- 1Key Laboratory of Precision Oncology of Shandong Higher Education, Institute of Precision Medicine, Jining Medical University, Jining, China
- 2Department of Biochemistry and Molecular Biology, School of Basic Medical Sciences, Southern Medical University, Guangzhou, China
- 3Research Institute of Medical Sciences, Chonnam National University Medical School, Gwangju, South Korea
Phytochemicals are natural small-molecule compounds derived from plants that have attracted attention for their anticancer activities. Some phytochemicals have been developed as first-line anticancer drugs, such as paclitaxel and vincristine. In addition, several phytochemicals show good tumor suppression functions in various cancer types. Bladder cancer is a malignant tumor of the urinary system. To date, few specific phytochemicals have been used for bladder cancer therapy, although many have been studied in bladder cancer cells and mouse models. Therefore, it is important to collate and summarize the available information on the role of phytochemicals in the prevention and treatment of bladder cancer. In this review, we summarize the effects of several phytochemicals including flavonoids, steroids, nitrogen compounds, and aromatic substances with anticancer properties and classify the mechanism of action of phytochemicals in bladder cancer. This review will contribute to facilitating the development of new anticancer drugs and strategies for the treatment of bladder cancer using phytochemicals.
Introduction
Cancer has multiple causes, such as genetic mutation and cellular dysregulation. The disease is characterized by uncontrolled cell growth, abnormal differentiation, proliferation, invasion, and metastasis (1). Several plant compounds inhibit cancer cell phenotypes. Based on this anticancer activity, several phytochemicals have been developed as FDA-approved drugs for cancer therapy (2, 3). For example, paclitaxel has been carried out the clinical trial and been a first-line treatment for lung, ovarian, and breast cancer (3). Paclitaxel promotes tubulin polymerization and assembly, and prevents depolymerization, thereby stabilizing tubulin, inhibiting cancer cell mitosis, and preventing cell apoptosis to effectively prevent cancer cell proliferation and exhibit anticancer activity (3). Paclitaxel has also entered the clinical trial stage III for bladder cancer with other chemotherapeutic drugs together - paclitaxel/gemcitabine/cisplatin (PGC): 142 patients were involved into this clinical trial (74 observation and 68 PGC treatment). This study indicated that PGC improves overall survival in high risk invasive bladder cancer (4). However paclitaxel alone has not been carried out clinical trial study on bladder cancer. Another phytochemical-derived antitumor drug, vincristine, is used to treat acute lymphoblastic leukemia and is effective in treating other acute leukemia, lymphatic sarcoma, reticular cell sarcoma, and breast cancer. It exerts these effects via suppression of microtubule formation in mitotic spindles, resulting in cell cycle arrest at the metaphase stage (5, 6).
Phytochemicals can be used for cancer treatment and prevention. Furthermore, these compounds can be used to treat patients whose cancer has recurred and can contribute to preventing carcinogenesis in those who do not have cancer during their lifetime. Resveratrol (3,5,4’-trihydroxy-trans-stilbene), a non-flavonoid polyphenol found in several food plants including grapes, peanuts, soy beans, berries, and pomegranates, possesses cancer prevention benefits via its anti-proliferative and anti-oxidative activities (7). Because phytochemicals are abundant in food sources and have low side effects, an increasing number have been developed as health supplements, including resveratrol and curcumin (8).
Bladder cancer is a common cancer of the urinary system. Because muscle invasive bladder cancer (MIBC) has a high mortality rate, and non-muscle invasive bladder cancer (NMIBC) has a high recurrence rate, both cancer types cause considerable physical and physiological pain to patients (9). Although phytochemicals have been studied for the treatment and prevention of bladder cancer, a systematic summary of their uses is lacking. Further, few drugs based on phytochemicals and their derivatives have been developed. Therefore, a comprehensive summary of previous studies on the activity of phytochemicals against bladder cancer is important for focusing on research priorities, achieving novel breakthroughs, and developing new anticancer drugs. In this review, two decades of research on phytochemical-based treatment of bladder cancer are systematically summarized.
Flavonoids
Flavonoids are natural compounds that have a 2-phenylfluorone structure with a ketone carbonyl group and a basic oxygen atom. These compounds form salts in the presence of strong acids and the resulting hydroxyl derivatives are usually yellow. Thus, these compounds are also called flavins or flavones (10). In plants, flavonoids usually combine with sugars to form glycosides with a small portion existing in a free state (aglycone). A considerable number of plants contain flavonoids, which play important roles in growth, development, flowering, fruit development, and disease prevention. Recently, the anticancer activities of flavonoids have been reported (11).
Flavone
Baicalein is a flavone extracted from Scutellaria altissima L or S. baicalensis Georgi (12). Previous studies showed that baicalin suppresses bladder cancer T24 cell proliferation by inhibiting the cell cycle at the G1/S phase (13). Moreover, baicalein increases apoptosis through a mitochondrial- and caspase-dependent pathway in T24 cells. Indeed, baicalin activates caspase 9 and caspase 3, decreases B-cell lymphoma-2 (Bcl-2) expression, and elevates Bcl-2 associated X protein (Bax) expression, thereby causing apoptosis of T24 cells (14). Chinese scholars reported that 40~80 μmol/L baicalein effectively kills bladder cancer cells by suppressing cell proliferation via downregulating cyclin B1 expression and decreasing CDC2 phosphorylation at Thr161 in bladder cancer T24 and HT1376 cells (15). In addition, baicalin inhibits bladder cancer cell invasion by attenuating matrix metallopeptidases (MMPs) levels including MMP-9 and MMP-2 in bladder carcinoma 5637 cells (16).
Apigenin, a flavonoid found in celery, is widely recognized for its anticancer activity. Our previous study demonstrated that apigenin suppresses cancer cell invasion by downregulating urokinase-type plasminogen activator receptor (uPAR) expression in bladder cancer T24 cells. In particular, apigenin showed substantial anticancer activity through the suppression of high levels of interleukin (IL)-1β-induced uPAR expression (17). These effects are mediated via the inhibition of mitogen-activated protein kinase (MAPK)-induced nuclear factor (NF)-κB and activator protein (AP)-1 activity, which are essential transcription factors for uPAR (17). Finally, apigenin suppresses T24 bladder cancer cell proliferation by arresting the cell cycle in the G2/M phase (18, 19).
Luteolin, widely found in nature, was originally isolated from the leaves, stems, and branches of plants of the Resedaceae family. Recently, luteolin was reported to inhibit bladder cancer cell viability and induce G2/M cell cycle arrest via upregulation of p21/WAF1 and reduction of phosphorylated S6 (p-S6), a key downstream molecule in the mechanistic target of rapamycin (mTOR) signaling pathway. Furthermore, luteolin also has an antioxidant effect that reduces intracellular reactive oxygen species (ROS) by elevating thioredoxin 1. Orally administered luteolin showed antitumor activity by markedly suppressing tumor growth in a xenograft mouse model by upregulating p21/WAF1 and repressing mTOR signaling (20).
Nobiletin, a methoxylated flavone extracted from citrus fruit peel, has anticancer activities. For example, nobiletin effectively inhibits BFTC-905 bladder cancer cell growth, increases DNA fragmentation, and expedites apoptosis. Additionally, this compound triggers mitochondrial dysfunction, leading to cytochrome C release into the cytosol, which in turn, upregulates the pro-apoptotic proteins Bcl-2 associated agonist of cell death (Bad), Bax, caspase 3, and caspase 9. These effects of nobiletin also downregulate the anti-apoptotic proteins Bcl-2 and myeloid cell leukemia sequence (MCL)-1 by affecting the phosphoinositide 3-kinase (PI3K)/AKT/mTOR axis and PERK/eukaryotic translation initiation factor 2α kinase (elF2α)/activating transcription factor 4 (ATF4)/C/EBP homologous protein axis (21).
Tangeretin is among the most abundant flavones in citrus peels, and its anticancer properties have been described, such as its inhibition of bladder carcinoma BFTC-905 cell viability through apoptosis. Besides, tangeretin induces calcium homeostasis in the mitochondria, activates caspase 3 and caspase-9, and causes cytochrome C release, which in turn, enhances apoptosis (22).
3′-Hydroxyflavone
3-Hydroxyflavone contains a 3-hydroxyl group and has an excellent resonance structure and chelating ability, as well as anti-inflammatory, antitumor, and antiviral properties (23). Casticin, a 3′-hydroxyflavone isolated from Vitex rotundifolia, induces DNA damage and inhibits TSGH-8301 bladder cancer cell viability (24). Additionally, casticin decreases ataxia telangiectasia mutated (ATM) andataxia telangiectasia and Rad3 related (ATR) phosphorylation and downregulates mediator of DNA damage checkpoint 1 (MDC1) and O-6-methylguanine-DNA methyltransferase (MGMT). Moreover, casticin increases p-p53, H2A.X variant histone, and poly-ADP ribose polymerase (PARP) levels, and affects phospho-p53 translocation from the cytoplasm to the nucleus in bladder cancer cells (24). This compound also increases intracellular ROS production, activates the caspase cascade, and disrupts the mitochondrial membrane potential (ΔΨm). X-linked inhibitor of apoptosis protein–associated factor 1 and transcriptionally active p73 are upregulated by casticin treatment in T24 bladder cancer cells (25).
The 3′-hydroxy-flavone kaempferol, widely found in various fruits, vegetables, and beverages, and its anticancer activities have been reported in several studies as follows. Dang et al. (26) showed that kaempferol inhibits bladder cancer proliferation and invasion by downregulating the c-Met/p38 signaling pathway in bladder cancer 5637, T24, 253J, and TCCSUP cells. Wu et al. (27) reported the anti-oxidative and anticancer activities of kaempferol in bladder cancer. Furthermore, kaempferol attenuates ROS-induced hemolysis and decreases cell proliferation by suppressing p-AKT, Bcl lymphoma extra-large (Bcl-xL), cyclin-dependent kinase 4 (CDK4), cyclin D1, and MCL-1. In addition, it enhances p21/WAF1, p38, p53, p-ATM, p-BRCA1 DNA repair-associated, Bax, and BH3 interacting domain death agonist (Bid) expression, leading to S phase arrest and the induction of apoptosis of EJ bladder cancer cells (28).
Fisetin, a flavonoid derived from Rhus succedanea L., induces apoptosis of human bladder cancer by increasing the ratio between pro-apoptotic and anti-apoptotic proteins by upregulating p53 and downregulating NF-κB activity in T24 and EJ bladder cancer cells. Additionally, fisetin elevates p53 and p21/WAF1 protein levels and decreases CDK2, CDK4, cyclin A, and cyclin D1 levels, thereby leading to G0/G1 cell cycle arrest. Moreover, fisetin increases Bcl antagonist/killer (Bak) and Bax expression and decreases Bcl-2 and Bcl-xL expression, which in turn triggers apoptosis (28).
Morinis a pale-yellow pigment extracted from the bark of plants of the mulberry family such as the yellow mulberry, mulberry orange tree, and many Chinese herbs. Morin has been shown to exhibit multiple anticancer activities such as inhibition of bladder cancer cell proliferation and invasion (29). Morin decreases CDK2, CDK4, cyclin D1, and cyclin E via modulation of the p21/WAF1 pathway, suppressing c-Jun N-terminal kinase (JNK) and AKT phosphorylation, and preventing MMP-9 expression by repressing NF-κB, SP-1, and AP-1 in EJ bladder cancer cells (29).
Quercetin is a flavonol that is widely distributed in the plant kingdom and exhibits various biological activities. Several studies have shown that quercetin inhibits cell survival and induces apoptosis of bladder cancer cell lines: This compound also upregulates tumor suppressors by inhibiting CDK inhibitor 2A (CDKN2A) and Ras association domain family member 1A (RASSF1A) gene methylation (30, 31). Quercetin inhibits cancer cell growth by arresting the cell cycle at the G0/G1 phase and decreases the protein expression of mutant p53 and survivin in EJ, J82, and T24 bladder cancer cells (30).
Isoflavone
Isoflavones are phenolic compounds formed by cyclization following the extension of the cinnamyl coenzyme A side chain during phenylalanine metabolism in plants (32). The isoflavone genistein mainly exists in legumes such as the locust horn and mountain bean root (33). Genistein inhibits bladder cancer cells proliferation by arresting the cell cycle at the G2/M phase via suppression of cyclin A and cyclin B1, and upregulation of CDKN1A (p21/WAF1). Moreover, genistein also inactivates the PI3K/AKT signaling pathway by increasing ROS accumulation in T24 bladder cancer cells (34). Interestingly, genistein also sensitizes bladder cancer cells to hydroxycamptothecin treatment in vitro and in vivo through ATM/NF-κB/inhibitor of NF-κB kinase (IKK)-mediated apoptosis (35). Another representative isoflavone is puerarin, which is isolated from Radix puerariae. Ye et al. (36) reported that puerarin inhibits proliferation and triggers apoptosis of T24 bladder cancer cells. The proposed underlying mechanism is the inhibition of the sirtuin 1 (SIRT1)/p53 signaling pathway (36). Puerarin also inhibits bladder cancer cell viability through cell cycle arrest at the G0/G1 phase by a mechanism that involves downregulation of mTOR and p70S6K phosphorylation, without affecting their protein levels (37). Moreover, puerarin upregulates the microRNA miR-16, which subsequently downregulates cyclooxygenase (COX)-2 expression via NF-κB signaling pathway inactivation, thereby decreasing the viability of T24 bladder cancer cells (38).
