- 1Pancreatic Disease Center, Department of General Surgery, Rui Jin Hospital Affiliated to Shanghai Jiao Tong University School of Medicine, Shanghai, China
- 2Department of General Surgery, Yichuan Community Health, Shanghai, China
- 3Department of Surgery, Ruijin Hospital, Shanghai Jiaotong University School of Medicine, Shanghai, China
- 4Research Institute of Digestive Surgery, Ruijin Hospital, Shanghai Jiaotong University School of Medicine, Shanghai, China
Pancreatic cancer (PC) is one of the deadliest gastrointestinal cancers, accounting for the fourth highest number of cancer-related fatalities. Increasing data suggests that mesenchymal stem cells (MSCs) might influence the drug resistance of GC cells in the tumor microenvironment and play essential roles in drug resistance development. However, the precise underlying process remains a mystery. The purpose of this study was to look at the control of MSC-induced SNHG7 in pancreatic cancer. In vitro and in vivo sphere formation, colony formation, and flow cytometry investigations revealed the stemness and Folfirinox resistance in pancreatic cancer cells. To confirm the direct connections between SNHG7 and other related targets, RNA pulldown and immunoprecipitation tests were performed. MSC co-culture enhanced the stemness and Folfirinox resistance in pancreatic cancer cells according to the findings. MSC co-culture increased SNHG7 expression in pancreatic cancer cells, contributing to the stemness and Folfirinox resistance. We demonstrated that Notch1 interacted with SNHG7 and could reverse the facilitative effect of SNHG7 on the stemness and Folfirinox resistance in pancreatic cancer cells. Finally, our findings showed that MSCs increased SNHG7 expression in pancreatic cancer cells, promoting the stemness and Folfirinox resistance via the Notch1/Jagged1/Hes-1 signaling pathway. These findings could provide a novel approach and therapeutic target for pancreatic cancer patients.
Introduction
Pancreatic cancer is one of the deadliest gastrointestinal malignancies, accounting for the fourth highest number of cancer-related deaths (1). Pancreatic cancer develops at a rapid, unique, and accelerated rate (2). There is no clinically sensitive early diagnosis indication or effective treatment point as a result of a biological process encompassing numerous phases (3). As a result, the quest for diagnostic indicators and tailored medicines to slow the growth of pancreatic cancer has become a major emphasis in pancreatic cancer treatment.
It has been shown that just 2% of the genome sequence is capable of coding proteins, whereas non-coding RNA accounts for more than 95% of the transcripts (4). Long non-coding RNAs (lncRNAs) are a kind of non-coding RNA that is longer than 200 nucleotides and lacks the ability to code for proteins (nt). Emerging data suggests that lncRNA has a role in a variety of malignant tumors, including pancreatic cancer (5–7). For example, the lncRNA HOTTIP promotes pancreatic cancer by enhancing the Wnt/-catenin pathway by binding to WDR5 (8). Recently, there has been a progressive discovery in the investigation of small nucleolar RNA host gene 7 (SNGH7) in ovarian cancer (9). However, the precise functions of SNGH7 in pancreatic cancer are yet unknown. Most patients with pancreatic cancer can now be fully resected as far as feasible because of the advances in surgery and medication therapy in the recent years. However, many patients still experience recurrence or metastasis after surgical resection, with a poor prognosis. It is not difficult to find out the reasons for this. In addition to the highly malignant characteristics of pancreatic cancer itself, chemotherapy resistance is also an important reason. Folfirinox regimen is a common combination chemotherapy regimen for pancreatic cancer based on fluurazepine, and drug resistance often directly affects the prognosis of patients. Therefore, finding a solution to the drug resistance of Folfirinox regimen is an urgent need to explore (10).
In the present study, we investigated the functional role and regulatory mechanism of SNGH7 in the stemness and Folfirinox resistance of pancreatic cancer cells. We found that SNGH7 was induced under MSC-culture in pancreatic cancer and elevated SNGH7 promoted the stemness and Folfirinox resistance. Mechanistic investigations revealed that SNGH7 interacted with Notch1 to regulate the stemness and Folfirinox resistance through the Notch1/Jagged1/Hes-1 signaling pathway in pancreatic cancer.
Materials and Methods
Patients
Pancreatic cancer tissues (n = 50) were obtained by surgery and normal tissues adjacent to cancer were obtained at the same time from Ruijin Hospital Affiliated to Medical College of Shanghai Jiaotong University. All patients have signed informed consents. The study was approved by the ethics committee of Ruijin Hospital Affiliated to Medical College of Shanghai Jiaotong University and conducted under its supervision.
Cell Culture
Human pancreatic cancer cells (PANC-1 and AsPC-1) and adult bone marrow MSCs were obtained from the Cell Resource Center of Shanghai Academy of Sciences. Cells were cultured in RPMI-1640 (Procell Life Science&Technology, Wuhan, China) with 10% fetal bovine (Thermo-Scientific, MA, USA) and 1% penicillin-streptomycin (MP Biomedicals, CA, USA). The cells were cultured in 5% CO2 and 37°C incubators. A Transwell cell culture room (Thermo, USA) was used for co-cultivation. In the co-culture system, MSCs were placed in the upper chamber and pancreatic cancer cells were placed in the lower chamber, allowing direct contact between MSCs and PC cells. Folfirinox is a common plan for pancreatic cancer chemotherapy to treat cells. It consists of four drugs, FOL-Folinicacid (CSNpharm, Shanghai, China), F-Fluorouracil (CSNpharm, Shanghai, China), IRIN-Irinotecan (CSNpharm, Shanghai), China) and OX-oxaliplatin (CSNpharm, Shanghai, China).
Sphere-Forming Assay
Used 1× stem cell culture medium to adjust the cell density to 2 × 104/mL, inoculated 500 microliters of cells per well in a 24-well ultra-low adsorption plate (Corning company, cat No. 3473), cultured at 37°C, 5% CO2, respectively. Added 10× stem cell culture medium (50µL/well) to culture for 3, 5, and 7 days. Collected cells after culture for 8 days. Centrifuged at 100×g for 2 min. Discarded the supernatant. Resuspended the spheroid cells in 200µlL trypsin digestion solution and incubated at 37°C. In 3 minutes, added 800µL of serum-containing medium, and counted live cells using Countstar automatic cell counter (Alite, China). The formula of 1× stem cell culture medium was serum-free DMEM/F12 medium (Thermo, USA), containing 20 ng/mL EGF (Thermo, USA), 20 ng/mL bFGF (Thermo, USA), 4 µg/mL heparin (XiYa reagent company, China) and 1×B27 (Thermo, USA).
Cell Transfection
When cells reached 60%–80% confluence, the transfection was performed. The SNHG7 vector and the control vector (GenePharma, Shanghai, China) were transfected using the Lipofectamine 2000 Reagent (Thermo-Scientific, MA, USA). After culturing for 48 h, cells were utilized for the follow-up study.
