- 1Hepatic Surgery Center, Tongji Hospital, Tongji Medical College, Huazhong University of Science and Technology, Wuhan, China
- 2Hubei Key Laboratory of Hepato-Pancreato-Biliary Diseases, Wuhan, China
- 3Key Laboratory of Organ Transplantation, NHC Key Laboratory of Organ Transplantation, Key Laboratory of Organ Transplantation, Ministry of Education, Chinese Academy of Medical Sciences, Wuhan, China
Exosomes are small membranous vesicles released by many kinds of cells, and are indispensable in cell-to-cell communication by delivering functional biological components both locally and systemically. Long non-coding RNAs (lncRNAs) are long transcripts over 200 nucleotides that exhibit no or limited protein-coding potentials. LncRNAs are dramatic gene expression regulators, and can be selectively sorted into exosomes. Exosomal lncRNAs derived from cancer cells and stromal cells can mediate the generation of pre-metastatic niches (PMNs) and thus promote the progression of cancer. In this review, we summarized the fundamental biology and characteristics of exosomal lncRNAs. Besides, we provided an overview of current research on functions of exosomal lncRNAs between cancer cells and non-cancer cells. A deep understanding of exosomal lncRNAs’ role in cancer will be facilitated to find important implications for cancer development and treatment.
Introduction
Exosomes are extracellular vesicles (EVs) with a size range of ~40 to 160 nm (average ~100 nm) in diameter from multivesicular bodies (MVBs) fusing with plasma membrane (1). In 1983, Johnstone RM et al. firstly discovered EVs in mature sheep reticulocytes, and named them as exosomes. They considered exosomes as cellular “debris” at that time (2). In 1996, Raposo et al. found that B lymphocytes secreted antigen-presenting exosomes which induced T cell response (3). In 2007, Valadi H. et al. put forward that exosomes containing both messenger RNAs (mRNAs) and microRNAs (miRNAs) can be transferred to another cell, and have function in the new location (4). Subsequently, more and more studies indicated that exosomes can mediate intercellular communication by carrying proteins, DNAs, and RNAs including non-coding RNAs (5). In addition, exosomes were presented in vast majority of body fluids, including plasma (6), urine (7), saliva (8), ascites (9). In tumor milieu, exosomes were derived among different kinds of cells like tumor cells, fibroblasts, and immune cells (10, 11), regulating tumor microenvironment (TME) mainly by autocrine, paracrine, or endocrine way (12). On account of the distinctive role in tumor development and the universality of existence, exosomes high prospects as therapeutic targets as well as the diagnosis biomarkers in cancer.
Long non-coding RNAs (lncRNAs) are defined as transcripts longer than 200 nucleotides that have no or limited protein-coding capacity (13). Owing to highly heterogeneous primary sequence and low expression level, lncRNAs were once believed as transcriptional “noise” (14). Thanks to high-throughput sequencing technology, it is now evident that lncRNAs have formidable functions in regulating gene expression and cell homeostasis. LncRNAs are located in either the cytoplasm or nucleus, which can interact with microRNAs, mRNAs, RNA-binding proteins (RBPs), transcription factors and chromatins, and act as enhancer-like RNAs (15, 16). The complex and extensive roles of lncRNAs in gene regulation are commonly separated into epigenetic, transcriptional and post-transcriptional levels (17). Beyond that, lncRNAs are reported to encode hidden polypeptides by the translation of small open reading frames (smORFs) (18–20). It should be emphasized that lncRNAs function as competing endogenous RNAs (ceRNAs) by sponging microRNAs, and hence inhibit microRNAs interacting with target mRNAs (21, 22). CeRNAs represent a new means of mechanism that involve in two kinds of non-coding RNAs in the same physiological process, which were largely showed in exosomal lncRNA regulation. A significant portion of lncRNAs are oncogenic lncRNAs that are associated with cancer occurrence, progression and outcome. Emerging evidence support the notion that lncRNAs play indispensable characters in proliferation (23), apoptosis (24), metastasis (25), angiogenesis (26), metabolism (27) of cancer (28). For example, lncRNA PTAR upregulated ZEB1 by competitively binding miR-101-3P like sponges, promoting TGF-β induced EMT and invasion in ovarian cancer (29). The diversity of lncRNAs function and mechanism implies a great potential in tumor malignant transformation, and exosomes amplify the function of lncRNAs by means of transporting them to distal region. As a novel way of acting, lncRNAs can be selectively sorted into exosomes and serve as signaling messengers in intercellular communication (4). LncRNAs were wrapped by exosomes and delivered to recipient cells, and then converted cell phenotypes by aforesaid mechanisms. In TME, exosomal lncRNAs have crucial impacts on proliferation, metastasis, angiogenesis, immunosuppression, and chemoresistance. It is fortunate that exosomes’ lipid membranes protect lncRNAs from degradation by ribonuclease. As a result, lncRNAs can be delivered to primary tumor tissue or distant organs safely (30). Therefore, the application of exosome-derived lncRNAs in tumorigenesis, development and treatment has attracted growing attention (31). In this review, we not only summarized basic information of exosomes, but also focused on the latest literatures related to the exosomal lncRNAs in cancers.
Exosome: Generation and Uptake
Exosomes originate from early and late-sorting endosomes formed by inward budding of the cell membranes (32). Subsequently, Late-sorting endosomes mature into MVBs (33). During this process, the endosomal limiting membranes inwardly invaginate and envelope proteins, RNAs and DNAs to form intraluminal vesicles (ILVs), which are the exosomes released to extracellular space subsequently. According to the condition and environment of the cells, MVBs would secret ILVs as exosomes by fusing with cell membrane, which is regulated by several RAB GTPases (including RAS-related protein RAB5, RAB7, RAB11, RAB27, and RAB35) as well as membrane fusion soluble N-ethylmaleimide-sensitive factor attachment protein receptor (SNARE) complex proteins (34). It’s worth mentioning that lncRNAs are involved in the biogenesis of exosomes and tumor development. For example, Wang, F. W. et al. illuminated that lncRNA-APC1 can bind Rab5b mRNA and reduce its stability, leading to reduction of exosomes production, thereby inhibiting colorectal cancer (CRC) growth, metastasis, and angiogenesis (35). Also, MVBs can fuse with lysosomes or autophagosomes to be degraded. The generation of exosomes mainly involves in two sorting mechanisms, including endosomal sorting complex required for transport (ESCRT) pathway and the ESCRT independent pathway (36, 37). However, it is still unclear whether the production of the same exosomes can contain these two sorting methods, or whether the two sorting methods can coexist in one type of cells.
The capturing and uptaking of exosomes are firstly divided into two ways according to whether they enter the cell. One is to rely on the interaction of glycans, lectins, integrins, and other cell adhesion molecules on the surface of exosomes with the cell membrane to directly activate the signaling pathway, or have fusion with the cytoplasm membrane and release cargos into the cytosol (38, 39). The other is be internalized by cells through endocytosis to form endosomes (40), which mainly includes clathrin-dependent endocytosis and clathrin-independent macropinocytosis (41) or phagocytosis (42). Endosomes can release exosomes into the cytoplasm and further release the contents including lncRNAs. The released lncRNAs can exert regulatory effect through various mechanisms, which include sponging miRNAs to regulate target genes, participating alternative splicing or RNA editing by matching with mRNAs, and integrating with RNPs. Besides, lncRNAs can even act as protein-coding RNAs to translate short peptides. In the nucleus, lncRNAs can also interact with transcription factors to promote or suppress gene transcription, induce chromatin remodeling and histone modification, as well as function as enhancers (43–45). What’s more, Endosomes can be degraded by fusing with the lysosomes, or even re-fusing with the cell membranes to release exosomes outside the cell again (Figure 1). However, it is unknown whether the release of endogenous generated and exogenous captured exosomes occur together or separately. In vitro and in vivo experiments have shown that exosomes were more likely to be absorbed by parent cells and can be used to make targeted drug carriers (46). Exosomes’ generation and uptake ultimately depend on the cell type and environment. Therefore, an acknowledged mechanism is requisite to help us further understand the role of exosomes in cell-to-cell communication.