Flavonol
Catechins are typical flavanols and (-)-epigallocatechin-3-gallate (EGCG), a bioactive compound extracted from green tea, is among the most widely studied anticancer catechin compounds. It has been extensively studied for its beneficial effects on various cancers, including bladder cancer (39). EGCG inhibits cancer cell proliferation and deactivates DNA methyltransferase activity of T24 bladder cancer cells (40). In SW780 bladder carcinoma cells, EGCG also showed anticancer activity by effectively inhibiting their proliferation and migration by suppressing NF-κB and downregulating MMP-9 (41). Lee et al. (41) studied the effects of EGCG on the transcriptome of BFTC-905 bladder cancer cells and found that it substantially changed the transcription of 108 genes. These genes are mainly involved in inflammatory responses, oxidation-reduction metabolism, and nicotinamide adenine dinucleotide biogenesis. The genes upregulated by EGCG include general transcription factor IIH subunit 2 family member C (GTF2H2C), proline rich transmembrane protein 2 (PRRT2), RAS p21 protein activator 4 (RASA4), transmembrane protein 92 (TMEM92), and RANBP2 like and GRIP domain containing 5 (RGPD5), whereas those downregulated include kynurenine 3-monooxygenase (KMO), NADH: ubiquinone oxidoreductase core subunit S1 (NDUFS1), gastric inhibitory polypeptide receptor (GIPR), and thioredoxin domain containing 2 (TXNDC2). Furthermore, many miRNA-mRNA interactions that mediate the response to EGCG treatment have been identified, including miR-22-3p-protein phosphatase 1K (miR-22-3p-PPM1K), miR-31-5p-tensin 1 (miR-31-5p-TNS1), and miR-185-3p-arrestin beta 1 (miR-185-3p-ARRB1) (42). Recently, a double-blind, randomized, clinical trial was performed in presurgical bladder cancer patients. The results showed EGCG modulated many cell proliferation related molecules e.g. PCNA, MMP2, VEGF, IGF-1, IGFBP-3 in bladder tumor tissue, which indicated EGCG would be an effective and safe cancer preventive agent for bladder cancer (43)
Silibinin is a dihydroflavonol with anticancer activity that inhibits transforming growth factor (TGF)−β1−induced cancer cell invasion by attenuating the epithelial-to-mesenchymal transition (EMT). TGF−β1−induced COX−2 expression is inhibited by silibinin (44). In addition, silibinin sensitizes bladder cancer cells to radiation treatment and photodynamic therapy (PDT) (45, 46). In vitro, silibinin sensitizes murine invasive cells to radiotherapy by inhibiting radiotherapy-activated NF-κB and PI3K signaling. In vivo, silibinin enhances responses to radiotherapy and overall survival rate in patients with invasive bladder tumors (45). Silibinin also enhances the anticancer effect of PDT, which is a photosensitization-based anticancer therapeutic strategy for malignant cells. This indicates that silibinin has potential as an adjuvant for PDT based on its activity against T24 human bladder cancer cells (46). In addition, silibinin markedly attenuates bladder cancer tumor chemoresistance through NF-κB-dependent and -independent mechanisms in T24 and J82 bladder cancer cells (47).
Flavanone
Flavanones are dihydroflavones found in the peels of citrus fruits such as lemon and pomelo fruits. Naringenin is an example an active flavanone found in citrus fruit extracts, and is known for its pharmacological utility (48, 49). Although the anticancer activities of naringenin have been widely reported, recent experimental evidence indicates that naringenin inhibits TSGH-8301 bladder cancer cell migration via deactivation of AKT signaling and MMP-2 downregulation (50).
Naringin dose-dependently inhibits the proliferation of 5637 bladder cancer cells by arresting the cell cycle in the G1 phase. Moreover, naringin strongly induces p21/WAF1 expression and decreases cyclins (cyclin D1 and cyclin E), and CDKs (CDK4 and CDK6) expression. Furthermore, naringin activates the MAPK signal transduction pathways by elevating the phosphorylation of extracellular signal-regulated kinase 1 and 2 (ERK1/2), p38 MAPK, and JNK in 5637 bladder cancer cells (51).
Flavonoid Glycosides
Baicalein, a flavonoid glycoside, has impressive anticancer activities (52). Baicalein induces apoptosis by activating apoptosis-related genes, including Bcl2, Bcl-xL, and XIAP, with effects on both their mRNA and protein levels in T24 and 253J bladder cancer cells (53). Other studies have shown that baicalein suppresses proliferation and migration while enhancing apoptosis. Further, baicalein strongly downregulates miR-106, which targets p21/WAF1 to inhibit JNK, MAPK kinase (MEK), and ERK in T24 bladder cancer cells (54). Baicalein also promotes apoptosis via an ROS-dependent pathway in human bladder cancer 5637 cells. Finally, baicalein downregulates several anti-apoptotic proteins such as cellular inhibitor of apoptosis protein (cIAP)-1 and cIAP-2, and activates caspase 9 and caspase 3 (55).
Orientin is a polyhydroxy-flavanone found in Lophatherum gracile and Trollius chinensis Bunge (56). This compound inhibits cancer cell proliferation, reduces cell viability, causes cell cycle arrest, and represses the expression of inflammatory mediators in T24 bladder cancer cells. In addition, orientin decreases the expression of NF-κB components and blocks the Hedgehog signaling pathway (57).
Biflavone
Ginkgetin, amentoflavone, sotetsuflavone, and hinokiflavone are representative biflavones. Ginkgetin, one of the best known biflavones, has been used to treat breast (58), lung (59), and prostate (60) cancers; however, its effects on bladder cancer have not been reported. Sotetsuflavone and hinokiflavone suppress lung cancer, but not bladder cancer. Amentoflavone markedly inhibits TSGH-8301 bladder cancer cell activity via the induction of mitochondria-dependent intrinsic apoptosis and Fas cell surface death receptor (FAS)/FAS ligand (FASL)-dependent extrinsic apoptosis. Further, amentoflavone reduces the expression of anti-apoptotic proteins including MCL-1 and cellular FLICE-like inhibitory protein, thereby enhancing apoptosis (61).
Steroids
Phytosterols
Phytosterols are plant sterols and mainly include those found in grain, rapeseed oil, beans, and rapeseed sterols, and corresponding alkanols. Phytosterols are active plant constituents that have numerous human health benefits (62). Studies have found that phytosterols reduce blood cholesterol, prevent and treat prostatic hypertrophy, inhibit tissue hyperplasia, and regulate immunity (62, 63). Recently, the anticancer activities of phytosterols have been described (64, 65).
Dioscin, a plant-based steroid, mainly comes from the roots of the ginger plant (66). This compound induces cancer cell apoptosis by demethylation of death-associated protein kinase 1 (DAPK-1) and Ras-association domain family 1α (RASSF-1α) genes via antioxidant activity in T24 and 5637 bladder cancer cells. In many carcinomas, DNA is often methylated at CpG rich regions (66), and many tumor suppressor genes are often hypermethylated including RASSF-1α and DAPK-1, whichhave been identified in studies conducted in some western countries. Dioscin regulates RASSF-1α and DAPK-1 methylation, which in turn elevates their expression. Moreover, dioscin specifically inhibits bladder tumor cells, but not normal bladder epithelial cells (66).
Solasonine is a steroidal glycoside alkaloid isolated from black nightshade, and its anticancer activity has not been widely studied because of its low water solubility. Miranda et al. (67) developed a nanotechnology-based strategy using poly (D, L-lactide) nanoparticles to improve the performance of solasonine for bladder cancer therapy. Interestingly, this study showed high nanoparticle uptake by RT4 bladder and MDA-MB-231 breast cancer cells, but not by normal HaCaT keratinocytes cells, suggesting targeted cancer cell uptake (67).
In addition to bladder and breast cancers, solasonine inhibits hepatocellular carcinoma cells via the non-apoptotic cell death pathway, ferroptosis. Solasonine suppresses glutathione synthetase (GSS) and glutathione peroxidase 4 (GPX4) (68). GSS, the key enzyme in the synthesis of the oxidation inhibitor GSH, suppresses ferroptosis by regulating the intracellular peroxidation environment. GPX4 prevents ferroptosis by converting hydroperoxide lipids into nontoxic lipid alcohols (68).
Monoterpenes
Monoterpenes are terpenoids usually derived from the polymerization of two isoprene molecules, and their oxygen-containing and saturated derivatives (69). Monoterpenes are divided into the following four categories according to the basic carbon skeleton of the molecule: acyclic monoterpenes, single-ring monoterpenes, double-ring monoterpenes, and tricyclic monoterpenes. Monoterpenes are important materials for the pharmaceutical, food, and cosmetic industries (69). Limonene, a monocyclic monoterpene, is found in citrus fruits, especially the peels. Recently, limonene was found to strongly suppress the viability of human T24 bladder cancer cells [half-maximal inhibitory concentration (IC50)= 9 μM] (70). In addition, it caused chromatin concentration and nuclear fragmentation, thereby inducing bladder cancer cell apoptosis by the downregulation of Bcl-2 expression and upregulation of Bax and caspase-3 expression. Moreover, limonene also induces cell cycle arrest in the G2/M phase and inhibits bladder cancer cell migration and invasion (70). The terpenoid linalool exhibits strong anticancer activity against various carcinoma cells, particularly cervical (IC50 = 0.37 μg/mL), stomach (IC50 = 14.1 μg/mL), skin (IC50 = 14.9 μg/mL), lung (IC50 = 21.5 μg/mL), and bone (IC50 = 21.7 μg/mL) carcinoma (71).
Sesquiterpenes
Sesquiterpenes are natural terpenes that contain three isoprene units and have various skeletal structures, such as chains and rings. Sesquiterpenes are mostly liquid and are mainly found in volatile plant oils. Curcumol, a natural sesquiterpene with anticancer activity, was originally isolated from Curcuma rhizomes (72). Zhou et al. (73) reported that curcumol inhibits EJ and T24 bladder cancer cell proliferation and induces apoptosis in a dose-dependent manner by decreasing the accumulation of enhancer of zeste homolog 2 (EZH2).
Atractylenolide I, an active sesquiterpene component extracted from Rhizoma atractylodis, inhibits T24 bladder cancer cell proliferation by arresting the cell cycle in the G2/M phase via downregulation of cell cycle-related proteins such as CDK1, cell division cycle 25C (CDC25c), and cyclin B1. Moreover, atractylenolide I promotes apoptosis via activation of the mitochondria-dependent apoptotic pathway and inhibition of the PI3K/AKT pathway in both T24 cells and xenografted tumors (74).
Parthenolide, a sesquiterpene lactone found in the herb, Brachynoma, has antitumor activity. Further, parthenolide substantially decreases bladder cancer cell viability via G1 phase cell cycle arrest by modulation of CDK2 phosphorylation. It also triggers apoptosis by enhancing Bcl-2 degradation and stabilizing PARP in 5637 bladder cancer cells (75).
A sesquiterpene lactone called costunolide exhibits anticancer properties in numerous cancers, including of the bladder. Costunolide dose-dependently inhibits cell viability, induces apoptosis, downregulates Bcl-2 and survivin expression, activates caspase 3, and elevates Bax expression in T24 bladder cancer cells (76).
Diterpenes
Yuanhuacine, an active diterpene from Daphne genkwa, has a wide range of antitumor activities. Zhang et al. (77) discovered that yuanhuacine inhibits T24T bladder cancer cell proliferation by arresting the cell cycle in the G2/M phase in an SP1-dependent manner. Yuanhuacine upregulates p21/WAF1 expression through a p38 pathway, but does not affect p53 or activate p53 protein levels.
Triptolide is a diterpenoid epoxide compound extracted from the roots, leaves, flowers, and fruits of Tripterygium wilfordii, with widely reported anticancer properties (78). Triptolide inhibits T24 bladder cancer cell proliferation with an IC50 of 68 ± 5 nmol/L after 72 h. Further, 25, 50, 100, and 250 nmol/L triptolide induced 27% ± 4%, 38% ± 5%, 50% ± 9%, and 65% ± 8% apoptosis, respectively. In this mechanism, triptolide decreases AKT and Forkhead box O3A phosphorylation and increases Bax, Bim, and cleaved caspase 3 levels (79). Triptolide also has excellent effects in drug cotherapy for bladder cancer, and in combination with gemcitabine, it is more effective against bladder cancer than gemcitabine alone. Further, it induces cell cycle arrest in the G1 phase through downregulation of cyclin A1, cyclin A2, CDK4, and CDK6. Moreover, apoptosis-related proteins such as Bcl-xL and caspase 8 are also elevated following triptolide and gemcitabine cotreatment (80).
