- 1Department of Anatomy and Structural Biology, Montefiore Medical Center, Einstein College of Medicine, Bronx, NY, United States
- 2Integrated Imaging Program, Montefiore Medical Center, Einstein College of Medicine, Bronx, NY, United States
- 3Department of Surgery, Montefiore Medical Center, Einstein College of Medicine, Bronx, NY, United States
- 4Gruss-Lipper Biophotonics Center, Montefiore Medical Center, Einstein College of Medicine, Bronx, NY, United States
- 5Department of Medicine (Oncology), Montefiore Medical Center, Einstein College of Medicine, Bronx, NY, United States
- 6School of Health & Life Sciences, Teesside University, Middlesbrough, United Kingdom
- 7National Horizons Centre, Teesside University, Darlington, United Kingdom
- 8Department of Pathology, Montefiore Medical Center, Einstein College of Medicine, Bronx, NY, United States
Breast cancer is the second most commonly diagnosed cancer in American women following skin cancer. Despite overall decrease in breast cancer mortality due to advances in treatment and earlier screening, black patients continue to have 40% higher risk of breast cancer related death compared to white patients. This disparity in outcome persists even when controlled for access to care and stage at presentation and has been attributed to differences in tumor subtypes or gene expression profiles. There is emerging evidence that the tumor microenvironment (TME) may contribute to the racial disparities in outcome as well. Here, we provide a comprehensive review of current literature available regarding race-dependent differences in the TME. Notably, black patients tend to have a higher density of pro-tumorigenic immune cells (e.g., M2 macrophages, regulatory T cells) and microvasculature. Although immune cells are classically thought to be anti-tumorigenic, increase in M2 macrophages and angiogenesis may lead to a paradoxical increase in metastasis by forming doorways of tumor cell intravasation called tumor microenvironment of metastasis (TMEM). Furthermore, black patients also have higher serum levels of inflammatory cytokines, which provide a positive feedback loop in creating a pro-metastatic TME. Lastly, we propose that the higher density of immune cells and angiogenesis observed in the TME of black patients may be a result of evolutionary selection for a more robust immune response in patients of African geographic ancestry. Better understanding of race-dependent differences in the TME will aid in overcoming the racial disparity in breast cancer mortality.
Introduction
Breast cancer is the second most common cancer in women in the U.S. following skin cancer, and is the second leading cause of cancer death (1, 2). In both female and male breast cancer, black race or African American (AA) ethnicity is associated with a worse prognosis compared to white race or European American (EA) ethnicity (3–6). Clinical and treatment factors associated with worse outcomes for black race in breast cancer are well-described (Table 1). Although breast cancer incidence and mortality have declined by ~40% in the U.S. between 1989 and 2017 (2), mortality rates have declined less in black women, which has contributed to persistently higher breast cancer mortality rate for black women (17). Furthermore, despite the lower incidence rate, the death rate for black women with breast cancer is now 40% higher than for white women (1, 2). For black women younger than 50 years of age, the death rate is double than that of white women of the same age group (2).
A widening racial gap in survival has also been observed for women in the US Department of Defense healthcare system (18), as well as for women undergoing NCI-sponsored clinical trials receiving contemporary therapy (Table 2), suggesting that factors other than disparities in care may be playing a role in contributing to inferior outcomes (20). A similar disparity in survival was also observed in patients with ER+/HER2- disease treated at Montefiore Medical Center, which serves a large African American population (13).
Indeed, several studies have indicated that racial disparity in breast cancer outcome between patients of African compared to those of Caucasian ancestry are due to biological factors including differences in gene expression patterns of tumor cells as well as differences in the local milieu (or context) in which cancer cells reside, typically referred to as the tumor microenvironment (TME) (21). TME encompasses a variety of cells including fibroblasts, adipocytes, immune cells, endothelial cells, as well as a plethora of signaling molecules and extracellular matrix (ECM) components. The non-cancerous stromal cells influence the behavior of cancer cells by direct contact, as well as by secreting ECM proteins, chemokines, cytokines and growth factors. Thus, it is the dynamic interplay between cancer cells, non-cancerous cells and other components of TME that dictates the growth and invasiveness of tumors and may contribute to racial disparity in breast cancer outcome. This review will focus on the racial disparities in TME as potential modulators of cancer progression, metastasis and response to therapy.
Racial/Ethnic Disparities in the Breast Cancer Microenvironment
Breast cancer is an extremely heterogeneous disease at multiple levels, including histologic subtype, grade, hormone and growth factor receptor status, as well as gene expression pattern (22). Molecular profiling based on the analysis of gene copy number, mRNA, microRNA and protein expression supports at least four (23), and up to ten intrinsic subtypes (24). Although an association of these intrinsic subtypes with disease outcome has been clearly demonstrated (22), it has been increasingly appreciated that the tumor microenvironment (TME) also plays an important role in regulating breast cancer biology at all stages of progression and ultimately influences disease outcome (25). Moreover, multiple lines of evidence indicate that black patients exhibit a TME with more pronounced pro-tumorigenic properties, which may be responsible for, and contribute to the disparity in breast cancer survival.
Disparity in Breast Cancer Immune Landscape
A number of immune cells reside within the TME and contribute to cancer progression. Among the well-studied ones are tumor-associated lymphocytes (TILs), regulatory T cells (T-regs), neutrophils, tumor-associated macrophages (TAMs), and myeloid-deprived suppressor cells (MDSCs).
Lymphocytes
TILs, the most abundant immune cells within breast TME, convey a good prognosis especially in patients with triple negative (TN) disease (26–28). In particular, high TIL count in TN disease has been associated with better survival, as well as better response to treatment (29, 30). Although the analysis of gene expression variants have shown higher expression of genes associated with immune response in tumors from African American (AA) compared to European American (EA) patients (31), the comparison of TIL counts, either as percent-area of stroma, or as percent-area of the whole section did not show any differences between these two racial groups (32). Likewise, the distribution of tumors that were lymphocyte-predominant (>50% TIL), lymphocyte-moderate (10–50% TIL) and lymphocyte-poor (<10% TILs) was not significantly different (32). The immunomodulatory score (33), which helps predict response to neoadjuvant chemotherapy, was also not different between AA and EA patients (32).
Unlike TILs, increased number of T-regs in breast TME has been associated with decreased relapse-free and overall survival (34, 35). This is not surprising as T-regs are suppressors of T cell responses and mediators of immune tolerance, and as such, T-regs may contribute to immune evasion of cancer cells. Indeed, ablation of T-regs leads to CD4 T-cell- and interferon-γ (INF-γ)-dependent reduction of primary and metastatic tumor growth in a transgenic mouse model of breast cancer (36). When analyzed as relative proportion among 9 immune cell populations (B-cells, dendritic cells, eosinophils, macrophages, mast cells, neutrophils, NK cells, CD4 and CD8 T-cells), T-regs were present in significantly higher proportion in TME of AA than of EA patients (32). It is therefore possible that more aggressive disease in AA compared to EA may be due to more pronounced immunosuppressive TME in breast cancers of AA patients. Since the recruitment of T-regs into the TME occurs partly via the C-X-C motif chemokine-12 (CXCL12) signaling factor, it would be interesting to see if TME in breast cancers from AA compared to EA patients produces more CXCL12.
Myeloid Cells
Neutrophils have been typically involved in the pathophysiology of acute infection and elimination of bacteria. However, about a decade ago, studies in pre-clinical models of cancer demonstrated that depending on the levels of chemokines in TME, tumor-associated neutrophils (TANs) may develop an either pro- or anti-tumor phenotype (37). More recent meta-analyses showed that a high neutrophils-to-lymphocytes ratio is associated with worse outcome (38–40). Neutrophils are also found to be potent suppressors of T-cell mediated immunity (41). Moreover, neutrophils can expulse their DNA to create so-called neutrophil extracellular traps (NETs), which can promote metastasis (42). Relative to other immune cell populations, the mean proportion of neutrophils was not found to be different between AA and EA (32). Quite interestingly, up to 12.5% of healthy women of AA descent were found to have neutropenia (43). However, it is not yet clear how that may affect breast cancer incidence and progression.