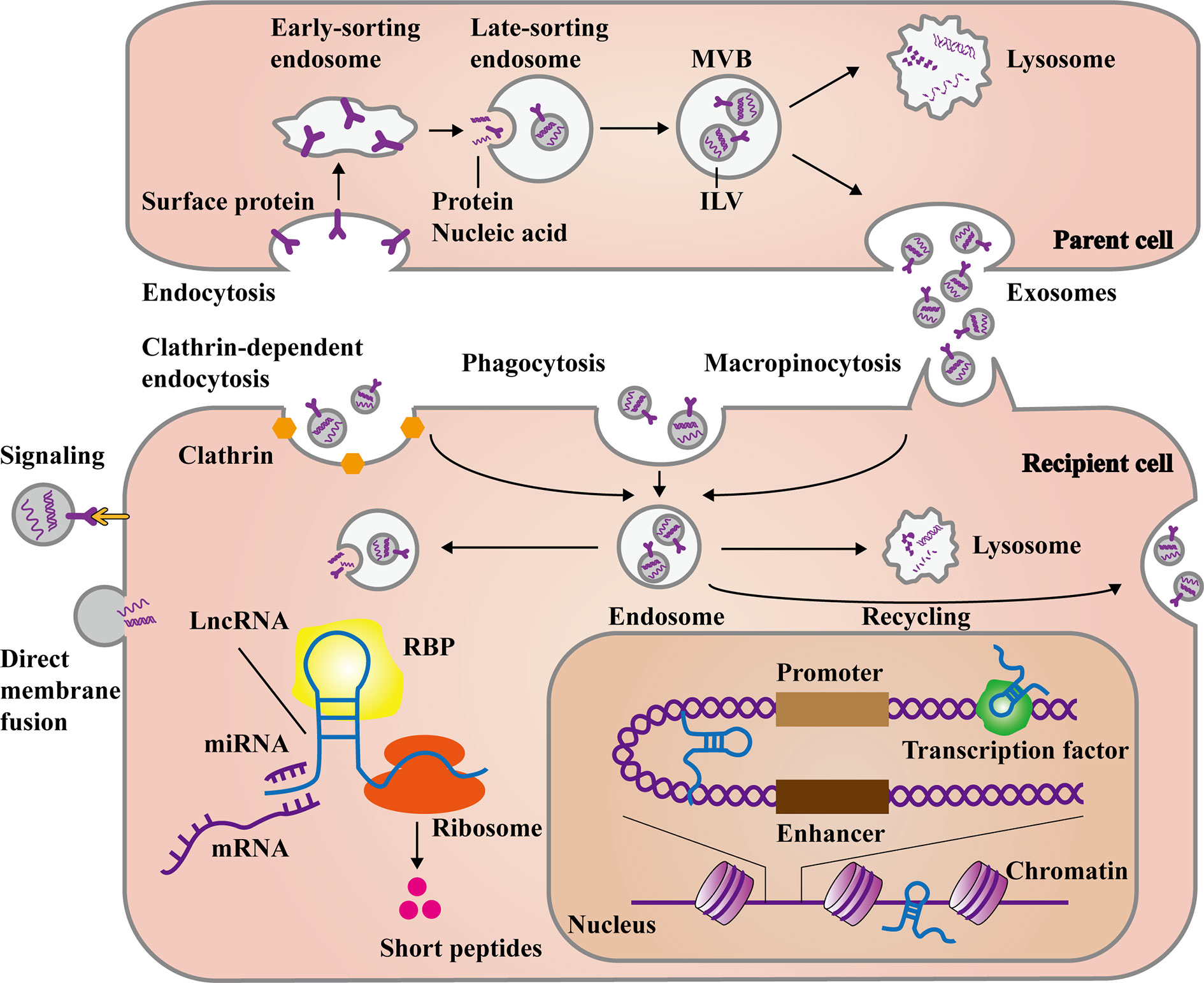
Figure 1 The main process of exosomal long non-coding RNAs (lncRNAs) formation and release. The formation of exosomes stems from the endocytosis of some membrane proteins at the plasma membrane, forming early and late-sorting endosomes. Then intraluminal vesicles (ILVs) are formed through the inward budding of the late-sorting endosomal membrane with encapsulating the substances like proteins, DNAs and RNAs. Finally, late-sorting endosomes mature to multivesicular bodies (MVBs), which release ILVs as exosomes. Otherwise, they fuse with lysosomes to be degraded. Exosomes can directly recognize and transmit signal to recipient cells, as well as fuse with plasma membrane to release cargos. In the other hand, exosomes can be internalized to form endosomes mainly through clathrin-dependent endocytosis, clathrin-independent macropinocytosis, or phagocytosis. Deeping on the needs of cells, the endosomes release exosomal cargos, fuse with lysosomes for degradation, or even fuse with plasma membrane again to recycle exosomes. The exosomal lncRNAs are subsequently released to regulate cell function in various ways. In the cytoplasm, lncRNAs can affect post-transcriptional levels by binding miRNAs, mRNAs, and proteins. Besides, some lncRNAs can even encode short peptides. In the nucleus, lncRNAs can also interact with transcription factors and chromatins, as well as act as enhancer-like RNAs.
Exosomal Long Non-Coding RNA: Sequencing and Database
The confirmation of exosome derived lncRNA is the first step to start the research, and the ways to obtain the appropriate lncRNA worth studying are various and evolutionary. Four to five years ago, microarray once occupied the mainstream, Qu, L. et al. utilized lncRNA microarray to compare lncRNA expression profiles between parental and sunitinib resistant RCC cells, and finally confirmed the most discrepant lncRNA (47). In recent years, as the cost of high-throughput sequencing decreased, its advantages of high sensitivity, whole-genome coverage, and the ability to explore unknown sequences have been amplified, resulting in a significant increase in high-throughput sequencing applications (48). For example, Yu, S. et al. performed extracellular vesicle long RNA-seq (including lncRNA) on plasma samples collected from 501 subjects, and developed 8 long RNAs for the detection of pancreatic cancer (49). In addition, there are also studies based on star lncRNAs such as HOTAIR and H19 to explore their roles with exosomes in TME (50, 51). Nowadays, a series of exosome databases that collect various public exosome sequencing data are constructed. In exoRBase (http://www.exoRBase.org), 58,330 circular RNAs (circRNAs), 15,501 lncRNAs, and 18,333 mRNAs derived from RNA-seq data analyses of human blood exosomes and experimental validations from published literature are concluded (52). Besides, The exRNA Atlas (http://exrna-atlas.org) is the data repository of the extracellular RNA communication consortium (ERCC), including 7,570 small RNA sequencing and qPCR-derived exRNA profiles from human and mouse biofluids (53). The combination of high-throughput sequencing and exosome databases is conducive to understand the profile of exosomal lncRNAs under specific pathophysiological conditions, facilitating efficient screening of exosomal lncRNAs worthy of study.
Exosomal Long Non-Coding Rna: Functional Roles Between Cancer And Non-Cancer
Intercellular signaling interaction are constructed between cancer cells and non-cancer cells to accelerate malignant progression of cancer. Furthermore, intracellular signaling networks operate by integrated circuits to reprogram gene expression, which induce hallmark capabilities of cancer, such as sustaining proliferation and activating invasion (54). Originally, cell communicated through direct cell-to-cell contact and soluble factors (55). Now, exosomes emerge as vital participants in the intercellular signaling transmission. LncRNAs are key functional molecules that mediate intercellular signaling interaction due to the role in genetic and epigenetic modulation. Hence, growing enthusiasm and energy are devoted to investigating the specific role and mechanism of exosomal lncRNAs in cancer.
In exosomes associated TME, “seed-and-soil” hypothesis (56) has far-reaching implications. The exosomes secreted by tumor cells carry various inflammatory factors and immunosuppressive factors (“fertilizer”), such as macrophage migration inhibitory factor (MIF) (57) and PD-L1 (58), which perform in the surrounding or distant normal tissues or organs, causing vascular leakiness (59), inflammation infiltration (60), extracellular matrix (ECM) remodeling (61), and immune suppression (62). A series of stimulation transform the non-tumor environments into pro-tumorigenic pre-metastatic niches (PMNs) (“soil”), attracting tumor cells (“seeds”) to colonize and grow. Concretely speaking, activated stromal cells can release a lot of cytokines and chemoattractants through exosomes, such as IL-6, IL-8 (63), and S100A9 (64), which trigger the proliferation, invasion, stemness, and chemoresistance of tumor cells. In addition, among tumor cells of different malignant degree, exosomes will also be delivered by paracrine to enhance the overall metastatic burden. Consequently, we divided exosomal lncRNA associated studies into three categories: cancer to non-cancer, cancer to cancer, and non-cancer to cancer (Table 1), which are beneficial to understand the unique and important roles of exosomal lncRNAs in each stage of the interaction between cancer cells and non-cancer cells, so as to provide ideas for the development of targeted diagnostic methods and treatment strategies.
Cancer to Non-Cancer
Cancer cells derived exosomes can promote non-cancer cells such as endothelial cells, mesenchymal stem cells (MSCs), carcinoma-associated fibroblasts (CAFs), and immune cells to generate PMNs (86). As a valuable kind of biomolecules, lncRNAs play important roles in various pathophysiological processes of forming PMNs, resulting in malignant tumors initiation and progression.
For example, cancer cells derived exosomes can influence endothelial cells to promote angiogenesis (87) which plays a momentous role in tumor proliferation, and induce vascular permeability (88) which is conducive to tumor metastasis (89). MiR-25-3p, a miRNA transferred from CRC cells to endothelial cells via exosomes, promoted vascular permeability and angiogenesis, finally led to hematogenous metastasis in CRC (90). Certainly, exosomal lncRNAs also show significant function in regulating endothelial cells. Bladder cancer (BCa) with lymph node (LN) metastasis has a poor prognosis (91), while PROX1 enhanced lymphatic endothelial cell differentiation and lymphatic budding through constructing interaction with p50 to upregulate VEGFR3 expression level (92). Chen, C. et al. pointed out lncRNA named LNMAT2, which interacted with heterogeneous nuclear ribonucleoprotein A2B1 (hnRNPA2B1) in BCa cells. Under the direction of hnRNPA2B1, LNMAT2 was selectively packed into exosomes and transmitted to human lymphatic endothelial cells (HLECs). Subsequently, LNMAT2 formed a triplex by interacting and integrating with the promoter of PROX1. After epigenetic modification by hnRNPA2B1-mediated H3 lysine 4 trimethylation (H3K4me3), PROX1 transcription was enhanced. Consequently, LNMAT2 mediated lymphangiogenesis and LN metastasis in BCa (67). What’s more, MALAT1 is a well-known lncRNA associated with cancer angiogenesis and metastasis (93). In metastatic epithelial ovarian cancer (EOC), MALAT1 can be transported to human umbilical vein endothelial cells (HUVECs) by exosomes, influencing HUVECs by stimulating the expression of angiogenesis-related genes, such as angiogenin and bFGF (74). Glioma is one of the most malignant cancers of the central nervous system (94). There are numerous literatures relating to exosomal lncRNAs in regulating the angiogenesis of glioma. For example, the long non-coding RNA HOTAIR wrapped by glioma cells’ exosomes induced the proliferation, migration, and tube formation of endothelial cells by increasing the expression of VEGFA (95), a well-known proangiogenic factor (51). Similarly, exosomal lncRNA POU3F3 and CCAT2 also induced angiogenesis in glioma (72, 73).
