- 1The First Clinical Medical College of Zhejiang Chinese Medical University, Hangzhou, China
- 2Department of Medical Oncology, The First Affiliated Hospital of Zhejiang Chinese Medical University, Hangzhou, China
- 3Department of Cancer Medicine, Hammersmith Hospital, Imperial College Healthcare NHS Trust, London, United Kingdom
Epithelial-to-mesenchymal transition (EMT), a complicated program through which polarized epithelial cells acquire motile mesothelial traits, is regulated by tumor microenvironment. EMT is involved in tumor progression, invasion and metastasis via reconstructing the cytoskeleton and degrading the tumor basement membrane. Accumulating evidence shows that resveratrol, as a non-flavonoid polyphenol, can reverse EMT and inhibit invasion and migration of human tumors via diverse mechanisms and signaling pathways. In the present review, we will summarize the detailed mechanisms and pathways by which resveratrol and its analogs (e.g. Triacetyl resveratrol, 3,5,4’-Trimethoxystilbene) might regulate the EMT process in cancer cells to better understand their potential as novel anti-tumor agents. Resveratrol can also reverse chemoresistance via EMT inhibition and improvement of the antiproliferative effects of conventional treatments. Therefore, resveratrol and its analogs have the potential to become novel adjunctive agents to inhibit cancer metastasis, which might be partly related to their blocking of the EMT process.
Introduction
In the past 10 years, a large number of studies have been conducted to determine cellular and molecular mechanisms in cancer invasion and metastasis (1). Epithelial-to-mesenchymal transition (EMT) has been widely reported to promote the acquisition of metastatic properties for tumor cells by enhancing mobility and invasion (2). EMT, as an invasive phenotype, is a reversible cellular program in which cells shed their epithelial features and adopt mesothelial traits (3), generating both morphological and molecular changes (4). Moreover, EMT is not only associated with cancer cell proliferation, invasion and metastasis, but also with chemoresistance and cancer cell stemness. EMT is known as the initial step of the development of metastasis (5), existing in various tumors such as lung, pancreatic, liver and prostate carcinoma (6–9). Resveratrol (RES) and its analogs reverse EMT to inhibit metastasis and mitigate chemoresistance via different pathways and mechanisms in human tumors (Tables 1 and 2). In the present review, we focus on summarizing and analyzing the mechanisms by which RES and its analogs affect EMT in different cancers, supporting their potential to be promising therapeutic agents.
The EMT Process
EMT is an evolutionarily conserved process whereby epithelial cells lose cell junctions and apical-basal polarity, and are eventually converted into migratory mesenchymal phenotype (64). As a key program, EMT participates in the development of embryogenesis such as gastrulation and tissue morphogenesis, as well as wound healing of adult (65). In addition, EMT has been related to different cancer functions, especially for tumor invasion and metastasis, tumor stemness and resistance to therapy (66). Correspondingly, the resulting mesenchymal cells after migration also can reverse the process, known as mesenchymal-to-epithelial transition (MET), transforming epithelial cells to colonize a particular location (67).
In the EMT process, polarized and immobile epithelial cells with cobblestone-like appearance acquire mesothelial traits with a spindle-like shape and increased cellular motility, by which they are separated from the epithelial basement membrane and invade through surrounding tissues (68) (Figures 1A, B). Then tumor cells intravasate into blood vessels and circulate in the bloodstream, eventually leading to extravasation to distant organs and form metastatic lesions (69) (Figure 1B). This process starts from dissociating of cell-cell connection via the loss of epithelial phenotype including adhesion molecule (E-cadherin), gap- and tight junctions (claudin-2) and desmoplakin (70). With the overexpression of mesenchymal markers (71) such as N-cadherin (N-cad), vimentin (VIM), fibronectin (FN) and α-smooth muscle actin (α-SMA), tumor cells could reconstruct the cytoskeleton, degrade the basement membrane, and remodel extracellular matrix (ECM) by inducing matrix metalloproteinases (MMPs), such as MMP-2 and MMP-9 (72), underlying metastasis to distant organs, stemness maintenance and reversion of chemoresistance (73).
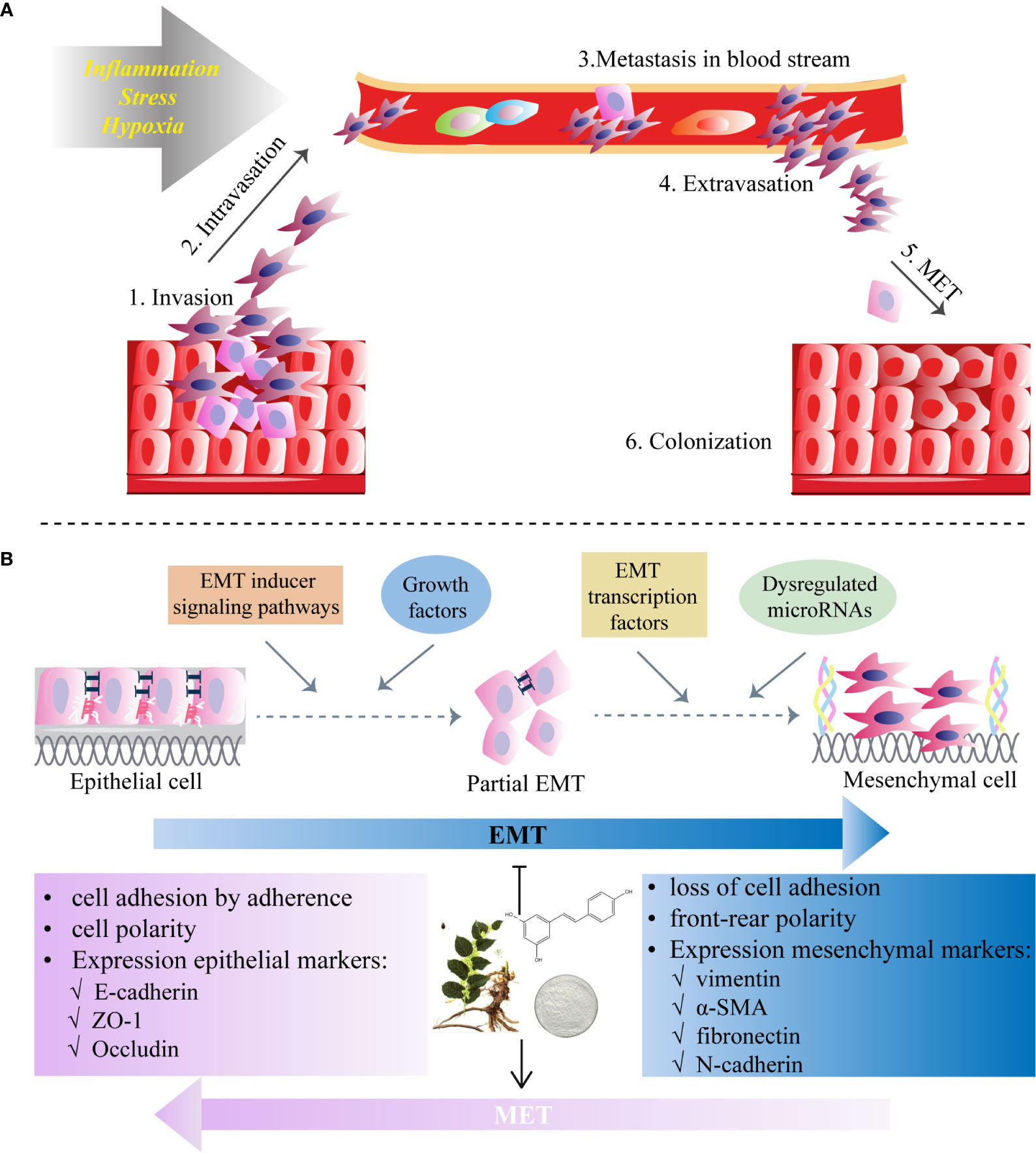
Figure 1 Epithelial-to-mesenchymal transition (EMT) programme in cancers and the inhibitory role of RES. (A) Invasion and migration initiated by the EMT process due to the factors (e.g., inflammation, stress and hypoxia) affect tumor microenvironment. (B) Resveratrol inhibits EMT which starts from diverse processes (EMT inducer signaling pathways, growth factors, dysregulation of microRNAs and EMT transcription factors) and by which polarized epithelial cells acquire motile mesothelial features. The EMT process is characterized by the loss of cell-cell contacts via downregulation of epithelial markers (i.e. E-cadherin in adherent junctions, zonula occludens-1 (ZO-1) in tight junctions and desmoplakin in desmosomes) and upregulation of mesenchymal markers (N-cadherin, vimentin, fibronectin and α-smooth muscle actin (α-SMA)). RES may also induce mesenchymal-to-epithelial transition (MET), a reversal of the EMT process.
Several critical EMT-inducing transcription factors (EMT-TFs), which include zinc-finger E-box binding homeobox 1 (ZEB1), ZEB2 (also known as SIp1), Snail1 (also known as Snail), Slug (also known as Snail2) and Twist-related protein 1(Twist1; also known as Twist), have been demonstrated to play significant regulatory roles in the EMT program of cancer cells (74). In addition to activating the classic EMT traits and promoting tumor metastasis, EMT-TFs are associated with the induction to many other features, like maintenance of cancer stem cells (CSCs) and resistance to therapeutic drugs (75). CSCs are referred to tumor cells with the ability for self-renewal and recapitulation of the tumor heterogeneity (76). The EMT process, as a key regulator of the cancer cell stemness, provides a chance to research the nature of intratumoral heterogeneity and offers a potential mechanistic basis for the resistance of anti-cancer drugs (77).
Transforming growth factor-β (TGF-β1), a secret multifunctional cytokine, is participated in the EMT process, regulating EMT-TFs (78). TGF-β1 can contribute to the EMT program through both Smad-mediated and non-Smad signaling. Smad2 and Smad3 are phosphorylated by TGF-β1 signals, then form hetero trimeric complexes about Smad4, translocating into the nucleus, activating the expression level of EMT-TFs, and cooperating with these transcription factors to regulate EMT-related genes (79). In addition, non-Smad pathways are also involved in the TGF-β1-induced EMT process, such as phosphatidylinositol 3-kinase (PI3K)/AKT/mammalian TOR complex 1 (mTORC1), tumor necrosis factor receptor-associated factor 6 (TRAF6)/TGF β-Activated Kinase 1 (TAK1), and Wnt/β-catenin signaling (80–82).
Resveratrol: A Bioactive Compound
Resveratrol (3,5,4’-trihydroxy-trans-stilbene), initially isolated in 1939 from Veratrum grandiflorum by Takaoka (83), is a phenolic substance present in various plants, including grapes, soy, peanuts and berries as well as the roots of Polygonum cuspidatum, a traditional medicine in China. It has been found to exert diverse pharmacological effects involving anti-inflammation, anti-oxidation, anti-aging, anti-diabetes, anti-obesity, anti-cancer, cardioprotection and neuroprotection (84–86). Interestingly, resveratrol (RES) can regulate various signaling pathways and target diverse effector molecules, as well as alter phenotypes of disease models. RES acts as a natural autophagy regulator for prevention and treatment of Alzheimer’s disease (87). RES also has shown promising effects on inflammatory bowel disease, as its anti-inflammatory and anti-oxidant activity (88). Moreover, RES moderately diminishes systolic blood pressure and blood glucose, exerts numerous vasculoprotective effects by suppressing vascular smooth muscle cell proliferation (89). Increasing evidence revealed that RES had the wide range of preventive and therapeutic roles against various types of tumor (90), including colorectal, breast and lung cancers (15, 23, 27), and involved various phases of cancer development, such as EMT. RES has been reported to regulate numerous functional proteins, including growth factors, inflammatory cytokines, transcription factors, as well as free radicals, which are all participated in the initiation and progression of human cancer (91).
