- 1B-cell Neoplasia Unit, Division of Experimental Oncology, IRCCS San Raffaele Scientific Institute, Milan, Italy
- 2Functional Genomics of Cancer Unit, Division of Experimental Oncology, IRCCS San Raffaele Scientific Institute, Milan, Italy
- 3Center for Omics Sciences (COSR), IRCCS San Raffaele Scientific Institute, Milan, Italy
The role of the WNT signaling pathway in key cellular processes, such as cell proliferation, differentiation and migration is well documented. WNT signaling cascade is initiated by the interaction of WNT ligands with receptors belonging to the Frizzled family, and/or the ROR1/ROR2 and RYK families. The downstream signaling cascade results in the activation of the canonical β-catenin dependent pathway, ultimately leading to transcriptional control of cell proliferation, or the non-canonical pathway, mainly acting on cell migration and cell polarity. The high level of expression of both WNT ligands and WNT receptors in cancer cells and in the surrounding microenvironment suggests that WNT may represent a central conduit of interactions between tumor cells and microenviroment. In this review we will focus on WNT pathways deregulation in hematological cancers, both at the ligand and receptor levels. We will review available literature regarding both the classical β-catenin dependent pathway as well as the non-canonical pathway, with particular emphasis on the possible exploitation of WNT aberrant activation as a therapeutic target, a notion supported by preclinical data.
Introduction
The WNT signaling pathway is central for development and homeostasis within tissues. The WNT signaling cascade is initiated by the interaction of WNT proteins (lipid-modified secreted glycoproteins) with various receptors and co-receptors whose activation elicit several processes, such as cell proliferation, differentiation, apoptosis, polarity, migration and invasion (1, 2).
WNT proteins are evolutionary conserved and in mammals WNT family genes comprise 19 members. These secreted, cystein-rich proteins exert their effects through the interaction with the Frizzled family of proteins (consisting of 10 members). Frizzled (FZD) are seven-pass transmembrane, and each FZD protein contains a cysteine-rich domain (CRD) with approximately 10 cysteine residues, essential for the binding to WNT proteins (3, 4).
Apart from FZD, other proteins are involved in WNT recognition: the co-receptor low-density lipoprotein receptor-related proteins (LRP 5 and 6), the receptor Tyr kinase-like orphan receptor family (ROR1 and ROR2), the Receptor Tyrosine Kinase (RYK) and the protein Tyr kinase 7 (PTK7).
The WNT pathway is broadly divided into canonical (β-catenin-dependent) and non-canonical (β-catenin-independent), depending on the agonists, the receptors and the intracellular players involved. Given the high number of both WNT ligands and receptors, the possible combinations are numerous, making its regulation exceedingly complex. The activation of either signaling pathway is receptor and ligand dependent and yet is also influenced by the cellular context. As an approximation, certain WNTs (WNT1, WNT3A and WNT8) activate the canonical β-catenin-dependent WNT signaling pathway by preferentially binding to FZD receptor coupled to LRP5/6 co-receptors. Conversely, WNT5A and WNT11 preferentially bind to ROR1, ROR2 and RYK receptors and are predominantly involved in the β-catenin-independent, non-canonical pathway.
Irrespectively from the signaling branch activated upon binding of WNT ligands to FZD or other receptors, the signal is commonly transduced to a protein called Disheveled (DVL) that represents the molecular hub executing both the canonical and non-canonical pathways (5). How can the same DVL protein be involved in different signaling pathways? The molecular wiring underlying this behaviour remains unknown, but various mechanisms have been proposed to confer specificity to DVL activity, including different phosphorylation events on DVL or the establishment of alternative interactions between DVL and its protein partners. This latter hypothesis is supported by the presence of three conserved domains in DVL protein (namely DIX, DEP and PDZ) which mediate its interaction with effector proteins involved in different signaling branches. Indeed, it has been suggested that DIX and PDZ domains are implicated in the activation of β-catenin signaling, whereas DEP and PDZ are preferentially engaged in the interactions with proteins (e.g. Daam1, Prickle) that trigger the non-canonical signaling pathway (6).
WNT/β-Catenin Signaling
As mentioned earlier, FZD proteins act as main receptors for WNT ligands, together with the co-receptor LRP5/6.
In the absence of exogenous WNT, the pathway is maintained in the “off” state by the so-called “β-catenin destruction complex”. This multiprotein complex, composed by Axin, β-catenin, APC, GSK3 and CK1, keeps β-catenin at low levels by phosphorylating it, and thus marking it for recognition by β-TRCP ubiquitin ligase, leading to its subsequent ubitiquination and degradation (7, 8). When a WNT molecule binds to FZD, it induces the phosphorylation of LRP6 (9), and the association of DVL proteins to the receptor. Both these events that are required for initiation of the signaling cascade resulting in the exposure of DVL’s DIX domain that works as a docking site for Axin pulling it away from the destruction complex. Moreover, the cytoplasmic tail of LRP5/6 contains several PPPSPxS domains that can bind Axin and become a competing substrate for GSK3. As a result, the destruction complex is disassembled and β-catenin is no longer marked for destruction. Therefore, β-catenin accumulates in the cytoplasm and then translocates in the nucleus (10), where it associates with the TCF/LEF transcription factors and activates the transcription of target genes such as Myc, Cyclin D1, Survivin and MMP (11) mainly implicated in the regulation of cell proliferation (Figure 1).