Triptolide can also be used in combination with hydroxycamptothecin to enhance the anticancer effect of chemotherapeutic drugs on bladder cancer cells. This effect is achieved by increasing apoptosis through the upregulation of apoptosis-related proteins (Bcl-xL and Caspase 8) and inhibition of cyclin D1, CDK4, and CDK6 expression, which arrested the cell cycle of EJ and UMUC3 bladder cancer cells in the G1/S phase (81). In addition, some diterpenes with antitumor activity, such as jolkinolide B, cafestol, and oridonin, exhibited excellent efficacy against prostate, esophageal, and lung cancer, but have not been studied in bladder cancer (82–84).
Triterpenes
Triterpenes are composed of several isoprenoids joined end to end by the removal of the hydroxyl group, and some of these compounds have antitumor activity. Ursolic acid, a triterpene presents in Prunella vulgaris L and Ilex rotunda Thunb, represses T24 bladder cancer cell growth by regulating anti-apoptotic NF-κB-p65 and AKT signaling, and suppressing IκBα, NF-κB-p65, and AKT phosphorylation. In addition, ursolic acid upregulates pro-apoptotic proteins such as caspase 3, indicating that it could serve as a candidate for the treatment and prevention of bladder cancer (85). Pachymic acid is an antitumor lanostane-type triterpenoid extracted from Poria cocos. Importantly, pachymic acid suppresses the growth of EJ bladder cancer cells by increasing the sub-G1 DNA ratio, with the accumulation of apoptotic bodies and promotion of DNA fragmentation. Molecularly, pachymic acid activates caspase3, caspase8, and caspase9, which in turn, dose-dependently accelerate apoptosis. Furthermore, pachymic acid elevates ROS and decreases the ΔΨm (86). Nimbolide, an active triterpene derived from Azadirachta indica, reportedly has several antitumor activities against bladder cancer. Nimbolide strongly inhibits bladder cancer cell proliferation with an IC50 of 3.0 μM. In bladder cancer cells treated with nimbolide, the G2/M phase cell cycle was arrested via the checkpoint kinase 2 (Chk2)/Cdc25C and Chk2/p21WAF1-related cyclin B1/Wee1 pathway. Moreover, nimbolide elevates JNK phosphorylation while reducing p38 and AKT phosphorylation in EJ and 5637 bladder cancer cells (87).
Iridoids
Iridoids are plant-derived monoterpenes, which usually form iridoid glycosides with sugars. Catalpol is an active iridoid glucoside that is abundantly present in the traditional Chinese medicinal plant Rehmannia glutinosa. Its anticancer activity was recently described by Jin et al. (88) who found that it strongly inhibited the proliferation, migration, and invasion of T24 bladder cancer cells. Moreover, many proteins involved in apoptosis are upregulated by catalpol such as active caspase 3 and PARP. Catalpol also promotes apoptosis by inducing pro-apoptotic protein expression and suppressing Bcl-2 protein expression by modulating the PI3K/AKT pathway (88). Genipin, a natural iridoid derived from Gardenia jasminoides fruit, inhibits the growth of T24 and 5637 bladder cancer cell lines in vitro and in vivo (89). Genipin decreases clonogenic growth and cell viability in a dose-dependent manner, arrests the cell cycle at the G0/G1 phase, and increases the percentage of apoptotic cells, leading to loss of the ΔΨm, promotion of cytochrome C release into the cytosol, and suppression of PI3K and AKT phosphorylation (90).
Alkaloids
Alkaloids are nitrogen-containing organic compounds found in nature, mainly in various plants and some animals. Most alkaloids have a complex ring structure with nitrogen located in the ring structure, which confers important biological activity. Alkaloids are also important bioactive ingredients of many traditional Chinese herbs. Many alkaloids screened from herbs or medicinal plants have shown anticancer and anti-proliferative effects in vivo and in vitro. Some have already been successfully developed as antitumor drugs, such as vinblastine, vinorelbine, vincristine, and vindesine (91). Goonewardene carried out a phase I/II clinical study using vincristine combined with methotrexate and cisplatin. This clinical trial result showed that vincristine combined chemotherapy significantly prolonged the survival period of invasive bladder cancer patients (92). The neoadjuvant cisplatin, methotrexate, and vinblastine (CMV) chemotherapy in muscle-invasive bladder cancer patients was studied in a randomized phase III trial. CMV treatment showed a statistically significant 16% reduction in the risk of death, indicating CMV chemotherapy improves outcome as first-line adjunctive treatment for invasive bladder cancer (93).
The alkaloid oxymatrine has been extracted from the dried roots, plants, and fruits of the leguminous matrine plant using organic solvents such as ethanol. The anti-bladder cancer activity of oxymatrine was recently discovered: Oxymatrine dose-dependently suppresses the proliferation of bladder cancer cells, arrests the cell cycle, and triggers apoptosis through Bax and caspase 3 upregulation and downregulation of p53, Bcl-2, and survivin expression in T24 bladder cancer cells (94).
Dauricine is a diphenyl isoquinoline alkaloid that exists in the rhizome of the Asiatic Moonseed. Dauricine inhibits the proliferation of urinary system cancer cells, including EJ bladder cancer cells and PC-3M prostate cancer cells. The minimum effective concentration was 3.81–5.15 g/mL, and the anticancer effect was concentration-dependent (95).
Capsaicin is an alkaloid found in chili peppers. Capsaicin markedly inhibited cell migration through the suppression of SIRT1 deacetylase via proteasome-mediated protein degradation, thereby elevating β-catenin acetylation and decreasing MMP-9 and MMP-2 activation (96) in T24 bladder cancer cells.
Other studies have shown that capsaicin inhibits bladder cancer cell migration and suppresses cell growth by enhancing apoptosis and inducing cell cycle arrest of TSGH-8301 and T24 bladder cancer cells. Capsaicin represses ERK, FAK, and paxillin phosphorylation, thereby inhibiting cancer cell migration. Moreover, capsaicin reduces the expression of tumor-associated NADH oxidase and SIRT1, thereby inhibiting proliferation, triggering apoptosis, and delaying cell cycle progression (97).
Piperlongumine, a naturally occurring alkaloid extracted from the longer pepper (Piper longum), was recently discovered to have selective anticancer activity. Piperlongumine inhibits bladder cancer cell proliferation, blocking the cell cycle at the G2/M phase and suppressing cell migration. Additionally, piperlongumine drastically increases ROS levels, which are closely related to the inhibition of cell cycle progression of cancer cells. In in vivo experiments, piperlongumine inhibited cancer cells via downregulation of Slug, N-cadherin, ZEB1, and β-catenin (98).
Organic Acids and Esters
Natural organic acids isolated from plants or agricultural byproducts have exhibited certain physiological activities. These acids are widely distributed in the leaves, roots, and fruits of Chinese herbs and fruits, such as black plums, schisandra chinensis and raspberries. Ellagic acid is a polyphenolic organic acid found in green tea, nuts, grapes, pomegranates, and berries, and shows antitumor activity in four different bladder cancer cell lines (UMUC3, 5637, T24, and HT1376). It exerts anti-proliferative activities and enhances the anticancer activity of mitomycin C in bladder cancer therapy. Ellagic acid also suppresses cancer cell invasion and represses chemotaxis, specifically via reduced vascular endothelial growth factor (VEGF)-A-induced VEGF receptor (VEGFR)-2 expression. More interestingly, ellagic acid reduces the expression of programmed cell death 1 ligand 1, an important protein in tumor cell immune escape. In addition, an in vivo study showed the antitumor activity of ellagic acid in human bladder cancer xenografts (99). In another study using a TSGH-8301 bladder cancer cell model, the antitumor activity of ellagic acid was reported to inhibit cell growth, leading to morphological changes, arrest of the cell cycle at the G0/G1 checkpoint, and triggering of apoptosis. Molecularly, ellagic acid enhances Ca2+ levels, elevates ROS, decreases the ΔΨm, and activates caspases including caspase 9 and caspase 3 (100).
Gallic acid is a polyphenol organic acid found in various plants such as rhubarb, dogwood, and eucalyptus. Gallic acid concentration-dependently inhibited T24 bladder cell viability, with IC50 values of 21.73, 18.62, and 11.59 µg/mL following treatment for 24, 48, and 72 h, respectively. In addition, gallic acid induces T24 cell apoptosis associated with ROS accumulation. Gallic acid-induced apoptosis involves ΔΨm depolarization and the caspase 3, Bax, p53, and cytoplasmic cytochrome C levels increase, whereas PI3K, AKT, IκBα, IKKα, and NF-κB p65 phosphorylation decrease. These results indicate that gallic acid is a potential anticancer drug candidate that inhibits cell proliferation, promotes apoptosis, and suppresses the PI3K/AKT/NF-κB signaling axis (101). In addition, the antitumor mechanism of action of gallic acid has been shown to be mediated via the MAPK and PI3K/AKT pathways. Finally, gallic acid inhibits fatty acid synthase and increases estrogen receptor (ER) alpha in TSGH-8301 bladder cancer cells (102).
Aromatic Phytochemicals
Anthrone and Its Derivatives
Gambogic acid, extracted from gamboges, has an anthrone-derived structure, induces ROS, and promotes a dramatic autophagic response via the JNK pathway. Gambogic acid induces ROS-mediated caspases activation, leading to the degradation of autophagic proteins, and causes mitochondrial hyperpolarization and caspase activation, which triggers the intrinsic apoptotic pathway. In addition, gambogic acid suppresses NF-κB activation through ROS-mediated suppression of IκBα phosphorylation in T24 and UMUC3 bladder cancer cell lines (103).
Gartanin, a naturally occurring xanthone, has anticancer activity in various bladder cancer cell lines including HT1376, T24, 5637, TCCSUP, RT4, UMUC3and J82 cells (104). The underlying molecular mechanism of gartanin involves a marked suppression of eukaryotic translation initiation factor 4E binding protein 1 (4EBP1) and p70S6 expression in T24 and RT4 cells. Further, triggering of mTOR pathway-mediated autophagy in T24 and RT4 cells has also been observed. In addition, gartanin downregulates protein expression of the apoptosis inhibitor Bcl-2, whereas it activates the p53 pathway, inducing apoptosis (104).
Cinnamate
3-Phenyl-2-acrylate (cinnamate), is an aromatic organic acid isolated from cinnamon bark or benzoin. Curcumin, a representative cinnamate derivative, widely exists in the rhizomes of some plants of the Zingiberaceae and Araceae families (105). The anticancer activity of curcumin has been widely investigated against various cancers, including that of the bladder, where it regulates numerous intracellular signaling molecules (106). The human trophoblast cell surface antigen 2 (Trop2) is a well-known cancer driver that is dysregulated in many cancers. Zhang et al. (107) found that curcumin strongly inhibits T24 and RT4 bladder cancer cell proliferation by decreasing Trop2 expression and its key downstream molecule cyclin E1. Recently, the anticancer effect of curcumin against bladder cancer stem cells has been reported: Curcumin reduces cell sphere formation by suppressing the expression of breast cancer stem cell markers such as CD133, CD44, Nanog, and octamer-binding transcription factor 4 in UMUC3 and EJ bladder cancer cells. In addition, curcumin inhibits proliferation and triggers apoptosis of bladder cancer cells. Interestingly, curcumin suppresses Sonic Hedgehog pathway activation, which is positively correlated with tumor growth (108). In addition, curcumin enhances the antitumor activity of cisplatin against bladder cancer. The apoptotic cell ratio of T24 and 253J-Bv cells cotreated with curcumin and cisplatin was higher than that of cells incubated with either agent alone. The ROS scavenger N-acetyl-L-cysteine and ERK inhibitor U0126 abolished the combined effect of curcumin and cisplatin. Thus, curcumin might induce apoptosis via a ROS-mediated MEK/ERK-dependent pathway (109). Ferulic acid, another cinnamate-type phytochemical, has also been studied for its activity against bladder cancer. For instance, Peng et al. (110) reported the effects of ferulic acid on T24 bladder cancer cells in two-dimensional (2D) and 3D cell culture systems where they found that it dramatically increased apoptosis, and induced much higher cytotoxicity in 3D than in 2D culture systems. Ferulic acid also upregulates anti-oxidative enzymes such as superoxide dismutase, catalase, and pro-apoptotic proteins such as caspase3, cleaved caspase 9, and Bax (111).
Stilbenes
Piceatannol is a phytochemical, similar to resveratrol, found in grapes, blueberries, passionfruit, and other fruits. Piceatannol inhibits EJ bladder cancer cell proliferation by arresting the cell cycle in the G0/G1 phase. Moreover, piceatannol induces EJ cell apoptosis through a mechanism associated with the increases in protein expression of phosphatase and tensin homolog in concert with a decrease in the phosphorylation of AKT (111).
Quinone Compounds
Anthraquinones
Emodin is an anthraquinone found in dried rhizomes and roots of Polygonum. This compound has anticancer effects against various cell lines, including bladder cancer cells (112). Ma et al. (113) reported that emodin dose-dependently suppresses proliferation and invasion of T24 and 5637 bladder cancer cells. Further, emodin downregulates the mRNA and protein expression of Notch1, Jagged1, VEGF, VEGFR2, and MMP2 (113). Additionally, Notch1 overexpression rescues emodin-induced cell growth suppression, indicating that emodin inhibits the proliferation of bladder cancer cells via the Notch1 pathway (113).
