- 1Department of Thoracic Surgery, The First Affiliated Hospital of University of Science and Technology of China (USTC), Division of Life Sciences and Medicine, University of Science and Technology of China, Hefei, China
- 2Department of Thoracic Surgery, Anhui Provincial Hospital Affiliated With Anhui Medical University, Hefei, China
- 3Department of Thoracic Surgery, Anhui Provincial Hospital, Wannan Medical College, Hefei, China
- 4Department of Geriatrics, Gerontology Institute of Anhui Province, The First Affiliated Hospital of University of Science and Technology of China (USTC), Division of Life Sciences and Medicine, University of Science and Technology of China, Hefei, China
- 5Anhui Provincial Key Laboratory of Tumor Immunotherapy and Nutrition Therapy, Hefei, China
- 6Cancer Immunotherapy Center, The First Affiliated Hospital of University of Science and Technology of China (USTC), Division of Life Sciences and Medicine, University of Science and Technology of China, Hefei, China
- 7Department of Clinical Laboratory, The First Affiliated Hospital of University of Science and Technology of China (USTC), Division of Life Sciences and Medicine, University of Science and Technology of China, Hefei, China
Malignant digestive tract tumors are a great threat to human public health. In addition to surgery, immunotherapy brings hope for the treatment of these tumors. Tissue-resident memory CD8+ T (Trm) cells are a focus of tumor immunology research and treatment due to their powerful cytotoxic effects, ability to directly kill epithelial-derived tumor cells, and overall impact on maintaining mucosal homeostasis and antitumor function in the digestive tract. They are a group of noncirculating immune cells expressing adhesion and migration molecules such as CD69, CD103, and CD49a that primarily reside on the barrier epithelium of nonlymphoid organs and respond rapidly to both viral and bacterial infection and tumorigenesis. This review highlights new research exploring the role of CD8+ Trm cells in a variety of digestive tract malignant tumors, including esophageal cancer, gastric cancer, colorectal cancer, and hepatocellular carcinoma. A summary of CD8+ Trm cell phenotypes and characteristics, tissue distribution, and antitumor functions in different tumor environments is provided, illustrating how these cells may be used in immunotherapies against digestive tract tumors.
Introduction
Malignant digestive tract tumors are a great threat to human public health. According to 2020 global cancer statistics, digestive tract tumors such as esophageal cancer (EC), gastric cancer (GC), colorectal cancer (CRC), and hepatocellular carcinoma (HCC) rank in the top 10 in cancer incidence and mortality and account for 23.4% of all new cases and 36.7% of deaths (1). The gastrointestinal mucosa is prone to inflammatory lesions and tumors resulting from long-term stimulation by physical and chemical factors and microorganisms (2). When tumors occur, although innate immune cells, as the vanguard, can induce rapid effector responses, powerful adaptive immunity involving various subsets of T cells, which is then triggered, is the main force to exert antitumor roles (3). As an important member of memory T cells, the tissue-resident memory T (Trm) subset is a group of noncirculating immune cells that reside in peripheral tissues and mediate tumor defense through cytokine secretion in humans and rodents (4–6). Trm cells include CD8+ Trm cells, CD4+ Trm cells, regulatory Trm cells, natural killer Trm cells, and γδ Trm cells, in which CD8+ Trm cells are extensively studied in antitumor research due to their powerful cytotoxic activity. CD8+ Trm cells mainly reside on the barrier epithelium of nonlymphoid organs and respond rapidly to both viral and bacterial infection and tumorigenesis. In human digestive tract mucosa, CD8+ Trm cells play a key role in anti-infection and antitumor immunity because they elicit a rapid immune response after antigen stimulation (7) .
Thus, CD8+ Trm cells play an important role in maintaining homeostasis and resisting tumorigenesis within the digestive tract mucosa. By recognizing homologous antigens, CD8+ Trm cells in the tumor microenvironment (TME) can rapidly secrete cytokines to activate innate immune cells and enhance the expression of chemokines and adhesion receptors, which in turn recruit circulating immune cells needed to exert essential antitumor functions. CD8+ Trm cell infiltration is associated with improved prognosis in common digestive tract tumors, such as EC, GC, CRC, and HCC (8–11).
Many treatments for malignant digestive tract tumors have shifted from traditional chemotherapy to a combination of chemotherapy and immunotherapy. In the TME of most digestive tract cancers, CD8+ Trm cells usually show an exhausted phenotype with the expression of inhibitory immune checkpoints such as programmed cell death protein-1 (PD-1) and T cell immunoglobulin and ITIM domain (TIGIT) (12–14). Although immune checkpoint inhibitors are widely used in the treatment of digestive tract tumors, there is still a high incidence of immune-related adverse events, and many patients do not respond well to immune checkpoint inhibitors due to the absence of prognostic markers, resulting in poor therapeutic outcomes (15–17). Therefore, adequate understanding of how variations in CD8+ Trm cells in the TME affect digestive tract tumor pathogenesis is of great practical significance for clinical treatment. However, until now, the roles of CD8+ Trm cells in digestive tract tumors have not been comprehensively described.
Herein, we review recent progress in understanding of the tissue distributions, biological characteristics and antitumor mechanisms of CD8+Trm cells in EC, GC, HCC and CRC to provide directions for combined precision targeted therapy strategies and prognosis prediction.
Biological Characteristics of CD8+ Trm Cells
The Origin and Maintenance of CD8+ Trm Cells
Trm cells are differentiated from naive T cells (18). The predominant phenotypes of CD8+Trm cells express CD69, CD103, and CD49a (19–21), but do not express lymphoid homing molecules CCR7 and CD62 L and cannot be recycled (22–24). For tumor immunity, cross-priming by type 1 classical dendritic cell (cDC1) subsets, whose development and/or function depends on basic leucine zipper ATF-like transcription factor 3 (Batf3) transcription, is necessary for optimal generation of Trm cells (25–27). Indeed, Batf3-lineage DCs migrate to the draining lymph node to mediate T cell cross-priming, while another subset remains in the tumor site to produce CXCR3 ligands CXCL9 and CXCL10 (CXCL11 in humans) used to recruit CD8+ effector T cells back to the target tissue (27). After cross-priming by Batf3-driven DCs, naive T cells and central memory T (Tcm) cells can differentiate into precursor Trm (pTrm) cells that enter the blood and circulate into targeted tissues. CD69 is upregulated on pTrm cells after exposure to IFN-α released by macrophages. After reaching the upper cortex, pTrm cells express CD103 and further differentiate in response to TGF-β. Kruppel-like factor 2 (KLF2) is a transcription factor encoding sphingosine-1 phosphate receptor 1 (S1PR1) and CD62 L, two molecules critical for naive T cell recirculation (28). Competition of CD69 and S1PR1 enables T lymphocytes to reside in peripheral tissue and differentiate into Trm cells. At the same time, T cells entering the epithelial tissue upregulate CD103 and downregulate the transcription factor KLF2 in response to TGF-β, promoting the residence of CD8+ T cells (29). TNF-α and type I interferon can upregulate the expression of CD69 on the surface of CD8+ Trm cells (24). In CD103-Trm cells, the memory lymphocyte cluster (MLC) can also provide signals to maintain CD103-Trm residence (23, 24) (Figure 1).