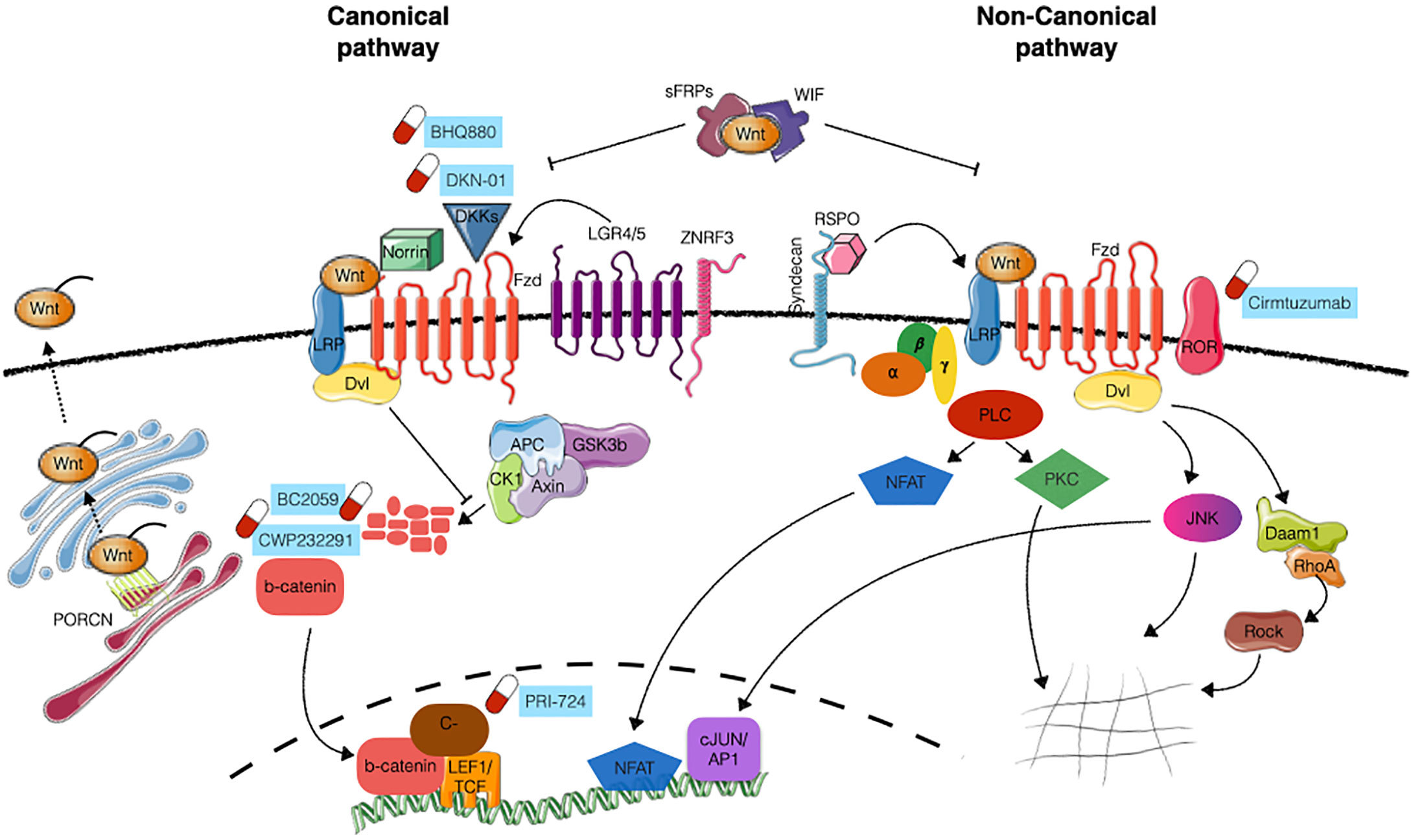
Figure 1 WNT pathaway signaling. Schematic representation of canonical (β-catenin dependent) and non-canonical (β-catenin independent) WNT signaling. WNT ligand interaction with FZD receptors together with LRP5/6 co-receptors inhibits the activity of the “destruction complex” (composed by Axin, APC, CK1 and GSK3β), allowing the stabilization of β-catenin, its translocation into the nucleus and the transcriptional activation of target genes. The activation of the non-canonical pathway involves additional co-receptors (e.g. ROR1 and ROR2) and is mediated by small GTPases RhoA, Daam1 and ROCK, acting on cytoskeleton remodelling, or PLC, JNK and NFAT, acting on transcriptional activity. Further layers of regulation of the pathway are exerted at the level of WNT ligands modification/secretion (mainly through PRCN), engagement of additional activator proteins (Norrin, Syndecans, RSPO, LGR4/5, ZNRF3) or the presence of antagonists (DKK, WIF, sFRPs). Compounds under clinical evaluation are indicated next to the appropriate target. Images have been created with Servier Medical Art (https://smart.servier.com/).
Non-Canonical WNT Signaling
The interactions of other WNT ligands (such as WNT5A and WNT11) and receptors (including ROR1 and ROR2) results in the activation of alternative signaling routes different from the canonical β-catenin dependent pathway, known as WNT non-canonical pathways. The first example of a non-canonical pathway is the Planar Cell Polarity (PCP)/Jun N-terminal kinase (JNK) signaling cascade that involves the activation of JNK, Rho, Rac and Rho kinase (ROCK) proteins (12). In this context, WNT ligand binding to FZD receptor stimulates the recruitment of DVL, which contrarily to what happens in the canonical-pathway, interacts with DAAM1 (Disheveled associated activator of morphogenesis 1) thereby stimulating Rac and Rho small GTPases and JNK, whose activation results in actin polymerization and cytoskeletal modifications (13) (Figure 1). In addition to the PCP pathway, another significant noncanonical pathway is the WNT/Ca2+ pathway, characterized by an increase in the intracellular calcium level as a result of WNT binding. This pathway subsequently activates heterotrimeric G proteins and Phospholipase-C (PLC) which hydrolyses membrane phospholipids to di-acyl glycerol and inositol 1,4,5-triphosphate (IP3). This neo-generated IP3 causes the release of calcium from the endoplasmic reticulum which activates Protein kinase C (PKC) and ultimately activating calcineurin and NFAT transcription factor, thus regulating transcriptional programs involved in cell fate and cell migration (14) (Figure 1).