Real-Time Quantitative Polymerase Chain Reaction (RT-qPCR)
Total RNAs were obtained from tissues and cells using TRIzol (Invitrogen, MA, USA). Reverse Transcription Kit (D1802, Haigene, Harbin, China) was used for the reverse transcription of RNA and obtain the cDNA. SYBR green PCR Kit (Vazyme, Nanjing, China) was used for RT-qPCR. U6 and GAPDH were used as the endogenous genes to normalize the relative expression of miRNAs and genes. Several studies have shown that the expression of SOX2, OCT-4, LIN28, and CD133 can be used as a marker for pancreatic cancer MSC, so we chose to detect the related expression of these four genes to reflect the change of stem cell characteristics (11).
Western Blot
RIPA lysis buffer with 1 mM PMSF was used to extract the total protein (Solarbio, Beijing, China). BCA protein assay kit (Thermo Fisher, MA, USA) was used to measure the protein concentration after protein extraction. A total of 30 g of total proteins was separated using 10% SDS-PAGE gels and transferred to a PVDF membrane (Millipore, USA). After 2 h of blocking with 5% skim milk, the membrane was incubated overnight at 4°C with a particular primary antibody. The particular primary antibodies were bought from Abcam, and the concentration used in this investigation was 1:1,000. The membrane was then treated for 2 h at room temperature with the matching secondary antibodies. The protein signals are seen using the ECL Western blotting substrate (Tanon, Shanghai, China). The internal gene to indicate the relative expression was GAPDH.
Colony Formation Assay
Cells were inoculated into a 6-well plate and the density was 500 cells/well. Then, the cells were cultured for 14 days in the appropriate medium. Following, the cells were fixed with methanol, washed twice with PBS, and stained with 0.1% crystal violet solution (Beyotime, Shanghai, China). The colonies were observed using a microscope (Nikon, Tokyo, Japan) and counted in six different fields.
Flow Cytometry
Cells were collected and passed through 100-mesh sieves. Then, the cells were incubated for 15 min with the Annexin V and PI solution at room temperature. The staining process needed to avoid light. The labeled cells were analyzed through the FACS flow cytometry (Leica, Wetzlar, Germany).
RNA Pulldown Assay
The pulldown assay was performed according to the previously described protocol (8). In brief, biotinylated miR-526b-3p wild type and biotinylated miR-526b-3p mutant or control probe were respectively transfected in PANC-1 and AsPC-1 cells. These probes were purchased from RiboBio (Guangzhou, China). A total of 107 PANC-1 and AsPC-1 cells were harvested and lysed by a lysis buffer. The total RNA solution was added DNaseI and incubated for 5 min at 65°C, followed by an instant ice bath. The solution was then incubated for 4 h with streptavidin-coated magnetic beads (New England BioLabs, USA) at 4°C. Then, the beads were washed with PBS, and RNA was extracted through the Trizol reagent.
Animal Assay
BALB/C nude mice (4 weeks old) were purchased from the Experimental Animal Center of Nanfang Hospital in Guangzhou, China for the following two experiments. Continuous dilutions of PANC-1 and AsPC-1 cell suspensions (5 105, 5 104, and 5 103 cells) with or without 5 106 MSCs were subcutaneously injected into nude mice in the first experiment. Six weeks later, the mice were killed, and tumor development was assessed. Furthermore, cells were transfected with pcDNA3.1/SNGH7 or pcDNA3.1, then, weekly intraperitoneally injected into mice treated with or without Folfirinox. Every 4 days, the volume of the tumors was determined. The mice were killed after 4 weeks, and the weight of the tumors was assessed.
Transwell Assay
Normal pancreatic cancer cells were taken, digested, and centrifuged, and the supernatant was discarded and added to the RPMI-1640 basic medium. The cells were mixed and counted, placed in an empty 6-well plate, and placed in an incubator. The second to fifth generation of MSCs with normal growth were taken, digested, and centrifuged, and the cells were mixed with the complete medium of MSCs and counted. The final number of cells per well was about 5 x 105. Four hours later, the culture medium in the 6-well plate was sucked out and cleaned with PBS, replaced with the same amount of RPMI-1640 basic medium, and the cells were placed in the empty 6-well plate and placed in the incubator. After cleaning with PBS, each chamber was placed in a 6-well plate with 1.5 mL of methanol solution and fixed for 20 minutes. The chambers were dried, the cells on the surface of the compartment were wiped, and the cells were placed in a 6-well plate with 1.5 mL of crystal violet dye solution and stained in the dark for 60 minutes. The chambers were observed under a 200-magnification microscope.
Statistical Analysis
Three different experiments yielded the following results. All of the results were provided as means standard deviations. GraphPad Prism 7.0 was used to analyze the data, which included one-way ANOVA and t-tests (GraphPad Inc., San Diego, CA, USA). P < 0.05 was regarded as statistically significant.
Results
MSCs Promote the Stemness and Folfirinox Resistance of Pancreatic Cancer Cells
In order to determine whether MSCs can promote the stemness and Folfirinox resistance of pancreatic cancer cells, pancreatic cancer cell lines, PANC-1 and AsPC-1, were co-cultured with MSCs via a transwell co-culture system. Consequently, the ability of PANC-1 and AsPC-1 cells to generate tumor spheres was enhanced under the co-culture with MSC (Figure 1A). Furthermore, co-culture of MSCs greatly increased the expression of stemness genes such as SOX2, Oct-4, LIN28, and CD133 at both the mRNA and protein levels (Figures 1B, C). According to the flow cytometry experiment, the number of CD44 positive (CD44+) pancreatic cancer cells, which are considered as cancer stem cell (CSC) characteristic indicators, was also enhanced (Figure 1D).