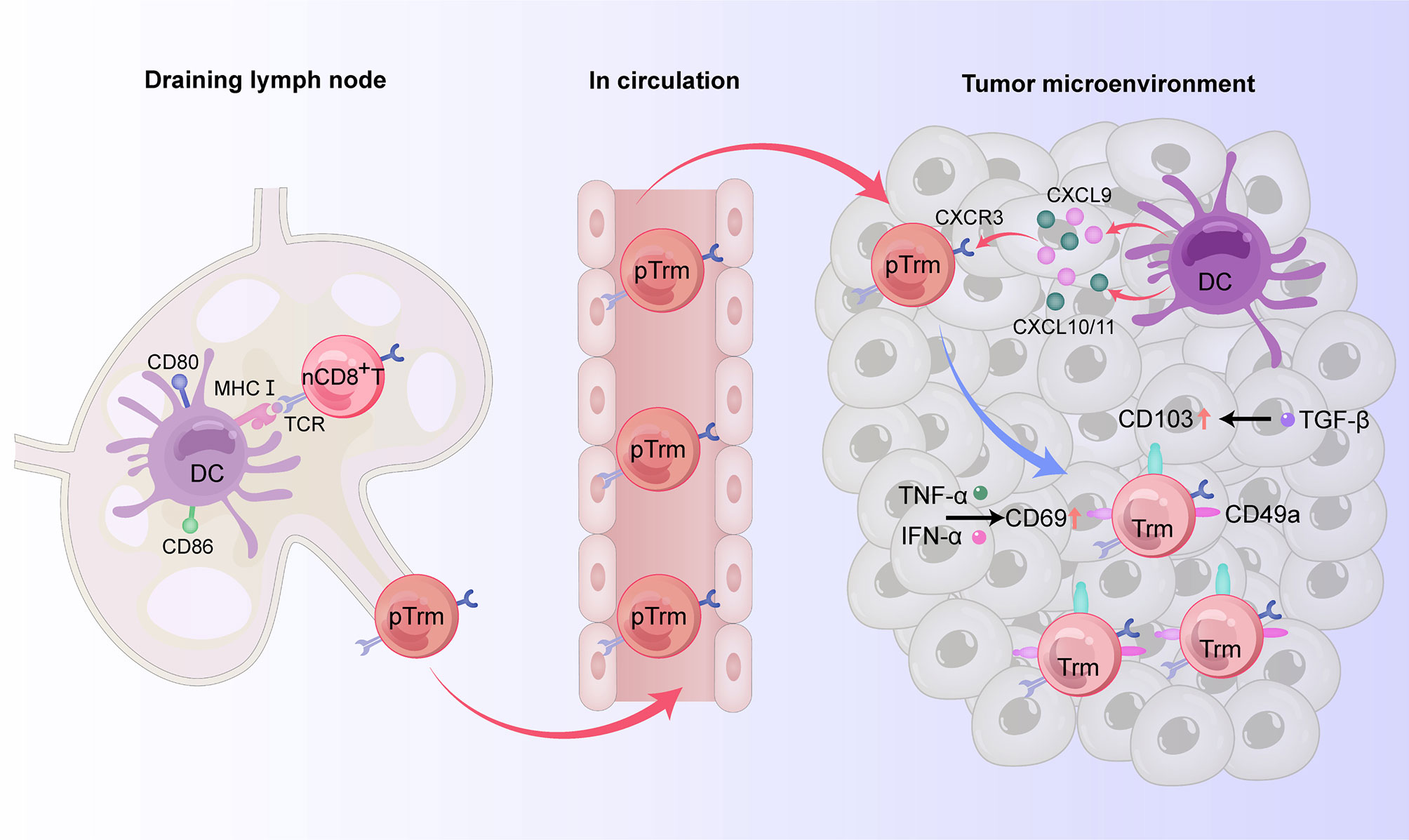
Figure 1 The origin and phenotypes of CD8+ Trm cells in human digestive tract tumors. In the draining lymph node, naive CD8+ T cells can differentiate into precursor Trm (pTrm) cells after cross-priming by Batf3-driven DCs and then enter the blood and circulate into the target tissue. By producing the CXCR3 ligands CXCL9 and CXCL10 (CXCL11 in humans), another subset of DCs remaining in the tumor site recruits pTrm cells into the tumor microenvironment. CD69 is upregulated on pTrm cells after exposure to TNF-α and IFN-α. After reaching the upper cortex, pTrm cells express CD103 and further differentiate in response to TGF-β. In addition to expressing CD69 and CD103, mature CD8+ Trm cells also express the adhesion molecule CD49a, thus possessing resident properties.
Although CD69 expression is upregulated in the early stage of Trm cell development, it cannot be used as a reliable marker of tissue residence because it is also expressed on other immune cells, and T cells expressing CD69 are still able to enter the circulation (30). CD103, also known as α E-integrin and human mucosal lymphocyte antigen, is an integrin expressed on intraepithelial T cells and some peripheral regulatory T cells. By binding to its ligand E-cadherin, CD103 can make antigen-specific T lymphocytes reside in epithelial tissue and is thus considered a reliable marker for Trm cells (23). CD49a, also known as very late antigen-1 (VLA-1), is a member of the integrin family. By binding to collagenase type IV, CD49a can prompt cells to be retained and survive in tissues (31). Furthermore, the maintenance of Trm cells in tissues is dependent on cytokines such as TNF-α, IL-15, TGF-β, and IL-33, while migration and retention are impacted by chemokines such as C-X-C motif chemokine receptor 6 (CXCR6), CCR10, and CXC chemokine ligand 17 (CXCL17) (30).
The Role of CD8+ Trm Cells in the Antitumor Immune Response
Tumor-infiltrating CD8+ T cells are effector T cells that can directly recognize and kill target cells, serving as the immune system’s frontline force against tumors. CD8+ T lymphocytes are represented by cytotoxic T lymphocyte (Tc1) subsets, which have antitumor and anti-infection functions by producing high levels of perforin, granzyme B, IFN-γ, and TNF-α (32). Of the immune cells that infiltrate the TME, the infiltration of CD8+ T lymphocytes, especially Tc1 subsets, is usually associated with a more favorable prognosis (33). The antitumor function of CD8+ T cells depends on both differentiation and transport into the TME (34). In the TME of solid tumors, factors such as abnormal chemokine secretion and tumor angiogenesis can hinder the transport and function of CD8+ T lymphocytes (35). When this occurs, CD8+ Trm cells play an extremely important role in the antitumor process (36). Among the various subsets of Trm cells, CD8+ Trm cells are considered the first line of defense for peripheral tissues to inhibit early exposed antigens and have thus received considerable attention. The response of CD8+ Trm cells to re-exposed homologous antigens in the barrier tissue is faster than the response of circulating memory T cells (37, 38), primarily as a result of the critical locations in which they reside. These regions are the most common sites exposed to pathogens such as bacteria and viruses and where epithelial cancers originate. When activated, CD8+ Trm cells can quickly release perforin and granzyme B to directly kill target cells (6, 39) and amplify the activation of a small number of cells into an organ-wide response (40). While Trm cells may have phenotypic heterogeneity based on their location in the epithelia or stroma and the tumor subtype, these cells can promote recruitment of T lymphocytes into the epithelial TME and enhance the early signal transduction of CD8+ T lymphocytes within tumors (41). During tumorigenesis, CD69+CD8+/CD103+CD8+/CD49a+CD8+ T lymphocytes are highly activated, showing better effector function than traditional CD8+ T cells, and are able to control tumor growth (42).
When persistently exposed to tumor antigens, upregulation of inhibitory receptors such as PD-1, cytotoxic T lymphocyte associated antigen-4 (CTLA-4), TIGIT, T cell immunoglobulin-and mucin-domain-containing molecule-3 (TIM3), and lymphocyte activation gene-3 (LAG3) can lead to impaired killing function and exhaustion of CD8+ T cells (43, 44). For example, as esophageal squamous cell carcinoma (ESCC) progresses, changes in the TME are accompanied by an increase in immunosuppressive cells such as regulatory T (Treg) cells, myeloid-derived suppressor cells (MDSCs), and immuno-suppressive DCs, as well as soluble inhibitory molecules such as indole-2,3 dioxygenase (IDO) (45) and fibroblast growth factor 2 (FGF2) (46), resulting in reduced infiltration and functional inhibition of CD8+ T cells (47). In recent years, it has been shown that tissue-resident T lymphocytes can overexpress PD-1 and other immune checkpoint molecules, such as TIGIT, LAG-3, and Tim-3, in some experimental animal and human tumor tissues (36, 48). There are two possibilities for this phenomenon: 1) tumor infiltrating CD8+ T lymphocytes express a variety of integrins, including CD49a, and remain in the TME in a quiescent/exhausted state, or 2) CD8+ T cells in the TME upregulate the expression of multiple integrins after exhaustion through an undetermined mechanism (Figure 2).