Furthermore, exosomal lncRNAs delivered to other stromal cells can also change the cells into pro-tumorigenic phenotypes (96). Cancer cells can induce immune tolerance and evade immune surveillance by secreting exosomes (97), which is the major component of PMN creation. For example, γδT cells occupied small proportion of all T lymphocytes but had significant immunosuppressive function as well as positive modulation of immunity (98). Evidence have demonstrated that γδT cell consists an important element of tumor-infiltrating lymphocytes (TILs) and is associated with poor progression and prognosis of breast cancer (99), but considering its positive effect in the innate and adaptive immune systems, a biomarker to identify the truly immunosuppressive subpopulations is urgently requisite. A recent study indicated that exosomal lncRNA SNHG16 was responsible for cross-talk between breast cancer cells and γδ1 T cells, exerting an effect in CD73 expression and resulting in the transformation of γδ1 T cells into the CD73+ immunosuppressive subtype. As a matter of fact, CD73+ γδ1 T cells play a crucial tumor-promoting function in breast cancer microenvironment. As for concrete mechanism, it was speculated that the extraneous SNHG16 activated TGF-β1/SMAD5 pathway by serving as a ceRNA with miR-16-5p in γδ1 T cell (66). Beyond that, macrophages can transform into tumor-associated macrophages (TAMs) under activation by chemokines, inflammatory, and growth factors (100), as well as M1/M2 polarization (101), contributing to the formation of PMNs (102). And evidence have shown exosomal lncRNAs were favorable for this progress. Li, X. et al. figured out that hepatocellular carcinoma (HCC) derived exosomal lncRNA TUC339 induced macrophage activation and polarization. TUC339 was enriched in HCC cells and corresponding exosomes, and over-expression of TUC339 in macrophage cells led to reduced pro-inflammatory cytokine production, decreased co-stimulatory molecule expression, and compromised phagocytosis. Moreover, TUC339 was indispensable for luring M2 polarization. Combined with the transcriptome-wide analysis, cytokine-cytokine receptor interaction et al. may explain the mechanism behind the role of TUC339 (71). LncRNA RUNX2-AS1 was highly expressed in MSCs extracted from multiple myeloma patients (MM-MSCs), and enriched in exosomes of human myeloma cell lines (HMCLs), while RUNX2 was lowly expressed in MM-MSCs. By forming an RNA duplex with RUNX2 pre-mRNA, RUNX2-AS1 interfered RUNX2 pre-mRNA splicing, resulting in the reduction of RUNX2 expression. Ultimately, exosomal lncRNA RUNX2-AS1 mediated decreased osteogenic potential of MSCs, which is the most outstanding character of multiple myeloma (68). In glioma, astrocytes were upon the activation phenotype by exosomes derived from glioma cells carrying lncRNA−ATB, which targeted and suppressed miR-204-3p. And in turn, activated astrocytes promoted the migration and invasion of glioma cells (70).
As noted above, different kinds of cells work together to develop cancer in TME. However, the mutual effects can be bidirectional in some situations. For instance, when HUVECs co-cultured with TAMs derived exosomes, the migration capacity was decreased by targeting miR-146b-5p, which led to the activation of NF-κB phosphorylation. Whereas, when TAMs derived exosomes stimulated HUVECs combining exosomes secreted from EOC cells, the inhibition of HUVECs was reversed. Wu, Q. et al. confirmed two lncRNAs associated with NF-κB phosphorylation in exosomes derived from EOC cells, while the detailed mechanism of exosomal lncRNAs remained unknown (103).
In summary, tumor derived exosomal lncRNAs can transform the state and phenotype of stromal cells to support tumor cells invasion and growth (104). The formation of PMNs triggered by exosomal lncRNAs has become a novel and important focus. Further understanding of their precise mechanisms in stromal cells will provide promising prospects for the prevention and treatment of tumors.
Cancer to Cancer
Except for connecting the communication between cancer cells and stromal cells, exosomes derived from one cancer cell can also transmitted to another cancer cell under different conditions. For instance, resistant cancer cells can confer drug resistance to sensitive cells through exosomes (77). As a result, the invasion and dissemination of cancers are continually proceeding like virus replication, leading to more malignant phenotypes. Recent studies have indicated that exosomal lncRNAs can contribute to this kind of cell-to-cell communication. For example, Qu, L. et al. demonstrated that lncARSR can disseminate sunitinib resistance in renal cell carcinoma (RCC). Mechanistically, upon the interaction with hnRNPA2B1, lncARSR was specifically sorted into exosomes to drug-sensitive cells. And lncARSR bound miR-34 and miR-449 which targeted to AXL and c-MET as a competing endogenous RNA. The activation of AXL/c-MET caused phosphorylation of AKT, ERK, and STAT3 signal pathway, which counteracted sunitinib’s effect. Therefore, sunitinib-sensitive cells were converted into resistant cells. Furthermore, transcription factors FOXO1 and FOXO3a were phosphorylated and degraded by activated AKT, resulting in increased expression of lncARSR. Intriguingly, the whole process formed a positive feedback loop in RCC cells (47). Long non-coding RNA MRPL23-AS1 was highly expressed in exosomes secreted from salivary adenoid cystic carcinoma (SACC) cells. Besides, the RNA-protein complex consisted of MRPL23-AS1 and EZH2 increased H3K27me3 of the E-cadherin promoter region, causing the initiation of epithelial-mesenchymal transition (EMT). Additionally, exosomal MRPL23-AS1 can also contribute to microvascular permeability in pulmonary microvascular endothelial cells. Altogether, SACC patients tended to lung metastasis and low overall survival upon the role of exosomal MRPL23-AS1 (76). Pancreatic ductal adenocarcinoma (PDAC)is one of the most aggressive cancers (105) because of its low diagnostic rate in the early stage and rapid metastasis (106). Li, Z. et al. elucidated that high invasive PDAC cells can release exosomes carrying lncRNA Sox2ot to low invasive PDAC cells. Then, the internalized Sox2ot promoted EMT and stem cell-like properties by competitively binding the miR-200 family. In addition, Sox2ot embedded in exosomes was validated by orthotopic xenograft assay to confirm that the lncRNA can be used as a special biomarker for PDAC diagnosis and prognosis (78). Accumulated evidence have proofed that hypoxia can remodel primary tumor microenvironment via protection from apoptosis (107), activation of EMT (108), abnormal metabolism, as well as microangiogenesis (109), finally, leading to the metastasis of cancer (110). Long non-coding RNA-UCA1 enriched in exosomes derived from hypoxic bladder cancer cells can promote tumor proliferation, migration and invasion though EMT. Regretfully, the authors rarely investigate the detailed function of exosomal lncRNA-UCA1 in bladder cancer cells (79).
As mentioned above, exosomal transmission between cancer cells can increase tumor chemoresistance and metastasis. Since the exosomal lncRNA-mediated intercellular communication occurs in the local area, the study of lncRNA specific antagonists like antisense oligonucleotides (ASOs) (111, 112) will provide powerful aid for conventional chemotherapeutic drugs.
Non-Cancer to Cancer
In the formation of PMNs, stromal cells like macrophages and fibroblasts were stimulated by “fertilizer” such as inflammatory and immunosuppressive factors, then converted into TAMs and CAFs (113). Reciprocally, the TAMs and CAFs would ulteriorly accelerate PMNs establishment and promote tumor dissemination (114). Exosomes play a significant role in such intercellular communication. Thus, considerable attention has been focused on exosomes in the area of non-cancer cells to cancer cells interflow. And here we emphatically stated the studies about exosomal lncRNAs. HIF-1α-stabilizing long non-coding RNA (HISLA) level was positive correlated with poor overall survival of patients with breast cancer in clinical trial. Chen, F. et al. claimed HISLA wrapped by exosomes derived from TAMs can promote aerobic glycolysis, apoptotic resistance and chemoresistance of breast cancer cells. During this pathophysiological process, HIF-1α, a transcription factor, also played a key role in determining glucose glycolysis or oxidation (115). It is well elucidated that TAMs’ exosomal HISLA competitively bound PHD2, preventing PHD2 having synergistic interaction with HIF-1α. Therefore, the hydroxylation and degradation of HIF-1α were inhibited, leading to enhanced aerobic glycolysis and lactate production. Surprisingly, as the highlight of this study, HISLA in macrophages was upregulated by lactate released from glycolytic cancer cells, which established a feed-forward loop between TAMs and cancer cells (83). It is believed that CAFs play a critical character of matrix remodeling in PMNs formation (116). Previous studies have reported that lncRNA H19 contributed to oncogenesis in many kinds of cancer (117). Moreover, it was found that H19 embedded in exosomes from CAFs enhanced stem cell-like features and chemoresistance in CRC. The RNA-binding protein immunoprecipitation (RIP) experiment and luciferase assay were performed to uncover that H19 sponged miR-141 as ceRNA, resulting in the activation of β-catenin pathway (84). A few lncRNAs that block tumorigenesis were reported as well. Exosome-carrying lncRNA PTENP1 transmitted from normal cells to tumor cells was able to increase cell apoptosis, but decrease motility of BCa cells (85).