The role of TAMs in the progression of breast cancer has been extensively studied (44–47). This is not surprising given that macrophages are the most abundant leukocytes in breast TME in both AA and EA patients (32). Macrophages are extremely plastic and under constant influence of TME, which can modify them to function as either tumor inhibitory (M1) or tumor promoting (M2) agents (48, 49). M1 macrophages, also called classically-activated macrophages, secrete pro-inflammatory cytokines such as INF-γ, TNF-α, IL-1, IL6, and IL-12, while M2 macrophages secrete anti-inflammatory cytokines, such as IL-10 and TGF-β. It is important to mention that both within and between the M1 and M2 polarization states, there exist several subcategories of macrophage phenotypes. Although most studies associate high macrophage density with poor outcome (50–52), macrophage density does not seem to play a role in the outcome of patients who have ER+ tumors smaller than 1 cm (53). However, TAMs seem to differ in TME of AA and EA patients not only in their density, but also in their composition. For instance, AA patients compared to EA and non-black Hispanic patients tend to have not only higher macrophage density (54), but also higher density of pro-tumorigenic M2, CD206-expressing macrophages in the TME (55). Consistent with the well-established role of M2 macrophages in promoting tumor invasion, angiogenesis, metastasis and immunosuppression (56–58), the density of CD206 M2 macrophages was found to be a significant predictor of progression-free survival independently of race (54). This even held true after adjusting for race and HER2 expression. Interestingly, if evaluated as a mean proportion of the leukocyte compartment within TME, tumors from AA compared to EA patients have a higher overall macrophage score, but tumors from EA patients score higher for M2 macrophages (32). One of the pro-tumorigenic properties of M2 macrophages is their ability to promote angiogenesis (58, 59). Indeed, M2 TAMs secrete various cytokines as well as matrix degrading enzymes that orchestrate not only cancer cell invasion, but also angiogenesis. In particular, a subset of M2 macrophages that expresses the tyrosine kinase receptor Tie2 produces large amounts of vascular endothelial growth factor (VEGF), which, in turn, regulates cancer cell dissemination (60, 61). Thus, macrophages serve as principal modifiers and regulators of blood vessel development and structure in the tumor microenvironment, suggesting that racial disparities in macrophage populations may indirectly shape the angiogenic milieu in different ethnic groups.
Myeloid-derived suppressor cells (MDSCs) represent well-established mediators of the immunosuppressive tumor microenvironment, and also serve as critical regulators of angiogenesis, cancer cell invasion and migration, as well as pre-metastatic niche formation (62–64). MDSCs are currently categorized into two distinct subtypes with clearly defined surface phenotype and functions, the granulocytic (G-MDSC) and the monocytic (Mo-MDSC) types (65).The levels of G-MDSCs in the peripheral blood of breast cancer patients receiving neo-adjuvant chemotherapy (doxorubicin and cyclophosphamide) are significantly elevated, especially for those who do not present with pathologic complete response (pCR) (66). Interestingly, this study additionally demonstrated that AA patients present with a comparably lower increase in G-MDSC levels following chemotherapy, compared to Caucasians (66).These observations suggest that racial disparities in MDSC responses and functions in the breast TME, especially in the context of chemotherapy treatment, may account for significant differences in tumor progression and even therapeutic outcome among different ethnic backgrounds.
Disparity in Breast Cancer Vascular Compartment
Microvascular density has been consistently associated with tumor progression and outcome in breast cancer (67), because blood vessels are critical for the development and progression of the primary breast cancer, cancer cell dissemination to distant sites (60, 68, 69), metastatic seeding, as well as outgrowth of metastatic nodules. Angiogenesis is a complex process regulated by a plethora of cytokines produced in response to hypoxia-induced activation of HIF-1 transcription factors (70). Given such importance of vasculature in tumor progression, it is not surprising given the disparity in outcome described above that one of the most striking differences in TME between patients of African and European ancestry is in the biology of angiogenesis (55). A comprehensive study by Martin et al. (55) looked at differences in gene enrichment in specific biological processes in tumor stroma and tumor epithelium, separated by laser capture micro-dissection, between black and white patients. The study found that patients of African ancestry had significantly higher expression of genes involved in cell cycle control and chemotaxis in tumor epithelium, while tumor stroma was enriched for genes involved in neovascularization. This study also found increased microvascular density in TME from AA patients. Interestingly however, an analysis of the National Cancer Database (NCDB) found that black race was not associated with higher risk of lymphovascular invasion in patients with early ER+/HER2- breast cancer (71). Given that a subset of TAMs stimulates angiogenesis, it is plausible that breast cancers in black patients release more macrophage chemotactic signals such as CSF-1, which could result in macrophage recruitment, increased density of proangiogenic TAMs and subsequent increase in microvascular density. Indeed, plasma levels of granulocyte colony-stimulating factor (G-CSF) was found to be elevated in African-American compared with Caucasian patients (72). Increased microvascular density along with increased density of proangiogenic CD206 expressing macrophages within TME likely contribute to an enhanced assembly of specialized doorways for cancer cell dissemination to distant sites called tumor microenvironment of metastasis (TMEM) doorways (60, 61). TMEM doorways are sites of localized transient vascular permeability. Each TMEM doorway is composed of one proangiogenic CD206 macrophage expressing high levels of Tie2 receptor, one tumor cell expressing high levels of actin regulatory protein Mena, and one endothelial cell expressing angiopoietin-2 (Ang2), all in direct physical contact (60). The TMEM doorway is a clinically validated prognostic biomarker for breast cancer metastasis to distant sites such as lung, bone or brain (73–75). It would be interesting to investigate if the density of TMEM doorways differs in breast TME of patients from different racial ancestry and if the difference in TMEM density contributes to the disparity in breast cancer outcome.
Cancer-Associated Fibroblasts (CAFs), Extracellular Matrix (ECM), and Breast Density in Patients of Different Racial/Ethnic Backgrounds
Cancer-associated fibroblasts (CAFs) are the most common stromal cell type of mesenchymal origin in the tumor microenvironment (76). However, due to the lack of molecular markers specific for CAFs, it is challenging to identify and study them (77). Nevertheless, it has been observed that breast CAFs secrete a large number of growth factors such as fibroblast growth factor (FGF), transforming growth factor beta (TGF-β), CXCL12, and hepatocyte growth factor (HGF), as well as various cytokines that contribute to cancer cell proliferation, invasiveness and angiogenesis (76). While, to the best of our knowledge, the effect of CAFs on cancer progression from patients of different racial backgrounds has not been investigated, one study describes the effect of ECM and fibroblasts isolated from healthy pre-menopausal women of various racial backgrounds on breast cancer cell growth and invasion both in vivo and in vitro (78). This study reports that fibroblasts from both AA and EA women enhanced cancer progression albeit in slightly different ways. In vitro, ECM from AA women induced invasiveness of TN cancer cells, while fibroblasts from EA women induced invasiveness of ER+/PR+ cancer cells. In xenograft models, ECM from EA women increased tumorigenesis of ER+/PR+ cells and enhanced metastasis. However, in vitro studies must be viewed with caution since in vitro assays suffer from uncertainty regarding the lack of TME associated factors which can lead to the observation of cell phenotypes that are unrelated to cell behavior in vivo.
According to several studies it seems that single nucleotide polymorphisms (SNPs) in the FGF family of genes may influence the risk for breast cancer in patients of various racial backgrounds. In particular, SNP variants in the fibroblast growth factor receptor-2 (FGFR-2) gene and/ or the FGFR-2 promoter are associated with an increased risk of breast cancer in Chinese women (79, 80), Northern Indian (81), Caucasian (82), and AA women (83). Thus, it is plausible that there are racial differences in which fibroblasts affect cancer susceptibility and progression via the secretion of protumoral and prometastatic cytokines.
Likewise, CAFs and ECM may affect breast tissue density, which has important clinical implication not only for cancer progression but also for mammographic screening. Indeed, in a large multiethnic study, it was shown that women of Hispanic ancestry had the highest mammographic breast density, followed by AA and EA women (84). To what extent this CAF-related phenotype is affected by differential deposition of collagen, collagen crosslinking, or regulation of interstitial pressure among the different racial groups needs to be determined using in vivo studies in the future.