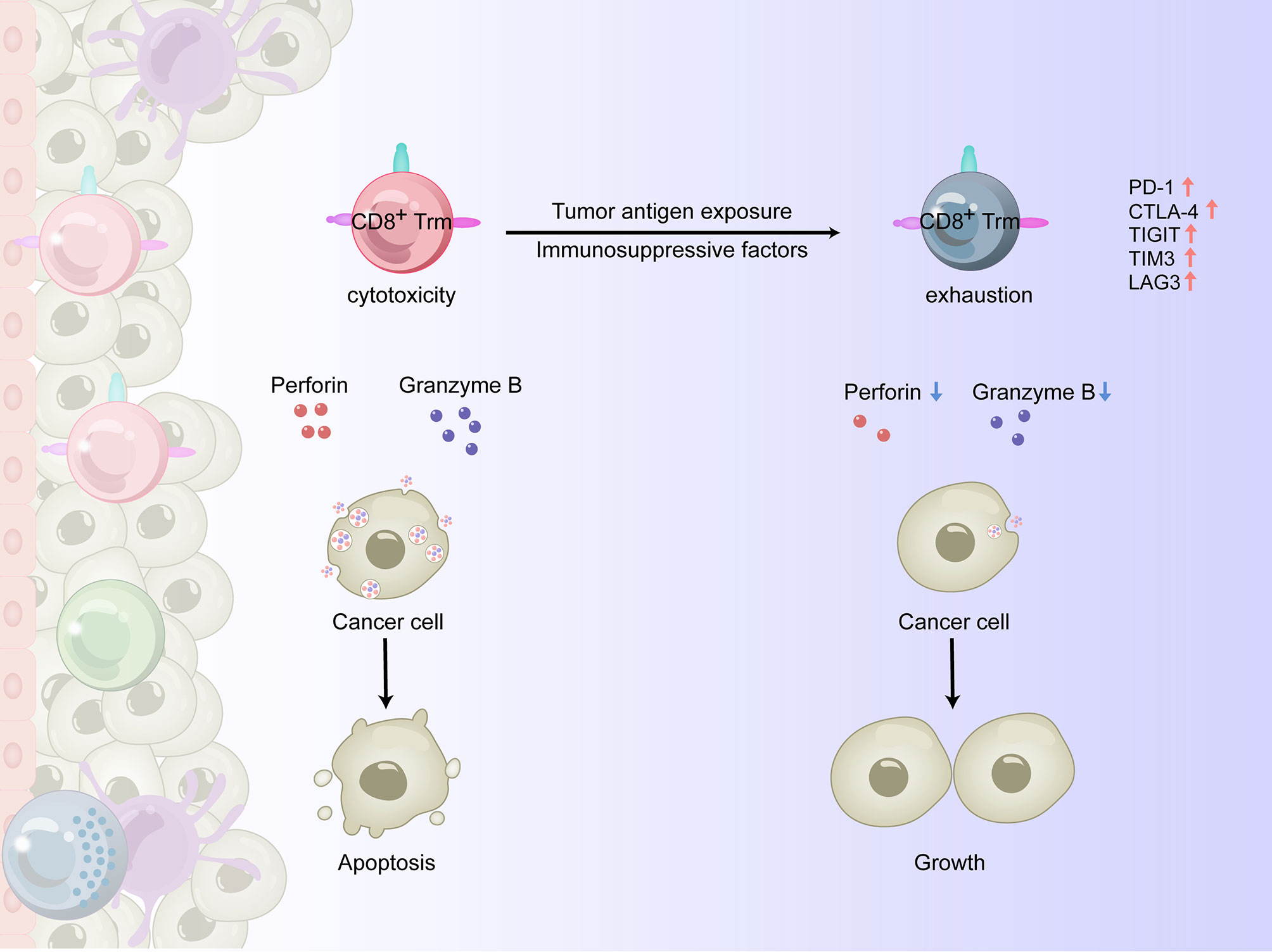
Figure 2 The antitumor effects of CD8+ Trm cells in the TME of human solid tumors. In the process of tumorigenesis, CD8+ Trm cells could be highly activated and show a higher effector function than traditional CD8+ T cells, releasing perforin and granzyme B and killing cancer cells. However, when persistently exposed to tumor antigens and immunosuppressive factors, the upregulation of inhibitory receptors such as PD-1, CTLA-4, TIGIT, TIM3 and LAG3 leads to impaired killing function and exhaustion of CD8+ Trm cells, making them unable to control tumor growth.
The following sections define the characteristics of CD8+Trm cells along with current research evaluating a role for CD8+Trm cells in antitumor therapy for four common digestive tract cancers, EC, GC, CRC, and HCC (Table 1).
Characteristics of CD8+ Trm Cells and Their Potential Use in the Treatment of Digestive Tract Cancers
CD8+ Trm Cells in EC
In 2020, EC ranked seventh in new cases and sixth among cancer-related deaths, with one in 18 deaths caused by EC (1). ESCC, which primarily occurs in Asian countries, accounts for about 90% of all pathological types of EC (63). Since it is directly exposed to foreign antigens in food, the esophageal mucosa has a special immune cell composition that plays an important role in maintaining esophageal homeostasis and mucosal anti-infective and antitumour processes. Strong expression of CD45RO, CD8, CD3, and CD107a in EC tissues indicates that there are cytotoxic memory CD8+ T cells in the stroma of these tumors (64). Although CD103+CD8+ T cells express PD-1 and TIM-3 in ESCC, they are relatively active cell subsets (12). Cells with the Trm phenotype have higher proliferation ability and express cytotoxicity-related molecules, indicating that there are highly activated antitumor subsets in CD8+ tumor infiltrating lymphocytes (TILs) in the TME.
The role of CD8+ Trm cells in EC is not well understood. Alterations in CD8+ Trm cell phenotypes and biological functions and the significance of these cells to EC prognosis and diagnosis remain obscure. Indeed, we have focused on the role of tissue-resident CD8+ T cells in EC for many years and found that CD49a, PD-1, and TIGIT molecules are highly expressed on CD8+ T cells in the TME of ESCC patients, indicating that there is also a population of tissue-resident CD8+ T cells with high expression of CD49a that shows the immune exhaustion phenotype in the ESCC TME. Multiple components of the ESCC TME can lead to immune exhaustion of CD103+CD8+ TILs, which can be repaired by αPD-1 blockers.
Clinical studies show that CD103+ CD8+ TILs are linked to the overall survival of ESCC patients (12). Thus, CD103 may be a suitable marker to evaluate the antitumor immune response of CD8+ T cells in ESCC, and infiltration of CD103+CD8+ TILs in the TME may be used as a biomarker to predict better prognosis in esophageal carcinoma (8, 12). It is worth noting that understanding the phenotype and function of CD8+ Trm cells in the occurrence and development of ESCC and exploring how best to reverse immune exhaustion and restore the antitumor function of CD8+Trm cells is an urgent issue that must be addressed by ESCC immunotherapeutic research. Establishing effective immune intervention strategies that target inhibitory molecules and reverse immune exhaustion will improve precision clinical immunotherapy for ESCC.
CD8+ Trm Cells in GC
GC is one of the most common cancers in the world. In 2020, this disease ranked fifth in morbidity, with more than one million new cases, and fourth in mortality, with an estimated 769,000 deaths (1). Helicobacter pylori infection is a major risk factor for the development of chronic gastritis to GC (65, 66), but the exact role of inflammatory components in disease progression remains unclear. Two types of gastric metaplasia, intestinal metaplasia and spasmodic cleavage peptide expression metaplasia (SPEM), are precancerous lesions of human gastric adenocarcinoma (51). The accumulation of CD8+ Trm cells in the gastric mucosa involves the regulation of absent in melanoma 2 (Aim2), one of the key components of the inflammasome. Previous studies show that the lack of Aim2 can promote the accumulation of CD8+ Trm cells in chronic inflammatory gastric mucosa by preventing CD62 L and S1PR1 function (67). While the high levels of IFN-γ produced by gastric CD8+ Trm cells can induce SPEM (68), these cells have antitumor cytotoxicity when a tumor occurs (67).
CD103+CD8+ Trm cells in GC have similar phenotypes to those in other nonlymphoid tissues, including downregulation of lymph node homing-related molecules such as CD62 L, CCR7, and T cell factor 1 (TCF-1) and upregulation of tissue inhabitation promoting molecules such as CD69, CD49a, and Runt-related transcription factor 3 (RUNX3) (20, 31, 50, 52, 69, 70). Approximately 30% of TILs in GC are CD69+CD103+ Trm cells, which highly express the inhibitory receptors PD-1, TIGIT, and CD39 (53). However, CD103+CD8+ T cells can produce high levels of cytolytic enzymes and IFN-γ in the presence of a wide variety of inhibitory receptors (9). Moreover, PD-1 blockade effectively restored the function of CD103+CD8+ T cells but not CD103-CD8+ T cells. Thus, CD103+CD8+ Trm cells represent highly activated T cell subsets in GC and play an important role in inhibiting tumors (9).
Trm cell metabolism in GC tissues does not utilize glucose but relies on fatty acid oxidation to maintain cell survival, such that loss of fatty acids results in Trm cell death. GC cells outperform Trm cells at lipid uptake and may induce Trm cell death. Targeting PD-L1 can promote the survival of Trm cells by reducing the expression of fatty acid binding protein (Fabp)4 and Fabp5 in gastric tumor cells, increasing the expression of Fabp4/5 in Trm cells, and promoting lipid uptake by Trm cells (53). Thus, metabolic reprogramming may be an effective way to prolong the life span of GC Trm cells and enhance antitumor immunity, including CD8+ Trm cell survival. In addition, B cells in the tumor can form cell masses known as tertiary lymphoid structures (TLSs), which can induce immune cells to effectively recognize and attack cancer cells. In the gastric TME, TLSs are positively correlated with tumor-infiltrating CD8+Trm cells. Studies have indicated that Trm cells may be related to the formation of TLSs, and both may improve the outcomes of targeted therapy for PD-1 inhibitors in GC (71–73).
CD8+Trm Cells in CRC
CRC ranks third in the world in incidence and second in mortality (1). As the organ with the largest interface with its environment, the gut is exposed to billions of antigens every day. The immune system needs to ensure tolerance to non-dangerous antigens and establish a strong immune response against potentially dangerous antigens (74). Immune cells are unevenly distributed in the gut. While CD8+ T cells (especially CD8+ Trm cells), monocytes, and CD19+ B cells are concentrated in the proximal colon, γδ T cells and NK cells are more abundant in the transverse colon, and CD4+ T cells and antibody-secreting cells are enriched in the distal colon and rectum (54). CD8+ T cells in the human intestinal tract are mainly Trm cells, which have CD103 and CD69 phenotypes and provide the first response to infection and tumors on the mucosal surface. TGF-β plays different roles in the formation and maintenance of Trm cells in the intestine. During secondary lymphoid organogenesis, TGF-β inhibits the migration of effector CD8+ T cells to the intestine, while during maintenance, TGF-β promotes the residence of CD8+ T cells (55). The regulatory function of Trm cells in the intestinal tract may be involved in intestinal homeostasis. It has been reported that promoting Trm and dendritic cell interactions can enhance tissue immunity, improve barrier function, and prevent microbiota-associated diseases (56). Due to the distinctiveness of the intestinal tract, CD8+ Trm cells have phenotypic and functional heterogeneity in response to infection and cancer, from pluripotent to differentiated, and show preferential protection at sites of imminent exposure to pathogens or persistent disease (75). In CRC, CD103 and CD69 are associated with immune recognition of Trm cells (57–59). CD103+CD39+CD8+ T cells have significant resident properties and tumor reactivity (10), with a unique methylome pattern in which the tumor reactivity markers CD39 and CD103 are specifically demethylated. This process provides these cells with distinct epigenetic properties (76).