RES exerts its anti-cancer and chemosensitivity effects through regulation of the tumor microenvironment and the malignant biological behaviors, including levels of reactive oxygen species (ROS), proliferation, antiapoptosis, invasion, migration, EMT progress, and stemness (92). As an effective antioxidant, RES can scavenge intracellular ROS via suppression of extracellular signal-regulated kinase 1/2 (ERK1/2) and p38 mitogen-activated protein kinase (p38 MAPK) (93). For the proliferation and viability of tumor cells, RES can induce cell cycle arrest and apoptosis by suppressing Wnt/β-cantenin signaling and downregulating antiapoptotic proteins (Bcl-2 and Bcl-XL), respectively (94, 95). Recent studies have been reported that RES can directly target cytokines, and cellular signaling molecules such as, forkhead box C2 (FOXC2), forkhead box O1 (FOXO1), human telomerase reverse transcriptase (hTERT), glioma-associated oncogene 1 (GLI1), β-catenin, ERK1/2 and nuclear factor-κB (NF-κB), which promote the EMT process in different disorders with a variety of cellular signaling pathways (Figure 2).
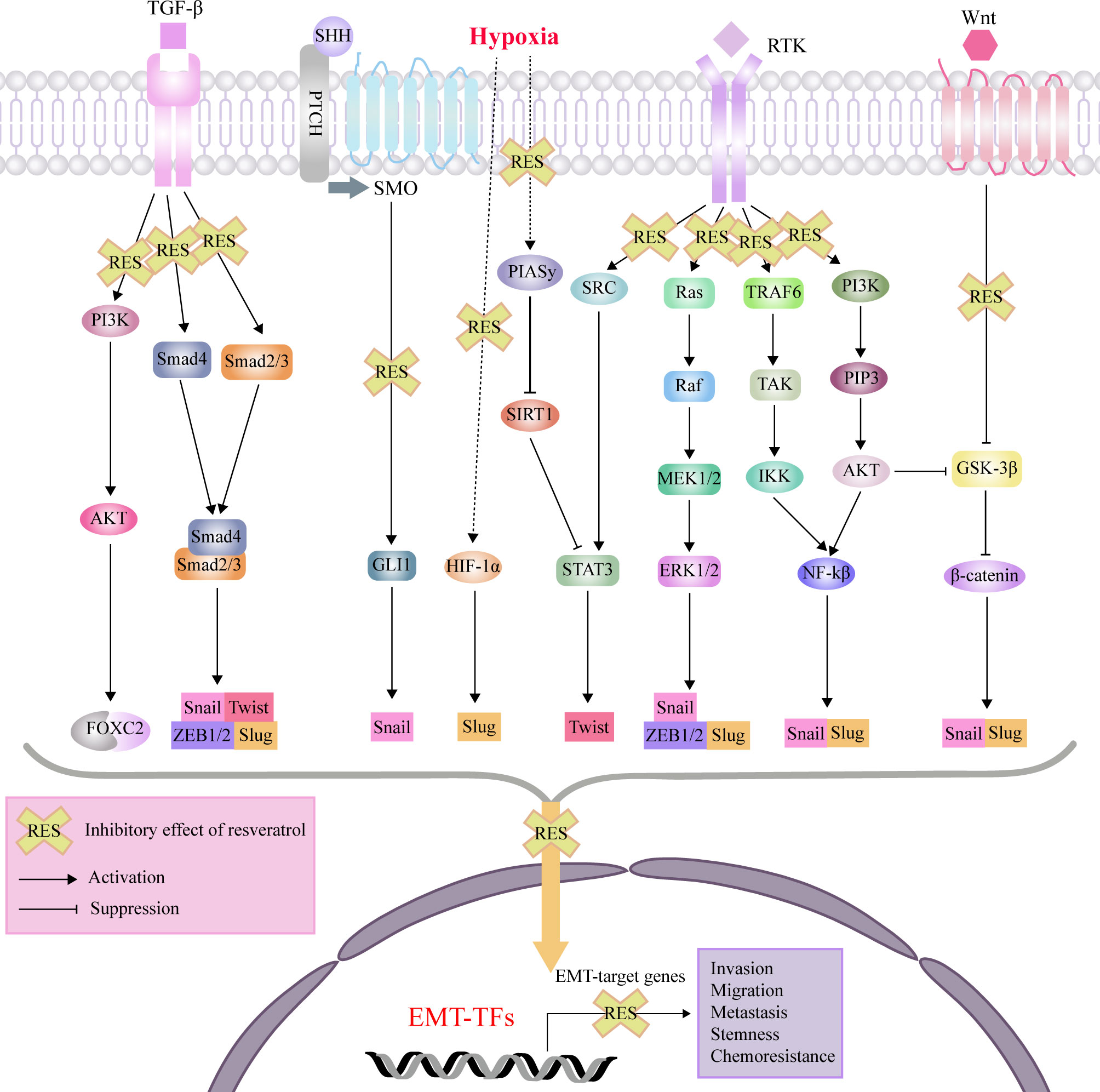
Figure 2 Mechanisms of effects of RES through interference with signaling pathways affecting EMT, invasion, migration, metastasis, tumor stemness and chemoresistance. protein kinase B (AKT); epithelial-mesenchymal transition (EMT); extracellular signal-regulated kinase (ERK); forkhead box C2 (FOXC2); glioma-associated oncogene 1 (GLI1); glycogen synthase kinase 3β (GSK-3β); hypoxia-inducible factor-1 (HIF-1); inhibitor of nuclear factor kappa-B kinase (IKK); nuclear factor-κB (NF-κB); MAP kinse-ERK kinase (MEK); protein inhibitor of activated STAT 4 (PIASy); patched (PTCH); phosphatidylinositol 3-kinase (PI3K); plasma membrane intrinsic protein 3 (PIP3); receptor tyrosine kinases (RTK); sonic hedgehog (SHH); sirtuin1(SIRT1); smoothened (SMO); signal transducer and activator of transcription 3 (STAT3); transforming growth factor-β (TGF-β); TGF beta-Activated Kinase (TAK); TNF-receptor associated factor 6 (TRAF6); zinc finger E-box binding homeobox (ZEB).
Effects of Resveratrol on EMT in Human Cancers
Digestive Cancers
Gastric Cancer
There have been accumulating clinical trials which demonstrated that doxorubicin (DOX)-based regimens failed to attain the favorable survival goal and brought about some side effects for patients with gastric cancer (96, 97). EMT participates not only in the cancer metastasis but also in the appearance of chemoresistance (98). EMT-mediated drug resistance is modulated by several signalings, among which PI3K/AKT pathway is of high attention (99). RES can reverse DOX resistance via the inhibition of EMT by suppressing mesenchymal markers (β-catenin and vimentin) through regulating phosphatase and tensin homolog deleted on chromosome ten (PTEN)/AKT signaling pathway (10). Besides RES can further promote apoptosis (10). That’s say, RES combined with DOX can not only suppress the growth of gastric cancer cells, but also alleviate the resistance of DOX and prohibit cell migration through the reverse of EMT by regulating PTEN/AKT pathway.
Metastasis-associated lung adenocarcinoma transcript 1 (MALAT1), which is also named by nuclear-enriched abundant transcript 2, has a strong function in EMT, proliferation, apoptosis and so on (100). In Yang’s gastric cancer cell model, RES was found to prevent EMT by down-regulating MALAT1 (11), which provided new evidence for anticancer mechanism of RES in vitro.
It is believed that the hedgehog signaling pathway could exert a critical role in vertebrate growth. e.g. the homeostatic process and tumorigenesis (101). Gli-1 is considered as a marker when the hedgehog signaling pathway activates abnormally (102). RES can prevent hypoxia-induced gastric cancer invasion and EMT by suppressing the hedgehog signaling as proved that RES can reduce the expression of Gli-1, Snail and N-cadherin, while enhancing the expression of E-cadherin (12, 13). The mechanism may be explained that Gli-1 directly induced Snail which marks the process of EMT (103), so the expression of Snail is decreased as soon as Gli-1 is suppressed. Moreover, RES can target mesenchymal stem cells derived from gastric cancer by inactivating the Wnt/β-catenin signaling pathway (14).
Colorectal Cancer
5-FU is routinely applied to the treatment of colorectal cancer, but its toxicity and ineffectiveness in many patients limit its wide application. Furthermore, over 50% of patients show resistance to 5-FU in the therapeutic course (15). RES has been found to inactive the NF-κB signaling pathway potently in earlier researches (104–106). The NF-κB signaling pathway not only closely links to inflammation and cancer (107), but also propels the process of EMT and metastasis (17). RES significantly attenuated 5-FU-induced EMT by down-regulation of NF-κB activation, which is proved by the evidence that RES suppresses EMT factors (down-regulating the expression of Slug and vimentin as well as up-regulating of E-cadherin) and overexpresses the gap and tight junctions (claudin-2) in colorectal cancer cells (15). Based on these findings, RES may perform as an anticancer agent in colorectal cancer.
The process of EMT can be triggered by many cytokines such as TGF-β1, HGF and so on (108–110). Different signaling pathways and mechanisms connected with EMT were studied, like TGF-β1/Smads pathway Qing Ji’s team focused on (16). They found that RES exerted an inhibitory role on EMT of colorectal cancer cells both in vivo and in vitro. RES has the potential to suppress EMT via TGF-β1/Smads signaling, as proved by the up-regulating of E-cadherin and the down-regulating of Snail and vimentin (16). Snail, a TGF-β1/Smad signaling pathway modulated gene (111, 112), restrains the E-cadherin expression and triggers the EMT process (16). Furthermore, they also found that with the treatment of RES, TGF-β1-induced MMP2 and MMP9 decreased through expression analysis (16). These findings may explain how RES inhibit invasion and metastases.
Previous studies reported that inflammatory cytokines like members of the tumor necrosis factor (TNF)-superfamily could change the tumor microenvironment and promote the progression of colorectal cancer through activation of EMT. These pro-inflammatory mediators are modulated via the transcription factor NF-κB (113–116). It has also been reported that focal adhesion kinase (FAK) associated with NF-κB regulates the cancer cells capacity of survival, invasiveness, and metastasis (117–120). Buhrmann and co-workers showed that RES inhibited the TNF-β-induced EMT as demonstrated by suppressing EMT factors (up-modulating vimentin and slug, down-modulating E-cadherin) in colorectal cancer cells through preventing the FAK/NF-κB activation as proved by down-regulation of MMP-9 and C-X-C motif chemokine receptor 4 (CXCR4) which are the NF-κB-dependent of tumor-promoting factors (17, 18). Moreover, it has been reported that cancer stem cells (CSCs) had stem cell characteristics like pluripotency, self-renewal, invasion, motility (121–124). CD133, CD44 and aldehyde dehydrogenase gene 1 (ALDH1), as molecules associate with CSCs, are widely used to mark CSCs (125, 126). The researchers also found that RES restrained CSC-like phenotype (CD133, CD44, ALDH1) to suppress the formation of CSCs (18). Targeting both stemness of cells and EMT underscores the potential of RES to develop a new therapeutic strategy for colorectal cancer.
Serine/threonine kinase (AKT) is closely linked to EMT (80) and is also related to some biological and pathological proceedings, like angiogenesis, invasion, and metastasis (127). Moreover, it has been found that the glycogen synthase kinase (GSK)−3β pathway often involves in the process of EMT because it is the downstream pathway of AKT (128). Furthermore, the AKT/GSK−3β signaling pathway can modulate the expression of Snail and promote EMT in some cancer cells (129, 130). Li and colleagues revealed that RES prevented EMT via AKT/GSK3β/Snail signaling pathway in colon cancer, and they observed markedly increased E−cadherin, whilst the decreased expression of N−cadherin both in vitro and in vivo (19). Concordantly, the activation of phospho (p)−AKT1, p−GSK−3β, and Snail also decreased (19). Taken together, these findings demonstrate that RES may provide new treatment for colon cancer.
More recently, it has been indicated that the miR-200 family exerts a vital role on the modulation of the EMT process (131). ZEB1, considered as the target gene of miR-200 family, can repress E-cadherin to promote EMT process, subsequently bring about cancer progression (132). Based on the data of Dermani’s team, RES could inhibit EMT by enhancing miR-200c expression in colorectal cancer cells, its mechanism might be explained as targeting ZEB1 and vimentin expression (20).
Up to now, there are involving some clinical study of resveratrol in cancer treatment. It was reported that long non-coding (Lnc) MALAT1 was upregulated in colorectal cancer tissues and RES inhibits invasion and metastasis via Lnc MALAT1 mediated Wnt/β-catenin signal pathway (133). Patel et al. found that daily oral doses of resveratrol at 0.5 or 1.0g makes the resected tissues keep resveratrol and its metabolite resveratrol-3-O-glucuronide with proper concentrations, exerting antitumor effects for patients with colorectal cancer (134). Moreover, Howells et al. conducted a phase I randomized, double-blind pilot study and assessed the safety, pharmacokinetics, and pharmacodynamics of micronized resveratrol (SRT501) for colorectal cancer patients with patients with hepatic metastases. They revealed that SRT501 was well tolerated and cleaved caspase-3, a marker of apoptosis, significantly increased by 39% in metastatic hepatic tissues from the SRT501 -treated patients compared with tissues following placebo treatment (135).