Cancer-Associated Adipocytes in Patients of Different Racial/Ethnic Backgrounds
The interplay between cancer cells and adipocytes has not been extensively studied. This may potentially be due to the fact that adipocytes, which represent a large portion of the healthy breast tissue, are frequently replaced by desmoplastic stroma during cancer progression. Nevertheless, cancer cells often invade the surrounding adipose tissues and such interplay may affect breast cancer outcome (85). Indeed, several studies indicate a positive correlation between cancer cell invasion into adipose breast tissue and poor patient outcome (86, 87). Recently, a microanatomical adipocyte-associated structure called crown-like structure (CLS) was described in breast TME (54). CLS are composed of macrophages surrounding dying adipocytes. A higher density of CLS was found in black compared to Caucasian and non-black Hispanic patients (54). Interestingly, CLS containing pro-inflammatory M1 macrophages are associated with worse survival in all racial groups. Thus, adipocytes may affect cancer outcome by influencing cancer behavior locally, as shown in several in vitro studies (88). Alternatively, adipocytes may be affecting overall inflammation at the systemic level, which is also cancer-promoting (89, 90). Since AA race is associated with higher obesity rates compared to EA (91), and obesity induces low-grade chronic inflammatory milieu, it is possible that CLSs are more frequently associated with M1 macrophages in AA than in EA patients due to obesity-induced inflammation. Indeed, obesity is not only associated with increased circulating fatty acids, but also with enrichment of chemo-attractants for immune cells into the TME (92). In particular, adipose tissue produces inflammatory cytokines such as TNF-α, interleukin (IL-6), IL-1β, and monocyte chemoattractant protein (MCP)-1. Moreover, adipocytes transdifferentiate into macrophages, which can be stimulated by fatty acids to produce inflammatory cytokines. High cytokine levels perpetuate chronic inflammation, which in turn, can promote tumor progression. Therefore, the interplay between TME and circulating cytokines may be responsible for the association of obesity with worse outcome in patients with breast cancer (93).
Serum Cytokine Profile in Breast Cancer Patients of Different Racial/Ethnic Backgrounds
Cytokines, the signaling molecules that mediate and regulate immunity, inflammation and hematopoiesis, are the biological milieu and constitute important components of the TME associated with breast cancer (94, 95). Cytokines have been used as biomarkers for prognosis and have been associated with clinical symptoms and adverse outcomes in breast cancer (95).
Studies indicate that certain cytokine levels may be influenced by racial background of the patient. For instance, plasma levels of IL-8 and granulocyte colony-stimulating factor were elevated in AAs compared with EAs (72), and TNF-α has been reported to be higher in non-obese Mexican Americans compared with matched non-Hispanic whites (96). Moreover, it was also demonstrated that plasma levels of circulating cytokines are influenced by both age and race (97). Most studies comparing racial differences in cancer at the cytokine levels investigated only a few cytokines. The reason may be the lack of sufficient numbers of AA patients in population-based case-control studies to observe significant differences in circulating cytokines and race-specific associations between cytokines and cancer (98). Studies in various cancer types demonstrated that there are substantial racial differences in inflammation between AA and EA patients. In lung cancer for instance, certain cytokines (IL-4, IL-5, IL-8, IL-10, IFN-γ, and TNF-α) were significantly elevated among EA compared to AA patients, whereas elevated IL-1β, IL-10, and TNF-α levels were associated with lung cancer only among AA patients (98). In other studies, AA compared to EA patients appeared to have higher levels of circulating C-reactive protein [a non-specific marker of inflammation (99)], higher levels of IL-6, and reduced levels of TNF-α (100). Of note, AA and EA patients were found to have significantly different frequencies of single nucleotide polymorphisms (SNPs) in cytokine genes, which may functionally alter and explain the differences in serum cytokine concentrations (99, 100).
A recent study demonstrated that race affects inflammatory cytokine levels (IL-6 and IFN-γ) and breast cancer risk. (101). Interestingly, other studies have shown that milk from healthy black women may contain higher levels of IL-1β than from white women even when controlled for BMI (102), which strengthens the hypothesis that increased inflammation within the breast of black women compared with white women may be linked to the higher rates of early onset breast cancer in black women (103). Therefore, potential strategies to reduce racial disparities in breast cancer risk could be through interventions such as short courses of anti-inflammatory agents (102). This is further supported by preclinical results reported by Lyons et al. (104) showing that a postpartum pro-inflammatory mechanism may promote development of aggressive breast cancer. Interestingly, TAMs, one of the major contributors of pro-inflammatory cytokines, are found in higher density in breast cancer specimens from AA compared to EA patients. Among other cytokines, TAMs produce resistin, which is the main mediator of obesity associated pro-inflammatory effects in various diseases, including cancer (105). Indeed, resistin, a main inducer of IL-6, was found to be expressed at greater levels in the TME of AA than of EA patients (106), specifically in breast cancer cells. This, in turn, may promote proliferation of breast cancer cells through STAT3 activation (105).
Since cytokines operate in integrated networks, a more complete understanding will be gained with the exploration and accurate measurements of multiple cytokines simultaneously (known as cytokine patterns or signatures), using advanced proteomic technologies (107). A wide range of cytokine assays is available for accurate measurements in biological fluids, e.g., immunoassays, cytokine bioassays, multiplex bead array assays, mass spectrometry, multi-parametric flow cytometry, among others (107). However, further research using bioanalytical techniques is needed to identify patterns of cytokine expression that may serve as biomarkers in clinical research, and to determine further differences in the cytokine landscape among patients of different racial backgrounds.
Racial/Ethnic Disparities in TME Elucidated Using High Throughput Tissue Analyses
Disparity in TME Gene Expression Pattern
Several groups have previously compared breast cancer gene expression patterns between AA and EA patients using high throughput approaches such as RNA sequencing and found differences in signaling pathways primarily related to angiogenesis, chemotaxis and immunity (32, 55). However, the two most significantly differentially expressed genes in the breast cancer epithelia between AA and EA patients are the phosphoserine phosphatase like (PSPHL) and Beta-crystallin B2 (CRYBB2) (55).Interestingly, PSPHL and CRYBB2 are also the most differentially expressed genes in prostate cancer patients from these two ancestral backgrounds (108). In fact, the racial ancestry of 94% of breast cancer epithelia could be correctly classified based only on the expression pattern of these 2 genes. The reasons for this are not understood.
A similar prediction could be made using five genes most differentially expressed in the breast cancer stroma, PSPHL, CXCL10, CXCL11, ISG20, and GMDS. Importantly, this separation was independent of estrogen receptor expression status. Interestingly, CXCL10, CXCL11, and ISG20 are IFN-γ-regulated genes, which is consistent with the presence of interferon signature found in breast cancer from AA patients (55). There are several reasons for the presence of interferon signature in tissues from AA, including chronic inflammation and/or presence of specific mutations in immune-related genes in tumors of AA patients (109). An extensive study by the Pusztai group performed a detailed analysis of immune gene expression in a multiracial patient cohort. The authors compared expression of 14 immune metagenes (patterns of gene expression) between AA and EA tumors, and found that although the median expression of all metagenes were higher in tumors from AA, only the major histocompatibility complex-1 (MHC1) was expressed at statistically significant higher levels. After looking deeper into the differences within tumor subtypes, it became evident that ER+ but not TN breast cancers from AA had higher median expression of the MHC1 metagene. Furthermore, the tumor immune dysfunction and exclusion (TIDE) analysis, which is used to assess the function and inclusion of T cells in the TME, showed only IFN-γ to be statistically higher in AA tumors, consistent with the presence of INF-γ signature in breast TME of AA women (55). Thus, IFN-γ network appears to be a main difference in breast cancer TME between AA and EA patients.
Disparity in Genomic Variations Affecting the TME
Racial differences in the immune TME have also been observed at a genomic level. It has been postulated and confirmed by several studies that populations with geographic ancestries that have been heavily exposed to environmental pathogens have variants in genes involved in innate immunity that protect them against infection, but negatively impact cancer incidence and progression (109). In a proof-of-principle, pilot study for example, it was shown that a Cypriot population displayed higher risk of developing cancer when there was a prior exposure to parasitic infections by Echinococcus granulosus (110). Such observations suggest that genomic variations may be prevalent in certain ethnic groups or patient populations of variable geographic origins, possibly as an inadvertent result of protection against local/endemic pathogens. Further evidence by Lazarus et al. (111) demonstrated that distinct SNPs patterns exist in innate immune genes in AA compared to EA patients. Likewise, Kwiatkowski et al. (111) found higher incidence of SNP variants in AA than in EA indicating that greater haplotype diversity exists within AA gene pool.
These observations collectively suggest that racial differences in transcriptomic/genomic landscape are indeed prevalent among breast cancer patients, which partially explain the intrinsic differences in the tumor microenvironment composition and disease progression.
Can Racial/Ethnic Disparities in Breast TME Help Personalize Breast Cancer Therapy?