CRC can be divided into microsatellite stable CRC (MSS) and high microsatellite unstable CRC (MSI-H). While tumor-infiltrating lymphocytes are abundant in MSI-H, which make up approximately 15% of CRCs, MSS CRC lacks tumor-infiltrating lymphocytes and is thus associated with a less favorable prognosis (77, 78). CD8+ Trm cell numbers were much higher in MSI-H than in MSS. Other studies show that deletion of the IL-15 gene, which is essential to maintaining intestinal Trm cells (79), is associated with poor prognosis, indicating that CD8+Trm cells play an important antitumor role in CRC. However, in MSI-H CRC, the expression of PD1 tended to increase in CD8+Trm cells, indicating that checkpoint inhibition therapy targeting Trm cells in MSI-H CRC may be of great significance (79).
CD8+ Trm Cells in HCC
In 2020, primary HCC was the sixth most frequently diagnosed cancer, with more than 900,000 new cases, and the third leading cause of cancer mortality, with 830,000 deaths (1). This malignant tumor usually occurs in chronic inflammatory liver disease, such as fibrosis or cirrhosis, and is associated with certain risk factors, including hepatitis B virus (HBV), hepatitis C virus (HCV), alcohol abuse, and metabolic diseases (80, 81). Increased infiltration of cytotoxic T, NK, and NKT cells in the liver plays an active antitumor role in primary HCC. To avoid unnecessary activation of innate immune cells during continuous exposure to food and microbial-derived antigens, the liver needs to maintain a relatively immunotolerant environment. When immunogenic stimulation occurs, liver CD103+ dendritic cells express high levels of MHC-II, CD80 and CD86, which result in massive activation of CD8+ T cells (82). For example, HBV induces IFNγ+CD8+ T cells to upregulate CD69 and CD103 and induces liver CD8+ T cells to show the Trm phenotype in situ (83). The presence of T cells and cytotoxic cells in TILs correlates with a favorable prognosis of patients with HCC. More than 50% of these tumor-infiltrating lymphocytes express CD69 (84), and about 20-30% are positive for CD103, thus showing resident characteristics. However, unlike other tumors, only about 5% of human hepatic CD69+CD8+ T cells express CD103 (85). Recent studies have shown that hepatic CD8+ Trm cells adhere to the liver via LFA-1, and the residence of CD8+ T cells in the hepatic sinusoid depends on the LFA-1-I/CAM-1 interaction (86). However, chronic tumor antigen stimulation and immunosuppressive cells and their production in the TME can put Trm cells into a “dysfunctional state”. Targeting immune checkpoint molecules such as PD-1, TIM-3, LAG-3, and CTLA-4 can restore the dysfunction of Trm cells (87). However, hepatic CD8+ Trm cells are a unique population with low cytotoxicity (60), which may be related to the immunotolerant ecological properties of the liver. Thus, anti-PD-L1 or anti-PD-1 alone may not restore this dysfunction, and other agents, such as IL-2, may have a synergistic effect in improving the antitumor immunity of CD8+ Trm cells in HCC (87). In addition, the development and maintenance of tumor-specific CD8+ Trm cells induced by adenoviral vector immunization vaccine in the liver can provide long-term protection for human papillomavirus-like virus (HPV)-induced HCC and can enhance the formation of CD8+ Trm cells by targeting CTLA-4 (61). Thus, CD8+ Trm cells may also play an active role in tumor vaccine therapy for HCC.
Application of CD8+ Trm Cells in Cancer Immunotherapy
The exhaustion phenotype of CD8+Trm cells in the TME does not prevent antitumor activity from being reactivated. In vitro studies of CD103+CD8+ T cells with high expression of PD-1 in lung cancer have shown that blocking the expression of PD-1 on these immune cells can restore their cytotoxicity against autologous tumor cells, suggesting that anti-PD-1 therapy may restore the killing function of CD8+ Trm cells toward autologous tumors (62). In the last few decades, anti-PD-1/PD-L1 therapies have shown remarkable efficacy in patients with malignant gastrointestinal neoplasms. For instance, the international randomized phase III KEYNOTE-181 and KEYNOTE-590 studies in EC patients showed that pembrolizumab provided a clinically meaningful overall survival (OS) benefit versus the control group (88, 89). Indeed, clinically meaningful improvements in overall response rate (ORR), progression-free survival and OS were observed in GC patients treated with pembrolizumab plus chemotherapy in the KEYNOTE-059 and KEYNOTE-062 trials (90, 91). However, although anti-PD-1 mAb is a promising approach for advanced GC patients, the response rate is still limited, with an ORR of only about 12.0% and a disease control ratio of about 34.7% (92). Although immunotherapy has produced durable responses in MSI-H CRC, with recent FDA approval of pembrolizumab in the first-line setting of metastatic CRC (93), MSS CRC has long been considered resistant to PD-1/PD-L1 blockade. However, combination therapy, such as co-inhibition of anti-PD-1 and STAT3 or regorafenib, a small molecule tyrosine kinase inhibitor, can elicit an effective antitumor response in a small subset of MSS CRC patients (49, 94). Disappointingly, the ORR of checkpoint inhibitors in HCC patients is only 15-20% (95). Recently, the Nivolumab (CheckMate-459) III phase trial failed to meet the primary endpoint, so an effective immunosuppressive therapy against HCC is still lacking (96).
There is no denying that the use of PD-1 inhibitors to reverse the exhaustion of immune cells such as CD8+ Trm cells, alone or with other checkpoint antibodies, has had controversial results. Due to tumor heterogeneity, a lack of reproducibility of results, and a complex scoring system, PD-L1 is not suitable as a predictive biomarker (97). While methods such as the combined positive score, which detects PD-L1 levels in tumors and lymphocytes, can be used clinically to evaluate patient response to PD-1/PD-L1-related inhibitors, their specificity for evaluating therapeutic impact is poor (98). Therefore, treatment options for patients with unresectable, locally advanced, or metastatic esophageal cancer are still limited, requiring the search for new predictive indicators and immunotherapy strategies (99).
Another way to increase the number of functional CD8+ Trm cells in tumors is by inducing their expansion using tumor vaccines. Studies demonstrate that vaccination can induce Trm cells in the tissue after natural infection and vaccination. For example, intravaginal immunization or systemic perfusion has been shown to boost vaginal mucosa by inducing Trm cells in the reproductive area (100). In addition, encoding respiratory syncytial virus mechanisms or recombinant cytomegalovirus vectors of Bacille Calmette-Guerin vaccine proteins for intranasal vaccination promotes immune cells to develop resident properties (101, 102). The vaccine-specific CD8+ T cell response can provide long-term protection against HPV-induced skin cancer and HCC but is dependent on the induction and accumulation of CD8+ Trm cells by blocking CTLA-4 early after immunization (61). Local radiotherapy by vaccination (103), which changes the expression of selectin, integrin, and chemokines, can also enhance the recruitment of resident CD8+ T lymphocytes in the tissue and tumor site.
Perspective
CD8+ Trm cell infiltration plays a critical role in the antitumor immune response in the digestive tract. CD69, CD103, CD39, and CD49a are the key biomarkers of tumor-reactive CD8+ Trm cells and can be used as prognostic molecules for different digestive tract tumors (57, 59). However, CD8+ Trm cells that have infiltrated digestive tract tumors can also express immune checkpoint molecules such as PD-1, CTLA-4, TIGIT, TIM3, and LAG3, which can damage their killing function and cause immune exhaustion (104, 105). While targeted application of immune checkpoint inhibitors has achieved good results, the lack of immune markers and disparate responses to immune checkpoint inhibitors diminish the efficacy of treatment. Determining how best to increase the number and function of tumor-associated CD8+ Trm cells helps to maximize antitumor immunity. There is also great diversity among CD8+Trm cell phenotypes found in different digestive tract organs. For example, while PD-1hi CD8+ Trm cells highly express cell adhesion and tissue positioning markers, including CD69 and integrins CD11c, CD49a, CD49b, and CD103 in HCC (87), CD103+CD8+ Trm cells express tissue residency-promoting molecules, such as CD69, CD49a, and RUNX3, in gastric cancer (9). CD103 is an important marker of CD8+ Trm in ESCC. ESCC patients with co-expression of PD-L1/TIM3 or PD-L1/TIGIT in CD8+ Trm cells have a lower survival rate than those expressing either marker alone (106). This may explain why only a small number of ECC patients benefit from treatment with PD-1 inhibitors. The absence of predictive indicators results in a high rate of immune-related adverse events in response to drugs targeting PD-1/PD-L1, with only a small number of patients showing positive outcomes. Nevertheless, a novel strategy to solve this problem is developing nanodrug delivery systems with a high drug loading capacity and targeting ability. It has been reported that biodegradable polymers such as poly (ursolic acid) are used as drug carriers for treating CRC and other cancers. The anticancer drug effectively loaded into poly(salicylic acid) nanoparticles shows ultrahigh blood vessel penetration, tumor penetration, and tumor accumulation due to the special prickly nanostructure (107, 108). Thus, the combination of a therapeutic polymer platform and immunotherapy to achieve precise targeted therapy may be a new attractive therapeutic strategy for treating digestive tract cancer.
