- Collaborative Innovation Platform for Modernization and Industrialization of Regional Characteristic Traditional Chinese Medicine, School of Integrated Traditional Chinese and Western Medicine, Binzhou Medical University, Yantai, China
Clinical data show that the incidence and mortality rates of cancer are rising continuously, and cancer has become an ongoing public health challenge worldwide. Excitingly, the extensive clinical application of traditional Chinese medicine may suggest a new direction to combat cancer, and the therapeutic effects of active ingredients from Chinese herbal medicine on cancer are now being widely studied in the medical community. As a traditional anticancer Chinese medicine, ChanSu has been clinically applied since the 1980s and has achieved excellent antitumor efficacy. Meanwhile, the ChanSu active components (e.g., telocinobufagin, bufotalin, bufalin, cinobufotalin, and cinobufagin) exert great antitumor activity in many cancers, such as breast cancer, colorectal cancer, hepatocellular carcinoma and esophageal squamous cell carcinoma. Many pharmaceutical scientists have investigated the anticancer mechanisms of ChanSu or the ChanSu active components and obtained certain research progress. This article reviews the research progress and antitumor mechanisms of ChanSu active components and proposes that multiple active components of ChanSu may be potential anticancer drugs.
Introduction
Cancer is a complex disease that affects the life quality and life expectancy of patients globally (1) and is one of the leading causes of death worldwide, with more than 10 million people dying from cancer each year. The hallmarks of cancer include persistent proliferative signaling, evading growth suppressors, resisting cell death, achieving replicative immortality, inducing angiogenesis, and activating invasion and metastasis (2). Fundamentally, cancer results from uncontrolled growth of cells. Radiation therapy and chemotherapy are among the main treatments for cancer at present (3). However, the efficacy of these cancer treatments is not remarkable, and they severely affect the life quality of cancer patients due to pronounced side effects (3). Traditional Chinese medicine may provide new ways to treat cancer and has gradually become an indispensable force in the fight against cancer (4). An increasing number of traditional Chinese medicines are applied against cancer; examples include barbed skullcap herb (Scutellaria barbata D. Don.), Solanum nigrum L., and Pseudobulb of Cremastra appendiculata (5–7). The toxic and side effects of Chinese medicines are relatively small, the efficacy is mild, and the continuity of medication can be maintained, which offers advantages in suppressing tumor angiogenesis as well as preventing and controlling tumor metastasis (8).
ChanSu (also known as toad venom or Venenum Bufonis) is a precious traditional Chinese medicine derived from the dried secretions of the Asiatic toad (Bufo gargarizans Cantor) or black-spectacled toad (Bufo melanostictus Schneider) of the Bufonidae family (9). The medical records of ChanSu firstly appeared in Yao Xing Lun of the Song Dynasty written by Quan Zhen (10) and be mentioned in following dynasties which described the processing method of ChanSu and its applications (11, 12). ChanSu is efficacious in detoxifying, reducing swelling, relieving pain, invigorating the spleen, and inducing resuscitation, and has been used mostly to treat furuncles, carbuncles, sore throat, faint, and infantile malnutrition (13). Historically, ChanSu has been used as a cardiotonic, diuretic, and hemostatic agent (14). With the development of extraction and detection technology, the components of ChanSu have been gradually identified including bufadienolides, indole alkaloids, sterols, epinephrine, proteins, amino acids, polysaccharide, etc. (15). Among these components of ChanSu, bufadienolides and indole alkaloids (bufotenines) were considered as two of the most important bioactive substances (16). Previous studies demonstrated the cyto-toxic activities of bufadienolides and verified in various cancers, including leukemia (Bufalin) (17), hepatocellular carcinoma (Bufothionine) (18), lung carcinoma (cinobufacini) (19), prostatic adenocarcinoma (20), and colon cancer (bufalin and cinobufagin) (21). In additional, bufotenines such as bufobutanoic acid and bufopyramide were demonstrated could inhibit mice P388 lymphocytic leukemia cell (22). Bufothionine, the most focused and widely studied indole alkaloids of ChanSu which have been verified possess notable anti-tumor effect on various cancer cells, including liver cancer (23), gastric cancer (24). Since the pharmaceutical active components of ChanSu and the pathogenic factors of cancers are overly complicated (25), despite an increasing number of medical scientists participating in the research of ChanSu active components, the action mechanisms of a portion of active components remain to be further studied.
This article reviews the latest research results on the modulation of tumor cells by active components of ChanSu in vitro and in vivo. Moreover, we explore and discuss the regulatory mechanisms of ChanSu active components for distinct cancer types, different signaling pathways, and various molecular targets.
Cancer and ChanSu active components
Cancer
Cancers have a genetic basis but are not necessarily hereditary diseases. When normal cells are influenced by carcinogens, genetic changes in the cells can result in the loss of normal regulatory functions for growth, thereby leading to abnormal cell proliferation in the human body. Therefore, drug-induced cancer cell apoptosis (also known as programmed cell death) and inhibition of cancer cell proliferation, migration, and metastasis are the most important means of treating tumors. Dysregulation of apoptosis has been linked to many human chronic disorders, including cancer (26), and inducing apoptosis in tumor cells has been validated as an effective way to treat cancer (27). Most antineoplastic drugs can achieve anticancer effects through modulating apoptosis (28), and the common ones include cisplatin (29), 5-fluorouracil (30), and paclitaxel (31).
