg. (2019) 6:30. doi: 10.3389/fsurg.2019.00030
22. Göbel W, Brucker D, Kienast Y, Johansson A, Kniebühler G, Rühm A, et al. Optical needle endoscope for safe and precise stereotactically guided biopsy sampling in neurosurgery. Optics Exp. (2012) 20:26117–26. doi: 10.1364/OE.20.026117
23. Ruhm A, Gobel W, Sroka R, Stepp H. ICG-assisted blood vessel detection during stereotactic neurosurgery: simulation study on excitation power limitations due to thermal effects in human brain tissue. Photodiagnosis Photodyn Ther. (2014) 11:307–18. doi: 10.1016/j.pdpdt.2014.03.007
24. Pichette J, Goyette A, Picot F, Tremblay M-A, Soulez G, Wilson BC, et al. Sensitivity analysis aimed at blood vessels detection using interstitial optical tomography during brain needle biopsy procedures. Biomed Optics Exp. (2015) 6:4238–54. doi: 10.1364/BOE.6.004238
25. Goyette A, Pichette J, Tremblay MA, Laurence A, Jermyn M, Mok K, et al. Sub-diffuse interstitial optical tomography to improve the safety of brain needle biopsies: a proof-of-concept study. Optics Lett. (2015) 40:170–3. doi: 10.1364/OL.40.000170
26. Markwardt NA, Stepp H, Franz G, Sroka R, Goetz M, Zelenkov P, et al. Remission spectrometry for blood vessel detection during stereotactic biopsy of brain tumors. J Biophoton. (2017) 10:1080–94. doi: 10.1002/jbio.201600193
27. Ramakonar H, Quirk BC, Kirk RW, Li J, Jacques A, Lind CRP, et al. Intraoperative detection of blood vessels with an imaging needle during neurosurgery in humans. Sci Adv. (2018) 4:eaav4992. doi: 10.1126/sciadv.aav4992
28. Haj-Hosseini N, Richter JCO, Milos P, Hallbeck M, Wårdell K. 5-ALA fluorescence and laser doppler flowmetry for guidance in a stereotactic brain tumor biopsy. Biomed Optics Exp. (2018) 9:2284–96. doi: 10.1364/BOE.9.002284
29. Wårdell K, Zsigmond P, Richter J, Hemm S. Relationship between laser doppler signals and anatomy during deep brain stimulation electrode implantation toward the ventral intermediate nucleus and subthalamic nucleus. Oper Neurosurg. (2013) 72:ons127–ons40. doi: 10.1227/NEU.0b013e31827e5821
30. Wårdell K, Hemm-Ode S, Rejmstad P, Zsigmond P. High-resolution laser Doppler measurements of microcirculation in the deep brain structures: a method for potential vessel tracking. Stereotact Funct Neurosurg. (2016) 94:1–9. doi: 10.1159/000442894
31. Stummer W, Pichlmeier U, Meinel T, Wiestler OD, Zanella F, Reulen HJ, et al. Fluorescence-guided surgery with 5-aminolevulinic acid for resection of malignant glioma: a randomised controlled multicentre phase III trial. Lancet Oncol. (2006) 7:392–401. doi: 10.1016/S1470-2045(06)70665-9
32. Hanel RA, Nakaji P, Spetzler RF. Use of microscope-integrated near-infrared indocyanine green videoangiography in the surgical treatment of spinal dural arteriovenous fistulae. Neurosurgery. (2010) 66:978–84. discussion: 84–5. doi: 10.1227/01.NEU.0000368108.94233.22
33. Raabe A, Beck J, Gerlach R, Zimmermann M, Seifert V. Near-infrared Indocyanine green video angiography: a new method for intraoperative assessment of vascular flow. Neurosurgery. (2003) 32–9. discussion: 139. doi: 10.1097/00006123-200301000-00017