In conclusion, stromal cells can deliver lncRNAs to cancer cells via exosomes, activating cellular signaling pathways and changing gene expression to accelerate tumor progression. Further studies of this field will offer a novel horizon in exosome associated tumor research, contributing to the delay of tumor deterioration and the improvement of drug efficacy.
Conclusions And Prospects
Cancer is the most lethal disease in the world due to poor diagnosis and prognosis. What’s more, it is still not fully clear how cancer grows and colonizes until now. Exosomes represent a new manner of transporting information between cancer cells and other functional cells. lncRNAs play indispensable characters in cancer by regulating gene expression in diverse approaches. Especially, lncRNAs are involved in exosome-mediated intercellular signaling. In this review, we mainly summarized recent literatures about the biological functions and mechanisms of exosomal lncRNAs in tumor microenvironment (Figure 2). Meanwhile, we introduced fundamental characteristics and research techniques of exosomes. LncRNA-carrying exosomes from cancer cells or stromal cells can deliver pro-tumorigenesis signals to target cells, contributing to the proliferation, metastasis, angiogenesis and chemoresistance of tumor. Exosomal lncRNAs provide us a novel horizon of tumor generation and development. Since exosomes are non-immunogenic, minimal toxic effects, as well as existed in nearly all of body fluids, they are promising to be applied in clinic such as drug transporters.
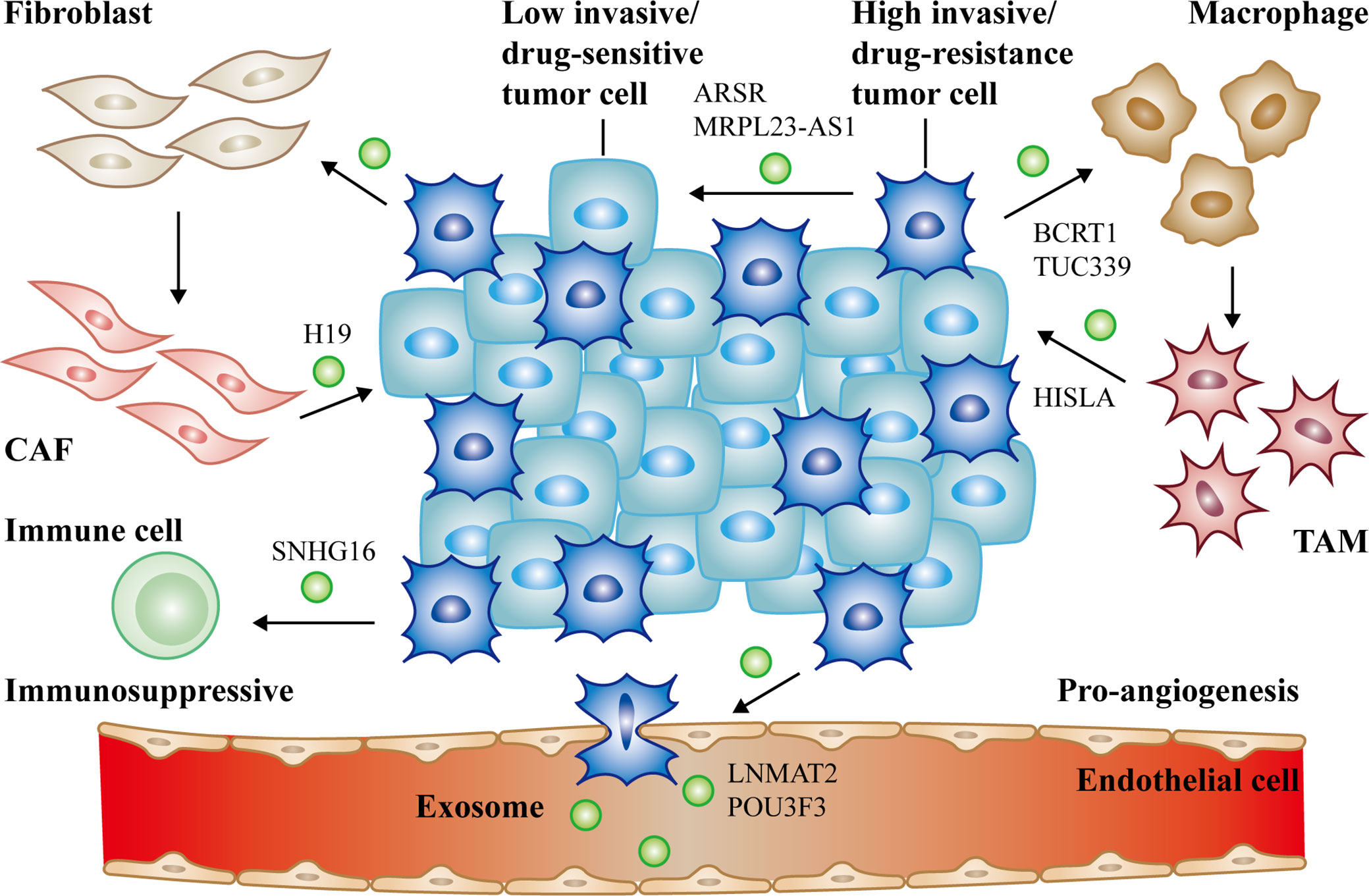
Figure 2 The communication of exosomal long non-coding RNAs (lncRNAs) between cancer cells and non-cancer cells in tumor microenvironment. Cancer cells derived exosomal lncRNAs active fibroblasts, macrophages (lncRNA BCRT1, lncRNA TUC339), endothelial cells (lncRNA LNMAT2, lncRNA POU3F3) and suppress immune cells to form the PMN (lncRNA SNHG16). Reciprocally, activated CAFs (lncRNA H19), TAMs (lncRNA HISLA) can also deliver exosomal lncRNAs to promote cancer progression. Moreover, high/low invasive or drug-resistance/sensitive tumor cells can communicate with each other via exosomal lncRNAs as well (lncRNA ARSR, lncRNA MRPL23-AS1). All of the above together promote tumor growth, metastasis, and chemoresistance.
However, there are still a number of issues remain poorly understood. For instance, a few studies have revealed that even when lncRNA expression was low in parental cells, it was enriched in exosomes (118, 119). This implicates that lncRNAs are selectively packaged into exosomes through active or passive mechanisms, such as binding to hnRNPA2B1 (47, 67). Nevertheless, the mechanism initially driving lncRNAs to be sorted into exosomes and its relevance with tumor progression are still elusive.
Additionally, a few limitations impede the in-depth exploration of exosomes, and the clinical translation of their functions. First, the results of many studies are only obtained through in vitro experiments between the two types of assigned cells and in vivo experiments of established animal models (67, 85), which mean that they cannot be confirmed in real pathophysiological conditions. Under these circumstances, if exosomes are served as transport vesicles, the transmission efficiency will not be guaranteed, and the treatment effect may be compromised if exosomes or lncRNAs are used as therapeutic targets. Beyond that, the same lncRNA has been reported to have inconsistent effects in exosomes from different cells and tumors (50, 69, 120). Therefore, it is necessary to control the dose and targeting of exosomal lncRNAs, otherwise, it will affect the homeostasis of the cells and cause great side effects. Based on the above limitations, multiple strategies have been designed for exosome labelling to trace the actual transport path in vivo, such as fluorescence (121, 122), bioluminescence (123), and radioactive isotope labelling (124, 125). In addition, the latest studies are focusing on targeted delivery to upgrade the capacity of recipient cells in capturing exosomes, including ligand-receptor binding (126, 127), pH/charge affinity (128, 129), and magnetic attraction (130). As these methods may be genetically altered or inefficient, we need to explore comprehensive techniques for exosome labelling and targeted delivery to maintain specificity, high efficiency, and native function of exosomes. Second, a large proportion of current studies are on the strength of the mechanism and function of cell and animal experiments, coupling with differences in the expression and prognosis of clinical samples (68, 78). Large-sample multicenter and prospective clinical studies are required to evaluate the authenticity and reliability of clinical application. Third, it is difficult to quantify and standardize exosomal lncRNAs in both experimental and clinical applications due to low abundance of lncRNAs in exosomes (131). Thus, a fast and sensitive analytical method is urgently needed.
With more researches conducted on exosome-derived lncRNAs, it is believed that exosomal lncRNAs will not only help us shed more light on the pathophysiology of tumors, but also be widely used in clinical diagnosis and therapeutics in the near future.