However, these chemotherapeutic agents can easily cause drug resistance of tumor cells. With the increased drug dose and prolonged duration of medication, the drug resistance of tumor cells becomes stronger and human body tolerance decreases, with severe side effects emerging, such as hair loss, reduced immune function, nausea, and vomiting. Although combined therapy regimens comprising multiple drugs are utilized in clinical practice, side effects and drug resistance remain major obstacles in cancer therapy (32). Emerging evidence demonstrate that the combined therapy of Chinese herbal medicine and chemotherapeutic drugs may minimize the side effects of chemotherapeutic drugs and maintain a certain continuity in medication, which may have important implications in the suppression of tumor metastasis, enhancement of patients’ physical fitness, and improvement of patients’ quality of life. Especially, ChanSu displays potent antineoplastic activity and has been extensively investigated as a new broad-spectrum anticancer drug.
ChanSu active components
The extraction and identification studies of ChanSu can be traced back to 1963, after thin layer and paper chromatography tracing, three sterols and 19 bufadienolides were isolated and identified from the skin of Bufo japonicus formosus (33). In the next 60 years, 23 indole alkaloids (34), 142 bufadienolides (15) were extracted and identified from the skin or dried powdered of toad venom. Indole alkaloids of ChanSu, characterized as high hydrophilicity, which are almost derived from serotonin (34). Bufobutanoic acid and bufopyramide were earliest reported indole alkaloids of ChanSu in 1999 (35). In one study, methanol extracts of ChanSu were separated by preparative HPLC with the conditions of H2O-Acetonitrile (98:2) solution containing 0.05% TFA and H2O-Acetonitrile (85:15-83:17), respectively, and identified by the 1H and 13C NMR spectral data (35). After that, other 21 indoles alkaloids such as serotonin, N′-methyl-serotonin, bufotenine, bufotenidine, dehydrobufotenine, bufothionine were extracted and identified by various isolation and identification methods including HPLC-ESI-Q-TOF-MS/MS, Phenyl-hexyl column with an ultraviolet detector, preparing thin-layer chromatography and HPLC, and atmospheric pressure chemical ionization tandem mass spectrometry (APCI-MS/MS) (12, 36–39). Unfortunately, scientific researchers seem to prefer extraction to in-depth study on the efficacy of these indoles’ alkaloids. Only a few studies focused on the bio-activities of bufothionine and bufotenine which demonstrated that bufothionine have potential activities in induced cell cycle arrest (G2/M phase arrest), inhibited tumor growth in liver cancer cells (40) and in H22 bearing mice (18). In terms of bufotenine, previous studies verified its powerful anti-inflammatory and psychotropic properties resulted from inhibited nuclear factor-kappa B (NF-κB) signaling pathway and its high affinity for the 5-hydroxytryptamine receptor, respectively (41, 42).
Except for indoles alkaloids, bufadienolides are a class of C-24 steroids, which are characterized by a diunsaturated six-membered lactone ring at C-17 (α-β) and considered as the most important components of ChanSu (43, 44) (Figure 1). In 1965, 19 bufadienolides including bufotalin, bufalin, bufotalinin, telocinobufagin, and cinobufotalin were isolated by thin layer and paper chromatography and identified from the skin of the Japanese toad bufo (33). Next, another 16 bufadienolides (such as resibufogenin, hellebrigenin, desacetylbufotalin, gamabufotalin, and cinobufagin) were isolated from chloroform extracts of ChanSu and subjected to silica gel chromatography (45, 46). Since that, reversed-phase preparative high-performance liquid chromatography (HPLC), TBE-300 high-speed counter-current chromatography, and ultra-high performance liquid chromatography (UHPLC) methods were used to separate bufadienolides from ethanol, methanol, and water extracts of ChanSu and identified by NMR or quadrupole time-of-flight mass spectrometry (Q-TOF/MS) technique (47–49). Meanwhile, anti-tumor activities of ChanSu bufadienolides were testified in various cancer cells. For example, bufalin and cinobufagin significantly induced cell apoptosis, cell cycle arrest, inhibit cell migration and invasion in liver cancer cells (50–52). The similar anti-tumor effects of ChanSu bufadienolides were observed in lung cancer (bufalin and gamabufotalin) (53, 54), osteosarcoma (cinobufagin, bufalin and bufotalin) (55, 56), and colorectal cancer (cinobufagin, resibufogenin, and doxorubicin) (57, 58). Among them, five bufadienolides including telocinobufagin, bufotalin, bufalin, cinobufotalin, and cinobufagin are the most focused and studied ChanSu bufadienolides and their anti-tumor activities have been verified in various cancers (Table 1).