Pancreatic Cancer and Cholangiocarcinoma
The CSCs have the potential of renewal, proliferation, clonogenicity, and multipotency as adult stem cells possess (136). From the cancer stem cell theory, the dissemination of a CSC induces sustained metastatic growth which represents the primary tumor re-establishment in a secondary site (21). Recent reports suggest the CSCs partly cause the emergence of EMT, through implications from tumor stromal components. In several human cancers including pancreatic cancer, CSCs and cells with EMT-type exert a critical role in drug resistance and early metastatic process (137, 138). In Shankar’s study, RES brought the decrement of EMT markers (ZEB-1, Slug and Snail) in CSCs (21). Moreover, Qin et al. reported that resveratrol suppressed CSC-like properties and EMT on of pancreatic cancer cells via downregulating the expression of nutrient-deprivation autophagy factor-1 (NAF-1) (25).
Tumor hypoxia, a microenvironment usually found in the central part of tumors (139), promotes the process of cancer invasion and metastasis (140). Hypoxia-inducible factor-1 (HIF-1) is the most critical transcription factor of hypoxia (141). Li et al. found that RES reversed EMT by inhibiting hypoxia-mediated activation of the Hedgehog pathway and observed RES restrained the expression of HIF-1α protein as well as uPA and MMP, which are known as metastatic-related factors (22). For the above-mentioned reasons, RES may be a potential choice of prevention and treatment for pancreatic cancer.
The PI3K/AKT signaling pathway has important influence in pancreatic cancer metastasis (23). Recent studies indicate that NF-κB transcription factor might regulate the PI3K/AKT signaling pathway to trigger the metastasis-mediated effect (142). The authors show that RES reduces the expressions of p-AKT and p-NF-κB to suppress the PI3K/AKT/NF-κB pathway, inhibiting the invasion and migration of pancreatic cells as proved by suppressing the level of EMT-related markers (N-cadherin and vimentin) (23). Pancreatic stellate cells (PSCs) are usually activated and become fibroblasts in the microenvironment of desmoplastic stroma. RES is reported to block hypoxia-induced PSCs activation and inhibit the interaction between PSCs and pancreatic cancer cells (24).
Cancer associated fibroblasts (CAFs) bring about persistent inflammation and an abnormal infiltration of a desmoplastic stroma in cholangiocarcinoma (CCA) (143). CAFs also release cytokine IL-6 drive the process of EMT (144). Recently, Ferraresi et al. reported that IL-6 drove EMT through suppression of autophagy (145). In further researches, they found that RES could decrease the secretion (IL-6) of CAFs and make CCA cells revert N- cadherin to E-cadherin switched by induction of autophagy (LC3-II/LC3I) (26). These findings provide a new view about CAFs secretion may change the malignant phenotype of CCA cells through a functional food.
Non-Digestive Cancers
Breast Cancer
TGF-β1, which is found in various tissues and has function of angiogenesis, provides cancer cells with oxygen and nutrients and becomes an immunosuppressive agent to protect tumor cells from the host immune system. So it has been linked to cell growth, invasion and metastasis in a variety of tumors (146). Tang et al. reported that RES could inhibit breast cancer metastasis by activating silent information regulator 7 (SIRT7) deacetylase activity toward Smad4 degradation (27), antagonizing TGF-β1 signaling. They found that mesenchymal markers involving Fibronectin 1 and vimentin, TGF-β responsive gene angiopoietin-like Protein 4 (ANGPTL4) and C-X-C motif chemokine ligand 8 (CXCL8) were significantly down-regulated by RES (27). Moreover, Sun et al. reported that RES could reverse TGF-β1-induced EMT of breast cancer cells by regulating PI3K/AKT and Smad signaling (28). The drugs targeting TGF-β1 ligand or receptor for the therapies of cancer are under clinical trials. However, systemic anti-TGF-β treatments might bring severe adverse effects (78). Drug development specifically targeting the TGF-β downstream signaling pathway is necessary. The findings can inspire that RES selectively targets SIRT7 and PI3K in breast cancer metastasis, which might provide new strategy for the treatment.
It is known to all that epidermal growth factor (EGF) can induce the EMT process by repressing the expression of E-cadherin, the main epithelial marker and up-regulating mesothelial markers vimentin and N-cadherin (147). González-González L. et al. reported that EGF stimulated the migratory capacity of breast cancer cells, by regulating the functional expression of NaV1.5 channels. And RES can also affect Na+ channel activity to prevent the stimulatory actions of EGF (29). Vergara D et al. also found that RES could repress EGF-induced EMT by prevention of ERK activation (30). These findings support the idea that RES prevents EGF-induced EMT through different mechanisms and can exert a preventive role in breast cancer metastasis.
Amphiregulin is high- and low-affinity ligand for EGF receptor and can maintain epithelial characteristics as efficiently as EGF (148). Cho et al. demonstrated that RES downregulated amphiregulin expression caused by LPA as well as blocked the Y-box binding protein-1 (YB-1)/enhancer of zeste homolog 2 (EZH2) signaling pathway to inhibit the invasion of breast cancer cell. They found EZH2 was associated with amphiregulin expression and overexpression of YB-1 increased EZH2 expression (31). Furthermore, Hartman et al. delineated that EZH2 was participated in NF-κB activation in triple negative breast cancer cells (TNBCs) and was critical for anchorage-independent growth of TNBCs (149). These phenomena reveal that RES has the potential to hamper EMT stimulated by LPA through suppression of YB-1/EZH2 signaling axis in breast cancer cells, preventing the invasion and metastasis, as well as sensitizing the treatment of 5-FU (31).
Interestingly, more new mechanisms are found to reverse EMT by RES. Rad9 belongs to the DNA damage response protein family, and inhibition of Rad9 can cause the corresponding downregulation of E-cadherin, and the upregulation of N-cadherin and vimentin. A research has shown that Rad9 could directly bind the Slug promoter and repress its transcriptional activity to inhibit EMT (150). Chen et al. found that RES could induce DNA damages and elevate ROS levels, which in turn upregulate Rad9, and suppress Slug expression to inhibit the EMT process in breast cancer cells (32). The alternative splicing of specific pre-RNAs, involving Cd44, Enah and fibroblast growth factor receptor 2 (FGFR2), exert a causative role in the EMT process (151–153). RES promotes the epithelial-type alternative splicing of Cd44, Enah, and FGFR2 pre-mRNAs in breast cancer cells by upregulating KH-type splicing regulatory protein (KHSRP) and heterogeneous nuclear ribonucleoproteins A1 (hnRNPA1) expression (33).
Doxorubicin (DOX) is one of the most commonly used chemotherapy drugs for patients of breast cancer. However, drug resistance to DOX, partly due to the occurrence of EMT, can lead to recurrence and metastasis of cancer (154). Jin et al. reported that RES successfully alleviated cell invasion and increased synergistic sensitivity to DOX via inhibiting the EMT process and modulating the association between silent information regulator 1 (SIRT1) and β‐catenin. They found that DOX could increase expression of vimentin, N-cadherin, β‐catenin and RES notably antagonized these EMT-markers, which showed that RES overcame DOX resistance and offered hope for breast cancer patients to extend their survival time (34). Tamoxifen, as a selective estrogen receptor (ER) modifier, is also widely used in patients with breast cancer. However, tamoxifen resistance contributes to a poor prognosis for breast cancer. Shi et al. reported that an increased production of endogenous TGF-β1 and constitutive activation of Smad signaling contributed to the cancer cell phenotype switch, meanwhile decreased the sensitivity to tamoxifen (35). The good news was that RES inhibited TGF-β1 secretion and reduced phosphorylation of Smad, eventually affecting the EMT process and reversing tamoxifen resistance (35). Targeting AKT can provide an important approach for cancer therapy and AKT inhibitor is widely studied. However, the clinically used AKT inhibitor has the side effect of promoting EMT, which might be part of drug resistance. Li et al. reported that RES could promote the β-transducin repeat containing protein (β-TrCP)-mediated Twist1 degradation and reduce MK-2206 (AKT inhibitor)-induced EMT (36). In addition, Yar et al. found that resveratrol could enhance the sensitivity of FL118 in triple-negative breast cancer cell lines by inhibiting EMT (37). It was reported that resveratrol decreased mammary promoter hypermethylation of the tumor suppressor gene Ras association domain family 1α (RASSF-1α) in women at increased risk for breast cancer (155).
Lung Cancer
Previous reports have pointed out that mitochondrial dysfunction has been linked to cancer metastasis (156). During the process of TGF-β1 induced EMT, mitochondria will be damaged. Under the treatment of TGF-β1, ROS level increase, while mitochondrial membrane potential and ATP content significantly decrease. Furthermore, the authors found that mitochondrial dysfunction promoted the expression level of vimentin and α-SMA, as well as decreased the expression level of E-cadherin and CK18. On the contrary, RES can protect mitochondrial function shown by an elevation in mitochondrial membrane potential, expression level of mitochondrial complex, and ATP content, so as to inhibit the occurrence of EMT (38). Wang et al. found that RES increased the expression of E-cadherin inhibited by TGF-β1 and decreased expression of fibronectin and vimentin, meanwhile RES also reduced the level of TGF-β1-induced Snail1 and Slug, thereby suppressing lung cancer invasion and metastasis (39).
Forkhead box C2 (FOXC2), a critical transcriptional factor that affects EMT, induces tumor angiogenesis and metastasis (157). Similarly, lung cancer patients with high expression of FOXC2 also experience a poor prognosis. A study has found that RES inhibited miRNA-520h, activated protein phosphatase 2 A/C (PP2A/C), then through the AKT-NF-κB signaling axis, finally down-regulated FOXC2 to inhibit distant metastasis of lung cancer cells (40).
Sun et al. found that SIRT1 transcription was regulated by a SUMOylation-dependent pathway, leading to lung cancer metastasis. Activation of SIRT1 can inhibit the occurrence of EMT. However, hypoxia repressed the transcription of SIRT1 in a SUMOylation-dependent manner. RES, the agonist of SIRT1, can antagonize the transformation of SIRT1 caused by hypoxia, thus inhibiting EMT in lung cancer cells (41).
Genitourinary Malignancy
A study reported that RES suppressed Snail expression and prevented cisplatin-induced EMT through the MAPK/ERK pathway in ovarian cancer cells. Meanwhile, RES can induce cell death and enhance the cytotoxicity of cisplatin. These findings suggest that RES combined with cisplatin may be a more effective antitumor regimen (42). Previous study has shown that human telomerase reverse transcriptase (hTERT) upregulated in tumor cells and is related to cancer development and poor prognosis. Noradrenaline (NE) can induce the expression of hTERT, and overexpression of hTERT can promote the expression of slug, thus speeding up the process of EMT (158). And RES happens to block the process by which NE causes EMT. Authors found that RES inhibited Src phosphorylation and HIF-1α expression, downregulating hTERT expression induced by NE. At the same time, RES also can reduce Slug expression and inhibit EMT of ovarian cancer cells. Moreover, the authors demonstrated that RES could enhance the effect of β adrenergic receptor inhibitors on NE-induced invasion of ovarian cancer cells (43). These results provide preclinical evidence for the effectiveness of RES in the treatment of ovarian cancer. Furthermore, it was reported that resveratrol could reverse EMT of cervical cancer cell via inhibiting STAT3Tyr705 phosphorylation (44).