Author Contributions
SH wrote the manuscript and designed the figures. YQ and YL collected the related references and edited the manuscript. XC and HL provided guidance and revised the manuscript. All authors contributed to the article and approved the submitted version.
Funding
This work is supported by grants from the National Natural Science Foundation of China (No. 81572855 to XC and No. 81202300 to HL) and a project of Hubei Natural Science Foundation of China (No. 2015CFB462).
Conflict of Interest
The authors declare that the research was conducted in the absence of any commercial or financial relationships that could be construed as a potential conflict of interest.
Acknowledgments
All authors thank Dr. Zhibin Liao, Dr. Hongwei Zhang, and Dr. Furong Liu for their valuable suggestions.
References
1. Kalluri R, LeBleu VS. The biology function and biomedical applications of exosomes. Sci (New York N.Y.) (2020) 367(6478):eeaau6977. doi: 10.1126/science.aau6977
2. Johnstone RM, Adam M, Hammond JR, Orr L, Turbide C. Vesicle formation during reticulocyte maturation. Association of plasma membrane activities with released vesicles (exosomes). J Biol Chem (1987) 262:9412–20.
3. Raposo G, Nijman HW, Stoorvogel W, Liejendekker R, Harding CV, Melief CJ, et al. B lymphocytes secrete antigen-presenting vesicles. J Exp Med (1996) 183:1161–72. doi: 10.1084/jem.183.3.1161
4. Valadi H, Ekström K, Bossios A, Sjöstrand M, Lee JJ, Lötvall JO. Exosome-mediated transfer of mRNAs and microRNAs is a novel mechanism of genetic exchange between cells. Nat Cell Biol (2007) 9:654–9. doi: 10.1038/ncb1596
5. Raposo G, Stoorvogel W. Extracellular vesicles: exosomes, microvesicles, and friends. J Cell Biol (2013) 200:373–83. doi: 10.1083/jcb.201211138
6. Caby M-P, Lankar D, Vincendeau-Scherrer C, Raposo G, Bonnerot C. Exosomal-like vesicles are present in human blood plasma. Int Immunol (2005) 17:879–87. doi: 10.1093/intimm/dxh267
7. Bryzgunova OE, Zaripov MM, Skvortsova TE, Lekchnov EA, Grigor’eva AE, Zaporozhchenko IA, et al. Comparative Study of Extracellular Vesicles from the Urine of Healthy Individuals and Prostate Cancer Patients. PloS One (2016) 11:e0157566. doi: 10.1371/journal.pone.0157566
8. Ogawa Y, Kanai-Azuma M, Akimoto Y, Kawakami H, Yanoshita R. Exosome-like vesicles with dipeptidyl peptidase IV in human saliva. Biol Pharm Bull (2008) 31:1059–62. doi: 10.1248/bpb.31.1059
9. Hu Y, Qi C, Liu X, Zhang C, Gao J, Wu Y, et al. Malignant ascites-derived exosomes promote peritoneal tumor cell dissemination and reveal a distinct miRNA signature in advanced gastric cancer. Cancer Lett (2019) 457:142–50. doi: 10.1016/j.canlet.2019.04.034
10. King HW, Michael MZ, Gleadle JM. Hypoxic enhancement of exosome release by breast cancer cells. BMC Cancer (2012) 12:421. doi: 10.1186/1471-2407-12-421
11. Chettimada S, Lorenz DR, Misra V, Dillon ST, Reeves RK, Manickam C, et al. Exosome markers associated with immune activation and oxidative stress in HIV patients on antiretroviral therapy. Sci Rep (2018) 8:7227. doi: 10.1038/s41598-018-25515-4
12. Teng Y, Ren Y, Hu X, Mu J, Samykutty A, Zhuang X, et al. MVP-mediated exosomal sorting of miR-193a promotes colon cancer progression. Nat Commun (2017) 8:14448. doi: 10.1038/ncomms14448
13. Gutschner T, Diederichs S. The hallmarks of cancer: a long non-coding RNA point of view. RNA Biol (2012) 9:703–19. doi: 10.4161/rna.20481
14. Struhl K. Transcriptional noise and the fidelity of initiation by RNA polymerase II. Nat Struct Mol Biol (2007) 14:103–5. doi: 10.1038/nsmb0207-103
15. Zhang Y, Tao Y, Liao Q. Long noncoding RNA: a crosslink in biological regulatory network. Briefings Bioinf (2018) 19:930–45. doi: 10.1093/bib/bbx042
16. Wilusz JE, Sunwoo H, Spector DL. Long noncoding RNAs: functional surprises from the RNA world. Genes Dev (2009) 23:1494–504. doi: 10.1101/gad.1800909
17. Yang G, Lu X, Yuan L. LncRNA: a link between RNA and cancer. Biochim Biophys Acta (2014) 1839:1097–109. doi: 10.1016/j.bbagrm.2014.08.012
18. Matsumoto A, Pasut A, Matsumoto M, Yamashita R, Fung J, Monteleone E, et al. mTORC1 and muscle regeneration are regulated by the LINC00961-encoded SPAR polypeptide. Nature (2017) 541:228–32. doi: 10.1038/nature21034
19. Anderson DM, Anderson KM, Chang C-L, Makarewich CA, Nelson BR, McAnally JR, et al. A micropeptide encoded by a putative long noncoding RNA regulates muscle performance. Cell (2015) 160:595–606. doi: 10.1016/j.cell.2015.01.009
20. Ruiz-Orera J, Messeguer X, Subirana JA, Alba MM. Long non-coding RNAs as a source of new peptides. eLife (2014) 3:e03523. doi: 10.7554/eLife.03523
21. Janakiraman H, House RP, Gangaraju VK, Diehl JA, Howe PH, Palanisamy V. The Long (lncRNA) and Short (miRNA) of It: TGFβ-Mediated Control of RNA-Binding Proteins and Noncoding RNAs. Mol Cancer Res MCR (2018) 16:567–79. doi: 10.1158/1541-7786.MCR-17-0547
22. Dykes IM, Emanueli C. Transcriptional and Post-transcriptional Gene Regulation by Long Non-coding RNA. Genomics Proteomics Bioinf (2017) 15:177–86. doi: 10.1016/j.gpb.2016.12.005
23. Prensner JR, Iyer MK, Sahu A, Asangani IA, Cao Q, Patel L, et al. The long noncoding RNA SChLAP1 promotes aggressive prostate cancer and antagonizes the SWI/SNF complex. Nat Genet (2013) 45:1392–8. doi: 10.1038/ng.2771
24. Huarte M, Guttman M, Feldser D, Garber M, Koziol MJ, Kenzelmann-Broz D, et al. A large intergenic noncoding RNA induced by p53 mediates global gene repression in the p53 response. Cell (2010) 142:409–19. doi: 10.1016/j.cell.2010.06.040
25. Gupta RA, Shah N, Wang KC, Kim J, Horlings HM, Wong DJ, et al. Long non-coding RNA HOTAIR reprograms chromatin state to promote cancer metastasis. Nature (2010) 464:1071–6. doi: 10.1038/nature08975
26. Jiang X, Yan Y, Hu M, Chen X, Wang Y, Dai Y, et al. Increased level of H19 long noncoding RNA promotes invasion, angiogenesis, and stemness of glioblastoma cells. J neurosurg (2016) 124:129–36. doi: 10.3171/2014.12.JNS1426
27. Hung C-L, Wang L-Y, Yu Y-L, Chen H-W, Srivastava S, Petrovics G, et al. A long noncoding RNA connects c-Myc to tumor metabolism. Proc Natl Acad Sci United States America (2014) 111:18697–702. doi: 10.1073/pnas.1415669112
28. Feng S, Yao J, Chen Y, Geng P, Zhang H, Ma X, et al. Expression and Functional Role of Reprogramming-Related Long Noncoding RNA (lincRNA-ROR) in Glioma. J Mol Neurosci MN (2015) 56:623–30. doi: 10.1007/s12031-014-0488-z
29. Liang H, Yu T, Han Y, Jiang H, Wang C, You T, et al. and invasion-metastasis in serous ovarian cancer by competitively binding miR-101-3p to regulate ZEB1 expression. Mol Cancer (2018) 17:119. doi: 10.1186/s12943-018-0870-5
30. Zhou R, Chen KK, Zhang J, Xiao B, Huang Z, Ju C, et al. The decade of exosomal long RNA species: an emerging cancer antagonist. Mol Cancer (2018) 17:75. doi: 10.1186/s12943-018-0823-z
31. Zhao R, Zhang Y, Zhang X, Yang Y, Zheng X, Li X, et al. Exosomal long noncoding RNA HOTTIP as potential novel diagnostic and prognostic biomarker test for gastric cancer. Mol Cancer (2018) 17:68. doi: 10.1186/s12943-018-0817-x
32. Shao H, Im H, Castro CM, Breakefield X, Weissleder R, Lee H. New Technologies for Analysis of Extracellular Vesicles. Chem Rev (2018) 118:1917–50. doi: 10.1021/acs.chemrev.7b00534
33. Théry C. Exosomes: secreted vesicles and intercellular communications. F1000 Biol Rep (2011) 3:15. doi: 10.3410/B3-15
34. Xu R, Rai A, Chen M, Suwakulsiri W, Greening DW, Simpson RJ. Extracellular vesicles in cancer - implications for future improvements in cancer care. Nat Rev Clin Oncol (2018) 15:617–38. doi: 10.1038/s41571-018-0036-9