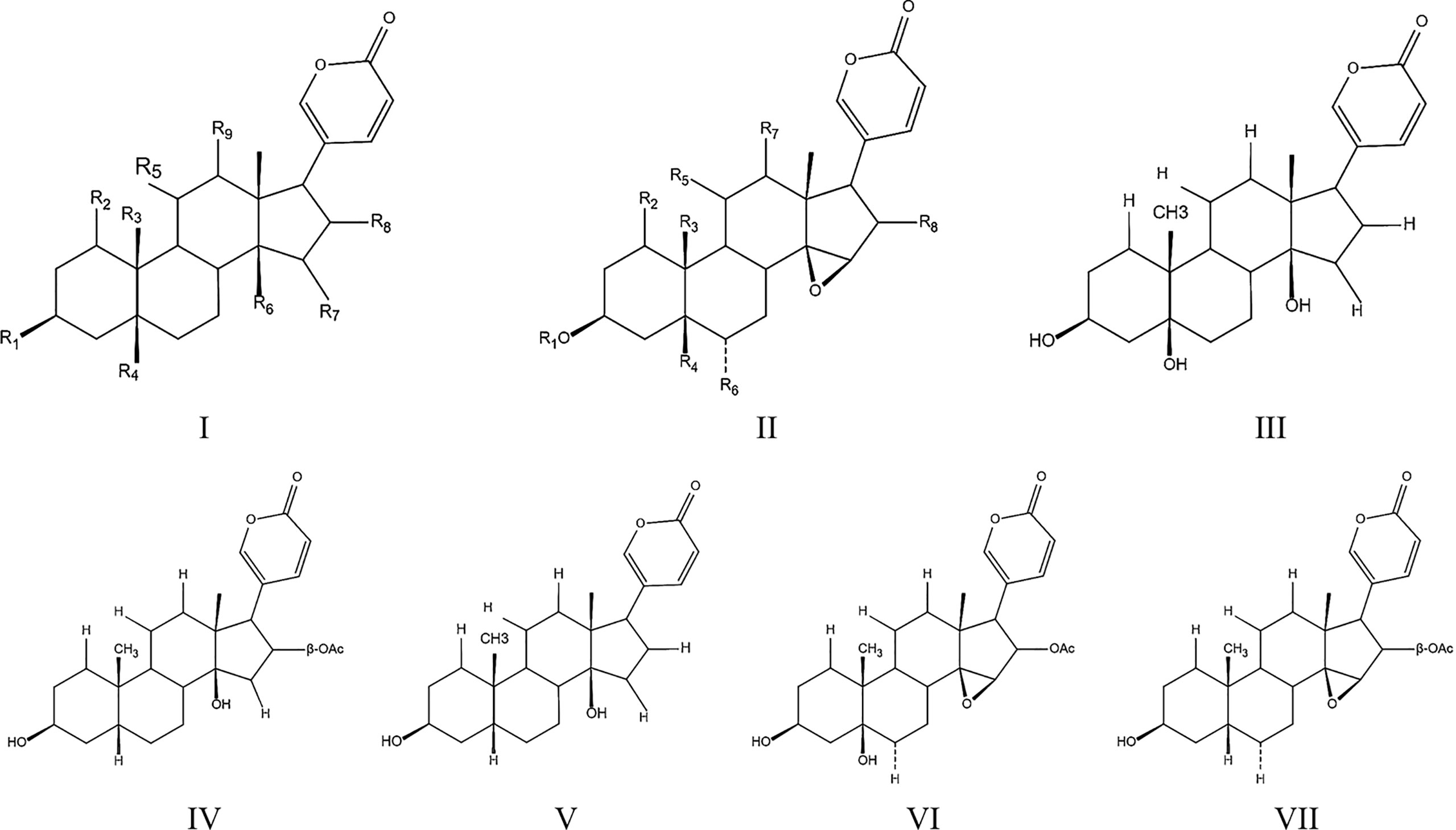
Figure 1 Toadienohydroxylate lactone parent nucleus (I, II), Telocinobufagin (III), Bufotalin (IV), Bufalin (V), Cinobufotalin (VI), Cinobufagin (VII).
The anti-tumor mechanisms of five ChanSu bufadienolides
Telocinobufagin
Telocinobufagin (TBG) is an active component isolated from the traditional Chinese medicine ChanSu and possesses the pharmacological properties of ChanSu, such as immunomodulation (93) and inhibition of Na+/K+-ATPase activities (60). Previous pharmacokinetic studies of TBG in rats demonstrated that after orally administered 120 mg/kg ChanSu extracts, the Cmax of TBG in plasma was 0.69 ± 0.26 μg/ml which imply its absorption is extremely low but rapid due to the Tmax was 35 ± 18 min. Meanwhile, 135 ± 43 min T1/2 demonstrated that TBG has moderate elimination rate in rats (94). In addition, TBG exhibits important antineoplastic activities, such as inhibiting tumor cell proliferation, constraining cell differentiation, arresting cell cycle, suppressing tumor angiogenesis, and hindering tumor cell metastasis. In vitro, telocinobufagin showed powerful cyto-toxic activities in various tumor cells (IC50 in LLC-PK1, A549, and H157 cells were 0.20 µM, 27.882 ± 17.291, and 23.606 ± 7.381 ng/mL, respectively (95). Numerous studies have focused on the antitumor effects of TBG on breast cancer. It has been shown that TBG (0.05 and 0.5 μg/ml) significantly inhibits migration and invasion of breast cancer cells via modulate PI3K/AKT/ERK/Snail pathway (59). Evaluation of anti-metastatic effect in a highly metastatic 4T1 mouse model has revealed that the tumor volume in mice after TBG treatment significantly decreased and the mass evidently decreased, without obvious changes in mice body weight, and H&E staining confirmed the lack of metastasis of breast cancer cells in the lungs (59). Further research has demonstrated that TBG represses epithelial–mesenchymal transition (EMT) via the Akt/ERK/Snail signaling pathway (59), thereby concluding that the Akt/ERK/Snail signaling pathway is a promising anticancer target of TBG. Additionally, it has been shown that the PI3K/Akt signal transduction pathway is closely related to tumorigenesis and development of breast cancer, and the expression levels of p-PI3K and p-Akt are increased in breast cancer cells under hypoxia, while the expression levels decrease after TBG intervention, suggesting that TBG may exert its function through the PI3K/Akt pathway and suppress the proliferation and invasion of breast cancer cells (59). According to another experiment, TBG can block breast cancer cell mobility through effectively impeding MMP-2 and MMP-9 synthesis, thereby achieving the inhibition of breast cancer cell proliferation and migration (96). Furthermore, some studies have reported that TBG is proficient in suppressing colon cancer cell viability and inducing apoptosis and is capable of significantly raising the necrosis and apoptosis rates of colorectal cancer (CRC) cells. Previous studies have also indicated that the suppression of CRC cells by TBG is related to the p53-mediated Bax pathway (97).