Arbutin, which occurs abundantly in the bearberry plant, is a glycosylated hydroquinone that inhibits the proliferation of TCCSUP bladder cancer cells in a concentration-dependent manner. However, arbutin did not show marked cytotoxicity against TCCSUP cells even at concentrations of 500 µg/mL. Arbutin inactivates the ERK pathway and downregulates cell proliferation. Moreover, arbutin markedly elevates p21/WAF1expression, thereby negatively regulating the cell cycle (114). Juglone is a quinone isolated from the root bark, pericarp, and branch bark of fresh Juglone catalpa and its anticancer activity includes inhibition of proliferation and induction of apoptosis of RT4 and TCCSUP bladder cancer cell lines (115).
Phenanthraquinone
Tanshinone IIA is a lipid-soluble phenanthraquinone compound extracted from Salvia miltiorrhiza Bunge. Tanshinone IIA has been reported to inhibit cell proliferation with an IC50< 5 μg/mL against various bladder cancer cells, including T24, 5637, and BFTC-905 cells. Moreover, tanshinone IIA suppresses cancer cell invasion by decreasing the expression and activity of MMP-9 and MMP-2, and suppresses the expression of C-C motif chemokine ligand 2. In addition, tanshinone IIA inhibits EMT by elevating the epithelial marker E-cadherin and reducing mesenchymal markers such as vimentin and N-cadherin. Tanshinone IIA also decreases transcription regulators such as Slug and Snail in bladder cancer cells (116).
Naphthoquinone
β-Lapachone is a natural naphthoquinone compound extracted from the bark of the lapacho tree that inhibits the viability of T24 bladder cancer cells by inducing apoptosis at the micromolar concentration range. Treatment of T24 cells with β-lapachone downregulated Bcl-2 expression and upregulated that of Bax. β-Lapachone-induced apoptosis is associated with the activation of caspase 9 and caspase 3. Moreover, β-lapachone also inhibits FAS and FASL in a dose-dependent manner in bladder cancer (117).
Based on the above review, phytochemicals exhibited anticancer activities through various mechanisms, including the MAPK, PI3K/AKT, and mTOR, cell cycle checkpoint, and apoptosis regulation signaling pathways (Figure 1). Proteins involved in these signaling pathways are affected by phytochemicals and are interrelated. For example, AKT influences cell proliferation by phosphorylating p21/WAF1 and regulates NF-κB signal transduction by phosphorylating IKKs (118). Caspases are a family of cysteine proteases that are central regulatory proteins of apoptosis (119). FAS is activated by FASL, leading to the activation of downstream caspases (e.g., caspase8 and caspase 9), which in turn, activate downstream molecules (e.g., caspase-3) to perform apoptosis (119). Various phytochemicals, such as nobiletin, tangeretin, genipin, and gallic acid, increase the release of cytochrome C in the mitochondria, triggering mitochondria-dependent apoptosis. The anti-apoptotic proteins Bcl-2 and Bcl-xL are localized in the mitochondrial outer membrane and inhibit the release of cytochrome C (120). Pro-apoptotic proteins, such as Bad, Bax, Bid, and Bim, are localized in the cytoplasm and translocate to the mitochondria after receiving a death signal, and promote the release of cytochrome C. When Bad is transferred to the mitochondria, it forms a pre-apoptotic complex with Bcl-xL, thereby accelerating the release of cytochrome C (120). Following its release, cytochrome C binds to apoptotic peptidase activating factor 1 and forms an apoptotic activation complex with caspase9, thereby accelerating apoptosis (121). The phytochemicals which are able to induce apoptosis have been shown as Figure 2.
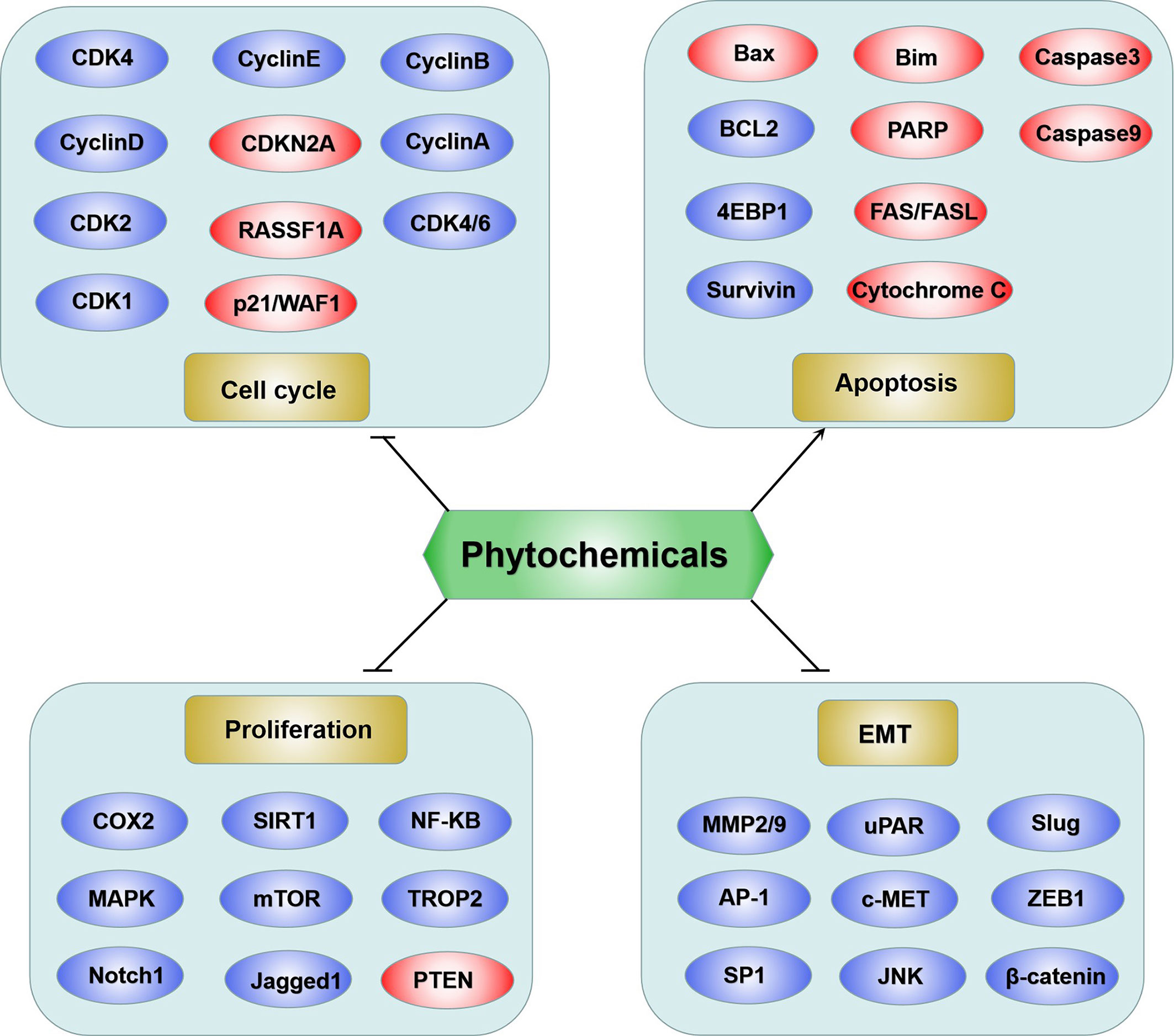
Figure 1 Schematic diagram of phytochemicals targeting molecules on bladder cancer. Generally the anticancer functions were summarized into four groups: suppression of cell cycle, inhibition of proliferation, induction of apoptosis and repression of MET. The red ovals represent upregulated molecules by phytochemicals and the blue ovals represent downregulated molecules by phytochemicals.
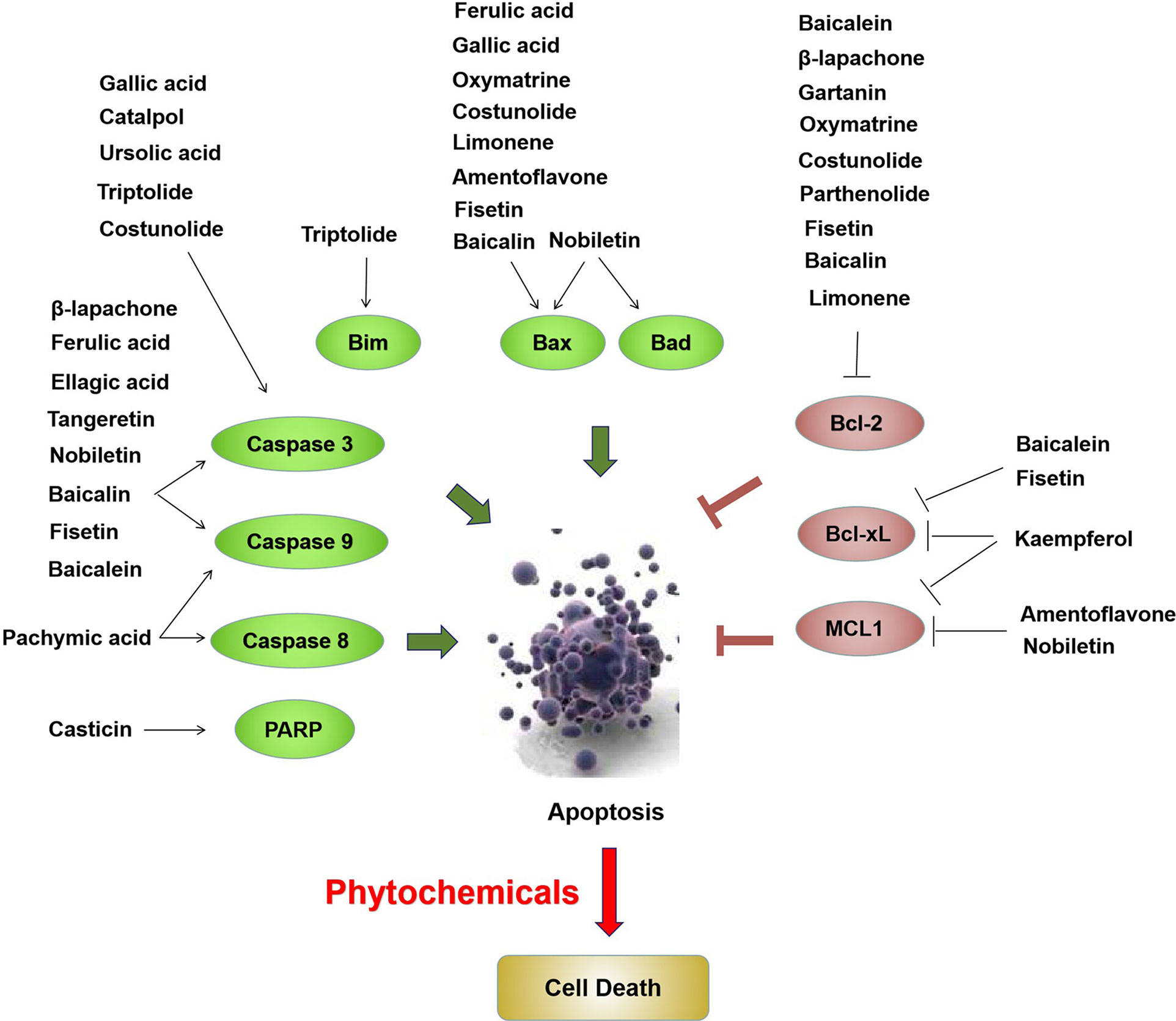
Figure 2 Phytochemicals induce apoptosis through upregulation of Bim, Bax, Bad, PARP, caspase 3, caspase 8 and caspase 9, as well as downregulation of Bcl-2, Bcl-xL and MCL1 in bladder cancer cells. The red ovals represent upregulated molecules by phytochemicals and the green ovals represent downregulated molecules by phytochemicals.
Various phytochemicals, such as tanshinone, silibinin, and piperlongumine, effectively inhibit cell invasion via attenuating MMPs and uPAR (Figure 3) and alleviation of NF-κB. Moreover, EMT is an important mechanism for cell invasion, and the tumor microenvironment and tumor cells secrete cytokines that lead to EMT. These cytokines activate intracellular signal transduction pathways that upregulate specific zinc-finger transcription factors such as Slug, ZEB1, and Snail, thereby enhancing EMT (Figure 3).