Regulation of WNT Signaling by Additional Agonists/Antagonists
The fine-tuning of WNT signaling activity is also determined by additional proteins that can either stimulate or inhibit WNT pathway. Two major families of growth factors are known to act as WNT agonists: Norrin, and R-spondins (RSPOs). As for the antagonists they can be broadly divided in two categories: the one interfering with WNT ligand (Secreted frizzled-related proteins- sFPRs, Wnt inhibitory factor- WIF) or other binding to the receptor complex (Dickkopf-related proteins- DKKs) (15).
WNT agonists: R-Spondin family of growth factors consists of 4 members (RSPO1 to 4), acting on both canonical (β-catenin dependent) and non-canonical signaling. Their mechanism of action is still controversial but it is suggested that RSPO proteins bind to leuchin-rich G protein 4 and 5 (LRG-4 and 5) to sequester ZNRF3, an E3 ubiquitin ligase involved in the turn-over of Fz-LRP6 receptor complex. By sequestering ZNRF3, RSPO-LRG interaction induces the accumulation of the receptor complex on the cell surface, thus enhancing WNT signaling (16). RSPOs can also amplify non-canonical PCP signaling, likely through the interaction of RSPO/Syndecans with WNT/Fz (17).
Another secreted factor capable of enhancing WNT activation is Norrin which was described to interact with Fz4 (18, 19) and to specifically activate canonical signaling.
WNT antagonists: The largest family of secreted WNT inhibitors is represented by sFRPs. In humans, it comprises five members (sFRP1-5), all sharing a conserved CRD at the N-terminus. They act binding WNT ligands and preventing their interaction with the receptor, although mouse sFRP3 has been shown to interact with FZD8 (20), suggesting an additional mode of inhibition (21). They potentially bind to different WNT proteins, so they can inhibit both canonical and non-canonical signaling.
WNT Inhibitor factor 1 (WIF-1) is another secreted factor that acts binding to WNT ligands and preventing their interaction with the receptors. In this way, WIF-1 can inhibit both canonical and non-canonical signaling.
Lastly, the DKK family includes four members (DKK1 to 4), with DDK1, 2 and 4 binding to LRP5/6 and inhibiting the canonical β-catenin pathway. DDK3 does not seem to bind to LRP5/6 and is likely acting on a different pathway such as the TGF-β one (22).
An Additional Layer of Regulation: Secretion of WNT Ligands
WNTS are secreted proteins exerting their function on a short- or long-range gradient in the neighboring cells. Their proper secretion and release in the extracellular space represents an additional level of signaling modulation. Indeed, WNT proteins need indeed to be posttranslational modified in order to be exported and to be biologically active. One of the most crucial modification is acylation, critical for the transportation from the Golgi to the cell surface (23) and for the correct and efficient binding to FZD receptors (24). WNT acylation occurs through the concerted action of three enzymes: stearoyl CoA desaturase (SCD), porcupine (PORCN) and Notum. Of these enzymes, the most studied is PORCN, a membrane-bound O-acyltransferase that acylates WNT molecules at specific sites. Given the prevalence of WNT signaling dysregulation in cancer, the search for drugs that specifically inhibit PORCN represents a possible treatment in those malignancies where WNT signaling is altered (25).
WNT Signaling in Hematological Cancer
The high level of expression of both WNT ligands and WNT receptors in cancer suggests that WNT may represent a central conduit of interactions between tumor cells and the microenvironment. Although most of the studies of WNT involvement in cancer come from solid tumors, WNT signaling deregulation has also been observed in hematological cancers.
WNT ligands are normally expressed in the hematopoietic stem cells (HSC) compartment and the surrounding microenvironment, and WNT signaling is crucial for HSC self-renewal and homeostasis (26), as well as for the maturation of hematopoietic progenitors (27). The normal hemopoiesis is maintained through the balance between extracellular factors (WNT ligands, agonists and antagonists), cell surface receptors, cytoplasmic components (adapters, destruction complex components etc.) and nuclear factors. Deregulation in any of the involved partners involved could cause hematopoietic disorders.
Aberrant WNT signaling has been reported for example in acute myeloid leukemia (AML). Common chromosomal translocations found in AML (AML1–ETO, PML–RARα and MLL–AF9) result in the stimulation of WNT signaling in AML cells (28, 29), inducing expression of WNT target genes and increasing proliferation. Moreover hyper-activation of the WNT pathway has also been associated to the overexpression of FZD4 (30) and pakoglobin (31). Other evidences suggest that modulation of endogenous WNT antagonists, such as DKK1 and DKK2 (32, 33) or SFRP (34) in AML patients increase the activity of WNT pathway and correlates with adverse clinical outcome.
In chronic myelogenous leukaemia (CML), increased levels of nuclear β- catenin were detected, thus enhancing the self-renewal capacity of CML cells both in vitro (35) and in animal models (36). Both canonical and non-canonical WNT signaling seem to be involved in the resistance to the tyrosine-kinase inhibitor Imatinib and in CML relapse as suggested by preclinical data on CML mouse models (37, 38).
WNT pathway deregulation is suggested to exert a crucial role in acute lymphoblastic leukemias (ALL) as well. In T-ALL, that is strictly dependent on Notch pathway alterations, a pivotal role for canonical WNT signaling has been postulated, based on the high expression of β-catenin, its cofactor LEF1 (39) and on the increased proliferation observed in cells expressing high levels of LEF1 (40). In B-ALL, elevated levels of WNT16 have been found in E2A-PBX1 translocated leukemias (41). Moreover, WNT2B, WNT5A, WNT10B and WNT16B ligands, FZD7 and FZD8 receptors and LRP5/6 co-receptors are overexpressed at different levels in B-ALL (42).