Moreover, TBG can modulate multiple immune cells that play key roles in immune responses. TBG induces the expression of Th1 cytokines and inhibit the secretion of Th2 cytokines, thereby enhancing the killing capacities of natural killer cells and macrophages, which become the first line of defense to protect the host from tumor and viral infection. Thus, TBG can exert anticancer activities through regulating immune cells and can be a potential therapeutic agent to treat cancer (93). The primary anti-tumor mechanisms of TBG in cancer cells were showed in Figure 2.
Bufotalin
Bufotalin is an extract from the traditional Chinese medicine ChanSu prepared from the dried secretion of the auricular and skin glands of Bufo gargarizans Cantor. In Kunming mice, single intravenous injection bufotalin showed rapid distributive and eliminated characters due to the T1/2 and MRT of bufotalin in plasma were 28.6 min and 14.7 min, respectively (98). The anti-tumor effects of bufalin have been testified in various cancer cells such as HepG2 Cells (IC50 = 130 ± 10 nM (48 h)), Eca-109 cells (IC50 = 0.8 µM (72 h)) (95). In details, bufotalin is able to induce apoptosis of human hepatocellular carcinoma (HCC) Hep3B cells (99), with experimental data suggesting that the inhibition rate of bufotalin for Hep3B cells could reach 80% (61). Another study showed that multiple active components of ChanSu had evident inhibitory effects on human HCC cell line (HepG2) and its doxorubicin-induced multidrug resistant liver cancer cells (R-HepG2); bufotalin had the best performance among these components and displayed extremely potent anti-hepatoma activity (64). Cell cycle analysis revealed that bufotalin induced apoptosis through the inhibition of the Akt-mediated signal pathway and induced cell cycle arrest at G2/M phase in HepG2 cells with upregulated p53 gene expression, thereby decreasing cellular viability and effectively suppressing the proliferation and migration of HepG2 cells.
It has been shown that bufotalin-induced apoptosis in osteoblastoma cells is associated with endoplasmic reticulum (ER) stress activation (65). Mild ER stress is usually considered a pro-survival and adaptive response, while prolonged or severe ER stress can promote apoptosis (62). After bufotalin treatment, MG63 cells are significantly less proliferative. Further experiments have shown that bufotalin promotes apoptosis via the activation of caspase-12, and the use of caspase inhibitors significantly represses the anticancer activity of bufotalin. Bufotalin sensitizes TNF-related apoptosis-inducing ligand (TRAIL)- and tumor necrosis factor-α (TNF-α)-induced apoptosis of HeLa cells. Through sensitizing death receptor–induced apoptosis via Bid- and STAT1-dependent pathways, bufotalin enhances chemotherapeutic drugs–induced apoptosis and downregulates antiapoptotic gene expression (63).
A study showed that bufotalin can simultaneously induce cell cycle arrest and apoptosis in A375 cells. Further experiments have revealed that bufotalin induces melanoma cell cycle arrest at G2/M phase via upregulation of ATM and Chk2 and downregulation of Cdc25C. Additionally, bufotalin may induce apoptosis via the mitochondrial apoptosis pathway and inhibition of AKT phosphorylation, which provides convincing evidence for bufotalin as a potential therapeutic drug for treating malignant melanoma of the skin (67). Another study has indicated that bufotalin induces p53-mediated apoptosis in esophageal squamous cell carcinoma (ESCC) cells. Bufotalin can effectively inhibit the viability of ESCC cells, enhance caspase protein activity, upregulate the expression of DNA damage-associated proteins, inhibit DNA repair, and markedly inhibit the expression of Ki-67, a biomarker of proliferation, suggesting that bufotalin exhibits therapeutic potential for ESCC through modulating the p53 signaling pathway (66). In addition, bufotalin has a proapoptotic effect on human gastric cancer BGC-823 cells (100).
Moreover, examination of the pharmacokinetics and tissue distribution following single-bolus injection and constant-rate infusion of bufotalin in mice showed that, except for the lungs and brain, bufotalin concentrations were low in most tissues. Constant-rate infusion resulted in higher bufotalin concentrations in the lungs and brain compared with single-bolus injection within the same time. These results suggest that bufotalin might be a promising antitumor candidate for lung cancer (98). The bufotalin induced mainly anti-tumor effects on cancer cells were showed in Figure 3.
Bufalin
Bufalin is a cardiotonic steroid and a principal component of the traditional Chinese medicine ChanSu extracted from the skins and parotid venom glands of Bufo gargarizans Cantor. After intravenous administration of ChanSu extracts in SD rats (0.8 mg/kg), rabbits (0.35 mg/kg), and beagle dogs (0.18 mg/kg), the T1/2 of bufalin were 24.32 ± 3.78, 21-31, and 14-28 min, respectively (101–103). In additional, oral administration of 10 mg/kg bufalin in SD rats, the pharmacokinetic parameters in plasma demonstrated that the Tmax and t1/2 were 22.50 ± 8.02 and 375.76 ± 243.85 min, respectively, which imply that bufalin have rapid absorptive and slowly eliminated properties (104). Numerous studies have reported that bufalin possesses very potent antitumor activities, and it is deemed one of the most valuable anticancer drugs. However, the mechanisms of bufalin’s antineoplastic actions are not fully understood. The mechanisms of bufalin effects on human bladder cancer are being particularly studied. It has been shown that bufalin can induce apoptotic cell death in human bladder cancer cells through the activation of both the intrinsic and extrinsic pathways. Bufalin induces cell cycle arrest at the G2/M phase, inhibits the growth of human bladder cancer cells, and suppresses cell proliferation, invasiveness, and metastasis. Additionally, it can induce apoptosis by activating the mitochondria-mediated intrinsic caspase pathway and the death receptor–mediated extrinsic pathway (70). Another study has reported that bufalin induces G0/G1 phase arrest in human bladder cancer T24 cells by reducing the levels of cyclin D, cyclin E, CDK2, and CDK4, and triggers apoptosis through the mitochondrial signaling pathway (71). Experimental data have also revealed that bufalin treatment results in repressed Akt activity and an increase in the proapoptotic Bax caused by dissociation from antiapoptotic Bcl-2, thereby leading to mitochondrial dysfunction, cytochrome c release, activation of caspase cascades, and consequently apoptosis in bladder cancer cells (71).