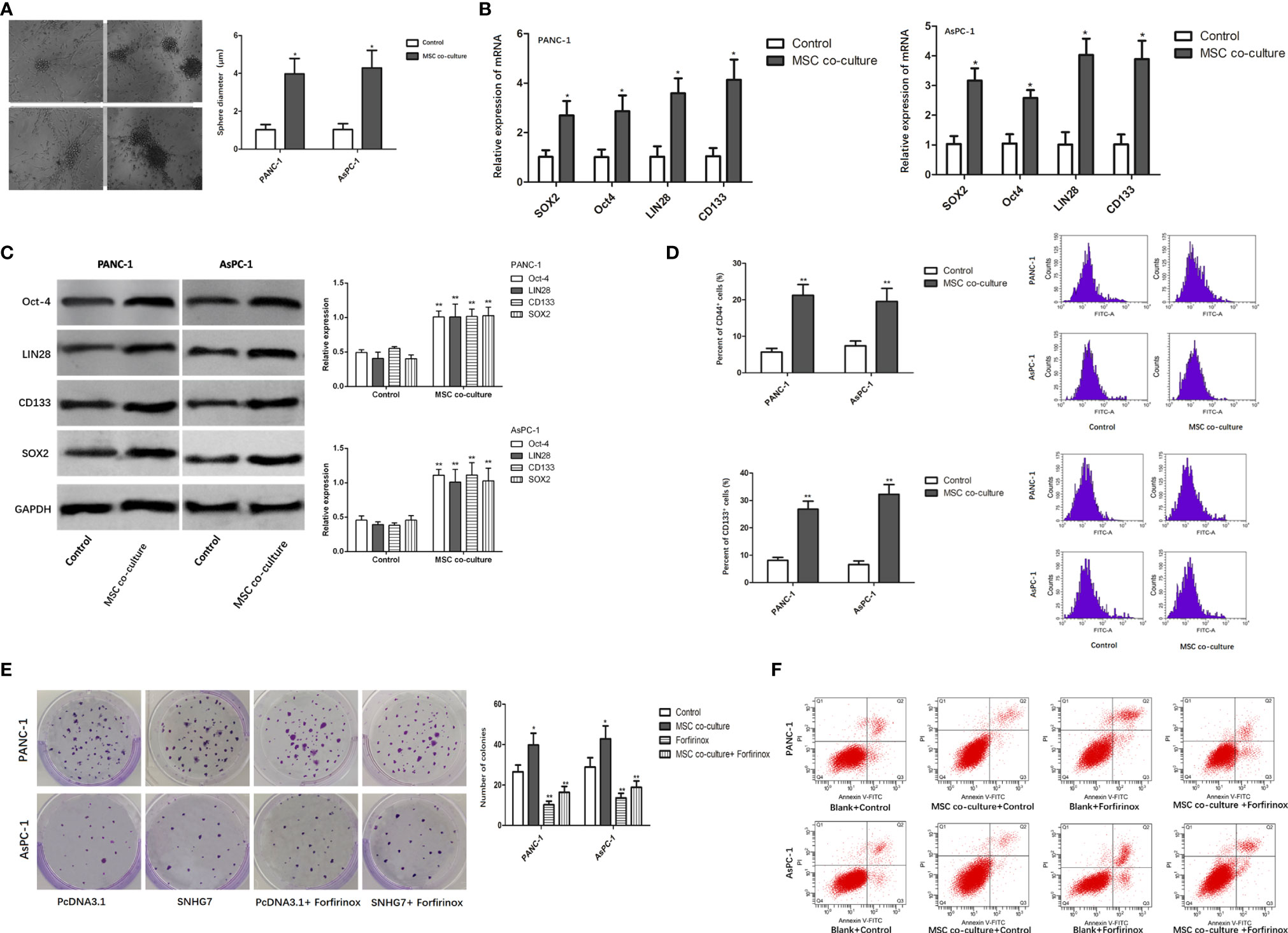
Figure 1 MSCs promote the stemness and Folfirinox resistance of pancreatic cancer cells. (A) Representative images and quantification of the tumor spheres generated by PANC-1, AsPC-1 cells with or without the co-culture with MSCs. (B, C) The expression of stemness markers were analyzed by using qRT-PCR and Western blot assays. (D) Flow cytometry analysis showed the rate of CD44+ cells upon MSC co-culture. (E, F) Colony-formation and flow cytometry analyses to detected the PANC-1, AsPC-1 cells with or without MSCs. *P < 0.05, **P < 0.01.
The findings of a colony formation experiment revealed that the growth inhibitory effects of Folfirinox resistance on pancreatic cancer cells were significantly reduced in the presence of MSCs (Figure 1E). Furthermore, MSC co-culture lowered GC cell apoptosis, and MSC co-culture inhibited the inductive impact of Folfirinox on pancreatic cancer cell apoptosis (Figure 1F). These findings indicated that MSCs can promote the stemness and Folfirinox resistance in pancreatic cancer cells.
Effect of MSC in Xenograft Models
To further investigate the effect of MSCs on tumor initiation, we subcutaneously injected a limiting dilution of PANC-1 cells at three dosages, 5 × 103, 5 × 104, and 5 × 105, with or without 5 × 106 admixed MSCs into nude mice. For 4 weeks, each group got Folfirinox intraperitoneal therapy once every 2 days. As a consequence, we discovered that PANC-1 cells alone at 5 103 and 5 104 cells failed to form xenografts. However, as compared to the injection of pancreatic cancer cells alone, cell combination with MSCs efficiently produced a xenograft and enhanced the weight of tumors transplanted, indicating that MSCs trigger pancreatic cancer cell de novo tumor development (Figure 2A). In addition, we used IHC labeling to identify the expression of MSC surface antigens, CD29 and CD90, in pancreatic cancer tissues. The results showed that the proportion of CD29+/CD90+ patients in pancreatic cancer tissues was substantially greater than in the responder group, and the rate of CD29+CD90+ in GC specimens was favorably connected with the clinical stage (Figures 2B, C). MSCs boosted the stem characteristics and chemo-resistance in pancreatic cancer cells according to the findings.
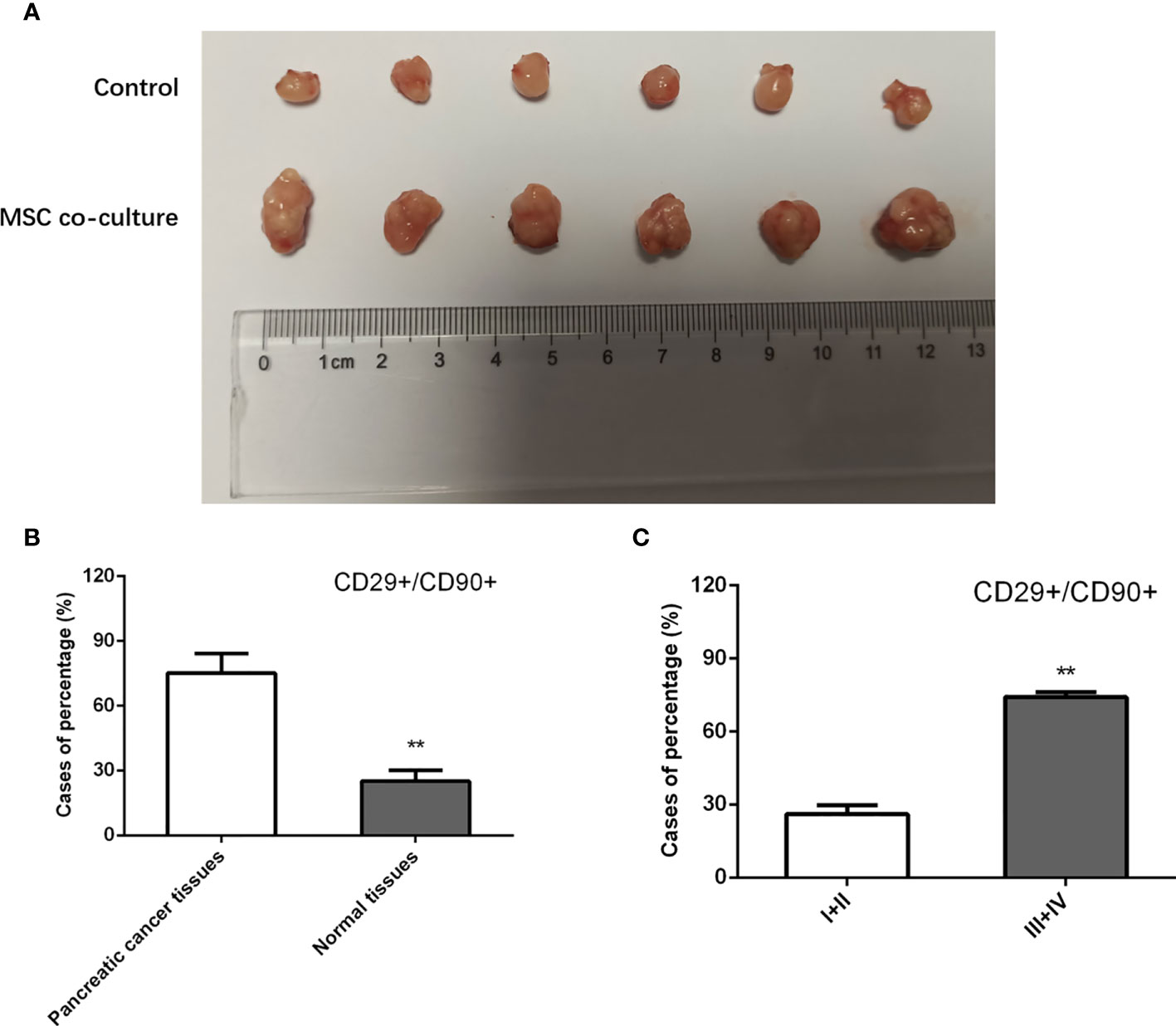
Figure 2 Effect of MSC in xenograft models. (A) Nude mice were injected with PANC-1 cells, and tumor weight in mice of each group were assessed. (B, C) Quantification of IHC staining rate of CD29+CD90+ in non-tumorous specimens or pancreatic cancer specimens from patients at stages I/II and III/IV. **P < 0.01.