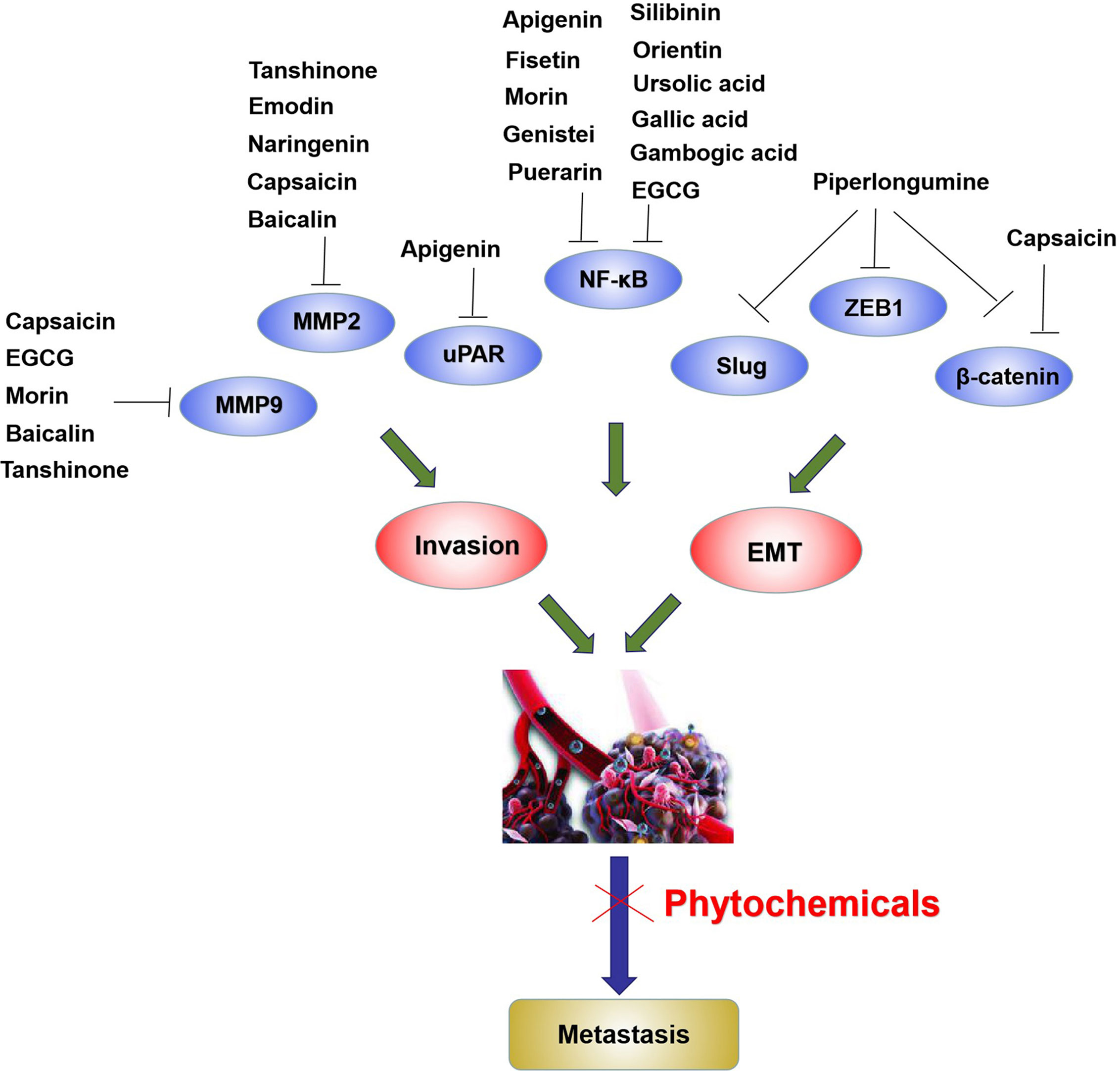
Figure 3 Phytochemicals inhibit metastasis by suppressing MMP2, MMP9, uPAR, Slug, and decreasing transcription factor activities including NF-κB, ZEB1 and β-catenin in bladder cancer cells. The blue ovals represent metastasis related molecules.
MAPKs are a family of protein kinases that are specific to serine, threonine, and tyrosine (122), and play important roles in complicated cellular processes such as embryonic development, cell differentiation, tumorigenesis, and cancer metastasis. Three MAPK families, ERK, JNK and p38 kinase, have been classified in mammalian cells (122), and activation of the ERK and JNK pathways is positively related to tumor cell proliferation (123). Since targeting MAPK has been considered as good anticancer strategy (124), this review summarizes the anticancer activities of numerous phytochemicals, such as arbutin, apigenin, capsaicin, curcumin, kaempferol, morin, naringin, nimbolide, and yuanhuacine, which modulate the MAPK signaling pathway.
The cell cycle is another key process related to cell proliferation and cell cycle related molecules are widely considered as anticancer targets (124). CDK4, CDK6, cyclin D, and cyclin E are essential for the transition of cells from the G1 phase to S phase, whereas p16Ink4A and p21/WAF1 inhibit cell cycle progression (125). During the G2/M transition, cells critically require CDC2 and cyclin B, and p53 is the checkpoint protein mediating this process (125). We summarized the anticancer activities of various phytochemicals, including fisetin, quercetin, genistein, puerarin, naringin, limonene, atractylenolide I, triptolide, nimbolide, capsaicin, piperlongumine, ellagic acid, piceatannol, and arbutin, which attenuate the cell cycle via different mechanisms, mainly by suppressing CDK and cyclin expression and increasing cell cycle inhibitor expression (Figure 4).
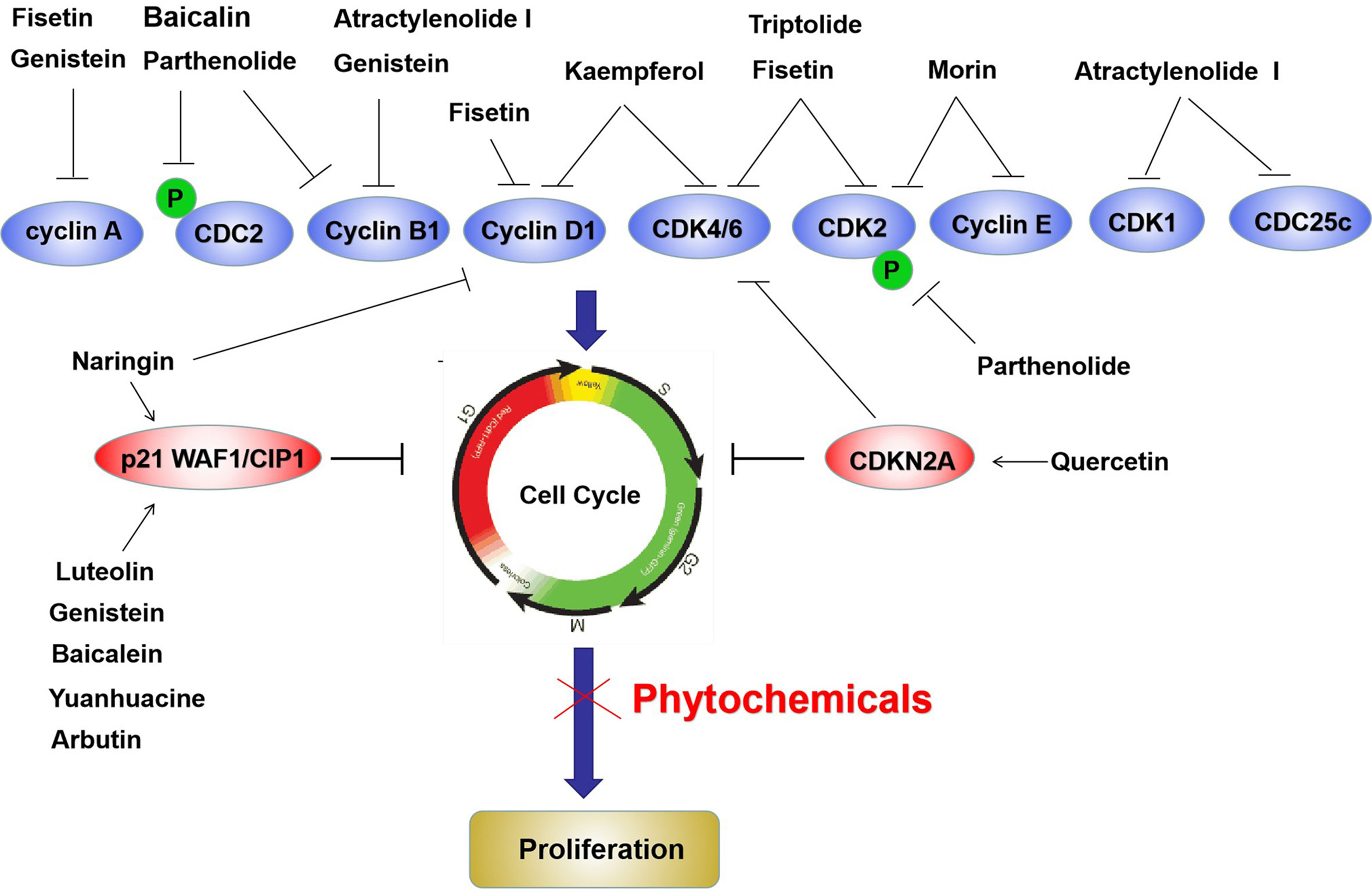
Figure 4 Phytochemicals inhibit bladder cancer cells proliferation by blocking cell cycle via suppressing various cyclins and CDKs, as well as upregulation of p21waf1 and p16-Ink4a in bladder cancer cells. The red ovals represent upregulated molecules by phytochemicals, and the blue ovals represent downregulated molecules by phytochemicals.
Because of various anti-tumor activities of phytochemicals, intake of plant-derived diets to prevent bladder cancer has also been extensively studied. Epidemiological studies showed high fluid intake reduce 49% bladder cancer risk compared with the low intake group, indicating phytochemical might be used for preventing bladder carcinogenesis (126). Moreover, the bladder cancer preventive function of phytochemical monomer was also studied: EGCG intake could reduce the tumor cell proliferation markers, which was consistent with potential chemo-preventive efficacy in bladder cancer patients (43).
Conclusion
In summary, phytochemicals can combat bladder cancer through various mechanisms, such as inhibition of proliferation, migration and invasion; induction of apoptosis; and promotion of autophagy. Table 1 provides an overview of the functions and corresponding molecular mechanisms of different phytochemicals. The use of phytochemicals could be a potentially effective approach for cancer prevention and treatment based on several unique properties. 1) Drug and food homology: many phytochemicals with anticancer activities are derived from vegetables and fruits, and dietary habits are closely linked to cancer. 2) Phytochemicals come from a wide range of sources and can either be extracted from plants or chemically synthesized. 3) Most phytochemicals are metabolized and excreted in the urine and, consequently, are more efficient at reaching the lesion location, the bladder. Overall, this review summarizes natural phytochemicals targeting bladder cancer cell proliferation, migration, and invasion via different signaling pathways in vitro and in vivo. Further, it provides an overview of previous studies on the effects of phytochemicals against bladder cancer, the development of new anticancer drugs, and novel strategies for the treatment of bladder cancer.
Author Contributions
Conceptualization: YX and YJ. Investigation: YX, RC, CL and SL. Figure and table organization: YX and GL. Original draft preparation: YX, RC, GL and SL. Writing and editing: YX and YJ. Manuscript amending: YX, GL and T-WK. Funding acquisition: YX and YJ. All authors contributed to the article and approved the submitted version.
Funding
This study was supported by the Taishan Scholars Program of Shandong Province (No. tsqn201909147), the Research Fund for Academician Lin He New Medicine (No. JYHL2019ZD01), and by a grant (2018R1D1A1B07049918) from the National Research Foundation of Korea (NRF) funded by the Ministry of Education, Science, and Technology.
Conflict of Interest
The authors declare that the research was conducted in the absence of any commercial or financial relationships that could be construed as a potential conflict of interest.