The experimental results have also shown that the antitumor effect of bufalin is related to the phosphorylation of the extracellular signal-regulated protein kinase (ERK) pathway, and the inhibitory effect of bufalin on T24 cell invasion may be partially fulfilled via the ERK pathway activation (72). Another study has demonstrated that bufalin can sensitize human bladder carcinoma cells to TRAIL-mediated apoptosis (76). TRAIL can induce programmed cell death (68, 74). TRAIL exerts minimal impact on normal cells but can selectively induce apoptosis of numerous transformed or malignant cells (73). However, certain malignant tumor cells are resistant to TRAIL. Combined usage of bufalin and TRAIL can markedly inhibit the viability of human bladder carcinoma cells, sensitizing the TRAIL-resistant bladder carcinoma cells to TRAIL-mediated apoptosis. Overall, bufalin may be a new anticancer drug in the future with features of low toxicity and rare side effects, which may provide a better therapeutic effect for patients with drug-resistant bladder cancer.
With regard to the treatment of neuroblastoma, researchers have constructed a chemically modified bufalin probe CS-P1 that retains the antitumor activity of bufalin in neuroblastoma, which is able to inhibit the proliferation and migration of neuroblastoma cells. Further investigation has confirmed that the antitumor effect of bufalin in neuroblastoma in vitro is achieved by targeting the electron transport chain (ETC). The accumulation of reactive oxygen species (ROS) induced by the disruption of the ETC decreases the mitochondrial membrane potential, increases the permeability of mitochondrial membrane, and causes the build-up of cytochrome c in cytoplasm, thereby inducing apoptosis (80). Furthermore, in glioblastoma, bufalin can augment the radiosensitivity of glioblastoma by repressing mitochondrial function and DNA damage repair (77) and can also improve the sensitivity to temozolamide (TMZ) (79), which provides the theoretical basis for the combined therapy of bufalin and radiation.
There are also research findings showing that bufalin induces apoptosis of human HCC cells through the Fas- and mitochondria-mediated pathways, and a caspase-10-dependent, Fas-mediated pathway might play a more crucial role (69). There are also related reports on the suppression of side effects from liver cancer chemotherapeutic drugs (78). Furthermore, bufalin has a certain inhibitory influence on pancreatic cancer, which is the most difficult tumor to target. Namely, bufalin exhibits strong inhibitory effect on pancreatic cancer cell proliferation both in vitro and in vivo, and induces cell cycle arrest at S phase via upregulating p27 level, consequently promoting apoptosis (75). The illustration of bufalin-triggered pathways that involved in tumor cell cycles and apoptosis were showed in Figure 4.
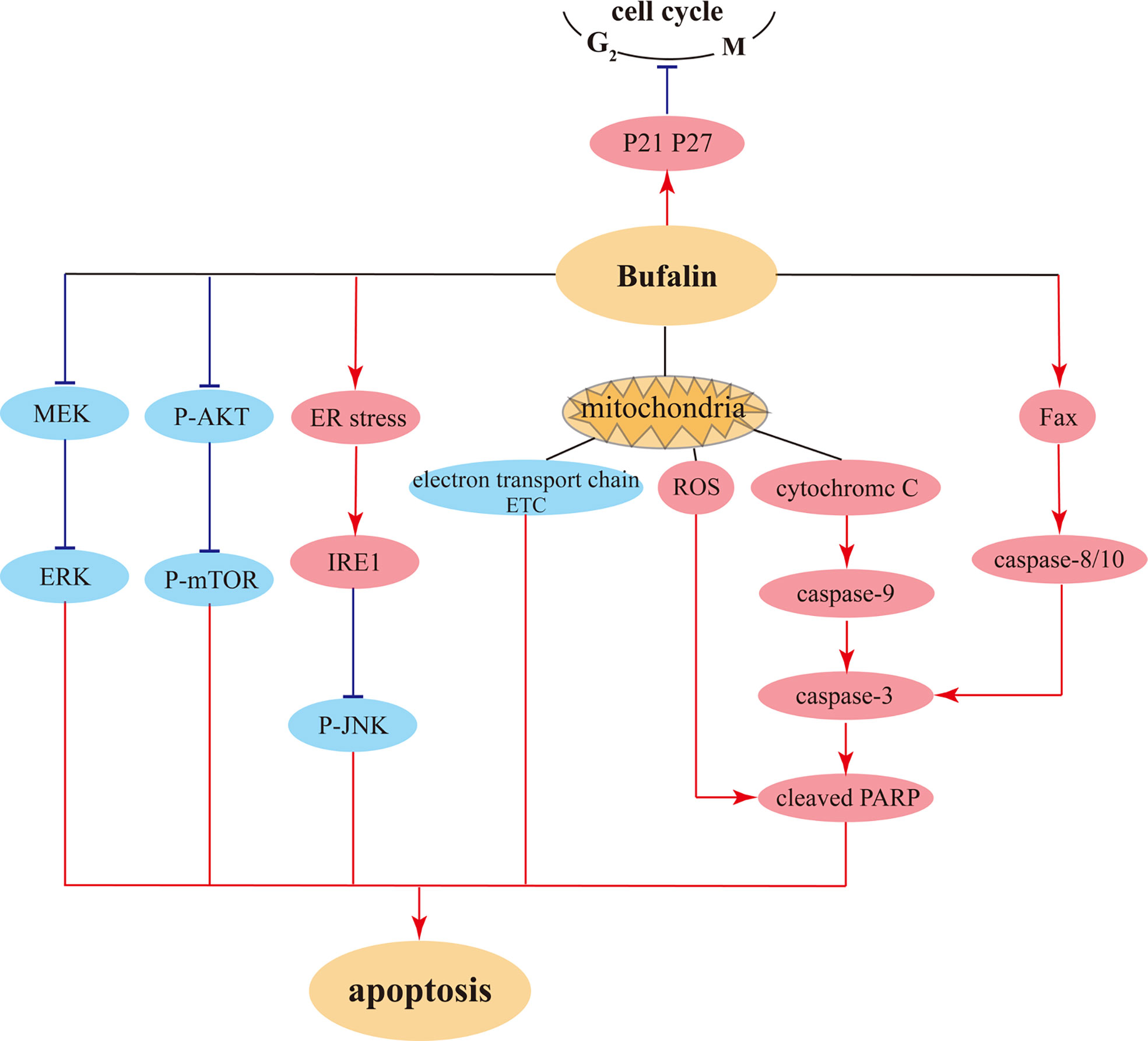
Figure 4 The possible pathways for bufalin induced cell cycle arrest and pro-apoptosis in tumor cells.
Cinobufotalin