It has been reported that changes of Mg2+ homeostasis affected cell functions, such as proliferation, invasion, migration, and angiogenesis (159). The transient receptor potential melastatin-subfamily member 7 (TRPM7) plays, as an Mg2+ influx channel, exerts tumor-promoting effects in prostate cancer. With the addition of TGF-β, the expression of TRPM7 increases, and TGF-β/TRPM7 axis is proved as one of the mechanisms of EMT. Thus, RES was found to inhibit the expression of TRPM7 induced by TGF-β, thereby inhibiting the occurrence of EMT (45). Khusbu et al. studied an unconventional E3 ligase named TNF-receptor associated factor 6 (TRAF6) and found that RES could inhibit growth and proliferation of prostate cancer cells by regulating the expression of TRAF6, suppressing the EMT process through NF-κB/Slug axis (46). Moreover, Li et al. found that RES could prevent LPS-induced EMT through the Hedhehog signaling pathway in prostate cancer cells (47). It is well known that prostate cancer can be easily affected by dihydrotestosterone (DHT) via androgen receptor (AR). Jang et al. reported that RES could prevent the metastasis of prostate cancer and reverse its process of EMT through AR and CXCR4 pathway (48). Hepatocyte growth factor (HGF), secreted by the stroma, can bind its receptor c-Met which is located in the epithelium, promoting cell growth and scattering of various epithelial cells. RES was found to suppress migration and invasion of prostate cancer epithelial cells through inhibiting HGF secretion from prostate stromal cells and to prevent the process of EMT (49).
Cigarette smoke (CS) is a main cause of bladder cancer. Sun et al. used cigarette smoke extract (CSE) to induced EMT and CSE can also promote the phosphorylation of signal transducer and activator of transcription 3 (STAT3) and the expression of Twist1, leading to the occurrence of EMT and cell invasion and metastasis. RES can promote the expression of E-cadherin and ZO-1, and reduce the expression of N-cadherin and vimentin through STAT3/Twist signaling pathway. These findings provide new ideas for clinical prevention and intervention of bladder cancer metastasis (50).
Brain Cancer
Song et al. reported that RES can inhibit TGF-β1-induced EMT process, migration and invasion ability in glioblastoma cells. They found that RES could downregulate EMT-related markers by inhibiting the phosphorylation of Smad2 and Smad3 which are the downstream of TGF-β1, thereby preventing the progress of EMT. And they also found that RES could inhibit the expression of cancer stem cell markers Bmil and Sox2, inhibit the stem cell-like traits of glioblastoma cells, and affect the self-renewal capacity of the cells. These findings provide experimental evidence of RES for glioblastoma treatment (51). In addition to the TGF-β1/Smad signaling axis, RES can also affect the proliferation and motility of glioma stem cells through the Wnt signaling pathway. It can also reduce the level of nuclear β-catenin and induce the transcriptional up-regulation of MYC. Finally, RES down-regulated the ribose of nuclear Twist1 and Snail1, indicating its effects as a novel anti-EMT agent (52).
A previous study demonstrated that cyclin B1 (CCNB1) can function as the control of the G2/M phase in the cell cycle (160). In addition, they found that patients with invasive pituitary adenomas share higher CCNB1 expression than these with non-invasive pituitary adenomas. Li et al. found that the knockdown of CCNB1 decreased the expression of N-cadherin, but increased the expression of E-cadherin and p120-catenin. Further analysis indicated that RES inhibited the expression level of CCNB1, regulated the proliferation and apoptosis of pituitary tumor cells, and changed the expression level of various EMT markers (53).
Head and Neck Cancer
More and more evidence has manifested that head and neck cancer has a subpopulation of cells which possess stem-like properties and ability to trigger tumor metastasis as well as the resistance of treatment (161). Cancer researchers pay huge attention to stemness signature interacted with EMT. For example, Hu et al. reported that RES could down-regulate the expression of Oct4, Nanog, and Nestin identified as stemness genes signatures and downregulate EMT markers (Slug, ZEB1, N-cadherin and vimentin) (54). Because of the capability to eliminate stem-like and EMT properties, RES may be a valuable therapeutic candidate. Additionally, researchers also found RES prevented the expression of Smad2/3 and down-regulated EMT markers but induced the expression of E-cadherin in oral squamous cell carcinoma (OSCC) (55). Rab coupling protein (RCP) promotes OSCC metastasis via elevating the expression level of ZEB1 and thereby upregulating membranetype 1 matrix metalloproteinases (MT1-MMP) expression, and this observation can be reversed by RES, revealing the potential therapeutic effects of RES on OSCC (56). Shen et al. proved that RES impeded EMT through p53 activation in CSCs of nasopharyngeal carcinoma (57).Conclusively, RES may give beneficial information for the new therapies for head and neck cancer treatment.
Melanoma, Osteosarcoma and Multiple Myeloma
Chen et al. found that RES exerted the antitumor effects in the metastasis melanoma mouse model, including reducing the tumor size and prolonging the survival. RES prevents tumor metastasis caused by LPS and inhibits the expression of EMT markers via the downregulation of NF-κB activity (58). In another study, Menicacci et al. proved that RES prevented MRC5 fibroblast senescence-associated secretory phenotype (SASP)-related protumoral effects on melanoma cells and inhibited the ability of EMT (59). In osteosarcoma cells, Sun et al. found that RES promoted the degradation of HIF-1α protein, abrogating the influence of hypoxia on proliferation, invasion and EMT (60). In conclusion, RES represents a bright prospect in the therapeutic strategies of cancers. A phase 2 study for patients with relapsed and or refractory multiple myeloma (MM) showed that the combination between SRT501 (resveratrol) and bortezomib exerted better overall response rate than SRT501 monotherapy, and renal failure seemed specific to MM patients (162).
Resveratrol Analogs
Recently, some analogs of RES have been constructed with enhanced anti-tumor effectiveness, bioavailability, and pharmacokinetic characteristics (163). Here, we reviewed several RES analogs which were associated with EMT in different cancers. Daniele et al. developed trans-restricted analogues in which the C–C double bond of RES is displaced by diaryl substituted imidazole analogues and studied anti-tumor effects of these analogues on ovarian cancer cells. Authors found these analogues blocked the AKT and MAPK signaling, inhibiting IL-6 and EGF-induced EMT in ovarian cancer cells. These findings indicate the potential of analogues in the fight against tumor metastasis (61).
3,5,4′-Trimethoxystilbene (MR-3), a natural methoxylated analog of RES, changes the EMT-related phenotypes more effectively than RES in MCF-7 cells (62). Authors found that MR-3 could restore membrane-bound β-catenin as well as suppress the nuclear localization and function of β-catenin in breast cancer cells. Further, MR-3 significantly decreases the phosphorylation of GSK-3β and the phosphorylated status of AKT is blocked. Altogether, MR-3 reverses the occurrence of EMT through the degradation of PI3K/AKT/GSK-3β signaling and the synthesis of β-catenin proteasome. Simultaneously, this finding suggests that methoxylation may increase the EMT-inhibiting effect of RES.
Another RES analog, Triacetyl-resveratrol (TCRV), which provides a better pharmacokinetics/pharmacodynamics traits than parent RES, was studied in pancreatic cancer cells by Junsheng Fu and his team (63). Authors found that TCRV inhibited EMT by suppressing the expression of ZEB1 via the upregulation of miR-200 family members and by the suppression of the sonic hedgehog (Shh) pathway. These findings suggest TCRV might be a promising candidate for the prevention and treatment of pancreatic cancer.
Conclusions and Perspectives
More and more studies have demonstrated that the EMT process exerts a significant role on tumor progression and metastasis (7–9). The EMT program is associated with various pathways and crosstalk between these signaling by key molecules. Interestingly, EMT induction promotes the CSC characteristics and contributes to recurrence and drug resistance. TGF-β1 has widely been regarded as an effective inducer of the EMT process via both Smad-dependent and other signaling. RTK pathways also play essential roles in the process of EMT. Thus, therapies targeting these signaling pathways might provide promising approaches to inhibit invasion of cancer cells and to prevent metastasis. RES has attracted increasing attention in recent 20 years with its impact on preventing cancer and its low toxicity for normal cells in vivo. In addition, the safety of oral administration has also been proved by many preclinical and clinical studies. Because of its low water-solubility and bioavailability, some RES analogs, such as 3,5,4’-Trimethoxystilbene and Triacetyl resveratrol, are constructed. Several studies have confirmed that RES and its analogues inhibit cancer cell growth, invasion, migration as well as promote the apoptosis of tumor cells. RES can also reverse and prevent EMT, increasing cell-cell tight junctions and eventually inhibiting the metastasis of cancers. Recent data reveal that RES can show anti-tumor effects by suppression of EMT via both TGF-β1-dependent (e.g. breast cancer, lung cancer and glioblastoma multiforme) and -independent (e.g. gastric cancer, pancreatic cancer, ovarian cancer, melanoma and osteosarcoma) signaling pathways. Moreover, it has been reported that RES and its analogs can abolish chemoresistance through EMT inhibition and improve the antiproliferative effects of conventional treatments (e.g. doxorubicin, 5-FU, tamoxifen and cisplatin). Therefore, RES and analogs have the potential ability to be applied as novel complementary and alternative agents to hamper cancer invasion and metastasis, and it may partly be attributed to their effectiveness to prevent EMT.
Author Contributions
Content design, KG, YF, XZ, LS, HW, MS and SR. Literature collection, KG, YF and XZ. Arrangement, KG. Figures and tables, KG and YF. Writing-original draft, KG, YF and XZ. Writing—review and editing, KG, YF, XZ, LS, HW, MS and SR. Writing—proofreading, LS. Funding, SR. All authors contributed to the article and approved the submitted version.
Funding
National Natural Science Foundation of China (81573902); Zhejiang provincial TCM scientific research fund project (2019ZQ015); China Postdoctoral Science Foundation (2017M612040; 2018T110610); Top ten thousand talents program of Zhejiang Province (SR, no.2019-97, http://www.zjzzgz.gov.cn/); Zhejiang Provincial Project for the key discipline of Traditional Chinese Medicine (YG, no,2017-XK-A09, http://www.zjwjw.gov.cn/); Science and technology innovation activity plan and new seedling of college students in Zhejiang Province (2019R410001).
Conflict of Interest
The authors declare that the research was conducted in the absence of any commercial or financial relationships that could be construed as a potential conflict of interest.