SNHG7 Is Induced by MSCs and Contributes to the Stemness and Folfirinox Resistance
Previous research has found that SNHG7 is overexpressed in pancreatic cancer tissues and promotes metabolic plasticity via antioxidant synthesis. As a result, we postulated that SNHG7 could have a role in the stemness and chemoresistance. SNHG7 was shown to be increased in pancreatic cancer tissues as compared to normal tissues (n = 50; Figure 3A). To identify whether SNHG7 was induced by MSCs, we detected its expression in PANC-1 and AsPC-1 cells co-cultured with or without MSCs. We found that the expression of SNHG7 was obviously increased both in pancreatic cancer after the co-culture with MSCs when compared to non-cultured cells (Figure 3B). In addition, we also performed in situ hybridization (ISH) to evaluate the correlation between SNHG7 expression and co-expression of CD29 and CD90 in pancreatic cancer tissues, which are well-known MSC surface antigen markers. We found that SNHG7 expression was higher in pancreatic cancer tissues that were both CD29 and CD90 positive (CD29+CD90+) than in pancreatic cancer tissues that were both CD29 and CD90 negative (CD29CD90) (Figure 3C). These findings suggest that SNHG7 may play a role in the effects of MSCs on pancreatic cancer cell stemness.
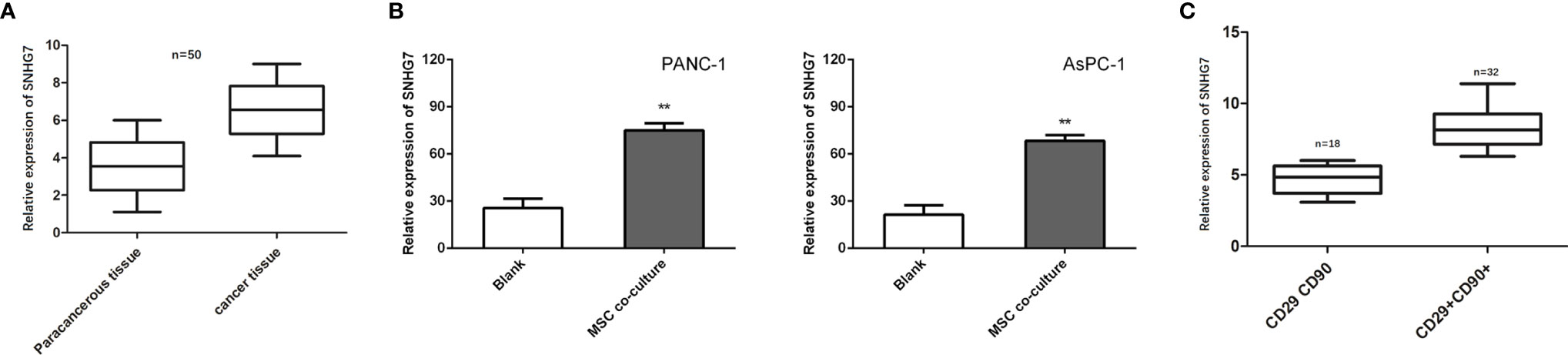
Figure 3 SNHG7 is induced by MSCs and contributes to the stemness and Folfirinox resistance. (A, B) SNHG7 expression was determined via qRT-PCR in pancreatic cancer tissues or cells with or without co-culture of MSCs. (C) The correlation between SNHG7 expression and co-expression of CD29 and CD90 in pancreatic cancer tissues. **P < 0.01.
We investigated the effect of SNHG7 on the stemness and Folfirinox resistance. SNHG7 was overexpressed in stable lentivirus transfected way, and the overexpression efficiency that was examined was verified by RT-qPCR analyses (Figure 4A). The findings of the RT-qPCR and Western blot studies revealed that the expression of SNHG7 and stemness genes was significantly elevated in PANC-1 and AsPC-1 cells after transfection with pcDNA3.1 SNHG7 compared to the NC group (Figures 4B, C). Flow cytometric analysis revealed that SNHG7 overexpression enhanced the fraction of CD44+ pancreatic cancer cells (Figure 4D). Besides, sphere-formation assay indicated that the sphere formation capability of PANC-1 and AsPC-1 cells was obviously increased after SNHG7 overexpression (Figure 4E). To evaluate whether SNHG7 is functionally involved in pancreatic cancer progression, transwell assay and flow cytometric analysis were performed. When treated with Folfirinox, the induced expression of SNHG7 promoted invasion and abolished growth inhibition, as demonstrated in Figure 4F. SNHG7 overexpression decreased cell apoptosis, and Folfirinox-induced apoptosis was reversed by SNHG7 ectopic expression in pancreatic cancer cells (Figure 4G). These results clearly indicated that MSC caused the stemness and Folfirinox resistance via inducing SNHG7.