The rationalized targeting of the tumor microenvironment has been proposed as early as the publication of the original “Hallmarks of Cancer” by Weinberg and Hanahan (112). In this review, we add a new dimension to this premise: different racial backgrounds are associated with different tumor microenvironments, which may partly explain the disparities in disease development and progression. This premise suggests that in the era of personalized oncology and rationalized targeting of the tumor microenvironment, race should clearly be taken into account as a major determinant of TME composition. Unfortunately, successful targeting of the components of TME have proven to be challenging. For example, anti-angiogenic drug bevacizumab (humanized monoclonal anti-VEGF antibody), failed to improve overall survival in either localized or metastatic breast cancer despite promising pre-clinical results (113). The key to successful bevacizumab treatment may lie in identifying patients with the appropriate TME, which could be racially determined (114). We postulate that studying racial disparities in the context of TME may facilitate identification of novel biomarkers for tailored treatment and for development of new therapeutics that specifically target the TME in AA. An example is the targeting of TMEM function using TMEM score as a prognostic for patients who would respond. Drugs specific for inhibition of macrophages supporting the assembly and function of TMEM its associated tumor cell dissemination, such as rebastinib (115), might present an opportunity if the TMEM score is elevated in AA patients.
Although prior epidemiologic and meta-analysis studies have documented that breast cancer patients treated with neoadjuvant (NAC) vs. adjuvant (AC) chemotherapy have no difference in survival (116, 117), a recent study by Pastoriza et al. (118) which stratified patients according to race, demonstrated that black patients treated with NAC have worse distant recurrence-free survival (DRFS) compared to matched white patients. Such racial disparities may partially be due to differences in the TME between AA and EA patients, including the increased density of prometastatic TAMs and microvascular density in black compared to white patients (54, 55). Since the main cause of breast cancer morbidity is metastatic disease, in addition to shrinking tumors with cytotoxic and anti-angiogenic therapies, targeting the sites of hematogenous dissemination at TMEM doorways may modify the TME and improve overall survival (115, 119, 120). Since AA compared to EA patients have higher microvascular and macrophage density as explained above, they may also have higher density of TMEM doorways, and thus respond better to anti-angiogenic and anti-TMEM therapy. Examining racial differences in TME may identify subpopulations of patients that do not receive full clinical benefit from current standardized therapies, and can define the need for novel, alternative treatment options in such patients.
Other promising therapies targeting the TME are immunotherapies (121). Although breast cancer is generally not highly immunogenic, the response to immunotherapies may vary according to the subtype: TN breast cancer, for example, is considered as the most immunogenic subtype, whereas ER+ disease is not (122). Since AA women tend to have higher incidence of TN disease as a population, one may speculate that AA patients may benefit more from immunotherapy. It would be interesting to evaluate if there is a racial disparity in patient response to immunotherapy. TCGA RNA sequencing data show significantly greater expression of the PD-L1 gene as compared to non-TNBC (123). Further studies established a link between androgen receptor (AR) expression in breast cancer and distinct gene signatures finding that those breast cancers with a lack of AR expression and triple negative biology had shorter time to progression and decreased overall survival with significantly elevated expression for immune checkpoint inhibitors PD-1, PD-L1, and CTLA 4. AR status was found to be a prognostic marker with increased capacity for AA patients (124). These findings show promise for the potential selective use of checkpoint inhibitors in this population. The lack of AR expression in the tumor can be used as a surrogate marker for increased expression in checkpoint inhibitors as PD-L1 expression in tumors has not been shown to be a reliable biomarker in regards to durable response to therapy (125). Further studies would need to be done in order to confirm whether AR status can be used in this way and if a correlation exists between AR expression and response to anti-PD-1/PD-L1-directed treatments.
Geographic Ancestry—The Ultimate Culprit for Disparity in TME of Breast Cancer?
Disparities encountered in the TME are a part of the dynamic interplay between local and systemic factors. As discussed above, the most pronounced differences in TME are associated with inflammation and angiogenesis. African ancestry is associated with higher inflammatory gene expression and enhanced bacterial clearance likely due to pathogen-rich geographic ancestry. While aggressive immune response is beneficial in defeating pathogens prevalent in certain geographical regions, it may also be promoting pro-tumorigenic properties in the TME as an unintended consequence. These protective innate immune variants are both disproportionately distributed among racial populations and are linked with racial disparities in cancer (109). Therefore, genetic and phenotypic characteristics that developed in response to environmental stressors specific to a particular geographic ancestry region may be the underlying cause for racial disparity in TME and ultimately outcome in patients with breast cancer (Figure 1) (126).
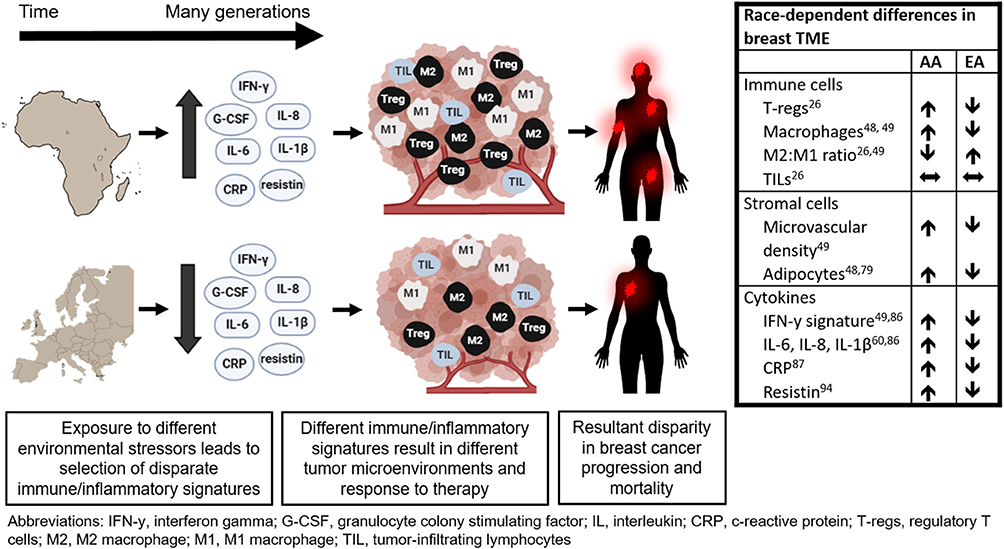
Figure 1. Potential link between tumor microenvironment and racial disparity in breast cancer outcome.
Conclusion
The TME is rapidly emerging as a key contributor to cancer progression, and patient outcome. The complex interplay between tumor cells and surrounding immune, vascular, and stromal components continue to be studied extensively. In this review, we highlight the racial differences in TME on cellular, molecular, and genetic levels. Furthermore, we explore systemic immune and cytokine signatures as contributors to the racial disparity in TME. The awareness of these differences and further research will lead to development of race-specific biomarkers and therapeutic targets and ultimately improved personalized cancer treatment.
Author Contributions
GKa and MO contributed conception and design of the review. GKi, JP, GKa, and MO wrote sections of the manuscript. JS, JC, and PF provided critcial revisions to the content. All authors contributed to the article and approved the submitted version.
Funding
This research was supported by the Department of Health Peter T. Rowley Breast Cancer Research Grant (DOH01-ROWLEY-2019-00037), the National Institute of Health/National Institute of Health Environmental Sciences T32 Grant (T32CA200561), Gruss-Lipper Biophotonics Center and its associated Integrated Imaging Program at Einstein College of Medicine, and Jane A. and Myles P. Dempsey.
Conflict of Interest
The authors declare that the research was conducted in the absence of any commercial or financial relationships that could be construed as a potential conflict of interest.