35. Wang FW, Cao CH, Han K, Zhao YX, Cai MY, Xiang ZC, et al. APC-activated long noncoding RNA inhibits colorectal carcinoma pathogenesis through reduction of exosome production. J Clin Invest (2019) 129:727–43. doi: 10.1172/JCI122478
36. Christ L, Raiborg C, Wenzel EM, Campsteijn C, Stenmark H. Cellular Functions and Molecular Mechanisms of the ESCRT Membrane-Scission Machinery. Trends Biochem Sci (2017) 42:42–56. doi: 10.1016/j.tibs.2016.08.016
37. Babst M. MVB vesicle formation: ESCRT-dependent, ESCRT-independent and everything in between. Curr Opin Cell Biol (2011) 23:452–7. doi: 10.1016/j.ceb.2011.04.008
38. Fraschilla I, Pillai S. Viewing Siglecs through the lens of tumor immunology. Immunol Rev (2017) 276:178–91. doi: 10.1111/imr.12526
39. Miyanishi M, Tada K, Koike M, Uchiyama Y, Kitamura T, Nagata S. Identification of Tim4 as a phosphatidylserine receptor. Nature (2007) 450:435–9. doi: 10.1038/nature06307
40. Christianson HC, Svensson KJ, van Kuppevelt TH, Li J-P, Belting M. Cancer cell exosomes depend on cell-surface heparan sulfate proteoglycans for their internalization and functional activity. Proc Natl Acad Sci United States America (2013) 110:17380–5. doi: 10.1073/pnas.1304266110
41. Feng D, Zhao W-L, Ye Y-Y, Bai X-C, Liu R-Q, Chang L-F, et al. Cellular internalization of exosomes occurs through phagocytosis. Traffic (Copenhagen Denmark) (2010) 11:675–87. doi: 10.1111/j.1600-0854.2010.01041.x
42. Bu H-F, Wang X, Tang Y, Koti V, Tan X-D. Toll-like receptor 2-mediated peptidoglycan uptake by immature intestinal epithelial cells from apical side and exosome-associated transcellular transcytosis. J Cell Physiol (2010) 222:658–68. doi: 10.1002/jcp.21985
43. Lee JT. Epigenetic regulation by long noncoding RNAs. Sci (New York N.Y.) (2012) 338:1435–9. doi: 10.1126/science.1231776
44. Kopp F, Mendell JT. Functional Classification and Experimental Dissection of Long Noncoding RNAs. Cell (2018) 172:393–407. doi: 10.1016/j.cell.2018.01.011
45. Quinn JJ, Chang HY. Unique features of long non-coding RNA biogenesis and function. Nat Rev Genet (2016) 17:47–62. doi: 10.1038/nrg.2015.10
46. Qiao L, Hu S, Huang K, Su T, Li Z, Vandergriff A, et al. Tumor cell-derived exosomes home to their cells of origin and can be used as Trojan horses to deliver cancer drugs. Theranostics (2020) 10:3474–87. doi: 10.7150/thno.39434
47. Qu L, Ding J, Chen C, Wu Z-J, Liu B, Gao Y, et al. Exosome-Transmitted lncARSR Promotes Sunitinib Resistance in Renal Cancer by Acting as a Competing Endogenous RNA. Cancer Cell (2016) 29:653–68. doi: 10.1016/j.ccell.2016.03.004
48. Stark R, Grzelak M, Hadfield J. RNA sequencing: the teenage years. Nature reviews. Genetics (2019) 20:631–56. doi: 10.1038/s41576-019-0150-2
49. Yu S, Li Y, Liao Z, Wang Z, Wang Z, Li Y, et al. Plasma extracellular vesicle long RNA profiling identifies a diagnostic signature for the detection of pancreatic ductal adenocarcinoma. Gut (2020) 69:540–50. doi: 10.1136/gutjnl-2019-318860
50. Chen Y, Ding H, Wei M, Zha W, Guan S, Liu N, et al. MSC-Secreted Exosomal H19 Promotes Trophoblast Cell Invasion and Migration by Downregulating let-7b and Upregulating FOXO1. Molecular therapy. Nucleic Acids (2020) 19:1237–49. doi: 10.1016/j.omtn.2019.11.031
51. Ma X, Li Z, Li T, Zhu L, Li Z, Tian N. Long non-coding RNA HOTAIR enhances angiogenesis by induction of VEGFA expression in glioma cells and transmission to endothelial cells via glioma cell derived-extracellular vesicles. Am J Trans Res (2017) 9:5012–21.
52. Li S, Li Y, Chen B, Zhao J, Yu S, Tang Y, et al. exoRBase: a database of circRNA, lncRNA and mRNA in human blood exosomes. Nucleic Acids Res (2018) 46:D106–12. doi: 10.1093/nar/gkx891
53. Rozowsky J, Kitchen RR, Park JJ, Galeev TR, Diao J, Warrell J, et al. exceRpt: A Comprehensive Analytic Platform for Extracellular RNA Profiling. Cell Syst (2019) 8(4):352–7.e3. doi: 10.1016/j.cels.2019.03.004
54. Hanahan D, Weinberg RA. Hallmarks of cancer: the next generation. Cell (2011) 144:646–74. doi: 10.1016/j.cell.2011.02.013
55. Lobb RJ, Lima LG, Möller A. Exosomes: Key mediators of metastasis and pre-metastatic niche formation. Semin Cell Dev Biol (2017) 67:3–10. doi: 10.1016/j.semcdb.2017.01.004
56. Paget S. The distribution of secondary growths in cancer of the breast. 1889. Cancer metastasis Rev (1989) 8(2):98–101.
57. Costa-Silva B, Aiello NM, Ocean AJ, Singh S, Zhang H, Thakur BK, et al. Pancreatic cancer exosomes initiate pre-metastatic niche formation in the liver. Nat Cell Biol (2015) 17:816–26. doi: 10.1038/ncb3169
58. Poggio M, Hu T, Pai CC, Chu B, Belair CD, Chang A, et al. Suppression of Exosomal PD-L1 Induces Systemic Anti-tumor Immunity and Memory. Cell (2019) 177:414–427 e413. doi: 10.1016/j.cell.2019.02.016
59. Huang Y, Song N, Ding Y, Yuan S, Li X, Cai H, et al. Pulmonary vascular destabilization in the premetastatic phase facilitates lung metastasis. Cancer Res (2009) 69:7529–37. doi: 10.1158/0008-5472.CAN-08-4382
60. Liu Y, Gu Y, Han Y, Zhang Q, Jiang Z, Zhang X, et al. Tumor Exosomal RNAs Promote Lung Pre-metastatic Niche Formation by Activating Alveolar Epithelial TLR3 to Recruit Neutrophils. Cancer Cell (2016) 30:243–56. doi: 10.1016/j.ccell.2016.06.021
61. Sleeman JP. The metastatic niche and stromal progression. Cancer metastasis Rev (2012) 31:429–40. doi: 10.1007/s10555-012-9373-9
62. Benito-Martin A, Di Giannatale A, Ceder S, Peinado H. The new deal: a potential role for secreted vesicles in innate immunity and tumor progression. Front Immunol (2015) 6:66. doi: 10.3389/fimmu.2015.00066
63. Fang T, Lv H, Lv G, Li T, Wang C, Han Q, et al. Tumor-derived exosomal miR-1247-3p induces cancer-associated fibroblast activation to foster lung metastasis of liver cancer. Nat Commun (2018) 9:191. doi: 10.1038/s41467-017-02583-0
64. Wang Y, Yin K, Tian J, Xia X, Ma J, Tang X, et al. Granulocytic Myeloid-Derived Suppressor Cells Promote the Stemness of Colorectal Cancer Cells through Exosomal S100A9. Adv Sci (Weinh) (2019) 6:1901278. doi: 10.1002/advs.201901278
65. Liang Y, Song X, Li Y, Chen B, Zhao W, Wang L, et al. LncRNA BCRT1 promotes breast cancer progression by targeting miR-1303/PTBP3 axis. Mol Cancer (2020) 19:85. doi: 10.1186/s12943-020-01206-5
66. Ni C, Fang Q-Q, Chen W-Z, Jiang J-X, Jiang Z, Ye J, et al. Breast cancer-derived exosomes transmit lncRNA SNHG16 to induce CD73+γδ1 Treg cells. Signal transduct targeted Ther (2020) 5:41. doi: 10.1038/s41392-020-0129-7
67. Chen C, Luo Y, He W, Zhao Y, Kong Y, Liu H, et al. Exosomal long noncoding RNA LNMAT2 promotes lymphatic metastasis in bladder cancer. J Clin Invest (2020) 130:404–21. doi: 10.1172/JCI130892
68. Li B, Xu H, Han H, Song S, Zhang X, Ouyang L, et al. Exosome-mediated transfer of lncRUNX2-AS1 from multiple myeloma cells to MSCs contributes to osteogenesis. Oncogene (2018) 37:5508–19. doi: 10.1038/s41388-018-0359-0
69. Conigliaro A, Costa V, Lo Dico A, Saieva L, Buccheri S, Dieli F, et al. CD90+ liver cancer cells modulate endothelial cell phenotype through the release of exosomes containing H19 lncRNA. Mol Cancer (2015) 14:155. doi: 10.1186/s12943-015-0426-x
70. Bian E-B, Chen E-F, Xu Y-D, Yang Z-H, Tang F, Ma C-C, et al. Exosomal lncRNA−ATB activates astrocytes that promote glioma cell invasion. Int J Oncol (2019) 54:713–21. doi: 10.3892/ijo.2018.4644
71. Li X, Lei Y, Wu M, Li N. Regulation of Macrophage Activation and Polarization by HCC-Derived Exosomal lncRNA TUC339. Int J Mol Sci (2018) 19(10):2958. doi: 10.3390/ijms19102958
72. Lang HL, Hu GW, Chen Y, Liu Y, Tu W, Lu YM, et al. Glioma cells promote angiogenesis through the release of exosomes containing long non-coding RNA POU3F3. Eur Rev Med Pharmacol Sci (2017) 21:959–72.