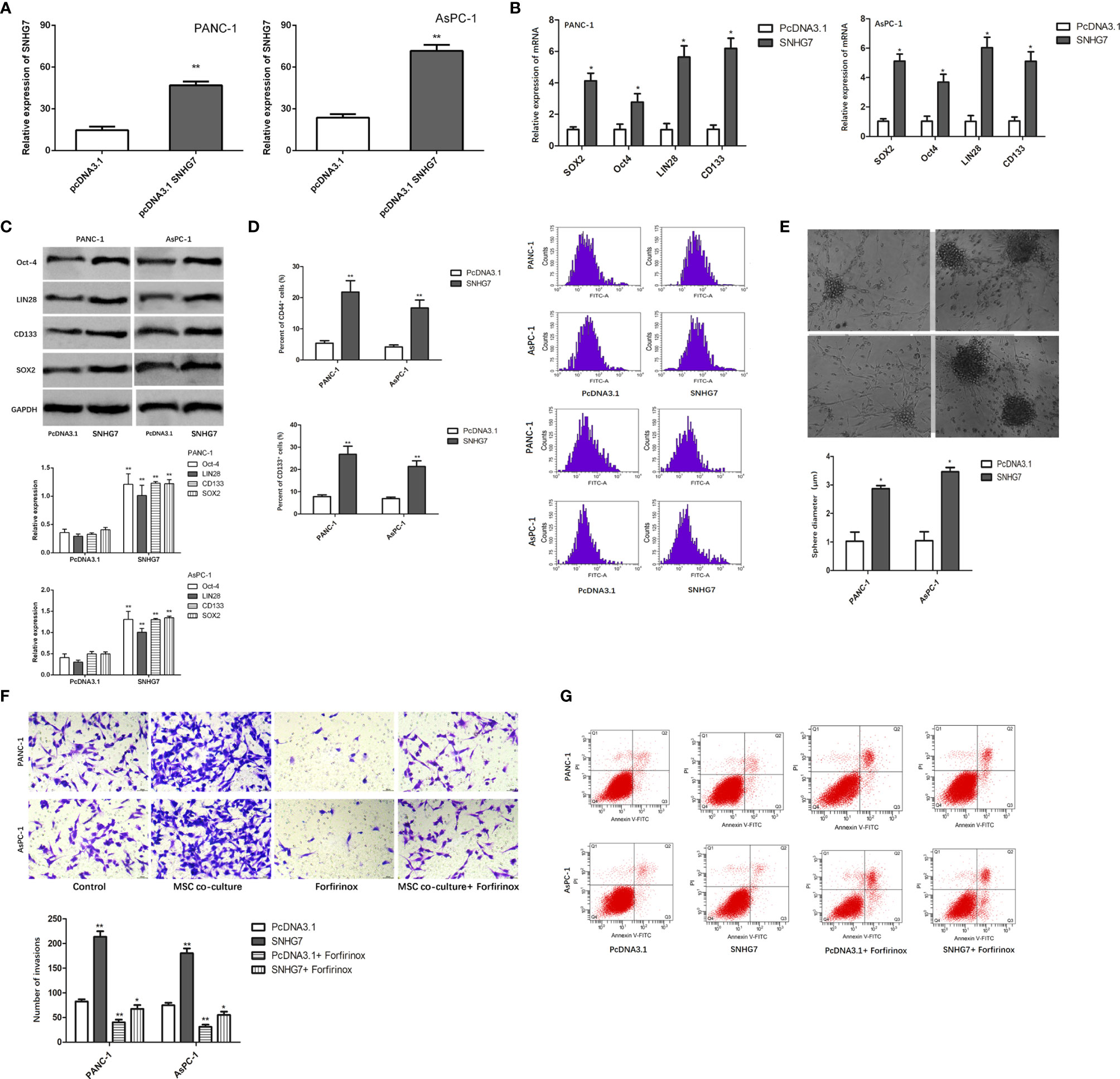
Figure 4 The effect of SNHG7 on the stemness and Folfirinox resistance. (A, B) overexpression efficiency was examined was verified by RT-qPCR and Western blot analyses. (C) Western blotting for stemness-associating genes in PANC-1 and AsPC-1 cell culture either alone or with MSCs and with or without Folfirinox. (D) The proportion of CD44+ pancreatic cancer cells. (E) Sphere-formation assay in PANC-1 and AsPC-1 cell culture either alone or with MSCs and with or without Folfirinox. (F, G) Colony-formation assay and flow cytometric analysis of PANC-1 and AsPC-1 cell culture either alone or with MSCs and with or without Folfirinox. *P < 0.05, **P < 0.01.
SNHG7 Is Interacted With Notch1 to Regulate the Stemness and Folfirinox Resistance in Pancreatic Cancer
To further explore the regulatory mechanism of SNHG7, we searched for the target mRNAs for SNHG7. Prediction results from starBase3.0 (http://starbase.sysu.edu.cn/) showed that SNHG7 potentially interacted with miRNA. Using RT-qPCR, the three most substantially elevated mRNAs in pancreatic cancer cells in response to co-culture with MSCs were Notch1, FMR1, and U2AF2 (Figure 5A). In a pulldown test, SNHG7 could only pull down Notch1 rather than antisense SNHG7 in pancreatic cancer cells (Figure 5B), showing that SNHG7 interacted with Notch1. RIP assay further confirmed the direct interaction between SNHG7 and Notch1 (Figure 5C). Therefore, we deduced that Notch1 was a target for SNHG7 in pancreatic cancer.

Figure 5 SNHG7 interacted with Notch1 to regulate the stemness and Folfirinox resistance in pancreatic cancer. (A) RT-qPCR western blot analyses revealed that three of the most upregulated miRNAs in pancreatic cancer cells respond to co-culture with MSC. (B) Pull down assay depicted that only Notch1 could be pulled down by SNHG7. (C) RIP analysis demonstrated the co-immunoprecipitation of SNHG7 and Notch1. *P < 0.05, **P < 0.01.
Moreover, we tried to examine whether SNHG7 modulated stemness and resistance in pancreatic cancer cells through Notch1. RT-qPCR findings revealed that SNHG7 overexpression promoted Notch1 expression, but si-Notch1 co-transfection restored Notch1 expression levels in PANC-1 and AsPC-1 cells (Figure 6A). The co-transfection of si-Notch1 suppressed the protein levels of the stemness markers caused by SNHG7 overexpression (Figure 6B). Ability of PANC-1 and AsPC-1 cells to form tumor spheres was enhanced by SNHG7 overexpression and repressed by Notch1 downexpression (Figure 6C). CCK8 assay demonstrated that the inhibitive effect of Folfirinox on cell viability was reduced by SNHG7 overexpression and regained by the silenced expression of Notch1 (Figure 6D). Also, under the treatment of Folfirinox, apoptosis in PANC-1 and AsPC-1 cells was reduced by SNHG7 overexpression, and rescued by co-transfection of si-Notch1 (Figure 6E). Hence, our findings indicated that SNHG7 interacted with Notch1 to regulate the stemness and Folfirinox resistance in pancreatic cancer.