References
1. Babaei G, Aziz SG, Jaghi NZZ. EMT, cancer stem cells and autophagy; The three main axes of metastasis. Biomed Pharmacother Biomed Pharmacother (2021) 133:110909. doi: 10.1016/j.biopha.2020.110909
2. Bakir B, Chiarella AM, Pitarresi JR, Rustgi AK. EMT, MET, Plasticity, and Tumor Metastasis. Trends Cell Biol (2020) 30(10):764–76. doi: 10.1016/j.tcb.2020.07.003
3. Kyuno D, Takasawa A, Kikuchi S, Takemasa I, Osanai M, Kojima T. Role of tight junctions in the epithelial-to-mesenchymal transition of cancer cells. Biochim Biophys Acta Biomembranes (2021) 1863(3):183503. doi: 10.1016/j.bbamem.2020.183503
4. Paolillo M, Schinelli S. Extracellular Matrix Alterations in Metastatic Processes. Int J Mol Sci (2019) 20(19):4947. doi: 10.3390/ijms20194947
5. Lambert AW, Weinberg RA. Linking EMT programmes to normal and neoplastic epithelial stem cells. Nat Rev Cancer (2021). doi: 10.1038/s41568-021-00332-6
6. Lin WH, Chang YW, Hong MX, Hsu TC, Lee KC, Lin C, et al. STAT3 phosphorylation at Ser727 and Tyr705 differentially regulates the EMT-MET switch and cancer metastasis. Oncogene (2021) 40(4):791–805. doi: 10.1038/s41388-020-01566-8
7. Liu M, Zhang Y, Yang J, Zhan H, Zhou Z, Jiang Y, et al. Zinc Dependent Regulation of ZEB1 and YAP1 Co-activation Promotes EMT Plasticity and Metastasis in Pancreatic Cancer. Gastroenterology (2021). doi: 10.1053/j.gastro.2020.12.077
8. Li L, Wang T, Li S, Chen Z, Wu J, Cao W, et al. TDO2 Promotes the EMT of Hepatocellular Carcinoma Through Kyn-AhR Pathway. Front Oncol (2020) 10:562823. doi: 10.3389/fonc.2020.562823
9. Xu F, Shangguan X, Pan J, Yue Z, Shen K, Ji Y, et al. HOXD13 Suppresses Prostate Cancer Metastasis and BMP4-induced EMT by Inhibiting SMAD1. Int J Cancer (2021). doi: 10.1002/ijc.33494
10. Xu J, Liu D, Niu H, Zhu G, Xu Y, Ye D, et al. Resveratrol reverses Doxorubicin resistance by inhibiting epithelial-mesenchymal transition (EMT) through modulating PTEN/Akt signaling pathway in gastric cancer. J Exp Clin Cancer Res CR (2017) 36(1):19. doi: 10.1186/s13046-016-0487-8
11. Yang Z, Xie Q, Chen Z, Ni H, Xia L, Zhao Q, et al. Resveratrol suppresses the invasion and migration of human gastric cancer cells via inhibition of MALAT1-mediated epithelial-to-mesenchymal transition. Exp Ther Med (2019) 17(3):1569–78. doi: 10.3892/etm.2018.7142
12. Gao Q, Yuan Y, Gan HZ, Peng Q. Resveratrol inhibits the hedgehog signaling pathway and epithelial-mesenchymal transition and suppresses gastric cancer invasion and metastasis. Oncol Lett (2015) 9(5):2381–7. doi: 10.3892/ol.2015.2988
13. Xu QH, Xiao Y, Li XQ, Fan L, Zhou CC, Cheng L, et al. Resveratrol Counteracts Hypoxia-Induced Gastric Cancer Invasion and EMT through Hedgehog Pathway Suppression. Anti-cancer Agents Med Chem (2020) 20(9):1105–14. doi: 10.2174/1871520620666200402080034
14. Yin L, Zhang R, Hu Y, Li W, Wang M, Liang Z, et al. Gastric-cancer-derived mesenchymal stem cells: a promising target for resveratrol in the suppression of gastric cancer metastasis. Hum Cell (2020) 33(3):652–62. doi: 10.1007/s13577-020-00339-5
15. Buhrmann C, Shayan P, Kraehe P, Popper B, Goel A, Shakibaei M. Resveratrol induces chemosensitization to 5-fluorouracil through up-regulation of intercellular junctions, Epithelial-to-mesenchymal transition and apoptosis in colorectal cancer. Biochem Pharmacol (2015) 98(1):51–68. doi: 10.1016/j.bcp.2015.08.105
16. Ji Q, Liu X, Han Z, Zhou L, Sui H, Yan L, et al. Resveratrol suppresses epithelial-to-mesenchymal transition in colorectal cancer through TGF-beta1/Smads signaling pathway mediated Snail/E-cadherin expression. BMC Cancer (2015) 15:97. doi: 10.1186/s12885-015-1119-y
17. Buhrmann C, Yazdi M, Popper B, Kunnumakkara AB, Aggarwal BB, Shakibaei M. Induction of the Epithelial-to-Mesenchymal Transition of Human Colorectal Cancer by Human TNF-β (Lymphotoxin) and its Reversal by Resveratrol. Nutrients (2019) 11(3):704. doi: 10.3390/nu11030704
18. Buhrmann C, Yazdi M, Popper B, Shayan P, Goel A, Aggarwal BB, et al. Resveratrol Chemosensitizes TNF-beta-Induced Survival of 5-FU-Treated Colorectal Cancer Cells. Nutrients (2018) 10(7):888. doi: 10.3390/nu10070888
19. Yuan L, Zhou M, Huang D, Wasan HS, Zhang K, Sun L, et al. Resveratrol inhibits the invasion and metastasis of colon cancer through reversal of epithelial mesenchymal transition via the AKT/GSK3beta/Snail signaling pathway. Mol Med Rep (2019) 20(3):2783–95. doi: 10.3892/mmr.2019.10528
20. Karimi Dermani F, Saidijam M, Amini R, Mahdavinezhad A, Heydari K, Najafi R. Resveratrol Inhibits Proliferation, Invasion, and Epithelial-Mesenchymal Transition by Increasing miR-200c Expression in HCT-116 Colorectal Cancer Cells. J Cell Biochem (2017) 118(6):1547–55. doi: 10.1002/jcb.25816
21. Shankar S, Nall D, Tang SN, Meeker D, Passarini J, Sharma J, et al. Resveratrol inhibits pancreatic cancer stem cell characteristics in human and KrasG12D transgenic mice by inhibiting pluripotency maintaining factors and epithelial-mesenchymal transition. PloS One (2011) 6(1):e16530. doi: 10.1371/journal.pone.0016530
22. Li W, Cao L, Chen X, Lei J, Ma Q. Resveratrol inhibits hypoxia-driven ROS-induced invasive and migratory ability of pancreatic cancer cells via suppression of the Hedgehog signaling pathway. Oncol Rep (2016) 35(3):1718–26. doi: 10.3892/or.2015.4504
23. Li W, Ma J, Ma Q, Li B, Han L, Liu J, et al. Resveratrol inhibits the epithelial-mesenchymal transition of pancreatic cancer cells via suppression of the PI-3K/Akt/NF-κB pathway. Curr Med Chem (2013) 20(33):4185–94. doi: 10.2174/09298673113209990251
24. Xiao Y, Qin T, Sun L, Qian W, Li J, Duan W, et al. Resveratrol Ameliorates the Malignant Progression of Pancreatic Cancer by Inhibiting Hypoxia-induced Pancreatic Stellate Cell Activation. Cell Transplant (2020) 29:963689720929987. doi: 10.1177/0963689720929987
25. Qin T, Cheng L, Xiao Y, Qian W, Li J, Wu Z, et al. NAF-1 Inhibition by Resveratrol Suppresses Cancer Stem Cell-Like Properties and the Invasion of Pancreatic Cancer. Front Oncol (2020) 10:1038. doi: 10.3389/fonc.2020.01038
26. Thongchot S, Ferraresi A, Vidoni C, Loilome W, Yongvanit P, Namwat N, et al. Resveratrol interrupts the pro-invasive communication between cancer associated fibroblasts and cholangiocarcinoma cells. Cancer Lett (2018) 430:160–71. doi: 10.1016/j.canlet.2018.05.031
27. Tang X, Shi L, Xie N, Liu Z, Qian M, Meng F, et al. SIRT7 antagonizes TGF-β signaling and inhibits breast cancer metastasis. Nat Commun (2017) 8(1):318. doi: 10.1038/s41467-017-00396-9
28. Sun Y, Zhou QM, Lu YY, Zhang H, Chen QL, Zhao M, et al. Resveratrol Inhibits the Migration and Metastasis of MDA-MB-231 Human Breast Cancer by Reversing TGF-β1-Induced Epithelial-Mesenchymal Transition. Mol (Basel Switzerland) (2019) 24(6):1131. doi: 10.3390/molecules24061131
29. Gonzalez-Gonzalez L, Gonzalez-Ramirez R, Flores A, Avelino-Cruz JE, Felix R, Monjaraz E. Epidermal Growth Factor Potentiates Migration of MDA-MB 231 Breast Cancer Cells by Increasing NaV1.5 Channel Expression. Oncology (2019) 97(6):373–82. doi: 10.1159/000501802
30. Vergara D, Valente CM, Tinelli A, Siciliano C, Lorusso V, Acierno R, et al. Resveratrol inhibits the epidermal growth factor-induced epithelial mesenchymal transition in MCF-7 cells. Cancer Lett (2011) 310(1):1–8. doi: 10.1016/j.canlet.2011.04.009
31. Cho KH, Jeong BY, Park CG, Lee HY. The YB-1/EZH2/amphiregulin signaling axis mediates LPA-induced breast cancer cell invasion. Arch Pharmacal Res (2019) 42(6):519–30. doi: 10.1007/s12272-019-01149-6
32. Chen KY, Chen CC, Chang YC, Chang MC. Resveratrol induced premature senescence and inhibited epithelial-mesenchymal transition of cancer cells via induction of tumor suppressor Rad9. PloS One (2019) 14(7):e0219317. doi: 10.1371/journal.pone.0219317
33. Moshiri A, Puppo M, Rossi M, Gherzi R, Briata P. Resveratrol limits epithelial to mesenchymal transition through modulation of KHSRP/hnRNPA1-dependent alternative splicing in mammary gland cells. Biochim Biophys Acta Gene Regul Mech (2017) 1860(3):291–8. doi: 10.1016/j.bbagrm.2017.01.001
34. Jin X, Wei Y, Liu Y, Lu X, Ding F, Wang J, et al. Resveratrol promotes sensitization to Doxorubicin by inhibiting epithelial-mesenchymal transition and modulating SIRT1/beta-catenin signaling pathway in breast cancer. Cancer Med (2019) 8(3):1246–57. doi: 10.1002/cam4.1993
35. Shi XP, Miao S, Wu Y, Zhang W, Zhang XF, Ma HZ, et al. Resveratrol sensitizes tamoxifen in antiestrogen-resistant breast cancer cells with epithelial-mesenchymal transition features. Int J Mol Sci (2013) 14(8):15655–68. doi: 10.3390/ijms140815655
36. Li CW, Xia W, Lim SO, Hsu JL, Huo L, Wu Y, et al. AKT1 Inhibits Epithelial-to-Mesenchymal Transition in Breast Cancer through Phosphorylation-Dependent Twist1 Degradation. Cancer Res (2016) 76(6):1451–62. doi: 10.1158/0008-5472.CAN-15-1941
37. Yar Saglam AS, Kayhan H, Alp E, Onen HI. Resveratrol enhances the sensitivity of FL118 in triple-negative breast cancer cell lines via suppressing epithelial to mesenchymal transition. Mol Biol Rep (2021) 48(1):475–89. doi: 10.1007/s11033-020-06078-y
38. Zhang J, Zhang W, Zhang T, Zhou Q, Liu J, Liu Y, et al. TGF-β1 induces epithelial-to-mesenchymal transition via inhibiting mitochondrial functions in A549 cells. Free Radical Res (2018) 52(11-12):1432–44. doi: 10.1080/10715762.2018.1500020
39. Wang H, Zhang H, Tang L, Chen H, Wu C, Zhao M, et al. Resveratrol inhibits TGF-β1-induced epithelial-to-mesenchymal transition and suppresses lung cancer invasion and metastasis. Toxicology (2013) 303:139–46. doi: 10.1016/j.tox.2012.09.017
40. Yu YH, Chen HA, Chen PS, Cheng YJ, Hsu WH, Chang YW, et al. MiR-520h-mediated FOXC2 regulation is critical for inhibition of lung cancer progression by resveratrol. Oncogene (2013) 32(4):431–43. doi: 10.1038/onc.2012.74