References
1. DeSantis CE, Fedewa SA, Goding Sauer A, Kramer JL, Smith RA, Jemal A. Breast cancer statistics, 2015: convergence of incidence rates between black and white women. CA Cancer J Clin. (2016) 66:31–42. doi: 10.3322/caac.21320
2. DeSantis CE, Ma J, Gaudet MM, Newman LA, Miller KD, Goding Sauer A, et al. Breast cancer statistics, (2019). CA Cancer J Clin. (2019) 69:438–51. doi: 10.3322/caac.21583
3. Albain KS, Unger JM, Crowley JJ, Coltman CA Jr, Hershman DL. Racial disparities in cancer survival among randomized clinical trials patients of the Southwest oncology group. J Natl Cancer Inst. (2009) 101:984–92. doi: 10.1093/jnci/djp175
4. Bach PB, Schrag D, Brawley OW, Galaznik A, Yakren S, Begg CB. Survival of blacks and whites after a cancer diagnosis. JAMA. (2002) 287:2106–13. doi: 10.1001/jama.287.16.2106
5. Crew KD, Neugut AI, Wang X, Jacobson JS, Grann VR, Raptis G, et al. Racial disparities in treatment and survival of male breast cancer. J Clin Oncol. (2007) 25:1089–98. doi: 10.1200/JCO.2006.09.1710
6. Newman LA, Mason J, Cote D, Vin Y, Carolin K, Bouwman D, et al. African-American ethnicity, socioeconomic status, and breast cancer survival: a meta-analysis of 14 studies involving over 10,000 African-American and 40,000 White American patients with carcinoma of the breast. Cancer. (2002) 94:2844–54. doi: 10.1002/cncr.10575
7. Joslyn SA, West MM. Racial differences in breast carcinoma survival. Cancer. (2000) 88:114–23. doi: 10.1002/(SICI)1097-0142(20000101)88:1<114::AID-CNCR16>3.0.CO;2-J
8. Carey LA, Perou CM, Livasy CA, Dressler LG, Cowan D, Conway K, et al. Race, breast cancer subtypes, and survival in the carolina breast cancer study. JAMA. (2006) 295:2492–502. doi: 10.1001/jama.295.21.2492
9. Sparano JA, Wang M, Zhao F, Stearns V, Martino S, Ligibel JA, et al. Race and hormone receptor-positive breast cancer outcomes in a randomized chemotherapy trial. J Natl Cancer Inst. (2012) 104:406–14. doi: 10.1093/jnci/djr543
10. Hershman D, McBride R, Jacobson JS, Lamerato L, Roberts K, Grann VR, et al. Racial disparities in treatment and survival among women with early-stage breast cancer. J Clin Oncol. (2005) 23:6639–46. doi: 10.1200/JCO.2005.12.633
11. Partridge AH, Wang PS, Winer EP, Avorn J. Nonadherence to adjuvant tamoxifen therapy in women with primary breast cancer. J Clin Oncol. (2003) 21:602–6. doi: 10.1200/JCO.2003.07.071
12. Schneider BP, Lai D, Shen F, Jiang G, Radovich M, Li L, et al. Charcot-marie-tooth gene, SBF2, associated with taxane-induced peripheral neuropathy in African Americans. Oncotarget. (2016) 7:82244–53. doi: 10.18632/oncotarget.12545
13. Kabat GC, Ginsberg M, Sparano JA, Rohan TE. Risk of recurrence and mortality in a multi-ethnic breast cancer population. J Racial Ethn Health Disparities. (2017) 4:1181–8. doi: 10.1007/s40615-016-0324-y
14. Schneider BP, Li L, Radovich M, Shen F, Miller KD, Flockhart DA, et al. Genome-wide association studies for taxane-induced peripheral neuropathy in ECOG-5103 and ECOG-1199. Clin Cancer Res. (2015) 21:5082–91. doi: 10.1158/1078-0432.CCR-15-0586
15. Schneider BP, Shen F, Jiang G, O'Neill A, Radovich M, Li L, et al. Impact of genetic ancestry on outcomes in ECOG-ACRIN-E5103. JCO Precision Oncol. (2017) 2017:10.1200/PO.17.00059. doi: 10.1200/PO.17.00059
16. Tammemagi CM, Nerenz D, Neslund-Dudas C, Feldkamp C, Nathanson D. Comorbidity and survival disparities among black and white patients with breast cancer. JAMA. (2005) 294:1765–72. doi: 10.1001/jama.294.14.1765
17. Menashe I, Anderson WF, Jatoi I, Rosenberg PS. Underlying causes of the black-white racial disparity in breast cancer mortality: a population-based analysis. J Natl Cancer Inst. (2009) 101:993–1000. doi: 10.1093/jnci/djp176
18. Jatoi I, Becher H, Leake CR. Widening disparity in survival between white and African-American patients with breast carcinoma treated in the U. S. department of defense healthcare system. Cancer. (2003) 98:894–9. doi: 10.1002/cncr.11604
19. Albain K, Gray RJ, Sparano JA, Makower DF, Pritchard KI, Hayes DF, et al. Abstract GS4-07: Race, ethnicity and clinical outcomes in hormone receptor-positive, HER2-negative, node-negative breast cancer: results from the TAILORx trial. Cancer Res. (2019) 79:GS4-07. (2018). doi: 10.1158/1538-7445.SABCS18-GS4-07
20. Wojcik BE, Spinks MK, Optenberg SA. Breast carcinoma survival analysis for African American and white women in an equal-access health care system. Cancer. (1998) 82:1310–8. doi: 10.1002/(SICI)1097-0142(19980401)82:7<1310::AID-CNCR14>3.0.CO;2-9
21. Deshmukh SK, Srivastava SK, Tyagi N, Ahmad A, Singh AP, Ghadhban AAL, et al. Emerging evidence for the role of differential tumor microenvironment in breast cancer racial disparity: a closer look at the surroundings. Carcinogenesis. (2017) 38:757–65. doi: 10.1093/carcin/bgx037
22. van 't Veer LJ, Dai H, van de Vijver MJ, He YD, Hart AA, Mao M, et al. Gene expression profiling predicts clinical outcome of breast cancer. Nature. (2002) 415:530–6. doi: 10.1038/415530a
23. Cancer Genome Atlas N. Comprehensive molecular portraits of human breast tumours. Nature. (2012) 490:61–70. doi: 10.1038/nature11412
24. Stephens PJ, Tarpey PS, Davies H, Van Loo P, Greenman C, Wedge DC, et al. The landscape of cancer genes and mutational processes in breast cancer. Nature. (2012) 486:400–4. doi: 10.1038/nature11017
25. Mittal S, Brown NJ, Holen I. The breast tumor microenvironment: role in cancer development, progression and response to therapy. Expert Rev Mol Diagn. (2018) 18:227–43. doi: 10.1080/14737159.2018.1439382
26. Burugu S, Asleh-Aburaya K, Nielsen TO. Immune infiltrates in the breast cancer microenvironment: detection, characterization and clinical implication. Breast Cancer. (2017) 24:3–15. doi: 10.1007/s12282-016-0698-z
27. Adams S, Gray RJ, Demaria S, Goldstein L, Perez EA, Shulman LN, et al. Prognostic value of tumor-infiltrating lymphocytes in triple-negative breast cancers from two phase III randomized adjuvant breast cancer trials: ECOG 2197 and ECOG (1199). J Clin Oncol. (2014) 32:2959–66. doi: 10.1200/JCO.2013.55.0491
28. Mahmoud SM, Paish EC, Powe DG, Macmillan RD, Grainge MJ, Lee AH, et al. Tumor-infiltrating CD8+ lymphocytes predict clinical outcome in breast cancer. J Clin Oncol. (2011) 29:1949–55. doi: 10.1200/JCO.2010.30.5037
29. Loi S, Michiels S, Salgado R, Sirtaine N, Jose V, Fumagalli D, et al. Tumor infiltrating lymphocytes are prognostic in triple negative breast cancer and predictive for trastuzumab benefit in early breast cancer: results from the FinHER trial. Ann Oncol. (2014) 25:1544–50. doi: 10.1093/annonc/mdu112
30. Loi S, Sirtaine N, Piette F, Salgado R, Viale G, Van Eenoo F, et al. Prognostic and predictive value of tumor-infiltrating lymphocytes in a phase III randomized adjuvant breast cancer trial in node-positive breast cancer comparing the addition of docetaxel to doxorubicin with doxorubicin-based chemotherapy: BIG 02-98. J Clin Oncol. (2013) 31:860–7. doi: 10.1200/JCO.2011.41.0902
31. Storz P. Reactive oxygen species in tumor progression. Front Biosci. (2005) 10:1881–96. doi: 10.2741/1667
32. O'Meara T, Safonov A, Casadevall D, Qing T, Silber A, Killelea B, et al. Immune microenvironment of triple-negative breast cancer in African-American and Caucasian women. Breast Cancer Res Treat. (2019) 175:247–59. doi: 10.1007/s10549-019-05156-5