Cinobufotalin (CB) is a bufadienolide discovered in toad venom. Previous PK studies used ChanSu extracts were performed in SD rats demonstrated that compared with intravenous injection (0.8 mg/kg), CB concentrations in oral administrated (120 mg.kg) rats plasma showed comfortable elimination rate (T1/2 of intravenous injection was 22.58 ± 5.58 min vs. 119 ± 26 min of oral administration). Meanwhile, oral administration exhibited rapid but low absorptive rate (Cmax and Tmax were 0.68 ± 0.33 μg/ml, and 17 ± 15 min, respectively) (94). Several studies have confirmed that CB possesses anticancer activities (81). The research on CB has never stopped, among which nasopharyngeal and liver cancers were mostly investigated. It has been revealed for the first time that EBV-miR-BART22 directly targets MAP2K4 and stimulates Myh9 expression, thereby inducing the ubiquitin degradation of GSK3β protein and consequently promoting tumor stemness, metastasis, and cisplatin chemoresistance via the activation of β-catenin-induced stemness and EMT signals (84). Further investigation has revealed that CB can powerfully reverse EBV-miR-BART22-induced cisplatin resistance via upregulating MAP2K4 to antagonize Myh9/GSK3β/β-catenin and its downstream tumor stemness and EMT signals in nasopharyngeal carcinoma. It has been proposed that the elevated miR-BART22 and decreased MAP2K4 expression may be important markers to predict poor prognosis in patients with nasopharyngeal carcinoma (84). It has also been found that CB can effectively induce FOXO1-stimulated cisplatin sensitivity by antagonizing FOXO1’s binding partner Myh9 (83). High Myh9 expression promotes the invasion and metastasis of tumor cells (105, 106), and CB suppresses tumor invasiveness via inhibiting Myh9 expression. Both in vitro and in vivo experiments have suggested that CB can improve the sensitivity of FOXO1-overexpressing nasopharyngeal carcinoma cells to cisplatin and promote their apoptosis, indicating that CB is a potential drug to fight against nasopharyngeal carcinoma (83).
With regard to the research on CB in the treatment of liver cancer, as a novel SREBP1 inhibitor, CB suppresses HCC proliferation by targeting lipogenesis, which significantly reduces the lipid levels in HepG2 cells and inhibits carcinoma growth, consequently exerting antitumor influence (86). In addition, another study has shown that CB induces HCC cells to produce ceramide, and ceramide production mediates CB-induced growth inhibition and apoptosis in HCC cells. It has been proposed that SphK1 suppression, ceramide production, and Akt/mTORC1 inactivation may be responsible for the inhibitory impact of CB on HCC cells (82). Scholars have also proposed that CB can induce apoptosis of liver cancer cells via the mitochondrial pathway.
A recent study has acquired data on CB-treated breast cancer cells from the Gene Expression Omnibus (GEO) database and has analyzed the profile of differentially expressed genes; the study suggested that CB may exert anticancer activities in MCF-7 cells in similar ways as miconazole. CB can suppress the proliferation of SKOV3 cancer cells through decreasing ARF6 protein expression, thereby accomplishing antitumor effect (107). Another animal experiment validated that as a substrate of P-glycoprotein, the distribution of CB in animal liver tissues can be modulated by P-glycoprotein. This suggests that the combined application with P-glycoprotein inhibitor can increase the accumulation of CB in plasma and liver in the treatment of liver cancer, thereby augmenting its antitumor efficacy (85). Overall, CB induced mainly anti-tumor pathways were showed in Figure 5.