34. Kut C, Chaichana KL, Xi J, Raza SM, Ye X, McVeigh ER, et al. Detection of human brain cancer infiltration ex vivo and in vivo using quantitative optical coherence tomography. Sci Transl Med. (2015) 7:292ra100. doi: 10.1126/scitranslmed.3010611
35. Haj-Hosseini N, Richter JCO, Hallbeck M, Wårdell K. Low dose 5-aminolevulinic acid: Implications in spectroscopic measurements during brain tumor surgery. Photodiagnosis Photodyn Ther. (2015) 12:209–14. doi: 10.1016/j.pdpdt.2015.03.004
36. Piquer J, Llácer JL, Rovira V, Riesgo P, Rodriguez R, Cremades A. Fluorescence-guided surgery and biopsy in gliomas with an exoscope system. BioMed Res Int. (2014) 2014:1–6. doi: 10.1155/2014/207974
37. Hefti M, von Campe G, Moschopulos M, Siegner A, Looser H, Landolt H. 5-Aminolevulinic acid induced protoporphyrin IX fluorescence in high-grade glioma surgery: a one-year experience at a single institutuion. Swiss Med Wkly. (2008) 138:180–5. doi: 10.4414/smw.2008.12077
38. Moriuchi S, Yamada K, Dehara M, Teramoto Y, Soda T, Imakita M, et al. Use of 5-aminolevulinic acid for the confirmation of deep-seated brain tumors during stereotactic biopsy. Report of 2 cases. J Neurosurg. (2011) 115:278–80. doi: 10.3171/2011.4.JNS102137
39. von Campe G, Moschopulos M, Hefti M. 5-Aminolevulinic acid-induced protoporphyrin IX fluorescence as immediate intraoperative indicator to improve the safety of malignant or high-grade brain tumor diagnosis in frameless stereotactic biopsies. Acta Neurochir. (2012) 154:585–8. doi: 10.1007/s00701-012-1290-8
40. Yamaguchi F, Takahashi H, Teramoto A. Photodiagnosis for frameless stereotactic biopsy of brain tumor. Photodiagnosis Photodyn Ther. (2007) 4:71–5. doi: 10.1016/j.pdpdt.2006.09.005
41. Kiesel B, Millesi M, Woehrer A, Furtner J, Bavand A, Roetzer T, et al. 5-ALA–induced fluorescence as a marker for diagnostic tissue in stereotactic biopsies of intracranial lymphomas: experience in 41 patients. Neurosurg Focus. (2018) 44:E7. doi: 10.3171/2018.3.FOCUS1859
42. Arita H, Kinoshita M, Kagawa N, Fujimoto Y, Kishima H, Hashimoto N, et al. 11C-methionine uptake and intraoperative 5-aminolevulinic acid-induced fluorescence as separate index markers of cell density in glioma. Cancer. (2012) 118:1619–27. doi: 10.1002/cncr.26445
43. Widhalm G, Minchev G, Woehrer A, Preusser M, Kiesel B, Furtner J, et al. Strong 5-aminolevulinic acid-induced fluorescence is a novel intraoperative marker for representative tissue samples in stereotactic brain tumor biopsies. Neurosurg Rev. (2012) 35:381–91. doi: 10.1007/s10143-012-0374-5
44. Potapov AA, Goryaynov SA, Okhlopkov VA, Pitskhelauri DI, Kobyakov GL, Zhukov VY, et al. [Clinical guidelines for the use of intraoperative fluorescence diagnosis in brain tumor surgery]. Zh Vopr Neirokhir Im N N Burdenko. (2015) 79:91–101. doi: 10.17116/neiro201579591-101
45. Markwardt NA, Haj-Hosseini N, Hollnburger B, Stepp H, Zelenkov P, Rühm A. 405 nm versus 633 nm for protoporphyrin IX excitation in fluorescence-guided stereotactic biopsy of brain tumors. J Biophoton. (2016) 9:901–12. doi: 10.1002/jbio.201500195
46. Potapov AA, Goriainov SA, Loshchenov VB, Savel'eva TA, Gavrilov AG, Okhlopkov VA, et al. [Intraoperative combined spectroscopy (optical biopsy) of cerebral gliomas]. Zh Vopr Neirokhir Im Burdenko. (2013) 77:3–10.