Aberrant WNT signaling is not only involved in the development of leukemias originating from the stem cell compartments, but it is also observed in disorders originating from mature cells.
Dysregulated WNT signaling has also been implicated in the development of Multiple Myeloma (MM). Indeed, β- catenin is often overexpressed and constitutively activated in MM cells, impacting on cell proliferation (43). Moreover, different WNT ligands are expressed in the bone-marrow microenvironment of MM patients, acting both in an autocrine and paracrine manner (44). As a result of these alterations, activation of both the canonical and non-canonical pathways has been observed, hence modulating proliferation capacity (45), migration/invasion (46, 47) and resistance to therapy (48). In this frame, we have recently shown that overexpression of the ROR2 receptor mediates myeloma cells interactions with the bone marrow and its depletion in vivo results in detachment of myeloma cells from their niche and delays disease progression. We also demonstrated, using in vitro and ex vivo 3D-culture systems, that ROR2 exerts a pivotal role in the adhesion of cancer cells to the microenvironment, mainly through the PI3K-AKT pathway, and that genetic and pharmacological inhibition of the AKT pathway is able to reduce ROR2-induced adhesion of malignant cells to bone marrow components (46). Deregulation of WNT pathway, also in terms of WNT antagonist expression, such SFRP2 and DKK1, also has relevant effects on the shaping of bone-marrow niche microenvironment, contributing to alter bone homeostasis and resulting in osteolytic bone disease (49, 50).
In chronic lymphocytic leukemia (CLL) several components of the WNT pathway have been found deregulated: WNT3, WNT5B, WNT6, WNT10A, WNT14, and WNT16, as well as the WNT receptor FZD3, are highly expressed in CLL when compared with normal B cells (51–53). Moreover, soluble inhibitors of the WNT pathway (DKKs and SFRPs) are downregulated (54, 55), further potentiating WNT signaling. More importantly the ROR1 receptor is specifically expressed in malignant CLL cells and not in normal B cells and has been implicated in both proliferation and migration of CLL cells (56, 57).
Aberrant WNT signaling can also be found also in lymphomas. Indeed, elevated levels of nuclear β-catenin have been detected in primary samples of different lymphoma sub-types, even in the absence of mutations in APC or β-catenin (58–60), suggesting that the pathway is activated by autocrine or paracrine mechanisms. Indeed, many WNT components are dysregulated in different type of lymphoma. For example, in Mantle Cell lymphoma (MCL) gene expression profiling studies revealed increased expression of FZD7, APC, LRP5, AXIN1and DVL3 (61).
Discussion
Targeting WNT Pathway: Is This the Way?
Many players of both the canonical and non-canonical WNT signaling are deregulated in hematological cancers, making the WNT pathway an interesting therapeutic opportunity for blood cancers. In this context, candidate drugs can act on WNT signaling pathway at different levels. The ideal druggable targets are both WNT ligands and the receptors or co-receptors, as they are easily accessible for a small compound. However, also intracellular mediators at different signaling levels or additional regulators of WNT pathways (extracellular regulators like DKKs or sFRPs or regulators of WNT secretion like PORCN) have been proposed as possible targets. In this section we report the main therapeutic agents targeting the WNT pathway and currently under clinical trials for the treatment of hematological malignancies (https://clinicaltrials.gov/, summarized in Table 1).
The most advanced clinical application of inhibitors of the WNT family is represented by the monoclonal antibody Cirmtuzumab, targeting the WNT receptor ROR1, that is overexpressed in CLL. Preclinical studies performed with Cirmtuzumab have shown excellent results in terms of induction of apoptosis and inhibition of cell migration both in in vitro and in vivo models of CLL (62, 63) and phase I clinical trial (NCT02860676) with this monoclonal antibody confirmed its safety and biological activity of Cirmtuzumab, even if a clear clinical benefit was not evident (64). Other clinical trials aimed to test the efficacy of this promising compound in different combination settings are currently ongoing (NCT03088878 Phase 1b/II clinical trial in combination with Ibrutinib) or planned (NCT04501939 phase II trial in combination with Venetoclax).
Promising results were also obtained with PRI-724/C-82 compound, a C-EBP/β-catenin inhibitor, on different models of leukemia (including T-ALL, AML and CML) also in the context of drug resistant cells (37, 65). A phase 1/2 clinical study with this candidate drug (NCT01606579) has been performed on AML and CML patients has been conducted, although the results are not available yet.
Another interesting molecule that impacts on WNT-β-catenin signaling is the CWP232291 inhibitor. This molecule targets β -catenin for degradation, thus inhibiting its signaling and exerting anti-apoptotic and anti-proliferative activities. CWP232291 is currently in use in different clinical trials in AMLs and MM, and it was recently reported that this compound is well tolerated and shows encouraging clinical activity (66).
Moreover, another β-catenin inhibitor, BC2059, was shown to reduce proliferation and induce apoptosis on both MM primary samples and cell lines, alone or in combination with proteasome inhibitors, and to delay tumor growth in vivo (67).