41. Sun L, Li H, Chen J, Dehennaut V, Zhao Y, Yang Y, et al. A SUMOylation-dependent pathway regulates SIRT1 transcription and lung cancer metastasis. J Natl Cancer Institute (2013) 105(12):887–98. doi: 10.1093/jnci/djt118
42. Baribeau S, Chaudhry P, Parent S, Asselin E. Resveratrol inhibits cisplatin-induced epithelial-to-mesenchymal transition in ovarian cancer cell lines. PloS One (2014) 9(1):e86987. doi: 10.1371/journal.pone.0086987
43. Kim SH, Cho KH, Kim YN, Jeong BY, Park CG, Hur GM, et al. Resveratrol attenuates norepinephrine-induced ovarian cancer invasiveness through downregulating hTERT expression. Arch Pharmacal Res (2016) 39(2):240–8. doi: 10.1007/s12272-015-0666-8
44. Sun X, Xu Q, Zeng L, Xie L, Zhao Q, Xu H, et al. Resveratrol suppresses the growth and metastatic potential of cervical cancer by inhibiting STAT3(Tyr705) phosphorylation. Cancer Med (2020) 9(22):8685–700. doi: 10.1002/cam4.3510
45. Sun Y, Schaar A, Sukumaran P, Dhasarathy A, Singh BB. TGFβ-induced epithelial-to-mesenchymal transition in prostate cancer cells is mediated via TRPM7 expression. Mol Carcinogen (2018) 57(6):752–61. doi: 10.1002/mc.22797
46. Khusbu FY, Zhou X, Roy M, Chen FZ, Cao Q, Chen HC. Resveratrol induces depletion of TRAF6 and suppresses prostate cancer cell proliferation and migration. Int J Biochem Cell Biol (2020) 118:105644. doi: 10.1016/j.biocel.2019.105644
47. Li J, Chong T, Wang Z, Chen H, Li H, Cao J, et al. A novel anticancer effect of resveratrol: reversal of epithelialmesenchymal transition in prostate cancer cells. Mol Med Rep (2014) 10(4):1717–24. doi: 10.3892/mmr.2014.2417
48. Jang YG, Go RE, Hwang KA, Choi KC. Resveratrol inhibits DHT-induced progression of prostate cancer cell line through interfering with the AR and CXCR4 pathway. J Steroid Biochem Mol Biol (2019) 192:105406. doi: 10.1016/j.jsbmb.2019.105406
49. Hsieh TC, Wu JM. Resveratrol Suppresses Prostate Cancer Epithelial Cell Scatter/Invasion by Targeting Inhibition of Hepatocyte Growth Factor (HGF) Secretion by Prostate Stromal Cells and Upregulation of E-cadherin by Prostate Cancer Epithelial Cells. Int J Mol Sci (2020) 21(5):1760. doi: 10.3390/ijms21051760
50. Sun H, Zhang Z, Zhang T, Geng H, Xie D, Wang Y, et al. Resveratrol Reverses Cigarette Smoke-Induced Urocystic Epithelial-Mesenchymal Transition via Suppression of STAT3 Phosphorylation in SV-HUC-1-Immortalized Human Urothelial Cells. Onco Targets Ther (2019) 12:10227–37. doi: 10.2147/OTT.S226580
51. Song Y, Chen Y, Li Y, Lyu X, Cui J, Cheng Y, et al. Resveratrol Suppresses Epithelial-Mesenchymal Transition in GBM by Regulating Smad-Dependent Signaling. BioMed Res Int (2019) 2019:1321973. doi: 10.1155/2019/1321973
52. Cilibrasi C, Riva G, Romano G, Cadamuro M, Bazzoni R, Butta V, et al. Lavitrano M et al: Resveratrol Impairs Glioma Stem Cells Proliferation and Motility by Modulating the Wnt Signaling Pathway. PloS One (2017) 12(1):e0169854. doi: 10.1371/journal.pone.0169854
53. Li B, Zhu HB, Song GD, Cheng JH, Li CZ, Zhang YZ, et al. Regulating the CCNB1 gene can affect cell proliferation and apoptosis in pituitary adenomas and activate epithelial-to-mesenchymal transition. Oncol Lett (2019) 18(5):4651–8. doi: 10.3892/ol.2019.10847
54. Hu FW, Tsai LL, Yu CH, Chen PN, Chou MY, Yu CC. Impairment of tumor-initiating stem-like property and reversal of epithelial-mesenchymal transdifferentiation in head and neck cancer by resveratrol treatment. Mol Nutr Food Res (2012) 56(8):1247–58. doi: 10.1002/mnfr.201200150
55. Kim SE, Shin SH, Lee JY, Kim CH, Chung IK, Kang HM, et al. Resveratrol Induces Mitochondrial Apoptosis and Inhibits Epithelial-Mesenchymal Transition in Oral Squamous Cell Carcinoma Cells. Nutr Cancer (2018) 70(1):125–35. doi: 10.1080/01635581.2018.1397708
56. Kim JY, Cho KH, Jeong BY, Park CG, Lee HY. Zeb1 for RCP-induced oral cancer cell invasion and its suppression by resveratrol. Exp Mol Med (2020) 52(7):1152–63. doi: 10.1038/s12276-020-0474-1
57. Shen YA, Lin CH, Chi WH, Wang CY, Hsieh YT, Wei YH, et al. Resveratrol Impedes the Stemness, Epithelial-Mesenchymal Transition, and Metabolic Reprogramming of Cancer Stem Cells in Nasopharyngeal Carcinoma through p53 Activation. Evidence-Based Complementary Altern Med eCAM (2013) 2013:590393. doi: 10.1155/2013/590393
58. Chen MC, Chang WW, Kuan YD, Lin ST, Hsu HC, Lee CH. Resveratrol inhibits LPS-induced epithelial-mesenchymal transition in mouse melanoma model. Innate Immun (2012) 18(5):685–93. doi: 10.1177/1753425912436589
59. Menicacci B, Laurenzana A, Chilla A, Margheri F, Peppicelli S, Tanganelli E, et al. Chronic Resveratrol Treatment Inhibits MRC5 Fibroblast SASP-Related Protumoral Effects on Melanoma Cells. J Gerontol Ser A Biol Sci Med Sci (2017) 72(9):1187–95. doi: 10.1093/gerona/glw336
60. Sun Y, Wang H, Liu M, Lin F, Hua J. Resveratrol abrogates the effects of hypoxia on cell proliferation, invasion and EMT in osteosarcoma cells through downregulation of the HIF-1alpha protein. Mol Med Rep (2015) 11(3):1975–81. doi: 10.3892/mmr.2014.2913
61. Vergara D, De Domenico S, Tinelli A, Stanca E, Del Mercato LL, Giudetti AM, et al. Manzini C et al: Anticancer effects of novel resveratrol analogues on human ovarian cancer cells. Mol Biosyst (2017) 13(6):1131–41. doi: 10.1039/C7MB00128B
62. Tsai JH, Hsu LS, Lin CL, Hong HM, Pan MH, Way TD, et al. 3,5,4’-Trimethoxystilbene, a natural methoxylated analog of resveratrol, inhibits breast cancer cell invasiveness by downregulation of PI3K/Akt and Wnt/beta-catenin signaling cascades and reversal of epithelial-mesenchymal transition. Toxicol Appl Pharmacol (2013) 272(3):746–56. doi: 10.1016/j.taap.2013.07.019
63. Fu J, Shrivastava A, Shrivastava SK, Srivastava RK, Shankar S. Triacetyl resveratrol upregulates miRNA−200 and suppresses the Shh pathway in pancreatic cancer: A potential therapeutic agent. Int J Oncol (2019) 54(4):1306–16. doi: 10.3892/ijo.2019.4700
64. Pastushenko I, Mauri F, Song Y, de Cock F, Meeusen B, Swedlund B, et al. Fat1 deletion promotes hybrid EMT state, tumour stemness and metastasis. Nature (2021) 589(7842):448–55. doi: 10.1038/s41586-020-03046-1
65. Pei D, Shu X, Gassama-Diagne A, Thiery JP. Mesenchymal-epithelial transition in development and reprogramming. Nat Cell Biol (2019) 21(1):44–53. doi: 10.1038/s41556-018-0195-z
66. Kudo-Saito C, Ozaki Y, Imazeki H, Hayashi H, Masuda J, Ozawa H, et al. Targeting Oncoimmune Drivers of Cancer Metastasis. Cancers (2021) 13(3):554. doi: 10.3390/cancers13030554
67. Cumin C, Huang YL, Everest-Dass A, Jacob F. Deciphering the Importance of Glycosphingolipids on Cellular and Molecular Mechanisms Associated with Epithelial-to-Mesenchymal Transition in Cancer. Biomolecules (2021) 11(1):62. doi: 10.3390/biom11010062
68. Coban B, Bergonzini C, Zweemer AJM, Danen EHJ. Metastasis: crosstalk between tissue mechanics and tumour cell plasticity. Br J Cancer (2021) 124(1):49–57. doi: 10.1038/s41416-020-01150-7
69. Novikov NM, Zolotaryova SY, Gautreau AM, Denisov EV. Mutational drivers of cancer cell migration and invasion. Br J Cancer (2021) 124(1):102–14. doi: 10.1038/s41416-020-01149-0
70. Kaszak I, Witkowska-Piłaszewicz O, Niewiadomska Z, Dworecka-Kaszak B, Ngosa Toka F, Jurka P. Role of Cadherins in Cancer-A Review. Int J Mol Sci (2020) 21(20):7624. doi: 10.3390/ijms21207624
71. Lin YT, Wu KJ. Epigenetic regulation of epithelial-mesenchymal transition: focusing on hypoxia and TGF-β signaling. J Biomed Sci (2020) 27(1):39. doi: 10.1186/s12929-020-00632-3
72. Mirzaei S, Gholami MH, Mahabady MK, Nabavi N, Zabolian A, Banihashemi SM, et al. : Pre-clinical investigation of STAT3 pathway in bladder cancer: Paving the way for clinical translation. Biomed Pharmacother Biomed Pharmacother (2021) 133:111077. doi: 10.1016/j.biopha.2020.111077
73. Jin W. Role of JAK/STAT3 Signaling in the Regulation of Metastasis, the Transition of Cancer Stem Cells, and Chemoresistance of Cancer by Epithelial-Mesenchymal Transition. Cells (2020) 9(1):217. doi: 10.3390/cells9010217
74. Stemmler MP, Eccles RL, Brabletz S, Brabletz T. Non-redundant functions of EMT transcription factors. Nat Cell Biol (2019) 21(1):102–12. doi: 10.1038/s41556-018-0196-y
75. Duan H, Liu Y, Gao Z, Huang W. Recent advances in drug delivery systems for targeting cancer stem cells. Acta Pharm Sin B (2021) 11(1):55–70. doi: 10.1016/j.apsb.2020.09.016
76. Richard V, Kumar TRS, Pillai RM. Transitional dynamics of cancer stem cells in invasion and metastasis. Trans Oncol (2021) 14(1):100909. doi: 10.1016/j.tranon.2020.100909
77. Gooding AJ, Schiemann WP. Epithelial-Mesenchymal Transition Programs and Cancer Stem Cell Phenotypes: Mediators of Breast Cancer Therapy Resistance. Mol Cancer Res MCR (2020) 18(9):1257–70. doi: 10.1158/1541-7786.MCR-20-0067
78. Hao Y, Baker D, Ten Dijke P. TGF-β-Mediated Epithelial-Mesenchymal Transition and Cancer Metastasis. Int J Mol Sci (2019) 20(11):2767. doi: 10.3390/ijms20112767
79. Aragón E, Wang Q, Zou Y, Morgani SM, Ruiz L, Kaczmarska Z, et al. Structural basis for distinct roles of SMAD2 and SMAD3 in FOXH1 pioneer-directed TGF-β signaling. Genes Dev (2019) 33(21-22):1506–24. doi: 10.1101/gad.330837.119
80. Karimi Roshan M, Soltani A, Soleimani A, Rezaie Kahkhaie K, Afshari AR, Soukhtanloo M. Role of AKT and mTOR signaling pathways in the induction of epithelial-mesenchymal transition (EMT) process. Biochimie (2019) 165:229–34. doi: 10.1016/j.biochi.2019.08.003
81. Yang M, Jin M, Li K, Liu H, Yang X, Zhang X, et al. TRAF6 Promotes Gastric Cancer Cell Self-Renewal, Proliferation, and Migration. Stem Cells Int (2020) 2020:3296192. doi: 10.1155/2020/3296192