In conclusion, alimentary tract neoplasms are a serious threat to human health. Immunotherapy for digestive tract tumors still has many problems, including blind treatment, side effects, and disparate individual responses. CD8+ Trm cells exist in various digestive tract tumors and are closely related to disease prognosis. However, current research on the utilization of CD8+ Trm cells in digestive tract tumors is still in the early stages. Thus, a comprehensive understanding of CD8+ Trm cell phenotypes and the characteristics of corresponding immune checkpoint molecules that are expressed in digestive tract tumors will be important to help guide accurate diagnosis and treatment of different tumor types. Specific drug therapy and tumor vaccine therapy that targets tumor-associated CD8+ Trm cells may become an important direction for antitumor research and tumor precision therapy.
Author Contributions
XM performed the study design and drafted the manuscript. HL and XZ participated in the manuscript writing. MC obtained the funding, participated in the paper design, and contributed fruitful discussions. KC conceived the study and participated in the paper design and writing. All authors contributed to the article and approved the submitted version.
Funding
The study was supported by Natural Science Foundation of Anhui Province, China (1708085MH182).
Conflict of Interest
The authors declare that the research was conducted in the absence of any commercial or financial relationships that could be construed as a potential conflict of interest.
The editor YM declared a shared parent affiliation with the author(s) XM, MC, KC at the time of the review.
Publisher’s Note
All claims expressed in this article are solely those of the authors and do not necessarily represent those of their affiliated organizations, or those of the publisher, the editors and the reviewers. Any product that may be evaluated in this article, or claim that may be made by its manufacturer, is not guaranteed or endorsed by the publisher.
Glossary
References
1. Sung H, Ferlay J, Siegel RL, Laversanne M, Soerjomataram I, Jemal A, et al. Global Cancer Statistics 2020: GLOBOCAN Estimates of Incidence and Mortality Worldwide for 36 Cancers in 185 Countries. CA Cancer J Clin (2021) 71(3):209–49. doi: 10.3322/caac.21660
2. Rao D, Parakrama R, Augustine T, Liu Q, Goel S, Maitra R. Immunotherapeutic Advances in Gastrointestinal Malignancies. NPJ Precis Oncol (2019) 3:4. doi: 10.1038/s41698-018-0076-8
3. Kather JN, Halama N. Harnessing the Innate Immune System and Local Immunological Microenvironment to Treat Colorectal Cancer. Br J Cancer (2019) 120(9):871–82. doi: 10.1038/s41416-019-0441-6
4. Mami-Chouaib F, Tartour E. Editorial: Tissue Resident Memory T Cells. Front Immunol (2019) 10:1018. doi: 10.3389/fimmu.2019.01018
5. Hogan RJ, Usherwood EJ, Zhong W, Roberts AA, Dutton RW, Harmsen AG, et al. Activated Antigen-Specific CD8+ T Cells Persist in the Lungs Following Recovery From Respiratory Virus Infections. J Immunol (2001) 166(3):1813–22. doi: 10.4049/jimmunol.166.3.1813
6. Masopust D, Vezys V, Marzo AL, Lefrancois L. Preferential Localization of Effector Memory Cells in Nonlymphoid Tissue. Science (2001) 291(5512):2413–7. doi: 10.1126/science.1058867
7. Mora-Buch R, Bromley SK. Discipline in Stages: Regulating CD8(+) Resident Memory T Cells. Front Immunol (2020) 11:624199. doi: 10.3389/fimmu.2020.624199
8. Chu Y, Liao J, Li J, Wang Y, Yu X, Wang J, et al. CD103(+) Tumor-Infiltrating Lymphocytes Predict Favorable Prognosis in Patients With Esophageal Squamous Cell Carcinoma. J Cancer (2019) 10(21):5234–43. doi: 10.7150/jca.30354
9. Li R, Liu H, Cao Y, Wang J, Chen Y, Qi Y, et al. Identification and Validation of an Immunogenic Subtype of Gastric Cancer With Abundant Intratumoural CD103(+)CD8(+) T Cells Conferring Favourable Prognosis. Br J Cancer (2020) 122(10):1525–34. doi: 10.1038/s41416-020-0813-y
10. de Vries NL, van Unen V, Ijsselsteijn ME, Abdelaal T, van der Breggen R, Farina Sarasqueta A, et al. High-Dimensional Cytometric Analysis of Colorectal Cancer Reveals Novel Mediators of Antitumour Immunity. Gut (2020) 69(4):691–703. doi: 10.1136/gutjnl-2019-318672
11. Lim CJ, Lee YH, Pan L, Lai L, Chua C, Wasser M, et al. Multidimensional Analyses Reveal Distinct Immune Microenvironment in Hepatitis B Virus-Related Hepatocellular Carcinoma. Gut (2019) 68(5):916–27. doi: 10.1136/gutjnl-2018-316510
12. Han L, Gao QL, Zhou XM, Shi C, Chen GY, Song YP, et al. Characterization of CD103(+) CD8(+) Tissue-Resident T Cells in Esophageal Squamous Cell Carcinoma: May be Tumor Reactive and Resurrected by Anti-PD-1 Blockade. Cancer Immunol Immunother (2020) 69(8):1493–504. doi: 10.1007/s00262-020-02562-3
13. Lee LH, Cavalcanti MS, Segal NH, Hechtman JF, Weiser MR, Smith JJ, et al. Patterns and Prognostic Relevance of PD-1 and PD-L1 Expression in Colorectal Carcinoma. Mod Pathol (2016) 29(11):1433–42. doi: 10.1038/modpathol.2016.139
14. Zhou X, Ding X, Li H, Yang C, Ma Z, Xu G, et al. Upregulation of TIGIT and PD-1 in Colorectal Cancer With Mismatch-Repair Deficiency. Immunol Invest (2021) 50(4):338–55. doi: 10.1080/08820139.2020.1758130
15. Esfahani K, Buhlaiga N, Thebault P, Lapointe R, Johnson NA, Miller WH Jr. Alemtuzumab for Immune-Related Myocarditis Due to PD-1 Therapy. N Engl J Med (2019) 380(24):2375–6. doi: 10.1056/NEJMc1903064
16. McElduff A. The Effect Of Pd-1 Inhibitors on Endocrine Function. Endocr Pract (2017) 23(2):239–40. doi: 10.4158/1934-2403-23.2.239
17. Kanz BA, Pollack MH, Johnpulle R, Puzanov I, Horn L, Morgans A, et al. Safety and Efficacy of Anti-PD-1 in Patients With Baseline Cardiac, Renal, or Hepatic Dysfunction. J Immunother Cancer (2016) 4:60. doi: 10.1186/s40425-016-0166-5
18. Budd RC, Cerottini JC, MacDonald HR. Phenotypic Identification of Memory Cytolytic T Lymphocytes in a Subset of Lyt-2+ Cells. J Immunol (1987) 138(4):1009–13.