33. Lehmann BD, Jovanovic B, Chen X, Estrada MV, Johnson KN, Shyr Y, et al. Refinement of triple-negative breast cancer molecular subtypes: implications for neoadjuvant chemotherapy selection. PLoS ONE. (2016) 11:e0157368. doi: 10.1371/journal.pone.0157368
34. Bohling SD, Allison KH. Immunosuppressive regulatory T cells are associated with aggressive breast cancer phenotypes: a potential therapeutic target. Mod Pathol. (2008) 21:1527–32. doi: 10.1038/modpathol.2008.160
35. Ohara M, Yamaguchi Y, Matsuura K, Murakami S, Arihiro K, Okada M. Possible involvement of regulatory T cells in tumor onset and progression in primary breast cancer. Cancer Immunol Immunother CII. (2009) 58:441–7. doi: 10.1007/s00262-008-0570-x
36. Bos PD, Plitas G, Rudra D, Lee SY, Rudensky AY. Transient regulatory T cell ablation deters oncogene-driven breast cancer and enhances radiotherapy. J Exp Med. (2013) 210:2435–66. doi: 10.1084/jem.20130762
37. Fridlender ZG, Sun J, Kim S, Kapoor V, Cheng G, Ling L, et al. Polarization of tumor-associated neutrophil phenotype by TGF-beta: “N1” versus “N2” TAN. Cancer Cell. (2009) 16:183–94. doi: 10.1016/j.ccr.2009.06.017
38. Wei B, Yao M, Xing C, Wang W, Yao J, Hong Y, et al. The neutrophil lymphocyte ratio is associated with breast cancer prognosis: an updated systematic review and meta-analysis. Onco Targets Therapy. (2016) 9:5567–75. doi: 10.2147/OTT.S108419
39. Chen J, Deng Q, Pan Y, He B, Ying H, Sun H, et al. Prognostic value of neutrophil-to-lymphocyte ratio in breast cancer. FEBS Open Bio. (2015) 5:502–7. doi: 10.1016/j.fob.2015.05.003
40. Ethier JL, Desautels D, Templeton A, Shah PS, Amir E. Prognostic role of neutrophil-to-lymphocyte ratio in breast cancer: a systematic review and meta-analysis. Breast Cancer Res BCR. (2017) 19:2. doi: 10.1186/s13058-016-0794-1
41. Spiegel A, Brooks MW, Houshyar S, Reinhardt F, Ardolino M, Fessler E, et al. Neutrophils suppress intraluminal NK cell-mediated tumor cell clearance and enhance extravasation of disseminated carcinoma cells. Cancer Discov. (2016) 6:630–49. doi: 10.1158/2159-8290.CD-15-1157
42. Park J, Wysocki RW, Amoozgar Z, Maiorino L, Fein MR, Jorns J, et al. Cancer cells induce metastasis-supporting neutrophil extracellular DNA traps. Sci Transl Med. (2016) 8:361ra138. doi: 10.1126/scitranslmed.aag1711
43. Grann VR, Bowman N, Joseph C, Wei Y, Horwitz MS, Jacobson JS, et al. Neutropenia in 6 ethnic groups from the caribbean and the U.S. Cancer. (2008) 113:854–60. doi: 10.1002/cncr.23614
44. Ojalvo LS, King W, Cox D, Pollard JW. High-density gene expression analysis of tumor-associated macrophages from mouse mammary tumors. Am J Pathol. (2009) 174:1048–64. doi: 10.2353/ajpath.2009.080676
45. Ojalvo LS, Whittaker CA, Condeelis JS, Pollard JW. Gene expression analysis of macrophages that facilitate tumor invasion supports a role for Wnt-signaling in mediating their activity in primary mammary tumors. J Immunol. (2010) 184:702–12. doi: 10.4049/jimmunol.0902360
46. Choi J, Gyamfi J, Jang H, Koo JS. The role of tumor-associated macrophage in breast cancer biology. Histol Histopathol. (2018) 33:133–45. doi: 10.14670/HH-11-916
47. Qiu SQ, Waaijer SJH, Zwager MC, de Vries EGE, van der Vegt B, Schroder CP. Tumor-associated macrophages in breast cancer: innocent bystander or important player? Cancer Treat Rev. (2018) 70:178–89. doi: 10.1016/j.ctrv.2018.08.010
48. Mills CD. Anatomy of a discovery: m1 and m2 macrophages. Front Immunol. (2015) 6:212. doi: 10.3389/fimmu.2015.00212
49. Italiani P, Boraschi D. From monocytes to M1/M2 macrophages: phenotypical vs. functional differentiation. Front Immunol. (2014) 5:514. doi: 10.3389/fimmu.2014.00514
50. Lin EY, Pollard JW. Role of infiltrated leucocytes in tumour growth and spread. Br J Cancer. (2004) 90:2053–8. doi: 10.1038/sj.bjc.6601705
51. Zhao X, Qu J, Sun Y, Wang J, Liu X, Wang F, et al. Prognostic significance of tumor-associated macrophages in breast cancer: a meta-analysis of the literature. Oncotarget. (2017) 8:30576–86. doi: 10.18632/oncotarget.15736
52. Jung KY, Cho SW, Kim YA, Kim D, Oh BC, Park DJ, et al. Cancers with higher density of tumor-associated macrophages were associated with poor survival rates. J Pathol Transl Med. (2015) 49:318–24. doi: 10.4132/jptm.2015.06.01
53. Carrio R, Koru-Sengul T, Miao F, Gluck S, Lopez O, Selman Y, et al. Macrophages as independent prognostic factors in small T1 breast cancers. Oncol Rep. (2013) 29:141–8. doi: 10.3892/or.2012.2088
54. Koru-Sengul T, Santander AM, Miao F, Sanchez LG, Jorda M, Gluck S, et al. Breast cancers from black women exhibit higher numbers of immunosuppressive macrophages with proliferative activity and of crown-like structures associated with lower survival compared to non-black latinas and caucasians. Breast Cancer Res Treat. (2016) 158:113–26. doi: 10.1007/s10549-016-3847-3
55. Martin DN, Boersma BJ, Yi M, Reimers M, Howe TM, Yfantis HG, et al. Differences in the tumor microenvironment between African-American and European-American breast cancer patients. PLoS ONE. (2009) 4:e4531. doi: 10.1371/journal.pone.0004531
56. Pollard JW. Tumour-educated macrophages promote tumour progression and metastasis. Nat Rev Cancer. (2004) 4:71–8. doi: 10.1038/nrc1256
57. Noy R, Pollard JW. Tumor-associated macrophages: from mechanisms to therapy. Immunity. (2014) 41:49–61. doi: 10.1016/j.immuni.2014.06.010
58. Sanchez LR, Borriello L, Entenberg D, Condeelis JS, Oktay MH, Karagiannis GS. The emerging roles of macrophages in cancer metastasis and response to chemotherapy. J Leukoc Biol. (2019) 106:259–74. doi: 10.1002/JLB.MR0218-056RR
59. Jetten N, Verbruggen S, Gijbels MJ, Post MJ, De Winther MP, Donners MM. Anti-inflammatory M2, but not pro-inflammatory M1 macrophages promote angiogenesis in vivo. Angiogenesis. (2014) 17:109–18. doi: 10.1007/s10456-013-9381-6
60. Harney AS, Arwert EN, Entenberg D, Wang Y, Guo P, Qian BZ, et al. Real-time imaging reveals local, transient vascular permeability, and tumor cell intravasation stimulated by TIE2hi macrophage-derived VEGFA. Cancer Discov. (2015) 5:932–43. doi: 10.1158/2159-8290.CD-15-0012
61. Karagiannis GS, Goswami S, Jones JG, Oktay MH, Condeelis JS. Signatures of breast cancer metastasis at a glance. J Cell Sci. (2016) 129:1751–8. doi: 10.1242/jcs.183129
62. Safarzadeh E, Orangi M, Mohammadi H, Babaie F, Baradaran B. Myeloid-derived suppressor cells: important contributors to tumor progression and metastasis. J Cell Physiol. (2018) 233:3024–36. doi: 10.1002/jcp.26075
63. Kumar V, Patel S, Tcyganov E, Gabrilovich DI. The nature of myeloid-derived suppressor cells in the tumor microenvironment. Trends Immunol. (2016) 37:208–20. doi: 10.1016/j.it.2016.01.004
64. Trovato R, Cane S, Petrova V, Sartoris S, Ugel S, De Sanctis F. The engagement between MDSCs and metastases: partners in crime. Front Oncol. (2020) 10:165. doi: 10.3389/fonc.2020.00165
65. Bergenfelz C, Leandersson K. The generation and identity of human myeloid-derived suppressor cells. Front Oncol. (2020) 10:109. doi: 10.3389/fonc.2020.00109