47. Rey-Dios R, Hattab EM, Cohen-Gadol AA. Use of intraoperative fluorescein sodium fluorescence to improve the accuracy of tissue diagnosis during stereotactic needle biopsy of high-grade gliomas. Acta Neurochir. (2014) 156:1071–5. doi: 10.1007/s00701-014-2097-6
48. Thien A, Rao JP, Ng WH, King NKK. The fluoropen: a simple low-cost device to detect intraoperative fluorescein fluorescence in stereotactic needle biopsy of brain tumors. Acta Neurochir. (2016) 159:371–5. doi: 10.1007/s00701-016-3041-8
49. Thien A, Han JX, Kumar K, Ng YP, Rao JP, Ng WH, et al. Investigation of the usefulness of fluorescein sodium fluorescence in stereotactic brain biopsy. Acta Neurochir. (2017) 160:317–24. doi: 10.1007/s00701-017-3429-0
50. Catapano G, Sgulo F, Laleva L, Columbano L, Dallan I, de Notaris M. Multimodal use of indocyanine green endoscopy in neurosurgery: a single-center experience and review of the literature. Neurosurg Rev. (2018) 41:985–98. doi: 10.1007/s10143-017-0858-4
51. Hitti FL, Lee JYK. Endoscopic resection of an intraventricular tumor with second window indocyanine green: 2-dimensional operative video. Oper Neurosurg. (2018) 15:E53–E4. doi: 10.1093/ons/opy053
52. Lee JY, Thawani JP, Pierce J, Zeh R, Martinez-Lage M, Chanin M, et al. Intraoperative near-infrared optical imaging can localize gadolinium-enhancing gliomas during surgery. Neurosurgery. (2016) 79:856–71. doi: 10.1227/NEU.0000000000001450
53. Haglund MM, Hochman DW, Spence AM, Berger MS. Enhanced optical imaging of rat gliomas and tumor margins. Neurosurgery. (1994) 35:930–40. discussion: 40–1. doi: 10.1097/00006123-199411000-00019
54. Watson JR, Martirosyan N, Lemole GM, Trouard TP, Romanowski M. Intraoperative brain tumor resection with indocyanine green using augmented microscopy. J Biomed Optics. (2018) 23:1–4. doi: 10.1117/1.JBO.23.9.090501
55. Butte PV, Mamelak A, Parrish-Novak J, Drazin D, Shweikeh F, Gangalum PR, et al. Near-infrared imaging of brain tumors using the tumor paint BLZ-100 to achieve near-complete resection of brain tumors. Neurosurg Focus. (2014) 36:E1. doi: 10.3171/2013.11.FOCUS13497
56. Martirosyan NL, Georges J, Eschbacher JM, Belykh E, Carotenuto A, Spetzler RF, et al. Confocal scanning microscopy provides rapid, detailed intraoperative histological assessment of brain neoplasms: experience with 106 cases. Clin Neurol Neurosurg. (2018) 169:21–8. doi: 10.1016/j.clineuro.2018.03.015
57. Martirosyan NL, Georges J, Eschbacher JM, Cavalcanti DD, Elhadi AM, Abdelwahab MG, et al. Potential application of a handheld confocal endomicroscope imaging system using a variety of fluorophores in experimental gliomas and normal brain. Neurosurg Focus. (2014) 36:E16. doi: 10.3171/2013.11.FOCUS13486
58. Martirosyan NL, Eschbacher JM, Kalani MY, Turner JD, Belykh E, Spetzler RF, et al. Prospective evaluation of the utility of intraoperative confocal laser endomicroscopy in patients with brain neoplasms using fluorescein sodium: experience with 74 cases. Neurosurg Focus. (2016) 40:E11. doi: 10.3171/2016.1.FOCUS15559
