- 1Department of Radiation Physics, Shandong Cancer Hospital and Institute, Shandong First Medical University and Shandong Academy of Medical Sciences, Jinan, China
- 2Department of Radiation Oncology, Hefei Ion Medical Center, Hefei, China
Background: Synchronous bilateral breast cancer (SBBC) is rare. The purpose of this study was to compare the dosimetric differences in intensity-modulated radiation therapy (IMRT), volumetric-modulated arc therapy (VMAT), helical tomotherapy (HT), and intensity-modulated proton therapy (IMPT) to find an optimal radiotherapy technique for bilateral breast cancer radiotherapy.
Methods: For 11 patients who received synchronous bilateral whole-breast irradiation without local lymph nodal regions, six plans were designed for each patient: IMRT with a single isocenter (IMRT-ISO1), IMRT with two isocenters (IMRT-ISO2), VMAT with a single isocenter (VMAT-ISO1), VMAT with two isocenters (VMAT-ISO2), HT, and IMPT. The differences between the single- and dual-isocentric plans for IMRT and VMAT were compared, and the plan with the better quality was selected for further dosimetric comparisons with IMPT and HT. The plan aimed for a target coverage of at least 95% with the prescription dose of 50 Gy [relative biological effectiveness (RBE)] while minimizing the dose of organs at risk (OARs).
Results: IMRT-ISO1 and VMAT-ISO2 plans were adopted for further dosimetric comparisons because of the reduced dose of the heart and/or lungs compared to IMRT-ISO2 and VMAT-ISO1 plans. The dose coverage of the planning target volume (PTV) was significantly higher in IMPT plans than that in all other plans. VMAT and IMPT plans showed the best conformity, whereas IMRT plans showed the worst conformity. Compared to IMRT and VMAT plans, IMPT and HT plans achieved significantly higher dose homogeneity. IMPT plans reduced the mean dose and low dose volume (V5, V10, and V20) of the heart, left anterior descending artery (LAD), and left ventricle (LV). In high-dose volumes of the heart and cardiac substructures, the IMPT, VMAT, and HT techniques showed similar advantages, and IMRT plans increased the values more than other techniques. IMPT plans had the maximal lung and normal tissue sparing but increased the skin dose compared to IMRT and VMAT plans.
Conclusions: IMPT plans improve both the target coverage and the OARs sparing, especially for the heart, cardiac substructures (LAD and LV), lungs and normal tissue, in synchronous bilateral breast radiotherapy. VMAT and HT could be selected as suboptimal techniques for SBBC patients.
Background
Breast cancer is the most common malignancy among women worldwide. The risk of local recurrence is halved, and the mortality of breast cancer is reduced by one-sixth in patients with breast cancer who receive adjuvant radiotherapy (1). However, radiotherapy is related to long-term cardiopulmonary toxicity and the risk of secondary malignancy, and cardiac toxicity may reduce survival (2–4).
Cardiovascular disease after radiation therapy has become the leading cause of non-breast cancer death in breast cancer patients (5). Therefore, the cardiotoxicity caused by radiotherapy is an important problem that needs to be studied extensively. Darby et al. (2) found that there was a linear relationship between the mean heart dose (MHD) and the incidence of ischemic heart disease, which increased by 7.4% per Gy of the MHD. Therefore, reducing the MHD is critical to reducing long-term cardiotoxicity. Most studies have found that the cardiotoxicity induced by radiotherapy is also closely related to the dose of key heart substructures, including the left anterior descending artery (LAD) and the left ventricle (LV), because studies have shown that high-grade coronary stenosis in LAD is increased in women receiving radiation for the left breast, suggesting a direct link between radiotherapy and coronary stenosis, so the dose to LAD is particularly important (6–9). Therefore, it is important to reduce the dose of the heart and cardiac substructures in patients receiving breast radiotherapy.
It is estimated that synchronous bilateral breast cancer (SBBC) accounts for 2.1% of all breast cancers (10). Compared to unilateral breast cancer, SBBC has a wider treatment volume, and it is closer to the lungs and heart. Because of these anatomical characteristics, designing a plan for radiotherapy for SBBC is a time-consuming and complex process. Three-dimensional conformal radiotherapy (3D-CRT) with tangential fields has some drawbacks for SBBC. Traditional tangential fields may overlap at the anterior border of the sternum, resulting in a high dose in the area in front of the sternum. The inhomogeneity of the target and poor cosmetic effects can be observed in 3D-CRT. Compared to 3D-CRT, intensity-modulated radiation therapy (IMRT), volumetric-modulated arc therapy (VMAT), or tomotherapy can improve the target dose coverage and can achieve acceptable cosmetic effects and cardiopulmonary sparing. Thus, the use of VMAT, IMRT, or tomotherapy was strongly suggested for SBBC (11–15). However, few studies have compared dosimetric differences between the three techniques of IMRT, VMAT, and tomotherapy in SBBC.
Past dosimetric studies have shown that proton therapy delivered a lower cardiac dose compared to photon techniques in unilateral breast cancer (16–19). Intensity-modulated proton therapy (IMPT) has the advantage of improving the dose distribution and reducing the dose of organs at risk (OARs) more without being limited by complex anatomical structures. However, the past studies did not include a dosimetric comparison forSBBC.
The aim of this study was to compare the dosimetric differences of IMRT, VMAT, tomotherapy and IMPT techniques and to establish optimal solutions with heart and substructure sparing for SBBC.
Methods
Patient Selection and Volume Delineation
From September 2006 to December 2018, patients who were diagnosed with SBBC and received bilateral breast radiotherapy at Shandong Cancer Hospital were identified. CT planning datasets of 11 patients (stage T1–T2; post-lumpectomy) were retrieved for dosimetric comparisons. This study was approved by the research ethics board of the Shandong Cancer Hospital.