As previously mentioned, it is possible to interfere with WNT signaling activation also by targeting extracellular regulators of the pathway. The example of DKK1, that has been exploited as therapeutic target especially in MM, is paradigmatic of how the WNT pathway may represent a real communication route between cells of the microenvironment and tumor cells. Indeed, MM-secreted DKK1 is indeed mainly active on osteoblasts, preventing their differentiation and contributing to the development of osteolytic lesions. The targeting of DKK1 could be achieved through the treatment with the DKN-01 inhibitor, in a phase I trial (NCT01711671 and NCT01457417) or with the anti-DKK1 monoclonal antibody BHQ880, which has been included in an already completed phase 1 (NCT01302886) and phase 2 trials (NCT01337752 and NCT00741377) showing potential clinical activity in myeloma patients (68). DKK1 has also been exploited in an immunotherapy setting in a myeloma mouse model (69) and also with primary myeloma samples (70), and an early phase 1 study (NCT03591614) is going to start soon.
Conclusions
In this review we tried to focus on the specific aberrations affecting the WNT pathway in different hematological cancer and how these aberrations could be exploited as therapeutic targets, developing drugs and compounds with specific effects on that particular biological process. If on the one hand the presence of many WNT components (19 WNT ligands and more than 15 receptors) offers several possibilities of tackling the pathway (though the problem of redundancy still remains), on the other hand the fact that WNT signaling is essential for many processes in development and homeostasis poses several challenges on the side of drug specificity and safety. The considerable bulk of knowledge accrued so far and the initial testing of several compounds impinging on this pathway suggest that the WNT network is both a crucial mediator of cancer cell survival and proliferation as well as an enticing target for new drugs. Nevertheless, much effort is still active on the identification of peculiar abnormalities and on the way to specifically target them, with the final aim of exploiting the WNT pathway alterations to stratify patients and provide novel tools to be exploited in a personalized medicine setting.
AUTHOR CONTRIBUTIONS
MF wrote the manuscript. GT reviewed and wrote the manuscript. All authors contributed to the article and approved the submitted version.
Funding
Funding for this research was provided by Fondazione Cariplo, Association for International Cancer Research (AICR no. 09-0713 to GT), Associazione Italiana per la Ricerca sul Cancro (AIRC Special Program Molecular Clinical Oncology, 5 per mille no. 9965 to GT), Multiple Myeloma Research Foundation (MMRF Research Fellow Award to MF), and Ministero della salute (GR-2011-02351686 to MF).
Conflict of Interest
The authors declare that the research was conducted in the absence of any commercial or financial relationships that could be construed as a potential conflict of interest.
References
1. Clevers H. Wnt/β-Catenin Signaling in Development and Disease. Cell (2006) 127:469–80. doi: 10.1016/j.cell.2006.10.018
2. van Amerongen R, Nusse R. Towards an integrated view of Wnt signaling in development. Development (2009) 136:3205–14. doi: 10.1242/dev.033910
3. Wu CH, Nusse R. Ligand receptor interactions in the Wnt signaling pathway in Drosophila. J Biol Chem (2002) 277:41762–9. doi: 10.1074/jbc.M207850200
4. Voloshanenko O, Gmach P, Winter J, Kranz D, Boutros M. Mapping of Wnt-Frizzled interactions by multiplex CRISPR targeting of receptor gene families. FASEB J (2017) 31:4832–44. doi: 10.1096/fj.201700144R
5. Gao C, Chen Y. Dishevelled : The hub of Wnt signaling. Cell Signal (2010) 22:717–27. doi: 10.1016/j.cellsig.2009.11.021
6. Wharton KA. Runnin’ with the Dvl: Proteins that associate with Dsh/Dvl and their significance to Wnt signal transduction. Dev Biol (2003) 253:1–17. doi: 10.1006/dbio.2002.0869
7. Gammons M, Bienz M. Multiprotein complexes governing Wnt signal transduction. Curr Opin Cell Biol (2018) 51:42–9. doi: 10.1016/j.ceb.2017.10.008
8. van Kappel EC, Maurice MM. Molecular regulation and pharmacological targeting of the β-catenin destruction complex. Br J Pharmacol (2017) 174:4575–88. doi: 10.1111/bph.13922
9. Niehrs C, Shen J. Regulation of Lrp6 phosphorylation. Cell Mol Life Sci (2010) 67:2551–62. doi: 10.1007/s00018-010-0329-3
10. Anthony CC, Robbins DJ, Ahmed Y, Lee E. Nuclear Regulation of Wnt/β-Catenin Signaling: It’s a Complex Situation. Genes (Basel) (2020) 11:886. doi: 10.3390/genes11080886
11. Cadigan KM, Waterman ML. TCF/LEFs and Wnt signaling in the nucleus. Cold Spring Harb Perspect Biol (2012) 4:a007906. doi: 10.1101/cshperspect.a007906