73. Lang H-L, Hu G-W, Zhang B, Kuang W, Chen Y, Wu L, et al. Glioma cells enhance angiogenesis and inhibit endothelial cell apoptosis through the release of exosomes that contain long non-coding RNA CCAT2. Oncol Rep (2017) 38:785–98. doi: 10.3892/or.2017.5742
74. Qiu J-J, Lin X-J, Tang X-Y, Zheng T-T, Lin Y-Y, Hua K-Q. Exosomal Metastasis−Associated Lung Adenocarcinoma Transcript 1 Promotes Angiogenesis and Predicts Poor Prognosis in Epithelial Ovarian Cancer. Int J Biol Sci (2018) 14:1960–73. doi: 10.7150/ijbs.28048
75. Han M, Gu Y, Lu P, Li J, Cao H, Li X, et al. Exosome-mediated lncRNA AFAP1-AS1 promotes trastuzumab resistance through binding with AUF1 and activating ERBB2 translation. Mol Cancer (2020) 19:26. doi: 10.1186/s12943-020-1145-5
76. Chen C-W, Fu M, Du Z-H, Zhao F, Yang W-W, Xu L-H, et al. Long noncoding RNA MRPL23-AS1 Promotes Adenoid Cystic Carcinoma Lung Metastasis. Cancer Res (2020) 80(11):3373–85. doi: 10.1158/0008-5472.CAN-19-0819
77. Zhang Z, Yin J, Lu C, Wei Y, Zeng A, You Y. Exosomal transfer of long non-coding RNA SBF2-AS1 enhances chemoresistance to temozolomide in glioblastoma. J Exp Clin Cancer Res CR (2019) 38:166. doi: 10.1186/s13046-019-1139-6
78. Li Z, Jiang P, Li J, Peng M, Zhao X, Zhang X, et al. Tumor-derived exosomal lnc-Sox2ot promotes EMT and stemness by acting as a ceRNA in pancreatic ductal adenocarcinoma. Oncogene (2018) 37:3822–38. doi: 10.1038/s41388-018-0237-9
79. Xue M, Chen W, Xiang A, Wang R, Chen H, Pan J, et al. Hypoxic exosomes facilitate bladder tumor growth and development through transferring long non-coding RNA-UCA1. Mol Cancer (2017) 16:143. doi: 10.1186/s12943-017-0714-8
80. Wu D-M, Deng S-H, Liu T, Han R, Zhang T, Xu Y. TGF-β-mediated exosomal lnc-MMP2-2 regulates migration and invasion of lung cancer cells to the vasculature by promoting MMP2 expression. Cancer Med (2018) 7:5118–29. doi: 10.1002/cam4.1758
81. Hardin H, Helein H, Meyer K, Robertson S, Zhang R, Zhong W, et al. Thyroid cancer stem-like cell exosomes: regulation of EMT via transfer of lncRNAs. Lab investig J Tech Methods Pathol (2018) 98:1133–42. doi: 10.1038/s41374-018-0065-0
82. Pan L, Liang W, Fu M, Huang Z-H, Li X, Zhang W, et al. Exosomes-mediated transfer of long noncoding RNA ZFAS1 promotes gastric cancer progression. J Cancer Res Clin Oncol (2017) 143(6):991–1004. doi: 10.1007/s00432-017-2361-2
83. Chen F, Chen J, Yang L, Liu J, Zhang X, Zhang Y, et al. Extracellular vesicle-packaged HIF-1α-stabilizing lncRNA from tumour-associated macrophages regulates aerobic glycolysis of breast cancer cells. Nat Cell Biol (2019) 21:498–510. doi: 10.1038/s41556-019-0299-0
84. Ren J, Ding L, Zhang D, Shi G, Xu Q, Shen S, et al. Carcinoma-associated fibroblasts promote the stemness and chemoresistance of colorectal cancer by transferring exosomal lncRNA H19. Theranostics (2018) 8:3932–48. doi: 10.7150/thno.25541
85. Zheng R, Du M, Wang X, Xu W, Liang J, Wang W, et al. Exosome-transmitted long non-coding RNA PTENP1 suppresses bladder cancer progression. Mol Cancer (2018) 17:143. doi: 10.1186/s12943-018-0880-3
86. Psaila B, Lyden D. The metastatic niche: adapting the foreign soil. Nat Rev Cancer (2009) 9:285–93. doi: 10.1038/nrc2621
87. Song W, Yan D, Wei T, Liu Q, Zhou X, Liu J. Tumor-derived extracellular vesicles in angiogenesis. Biomedi pharmacother = Biomed pharmacother (2018) 102:1203–8. doi: 10.1016/j.biopha.2018.03.148
88. Todorova D, Simoncini S, Lacroix R, Sabatier F, Dignat-George F. Extracellular Vesicles in Angiogenesis. Circ Res (2017) 120:1658–73. doi: 10.1161/CIRCRESAHA.117.309681
89. Peinado H, Alečković M, Lavotshkin S, Matei I, Costa-Silva B, Moreno-Bueno G, et al. Melanoma exosomes educate bone marrow progenitor cells toward a pro-metastatic phenotype through MET. Nat Med (2012) 18:883–91. doi: 10.1038/nm.2753
90. Zeng Z, Li Y, Pan Y, Lan X, Song F, Sun J, et al. Cancer-derived exosomal miR-25-3p promotes pre-metastatic niche formation by inducing vascular permeability and angiogenesis. Nat Commun (2018) 9:5395. doi: 10.1038/s41467-018-07810-w
91. Hautmann RE, de Petriconi RC, Pfeiffer C, Volkmer BG. Radical cystectomy for urothelial carcinoma of the bladder without neoadjuvant or adjuvant therapy: long-term results in 1100 patients. Eur Urol (2012) 61:1039–47. doi: 10.1016/j.eururo.2012.02.028
92. Saharinen P, Tammela T, Karkkainen MJ, Alitalo K. Lymphatic vasculature: development, molecular regulation and role in tumor metastasis and inflammation. Trends Immunol (2004) 25:387–95. doi: 10.1016/j.it.2004.05.003
93. Li X, Song Y, Liu F, Liu D, Miao H, Ren J, et al. Long Non-Coding RNA MALAT1 Promotes Proliferation, Angiogenesis, and Immunosuppressive Properties of Mesenchymal Stem Cells by Inducing VEGF and IDO. J Cell Biochem (2017) 118:2780–91. doi: 10.1002/jcb.25927
94. Molinaro AM, Taylor JW, Wiencke JK, Wrensch MR. Genetic and molecular epidemiology of adult diffuse glioma. Nat Rev Neurol (2019) 15:405–17. doi: 10.1038/s41582-019-0220-2
95. Winder T, Lenz H-J. Vascular endothelial growth factor and epidermal growth factor signaling pathways as therapeutic targets for colorectal cancer. Gastroenterology (2010) 138:2163–76. doi: 10.1053/j.gastro.2010.02.005
96. Liu C, Yu S, Zinn K, Wang J, Zhang L, Jia Y, et al. Murine mammary carcinoma exosomes promote tumor growth by suppression of NK cell function. J Immunol (Baltimore Md. 1950) (2006) 176:1375–85. doi: 10.4049/jimmunol.176.3.1375
97. Whiteside TL. Exosomes and tumor-mediated immune suppression. J Clin Invest (2016) 126:1216–23. doi: 10.1172/JCI81136
98. Wu D, Wu P, Wu X, Ye J, Wang Z, Zhao S, et al. expanded human circulating Vδ1 γδT cells exhibit favorable therapeutic potential for colon cancer. Oncoimmunology (2015) 4:e992749. doi: 10.4161/2162402X.2014.992749
99. Peng G, Wang HY, Peng W, Kiniwa Y, Seo KH, Wang R-F. Tumor-infiltrating gammadelta T cells suppress T and dendritic cell function via mechanisms controlled by a unique toll-like receptor signaling pathway. Immunity (2007) 27:334–48. doi: 10.1016/j.immuni.2007.05.020
100. Lewis CE, Pollard JW. Distinct role of macrophages in different tumor microenvironments. Cancer Res (2006) 66:605–12. doi: 10.1158/0008-5472.CAN-05-4005
101. Chen P, Bonaldo P. Role of macrophage polarization in tumor angiogenesis and vessel normalization: implications for new anticancer therapies. Int Rev Cell Mol Biol (2013) 301:1–35. doi: 10.1016/B978-0-12-407704-1.00001-4
102. Gil-Bernabé AM, Ferjancic S, Tlalka M, Zhao L, Allen PD, Im JH, et al. Recruitment of monocytes/macrophages by tissue factor-mediated coagulation is essential for metastatic cell survival and premetastatic niche establishment in mice. Blood (2012) 119:3164–75. doi: 10.1182/blood-2011-08-376426
103. Wu Q, Wu X, Ying X, Zhu Q, Wang X, Jiang L, et al. Suppression of endothelial cell migration by tumor associated macrophage-derived exosomes is reversed by epithelial ovarian cancer exosomal lncRNA. Cancer Cell Int (2017) 17:62. doi: 10.1186/s12935-017-0430-x