66. Wesolowski R, Duggan MC, Stiff A, Markowitz J, Trikha P, Levine KM, et al. Circulating myeloid-derived suppressor cells increase in patients undergoing neo-adjuvant chemotherapy for breast cancer. Cancer Immunol Immunother. (2017) 66:1437–47. doi: 10.1007/s00262-017-2038-3
67. Carmeliet P, Jain R. Angiogenesis in cancer and other diseases. Nature. (2000) 407:249–57. doi: 10.1038/35025220
68. Brown M, Assen FP, Leithner A, Abe J, Schachner H, Asfour G, et al. Lymph node blood vessels provide exit routes for metastatic tumor cell dissemination in mice. Science. (2018) 359:1408–11. doi: 10.1126/science.aal3662
69. Pereira ER, Kedrin D, Seano G, Gautier O, Meijer EFJ, Jones D, et al. Lymph node metastases can invade local blood vessels, exit the node, and colonize distant organs in mice. Science. (2018) 359:1403–7. doi: 10.1126/science.aal3622
70. Carmeliet P, Dor Y, Herbert JM, Fukumura D, Brusselmans K, Dewerchin M, et al. Role of HIF-1alpha in hypoxia-mediated apoptosis, cell proliferation and tumour angiogenesis. Nature. (1998) 394:485–90. doi: 10.1038/28867
71. Della Makower JL, Xue X, Sparano J. Lymphovascular invasion (LVI), the 21-gene recurrence score, and race in early estrogen receptor-positive, HER2-negative breast cancer: a national cancer database (NCDB) analysis. Proceddings of the 2019. San Antonio Breast Cancer Symposium 2019. San Antonio, TX, Philadelphia, PA: AACR (2020). doi: 10.1158/1538-7445.SABCS19-P3-08-06
72. Mayr FB, Spiel AO, Leitner JM, Firbas C, Kliegel T, Jilma B. Ethnic differences in plasma levels of interleukin-8 (IL-8) and granulocyte colony stimulating factor (G-CSF). Transl Res. (2007) 149:10–4. doi: 10.1016/j.trsl.2006.06.003
73. Robinson BD, Sica GL, Liu YF, Rohan TE, Gertler FB, Condeelis JS, et al. Tumor microenvironment of metastasis in human breast carcinoma: a potential prognostic marker linked to hematogenous dissemination. Clin Cancer Res. (2009) 15:2433–41. doi: 10.1158/1078-0432.CCR-08-2179
74. Rohan TE, Xue X, Lin HM, D'Alfonso TM, Ginter PS, Oktay MH, et al. Tumor microenvironment of metastasis and risk of distant metastasis of breast cancer. J Natl Cancer Inst. (2014) 106:dju136. doi: 10.1093/jnci/dju136
75. Sparano JA, Gray R, Oktay MH, Entenberg D, Rohan T, Xue X, et al. A metastasis biomarker (MetaSite Breast Score) is associated with distant recurrence in hormone receptor-positive, HER2-negative early-stage breast cancer. Nat PJ Breast Cancer. (2017) 3:42. doi: 10.1038/s41523-017-0043-5
76. Qiao A, Gu F, Guo X, Zhang X, Fu L. Breast cancer-associated fibroblasts: their roles in tumor initiation, progression and clinical applications. Front Med. (2016) 10:33–40. doi: 10.1007/s11684-016-0431-5
77. Buchsbaum RJ, Oh SY. Breast cancer-associated fibroblasts: where we are and where we need to go. Cancers. (2016) 8:19. doi: 10.3390/cancers8020019
78. Fleming JM, Miller TC, Quinones M, Xiao Z, Xu X, Meyer MJ, et al. The normal breast microenvironment of premenopausal women differentially influences the behavior of breast cancer cells in vitro and in vivo. BMC Med. (2010) 8:27. doi: 10.1186/1741-7015-8-27
79. Pan Z, Bao Y, Zheng X, Cao W, Cheng W, Xu X. Association of polymorphisms in intron 2 of FGFR2 and breast cancer risk in capital ES, Cyrillichinese women. Tsitol Genet. (2016) 50:59–64. doi: 10.3103/S009545271605008X
80. Yang YB, Zhao ZX, Huang W, Liu H, Tan YL, Wang WM. Association between fibroblast growth factor receptor-2 gene polymorphism and risk of breast cancer in Chinese populations: a HuGE review and meta-analysis. J Cancer Res Ther. (2016) 12:543–9. doi: 10.4103/0973-1482.148715
81. Siddiqui S, Chattopadhyay S, Akhtar MS, Najm MZ, Deo SV, Shukla NK, et al. A study on genetic variants of Fibroblast growth factor receptor 2 (FGFR2) and the risk of breast cancer from North India. PLoS ONE. (2014) 9:e110426. doi: 10.1371/journal.pone.0110426
82. Zhou L, Yao F, Luan H, Wang Y, Dong X, Zhou W, et al. Three novel functional polymorphisms in the promoter of FGFR2 gene and breast cancer risk: a HuGE review and meta-analysis. Breast Cancer Res Treat. (2012) 136:885–97. doi: 10.1007/s10549-012-2300-5
83. Ruiz-Narvaez EA, Haddad SA, Lunetta KL, Yao S, Bensen JT, Sucheston-Campbell LE, et al. Gene-based analysis of the fibroblast growth factor receptor signaling pathway in relation to breast cancer in African American women: the AMBER consortium. Breast Cancer Res Treat. (2016) 155:355–63. doi: 10.1007/s10549-015-3672-0
84. Oppong BA, Dash C, O'Neill S, Li Y, Makambi K, Pien E, et al. Breast density in multiethnic women presenting for screening mammography. Breast J. (2018) 24:334–8. doi: 10.1111/tbj.12941
85. Tan J, Buache E, Chenard MP, Dali-Youcef N, Rio MC. Adipocyte is a non-trivial, dynamic partner of breast cancer cells. Int J Dev Biol. (2011) 55:851–9. doi: 10.1387/ijdb.113365jt
86. Kimijima I, Ohtake T, Sagara H, Watanabe T, Takenoshita S. Scattered fat invasion: an indicator for poor prognosis in premenopausal, and for positive estrogen receptor in postmenopausal breast cancer patients. Oncology. (2000) 59(Suppl. 1):25–30. doi: 10.1159/000055284
87. Yamaguchi J, Ohtani H, Nakamura K, Shimokawa I, Kanematsu T. Prognostic impact of marginal adipose tissue invasion in ductal carcinoma of the breast. Am J Clin Pathol. (2008) 130:382–8. doi: 10.1309/MX6KKA1UNJ1YG8VN
88. Dirat B, Bochet L, Dabek M, Daviaud D, Dauvillier S, Majed B, et al. Cancer-associated adipocytes exhibit an activated phenotype and contribute to breast cancer invasion. Cancer Res. (2011) 71:2455–65. doi: 10.1158/0008-5472.CAN-10-3323
89. Jiang X, Shapiro DJ. The immune system and inflammation in breast cancer. Mol Cell Endocrinol. (2014) 382:673–82. doi: 10.1016/j.mce.2013.06.003
90. Balkwill F, Mantovani A. Inflammation and cancer: back to virchow? Lancet. (2001) 357:539–45. doi: 10.1016/S0140-6736(00)04046-0
91. Byrd AS, Toth AT, Stanford FC. Racial disparities in obesity treatment. Curr Obes Rep. (2018) 7:130–8. doi: 10.1007/s13679-018-0301-3
92. Harvey AE, Lashinger LM, Hursting SD. The growing challenge of obesity and cancer: an inflammatory issue. Ann N Y Acad Sci. (2011) 1229:45–52. doi: 10.1111/j.1749-6632.2011.06096.x
93. Calle EE, Kaaks R. Overweight, obesity and cancer: epidemiological evidence and proposed mechanisms. Nat Rev Cancer. (2004) 4:579–91. doi: 10.1038/nrc1408
94. Dranoff G. Cytokines in cancer pathogenesis and cancer therapy. Nat Rev Cancer. (2004) 4:11–22. doi: 10.1038/nrc1252
95. Kawaguchi K, Sakurai M, Yamamoto Y, Suzuki E, Tsuda M, Kataoka TR, et al. Alteration of specific cytokine expression patterns in patients with breast cancer. Sci Rep. (2019) 9:2924. doi: 10.1038/s41598-019-39476-9
96. Ho RC, Davy KP, Hickey MS, Melby CL. Circulating tumor necrosis factor alpha is higher in non-obese, non-diabetic Mexican Americans compared to non-Hispanic white adults. Cytokine. (2005) 30:14–21. doi: 10.1016/j.cyto.2004.10.015
97. Stowe RP, Peek MK, Cutchin MP, Goodwin JS. Plasma cytokine levels in a population-based study: relation to age and ethnicity. J Gerontol A Biol Sci Med Sci. (2010) 65:429–33. doi: 10.1093/gerona/glp198
98. Pine SR, Mechanic LE, Enewold L, Bowman ED, Ryan BM, Cote ML, et al. Differential serum cytokine levels and risk of lung cancer between african and european americans. Cancer Epidemiol Biomarkers Prev. (2016) 25:488–97. doi: 10.1158/1055-9965.EPI-15-0378