The clinical target volume (CTV) for the bilateral breast was contoured according to the Radiation Therapy Oncology Group contouring atlas group (20). The planning target volumes (PTVs) were created by expanding a 5-mm margin to the CTV. All PTVs were clipped 5 mm from the skin surface. The whole lung, heart, LV, LAD, liver, and skin were considered organs at risk (OARs). The normal structures were defined as the body minus the PTV (B-P). The skin was defined as a 3-mm area under the body outside of the PTV.
Treatment Planning
For each patient, six plans were generated: IMRT with a single isocenter (IMRT-ISO1), IMRT with two isocenters (IMRT-ISO2), VMAT with a single isocenter (VMAT-ISO1), VMAT with two isocenters (VMAT-ISO2), helical tomotherapy (HT), and IMPT.
IMRT and VMAT plans were designed with the Eclipse version 13.6 treatment planning system (Varian Medical Systems, Palo Alto, CA, USA) using a Varian Trilogy linear accelerator. Plans were optimized using a progressive resolution optimization (PRO) algorithm, and the dose calculation algorithm was an analytic anisotropic algorithm (AAA) with a 0.25-cm grid size. Six MV photon beams were used for the two plans.
For IMRT-ISO1 plans, a single isocenter was placed under the middle of the sternum. Two tangential photon beams were designed for each breast. If the chest wall was too curved, 1–2 additional segment fields were added to protect the lungs and heart. For IMRT-ISO2 plans, two isocenters were placed in the center of the half circle formed by the lung-breast interface at the same CT section. The field angles were the same as those in the IMRT-ISO1 plans.
The isocenters of the VMAT plans were the same as those of the IMRT plans. A bowtie technique, as described by Viren et al. (21), was employed. Four partial arcs, each consisting of ~80° of rotation, were used for one breast. A total of eight partial arcs were designed for the VMAT-ISO1 and VMAT-ISO2 plans. The medial x-jaw shielding the lungs and heart was set to the minimum site (−2 cm), and the lateral x-jaw was opened to include all breast tissue in the beam's-eye-view (BEV). The appropriate starting and stopping angles were selected according to the anatomy of individual patients. To minimize the exposure of the lungs and heart, paring apple-like tangential beams were designed for VMAT plans. VMAT-ISO1 and VMAT-ISO2 plans used different arc degrees to obtain the lowest OARs dose.
HT plans were designed in the Tomotherapy version 5.1.3 treatment planning system (Accuray® Planning Station, Madison, WI, USA). A 2.51-cm field width and a 0.287 pitch were used in the plan. The modulation factor was initially set to 3 and was adjusted throughout optimization. Most areas behind the bilateral breast, including most of the lungs and heart, were all directly blocked for planning.
The IMPT plans were generated in the Varian Eclipse ProBeam proton system. To maximize the robustness of IMPT plans, two en face fields (45/315°) were designed for the bilateral breast. The en face beams were in the direction of respiratory movement, thus reducing the risk of target coverage loss caused by respiratory movement. A range shifter with a thickness of 5 cm was used in the proton fields to cover the superficial target areas at the surface. In IMPT plans, multiple-field optimization and selective robust optimization (22) were used. CTV plus robustness optimization with a 5-mm setup uncertainty and 3.5% range uncertainty was used, whereas normal objectives were applied to the PTV. Plans were generated using a non-linear universal proton optimizer algorithm (NUPO) (23). A proton convolution superposition algorithm was used for the dose calculation with a 0.25-cm grid size.
The prescribed dose was 50 Gy in 2-Gy fractions of relative biological effectiveness (RBE). Plans were designed to first achieve at least 95% coverage of the target to a dose of 50 Gy (RBE) and second to achieve maximal sparing of the OARs, especially the lungs, heart, and substructures. Doses to the OARs were minimized without compromising the PTV coverage. Optimization was performed to reduce the mean total-lung dose (MLD) and mean heart dose (MHD), as well as the mean dose to the LAD. The volume of PTV wrapped in 110% of the prescription dose was <1%. The plan objectives were to implement V20Gy ≤30% for both lungs (24) and an MHD ≤ 5 Gy (3) while synchronously reducing the dose to the OARs as much as possible.
Dosimetric Evaluation
The dose statistics of the plans were based on dose-volume histogram (DVH) analysis. For PTV, D2, D98 (the dose of 2% and 98% volume of PTV) and the values of V100 and V110 (the volumes receiving 100% and 110% of the prescribed dose) were analyzed. The conformity index (CI) and homogeneity index (HI) of the PTV were analyzed. The CI was defined as follows:
The TVPV represents the volume of the PTV covered by the prescribed dose, TV represents the volume of the PTV, and PV represents the total volume covered by the prescribed dose (25).
The HI was defined as follows:
D2 represents the dose of 2% of the volume of the PTV, and D98 represents the dose of 98% of the volume of the PTV. Dp represents the prescribed dose (26). Lower HI values indicate more homogeneous target doses.
To evaluate the irradiated dose to the OARs, the analysis included the mean dose and VXGy (OARs volume receiving X Gy). For the whole lung, V5Gy, V10Gy, V20Gy, V30Gy, V40Gy, and the mean dose were compared. The V5Gy, V10Gy, V20Gy, V30Gy, V40Gy, and Dmean for the heart and LV were compared. V5Gy, V10Gy, V20Gy, V30Gy, V40Gy, Dmax, and Dmean were also compared for the LAD. The V5Gy, V10Gy, V20Gy, V30Gy, V40Gy, V50Gy, and Dmean for B-P and V30Gy, V40Gy, V45Gy, V50Gy, and Dmean for the skin were analyzed. The Dmean for the liver was analyzed. The monitor units (MUs) for the IMRT and VMAT plans were compared.
First, the differences between the single- and dual-isocentric plans for IMRT and VMAT were compared, and the plan with the higher quality was selected from the IMRT and VMAT plans for further dosimetric comparisons with IMPT and HT.