19. Cepek KL, Shaw SK, Parker CM, Russell GJ, Morrow JS, Rimm DL, et al. Adhesion Between Epithelial Cells and T Lymphocytes Mediated by E-Cadherin and the Alpha E Beta 7 Integrin. Nature (1994) 372(6502):190–3. doi: 10.1038/372190a0
20. Mackay LK, Braun A, Macleod BL, Collins N, Tebartz C, Bedoui S, et al. Cutting Edge: CD69 Interference With Sphingosine-1-Phosphate Receptor Function Regulates Peripheral T Cell Retention. J Immunol (2015) 194(5):2059–63. doi: 10.4049/jimmunol.1402256
21. Meharra EJ, Schon M, Hassett D, Parker C, Havran W, Gardner H. Reduced Gut Intraepithelial Lymphocytes in VLA1 Null Mice. Cell Immunol (2000) 201(1):1–5. doi: 10.1006/cimm.2000.1630
22. Casey KA, Fraser KA, Schenkel JM, Moran A, Abt MC, Beura LK, et al. Antigen-Independent Differentiation and Maintenance of Effector-Like Resident Memory T Cells in Tissues. J Immunol (2012) 188(10):4866–75. doi: 10.4049/jimmunol.1200402
23. Iijima N, Iwasaki A. Tissue Instruction for Migration and Retention of TRM Cells. Trends Immunol (2015) 36(9):556–64. doi: 10.1016/j.it.2015.07.002
24. Park CO, Kupper TS. The Emerging Role of Resident Memory T Cells in Protective Immunity and Inflammatory Disease. Nat Med (2015) 21(7):688–97. doi: 10.1038/nm.3883
25. Enamorado M, Khouili SC, Iborra S, Sancho D. Genealogy, Dendritic Cell Priming, and Differentiation of Tissue-Resident Memory CD8(+) T Cells. Front Immunol (2018) 9:1751. doi: 10.3389/fimmu.2018.01751
26. Seillet C, Jackson JT, Markey KA, Brady HJ, Hill GR, Macdonald KP, et al. CD8alpha+ DCs Can Be Induced in the Absence of Transcription Factors Id2, Nfil3, and Batf3. Blood (2013) 121(9):1574–83. doi: 10.1182/blood-2012-07-445650
27. Spranger S, Dai D, Horton B, Gajewski TF. Tumor-Residing Batf3 Dendritic Cells Are Required for Effector T Cell Trafficking and Adoptive T Cell Therapy. Cancer Cell (2017) 31(5):711–23.e714. doi: 10.1016/j.ccell.2017.04.003
28. Carlson CM, Endrizzi BT, Wu J, Ding X, Weinreich MA, Walsh ER, et al. Kruppel-Like Factor 2 Regulates Thymocyte and T-Cell Migration. Nature (2006) 442(7100):299–302. doi: 10.1038/nature04882
29. Skon CN, Lee JY, Anderson KG, Masopust D, Hogquist KA, Jameson SC. Transcriptional Downregulation of S1pr1 Is Required for the Establishment of Resident Memory CD8+ T Cells. Nat Immunol (2013) 14(12):1285–93. doi: 10.1038/ni.2745
30. Sun H, Sun C, Xiao W, Sun R. Tissue-Resident Lymphocytes: From Adaptive to Innate Immunity. Cell Mol Immunol (2019) 16(3):205–15. doi: 10.1038/s41423-018-0192-y
31. Richter MV, Topham DJ. The Alpha1beta1 Integrin and TNF Receptor II Protect Airway CD8+ Effector T Cells From Apoptosis During Influenza Infection. J Immunol (2007) 179(8):5054–63. doi: 10.4049/jimmunol.179.8.5054
32. St Paul M, Ohashi PS. The Roles of CD8(+) T Cell Subsets in Antitumor Immunity. Trends Cell Biol (2020) 30(9):695–704. doi: 10.1016/j.tcb.2020.06.003
33. Fridman WH, Pages F, Sautes-Fridman C, Galon J. The Immune Contexture in Human Tumours: Impact on Clinical Outcome. Nat Rev Cancer (2012) 12(4):298–306. doi: 10.1038/nrc3245
34. Nolz JC. Molecular Mechanisms of CD8(+) T Cell Trafficking and Localization. Cell Mol Life Sci (2015) 72(13):2461–73. doi: 10.1007/s00018-015-1835-0
35. Bellone M, Calcinotto A. Ways to Enhance Lymphocyte Trafficking Into Tumors and Fitness of Tumor Infiltrating Lymphocytes. Front Oncol (2013) 3231:231. doi: 10.3389/fonc.2013.00231
36. Mami-Chouaib F, Blanc C, Corgnac S, Hans S, Malenica I, Granier C, et al. Resident Memory T Cells, Critical Components in Tumor Immunology. J Immunother Cancer (2018) 6(1):87. doi: 10.1186/s40425-018-0399-6
37. Jiang X, Clark RA, Liu L, Wagers AJ, Fuhlbrigge RC, Kupper TS. Skin Infection Generates non-Migratory Memory CD8+ T(RM) Cells Providing Global Skin Immunity. Nature (2012) 483(7388):227–31. doi: 10.1038/nature10851
38. Steinbach K, Vincenti I, Kreutzfeldt M, Page N, Muschaweckh A, Wagner I, et al. Brain-Resident Memory T Cells Represent an Autonomous Cytotoxic Barrier to Viral Infection. J Exp Med (2016) 213(8):1571–87. doi: 10.1084/jem.20151916
39. Cheuk S, Schlums H, Gallais Serezal I, Martini E, Chiang SC, Marquardt N, et al. CD49a Expression Defines Tissue-Resident CD8(+) T Cells Poised for Cytotoxic Function in Human Skin. Immunity (2017) 46(2):287–300. doi: 10.1016/j.immuni.2017.01.009
40. Ariotti S, Hogenbirk MA, Dijkgraaf FE, Visser LL, Hoekstra ME, Song JY, et al. T Cell Memory. Skin-Resident Memory CD8(+) T Cells Trigger a State of Tissue-Wide Pathogen Alert. Science (2014) 346(6205):101–5. doi: 10.1126/science.1254803
41. Boutet M, Gauthier L, Leclerc M, Gros G, de Montpreville V, Theret N, et al. TGFbeta Signaling Intersects With CD103 Integrin Signaling to Promote T-Lymphocyte Accumulation and Antitumor Activity in the Lung Tumor Microenvironment. Cancer Res (2016) 76(7):1757–69. doi: 10.1158/0008-5472.CAN-15-1545
42. Ganesan AP, Clarke J, Wood O, Garrido-Martin EM, Chee SJ, Mellows T, et al. Tissue-Resident Memory Features Are Linked to the Magnitude of Cytotoxic T Cell Responses in Human Lung Cancer. Nat Immunol (2017) 18(8):940–50. doi: 10.1038/ni.3775
43. Verdeil G, Fuertes Marraco SA, Murray T, Speiser DE. From T Cell "Exhaustion" to Anti-Cancer Immunity. Biochim Biophys Acta (2016) 1865(1):49–57. doi: 10.1016/j.bbcan.2015.06.007
44. Wherry EJ, Kurachi M. Molecular and Cellular Insights Into T Cell Exhaustion. Nat Rev Immunol (2015) 15(8):486–99. doi: 10.1038/nri3862
45. Zhang G, Liu WL, Zhang L, Wang JY, Kuang MH, Liu P, et al. Involvement of Indoleamine 2,3-Dioxygenase in Impairing Tumor-Infiltrating CD8 T-Cell Functions in Esophageal Squamous Cell Carcinoma. Clin Dev Immunol (2011) 2011:384726. doi: 10.1155/2011/384726
46. Chen QY, Li YN, Wang XY, Zhang X, Hu Y, Li L, et al. Tumor Fibroblast-Derived FGF2 Regulates Expression of SPRY1 in Esophageal Tumor-Infiltrating T Cells and Plays a Role in T-Cell Exhaustion. Cancer Res (2020) 80(24):5583–96. doi: 10.1158/0008-5472.CAN-20-1542
47. Yang L, Zhang Y. Tumor-Associated Macrophages, Potential Targets for Cancer Treatment. biomark Res (2017) 5:25. doi: 10.1186/s40364-017-0106-7
48. Melssen MM, Olson W, Wages NA, Capaldo BJ, Mauldin IS, Mahmutovic A, et al. Formation and Phenotypic Characterization of CD49a, CD49b and CD103 Expressing CD8 T Cell Populations in Human Metastatic Melanoma. Oncoimmunology (2018) 7(10):e1490855. doi: 10.1080/2162402X.2018.1490855
49. Wang C, Chevalier D, Saluja J, Sandhu J, Lau C, Fakih M. Regorafenib and Nivolumab or Pembrolizumab Combination and Circulating Tumor DNA Response Assessment in Refractory Microsatellite Stable Colorectal Cancer. Oncologist (2020) 25(8):e1188–94. doi: 10.1634/theoncologist.2020-0161
50. Zhou X, Yu S, Zhao DM, Harty JT, Badovinac VP, Xue HH. Differentiation and Persistence of Memory CD8(+) T Cells Depend on T Cell Factor 1. Immunity (2010) 33(2):229–40. doi: 10.1016/j.immuni.2010.08.002
51. Schmidt PH, Lee JR, Joshi V, Playford RJ, Poulsom R, Wright NA, et al. Identification of a Metaplastic Cell Lineage Associated With Human Gastric Adenocarcinoma. Lab Invest (1999) 79(6):639–46.