59. Foersch S, Heimann A, Ayyad A, Spoden GA, Florin L, Mpoukouvalas K, et al. Confocal laser endomicroscopy for diagnosis and histomorphologic imaging of brain tumors in vivo. PLoS ONE. (2012) 7:e41760. doi: 10.1371/journal.pone.0041760
60. Breuskin D, Szczygielski J, Urbschat S, Kim YJ, Oertel J. Confocal laser endomicroscopy in neurosurgery—An alternative to instantaneous sections? World Neurosurg. (2017) 100:180–5. doi: 10.1016/j.wneu.2016.12.128
61. Sankar T, Delaney PM, Ryan RW, Eschbacher J, Abdelwahab M, Nakaji P, et al. Miniaturized handheld confocal microscopy for neurosurgery: results in an experimental glioblastoma model. Neurosurgery. (2010) 66:410–7. discussion: 7–8. doi: 10.1227/01.NEU.0000365772.66324.6F
62. Sanai N, Eschbacher J, Hattendorf G, Coons SW, Preul MC, Smith KA, et al. Intraoperative confocal microscopy for brain tumors: a feasibility analysis in humans. Neurosurgery. (2011) 68(2 Suppl Operative):282–90. discussion: 90. doi: 10.1227/NEU.0b013e318212464e
63. Giovannini M. Needle-based confocal laser endomicroscopy. Endosc Ultrasound. (2015) 4:284–8. doi: 10.4103/2303-9027.170405
64. Schneider C, Johnson SP, Gurusamy K, Cook RJ, Desjardins AE, Hawkes DJ, et al. Identification of liver metastases with probe-based confocal laser endomicroscopy at two excitation wavelengths. Lasers Surg Med. (2017) 49:280–92. doi: 10.1002/lsm.22617
65. Pavlov V, Meyronet D, Meyer-Bisch V, Armoiry X, Pikul B, Dumot C, et al. Intraoperative probe-based confocal laser endomicroscopy in surgery and stereotactic biopsy of low-grade and high-grade gliomas: a feasibility study in humans. Neurosurgery. (2016) 79:604–12. doi: 10.1227/NEU.0000000000001365
66. Lynagh R, Ishak M, Georges J, Lopez D, Osman H, Kakareka M, et al. Fluorescence-guided stereotactic biopsy: a proof-of-concept study. J Neurosurg. (2019) 22:1–7. doi: 10.3171/2018.11.JNS18629
67. Lin W-C, Mahadevan-Jansen A, Johnson MD, Weil RJ, Toms SA. In vivo optical spectroscopy detects radiation damage in brain tissue. Neurosurgery. (2005) 57:518–25. doi: 10.1227/01.NEU.0000170559.48166.AC
68. Eschbacher JM, Georges JF, Belykh E, Yazdanabadi MI, Martirosyan NL, Szeto E, et al. Immediate label-free ex vivo evaluation of human brain tumor biopsies with confocal reflectance microscopy. J Neuropathol Exp Neurol. (2017) 76:1008–22. doi: 10.1093/jnen/nlx089
69. Georges J, Zehri A, Carlson E, Nichols J, Mooney MA, Martirosyan NL, et al. Label-free microscopic assessment of glioblastoma biopsy specimens prior to biobanking [corrected]. Neurosurg Focus. (2014) 36:E8. doi: 10.3171/2013.11.FOCUS13478
70. Lee JH, Kim DH, Song WK, Oh M-K, Ko D-K. Label-free imaging and quantitative chemical analysis of Alzheimer's disease brain samples with multimodal multiphoton nonlinear optical microspectroscopy. J Biomed Optics. (2015) 20:056013. doi: 10.1117/1.JBO.20.5.056013
71. Krafft C, Belay B, Bergner N, Romeike BFM, Reichart R, Kalff R, et al. Advances in optical biopsy—Correlation of malignancy and cell density of primary brain tumors using Raman microspectroscopic imaging. Analyst. (2012) 137:5533–7. doi: 10.1039/c2an36083g