99. Albert MA, Ridker PM. C-reactive protein as a risk predictor: do race/ethnicity and gender make a difference? Circulation. (2006) 114:e67–74. doi: 10.1161/CIRCULATIONAHA.106.613570
100. Van Dyke AL, Cote ML, Wenzlaff AS, Land S, Schwartz AG. Cytokine SNPs: comparison of allele frequencies by race and implications for future studies. Cytokine. (2009) 46:236–44. doi: 10.1016/j.cyto.2009.02.003
101. Park NJ, Kang DH. Inflammatory cytokine levels and breast cancer risk factors: racial differences of healthy Caucasian and African American women. Oncol Nurs Forum. (2013) 40:490–500. doi: 10.1188/13.ONF.40-05AP
102. Murphy J, Pfeiffer RM, Lynn BCD, Caballero AI, Browne EP, Punska EC, et al. Pro-inflammatory cytokines and growth factors in human milk: an exploratory analysis of racial differences to inform breast cancer etiology. Breast Cancer Res Treat. (2018) 172:209–19. doi: 10.1007/s10549-018-4907-7
103. Fornetti J, Martinson HA, Betts CB, Lyons TR, Jindal S, Guo Q, et al. Mammary gland involution as an immunotherapeutic target for postpartum breast cancer. J Mammary Gland Biol Neoplasia. (2014) 19:213–28. doi: 10.1007/s10911-014-9322-z
104. Lyons TR, O'Brien J, Borges VF, Conklin MW, Keely PJ, Eliceiri KW, et al. Postpartum mammary gland involution drives progression of ductal carcinoma in situ through collagen and COX-2. Nat Med. (2011) 17:1109–15. doi: 10.1038/nm.2416
105. Deshmukh SK, Srivastava SK, Bhardwaj A, Singh AP, Tyagi N, Marimuthu S, et al. Resistin and interleukin-6 exhibit racially-disparate expression in breast cancer patients, display molecular association and promote growth and aggressiveness of tumor cells through STAT3 activation. Oncotarget. (2015) 6:11231–41. doi: 10.18632/oncotarget.3591
106. Stewart PA, Luks J, Roycik MD, Sang QX, Zhang J. Differentially expressed transcripts and dysregulated signaling pathways and networks in African American breast cancer. PLoS ONE. (2013) 8:e82460. doi: 10.1371/journal.pone.0082460
107. Stenken JA, Poschenrieder AJ. Bioanalytical chemistry of cytokines–a review. Anal Chim Acta. (2015) 853:95–115. doi: 10.1016/j.aca.2014.10.009
108. Wallace TA, Prueitt RL, Yi M, Howe TM, Gillespie JW, Yfantis HG, et al. Tumor immunobiological differences in prostate cancer between African-American and European-American men. Cancer Res. (2008) 68:927–36. doi: 10.1158/0008-5472.CAN-07-2608
109. Yeyeodu ST, Kidd LR, Kimbro KS. Protective innate immune variants in racial/ethnic disparities of breast and prostate cancer. Cancer Immunol Res. (2019) 7:1384–9. doi: 10.1158/2326-6066.CIR-18-0564
110. Oikonomopoulou K, Yu H, Wang Z, Vasiliou SK, Brinc D, Christofi G, et al. Association between Echinococcus granulosus infection and cancer risk - a pilot study in cyprus. Clin Chem Lab Med. (2016) 54:1955–61. doi: 10.1515/cclm-2016-0125
111. Lazarus R, Vercelli D, Palmer LJ, Klimecki WJ, Silverman EK, Richter B, et al. Single nucleotide polymorphisms in innate immunity genes: abundant variation and potential role in complex human disease. Immunol Rev. (2002) 190:9–25. doi: 10.1034/j.1600-065X.2002.19002.x
112. Hanahan D, Weinberg RA. The hallmarks of cancer. Cell. (2000) 100:57–70. doi: 10.1016/S0092-8674(00)81683-9
113. Varella L, Abraham J, Kruse M. Revisiting the role of bevacizumab in the treatment of breast cancer. Semin Oncol. (2017) 44:273–85. doi: 10.1053/j.seminoncol.2017.10.010
114. Aalders KC, Tryfonidis K, Senkus E, Cardoso F. Anti-angiogenic treatment in breast cancer: facts, successes, failures and future perspectives. Cancer Treat Rev. (2017) 53:98–110. doi: 10.1016/j.ctrv.2016.12.009
115. Harney AS, Karagiannis GS, Pignatelli J, Smith BD, Kadioglu E, Wise SC, et al. The selective Tie2 inhibitor rebastinib blocks recruitment and function of Tie2(Hi) macrophages in breast cancer and pancreatic neuroendocrine tumors. Mol Cancer Ther. (2017) 16:2486–501. doi: 10.1158/1535-7163.MCT-17-0241
116. Rastogi P, Anderson SJ, Bear HD, Geyer CE, Kahlenberg MS, Robidoux A, et al. Preoperative chemotherapy: updates of national surgical adjuvant breast and bowel project protocols B-18 and B-27. J Clin Oncol. (2008) 26:778–85. doi: 10.1200/JCO.2007.15.0235
117. Mauri D, Pavlidis N, Ioannidis JP. Neoadjuvant versus adjuvant systemic treatment in breast cancer: a meta-analysis. J Natl Cancer Inst. (2005) 97:188–94. doi: 10.1093/jnci/dji021
118. Pastoriza JM, Karagiannis GS, Lin J, Lanjewar S, Entenberg D, Condeelis JS, et al. Black race and distant recurrence after neoadjuvant or adjuvant chemotherapy in breast cancer. Clin Exp Metastasis. (2018) 35:613–23. doi: 10.1007/s10585-018-9932-8
119. Karagiannis GS, Pastoriza JM, Wang Y, Harney AS, Entenberg D, Pignatelli J, et al. Neoadjuvant chemotherapy induces breast cancer metastasis through a TMEM-mediated mechanism. Sci Transl Med. (2017) 9:eaan0026. doi: 10.1126/scitranslmed.aan0026
120. Karagiannis GS, Condeelis JS, Oktay MH. Chemotherapy-induced metastasis: molecular mechanisms, clinical manifestations, therapeutic interventions. Cancer Res. (2019) 79:4567–76. doi: 10.1158/0008-5472.CAN-19-1147
121. Farkona S, Diamandis EP, Blasutig IM. Cancer immunotherapy: the beginning of the end of cancer? BMC Med. (2016) 14:73. doi: 10.1186/s12916-016-0623-5
122. Emens LA. Breast cancer immunotherapy: facts and hopes. Clin Cancer Res. (2018) 24:511–20. doi: 10.1158/1078-0432.CCR-16-3001
123. Mittendorf EA, Philips AV, Meric-Bernstam F, Qiao N, Wu Y, Harrington S, et al. PD-L1 expression in triple-negative breast cancer. Cancer Immunol Res. (2014) 2:361–70. doi: 10.1158/2326-6066.CIR-13-0127
124. Davis M, Tripathi S, Hughley R, He Q, Bae S, Karanam B, et al. AR negative triple negative or “quadruple negative” breast cancers in African American women have an enriched basal and immune signature. PLoS ONE. (2018) 13:e0196909. doi: 10.1371/journal.pone.0196909
125. Patel SP, Kurzrock R. PD-L1 expression as a predictive biomarker in cancer immunotherapy. Mol Cancer Ther. (2015) 14:847–56. doi: 10.1158/1535-7163.MCT-14-0983
Keywords: breast cancer, tumor microenvironment, breast cancer racial disparity, breast cancer outcome, tumor microenvironment of metastasis (TMEM)
Citation: Kim G, Pastoriza JM, Condeelis JS, Sparano JA, Filippou PS, Karagiannis GS and Oktay MH (2020) The Contribution of Race to Breast Tumor Microenvironment Composition and Disease Progression. Front. Oncol. 10:1022. doi: 10.3389/fonc.2020.01022
Received: 11 March 2020; Accepted: 22 May 2020;
Published: 30 June 2020.
Edited by:
Cesar Augusto Santa-Maria, Johns Hopkins Medicine, United StatesReviewed by:
Prashant Trikha, Nationwide Children's Hospital, United StatesDaniel Christian Hoessli, University of Karachi, Pakistan
Copyright © 2020 Kim, Pastoriza, Condeelis, Sparano, Filippou, Karagiannis and Oktay. This is an open-access article distributed under the terms of the Creative Commons Attribution License (CC BY). The use, distribution or reproduction in other forums is permitted, provided the original author(s) and the copyright owner(s) are credited and that the original publication in this journal is cited, in accordance with accepted academic practice. No use, distribution or reproduction is permitted which does not comply with these terms.
*Correspondence: Maja H. Oktay, maja.oktay@einsteinmed.org