Statistical Analysis
All data were analyzed using the Statistical Package for Social Sciences v19.0 software (SPSS Inc., Chicago, IL, USA). The Wilcoxon matched-paired signed-rank test was used to evaluate the significance of the observed differences between the single- and dual-isocentric plans for IMRT and VMAT. One-way analysis of variance with Tukey's multiple comparison post hoc test was applied to compare the four techniques. The differences were considered statistically significant when p < 0.05.
Results
PTV
The average PTV was 1,364.1 ± 302.0 cm3, ranging from 966.8 to 1,916.6 cm3.
Dose Comparisons for IMRT and VMAT Plans
Table 1 shows the dose parameters for the ISO1 and ISO2 plans of IMRT and VMAT. No significant differences were detected between the ISO1 and ISO2 plans for IMRT and VMAT in regard to the dose coverage, D2, and D98 of the PTV. The IMRT-ISO1 plans achieved significantly higher dose homogeneity (p < 0.05) than the IMRT-ISO2 plans. Significant heart dose (V10, V20, V30, V40, and Dmean) sparing was achieved with IMRT-ISO1 plans compared to IMRT-ISO2 plans. No significant differences were detected in the dose of the lungs, LV, LAD, liver, and MUs between the IMRT-ISO1 and IMRT-ISO2 plans. Among the VMAT-ISO1 and VMAT-ISO2 plans, the VMAT-ISO2 plans showed higher conformity. Lung doses (V10, V20, V30, V40, and Dmean), heart doses (V20, V30, and V40), and LV (Dmean) and LAD (Dmean and Dmax) doses were significantly reduced in VMAT-ISO2 plans. No statistically significant differences were found in the V5 of lungs, V10 and Dmean of the heart, and Dmean of the liver and MUs between VMAT-ISO1 and VMAT-ISO2 plans. However, VMAT-ISO2 plans showed higher values on the V5 of the heart.
Based on these data, IMRT-ISO1 and VMAT-ISO2 plans were selected for further dosimetric comparisons with IMPT and HT.
Dose Comparisons for PTVs
The dose coverage (V100) of the PTV was significantly better in IMPT plans than in all other plans (Table 2). No significant difference in the V100 was observed between the VMAT, IMRT, and HT plans. Among the four techniques, no significant differences were observed in the V110 of the PTV. IMPT and HT plans showed a lower maximum dose of the PTV (D2). VMAT plans showed the lowest minimum dose of the PTV (D98). VMAT and IMPT plans showed the best conformity, whereas IMRT plans showed the worst conformity. IMPT and HT plans achieved significantly higher dose homogeneity than IMRT and VMAT plans. Figure 1 shows the dose distributions of the four plans for a typical patient in our study. Figure 2 shows the DVHs of the four plans for the same patient.
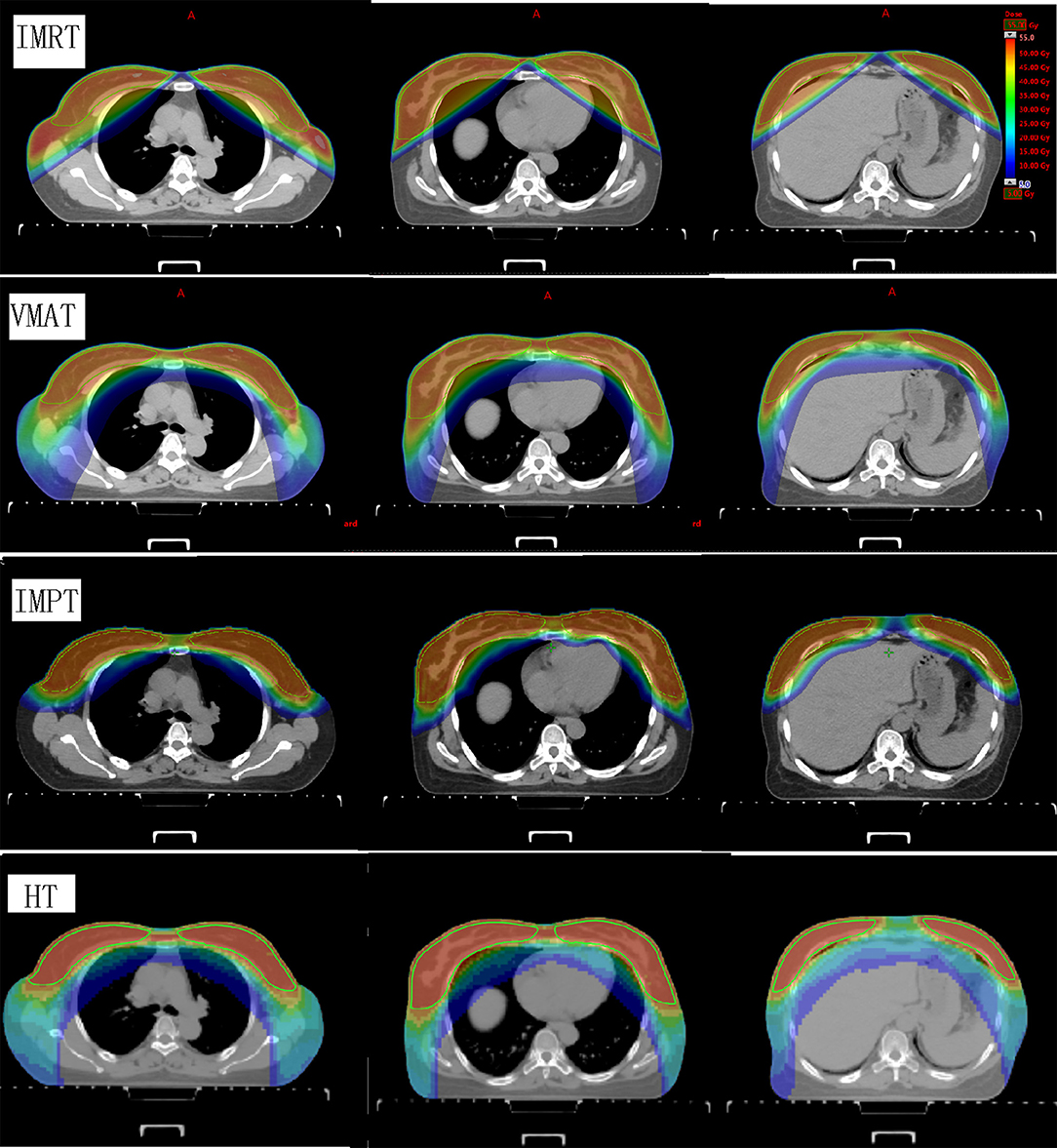
Figure 1. Dose distributions of the four plans for a representative patient. The images show IMRT, VMAT, IMPT, and HT techniques from the top row to the bottom row. The structures outlined in green are the bilateral whole breast planning target volumes. IMRT, intensity-modulated radiation therapy; VMAT, volumetric-modulated arc therapy; IMPT, intensity-modulated proton therapy; HT, helical tomotherapy.