Cinobufagin
Cinobufagin is one of the principle active components in the traditional Chinese medicine ChanSu and an effective traditional Chinese medicine monomer extracted from Bufo gargarizans Cantor and Bufo melanostictus Schneider (108). Similar as CB, the PK parameters of cinobufagin showed its absorptive and eliminated rates were less than 30 min (the Tmax of oral administration 120 mg/kg ChanSu extracts was 20 ± 12 min) and great than 2 hours (T1/2 was 138 ± 30 min), respectively, although with weak absorptive amount (Cmax was 0.77 ± 0.12 μg/ml) (94). Numerous studies testified the anti-tumor cell properties of cinobufagin, such as U2OS cells (IC50 = 100 nM, 48 h), SMMC-7721 Cells (IC50 = 92 ng/mL, 48 h), EC9706 cells (IC50 = 3.2 µM, 72hrs), Hec2 cells (IC50 = 2.4 µM, 72hrs), and MCF7 Cells (IC50 = 0.44 ± 0.12 µM, 48 h) (95). A study reported that cinobufagin was able to decrease the viability of OS cells and induce their apoptosis both in vitro and in vivo. Under the influence of cinobufagin, Notch-1 gene was downregulated, indicating that cinobufagin may induce apoptosis of OS cells through the inactivation of the Notch signaling pathway (55). Another study showed that cinobufagin may inhibit the characteristics of OS cancer cells by suppressing the IL-6-OPN-STAT3 pathway and may also inhibit the metastasis and invasiveness of OS cells. Additionally, when OS cells were repressed, the normal human osteoblast hFOB1.19 cells were not obviously impacted (90). There was also research showing that cinobufagin manifested potent antitumor activities by inducing G2/M phase arrest and apoptosis in OS cells. Further mechanistic research has suggested that cinobufagin-induced apoptosis is partially achieved through the suppression of the GSK-3/NF-κB pathway (87). To sum up, cinobufagin is likely to be a promising drug candidate for OS treatment.
Moreover, regarding the research on the action mechanisms of cinobufagin in the inhibition of CRC, experimental data have indicated that cinobufagin evidently suppresses CRC cell viability via the STAT3 pathway inhibition, promotes apoptosis, and represses proliferation and migration of CRC cells. Besides, cinobufagin showed therapeutic effects in a CRC xenograft model without damaging major organs (92). Cinobufagin can also impede the growth of CRC cells by suppressing angiogenesis (109). Further experimental results have indicated that cinobufagin can inhibit angiogenesis in CRC through downregulating the Akt/mTORC1/HIF-1α pathway and triggering MOMP-mediate apoptosis, consequently reaching antitumor outcome (88). Thus, cinobufagin is a very promising biotherapeutic drug to treat CRC.
Cinobufagin can also promote apoptosis in nasopharyngeal carcinoma cells. By downregulating the levels of CDK2 and cyclin E, cinobufagin induces cell cycle arrest at the S phase in HK-1 cells and increases ROS levels, leading to DNA damage (110). In addition, cinobufagin promotes apoptosis in nasopharyngeal carcinoma HK-1 cells through downregulating the protein levels of Bcl-2 and the apoptotic protein PARP1 (91). It has also been shown that cinobufagin-induced apoptosis in gastric cancer is enhanced via inhibition of autophagy (111, 112). At the same time, cinobufagin can induce ROS production, further augment the effect of autophagy inhibition, increase proapoptotic gene expression, and disrupt mitochondrial membrane potential, thereby inducing apoptosis in gastric cancer cells (89). The illustration of primary anti-tumor effects of cinobufagin on cancer cells were showed in Figure 6.
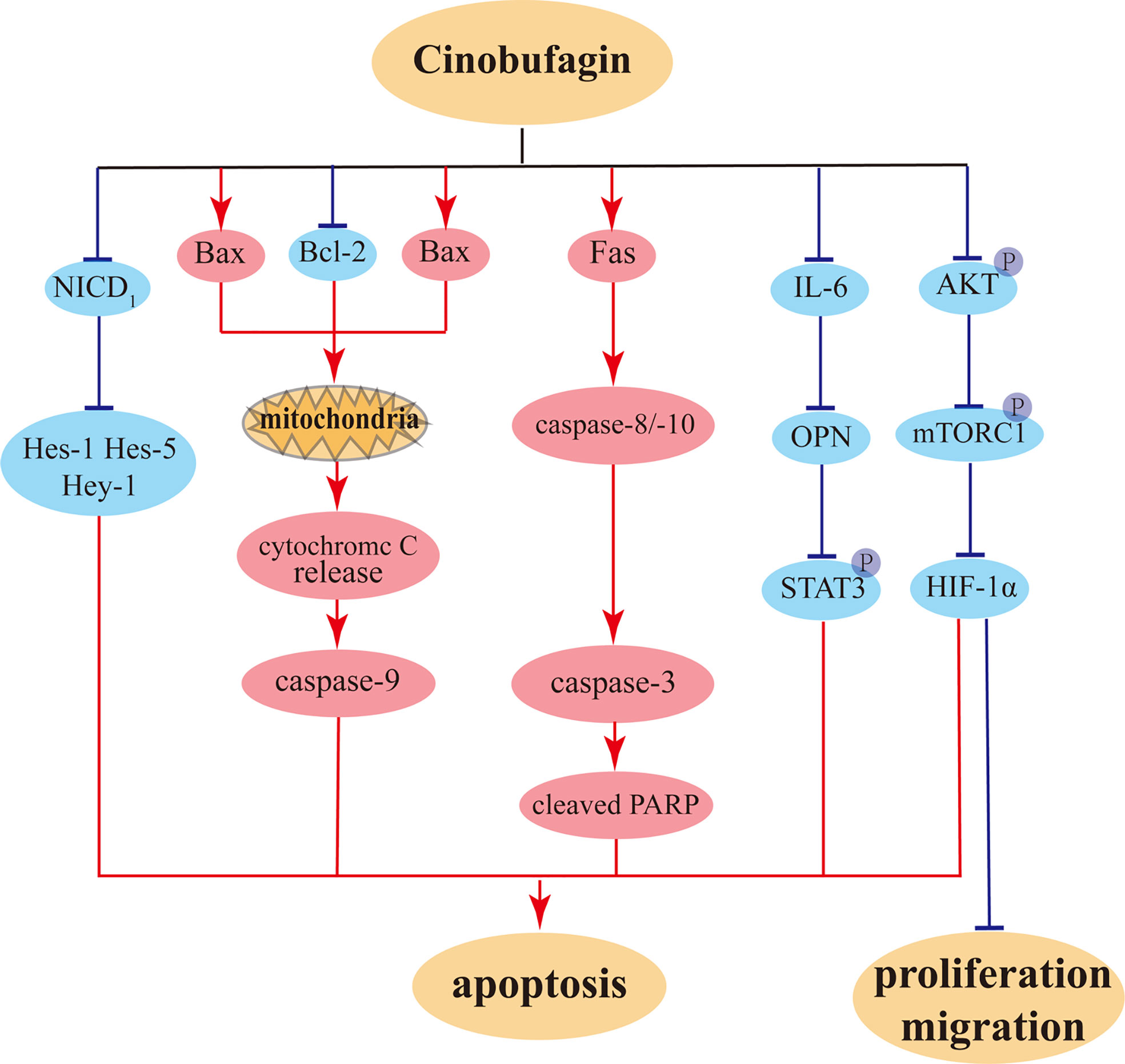
Figure 6 The potential anti-proliferation, anti-migration, and pro-apoptosis pathways of cinobufagin on tumor cells.
Clinical applications of cinobufacini combination therapy