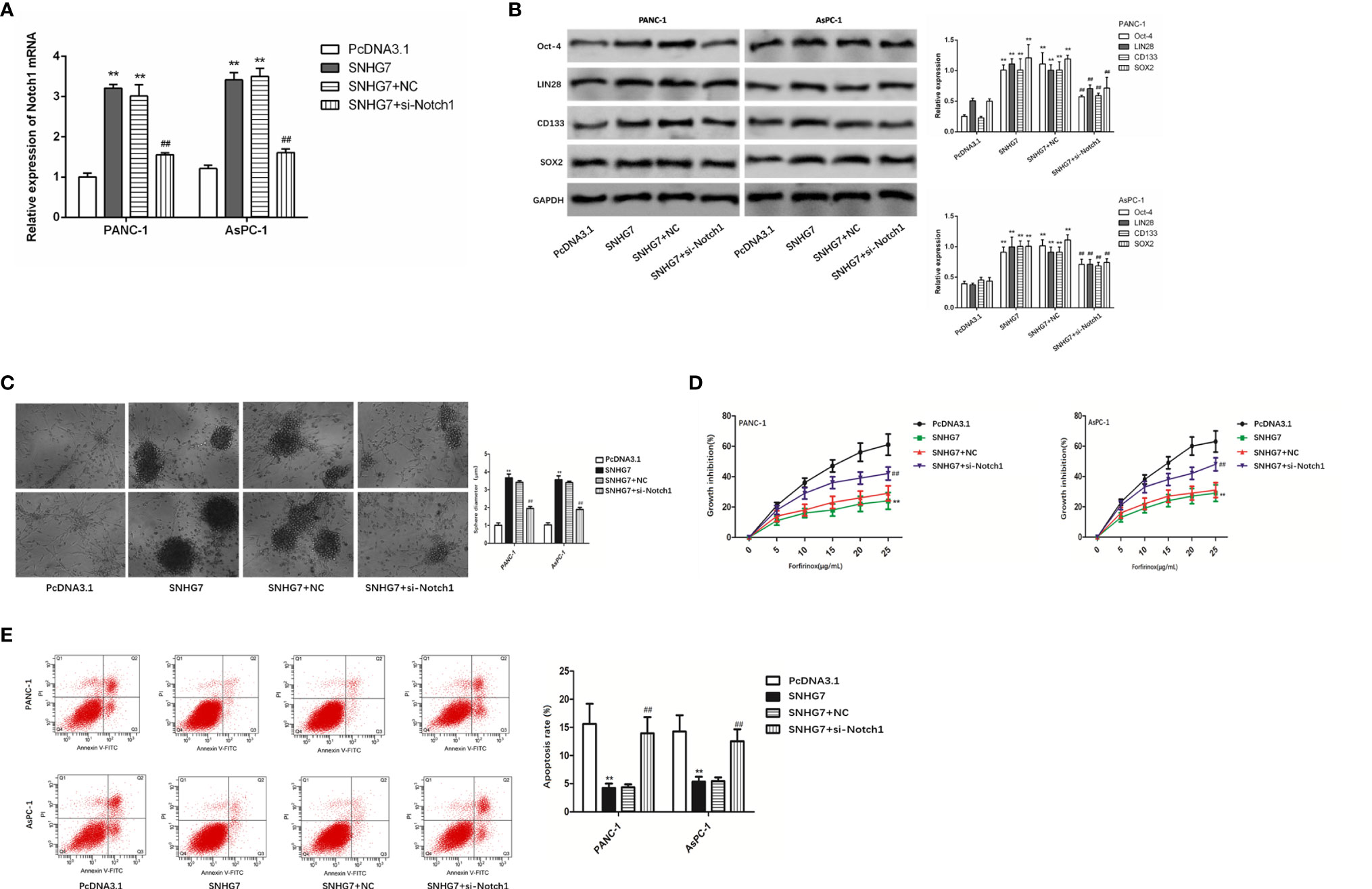
Figure 6 Notch1 to regulate the stemness and Folfirinox resistance in pancreatic cancer. (A) Notch1 expression was assessed via RT-qPCR assay. (B) The expression of stemness markers were analyzed by using Western blot assay. (C–E) Sphere-formation assay, Colony-formation, and flow cytometry analyses. **P < 0.01 (compared with pcDNA3.1), ##P < 0.01 (compared SNHG7+NC with SNHG7+si-Notch1).
MSC-Induced SNHG7 Facilitate Stemness and Folfirinox Resistance Through the Notch1/Jagged1/Hes-1 Signaling Pathway in Pancreatic Cancer
The expressions of the Notch1/Jagged1/Hes-1 signaling pathway components (Notch1, Jagged1, and Hes1) in PANC-1 and AsPC-1 cells were examined by qRT-PCR and Western blot tests to assess the biological relevance of SNHG7 in pancreatic cancer stemness and Folfirinox resistance. The results showed that the expressions of Notch1, Jagged1, and Hes1at mRNA and protein levels were significantly upregulated in PANC-1 and AsPC-1 cells with the transfection of pcDNA3.1 SNHG7 compared with the NC group (Figures 7A, B).
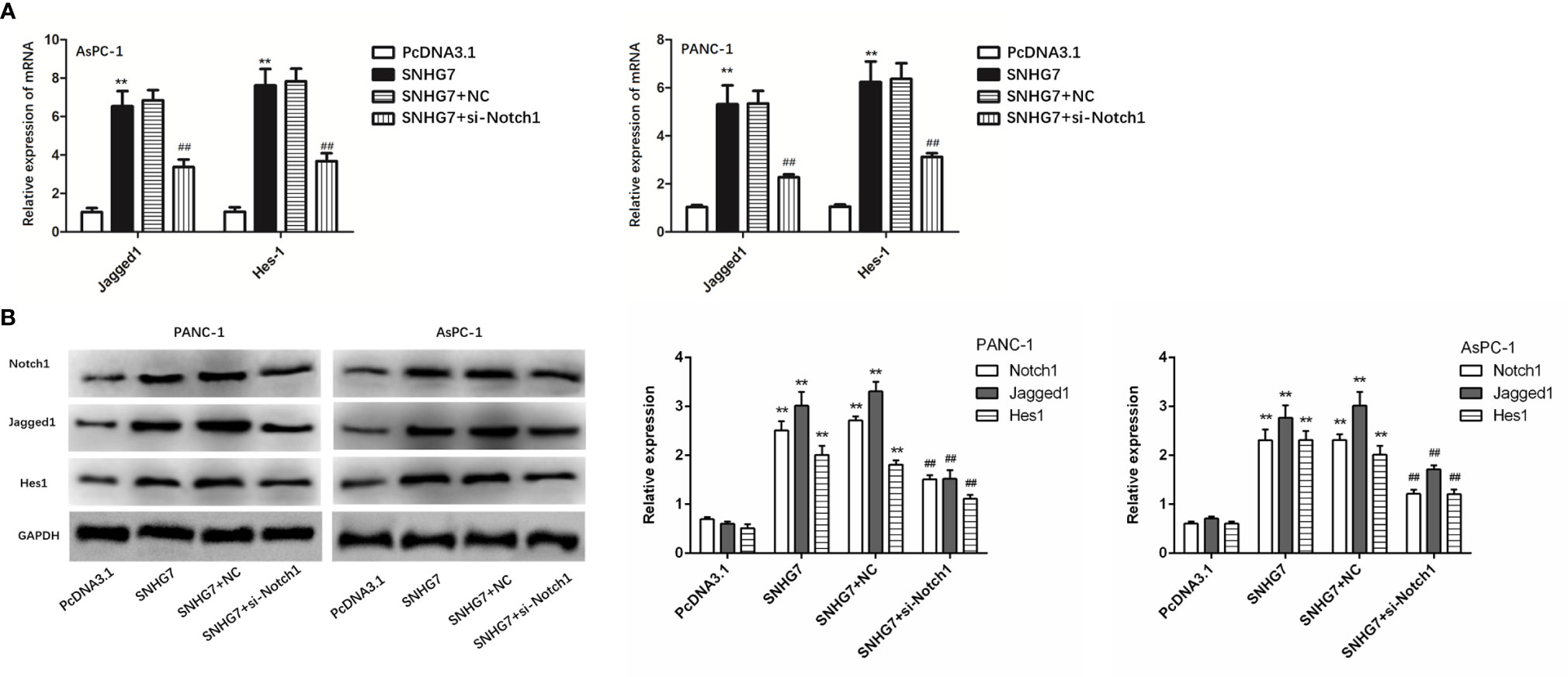
Figure 7 MSC-induced SNHG7 facilitate the stemness and Folfirinox resistance through the Notch1/Jagged1/Hes-1 signaling pathway in pancreatic cancer. (A, B) The expressions of the Notch1/Jagged1/Hes-1 signaling pathway related mRNA and proteins were determined by qRT-PCR and Western blot assays. **P < 0.01 (compared control with pcDNA3.1), ##P < 0.01 (compared SNHG7+NC with SNHG7+si-Notch1).