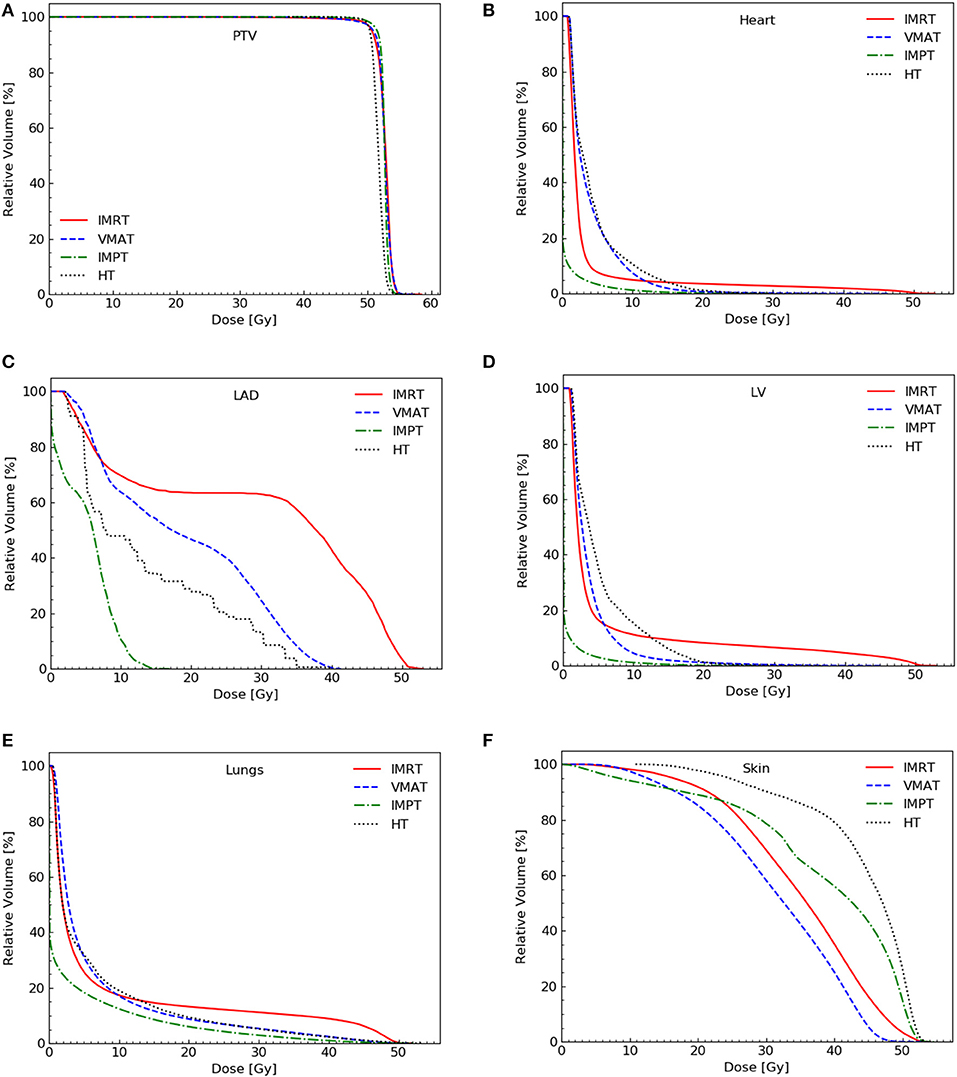
Figure 2. Dose-volume histograms for the four plans for a representative patient. PTV (A), heart (B), LAD (C), LV (D), lungs (E), and skin (F). PTV, planning target volume; LV, left ventricle; LAD, left anterior descending artery.
Dose Comparisons for the Heart and Substructures
Table 3 shows the dose parameters of the heart and substructures.
IMPT plans showed the lowest values for the V5, V10, V20, and Dmean of the heart (p < 0.05). The VMAT and HT plans showed the highest values for the V5 of the heart. IMRT significantly increased the V20, V30, and V40 of the heart compared with other techniques. No statistically significant differences were found in the V10 and Dmean of the heart among the VMAT, IMRT, or HT techniques. No statistically significant differences were found in the V30 and V40 of the heart among the VMAT, IMPT, or HT techniques.
Compared to other plans, IMPT plans reduced the V5 and mean dose of the LV significantly (p < 0.05). IMPT reduced the V10 and V20 of the LV slightly but non-significantly compared with VMAT (IMPT plans showed the lowest average values). IMRT plans showed the highest values for the V20, V30, V40, and Dmean of the LV. No statistically significant differences were found in the V30 and V40 of the LV among the VMAT, IMPT, or HT techniques.