References
1. Seebacher NA, Stacy AE, Porter GM, Merlot AM. Clinical Development of Targeted and Immune Based Anti-Cancer Therapies. J Exp Clin Cancer Res (2019) 38(1):156. doi: 10.1186/s13046-019-1094-2
2. Soflaei SS, Momtazi-Borojeni AA, Majeed M, Derosa G, Maffioli P, Sahebkar A. Curcumin: A Natural Pan-HDAC Inhibitor in Cancer. Curr Pharm Design (2018) 24(2):123–9. doi: 10.2174/1381612823666171114165051
3. Hardin C, Shum E, Singh AP, Perez-Soler R, Cheng H. Emerging Treatment Using Tubulin Inhibitors in Advanced non-Small Cell Lung Cancer. Expert Opin Pharmacother (2017) 18(7):701–16. doi: 10.1080/14656566.2017.1316374
4. Paz-Ares LG, Solsona E, Esteban E, Saez A, Gonzalez-Larriba J, Anton A, et al. Randomized Phase III Trial Comparing Adjuvant Paclitaxel/Gemcitabine/Cisplatin (PGC) to Observation in Patients With Resected Invasive Bladder Cancer: Results of the Spanish Oncology Genitourinary Group (SOGUG) 99/01 Study. J Clin Oncol (2010) 28(18):LBA4518. doi: 10.1200/jco.2010.28.18_suppl.lba4518
5. August KJ, Guest EM, Lewing K, Hays JA, Gamis AS. Treatment of Children With Relapsed and Refractory Acute Lymphoblastic Leukemia With Mitoxantrone, Vincristine, Pegaspargase, Dexamethasone, and Bortezomib. Pediatr Blood Cancer (2020) 67(3):e28062. doi: 10.1002/pbc.28062
6. Zeng F, Ju RJ, Liu L, Xie HJ, Mu LM, Lu WL. Efficacy in Treating Lung Metastasis of Invasive Breast Cancer With Functional Vincristine Plus Dasatinib Liposomes. Pharmacology (2018) 101(1-2):43–53. doi: 10.1159/000480737
7. Yammine A, Zarrouk A, Nury T, Vejux A, Latruffe N, Vervandier-Fasseur D, et al. Prevention by Dietary Polyphenols (Resveratrol, Quercetin, Apigenin) Against 7-Ketocholesterol-Induced Oxiapoptophagy in Neuronal N2a Cells: Potential Interest for the Treatment of Neurodegenerative and Age-Related Diseases. Cells (2020) 9(11):2346. doi: 10.3390/cells9112346
8. Selvam C, Prabu SL, Jordan BC, Purushothaman Y, Umamaheswari A, Hosseini Zare MS, et al. Molecular Mechanisms of Curcumin and its Analogs in Colon Cancer Prevention and Treatment. Life Sci (2019) 239:117032. doi: 10.1016/j.lfs.2019.117032
9. McConkey DJ, Choi W. Molecular Subtypes of Bladder Cancer. Curr Oncol Rep (2018) 20(10):77. doi: 10.1007/s11912-018-0727-5
10. Perez-Vizcaino F, Fraga CG. Research Trends in Flavonoids and Health. Arch Biochem Biophys (2018) 646:107–12. doi: 10.1016/j.abb.2018.03.022
11. George VC, Dellaire G, Rupasinghe HPV. Plant Flavonoids in Cancer Chemoprevention: Role in Genome Stability. J Nutr Biochem (2017) 45:1–14. doi: 10.1016/j.jnutbio.2016.11.007
12. Dinda B, Dinda S, DasSharma S, Banik R, Chakraborty A, Dinda M. Therapeutic Potentials of Baicalin and its Aglycone, Baicalein Against Inflammatory Disorders. Eur J Med Chem (2017) 131:68–80. doi: 10.1016/j.ejmech.2017.03.004
13. Lin CJ, Tsai SC, Tseng MT, Peng SF, Kuo SC, Lin MW, et al. AKT Serine/Threonine Protein Kinase Modulates Baicalin-Triggered Autophagy in Human Bladder Cancer T24 Cells. Int J Oncol (2013) 42(3):993–1000. doi: 10.3892/ijo.2013.1791
14. Li HL, Zhang S, Wang Y, Liang RR, Li J, An P, et al. Baicalein Induces Apoptosis Via a Mitochondrial-Dependent Caspase Activation Pathway in T24 Bladder Cancer Cells. Mol Med Rep (2013) 7(1):266–70. doi: 10.3892/mmr.2012.1123
15. Chao JI, Su WC, Liu HF. Baicalein Induces Cancer Cell Death and Proliferation Retardation by the Inhibition of CDC2 Kinase and Survivin Associated With Opposite Role of p38 Mitogen-Activated Protein Kinase and AKT. Mol Cancer Ther (2007) 6(11):3039–48. doi: 10.1158/1535-7163.MCT-07-0281
16. Wu JY, Tsai KW, Li YZ, Chang YS, Lai YC, Laio YH, et al. Anti-Bladder-Tumor Effect of Baicalein From Scutellaria Baicalensis Georgi and Its Application In Vivo. Evidence-Based Complementary Altern Med (2013) 2013:579751. doi: 10.1155/2013/579751
17. Xia Y, Yuan MM, Li SA, Thuan UT, Nguyen TT, Kang TW, et al. Apigenin Suppresses the IL-1 Beta-Induced Expression of the Urokinase Type Plasminogen Activator Receptor by Inhibiting Mapk-Mediated AP-1 and NF-Kappa B Signaling in Human Bladder Cancer T24 Cells. J Agric Food Chem (2018) 66(29):7663–73. doi: 10.1021/acs.jafc.8b02351
18. Zhu Y, Mao YQ, Chen H, Lin YW, Hu ZH, Wu J, et al. Apigenin Promotes Apoptosis, Inhibits Invasion and Induces Cell Cycle Arrest of T24 Human Bladder Cancer Cells. Cancer Cell Int (2013) 13(1):54. doi: 10.1186/1475-2867-13-54
19. Shi MD, Shiao CK, Lee YC, Shih YW. Apigenin, a Dietary Flavonoid, Inhibits Proliferation of Human Bladder Cancer T-24 Cells Via Blocking Cell Cycle Progression and Inducing Apoptosis. Cancer Cell Int (2015) 15:33. doi: 10.1186/s12935-015-0186-0
20. Iida K, Naiki T, Naiki-Ito A, Suzuki S, Kato H, Nozaki S, et al. Luteolin Suppresses Bladder Cancer Growth Via Regulation of Mechanistic Target of Rapamycin Pathway. Cancer Sci (2020) 111(4):1165–79. doi: 10.1111/cas.14334
21. Goan YG, Wu WT, Liu CI, Neoh CA, Wu YJ. Involvement of Mitochondrial Dysfunction, Endoplasmic Reticulum Stress, and the PI3K/AKT/mTOR Pathway in Nobiletin-Induced Apoptosis of Human Bladder Cancer Cells. Molecules (2019) 24(16):2881. doi: 10.3390/molecules24162881
22. Lin JJ, Huang CC, Su YL, Luo HL, Lee NL, Sung MT, et al. Proteomics Analysis of Tangeretin-Induced Apoptosis Through Mitochondrial Dysfunction in Bladder Cancer Cells. Int J Mol Sci (2019) 20(5):1017. doi: 10.3390/ijms20051017
23. Butun B, Topcu G, Ozturk T. Recent Advances on 3-Hydroxyflavone Derivatives: Structures and Properties. Mini Rev Med Chem (2018) 18(2):98–103. doi: 10.2174/1389557517666170425102827
24. Huang AC, Cheng YD, Huang LH, Hsiao YT, Peng SF, Lu KW, et al. Casticin Induces Dna Damage and Impairs Dna Repair in Human Bladder Cancer Tsgh-8301 Cells. Anticancer Res (2019) 39(4):1839–47. doi: 10.21873/anticanres.13291
25. Chung YH, Kim D. RIP Kinase-Mediated ROS Production Triggers XAF1 Expression Through Activation of TAp73 in Casticin-Treated Bladder Cancer Cells. Oncol Rep (2016) 36(2):1135–42. doi: 10.3892/or.2016.4895
26. Dang Q, Song WB, Xu DF, Ma YM, Li F, Zeng J, et al. Kaempferol Suppresses Bladder Cancer Tumor Growth by Inhibiting Cell Proliferation and Inducing Apoptosis. Mol Carcinogen (2015) 54(9):831–40. doi: 10.1002/mc.22154
27. Wu P, Meng XF, Zheng HD, Zeng Q, Chen TF, Wang W, et al. Kaempferol Attenuates Ros-Induced Hemolysis and the Molecular Mechanism of Its Induction of Apoptosis on Bladder Cancer. Molecules (2018) 23(10):2592. doi: 10.3390/molecules23102592
28. Li J, Cheng YY, Qu WX, Sun Y, Wang ZP, Wang HZ, et al. Fisetin, a Dietary Flavonoid, Induces Cell Cycle Arrest and Apoptosis Through Activation of p53 and Inhibition of NF-Kappa B Pathways in Bladder Cancer Cells. Basic Clin Pharmacol Toxicol (2011) 108(2):84–93. doi: 10.1111/j.1742-7843.2010.00613.x
29. Shin SS, Won SY, Noh DH, Hwang B, Kim WJ, Moon SK. Morin Inhibits Proliferation, Migration, and Invasion of Bladder Cancer EJ Cells Via Modulation of Signaling Pathways, Cell Cycle Regulators, and Transcription Factor-Mediated MMP-9 Expression. Drug Dev Res (2017) 78(2):81–90. doi: 10.1002/ddr.21377
30. Ma L, Feugang JM, Konarski P, Wang J, Lu JZ, Fu SJ, et al. Growth Inhibitory Effects of Quercetin on Bladder Cancer Cell. Front Biosci-Landmark (2006) 11:2275–85. doi: 10.2741/1970
31. Su QL, Peng M, Zhang YQ, Xu WJ, Darko KO, Tao T, et al. Quercetin Induces Bladder Cancer Cells Apoptosis by Activation of AMPK Signaling Pathway. Am J Cancer Res (2016) 6(2):498–508.
32. Krizova L, Dadakova K, Kasparovska J, Kasparovsky T. Isoflavones. Molecules (2019) 24(6):1076. doi: 10.3390/molecules24061076
33. Mazumder MAR, Hongsprabhas P. Genistein as Antioxidant and Antibrowning Agents in In Vivo and In Vitro: A Review. Biomed Pharmacother (2016) 82:379–92. doi: 10.1016/j.biopha.2016.05.023
34. Park C, Cha HJ, Lee H, Hwang-Bo H, Ji SY, Kim MY, et al. Induction of G2/M Cell Cycle Arrest and Apoptosis by Genistein in Human Bladder Cancer T24 Cells Through Inhibition of the ROS-Dependent Pi3k/Akt Signal Transduction Pathway. Antioxidants (2019) 8(9):327. doi: 10.3390/antiox8090327
35. Wang Y, Wang H, Zhang W, Shao C, Xu P, Shi CH, et al. Genistein Sensitizes Bladder Cancer Cells to HCPT Treatment In Vitro and In Vivo Via ATM/NF-kappa B/Ikk Pathway-Induced Apoptosis. PloS One (2013) 8(1):e50175. doi: 10.1371/journal.pone.0050175
36. Ye GM, Kan SF, Chen JF, Lu X. Puerarin in Inducing Apoptosis of Bladder Cancer Cells Through Inhibiting SIRT1/p53 Pathway. Oncol Lett (2019) 17(1):195–200. doi: 10.3892/ol.2018.9600
37. Jiang KH, Chen HB, Tang K, Guan W, Zhou H, Guo XL, et al. Puerarin Inhibits Bladder Cancer Cell Proliferation Through the mTOR/p70S6K Signaling Pathway. Oncol Lett (2018) 15(1):167–74. doi: 10.3892/ol.2017.7298
38. Liu XY, Li SG, Li YY, Cheng B, Tan B, Wang G. Puerarin Inhibits Proliferation and Induces Apoptosis by Upregulation of miR-16 in Bladder Cancer Cell Line T24. Oncol Res (2018) 26(8):1227–34. doi: 10.3727/096504018x15178736525106
39. Gan RY, Li HB, Sui ZQ, Corke H. Absorption, Metabolism, Anti-Cancer Effect and Molecular Targets of Epigallocatechin Gallate (EGCG): An Updated Review. Crit Rev Food Sci Nutr (2018) 58(6):924–41. doi: 10.1080/10408398.2016.1231168
40. Feng CC, Ho Y, Sun CY, Xia GW, Ding Q, Gu B. Epigallocatechin Gallate Inhibits the Growth and Promotes the Apoptosis of Bladder Cancer Cells. Exp Ther Med (2017) 14(4):3513–8. doi: 10.3892/etm.2017.4981
41. Luo KW, Chen W, Lung WY, Wei XY, Cheng BH, Cai ZM, et al. EGCG Inhibited Bladder Cancer SW780 Cell Proliferation and Migration Both In Vitro and In Vivo Via Down-Regulation of NF-Kappa B and MMP-9. J Nutr Biochem (2017) 41:56–64. doi: 10.1016/j.jnutbio.2016.12.004
42. Lee HY, Chen YJ, Chang WA, Li WM, Ke HL, Wu WJ, et al. Effects of Epigallocatechin Gallate (EGCG) on Urinary Bladder Urothelial Carcinoma? Next-Generation Sequencing and Bioinformatics Approaches. Medicina-Lithuania (2019) 55(12):768. doi: 10.3390/medicina55120768
43. Gee JR, Saltzstein DR, Kim K, Kolesar J, Huang W, Havighurst TC, et al. A Phase Ii Randomized, Double-Blind, Presurgical Trial of Polyphenon E in Bladder Cancer Patients to Evaluate Pharmacodynamics and Bladder Tissue Biomarkers. Cancer Prev Res (2017) 10(5):298–307. doi: 10.1158/1940-6207
44. Li F, Sun Y, Jia J, Yang C, Tang XS, Jin B, et al. Silibinin Attenuates TGF-beta 1-Induced Migration and Invasion Via EMT Suppression and is Associated With COX-2 Downregulation in Bladder Transitional Cell Carcinoma. Oncol Rep (2018) 40(6):3543–50. doi: 10.3892/or.2018.6728
45. Mc Cormick BP, Langle Y, Belgorosky D, Vanzulli S, Balarino N, Sandes E, et al. Flavonoid Silybin Improves the Response to Radiotherapy in Invasive Bladder Cancer. J Cell Biochem (2018) 119(7):5402–12. doi: 10.1002/jcb.26693
46. Gandara L, Sandes E, Di Venosa G, Mc Cormick BP, Rodriguez L, Mamone L, et al. The Natural Flavonoid Silybin Improves the Response to Photodynamic Therapy of Bladder Cancer Cells. J Photochem Photobiol B-Biol (2014) 133:55–64. doi: 10.1016/j.jphotobiol.2014.03.006
47. Sun Y, Guan ZF, Zhao WC, Jiang YZ, Li Q, Cheng YY, et al. Silibinin Suppresses Bladder Cancer Cell Malignancy and Chemoresistance in an NF-Kappa B Signal-Dependent and Signal-Independent Manner. Int J Oncol (2017) 51(4):1219–26. doi: 10.3892/ijo.2017.4089
48. Mir IA, Tiku AB. Chemopreventive and Therapeutic Potential of “Naringenin,” a Flavanone Present in Citrus Fruits. Nutr Cancer-an Int J (2015) 67(1):27–42. doi: 10.1080/01635581.2015.976320