52. Milner JJ, Toma C, Yu B, Zhang K, Omilusik K, Phan AT, et al. Runx3 Programs CD8(+) T Cell Residency in non-Lymphoid Tissues and Tumours. Nature (2017) 552(7684):253–7. doi: 10.1038/nature24993
53. Lin R, Zhang H, Yuan Y, He Q, Zhou J, Li S, et al. Fatty Acid Oxidation Controls CD8(+) Tissue-Resident Memory T-Cell Survival in Gastric Adenocarcinoma. Cancer Immunol Res (2020) 8(4):479–92. doi: 10.1158/2326-6066.CIR-19-0702
54. Tyler CJ, Guzman M, Lundborg LR, Yeasmin S, Perez-Jeldres T, Yarur A, et al. Inherent Immune Cell Variation Within Colonic Segments Presents Challenges for Clinical Trial Design. J Crohns Colitis (2020) 14(10):1364–77. doi: 10.1093/ecco-jcc/jjaa067
55. Zhang N, Bevan MJ. Transforming Growth Factor-Beta Signaling Controls the Formation and Maintenance of Gut-Resident Memory T Cells by Regulating Migration and Retention. Immunity (2013) 39(4):687–96. doi: 10.1016/j.immuni.2013.08.019
56. Noble A, Durant L, Hoyles L, McCartney AL, Man R, Segal J, et al. Deficient Resident Memory T Cell and CD8 T Cell Response to Commensals in Inflammatory Bowel Disease. J Crohns Colitis (2020) 14(4):525–37. doi: 10.1093/ecco-jcc/jjz175
57. Corgnac S, Boutet M, Kfoury M, Naltet C, Mami-Chouaib F. The Emerging Role of CD8(+)(TRM) Cells in Antitumor Immunity: A Unique Functional Contribution of the CD103 Integrin. Front Immunol (2018) 9:1904. doi: 10.3389/fimmu.2018.01904
58. Duhen T, Duhen R, Montler R, Moses J, Moudgil T, de Miranda NF, et al. Co-Expression of CD39 and CD103 Identifies Tumor-Reactive CD8 T Cells in Human Solid Tumors. Nat Commun (2018) 9(1):2724. doi: 10.1038/s41467-018-05072-0
59. Gallerano D, Ciminati S, Grimaldi A, Piconese S, Cammarata I, Focaccetti C, et al. Genetically Driven CD39 Expression Shapes Human Tumor-Infiltrating CD8(+) T-Cell Functions. Int J Cancer (2020) 147(9):2597–610. doi: 10.1002/ijc.33131
60. Ma J, Zheng B, Goswami S, Meng L, Zhang D, Cao C, et al. PD1(Hi) CD8(+) T Cells Correlate With Exhausted Signature and Poor Clinical Outcome in Hepatocellular Carcinoma. J Immunother Cancer (2019) 7(1):331. doi: 10.1186/s40425-019-0814-7
61. van der Gracht ET, Schoonderwoerd MJ, van Duikeren S, Yilmaz AN, Behr FM, Colston JM, et al. Adenoviral Vaccines Promote Protective Tissue-Resident Memory T Cell Populations Against Cancer. J Immunother Cancer (2020) 8(2):e001133. doi: 10.1136/jitc-2020-001133
62. Djenidi F, Adam J, Goubar A, Durgeau A, Meurice G, de Montpreville V, et al. CD8+CD103+ Tumor-Infiltrating Lymphocytes are Tumor-Specific Tissue-Resident Memory T Cells and a Prognostic Factor for Survival in Lung Cancer Patients. J Immunol (2015) 194(7):3475–86. doi: 10.4049/jimmunol.1402711
63. Stoner GD, Gupta A. Etiology and Chemoprevention of Esophageal Squamous Cell Carcinoma. Carcinogenesis (2001) 22(11):1737–46. doi: 10.1093/carcin/22.11.1737
64. Conroy MJ, Kennedy SA, Doyle SL, Hayes B, Kavanagh M, van der Stok EP, et al. A Study of the Immune Infiltrate and Patient Outcomes in Esophageal Cancer. Carcinogenesis (2021) 42(3):395–404. doi: 10.1093/carcin/bgaa101
65. Plummer M, Franceschi S, Vignat J, Forman D, de Martel C. Global Burden of Gastric Cancer Attributable to Helicobacter Pylori. Int J Cancer (2015) 136(2):487–90. doi: 10.1002/ijc.28999
66. Fox JG, Wang TC. Inflammation, Atrophy, and Gastric Cancer. J Clin Invest (2007) 117(1):60–9. doi: 10.1172/JCI30111
67. El-Zaatari M, Bishu S, Zhang M, Grasberger H, Hou G, Haley H, et al. Aim2-Mediated/IFN-Beta-Independent Regulation of Gastric Metaplastic Lesions via CD8+ T Cells. JCI Insight (2020) 5(5):e94035. doi: 10.1172/jci.insight.94035
68. Syu LJ, El-Zaatari M, Eaton KA, Liu Z, Tetarbe M, Keeley TM, et al. Transgenic Expression of Interferon-Gamma in Mouse Stomach Leads to Inflammation, Metaplasia, and Dysplasia. Am J Pathol (2012) 181(6):2114–25. doi: 10.1016/j.ajpath.2012.08.017
69. Bromley SK, Thomas SY, Luster AD. Chemokine Receptor CCR7 Guides T Cell Exit From Peripheral Tissues and Entry Into Afferent Lymphatics. Nat Immunol (2005) 6(9):895–901. doi: 10.1038/ni1240
70. Hombrink P, Helbig C, Backer RA, Piet B, Oja AE, Stark R, et al. Programs for the Persistence, Vigilance and Control of Human CD8(+) Lung-Resident Memory T Cells. Nat Immunol (2016) 17(12):1467–78. doi: 10.1038/ni.3589
71. Mori T, Tanaka H, Suzuki S, Deguchi S, Yamakoshi Y, Yoshii M, et al. Tertiary Lymphoid Structures Show Infiltration of Effective Tumor-Resident T Cells in Gastric Cancer. Cancer Sci (2021) 112(5):1746–57. doi: 10.1111/cas.14888
72. Edwards J, Wilmott JS, Madore J, Gide TN, Quek C, Tasker A, et al. CD103(+) Tumor-Resident CD8(+) T Cells Are Associated With Improved Survival in Immunotherapy-Naive Melanoma Patients and Expand Significantly During Anti-PD-1 Treatment. Clin Cancer Res (2018) 24(13):3036–45. doi: 10.1158/1078-0432.CCR-17-2257
73. Cabrita R, Lauss M, Sanna A, Donia M, Skaarup Larsen M, Mitra S, et al. Tertiary Lymphoid Structures Improve Immunotherapy and Survival in Melanoma. Nature (2020) 577(7791):561–5. doi: 10.1038/s41586-019-1914-8
74. Paap EM, Muller TM, Sommer K, Neurath MF, Zundler S. Total Recall: Intestinal TRM Cells in Health and Disease. Front Immunol (2020) 11623072:623072. doi: 10.3389/fimmu.2020.623072
75. Milner JJ, Toma C, He Z, Kurd NS, Nguyen QP, McDonald B, et al. Heterogenous Populations of Tissue-Resident CD8(+) T Cells Are Generated in Response to Infection and Malignancy. Immunity (2020) 52(5):808–824 e807. doi: 10.1016/j.immuni.2020.04.007
76. Yang R, Cheng S, Luo N, Gao R, Yu K, Kang B, et al. Distinct Epigenetic Features of Tumor-Reactive CD8+ T Cells in Colorectal Cancer Patients Revealed by Genome-Wide DNA Methylation Analysis. Genome Biol (2019) 21(1):2. doi: 10.1186/s13059-019-1921-y
77. Popat S, Hubner R, Houlston RS. Systematic Review of Microsatellite Instability and Colorectal Cancer Prognosis. J Clin Oncol (2005) 23(3):609–18. doi: 10.1200/JCO.2005.01.086
78. Guastadisegni C, Colafranceschi M, Ottini L, Dogliotti E. Microsatellite Instability as a Marker of Prognosis and Response to Therapy: A Meta-Analysis of Colorectal Cancer Survival Data. Eur J Cancer (2010) 46(15):2788–98. doi: 10.1016/j.ejca.2010.05.009
79. Mlecnik B, Bindea G, Angell HK, Sasso MS, Obenauf AC, Fredriksen T, et al. Functional Network Pipeline Reveals Genetic Determinants Associated With in Situ Lymphocyte Proliferation and Survival of Cancer Patients. Sci Transl Med (2014) 6(228):228ra237. doi: 10.1126/scitranslmed.3007240
80. Zheng C, Zheng L, Yoo JK, Guo H, Zhang Y, Guo X, et al. Landscape of Infiltrating T Cells in Liver Cancer Revealed by Single-Cell Sequencing. Cell (2017) 169(7):1342–56.e1316. doi: 10.1016/j.cell.2017.05.035
81. Sia D, Jiao Y, Martinez-Quetglas I, Kuchuk O, Villacorta-Martin C, Castro de Moura M, et al. Identification of an Immune-Specific Class of Hepatocellular Carcinoma, Based on Molecular Features. Gastroenterology (2017) 153(3):812–26. doi: 10.1053/j.gastro.2017.06.007
82. Krueger PD, Kim TS, Sung SS, Braciale TJ, Hahn YS. Liver-Resident CD103+ Dendritic Cells Prime Antiviral CD8+ T Cells in Situ. J Immunol (2015) 194(7):3213–22. doi: 10.4049/jimmunol.1402622