A significant decrease in the V5, V10, V20, V30, Dmean, and Dmax of the LAD was achieved with the IMPT technique compared with the other techniques (p < 0.01). IMRT significantly increased the V20, V30, V40, Dmean, and Dmax of the LAD compared with the other techniques. The VMAT and IMRT plans showed the highest values for the V5 and V10 of the LAD. The V40 to the LAD was significantly lower in IMPT and HT than in the IMRT and VMAT techniques (p < 0.05).
Dose Comparisons for OARs
For whole lungs, IMPT plans reduced the V5, V10, V20, and mean dose significantly compared with the other plans (p < 0.05). IMPT plans also showed the lowest average values for the V30 and V40 of the lungs among the four techniques, but no statistically significant differences were found compared with VMAT and HT. The VMAT and HT plans showed the highest values for the V5 of the lungs. IMRT plans increased the V20, V30, V40, and Dmean of the lungs significantly compared with other techniques. Table 4 shows the dose parameters of the lungs.
For the skin, statistically significant differences were found in the V30, V40, V45, and Dmean of the skin when comparing any two techniques. HT plans increased the V30, V40, V45, V50, and Dmean of the skin significantly compared with the other techniques. IMPT plans increased the V30, V40, V45, and Dmean of the skin significantly compared with IMRT and VMAT techniques. A significant decrease in the V30, V40, V45, V50, and Dmean of the skin was achieved with the VMAT technique compared with the other techniques (p < 0.01). Table 5 shows the dose parameters of the skin.
For the liver, IMPT plans reduced and HT plans increased the mean dose significantly compared with the other plans (p < 0.01). Table 4 shows the average mean dose of the liver in the four techniques.
Table 6 shows the dose parameters of B-P. For the B-P, IMPT plans reduced the dose (V5, V10, V20, V30, V40, V50, and Dmean) significantly compared with the other plans. HT plans increased the V5, V10, V20, and Dmean of the B-P significantly, and IMRT plans increased the V30, V40, and V50 of the B-P significantly.
Discussion
SBBC is a rare disease. There are no standard guidelines for the treatment of SBBC, and due to the increased demand for breast-conserving treatment, synchronous irradiation of the bilateral breast is imperative. Synchronous bilateral breast irradiation is challenging due to the large and complex target volume and the need to minimize the dose to the heart and lungs. IMRT, VMAT, and HT techniques have been reported to improve dosimetry in SBBC patients, and past dosimetric studies have shown that IMPT can reduce the cardiac dose significantly in unilateral breast cancer (11–19). Previous studies have not compared dosimetric differences between IMRT, VMAT, and HT in SBBC. To the best of our knowledge, this is the first report on the dosimetry of IMRT, VMAT, HT, and IMPT for SBBC.
First, we compared the dosimetric differences between single- and dual-isocentric IMRT and VMAT plans. Compared to the IMRT-ISO2 plans, significantly higher dose homogeneity and heart dose sparing were achieved with IMRT-ISO1 plans. VMAT-ISO2 plans showed higher conformity and significantly reduced lung, heart, LV, and LAD doses than VMAT-ISO1 plans. Boman et al. (27) compared mono-isocenter and dual-isocenter VMAT plans for 11 SBBC patients with lymph node positivity. When compared to Iso1 plans, Iso2 plans reduced the mean dose for the lungs and heart from 11.3 Gy and 3.8 Gy to 10.9 Gy and 3.6 Gy, respectively. In single-isocenter plans, there was a statistically positive correlation between the PTV volume and the maximum PTV dose, but this was not observed in the dual-isocenter plans. This suggests that the use of a single isocenter may not be the best solution for very large bilateral breasts and that a dual-isocentric solution may be required. However, the LAD and LV were not delineated in the above study. In our study, paring apple-like tangential beams were designed for VMAT plans to minimize the exposure of the lungs and heart. The medial x-jaw shielding of the lungs and heart was set to the minimum site (−2 cm). Because the minimum setting of the medial x-jaw value can only be −2 cm, VMAT-ISO2 plans were better at blocking the lungs and heart than VMAT-ISO1 plans. Therefore, the VMAT-ISO2 plans in this study had better dosimetric advantages. The IMRT plans were mainly based on tangential fields, which is similar to the field-in-field technique. Therefore, the IMRT-ISO2 plans did not show a dosimetric advantage, and IMRT-ISO1 plans were superior. IMRT-ISO1 and VMAT-ISO2 plans were selected for further dosimetric comparisons with IMPT and HT plans.
IMPT plans achieved the highest dose coverage of the PTV compared with all other plans. VMAT and IMPT plans showed the best conformity, whereas IMRT plans showed the worst conformity. IMPT and HT plans achieved significantly higher dose homogeneity and a lower maximum dose of the PTV (D2) than IMRT and VMAT plans. The dosimetric advantage for the target of proton therapy has been recognized (16–19). In our study, we found that VMAT, IMRT, and HT techniques achieved comparable dose coverage of the PTV.