82. Shome R, Ghosh SS. Tweaking EMT and MDR dynamics to constrain triple-negative breast cancer invasiveness by EGFR and Wnt/β-catenin signaling regulation. Cell Oncol (Dordrecht) (2021) 44(2):405–22. doi: 10.1007/s13402-020-00576-8
83. Pezzuto JM. Resveratrol: Twenty Years of Growth, Development and Controversy. Biomol Ther (2019) 27(1):1–14. doi: 10.4062/biomolther.2018.176
84. Torregrosa-Muñumer R, Vara E, Fernández-Tresguerres J, Gredilla R. Resveratrol supplementation at old age reverts changes associated with aging in inflammatory, oxidative and apoptotic markers in rat heart. Eur J Nutr (2021). doi: 10.1007/s00394-020-02457-0
85. Nunes S, Danesi F, Del Rio D, Silva P. Resveratrol and inflammatory bowel disease: the evidence so far. Nutr Res Rev (2018) 31(1):85–97. doi: 10.1017/S095442241700021X
86. Rahman MH, Akter R, Bhattacharya T, Abdel-Daim MM, Alkahtani S, Arafah MW, et al. Resveratrol and Neuroprotection: Impact and Its Therapeutic Potential in Alzheimer’s Disease. Front Pharmacol (2020) 11:619024. doi: 10.3389/fphar.2020.619024
87. Kou X, Chen N. Resveratrol as a Natural Autophagy Regulator for Prevention and Treatment of Alzheimer’s Disease. Nutrients (2017) 9(9):927. doi: 10.3390/nu9090927
88. Shi Y, Zhou J, Jiang B, Miao M. Resveratrol and inflammatory bowel disease. Ann New Y Acad Sci (2017) 1403(1):38–47. doi: 10.1111/nyas.13426
89. Breuss JM, Atanasov AG, Uhrin P. Resveratrol and Its Effects on the Vascular System. Int J Mol Sci (2019) 20(7). doi: 10.3390/ijms20071523
90. Rauf A, Imran M, Butt MS, Nadeem M, Peters DG, Mubarak MS. Resveratrol as an anti-cancer agent: A review. Crit Rev Food Sci Nutr (2018) 58(9):1428–47. doi: 10.1080/10408398.2016.1263597
91. Chen CY, Kao CL, Liu CM. The Cancer Prevention, Anti-Inflammatory and Anti-Oxidation of Bioactive Phytochemicals Targeting the TLR4 Signaling Pathway. Int J Mol Sci (2018) 19(9):2729. doi: 10.3390/ijms19092729
92. Jiang Z, Chen K, Cheng L, Yan B, Qian W, Cao J, et al. Resveratrol and cancer treatment: updates. Ann New Y Acad Sci (2017) 1403(1):59–69. doi: 10.1111/nyas.13466
93. Cao L, Chen X, Xiao X, Ma Q, Li W. Resveratrol inhibits hyperglycemia-driven ROS-induced invasion and migration of pancreatic cancer cells via suppression of the ERK and p38 MAPK signaling pathways. Int J Oncol (2016) 49(2):735–43. doi: 10.3892/ijo.2016.3559
94. Rasheduzzaman M, Jeong JK, Park SY. Resveratrol sensitizes lung cancer cell to TRAIL by p53 independent and suppression of Akt/NF-κB signaling. Life Sci (2018) 208:208–20. doi: 10.1016/j.lfs.2018.07.035
95. Palomera-Avalos V, Griñán-Ferré C, Puigoriol-Ilamola D, Camins A, Sanfeliu C, Canudas AM, et al. Resveratrol Protects SAMP8 Brain Under Metabolic Stress: Focus on Mitochondrial Function and Wnt Pathway. Mol Neurobiol (2017) 54(3):1661–76. doi: 10.1007/s12035-016-9770-0
96. Reutovich MY, Krasko OV, Sukonko OG. Hyperthermic intraperitoneal chemotherapy in serosa-invasive gastric cancer patients. Eur J Surg Oncol J Eur Soc Surg Oncol Br Assoc Surg Oncol (2019) 45(12):2405–11. doi: 10.1016/j.ejso.2019.07.030
97. Touchefeu Y, Guimbaud R, Louvet C, Dahan L, Samalin E, Barbier E, et al. Prognostic factors in patients treated with second-line chemotherapy for advanced gastric cancer: results from the randomized prospective phase III FFCD-0307 trial. Gastric Cancer Off J Int Gastric Cancer Assoc Japan Gastric Cancer Assoc (2019) 22(3):577–86. doi: 10.1007/s10120-018-0885-z
98. Dudas J, Ladanyi A, Ingruber J, Steinbichler TB, Riechelmann H. Epithelial to Mesenchymal Transition: A Mechanism that Fuels Cancer Radio/Chemoresistance. Cells (2020) 9(2):428. doi: 10.3390/cells9020428
99. Guo Q, Jing FJ, Xu W, Li X, Li X, Sun JL, et al. Ubenimex induces autophagy inhibition and EMT suppression to overcome cisplatin resistance in GC cells by perturbing the CD13/EMP3/PI3K/AKT/NF-κB axis. Aging (2019) 12(1):80–105. doi: 10.18632/aging.102598
100. Li Z, Lü M, Zhou Y, Xu L, Jiang Y, Liu Y, et al. Role of Long Non-Coding RNAs in the Chemoresistance of Gastric Cancer: A Systematic Review. OncoTarg Ther (2021) 14:503–18. doi: 10.2147/OTT.S294378
101. Groves I, Placzek M, Fletcher AG. Of mitogens and morphogens: modelling Sonic Hedgehog mechanisms in vertebrate development. Philos Trans R Soc London Ser B Biol Sci (2020) 375(1809):20190660. doi: 10.1098/rstb.2019.0660
102. Guimaraes VSN, Vidal MTA, de Faro Valverde L, de Oliveira MG, de Oliveira Siquara da Rocha L, Coelho PLC, et al. Hedgehog pathway activation in oral squamous cell carcinoma: cancer-associated fibroblasts exhibit nuclear GLI-1 localization. J Mol Histol (2020) 51(6):675–84. doi: 10.1007/s10735-020-09913-5
103. Dantas RCM, Guimarães VSN, de Souza RO, Valverde LF, Vidal MTA, Nogueira RLR, et al. Immunodetection of Epithelial-Mesenchymal Transition and Tumor Proliferation Markers in GLi-1-positive Oral Squamous Cell Carcinoma. Appl Immunohistochem Mol Morphol AIMM (2020). doi: 10.1097/PAI.0000000000000866
104. Wang Z, Li X, Chen H, Han L, Ji X, Wang Q, et al. Resveratrol alleviates bleomycin-induced pulmonary fibrosis via suppressing HIF-1α and NF-κB expression. Aging (2021) 13(3):4605–16. doi: 10.18632/aging.202420
105. Tan YY, Chen LX, Fang L, Zhang Q. Cardioprotective effects of polydatin against myocardial injury in diabetic rats via inhibition of NADPH oxidase and NF-κB activities. BMC Complementary Med Ther (2020) 20(1):378. doi: 10.1186/s12906-020-03177-y
106. Buhrmann C, Shayan P, Brockmueller A, Shakibaei M. Resveratrol Suppresses Cross-Talk between Colorectal Cancer Cells and Stromal Cells in Multicellular Tumor Microenvironment: A Bridge between In Vitro and In Vivo Tumor Microenvironment Study. Mol (Basel Switzerland) (2020) 25(18):4292. doi: 10.3390/molecules25184292
107. He Y, Lu X, Chen T, Yang Y, Zheng J, Chen C, et al. Resveratrol protects against myocardial ischemic injury via the inhibition of NF−κB−dependent inflammation and the enhancement of antioxidant defenses. Int J Mol Med (2021) 47(3):1. doi: 10.3892/ijmm.2021.4862
108. Huang X, Wang Y, Zhang P, Zou H. A HGF−derived peptide suppresses EMT in human lens epithelial cells via the TGF−β/Smad and Akt/mTOR signaling pathways. Mol Med Rep (2020) 22(1):551–8. doi: 10.3892/mmr.2020.11097
109. Wu JR, You RI, Hu CT, Cheng CC, Rudy R, Wu WS. Hydrogen peroxide inducible clone-5 sustains NADPH oxidase-dependent reactive oxygen species-c-jun N-terminal kinase signaling in hepatocellular carcinoma. Oncogenesis (2019) 8(8):40. doi: 10.1038/s41389-019-0149-8
110. Almalé L, García-Álvaro M, Martínez-Palacián A, García-Bravo M, Lazcanoiturburu N, Addante A, et al. c-Met Signaling Is Essential for Mouse Adult Liver Progenitor Cells Expansion After Transforming Growth Factor-β-Induced Epithelial-Mesenchymal Transition and Regulates Cell Phenotypic Switch. Stem Cells (Dayton Ohio) (2019) 37(8):1108–18. doi: 10.1002/stem.3038
111. Su J, Morgani SM, David CJ, Wang Q, Er EE, Huang YH, et al. TGF-β orchestrates fibrogenic and developmental EMTs via the RAS effector RREB1. Nature (2020) 577(7791):566–71. doi: 10.1038/s41586-019-1897-5
112. Fan P, Li Z, Zuo C, Fang M. Promotion effects of mono-2-ethyhexyl phthalate (MEHP) on migration and invasion of human melanoma cells via activation of TGF-β signals. Cell Biochem Funct (2020) 38(1):38–46. doi: 10.1002/cbf.3447
113. Chen X, Huang C, Sun H, Hong H, Jin J, Bei C, et al. Puerarin suppresses inflammation and ECM degradation through Nrf2/HO-1 axis in chondrocytes and alleviates pain symptom in osteoarthritic mice. Food Funct (2021) 12(5):2075–89. doi: 10.1039/D0FO03076G
114. Luo Y, Xiong B, Liu H, Chen Z, Huang H, Yu C, et al. Koumine Suppresses IL-1β Secretion and Attenuates Inflammation Associated With Blocking ROS/NF-κB/NLRP3 Axis in Macrophages. Front Pharmacol (2020) 11:622074. doi: 10.3389/fphar.2020.622074
115. Zhang Q, Duan HX, Li RL, Sun JY, Liu J, Peng W, et al. Inducing Apoptosis and Suppressing Inflammatory Reactions in Synovial Fibroblasts are Two Important Ways for Guizhi-Shaoyao-Zhimu Decoction Against Rheumatoid Arthritis. J Inflammation Res (2021) 14:217–36. doi: 10.2147/JIR.S287242
116. Hayakawa Y, Hirata Y, Hata M, Tsuboi M, Oya Y, Kurokawa K, et al. Dysregulated Immune Responses by ASK1 Deficiency Alter Epithelial Progenitor Cell Fate and Accelerate Metaplasia Development during H. pylori Infection. Microorganisms (2020) 8(12):1995. doi: 10.3390/microorganisms8121995
117. Sun T, Zhang K, Pangeni RP, Wu J, Li W, Du Y, et al. G9a Promotes Invasion and Metastasis of Non-Small Cell Lung Cancer through Enhancing Focal Adhesion Kinase Activation via NF-κB Signaling Pathway. Mol Cancer Res MCR (2020) 19(3):429–40. doi: 10.21203/rs.3.rs-35579/v1
118. Li B, Li Y, Tomkiewicz-Raulet C, Dao P, Lietha D, Yen-Pon E, et al. Design, Synthesis, and Biological Evaluation of Covalent Inhibitors of Focal Adhesion Kinase (FAK) against Human Malignant Glioblastoma. J Med Chem (2020) 63(21):12707–24. doi: 10.1021/acs.jmedchem.0c01059
119. Zimmermann RC, Welch DR. BRMS1: a multifunctional signaling molecule in metastasis. Cancer Metastasis Rev (2020) 39(3):755–68. doi: 10.1007/s10555-020-09871-0
120. Deng Z, Zeng Q, Chai J, Zhang B, Zheng W, Xu X, et al. Disintegrin Tablysin-15 Suppresses Cancer Hallmarks in Melanoma Cells by Blocking FAK/Akt/ERK and NF-κB Signaling. Curr Cancer Drug Targets (2020) 20(4):306–15. doi: 10.2174/1568009620666200101094736
121. Chen PC, Kuo YC, Chuong CM, Huang YH. Niche Modulation of IGF-1R Signaling: Its Role in Stem Cell Pluripotency, Cancer Reprogramming, and Therapeutic Applications. Front Cell Dev Biol (2020) 8:625943. doi: 10.3389/fcell.2020.625943
122. Fernandes GMM, Galbiatti-Dias ALS, Ferreira LAM, Serafim Junior V, Rodrigues-Fleming GH, de Oliveira-Cucolo JG, et al. Anti-EGFR treatment effects on laryngeal cancer stem cells. Am J Trans Res (2021) 13(1):143–55.