12. Butler MT, Wallingford JB. Planar cell polarity in development and disease. Nat Rev Mol Cell Biol (2017) 18:375–88. doi: 10.1038/nrm.2017.11
13. Hall A. Rho GTPases and the control of cell behaviour. Biochem Soc Trans (2005) 33:891–5. doi: 10.1042/BST20050891
14. De A. Wnt/Ca 2 signaling pathway: A brief overview. Acta Biochim Biophys Sin (Shanghai) (2011) 43:745–56. doi: 10.1093/abbs/gmr079
15. Cruciat CM, Niehrs C. Secreted and transmembrane Wnt inhibitors and activators. Cold Spring Harb Perspect Biol (2013) 5(3):a015081. doi: 10.1101/cshperspect.a015081
16. Hao HX, Xie Y, Zhang Y, Zhang O, Oster E, Avello M, et al. ZNRF3 promotes Wnt receptor turnover in an R-spondin-sensitive manner. Nature (2012) 485:195–202. doi: 10.1038/nature11019
17. Ohkawara B, Glinka A, Niehrs C. Rspo3 Binds Syndecan 4 and Induces Wnt/PCP Signaling via Clathrin-Mediated Endocytosis to Promote Morphogenesis. Dev Cell (2011) 20:303–14. doi: 10.1016/j.devcel.2011.01.006
18. Wang Y, Rattner A, Zhou Y, Williams J, Smallwood PM, Nathans J. Norrin/Frizzled4 signaling in retinal vascular development and blood brain barrier plasticity. Cell (2012) 151:1332–44. doi: 10.1016/j.cell.2012.10.042
19. Ke J, Harikumar KG, Erice C, Chen C, Gu X, Wang L, et al. Structure and function of Norrin in assembly and activation of a Frizzled 4-Lrp5/6 complex. Genes Dev (2013) 27:2305–19. doi: 10.1101/gad.228544.113
20. Bafico A, Gazit A, Pramila T, Finch PW, Yaniv A, Aaronson SA. Interaction of frizzled related protein (FRP) with Wnt ligands and the frizzled receptor suggests alternative mechanisms for FRP inhibition of Wnt signaling. J Biol Chem (1999) 274:16180–7. doi: 10.1074/jbc.274.23.16180
21. Xavier CP, Melikova M, Chuman Y, Üren A, Baljinnyam B, Rubin JS. Secreted Frizzled-related protein potentiation versus inhibition of Wnt3a/β-catenin signaling. Cell Signal (2014) 26:94–101. doi: 10.1016/j.cellsig.2013.09.016
22. Romero D, Al-Shareef Z, Gorroño-Etxebarria I, Atkins S, Turrell F, Chhetri J, et al. Dickkopf-3 regulates prostate epithelial cell acinar morphogenesis and prostate cancer cell invasion by limiting TGF-β-dependent activation of matrix metalloproteases. Carcinogenesis (2016) 37:18–29. doi: 10.1093/carcin/bgv153
23. Port F, Basler K. Wnt Trafficking: New Insights into Wnt Maturation, Secretion and Spreading. Traffic (2010) 11:1265–71. doi: 10.1111/j.1600-0854.2010.01076.x
24. Janda CY, Garcia KC. Wnt acylation and its functional implication in Wnt signalling regulation. Biochem Soc Trans (2015) 43:211–6. doi: 10.1042/BST20140249
25. Torres VI, Godoy JA, Inestrosa NC. Modulating Wnt signaling at the root: Porcupine and Wnt acylation. Pharmacol Ther (2019) 198:34–45. doi: 10.1016/j.pharmthera.2019.02.009
26. Malhotra S, Kincade PW. Wnt-Related Molecules and Signaling Pathway Equilibrium in Hematopoiesis. Cell Stem Cell (2009) 4:27–36. doi: 10.1016/j.stem.2008.12.004
27. Staal FJT, Luis TC, Tiemessen MM. WNT signalling in the immune system: WNT is spreading its wings. Nat Rev Immunol (2008) 8:581–93. doi: 10.1038/nri2360
28. Müller-Tidow C, Steffen B, Cauvet T, Tickenbrock L, Ji P, Diederichs S, et al. Translocation Products in Acute Myeloid Leukemia Activate the Wnt Signaling Pathway in Hematopoietic Cells. Mol Cell Biol (2004) 24:2890–904. doi: 10.1128/mcb.24.7.2890-2904.2004
29. Lane SW, Wang YJ, Lo Celso C, Ragu C, Bullinger L, Sykes SM, et al. Differential niche and Wnt requirements during acute myeloid leukemia progression. Blood (2011) 118:2849–56. doi: 10.1182/blood-2011-03-345165
30. Tickenbrock L, Hehn S, Sargin B, Choudhary C, Bäumer N, Buerger H, et al. Activation of Wnt signalling in acute myeloid leukemia by induction of Frizzled-4. Int J Oncol (2008) 33:1215–21. doi: 10.3892/ijo_00000111
31. Morgan RG, Pearn L, Liddiard K, Pumford SL, Burnett AK, Tonks A, et al. γ-Catenin is overexpressed in acute myeloid leukemia and promotes the stabilization and nuclear localization of β-catenin. Leukemia (2013) 27:336–43. doi: 10.1038/leu.2012.221
32. Valencia A, Román-Gómez J, Cervera J, Such E, Barragán E, Bolufer P, et al. Wnt signaling pathway is epigenetically regulated by methylation of Wnt antagonists in acute myeloid leukemia. Leukemia (2009) 23:1658–66. doi: 10.1038/leu.2009.86
33. Griffiths EA, Gore SD, Hooker C, McDevitt MA, Karp JE, Smith BD, et al. Acute myeloid leukemia is characterized by Wnt pathway inhibitor promoter hypermethylation. Leuk Lymphoma (2010) 51:1711–9. doi: 10.3109/10428194.2010.496505
34. Jost E, Schmid J, Wilop S, Schubert C, Suzuki H, Herman JG, et al. Epigenetic inactivation of secreted Frizzled-related proteins in acute myeloid leukaemia. Br J Haematol (2008) 142:745–53. doi: 10.1111/j.1365-2141.2008.07242.x