Despite significant reductions in cardiac doses over the past few years (6, 28), radiation-induced heart disease remains the leading cause of death among long-term breast cancer survivors after radiation therapy. Darby et al. (2) found that there was a linear relationship between the MHD and the incidence of ischemic heart disease, which increased by 7.4% per Gy of the MHD. Therefore, the MHD is commonly used as a reference for cardiotoxicity studies. However, there is increasing evidence that the dose of heart substructures needs to be considered. Some studies have focused on the LAD and LV as important parts of the heart associated with radiation-induced heart disease (8, 29). Therefore, an appropriate technique that could minimize cardiac and substructure doses in breast cancer radiation therapy may be beneficial for breast patients. Proton therapy has been confirmed to reduce the dose of the heart and LAD in patients with left breast cancer especially because high cardiac doses remain in advanced photon techniques (17–19). In our study, we also found a dosimetric advantage of IMPT for sparing the heart, LAD, and LV doses in SBBC patients. IMPT plans reduced the mean dose and low dose volume of the heart, LAD, and LV. In high dose volumes of the heart and cardiac substructures, IMPT, VMAT, and HT techniques showed similar advantages, and IMRT plans increased the values more than other techniques. IMPT is the only technique capable of delivering an MHD <1 Gy, whereas the three other techniques delivered <5 Gy. Kim et al. (15) compared 3DCRT, IMRT, and VMAT treatment plans for 10 SBBC patients. In their study, 3DCRT reduced the mean dose and V10Gy of the heart, and IMRT reduced the V20Gy and V40Gy of the heart. These results are different from the results of our study mainly because of the different irradiation field angles. In their study, 3DCRT comprised eight fields with multiple isocenters, whereas IMRT and VMAT comprised 12 fields and two partial arcs with a single isocenter. The increase in the number and angle of irradiation fields will increase the exposure to a low dose and thus increase the cardiac mean dose. In their study, the Dmean of the heart in 3DCRT, IMRT, and VMAT were 818.00 ± 306.09 cGy, 946.08 ± 166.75 cGy, and 1,447.65 ± 239.94 cGy, respectively. The values in our study were lower than the values in their study probably because of the fields that we used. In our study, no breath-holding was used. To further reduce the dose to the heart, deep-inspiration breath hold (DIBH) could be used in IMRT and VMAT plans.
Past studies have demonstrated that proton therapy can significantly reduce the V5 and V20 values in the ipsilateral lung by nearly 50% compared to traditional 3D-CRT and IMRT, and the low-dose radiation volume could be reduced significantly while providing a reduced or similarly high-dose radiation volume with proton therapy in unilateral breast cancer (19, 30, 31). In our study, IMPT plans reduced the mean dose and all dose-volume parameters of lungs. Lung cancer data showed a correlation between the multi-dose-volume parameters and late pulmonary fibrosis. The dosimetric factors of the mean lung dose and the V10Gy, V20Gy, V30Gy, V40Gy, and V50Gy of the lung were highly correlated with late radiation fibrosis (32). Therefore, IMPT may reduce the incidence of late pulmonary complications compared to the photon techniques. In the four techniques, the values of the V20 for the lungs did not exceed 20%. The VMAT and HT plans increased the low dose (V5), and the IMRT plans increased the high dose (V20, V30, and V40) and Dmean of the lungs significantly. No differences were observed between VMAT and HT techniques in all dose-volume parameters of the lungs.
Compared with photon therapy, the disadvantages of relatively high skin doses and different levels of dermatitis have been reported in proton therapy clinical studies (33, 34). Therefore, in this study, the skin dose was included in the results. The results showed that IMPT plans increased the skin dose significantly compared with IMRT and VMAT techniques. However, HT plans showed the highest skin dose in the four techniques, and the lowest dose was achieved in VMAT plans. In our study, we did not optimize the skin dose. Tommasino et al. (35) published a model-based approach that showed that optimizing skin doses in IMPT may reduce the risk of acute skin toxicity compared to IMRT. If we add the skin to the optimization process, the skin dose could be reduced in the four techniques. This will be examined in future research.
IMPT plans reduced the mean dose of the liver significantly, and HT plans increased the mean dose compared with other plans (p < 0.01). The HT plans showed the highest liver dose, but the Dmean was <5 Gy. Patients with radiation-induced liver disease (RILD) receive a significantly higher mean dose to the liver, and the incidence rate of RILD was <5% when the dose of the whole liver was <30–32 Gy (36). So, we believe that in terms of liver protection, all four techniques are feasible.
IMPT plans reduced the dose of non-target tissues significantly compared with other plans. The reduction in these doses may result in a decreased incidence of secondary malignancies after adjuvant radiotherapy (37).
As discussed above, IMPT plans achieved superior cardiac and lung sparing in synchronous bilateral breast radiotherapy compared to photon therapy, which may lead to a reduction in cardiopulmonary toxicity and the potential benefits related to this reduction. Because proton therapy is still a less common and more expensive treatment technique, VMAT and HT could be used as a suboptimal technique for SBBC patients. DIBH could be used in photon plans to reduce the dose to the heart and lungs.
Conclusion
In SBBC radiotherapy, IMPT plans improve both the target coverage and OARs sparing, especially for the heart, cardiac substructures (LAD and LV), lungs, and normal tissue. Long-term follow-up is required to confirm the benefits of IMPT over photon techniques for late toxicity and secondary malignancies. IMRT technique increases the mean dose and high dose volume of all OARs while only reducing the V5 of the lungs and heart compared to VMAT and HT techniques. VMAT and HT techniques show better dosimetric advantages compared to IMRT technique. VMAT and HT could be used as a suboptimal technique for SBBC patients.
Data Availability Statement
The datasets generated for this study are available on request to the corresponding author.
Ethics Statement
This study was approved by the Research Ethics Board of the Shandong Cancer Hospital. Written informed consent was not required in accordance with national and institutional guidelines.
Author Contributions
TS and YY designed the study. YT collected the CT data and delineated OARs volumes. TS, XLin, and XLiu designed the treatment plans. LP, CT, and JD analyzed the data. TS wrote the paper. All authors read and approved the final manuscript.
Funding
This study was supported by the National Key Research and Development Programs of China (No. 2017YFC0113202).
Conflict of Interest
The authors declare that the research was conducted in the absence of any commercial or financial relationships that could be construed as a potential conflict of interest.