123. Di Matteo S, Nevi L, Overi D, Landolina N, Faccioli J, Giulitti F, et al. Metformin exerts anti-cancerogenic effects and reverses epithelial-to-mesenchymal transition trait in primary human intrahepatic cholangiocarcinoma cells. Sci Rep (2021) 11(1):2557. doi: 10.1038/s41598-021-81172-0
124. Kokkorakis N, Gaitanou M. Minibrain-related kinase/dual-specificity tyrosine-regulated kinase 1B implication in stem/cancer stem cells biology. World J Stem Cells (2020) 12(12):1553–75. doi: 10.4252/wjsc.v12.i12.1553
125. Teixeira F, Vijaya Kumar A, Kumar Katakam S, Cocola C, Pelucchi P, Graf M, et al. The Heparan Sulfate Sulfotransferases HS2ST1 and HS3ST2 Are Novel Regulators of Breast Cancer Stem-Cell Properties. Front Cell Dev Biol (2020) 8:559554. doi: 10.3389/fcell.2020.559554
126. Liang X, Xu C, Cao X, Wang W. Isovitexin Suppresses Cancer Stemness Property And Induces Apoptosis Of Osteosarcoma Cells By Disruption Of The DNMT1/miR-34a/Bcl-2 Axis. Cancer Manage Res (2019) 11:8923–36. doi: 10.2147/CMAR.S222708
127. Sheng X, Zhu P, Zhao Y, Zhang J, Li H, Zhao H, et al. Effect of PI3K/AKT/mTOR Signaling Pathway on Regulating and Controlling the Anti-Invasion and Metastasis of Hepatoma Cells by Bufalin. Recent Patents Anti-cancer Drug Discovery (2021). doi: 10.2174/1574892816666210201120324
128. Chen L, Wu Q, Xu X, Yang C, You J, Chen F, et al. Cancer/testis antigen LDHC promotes proliferation and metastasis by activating the PI3K/Akt/GSK-3β-signaling pathway and the in lung adenocarcinoma. Exp Cell Res (2021) 398(2):112414. doi: 10.1016/j.yexcr.2020.112414
129. Tian J, Zhang H, Mu L, Wang M, Li X, Zhang X, et al. The miR-218/GAB2 axis regulates proliferation, invasion and EMT via the PI3K/AKT/GSK-3β pathway in prostate cancer. Exp Cell Res (2020) 394(1):112128. doi: 10.1016/j.yexcr.2020.112128
130. Yang XX, Ma M, Sang MX, Zhang XY, Zou NY, Zhu SC. Knockdown of FAM83D Enhances Radiosensitivity in Coordination with Irradiation by Inhibiting EMT via the Akt/GSK-3β/Snail Signaling Pathway in Human Esophageal Cancer Cells. OncoTarg Ther (2020) 13:4665–78. doi: 10.2147/OTT.S245681
131. Nabih HK. Crosstalk between NRF2 and Dicer through metastasis regulating MicroRNAs; mir-34a, mir-200 family and mir-103/107 family. Arch Biochem Biophysics (2020) 686:108326. doi: 10.1016/j.abb.2020.108326
132. Zhang M, Jabbari N, Satpathy M, Matyunina LV, Wang Y, McDonald LD, et al. Sequence diverse miRNAs converge to induce mesenchymal-to-epithelial transition in ovarian cancer cells through direct and indirect regulatory controls. Cancer Lett (2019) 459:168–75. doi: 10.1016/j.canlet.2019.05.039
133. Ji Q, Liu X, Fu X, Zhang L, Sui H, Zhou L, et al. Resveratrol inhibits invasion and metastasis of colorectal cancer cells via MALAT1 mediated Wnt/β-catenin signal pathway. PloS One (2013) 8(11):e78700. doi: 10.1371/journal.pone.0078700
134. Patel KR, Brown VA, Jones DJ, Britton RG, Hemingway D, Miller AS, et al. Clinical pharmacology of resveratrol and its metabolites in colorectal cancer patients. Cancer Res (2010) 70(19):7392–9. doi: 10.1158/0008-5472.CAN-10-2027
135. Howells LM, Berry DP, Elliott PJ, Jacobson EW, Hoffmann E, Hegarty B, et al. Phase I randomized, double-blind pilot study of micronized resveratrol (SRT501) in patients with hepatic metastases–safety, pharmacokinetics, and pharmacodynamics. Cancer Prev Res (Philadelphia Pa) (2011) 4(9):1419–25. doi: 10.1158/1940-6207.CAPR-11-0148
136. Thomas SK, Lee J, Beatty GL. Paracrine and cell autonomous signalling in pancreatic cancer progression and metastasis. EBioMedicine (2020) 53:102662. doi: 10.1016/j.ebiom.2020.102662
137. Li J, Qi D, Hsieh TC, Huang JH, Wu JM, Wu E. Trailblazing perspectives on targeting breast cancer stem cells. Pharmacol Ther (2021) 223:107800. doi: 10.1016/j.pharmthera.2021.107800
138. Rodriguez-Aznar E, Wiesmüller L, Sainz B Jr., Hermann PC. EMT and Stemness-Key Players in Pancreatic Cancer Stem Cells. Cancers (2019) 11(8):1136. doi: 10.3390/cancers11081136
139. Tao YK, Hou XY, Gao H, Zhang X, Zuo FM, Wang Y, et al. Grade-targeted nanoparticles for improved hypoxic tumor microenvironment and enhanced photodynamic cancer therapy. Nanomed (London England) (2021) 16(3):221–35. doi: 10.2217/nnm-2020-0096
140. Emami Nejad A, Najafgholian S, Rostami A, Sistani A, Shojaeifar S, Esparvarinha M, et al. The role of hypoxia in the tumor microenvironment and development of cancer stem cell: a novel approach to developing treatment. Cancer Cell Int (2021) 21(1):62. doi: 10.1186/s12935-020-01719-5
141. Samec M, Liskova A, Koklesova L, Mersakova S, Strnadel J, Kajo K, et al. Flavonoids Targeting HIF-1: Implications on Cancer Metabolism. Cancers (2021) 13(1):130. doi: 10.3390/cancers13010130
142. Chen H, Wang H, Yu X, Zhou S, Zhang Y, Wang Z, et al. ERCC6L promotes the progression of hepatocellular carcinoma through activating PI3K/AKT and NF-κB signaling pathway. BMC Cancer (2020) 20(1):853. doi: 10.1186/s12885-020-07367-2
143. Vaquero J, Aoudjehane L, Fouassier L. Cancer-associated fibroblasts in cholangiocarcinoma. Curr Opin Gastroenterol (2020) 36(2):63–9. doi: 10.1097/MOG.0000000000000609
144. Jia C, Wang G, Wang T, Fu B, Zhang Y, Huang L, et al. Cancer-associated Fibroblasts induce epithelial-mesenchymal transition via the Transglutaminase 2-dependent IL-6/IL6R/STAT3 axis in Hepatocellular Carcinoma. Int J Biol Sci (2020) 16(14):2542–58. doi: 10.7150/ijbs.45446
145. Ferraresi A, Phadngam S, Morani F, Galetto A, Alabiso O, Chiorino G, et al. Resveratrol inhibits IL-6-induced ovarian cancer cell migration through epigenetic up-regulation of autophagy. Mol Carcinogen (2017) 56(3):1164–81. doi: 10.1002/mc.22582
146. Huang CY, Chung CL, Hu TH, Chen JJ, Liu PF, Chen CL. Recent progress in TGF-β inhibitors for cancer therapy. Biomed Pharmacother Biomed Pharmacother (2021) 134:111046. doi: 10.1016/j.biopha.2020.111046
147. Chen W, Huang B, Wang E, Wang X. MiR-145 inhibits EGF-induced epithelial-to-mesenchymal transition via targeting Smad2 in human glioblastoma. OncoTarg Ther (2019) 12:3099–107. doi: 10.2147/OTT.S202129
148. Fukuda S, Nishida-Fukuda H, Nanba D, Nakashiro K, Nakayama H, Kubota H, et al. Reversible interconversion and maintenance of mammary epithelial cell characteristics by the ligand-regulated EGFR system. Sci Rep (2016) 6:20209. doi: 10.1038/srep20209
149. Hartman ZC, Poage GM, den Hollander P, Tsimelzon A, Hill J, Panupinthu N, et al. Growth of triple-negative breast cancer cells relies upon coordinate autocrine expression of the proinflammatory cytokines IL-6 and IL-8. Cancer Res (2013) 73(11):3470–80. doi: 10.1158/0008-5472.CAN-12-4524-T
150. Wen FC, Chang TW, Tseng YL, Lee JC, Chang MC. hRAD9 functions as a tumor suppressor by inducing p21-dependent senescence and suppressing epithelial-mesenchymal transition through inhibition of Slug transcription. Carcinogenesis (2014) 35(7):1481–90. doi: 10.1093/carcin/bgu009
151. Vadlamudi Y, Dey DK, Kang SC. Emerging Multi-cancer Regulatory Role of ESRP1: Orchestration of Alternative Splicing to Control EMT. Curr Cancer Drug Targets (2020) 20(9):654–65. doi: 10.2174/1568009620666200621153831
152. Qiu Y, Ching WK, Zou Q. Prediction of RNA-binding protein and alternative splicing event associations during epithelial-mesenchymal transition based on inductive matrix completion. Briefings Bioinf (2021). doi: 10.1093/bib/bbaa440
153. Osada AH, Endo K, Kimura Y, Sakamoto K, Nakamura R, Sakamoto K, et al. Addiction of mesenchymal phenotypes on the FGF/FGFR axis in oral squamous cell carcinoma cells. PloS One (2019) 14(11):e0217451. doi: 10.1371/journal.pone.0217451
154. Liu X, Zhou XQ, Shang XW, Wang L, Li Y, Yuan H, et al. Inhibition of chemotherapy-related breast tumor EMT by application of redox-sensitive siRNA delivery system CSO-ss-SA/siRNA along with doxorubicin treatment. J Zhejiang Univ Sci B (2020) 21(3):218–33. doi: 10.1631/jzus.B1900468
155. Zhu W, Qin W, Zhang K, Rottinghaus GE, Chen YC, Kliethermes B, et al. Trans-resveratrol alters mammary promoter hypermethylation in women at increased risk for breast cancer. Nutr Cancer (2012) 64(3):393–400. doi: 10.1080/01635581.2012.654926
156. López-Grueso MJ, Lagal DJ, García-Jiménez ÁF, Tarradas RM, Carmona-Hidalgo B, Peinado J, et al. Knockout of PRDX6 induces mitochondrial dysfunction and cell cycle arrest at G2/M in HepG2 hepatocarcinoma cells. Redox Biol (2020) 37:101737. doi: 10.1016/j.redox.2020.101737
157. Børretzen A, Gravdal K, Haukaas SA, Beisland C, Akslen LA, Halvorsen OJ. FOXC2 expression and epithelial-mesenchymal phenotypes are associated with castration resistance, metastasis and survival in prostate cancer. J Pathol Clin Res (2019) 5(4):272–86. doi: 10.1002/cjp2.142
158. Choi MJ, Cho KH, Lee S, Bae YJ, Jeong KJ, Rha SY, et al. hTERT mediates norepinephrine-induced Slug expression and ovarian cancer aggressiveness. Oncogene (2015) 34(26):3402–12. doi: 10.1038/onc.2014.270
159. Broertjes J, Klarenbeek J, Habani Y, Langeslag M, Jalink K. TRPM7 residue S1269 mediates cAMP dependence of Ca2+ influx. PloS One (2019) 14(1):e0209563. doi: 10.1371/journal.pone.0209563
160. Xie B, Wang S, Jiang N, Li JJ. Cyclin B1/CDK1-regulated mitochondrial bioenergetics in cell cycle progression and tumor resistance. Cancer Lett (2019) 443:56–66. doi: 10.1016/j.canlet.2018.11.019
161. Wang X, Guo J, Yu P, Guo L, Mao X, Wang J, et al. The roles of extracellular vesicles in the development, microenvironment, anticancer drug resistance, and therapy of head and neck squamous cell carcinoma. J Exp Clin Cancer Res CR (2021) 40(1):35. doi: 10.1186/s13046-021-01840-x
162. Popat R, Plesner T, Davies F, Cook G, Cook M, Elliott P, et al. A phase 2 study of SRT501 (resveratrol) with bortezomib for patients with relapsed and or refractory multiple myeloma. Br J Haematol (2013) 160(5):714–7. doi: 10.1111/bjh.12154
Keywords: epithelial-to-mesenchymal transition, metastasis, chemoresistance, cancer cell stemness, resveratrol
Citation: Guo K, Feng Y, Zheng X, Sun L, Wasan HS, Ruan S and Shen M (2021) Resveratrol and Its Analogs: Potent Agents to Reverse Epithelial-to-Mesenchymal Transition in Tumors. Front. Oncol. 11:644134. doi: 10.3389/fonc.2021.644134
Received: 20 December 2020; Accepted: 17 March 2021;
Published: 16 April 2021.
Edited by:
Darren Boehning, Cooper Medical School of Rowan University, United StatesReviewed by:
Qingyong Ma, First Affiliated Hospital of Xi’an Jiaotong University, ChinaShayahati Bieerkehazhi, University of Texas Health Science Center at Houston, United States
Copyright © 2021 Guo, Feng, Zheng, Sun, Wasan, Ruan and Shen. This is an open-access article distributed under the terms of the Creative Commons Attribution License (CC BY). The use, distribution or reproduction in other forums is permitted, provided the original author(s) and the copyright owner(s) are credited and that the original publication in this journal is cited, in accordance with accepted academic practice. No use, distribution or reproduction is permitted which does not comply with these terms.
*Correspondence: Shanming Ruan, c2hhbm1pbmdydWFuQHpjbXUuZWR1LmNu; Minhe Shen, c2hlbm1pbmhlQHpjbXUuZWR1LmNu
†These authors have contributed equally to this work and share first authorship