Discussion
Pancreatic cancer is a lethal solid tumor that usually causes local invasion and early spread, killing more than 300,000 individuals each year. PC patients have a poor prognosis, with less than 5% of patients living longer than 5 years (12). As a result, developing possible diagnostic markers or targeted medications to halt the progression of pancreatic cancer is important.
As an important component of the tumor environment, MSCs are a heterogeneous stroma/stem-like phenotype with the ability to differentiate along the mesodermal lineage (13). They may promote chemotherapy resistance by secreting protective cytokines or even generating genetic mutations and altering transcriptional expression to assist cancer cells in overcoming the anticancer effect of chemotherapeutic agents (14, 15). Recently, it has been demonstrated that MSCs play an important role in tumor chemoresistance (16). MSCs have been shown to release polyunsaturated fatty acids KHT and 16:4(n3), which can lead to resistance to platinum-based chemotherapy (17). Thus, it is unclear whether MSCs play a substantial role in metabolic reprogramming in the control of chemotherapy resistance.
As sequencing technology has advanced, more non-coding RNA has been identified. Among these, lncRNAs have gotten a lot of interest because of their diverse roles in all stages of carcinogenesis and tumor development, and lncRNAs might be exploited as novel prognostic indicators (18, 19). By influencing oncogenes or tumor suppressor genes, they contribute to tumor development, proliferation, and metastasis (20, 21). A growing body of data suggests that lncRNAs have a role in cancer stemness and treatment resistance (22, 23).
Small nucleolar RNA host gene 7 (SNHG7) has been shown to be carcinogenic in ovarian cancer (9). In this study, we looked at the unique biological effects of SNHG7 in pancreatic cancer. The key finding of this study is that SNHG7 plays a significant role in pancreatic cancer. We explored the critical function and post-transcriptional regulation of SNHG7 in MSC-induced stemness and Folfirinox resistance in this work. We found that the overexpression of SNHG7 confers Folfirinox resistance and enhances the stemness of pancreatic cancer cells.
Mechanistically, the interaction of lncRNAs with mRNAs in cancer progression and cellular response to chemotherapy has been widely documented. The Notch signaling system is an evolutionarily conserved system in the tumor microenvironment that is known to modulate the expression of its target genes and consequently plays an important role in various cellular processes such as cell proliferation, migration, and death (24). Notch receptors have been reported to be commonly dysregulated in several neoplastic lesions, demonstrating that Notch has an oncogenic function in a variety of malignancies (25). More crucially, mounting data suggests that the Notch signaling pathway is implicated in the chemoresistance of many tumor cells and also promotes radiation resistance in certain malignancies (26–28). We demonstrated that Notch1 interacted with SNHG7 and could reverse the facilitative effect of SNHG7 on the stemness and Folfirinox resistance in pancreatic cancer cells.
In conclusion, our findings show that MSCs increased SNHG7 expression in pancreatic cancer cells, promoting the stemness and Folfirinox resistance via the Notch1/Jagged1/Hes-1 signaling pathway. Our result not only helps us to better understand the regulatory potential of SNHG7 in pancreatic cancer stemness and Folfirinox resistance, but it is also important for identifying new pharmacological targets and devising innovative treatment techniques to overcome resistance.
Data Availability Statement
The original contributions presented in the study are included in the article. Further inquiries can be directed to the corresponding authors.
Author Contributions
All authors contributed to the article and approved the submitted version.
Funding
This work was supported by the Interdisciplinary Program of Shanghai Jiao Tong University under grant No. YG2019QNB26.
Conflict of Interest
The authors declare that the research was conducted in the absence of any commercial or financial relationships that could be construed as a potential conflict of interest.
Publisher’s Note
All claims expressed in this article are solely those of the authors and do not necessarily represent those of their affiliated organizations, or those of the publisher, the editors and the reviewers. Any product that may be evaluated in this article, or claim that may be made by its manufacturer, is not guaranteed or endorsed by the publisher.
References
1. Hui B, Ji H, Xu Y, Wang J, Ma Z, Zhang C, et al. RREB1-Induced Upregulation of the lncRNA AGAP2-AS1 Regulates the Proliferation and Migration of Pancreatic Cancer Partly Through Suppressing ANKRD1 and ANGPTL4. Cell Death Dis (2019) 10(3):207. doi: 10.1038/s41419-019-1384-9
2. Siegel RL, Miller KD, Jemal A. Cancer Statistics, 2019. CA Cancer J Clin (2019) 69(1):7–34. doi: 10.3322/caac.21551
3. Goggins M, Overbeek KA, Brand R, Syngal S, Del Chiaro M, Bartsch DK. C. International Cancer of the Pancreas Screening. Management of Patients With Increased Risk for Familial Pancreatic Cancer: Updated Recommendations From the International Cancer of the Pancreas Screening (CAPS) Consortium. Gut (2020) 69(1):7–17. doi: 10.1136/gutjnl-2019-319352corr1
4. Martens-Uzunova ES, Bottcher R, Croce CM, Jenster G, Visakorpi T, Calin GA. Long Noncoding RNA in Prostate, Bladder, and Kidney Cancer. Eur Urol (2014) 65(6):1140–51. doi: 10.1016/j.eururo.2013.12.003
5. Zuo Z, Hu H, Xu Q, Luo X, Peng D, Zhu K, et al. BBCancer: An Expression Atlas of Blood-Based Biomarkers in the Early Diagnosis of Cancers. Nucleic Acids Res (2020) 48(D1):D789–96. doi: 10.1093/nar/gkz942
6. Jin X, Xu XE, Jiang YZ, Liu YR, Sun W, Guo YJ, et al. The Endogenous Retrovirus-Derived Long Noncoding RNA TROJAN Promotes Triple-Negative Breast Cancer Progression via ZMYND8 Degradation. Sci Adv (2019) 5(3):eaat9820. doi: 10.1126/sciadv.aat9820