References
1. EBCTCG, Darby S, McGale P, Correa C, Taylor C, Arriagada R, et al. Effect of radiotherapy after breast-conserving surgery on 10-year recurrence and 15-year breast cancer death: meta-analysis of individual patient data for 10801 women in 17 randomised trials. Lancet. (2011) 378:1707–16. doi: 10.1016/S0140-6736(11)61629-2
2. Darby S, Ewertz M, McGale P, Bennet AM, Blom-Goldman U, Brønnum D, et al. Risk of ischemic heart disease in women after radiotherapy for breast cancer. N Engl J Med. (2013) 368:987–98. doi: 10.1056/NEJMoa1209825
3. Shilkrut M, Belkacemi Y, Kuten A. Secondary malignancies in survivors of breast cancer: how to overcome the risk. Crit Rev Oncol Hematol. (2012) 84:e86–9. doi: 10.1016/j.critrevonc.2010.06.007
4. Lee MS, Finch W, Mahmud E. Cardiovascular complications of radiotherapy. Am J Cardiol. (2013) 112:1688–96. doi: 10.1016/j.amjcard.2013.07.031
5. Fan LL, Luo YK, Xu JH, He L, Wang J, Du XB. A dosimetry study precisely outlining the heart substructure of left breast cancer patients using intensity-modulated radiation therapy. J Appl Clin Med Phys. (2014) 15:4624. doi: 10.1120/jacmp.v15i5.4624
6. Taylor CW, Nisbet A, Mcgale P, Darby SC. Cardiac exposures in breast cancer radiotherapy: 1950s−1990s. Int J Radiat Oncol Biol Phy. (2007) 69:1484–95. doi: 10.1016/j.ijrobp.2007.05.034
7. Correa CR, Das IJ, Litt HI, Hwang WT, Solin LJ, Harris EE. Association between tangential beam treatment parameters and cardiac abnormalities after definitive radiation treatment for left-sided breast cancer. Int J Radiat Oncol Biol Phys. (2008) 72:508–16. doi: 10.1016/j.ijrobp.2007.12.037
8. Nilsson G, Holmberg L, Garmo H, Duvernoy O, Sjogren I, Lagerqvist B. Distribution of coronary artery stenosis after radiation for breast cancer. J Clin Oncol. (2012) 30:380–6. doi: 10.1200/JCO.2011.34.5900
9. Gagliardi G, Constine LS, Moiseenko V, Correa C, Pierce LJ, Allen AM, et al. Radiation dose-volume effects in the heart. Int J Radiat Oncol Biol Phys. (2010)76(3 Suppl):S77–85. doi: 10.1016/j.ijrobp.2009.04.093
10. Kheirelseid A, Jumustafa H, Miller N, Curran C, Sweeney K, Malone C, et al. Bilateral breast cancer: analysis of incidence, outcome, survival and disease characteristics. Br Cancer Res Treat. (2011) 126:131–40. doi: 10.1007/s10549-010-1057-y
11. Nicolini G, Clivio A, Fogliata A, Vanetti E, Cozzi L. Simultaneous integrated boost radiotherapy for bilateral breast: a treatment planning and dosimetric comparison for volumetric modulated arc and fixed field intensity modulated therapy. Radiat Oncol. (2009) 4:27. doi: 10.1186/1748-717X-4-27
12. Wadasadawala T, Visariya B, Sarin R, Upreti RR, Paul S, Phurailatpam R. Use of tomotherapy in treatment of synchronous bilateral breast cancer: dosimetric comparison study. Br J Radiol. (2015) 88:20140612. doi: 10.1259/bjr.20140612
13. Kaidar-Person O, Kostich M, Zagar TM, Jones E, Gupta G, Mavroidis P, et al. Helical tomotherapy for bilateral breast cancer: clinical experience. Breast. (2016) 28:79–83. doi: 10.1016/j.breast.2016.05.004
14. Seppälä J, Heikkilä J, Myllyoja K, Koskela K. Volumetric modulated arc therapy for synchronous bilateral whole breast irradiation—a case study. Rep Pract Oncol Radiother. (2015) 20:398–402. doi: 10.1016/j.rpor.2015.05.011
15. Kim SJ, Lee MJ, Youn SM. Radiation therapy of synchronous bilateral breast carcinoma(SBBC) using multiple techniques. Med Dosim. (2018) 43:55–68. doi: 10.1016/j.meddos.2017.08.003
16. Weber DC, Ares C, Lomax AJ, Kurtz JM. Radiation therapy planning with photons and protons for early and advanced breast cancer: an overview. Radiat Oncol. (2006) 20:22. doi: 10.1186/1748-717X-1-22
17. Mast ME, Vredeveld EJ, Credoe HM, van Egmond J, Heijenbrok MW, Hug EB, et al. Whole breast proton irradiation for maximal reduction of heart dose in breast cancer patients. Br Cancer Res Treat. (2014) 148:33–9. doi: 10.1007/s10549-014-3149-6
18. Jimenez RB, Goma C, Nyamwanda J, Kooy HM, Halabi T, Napolitano BN, et al. Intensity modulated proton therapy for postmastectomy radiation of bilateral implant reconstructed breasts: a treatment planning study. Radiother Oncol. (2013) 107:213–7. doi: 10.1016/j.radonc.2013.03.028
19. Ares C, Khan S, MacArtain AM, Heuberger J, Goitein G, Gruber G, et al. Postoperative proton radiotherapy for localized and locoregional breast cancer: potential for clinically relevant improvements? Int J Radiat Oncol Biol Phys. (2010) 76:685–97. doi: 10.1016/j.ijrobp.2009.02.062
21. Viren T, Heikkiä J, Myllyoja K, Koskela K, Lahtinen T, Seppälä J. Tangential volumetric modulated arc therapy technique for left-sided breast cancer radiotherapy. Radiat Oncol. (2015) 10:79. doi: 10.1186/s13014-015-0392-x
22. Li Y, Niemela P, Liao L, Jiang S, Li H, Poenisch F, et al. Selective robust optimization: a new intensity-modulated proton therapy optimization strategy. Med Phys. (2015) 42:4840–7. doi: 10.1118/1.4923171