49. Chen R, Qi QL, Wang MT, Li QY. Therapeutic Potential of Naringin: An Overview. Pharm Biol (2016) 54(12):3203–10. doi: 10.1080/13880209.2016.1216131
50. Liao ACH, Kuo CC, Huang YC, Yeh CW, Hseu YC, Liu JY, et al. Naringenin Inhibits Migration of Bladder Cancer Cells Through Downregulation of AKT and MMP-2. Mol Med Rep (2014) 10(3):1531–6. doi: 10.3892/mmr.2014.2375
51. Kim DI, Lee SJ, Lee SB, Park K, Kim WJ, Moon SK. Requirement for Ras/Raf/ERK Pathway in Naringin-Induced G(1)-cell-cycle Arrest Via p21WAF1 Expression. Carcinogenesis (2008) 29(9):1701–9. doi: 10.1093/carcin/bgn055
52. Liu H, Dong YH, Gao YT, Du ZP, Wang YT, Cheng P, et al. The Fascinating Effects of Baicalein on Cancer: A Review. Int J Mol Sci (2016) 17(10):1681. doi: 10.3390/ijms17101681
53. Yang Y, Liu K, Yang LB, Zhang GY. Bladder Cancer Cell Viability Inhibition and Apoptosis Induction by Baicalein Through Targeting the Expression of Anti-Apoptotic Genes. Saudi J Biol Sci (2018) 25(7):1478–82. doi: 10.1016/j.sjbs.2017.03.014
54. Jiang LZ, Song HB, Guo HB, Wang C, Lu ZP. Baicalein Inhibits Proliferation and Migration of Bladder Cancer Cell Line T24 by Down-Regulation of Microrna-106. Biomed Pharmacother (2018) 107:1583–90. doi: 10.1016/j.biopha.2018.08.107
55. Choi EO, Park C, Hwang HJ, Hong SH, Kim GY, Cho EJ, et al. Baicalein Induces Apoptosis Via ROS-dependent Activation of Caspases in Human Bladder Cancer 5637 Cells. Int J Oncol (2016) 49(3):1009–18. doi: 10.3892/ijo.2016.3606
56. Choi EM, Suh KS, Park SY, Yun S, Chin SO, Rhee SY, et al. Orientin Reduces the Inhibitory Effects of 2,3,7,8-Tetrachlorodibenzo-P-Dioxin on Adipogenic Differentiation and Insulin Signaling Pathway in Murine 3T3-L1 Adipocytes. Chem-Biol Interact (2020) 318:108978. doi: 10.1016/j.cbi.2020.108978
57. Tian FH, Tong M, Li ZZ, Huang WC, Jin YY, Cao QF, et al. The Effects of Orientin on Proliferation and Apoptosis of T24 Human Bladder Carcinoma Cells Occurs Through the Inhibition of Nuclear Factor-Kappab and the Hedgehog Signaling Pathway. Med Sci Monitor (2019) 25:9547–54. doi: 10.12659/Msm.919203
58. Stockwell BR, Angeli JPF, Bayir H, Bush AI, Conrad M, Dixon SJ, et al. Ferroptosis: A Regulated Cell Death Nexus Linking Metabolism, Redox Biology, and Disease. Cell (2017) 171(2):273–85. doi: 10.1016/j.cell.2017.09.021
59. Lou JS, Bi WC, Chan GKL, Jin Y, Wong CW, Zhou ZY, et al. Ginkgetin Induces Autophagic Cell Death Through p62/SQSTM1-mediated Autolysosome Formation and Redox Setting in non-Small Cell Lung Cancer. Oncotarget (2017) 8(54):93131–48. doi: 10.18632/oncotarget.21862
60. Jeon YJ, Jung SN, Yun J, Lee CW, Choi J, Lee YJ, et al. Ginkgetin Inhibits the Growth of DU-145 Prostate Cancer Cells Through Inhibition of Signal Transducer and Activator of Transcription 3 Activity. Cancer Sci (2015) 106(4):413–20. doi: 10.1111/cas.12608
61. Chiang CH, Yeh CY, Chung JG, Chiang IT, Hsu FT. Amentoflavone Induces Apoptosis and Reduces Expression of Anti-apoptotic and Metastasis-associated Proteins in Bladder Cancer. Anticancer Res (2019) 39(7):3641–9. doi: 10.21873/anticanres.13512
62. Moreau RA, Nystrom L, Whitaker BD, Winkler-Moser JK, Baer DJ, Gebauer SK, et al. Phytosterols and Their Derivatives: Structural Diversity, Distribution, Metabolism, Analysis, and Health-Promoting Uses. Prog Lipid Res (2018) 70:35–61. doi: 10.1016/j.plipres.2018.04.001
63. Jaramillo-Madrid AC, Ashworth J, Fabris M, Ralph PJ. Phytosterol Biosynthesis and Production by Diatoms (Bacillariophyceae). Phytochemistry (2019) 163:46–57. doi: 10.1016/j.phytochem.2019.03.018
64. Huang J, Xu M, Fang YJ, Lu MS, Pan ZZ, Huang WQ, et al. Association Between Phytosterol Intake and Colorectal Cancer Risk: A Case-Control Study. Br J Nutr (2017) 117(6):839–50. doi: 10.1017/S0007114517000617
65. Ramprasath VR, Awad AB. Role of Phytosterols in Cancer Prevention and Treatment. J Aoac Int (2015) 98(3):735–8. doi: 10.5740/jaoacint.SGERamprasath
66. Zhou Q, Song W, Xiao W. Dioscin Induces Demethylation of DAPK-1 and RASSF-1alpha Genes Via the Antioxidant Capacity, Resulting in Apoptosis of Bladder Cancer T24 Cells. Excli J (2017) 16:101–12. doi: 10.17179/excli2016-571
67. Miranda MA, Silva LB, Carvalho IPS, Amaral R, de Paula MH, Swiech K, et al. Targeted Uptake of Folic Acid-Functionalized Polymeric Nanoparticles Loading Glycoalkaloidic Extract In Vitro and In Vivo Assays. Colloids Surf B Biointerfaces (2020) 192:111106. doi: 10.1016/j.colsurfb.2020.111106
68. Jin MM, Shi CZ, Li T, Wu Y, Hu C, Huang G. Solasonine Promotes Ferroptosis of Hepatoma Carcinoma Cells Via Glutathione Peroxidase 4-Induced Destruction of the Glutathione Redox System. Biomed Pharmacother (2020) 129:110282. doi: 10.1016/j.biopha.2020.110282
69. Wojtunik-Kulesza KA, Kasprzak K, Oniszczuk T, Oniszczuk A. Natural Monoterpenes: Much More Than Only a Scent. Chem Biodiversity (2019) 16(12):e1900434. doi: 10.1002/cbdv.201900434
70. Ye ZH, Liang ZL, Mi QW, Guo YL. Limonene Terpenoid Obstructs Human Bladder Cancer Cell (T24 Cell Line) Growth by Inducing Cellular Apoptosis, Caspase Activation, G2/M Phase Cell Cycle Arrest and Stops Cancer Metastasis. J Buon (2020) 25(1):280–5.
71. Cherng JM, Shieh DE, Chiang W, Chang MY, Chiang LC. Chemopreventive Effects of Minor Dietary Constituents in Common Foods on Human Cancer Cells. Biosci Biotechnol Biochem (2007) 71(6):1500–4. doi: 10.1271/bbb.70008
72. Ding J, Wang JJ, Huang C, Wang L, Deng SN, Xu TL, et al. Curcumol From Rhizoma Curcumae Suppresses Epileptic Seizure by Facilitation of GABA (a) Receptors. Neuropharmacology (2014) 81:244–55. doi: 10.1016/j.neuropharm.2014.02.009
73. Zhou L, Wei ES, Zhou B, Bi GW, Gao L, Zhang TY, et al. Anti-Proliferative Benefit of Curcumol on Human Bladder Cancer Cells Via Inactivating EZH2 Effector. Biomed Pharmacother (2018) 104:798–805. doi: 10.1016/j.biopha.2018.05.101
74. Yu R, Yu BX, Chen JF, Lv XY, Yan ZJ, Cheng Y, et al. Anti-Tumor Effects of Atractylenolide I on Bladder Cancer Cells. J Exp Clin Cancer Res (2016) 35:40. doi: 10.1186/s13046-016-0312-4
75. Cheng G, Xie LP. Parthenolide Induces Apoptosis and Cell Cycle Arrest of Human 5637 Bladder Cancer Cells In Vitro. Molecules (2011) 16(8):6758–68. doi: 10.3390/molecules16086758
76. Rasul A, Bao R, Malhi M, Zhao B, Tsuji I, Li J, et al. Induction of Apoptosis by Costunolide in Bladder Cancer Cells is Mediated Through ROS Generation and Mitochondrial Dysfunction. Molecules (2013) 18(2):1418–33. doi: 10.3390/molecules18021418
77. Zhang RW, Wang YL, Li JX, Jin HL, Song SJ, Huang CS. The Chinese Herb Isolate Yuanhuacine (Yhl-14) Induces G(2)/M Arrest in Human Cancer Cells by Up-regulating P21 Protein Expression Through an P53 Protein-independent Cascade. J Biol Chem (2014) 289(10):6394–403. doi: 10.1074/jbc.M113.513960
78. Noel P, Von Hoff DD, Saluja AK, Velagapudi M, Borazanci E, Han HY. Triptolide and Its Derivatives as Cancer Therapies. Trends Pharmacol Sci (2019) 40(5):327–41. doi: 10.1016/j.tips.2019.03.002
79. Yang LL, Wang XY, Zheng LY, Fang SJ, Xu M, Zhao ZW, et al. The Role of FOXO3a-Bim Signaling in Triptolide Induced Bladder Cancer T24 Cells Apoptosis. Zhonghua Yi Xue Za Zhi (2017) 97(15):1187–90. doi: 10.3760/cma.j.issn.0376-2491.2017.15.016
80. Yang Y, Zhang LJ, Bai XG, Xu HJ, Jin ZL, Ding M. Synergistic Antitumour Effects of Triptolide Plus Gemcitabine in Bladder Cancer. Biomed Pharmacother (2018) 106:1307–16. doi: 10.1016/j.biopha.2018.07.083
81. Wang TAN, Ding Y, Yang YF, Wang ZY, Gao WS, Li DS, et al. Synergistic Antitumour Effects of Triptolide Plus 10-Hydroxycamptothecin on Bladder Cancer. Biomed Pharmacother (2019) 115:108899. doi: 10.1016/j.biopha.2019.108899
82. Jiang JH, Pi J, Jin H, Cai JY. Oridonin-Induced Mitochondria-Dependent Apoptosis in Esophageal Cancer Cells by Inhibiting PI3K/AKT/mTOR and Ras/Raf Pathways. J Cell Biochem (2019) 120(3):3736–46. doi: 10.1002/jcb.27654
83. Iwamoto H, Izumi K, Natsagdorj A, Naito R, Makino T, Kadomoto S, et al. Coffee Diterpenes Kahweol Acetate and Cafestol Synergistically Inhibit the Proliferation and Migration of Prostate Cancer Cells. Prostate (2019) 79(5):468–79. doi: 10.1002/pros.23753
84. Gao X, Han H. Jolkinolide B Inhibits Glycolysis by Downregulating Hexokinase 2 Expression Through Inactivating the Akt/mTOR Pathway in non-Small Cell Lung Cancer Cells. J Cell Biochem (2018) 119(6):4967–74. doi: 10.1002/jcb.26742
85. Gai L, Cai N, Wang LL, Xu XY, Kong XB. Ursolic Acid Induces Apoptosis Via Akt/NF-Kappa B Signaling Suppression in T24 Human Bladder Cancer Cells. Mol Med Rep (2013) 7(5):1673–7. doi: 10.3892/mmr.2013.1364
86. Jeong JW, Lee WS, Go SI, Nagappan A, Baek JY, Lee JD, et al. Pachymic Acid Induces Apoptosis of EJ Bladder Cancer Cells by DR5 Up-Regulation, ROS Generation, Modulation of Bcl-2 and IAP Family Members. Phytother Res (2015) 29(10):1516–24. doi: 10.1002/ptr.5402
87. Shin SS, Hwang B, Muhammad K, Gho Y, Song JH, Kim WJ, et al. Nimbolide Represses the Proliferation, Migration, and Invasion of Bladder Carcinoma Cells Via Chk2-Mediated G2/M Phase Cell Cycle Arrest, Altered Signaling Pathways, and Reduced Transcription Factors-Associated Mmp-9 Expression. Evidence-Based Complementary Altern Med (2019) 2019:3753587. doi: 10.1155/2019/3753587
88. Jin D, Cao M, Mu X, Yang GL, Xue W, Huang YR, et al. Catalpol Inhibited the Proliferation of T24 Human Bladder Cancer Cells by Inducing Apoptosis Through the Blockade of Akt-Mediated Anti-Apoptotic Signaling. Cell Biochem Biophysics (2015) 71(3):1349–56. doi: 10.1007/s12013-014-0355-0
89. Mahgoub E, Kumaraswamy SM, Kader KH, Venkataraman B, Ojha S, Adeghate E, et al. Genipin Attenuates Cisplatin-Induced Nephrotoxicity by Counteracting Oxidative Stress, Inflammation, and Apoptosis. Biomed Pharmacother (2017) 93:1083–97. doi: 10.1016/j.biopha.2017.07.018
90. Li Z, Zhang TB, Jia DH, Sun WQ, Wang CL, Gu AZ, et al. Genipin Inhibits the Growth of Human Bladder Cancer Cells Via Inactivation of PI3K/Akt Signaling. Oncol Lett (2018) 15(2):2619–24. doi: 10.3892/ol.2017.7588
91. Mondal A, Gandhi A, Fimognari C, Atanasov AG, Bishayee A. Alkaloids for Cancer Prevention and Therapy: Current Progress and Future Perspectives. Eur J Pharmacol (2019) 858:172472. doi: 10.1016/j.ejphar.2019.172472
92. Goonewardene TI, Bozcuk H, Oliver RTD, Barua J, Nargund V, Philip T, et al. Phase 1/2 Study of Synchronous Methotrexate, Cisplatin, Vincristine (Mopq10) Chemotherapy and Radiation for Patients With Locally Advanced Bladder Cancer. Urol Internationalis (2001) 67(4):293–7. doi: 10.1159/000051006
93. Griffiths G, M. R. C. A. B. Canc, N. B. C. S. Grp, C. U. E. Tratamiento. International Phase Iii Trial Assessing Neoadjuvant Cisplatin, Methotrexate, and Vinblastine Chemotherapy for Muscle-Invasive Bladder Cancer: Long-Term Results of the BA06 30894 Trial. J Clin Oncol (2011) 29(16):2171–7. doi: 10.1200/Jco.2010.32.3139
94. Li S, Zhang Y, Liu QY, Zhao QL, Xu LY, Huang SL, et al. Oxymatrine Inhibits Proliferation of Human Bladder Cancer T24 Cells by Inducing Apoptosis and Cell Cycle Arrest. Oncol Lett (2017) 13(6):4453–8. doi: 10.3892/ol.2017.6013
95. Wang J, Li Y, Zu XB, Chen MF, Qi L. Dauricine can Inhibit the Activity of Proliferation of Urinary Tract Tumor Cells. Asian Pacific J Trop Med (2012) 5(12):973–6. doi: 10.1016/S1995-7645(12)60185-0
96. Islam A, Yang YT, Wu WH, Chueh PJ, Lin MH. Capsaicin Attenuates Cell Migration Via SIRT1 Targeting and Inhibition to Enhance Cortactin and Beta-Catenin Acetylation in Bladder Cancer Cells. Am J Cancer Res (2019) 9(6):1172–82.