7. Qu D, Sun WW, Li L, Ma L, Sun L, Jin X, et al. Long Noncoding RNA MALAT1 Releases Epigenetic Silencing of HIV-1 Replication by Displacing the Polycomb Repressive Complex 2 From Binding to the LTR Promoter. Nucleic Acids Res (2019) 47(6):3013–27. doi: 10.1093/nar/gkz117
8. Fu Z, Chen C, Zhou Q, Wang Y, Zhao Y, Zhao X, et al. LncRNA HOTTIP Modulates Cancer Stem Cell Properties in Human Pancreatic Cancer by Regulating HOXA9. Cancer Lett (2017) 410:68–81. doi: 10.1016/j.canlet.2017.09.019
9. Bai Z, Wu Y, Bai S, Yan Y, Kang H, Ma W, et al. Long non-Coding RNA SNGH7 Is Activated by SP1 and Exerts Oncogenic Properties by Interacting With EZH2 in Ovarian Cancer. J Cell Mol Med (2020) 24(13):7479–89. doi: 10.1111/jcmm.15373
10. Sarvepalli D, Schultheiss M, Rahman A. Gemcitabine: A Review of Chemoresistance in Pancreatic Cancer. Crit Rev Oncog (2019) 24(2):199–212. doi: 10.1615/CritRevOncog.2019031641
11. Calloni R, Cordero E, Henriques J. Reviewing and Updating the Major Molecular Markers for Stem Cells. Stem Cells Dev (2013) 22(9):1455–76. doi: 10.1089/scd.2012.0637
12. Wei W, Liu Y, Lu Y, Yang B, Tang L. LncRNA XIST Promotes Pancreatic Cancer Proliferation Through miR-133a/EGFR. J Cell Biochem (2017) 118(10):3349–58. doi: 10.1002/jcb.25988
13. Cuiffo BG, Campagne A, Bell GW, Lembo A, Orso F, Lien EC, et al. MSC-Regulated microRNAs Converge on the Transcription Factor FOXP2 and Promote Breast Cancer Metastasis. Cell Stem Cell (2014) 15(6):762–74. doi: 10.1016/j.stem.2014.10.001
14. Houthuijzen JM, Daenen LG, Roodhart JM, Voest EE. The Role of Mesenchymal Stem Cells in Anti-Cancer Drug Resistance and Tumour Progression. Br J Cancer (2012) 106(12):1901–6. doi: 10.1038/bjc.2012.201
15. Meads MB, Hazlehurst LA, Dalton WS. The Bone Marrow Microenvironment as a Tumor Sanctuary and Contributor to Drug Resistance. Clin Cancer Res (2008) 14(9):2519–26. doi: 10.1158/1078-0432.CCR-07-2223
16. Seebach C, Schultheiss J, Wilhelm K, Frank J, Henrich D. Comparison of Six Bone-Graft Substitutes Regarding to Cell Seeding Efficiency, Metabolism and Growth Behaviour of Human Mesenchymal Stem Cells (MSC) In Vitro. Injury (2010) 41(7):731–8. doi: 10.1016/j.injury.2010.02.017
17. Roodhart JM, Daenen LG, Stigter EC, Prins HJ, Gerrits J, Houthuijzen JM, et al. Mesenchymal Stem Cells Induce Resistance to Chemotherapy Through the Release of Platinum-Induced Fatty Acids. Cancer Cell (2011) 20(3):370–83. doi: 10.1016/j.ccr.2011.08.010
18. Ghoveud E, Teimuri S, Vatandoost J, Hosseini A, Ghaedi K, Etemadifar M, et al. Potential Biomarker and Therapeutic LncRNAs in Multiple Sclerosis Through Targeting Memory B Cells. Neuromol Med (2020) 22:111–20. doi: 10.1007/s12017-019-08570-6
19. Storti CB, de Oliveira RA, de Carvalho M, Hasimoto EN, Cataneo DC, Cataneo AJM, et al. Telomere-Associated Genes and Telomeric lncRNAs are Biomarker Candidates in Lung Squamous Cell Carcinoma (LUSC). Exp Mol Pathol (2020) 112:104354. doi: 10.1016/j.yexmp.2019.104354
20. Kang M, Ren M, Li Y, Fu Y, Deng M, Li C. Exosome-Mediated Transfer of lncRNA PART1 Induces Gefitinib Resistance in Esophageal Squamous Cell Carcinoma via Functioning as a Competing Endogenous RNA. J Exp Clin Cancer Res (2018) 37:171. doi: 10.1186/s13046-018-0845-9
21. Zhang W, Cai X, Yu J, Lu X, Qian Q, Qian W. Exosome-Mediated Transfer of lncRNA RP11838N2.4 Promotes Erlotinib Resistance in Non-Small Cell Lung Cancer. Int J Oncol (2018) 53:527–38. doi: 10.3892/ijo.2018.4412
22. Song X, Zhang X, Wang X, Chen L, Jiang L, Zheng A, et al. LncRNA SPRY4-IT1 Regulates Breast Cancer Cell Stemness Through Competitively Binding miR-6882-3p With TCF7L2. J Cell Mol Med (2020) 24:772–84. doi: 10.1111/jcmm.14786
23. Yang X, Tao H, Wang C, Chen W, Hua F, Qian H. lncRNA-ATB Promotes Stemness Maintenance in Colorectal Cancer by Regulating Transcriptional Activity of the Beta-Catenin Pathway. Exp Ther Med (2020) 19:3097–103. doi: 10.3892/etm.2020.8558
24. Fazio C, Ricciardiello L. Inflammation and Notch Signaling: A Crosstalk With Opposite Effects on Tumorigenesis. Cell Death Dis (2016) 7:e2515. doi: 10.1038/cddis.2016.408
25. Wang Z, Li Y, Banerjee S, Sarkar FH. Emerging Role of Notch in Stem Cells and Cancer. Cancer Lett (2009) 279:8–12. doi: 10.1016/j.canlet.2008.09.030
26. Zou W, Ma X, Hua W, Chen B, Cai G. Caveolin-1 Mediates Chemoresistance in Cisplatin-Resistant Ovarian Cancer Cells by Targeting Apoptosis Through the Notch-1/Akt/NF-kappaB Pathway. Oncol Rep (2015) 34:3256–63. doi: 10.3892/or.2015.4320
27. Kim B, Stephen SL, Hanby AM, Horgan K, Perry SL, Richardson J, et al. Chemotherapy Induces Notch1-Dependent MRP1 Up-Regulation, Inhibition of Which Sensitizes Breast Cancer Cells to Chemotherapy. BMC Cancer (2015) 15:634. doi: 10.1186/s12885-015-1625-y
Keywords: SNHG7, Notch1, pancreatic cancer, stemness, resistance
Citation: Cheng D, Fan J, Qin K, Zhou Y, Yang J, Ma Y, Shi M and Jin J (2021) LncRNA SNHG7 Regulates Mesenchymal Stem Cell Through the Notch1/Jagged1/Hes-1 Signaling Pathway and Influences Folfirinox Resistance in Pancreatic Cancer. Front. Oncol. 11:719855. doi: 10.3389/fonc.2021.719855
Received: 03 June 2021; Accepted: 30 July 2021;
Published: 22 September 2021.
Edited by:
Ye Wang, Qingdao University Medical College, ChinaReviewed by:
Ying Huang, Charité – Universitätsmedizin Berlin, GermanyLili Zhou, First Affiliated Hospital of Jilin University, China
Copyright © 2021 Cheng, Fan, Qin, Zhou, Yang, Ma, Shi and Jin. This is an open-access article distributed under the terms of the Creative Commons Attribution License (CC BY). The use, distribution or reproduction in other forums is permitted, provided the original author(s) and the copyright owner(s) are credited and that the original publication in this journal is cited, in accordance with accepted academic practice. No use, distribution or reproduction is permitted which does not comply with these terms.
*Correspondence: Dongfeng Cheng, cdf11426@rjh.com.cn; Jiabin Jin, jjb11501@rjh.com.cn
†These authors share first authorship