35. Jamieson CHM, Ailles LE, Dylla SJ, Muijtjens M, Jones C, Zehnder JL, et al. Granulocyte-macrophage progenitors as candidate leukemic stem cells in blast-crisis CML. N Engl J Med (2004) 351:657–67. doi: 10.1056/NEJMoa040258
36. Zhao C, Blum J, Chen A, Kwon HY, Jung SH, Cook JM, et al. Loss of β-Catenin Impairs the Renewal of Normal and CML Stem Cells In Vivo. Cancer Cell (2007) 12:528–41. doi: 10.1016/j.ccr.2007.11.003
37. Heidel FH, Bullinger L, Feng Z, Wang Z, Neff TA, Stein L, et al. Genetic and pharmacologic inhibition of β-catenin targets imatinib-resistant leukemia stem cells in CML. Cell Stem Cell (2012) 10:412–24. doi: 10.1016/j.stem.2012.02.017
38. Gregory MA, Phang TL, Neviani P, Alvarez-Calderon F, Eide CA, O’Hare T, et al. Wnt/Ca(2+)/NFAT Signaling Maintains Survival of Ph(+) Leukemia Cells upon Inhibition of Bcr-Abl. Cancer Cell (2010) 18:74–87. doi: 10.1016/j.ccr.2010.04.025
39. Ng OH, Erbilgin Y, Firtina S, Celkan T, Karakas Z, Aydogan G, et al. Deregulated WNT signaling in childhood T-cell acute lymphoblastic leukemia. Blood Cancer J (2014) 4:e192. doi: 10.1038/bcj.2014.12
40. Guo X, Zhang R, Liu J, Li M, Song C, Dovat S, et al. Characterization of LEF1 high expression and novel mutations in adult acute lymphoblastic leukemia. PloS One (2015) 10(5):e0125429. doi: 10.1371/journal.pone.0125429
41. Mcwhirter JR, Neuteboom STC, Wancewicz EV, Monia BP, Downing JR, Murre C. Oncogenic homeodomain transcription factor E2A-Pbx1 activates a novel WNT gene in pre-B acute lymphoblastoid leukemia. Proc Natl Acad Sci U S A (1999) 96:11464–9. doi: 10.1073/pnas.96.20.11464
42. Khan NI, Bradstock KF, Bendall LJ. Activation of Wnt/β-catenin pathway mediates growth and survival in B-cell progenitor acute lymphoblastic leukaemia. Br J Haematol (2007) 138:338–48. doi: 10.1111/j.1365-2141.2007.06667.x
43. Derksen PWB, Tjin E, Meijer HP, Klok MD, Mac Gillavry HD, Van Oers MHJ, et al. Illegitimate WNT signaling promotes proliferation of multiple myeloma cells. Proc Natl Acad Sci U S A (2004) 101:6122–7. doi: 10.1073/pnas.0305855101
44. Mahtouk K, Moreaux J, Hose D, Rème T, Meissner T, Jourdan M, et al. Growth factors in multiple myeloma: a comprehensive analysis of their expression in tumor cells and bone marrow environment using Affymetrix microarrays. BMC Cancer (2010) 10:198. doi: 10.1186/1471-2407-10-198
45. van Andel H, Kocemba KA, Spaargaren M, Pals ST. Aberrant Wnt signaling in multiple myeloma: molecular mechanisms and targeting options. Leukemia (2019) 33:1063–75. doi: 10.1038/s41375-019-0404-1
46. Frenquelli M, Caridi N, Antonini E, Storti F, Viganò V, Gaviraghi M, et al. The WNT receptor ROR2 drives the interaction of multiple myeloma cells with the microenvironment through AKT activation. Leukemia (2020) 34:257–70. doi: 10.1038/s41375-019-0486-9
47. Qiang YW, Walsh K, Yao L, Kedei N, Blumberg PM, Rubin JS, et al. Wnts induce migration and invasion of myeloma plasma cells. Blood (2005) 106:1786–93. doi: 10.1182/blood-2005-01-0049
48. Bjorklund CC, Ma W, Wang ZQ, Davis RE, Kuhn DJ, Kornblau SM, et al. Evidence of a role for activation of Wnt/β-catenin signaling in the resistance of plasma cells to lenalidomide. J Biol Chem (2011) 286:11009–20. doi: 10.1074/jbc.M110.180208
49. Kaiser M, Mieth M, Liebisch P, Oberländer R, Rademacher J, Jakob C, et al. Serum concentrations of DKK-1 correlate with the extent of bone disease in patients with multiple myeloma. Eur J Haematol (2008) 80:490–4. doi: 10.1111/j.1600-0609.2008.01065.x
50. Terpos E, Ntanasis-Stathopoulos I, Gavriatopoulou M, Dimopoulos MA. Pathogenesis of bone disease in multiple myeloma: From bench to bedside. Blood Cancer J (2018) 8:7. doi: 10.1038/s41408-017-0037-4
51. Kaucká M, Plevová K, Pavlová Š, Janovská P, Mishra A, Verner J, et al. The planar cell polarity pathway drives pathogenesis of chronic lymphocytic leukemia by the regulation of b-lymphocyte migration. Cancer Res (2013) 73:1491–501. doi: 10.1158/0008-5472.CAN-12-1752
52. Poppova L, Janovska P, Plevova K, Radova L, Plesingerova H, Borsky M, et al. Decreased WNT3 expression in chronic lymphocytic leukaemia is a hallmark of disease progression and identifies patients with worse prognosis in the subgroup with mutated IGHV. Br J Haematol (2016) 175:851–9. doi: 10.1111/bjh.14312
53. Janovská P, Bryja V. Wnt signalling pathways in chronic lymphocytic leukaemia and B-cell lymphomas. Br J Pharmacol (2017) 174:4701–15. doi: 10.1111/bph.13949
54. Moskalev EA, Luckert K, Vorobjev IA, Mastitsky SE, Gladkikh AA, Stephan A, et al. Concurrent epigenetic silencing of wnt/β-catenin pathway inhibitor genes in B cell chronic lymphocytic leukaemia. BMC Cancer (2012) 12:213. doi: 10.1186/1471-2407-12-213