72. Hollon TC, Lewis S, Pandian B, Niknafs YS, Garrard MR, Garton H, et al. Rapid intraoperative diagnosis of pediatric brain tumors using stimulated Raman histology. Cancer Res. (2018) 78:278–89. doi: 10.1158/0008-5472.CAN-17-1974
73. Orringer DA, Pandian B, Niknafs YS, Hollon TC, Boyle J, Lewis S, et al. Rapid intraoperative histology of unprocessed surgical specimens via fibre-laser-based stimulated Raman scattering microscopy. Nat Biomed Eng. (2017) 1:0027. doi: 10.1038/s41551-016-0027
74. Romeike BFM, Meyer T, Reichart R, Kalff R, Petersen I, Dietzek B, et al. Coherent anti-stokes raman scattering and two photon excited fluorescence for neurosurgery. Clin Neurol Neurosurg. (2015) 131:42–6. doi: 10.1016/j.clineuro.2015.01.022
75. Mahadevan-Jansen A, Petrich W, Stevens OAC, Hutchings J, Gray W, Day JC. A low background Raman probe for optical biopsy of brain tissue. Biomed Vibrational Spectrosc. (2014). doi: 10.1117/12.2044139. [Epub ahead of print].
76. Stevens OAC, Hutchings J, Gray W, Vincent RL, Day JC. Miniature standoff Raman probe for neurosurgical applications. J Biomed Optics. (2016) 21:087002. doi: 10.1117/1.JBO.21.8.087002
77. Giller CA, Liu H, German DC, Kashyap D, Dewey RB. A stereotactic near-infrared probe for localization during functional neurosurgical procedures: further experience. J Neurosurg. 110:263–73. doi: 10.3171/2008.8.JNS08728
78. Lee I, Kalkanis S, Hadjipanayis CG. Stereotactic laser interstitial thermal therapy for recurrent high-grade gliomas. Neurosurgery. (2016) 79 (Suppl 1):S24–S34. doi: 10.1227/NEU.0000000000001443
79. Ashraf O, Patel NV, Hanft S, Danish SF. Laser-induced thermal therapy in neuro-oncology: a review. World Neurosurg. (2018) 112:166–77. doi: 10.1016/j.wneu.2018.01.123
Keywords: fluorescence, 5-aminolevulinic acid, stereotactic, spectroscopy, optical, biopsy, fluorescein sodium
Citation: Akshulakov SK, Kerimbayev TT, Biryuchkov MY, Urunbayev YA, Farhadi DS and Byvaltsev VA (2019) Current Trends for Improving Safety of Stereotactic Brain Biopsies: Advanced Optical Methods for Vessel Avoidance and Tumor Detection. Front. Oncol. 9:947. doi: 10.3389/fonc.2019.00947
Received: 02 April 2019; Accepted: 09 September 2019;
Published: 02 October 2019.
Edited by:
Mark Preul, Barrow Neurological Institute (BNI), United StatesReviewed by:
Seyedmehdi Payabvash, School of Medicine, Yale University, United StatesArvind P. Pathak, School of Medicine, Johns Hopkins University, United States
Copyright © 2019 Akshulakov, Kerimbayev, Biryuchkov, Urunbayev, Farhadi and Byvaltsev. This is an open-access article distributed under the terms of the Creative Commons Attribution License (CC BY). The use, distribution or reproduction in other forums is permitted, provided the original author(s) and the copyright owner(s) are credited and that the original publication in this journal is cited, in accordance with accepted academic practice. No use, distribution or reproduction is permitted which does not comply with these terms.
*Correspondence: Vadim A. Byvaltsev, ZW1haWxAdW5pLmVkdQ==; Ynl2YWw3NXZhZGltQHlhbmRleC5ydQ==