83. Pallett LJ, Davies J, Colbeck EJ, Robertson F, Hansi N, Easom NJW, et al. IL-2(High) Tissue-Resident T Cells in the Human Liver: Sentinels for Hepatotropic Infection. J Exp Med (2017) 214(6):1567–80. doi: 10.1084/jem.20162115
84. Langhans B, Nischalke HD, Kramer B, Hausen A, Dold L, van Heteren P, et al. Increased Peripheral CD4(+) Regulatory T Cells Persist After Successful Direct-Acting Antiviral Treatment of Chronic Hepatitis C. J Hepatol (2017) 66(5):888–96. doi: 10.1016/j.jhep.2016.12.019
85. Kim JH, Han JW, Choi YJ, Rha MS, Koh JY, Kim KH, et al. Functions of Human Liver CD69(+)CD103(-)CD8(+) T Cells Depend on HIF-2alpha Activity in Healthy and Pathologic Livers. J Hepatol (2020) 72(6):1170–81. doi: 10.1016/j.jhep.2020.01.010
86. McNamara HA, Cai Y, Wagle MV, Sontani Y, Roots CM, Miosge LA, et al. Up-Regulation of LFA-1 Allows Liver-Resident Memory T Cells to Patrol and Remain in the Hepatic Sinusoids. Sci Immunol (2017) 2(9):eaaj1996. doi: 10.1126/sciimmunol.aaj1996
87. Han JW, Yoon SK. Tissue-Resident Lymphocytes: Implications in Immunotherapy for Hepatocellular Carcinoma. Int J Mol Sci (2020) 22(1):232. doi: 10.3390/ijms22010232
88. Kojima T, Shah MA, Muro K, Francois E, Adenis A, Hsu CH, et al. Randomized Phase III KEYNOTE-181 Study of Pembrolizumab Versus Chemotherapy in Advanced Esophageal Cancer. J Clin Oncol (2020) 38(35):4138–48. doi: 10.1200/JCO.20.01888
89. Sun JM, Shen L, Shah MA, Enzinger P, Adenis A, Doi T, et al. Pembrolizumab Plus Chemotherapy Versus Chemotherapy Alone for First-Line Treatment of Advanced Oesophageal Cancer (KEYNOTE-590): A Randomised, Placebo-Controlled, Phase 3 Study. Lancet (2021) 398(10302):759–71. doi: 10.1016/S0140-6736(21)01234-4
90. Bang YJ, Kang YK, Catenacci DV, Muro K, Fuchs CS, Geva R, et al. Pembrolizumab Alone or in Combination With Chemotherapy as First-Line Therapy for Patients With Advanced Gastric or Gastroesophageal Junction Adenocarcinoma: Results From the Phase II Nonrandomized KEYNOTE-059 Study. Gastric Cancer (2019) 22(4):828–37. doi: 10.1007/s10120-018-00909-5
91. Shitara K, Van Cutsem E, Bang YJ, Fuchs C, Wyrwicz L, Lee KW, et al. Efficacy and Safety of Pembrolizumab or Pembrolizumab Plus Chemotherapy vs Chemotherapy Alone for Patients With First-Line, Advanced Gastric Cancer: The KEYNOTE-062 Phase 3 Randomized Clinical Trial. JAMA Oncol (2020) 6(10):1571–80. doi: 10.1001/jamaoncol.2020.3370
92. Kono K, Nakajima S, Mimura K. Current Status of Immune Checkpoint Inhibitors for Gastric Cancer. Gastric Cancer (2020) 23(4):565–78. doi: 10.1007/s10120-020-01090-4
93. Ganesh K, Stadler ZK, Cercek A, Mendelsohn RB, Shia J, Segal NH, et al. Immunotherapy in Colorectal Cancer: Rationale, Challenges and Potential. Nat Rev Gastroenterol Hepatol (2019) 16(6):361–75. doi: 10.1038/s41575-019-0126-x
94. Nusrat M. Response to Anti-PD-1 in Microsatellite-Stable Colorectal Cancer: A STAT Need. Clin Cancer Res (2020) 26(22):5775–7. doi: 10.1158/1078-0432.CCR-20-2901
95. El-Khoueiry AB, Sangro B, Yau T, Crocenzi TS, Kudo M, Hsu C, et al. Nivolumab in Patients With Advanced Hepatocellular Carcinoma (CheckMate 040): An Open-Label, Non-Comparative, Phase 1/2 Dose Escalation and Expansion Trial. Lancet (2017) 389(10088):2492–502. doi: 10.1016/S0140-6736(17)31046-2
96. Kudo M. Immuno-Oncology Therapy for Hepatocellular Carcinoma: Current Status and Ongoing Trials. Liver Cancer (2019) 8(4):221–38. doi: 10.1159/000501501
97. Cui K, Hu S, Mei X, Cheng M. Innate Immune Cells in the Esophageal Tumor Microenvironment. Front Immunol (2021) 12:654731. doi: 10.3389/fimmu.2021.654731
98. Kato K, Shah MA, Enzinger P, Bennouna J, Shen L, Adenis A, et al. KEYNOTE-590: Phase III Study of First-Line Chemotherapy With or Without Pembrolizumab for Advanced Esophageal Cancer. Future Oncol (2019) 15(10):1057–66. doi: 10.2217/fon-2018-0609
99. Shah MA. Update on Metastatic Gastric and Esophageal Cancers. J Clin Oncol (2015) 33(16):1760–9. doi: 10.1200/JCO.2014.60.1799
100. Nizard M, Roussel H, Tartour E. Resident Memory T Cells as Surrogate Markers of the Efficacy of Cancer Vaccines. Clin Cancer Res (2016) 22(3):530–2. doi: 10.1158/1078-0432.CCR-15-2364
101. Perdomo C, Zedler U, Kuhl AA, Lozza L, Saikali P, Sander LE, et al. Mucosal BCG Vaccination Induces Protective Lung-Resident Memory T Cell Populations Against Tuberculosis. mBio (2016) 7(6):e01686–16. doi: 10.1128/mBio.01686-16
102. Morabito KM, Ruckwardt TR, Redwood AJ, Moin SM, Price DA, Graham BS. Intranasal Administration of RSV Antigen-Expressing MCMV Elicits Robust Tissue-Resident Effector and Effector Memory CD8+ T Cells in the Lung. Mucosal Immunol (2017) 10(2):545–54. doi: 10.1038/mi.2016.48
103. Komdeur FL, Prins TM, van de Wall S, Plat A, Wisman GBA, Hollema H, et al. CD103+ Tumor-Infiltrating Lymphocytes are Tumor-Reactive Intraepithelial CD8+ T Cells Associated With Prognostic Benefit and Therapy Response in Cervical Cancer. Oncoimmunology (2017) 6(9):e1338230. doi: 10.1080/2162402X.2017.1338230
104. Dumauthioz N, Labiano S, Romero P. Tumor Resident Memory T Cells: New Players in Immune Surveillance and Therapy. Front Immunol (2018) 9:2076. doi: 10.3389/fimmu.2018.02076
105. Ge Z, Zhou G, Campos Carrascosa L, Gausvik E, Boor PPC, Noordam L, et al. TIGIT and PD1 Co-Blockade Restores Ex Vivo Functions of Human Tumor-Infiltrating CD8(+) T Cells in Hepatocellular Carcinoma. Cell Mol Gastroenterol Hepatol (2021) 12(2):443–64. doi: 10.1016/j.jcmgh.2021.03.003
106. Wang P, Chen Y, Long Q, Li Q, Tian J, Liu T, et al. Increased Coexpression of PD-L1 and TIM3/TIGIT Is Associated With Poor Overall Survival of Patients With Esophageal Squamous Cell Carcinoma. J Immunother Cancer (2021) 9(10):e002836. doi: 10.1136/jitc-2021-002836
107. Ou K, Xu X, Guan S, Zhang R, Zhang X, Kang Y, et al. Nanodrug Carrier Based on Poly (Ursolic Acid) With Self-Anticancer Activity Against Colorectal Cancer. Adv Funct Mater (2020) 30(9):1907857. doi: 10.1002/adfm.201907857
Keywords: CD8+ Trm cells, characteristics, antitumor effects, immunotherapy, digestive tract tumors
Citation: Mei X, Li H, Zhou X, Cheng M and Cui K (2022) The Emerging Role of Tissue-Resident Memory CD8+ T Lymphocytes in Human Digestive Tract Cancers. Front. Oncol. 11:819505. doi: 10.3389/fonc.2021.819505
Received: 21 November 2021; Accepted: 23 December 2021;
Published: 14 January 2022.
Edited by:
Yuanzeng Min, University of Science and Technology of China, ChinaCopyright © 2022 Mei, Li, Zhou, Cheng and Cui. This is an open-access article distributed under the terms of the Creative Commons Attribution License (CC BY). The use, distribution or reproduction in other forums is permitted, provided the original author(s) and the copyright owner(s) are credited and that the original publication in this journal is cited, in accordance with accepted academic practice. No use, distribution or reproduction is permitted which does not comply with these terms.
*Correspondence: Kele Cui, Y2tlbGVAbWFpbC51c3RjLmVkdS5jbg==; Min Cheng, Y2hlbmdtaW5AdXN0Yy5lZHUuY24=