97. Lin MH, Lee YH, Cheng HL, Chen HY, Jhuang FH, Chueh PJ. Capsaicin Inhibits Multiple Bladder Cancer Cell Phenotypes by Inhibiting Tumor-Associated NADH Oxidase (tNOX) and Sirtuin1 (Sirt1). Molecules (2016) 21(7):849. doi: 10.3390/molecules21070849
98. Liu D, Qiu XY, Wu X, Hu DX, Li CY, Yu SB, et al. Piperlongumine Suppresses Bladder Cancer Invasion Via Inhibiting Epithelial Mesenchymal Transition and F-actin Reorganization. Biochem Biophys Res Commun (2017) 494(1-2):165–72. doi: 10.1016/j.bbrc.2017.10.061
99. Ceci C, Tentori L, Atzori MG, Lacal PM, Bonanno E, Scimeca M, et al. Ellagic Acid Inhibits Bladder Cancer Invasiveness and In Vivo Tumor Growth. Nutrients (2016) 8(11):744. doi: 10.3390/nu8110744
100. Ho CC, Huang AC, Yu CS, Lien JC, Wu SH, Huang YP, et al. Ellagic Acid Induces Apoptosis in TSGH8301 Human Bladder Cancer Cells Through the Endoplasmic Reticulum Stress- and Mitochondria-Dependent Signaling Pathways. Environ Toxicol (2014) 29(11):1262–74. doi: 10.1002/tox.21857
101. Zeng ML, Su Y, Li KY, Jin D, Li QL, Li Y, et al. Gallic Acid Inhibits Bladder Cancer T24 Cell Progression Through Mitochondrial Dysfunction and PI3K/Akt/NF-Kappa B Signaling Suppression. Front Pharmacol (2020) 11:1222. doi: 10.3389/fphar.2020.01222
102. Liao CC, Chen SC, Huang HP, Wang CJ. Gallic Acid Inhibits Bladder Cancer Cell Proliferation and Migration Via Regulating Fatty Acid Synthase (FAS). J Food Drug Anal (2018) 26(2):620–7. doi: 10.1016/j.jfda.2017.06.006
103. Ishaq M, Khan MA, Sharma K, Sharma G, Dutta RK, Majumdar S. Gambogic Acid Induced Oxidative Stress Dependent Caspase Activation Regulates Both Apoptosis and Autophagy by Targeting Various Key Molecules (NF-Kappa B, Beclin-1, p62 and NBR1) in Human Bladder Cancer Cells. Biochim Et Biophys Acta-General Subj (2014) 1840(12):3374–84. doi: 10.1016/j.bbagen.2014.08.019
104. Liu ZB, Antalek M, Nguyen L, Li XS, Tian XJ, Le A, et al. The Effect of Gartanin, a Naturally Occurring Xanthone in Mangosteen Juice, on the Mtor Pathway, Autophagy, Apoptosis, and the Growth of Human Urinary Bladder Cancer Cell Lines. Nutr Cancer-an Int J (2013) 65:68–77. doi: 10.1080/01635581.2013.785011
105. Tsuda T. Curcumin as a Functional Food-Derived Factor: Degradation Products, Metabolites, Bioactivity, and Future Perspectives. Food Funct (2018) 9(2):705–14. doi: 10.1039/c7fo01242j
106. Imran M, Ullah A, Saeed F, Nadeem M, Arshad MU, Suleria HAR. Cucurmin, Anticancer, & Antitumor Perspectives: A Comprehensive Review. Crit Rev Food Sci Nutr (2018) 58(8):1271–93. doi: 10.1080/10408398.2016.1252711
107. Zhang LH, Yang GL, Zhang RY, Dong L, Chen HG, Bo JJ, et al. Curcumin Inhibits Cell Proliferation and Motility Via Suppression of TROP2 in Bladder Cancer Cells. Int J Oncol (2018) 53(2):515–26. doi: 10.3892/ijo.2018.4423
108. Wang DD, Kong XC, Li Y, Qian WW, Ma JX, Wang DM, et al. Curcumin Inhibits Bladder Cancer Stem Cells by Suppressing Sonic Hedgehog Pathway. Biochem Biophys Res Commun (2017) 493(1):521–7. doi: 10.1016/j.bbrc.2017.08.158
109. Park BH, Lim JE, Jeon HG, Seo SI, Lee HM, Choi HY, et al. Curcumin Potentiates Antitumor Activity of Cisplatin in Bladder Cancer Cell Lines Via ROS-mediated Activation of ERK1/2. Oncotarget (2016) 7(39):63870–86. doi: 10.18632/oncotarget.11563
110. Peng CC, Chyau CC, Wang HE, Chang CH, Chen KC, Chou KY, et al. Cytotoxicity of Ferulic Acid on T24 Cell Line Differentiated by Different Microenvironments. BioMed Res Int (2013) 2013:579859. doi: 10.1155/2013/579859
111. Li XG, Zhang WS, Yan XP. Piceatannol Inhibits Proliferation and Induces Apoptosis of Bladder Cancer Cells Through Regulation of the PTEN/AKT Signal Pathway. Cell Mol Biol (2020) 66(3):181–4. doi: 10.14715/cmb/2020.66.3.29
112. Jelassi B, Anchelin M, Chamouton J, Cayuela ML, Clarysse L, Li JY, et al. Anthraquinone Emodin Inhibits Human Cancer Cell Invasiveness by Antagonizing P2X7 Receptors. Carcinogenesis (2013) 34(7):1487–96. doi: 10.1093/carcin/bgt099
113. Ma LB, Chen K, Jiang K, Deng G, Jiang PW, Shao J, et al. Emodin Inhibits the Proliferation and Invasion of Bladder Cancer Cells Via Down-Regulating Notch1. Int J Clin Exp Pathol (2017) 10(9):9452–9.
114. Li H, Jeong YM, Kim SY, Kim MK, Kim DS. Arbutin Inhibits TCCSUP Human Bladder Cancer Cell Proliferation Via Up-Regulation of P21. Pharmazie (2011) 66(4):306–9. doi: 10.1691/ph.2011.0785
115. Bayram D, Armagan I, Ozgocmen M, Senol N, Calapoglu M. Determination of Apoptotic Effect of Juglone on Human Bladder Cancer Tcc-SUP and RT-4 Cells: An in Vitro Study. J Environ Pathol Toxicol Oncol (2018) 37(2):173–81. doi: 10.1615/JEnvironPatholToxicolOncol.2018025226
116. Huang SY, Chang SF, Liao KF, Chiu SC. Tanshinone IIA Inhibits Epithelial-Mesenchymal Transition in Bladder Cancer Cells Via Modulation of STAT3-CCL2 Signaling. Int J Mol Sci (2017) 18(8):616. doi: 10.3390/ijms18081616
117. Perabo FGE, Wirger A, Kamp S, Lindner H, Schmidt DH, Muller SC, et al. Carboxyamido-Triazole (CAI), a Signal Transduction Inhibitor Induces Growth Inhibition and Apoptosis in Bladder Cancer Cells by Modulation of Bcl-2. Anticancer Res (2004) 24(5a):2869–77.
118. Hoxhaj G, Manning BD. The PI3K-AKT Network At the Interface of Oncogenic Signalling and Cancer Metabolism. Nat Rev Cancer (2020) 20(2):74–88. doi: 10.1038/s41568-019-0216-7
119. Riedl SJ, Shi YG. Molecular Mechanisms of Caspase Regulation During Apoptosis. Nat Rev Mol Cell Biol (2004) 5(11):897–907. doi: 10.1038/nrm1496
120. Iguchi M, Hiroi M, Kanegae H, Ohmori Y. Costimulation of Murine Osteoblasts With Interferon-gamma and Tumor Necrosis Factor-alpha Induces Apoptosis Through Downregulation of Bcl-2 and Release of Cytochrome C From Mitochondria. Mediators Inflammation (2018) 2018:9, 3979606. doi: 10.1155/2018/3979606
121. Mendez DL, Akey IV, Akey CW, Kranz RG. Oxidized or Reduced Cytochrome C and Axial Ligand Variants All Form the Apoptosome In Vitro. Biochemistry (2017) 56(22):2766–9. doi: 10.1021/acs.biochem.7b00309
122. Burotto M, Chiou VL, Lee JM, Kohn EC. The MAPK Pathway Across Different Malignancies: A New Perspective. Cancer (2014) 120(22):3446–56. doi: 10.1002/cncr.28864
123. Papa S, Choy PM, Bubici C. The ERK and JNK Pathways in the Regulation of Metabolic Reprogramming. Oncogene (2019) 38(13):2223–40. doi: 10.1038/s41388-018-0582-8
124. Wu QS, Wong JPC, Kwok HF. Putting the Brakes on Tumorigenesis With Natural Products of Plant Origin: Insights Into the Molecular Mechanisms of Actions and Immune Targets for Bladder Cancer Treatment. Cells (2020) 9(5):1213. doi: 10.3390/cells9051213
Keywords: phytochemical, bladder cancer, apoptosis, proliferation, cell cycle
Citation: Xia Y, Chen R, Lu G, Li C, Lian S, Kang T-W and Jung YD (2021) Natural Phytochemicals in Bladder Cancer Prevention and Therapy. Front. Oncol. 11:652033. doi: 10.3389/fonc.2021.652033
Received: 13 January 2021; Accepted: 12 April 2021;
Published: 30 April 2021.
Edited by:
Xiaobing Tian, Brown University, United StatesCopyright © 2021 Xia, Chen, Lu, Li, Lian, Kang and Jung. This is an open-access article distributed under the terms of the Creative Commons Attribution License (CC BY). The use, distribution or reproduction in other forums is permitted, provided the original author(s) and the copyright owner(s) are credited and that the original publication in this journal is cited, in accordance with accepted academic practice. No use, distribution or reproduction is permitted which does not comply with these terms.
*Correspondence: Yong Xia, eGlheW9uZ0BtYWlsLmpubWMuZWR1LmNu; Young Do Jung, eWRqdW5nQGNob25uYW0uYWMua3I=