104. Kaplan RN, Riba RD, Zacharoulis S, Bramley AH, Vincent L, Costa C, et al. VEGFR1-positive haematopoietic bone marrow progenitors initiate the pre-metastatic niche. Nature (2005) 438:820–7. doi: 10.1038/nature04186
105. Miller KD, Nogueira L, Mariotto AB, Rowland JH, Yabroff KR, Alfano CM, et al. Cancer treatment and survivorship statistics, 2019. CA: Cancer J clinicians (2019) 69:363–85. doi: 10.3322/caac.21565
106. Wolfgang CL, Herman JM, Laheru DA, Klein AP, Erdek MA, Fishman EK, et al. Recent progress in pancreatic cancer. CA: Cancer J clinicians (2013) 63:318–48. doi: 10.3322/caac.21190
107. Graeber TG, Osmanian C, Jacks T, Housman DE, Koch CJ, Lowe SW, et al. Hypoxia-mediated selection of cells with diminished apoptotic potential in solid tumours. Nature (1996) 379:88–91. doi: 10.1038/379088a0
108. Jung H-Y, Fattet L, Yang J. Molecular pathways: linking tumor microenvironment to epithelial-mesenchymal transition in metastasis. Clin Cancer Res an Off J Am Assoc Cancer Res (2015) 21:962–8. doi: 10.1158/1078-0432.CCR-13-3173
109. Lu X, Kang Y. Hypoxia and hypoxia-inducible factors: master regulators of metastasis. Clin Cancer Res an Off J Am Assoc Cancer Res (2010) 16:5928–35. doi: 10.1158/1078-0432.CCR-10-1360
110. Jing X, Yang F, Shao C, Wei K, Xie M, Shen H, et al. Role of hypoxia in cancer therapy by regulating the tumor microenvironment. Mol Cancer (2019) 18:157. doi: 10.1186/s12943-019-1089-9
111. Lin Y-H, Wu M-H, Yeh C-T, Lin K-H. Long Non-Coding RNAs as Mediators of Tumor Microenvironment and Liver Cancer Cell Communication. Int J Mol Sci (2018) 19(12):3742. doi: 10.3390/ijms19123742
112. Ling H, Fabbri M, Calin GA. MicroRNAs and other non-coding RNAs as targets for anticancer drug development. Nature reviews. Drug Discovery (2013) 12:847–65. doi: 10.1038/nrd4140
113. Guo Y, Ji X, Liu J, Fan D, Zhou Q, Chen C, et al. Effects of exosomes on pre-metastatic niche formation in tumors. Mol Cancer (2019) 18:39. doi: 10.1186/s12943-019-0995-1
114. Kalluri R, Zeisberg M. Fibroblasts in cancer. Nat Rev Cancer (2006) 6:392–401. doi: 10.1038/nrc1877
115. Hsu PP, Sabatini DM. Cancer cell metabolism: Warburg and beyond. Cell (2008) 134:703–7. doi: 10.1016/j.cell.2008.08.021
116. Kong J, Tian H, Zhang F, Zhang Z, Li J, Liu X, et al. Extracellular vesicles of carcinoma-associated fibroblasts creates a pre-metastatic niche in the lung through activating fibroblasts. Mol Cancer (2019) 18:175. doi: 10.1186/s12943-019-1101-4
117. Cui H, Onyango P, Brandenburg S, Wu Y, Hsieh C-L, Feinberg AP. Loss of imprinting in colorectal cancer linked to hypomethylation of H19 and IGF2. Cancer Res (2002) 62:6442–6.
118. Hinger SA, Cha DJ, Franklin JL, Higginbotham JN, Dou Y, Ping J, et al. Diverse Long RNAs Are Differentially Sorted into Extracellular Vesicles Secreted by Colorectal Cancer Cells. Cell Rep (2018) 25(3):715–25.e4. doi: 10.1016/j.celrep.2018.09.054
119. Ahadi A, Brennan S, Kennedy PJ, Hutvagner G, Tran N. Long non-coding RNAs harboring miRNA seed regions are enriched in prostate cancer exosomes. Sci Rep (2016) 6:24922. doi: 10.1038/srep24922
120. Liu R, Li X, Zhu W, Wang Y, Zhao D, Wang X, et al. Cholangiocyte-Derived Exosomal Long Noncoding RNA H19 Promotes Hepatic Stellate Cell Activation and Cholestatic Liver Fibrosis. Hepatology (2019) 70:1317–35. doi: 10.1002/hep.30662
121. Yang B, Feng X, Liu H, Tong R, Wu J, Li C, et al. High-metastatic cancer cells derived exosomal miR92a-3p promotes epithelial-mesenchymal transition and metastasis of low-metastatic cancer cells by regulating PTEN/Akt pathway in hepatocellular carcinoma. Oncogene (2020) 39:6529–43. doi: 10.1038/s41388-020-01450-5
122. Wiklander OPB, Nordin JZ, O’Loughlin A, Gustafsson Y, Corso G, Mäger I, et al. Extracellular vesicle in vivo biodistribution is determined by cell source, route of administration and targeting. J extracell vesicles (2015) 4:26316. doi: 10.3402/jev.v4.26316
123. Takahashi Y, Nishikawa M, Shinotsuka H, Matsui Y, Ohara S, Imai T, et al. Visualization and in vivo tracking of the exosomes of murine melanoma B16-BL6 cells in mice after intravenous injection. J Biotechnol (2013) 165:77–84. doi: 10.1016/j.jbiotec.2013.03.013
124. Salunkhe S, Dheeraj, Basak M, Chitkara D, Mittal A. Surface functionalization of exosomes for target-specific delivery and in vivo imaging & tracking: Strategies and significance. J Controlled release Off J Controlled Release Soc (2020) 326:599–614. doi: 10.1016/j.jconrel.2020.07.042
125. Rashid MH, Borin TF, Ara R, Angara K, Cai J, Achyut BR, et al. Differential in vivo biodistribution of I-labeled exosomes from diverse cellular origins and its implication for theranostic application. Nanomedicine nanotechnol biol Med (2019) 21:102072. doi: 10.1016/j.nano.2019.102072
126. Wang J, Li W, Zhang L, Ban L, Chen P, Du W, et al. Chemically Edited Exosomes with Dual Ligand Purified by Microfluidic Device for Active Targeted Drug Delivery to Tumor Cells. ACS Appl mater interfaces (2017) 9:27441–52. doi: 10.1021/acsami.7b06464
127. Ohno S-i, Takanashi M, Sudo K, Ueda S, Ishikawa A, Matsuyama N, et al. Systemically injected exosomes targeted to EGFR deliver antitumor microRNA to breast cancer cells. Mol Ther J Am Soc Gene Ther (2013) 21:185–91. doi: 10.1038/mt.2012.180
128. Hwang DW, Jo MJ, Lee JH, Kang H, Bao K, Hu S, et al. Chemical Modulation of Bioengineered Exosomes for Tissue-Specific Biodistribution. Adv Ther (2019) 2(11):1900111. doi: 10.1002/adtp.201900111
129. Yu X, Yang X, Horte S, Kizhakkedathu JN, Brooks DE. A pH and thermosensitive choline phosphate-based delivery platform targeted to the acidic tumor microenvironment. Biomaterials (2014) 35:278–86. doi: 10.1016/j.biomaterials.2013.09.052
130. Qi H, Liu C, Long L, Ren Y, Zhang S, Chang X, et al. Blood Exosomes Endowed with Magnetic and Targeting Properties for Cancer Therapy. ACS nano (2016) 10:3323–33. doi: 10.1021/acsnano.5b06939
Keywords: extracellular vesicle, exosome, long non-coding RNA, tumor microenvironment, cancer
Citation: Han S, Qi Y, Luo Y, Chen X and Liang H (2021) Exosomal Long Non-Coding RNA: Interaction Between Cancer Cells and Non-Cancer Cells. Front. Oncol. 10:617837. doi: 10.3389/fonc.2020.617837
Received: 15 October 2020; Accepted: 30 November 2020;
Published: 14 January 2021.
Edited by:
You Zhou, First People’s Hospital of Changzhou, ChinaCopyright © 2021 Han, Qi, Luo, Chen and Liang. This is an open-access article distributed under the terms of the Creative Commons Attribution License (CC BY). The use, distribution or reproduction in other forums is permitted, provided the original author(s) and the copyright owner(s) are credited and that the original publication in this journal is cited, in accordance with accepted academic practice. No use, distribution or reproduction is permitted which does not comply with these terms.
*Correspondence: Xiaoping Chen, chenxpchenxp@163.com; Huifang Liang, lianghuifang1997@126.com