23. Jacques R, Taylor R, Wong J, McNutt T. Towards real-time radiation therapy: GpU accelerated superposition/convolution. Comput Methods Programs Biomed. (2010) 98:285–92. doi: 10.1016/j.cmpb.2009.07.004
24. Marks LB, Bentzen SM, Deasy JO, Kong FM, Bradley JD, Vogelius IS, et al. Radiation dose-volume effects in the lung. Int J Radiat Oncol Biol Phys. (2010) 76(suppl 3):S70–6. doi: 10.1016/j.ijrobp.2009.06.091
25. Paddick IA. Simple scoring ratio to index the conformity of radiosurgical treatment plans, technical note. J Neurosurg. (2000) 93:S219–22. doi: 10.3171/jns.2000.93.supplement_3.0219
26. Wu Q, Mohan R, Morris M, Lauve A, Schmidt-Ullrich R. Simultaneous integrated boost intensity-modulated radiotherapy for locally advanced head-and-neck squamous cell carcinomas. I: dosimetric results. Int J Radiat Oncol Biol Phys. (2003) 56:573–85. doi: 10.1016/s0360-3016(02)04617-5
27. Boman E, Maija Rossia M, Kapanen M. The robustness of dual isocenter VMAT radiation therapy for bilateral lymph node positive breast cancer. Phys Med. (2017) 14:11–7. doi: 10.1016/j.ejmp.2017.11.006
28. Taylor AM, Wang Z, Macaulay E, Jagsi R, Duane F, Darby SC. Exposure of the heart in breast cancer radiotherapy: a systematic review of heart doses published during 2003–2013. Int J Radiat Oncol Biol Phys. (2015) 93:845–53. doi: 10.1016/j.ijrobp.2015.07.2292
29. van den Bogaard VA, Ta BD, van der Schaaf A, Bouma AB, Middag AM, Bantema-Joppe EJ, et al. Validation and modification of a prediction model for acute cardiac events in patients with breast Cancer treated with radiotherapy based on three-dimensional dose distributions to cardiac substructures. J Clin Oncol. (2017) 35:1171–8. doi: 10.1200/JCO.2016.69.8480
30. MacDonald SM, Patel SA, Hickey S, Specht M, Isakoff SJ, Gadd M, et al. Proton therapy for breast cancer after mastectomy: early outcomes of a prospective clinical trial. Int J Radiat Oncol Biol Phys. (2013) 86:484–90. doi: 10.1016/j.ijrobp.2013.01.038
31. Xu N, Ho MW, Li Z, Morris CG, Mendenhall NP. Can proton therapy improve the therapeutic ratio in breast cancer patients at risk for nodal disease? Am J Clin Oncol. (2014) 37:568–74. doi: 10.1097/COC.0b013e318280d614
32. Mazeron R, Etienne-Mastroianni B, Perol D, Arpin D, Vincent M, Falchero L, et al. Predictive factors of late radiation fibrosis: a prospective study in non-small cell lung cancer. Int J Radiat Oncol Biol Phys. (2010) 77:38–43. doi: 10.1016/j.ijrobp.2009.04.019
33. Liang X, Bradley JA, Zheng D, Rutenberg M, Yeung D, Mendenhall N, et al. Prognostic factors of radiation dermatitis following passive-scattering proton therapy for breast cancer. Radiat Oncol. (2018) 13:72. doi: 10.1186/s13014-018-1004-3
34. Verma V, Iftekaruddin Z, Badar N, Hartsell W, Han-Chih Chang J, Gondi V, et al. Proton beam radiotherapy as part of comprehensive regional nodal irradiation for locally advanced breast cancer. Radiother Oncol. (2017) 123:294–8. doi: 10.1016/j.radonc.2017.04.007
35. Tommasino F, Durante M, D'Avino V, Liuzzi R, Conson M, Farace P, et al. Modelbased approach for quantitative estimates of skin, heart, and lung toxicity risk for left-side photon and proton irradiation after breast-conserving surgery. Acta Oncol. (2017) 56:730–6. doi: 10.1080/0284186X.2017.1299218
36. Cheng JC, Wu JK, Huang CM, Liu HS, Huang DY, Cheng SH, et al. Radiation induced liver disease after three-dimensional conformal radiotherapy for patients with hepatocellular carcinoma: dosimetric analysis and implication. Int J Radiat Oncol Biol Phys. (2002) 54:156–62. doi: 10.1016/S0360-3016(02)02915-2
Keywords: synchronous bilateral breast cancer, intensity-modulated radiation therapy, volumetric-modulated arc therapy, tomotherapy, proton beam therapy, dosimetry
Citation: Sun T, Lin X, Tong Y, Liu X, Pan L, Tao C, Duan J and Yin Y (2020) Heart and Cardiac Substructure Dose Sparing in Synchronous Bilateral Breast Radiotherapy: A Dosimetric Study of Proton and Photon Radiation Therapy. Front. Oncol. 9:1456. doi: 10.3389/fonc.2019.01456
Received: 27 June 2019; Accepted: 05 December 2019;
Published: 10 January 2020.
Edited by:
Drexell Hunter Boggs, University of Alabama at Birmingham, United StatesReviewed by:
Allison Paige Dalton, University of Alabama at Birmingham, United StatesAdam Kole, University of Alabama at Birmingham, United States
Jehee Isabelle Choi, Memorial Sloan Kettering Cancer Center, United States
Copyright © 2020 Sun, Lin, Tong, Liu, Pan, Tao, Duan and Yin. This is an open-access article distributed under the terms of the Creative Commons Attribution License (CC BY). The use, distribution or reproduction in other forums is permitted, provided the original author(s) and the copyright owner(s) are credited and that the original publication in this journal is cited, in accordance with accepted academic practice. No use, distribution or reproduction is permitted which does not comply with these terms.
*Correspondence: Yong Yin, yinyongsd@126.com