55. Pei L, Choi JH, Liu J, Lee EJ, McCarthy B, Wilson JM, et al. Genome-wide DNA methylation analysis reveals novel epigenetic changes in chronic lymphocytic leukemia. Epigenetics (2012) 7:567–78. doi: 10.4161/epi.20237
56. Cui B, Ghia EM, Chen L, Rassenti LZ, DeBoever C, Widhopf GF, et al. High-level ROR1 associates with accelerated disease progression in chronic lymphocytic leukemia. Blood (2016) 128:2931–40. doi: 10.1182/blood-2016-04-712562
57. Aghebati-Maleki L, Shabani M, Baradaran B, Motallebnezhad M, Majidi J, Yousefi M. Receptor tyrosine kinase-like orphan receptor 1 (ROR-1): An emerging target for diagnosis and therapy of chronic lymphocytic leukemia. BioMed Pharmacother (2017) 88:814–22. doi: 10.1016/j.biopha.2017.01.070
58. Zhang D, O’Neil MF, Cunningham MT, Fan F, Olyaee M, Li L. Abnormal Wnt signaling and stem cell activation in reactive lymphoid tissue and low-grade marginal zone lymphoma. Leuk Lymphoma (2010) 51:906–10. doi: 10.3109/10428191003695645
59. Gelebart P, Anand M, Armanious H, Peters AC, Bard JD, Amin HM, et al. Constitutive activation of the Wnt canonical pathway in mantle cell lymphoma. Blood (2008) 112:5171–9. doi: 10.1182/blood-2008-02-139212
60. Ge X, Lv X, Feng L, Liu X, Wang X. High expression and nuclear localization of β-catenin in diffuse large B-cell lymphoma. Mol Med Rep (2012) 5:1433–7. doi: 10.3892/mmr.2012.835
61. Rizzatti EG, Falcão RP, Panepucci RA, Proto-Siqueira R, Anselmo-Lima WT, Okamoto OK, et al. Gene expression profiling of mantle cell lymphoma cells reveals aberrant expression of genes from the PI3K-AKT, WNT and TGFβ signalling pathways. Br J Haematol (2005) 130:516–26. doi: 10.1111/j.1365-2141.2005.05630.x
62. Yu J, Chen L, Cui B, Wu C, Choi MY, Chen Y, et al. Cirmtuzumab inhibits Wnt5a-induced Rac1 activation in chronic lymphocytic leukemia treated with ibrutinib. Leukemia (2017) 31:1333–9. doi: 10.1038/leu.2016.368
63. Choi MY, Widhopf GF, Wu CCN, Cui B, Lao F, Sadarangani A, et al. Pre-clinical Specificity and Safety of UC-961, a First-In-Class Monoclonal Antibody Targeting ROR1. Clin Lymphoma Myeloma Leuk (2015) 15:S167–9. doi: 10.1016/j.clml.2015.02.010
64. Choi MY, Widhopf GF, Ghia EM, Kidwell RL, Hasan MK, Yu J, et al. Phase I Trial: Cirmtuzumab Inhibits ROR1 Signaling and Stemness Signatures in Patients with Chronic Lymphocytic Leukemia. Cell Stem Cell (2018) 22:951–959.e3. doi: 10.1016/j.stem.2018.05.018
65. Zhou H, Mak PY, Mu H, Mak DH, Zeng Z, Cortes J, et al. Combined inhibition of β-catenin and Bcr–Abl synergistically targets tyrosine kinase inhibitor-resistant blast crisis chronic myeloid leukemia blasts and progenitors in vitro and in vivo. Leukemia (2017) 31(10):2065–74. doi: 10.1038/leu.2017.87
66. Lee JH, Faderl S, Pagel JM, Jung CW, Yoon SS, Pardanani AD, et al. Phase 1 study of CWP232291 in patients with relapsed or refractory acute myeloid leukemia and myelodysplastic syndrome. Blood Adv (2020) 4:2032–43. doi: 10.1182/bloodadvances.2019000757
67. Savvidou I, Khong T, Cuddihy A, McLean C, Horrigan S, Spencer A. β-catenin inhibitor BC2059 is efficacious as monotherapy or in combination with proteasome inhibitor bortezomib in multiple myeloma. Mol Cancer Ther (2017) 16:1765–78. doi: 10.1158/1535-7163.MCT-16-0624
68. Iyer SP, Beck JT, Stewart AK, Shah J, Kelly KR, Isaacs R, et al. A Phase IB multicentre dose-determination study of BHQ880 in combination with anti-myeloma therapy and zoledronic acid in patients with relapsed or refractory multiple myeloma and prior skeletal-related events. Br J Haematol (2014) 167:366–75. doi: 10.1111/bjh.13056
69. Lu C, Meng S, Jin Y, Zhang W, Li Z, Wang F, et al. A novel multi-epitope vaccine from MMSA-1 and DKK1 for multiple myeloma immunotherapy. Br J Haematol (2017) 178:413–26. doi: 10.1111/bjh.14686
Keywords: multiple myeloma, WNT, ROR2, Wnt/b-catenin, microenvironment
Citation: Frenquelli M and Tonon G (2020) WNT Signaling in Hematological Malignancies. Front. Oncol. 10:615190. doi: 10.3389/fonc.2020.615190
Received: 08 October 2020; Accepted: 16 November 2020;
Published: 21 December 2020.
Edited by:
Marco Rossi, University of Catanzaro, ItalyReviewed by:
Pádraig D'Arcy, Linköping University, SwedenSilvia Soddu, Regina Elena National Cancer Institute (IRCCS), Italy
Copyright © 2020 Frenquelli and Tonon. This is an open-access article distributed under the terms of the Creative Commons Attribution License (CC BY). The use, distribution or reproduction in other forums is permitted, provided the original author(s) and the copyright owner(s) are credited and that the original publication in this journal is cited, in accordance with accepted academic practice. No use, distribution or reproduction is permitted which does not comply with these terms.
*Correspondence: Michela Frenquelli, ZnJlbnF1ZWxsaS5taWNoZWxhQGhzci5pdA==; Giovanni Tonon, dG9ub24uZ2lvdmFubmlAaHNyLml0