It has been reported that the anti-tumor effects of various active ingredients of Chansu were significantly better than those of other anti-tumor drugs, such as the herbal extract paclitaxel and the chemotherapeutic drug cisplatin (32, 113). The combination of Chansu active ingredients and other chemotherapeutic drugs is significantly more effective than the single application of two drugs, and also increases the sensitivity of drug-resistant cells to chemotherapeutic drugs (114). As a traditional medicine in China, the Chinese medicine extracts of ChanSu have been made into cinobufacini injection for clinical application. Cinobufacini is an aqueous injection solution processed from the shaded dried skin of the traditional Chinese medicine Bufo gargarizans Cantor and has been approved by the China Food and Drug Administration as a chemotherapeutic drug to treat liver and prostate cancers in China (115). Previous studies investigated the chemical constituents in cinobufacini injection by UPLC-ESI-QTOF/MSE method and the results showed that 76 components were identified including 12 alkaloids, 13 peptides, 36 bufadienolides, and 15 other components (such as organic acids, amides, and sterols). In terms of bufadienolides, telocinobufagin, bufotalin, bufalin, cinobufotalin, and cinobufagin were identified (116). Similarly, these five components were also identified in cinobufacini treated rats plasma (117).
Cinobufacini injection showed satisfied properties in detoxification, swelling reduction, pain relief, and illnesses including middle and advanced stage tumors as well as chronic hepatitis B. Clinical study data have shown that combination application of cinobufacini with radiotherapy and chemotherapy evidently elevates efficacy compared with chemotherapy (gemcitabine-oxaliplatin) alone in patients with locally advanced or metastatic gallbladder carcinoma, and mitigates the toxic or side effects of radiation and chemotherapy (118). Another study indicated a possible effective component in cinobufacini that could exert anti-tumor effect in HCC patients (40). In the treatment of a case of advanced lung cancer with malignant pericardial effusion, after intrapericardial cinobufacini instillation, the patient’s pain was significantly relieved and life quality improved with hardly any adverse reactions, which provided a new approach for the treatment of patients with advanced cancer who cannot tolerate chemotherapeutic drugs (119). In the treatment of primary liver cancer after transarterial chemoembolization (TACE), intra-arterial infusion of cinobufacini combined with sorafenib competently improved short-term antitumor efficacy, reduced the levels of inflammatory cytokines and tumor markers, and was well tolerated with minimal adverse reactions (120). Clinical adjuvant therapy with cinobufacini in elderly patients with colorectal cancer enhanced short-term efficacy of chemotherapy, largely reduced the toxic or side effects of radiotherapy and chemotherapy, and improved the body’s immune function, exhibiting high clinical application values (121).
Future research perspectives
The incidence and mortality of cancer are increasing each year, and treatments with chemotherapy or radiation alone generate little desirable outcomes, which is why finding new ways to fight cancer is imperative. In this situation, traditional Chinese medicine, which has been passed on for thousands of years, is gradually being increasingly recognized by most physicians and patients. Years of clinical applications confirm the definite antitumor effect of ChanSu, and cinobufacini in combination with other chemotherapeutic drugs shows very good clinical efficacy, which has great implications for the scientific and rational utilization of cinobufacini and full employment of the unique antitumor effect of traditional Chinese medicine.
In addition, several studies showed that Chansu has adverse effects such as cardiotoxicity (122), hepatotoxicity (123), and central system toxicity (124). Chansu also can rapidly alter intracellular calcium stores Na+/K+ concentrations in cardiac myocytes, causing them to stop beating within seconds (125). However, the cardiac toxicity of ChanSu could be effectively alleviated through intravenous infusion, which provides an efficient way to take advantage of the multiple extracts of ChanSu in clinical application (126). At present, clinical research on ChanSu is primarily conducted in China, with rare related clinical studies conducted abroad. Future developments should aim at enhancing antitumor activities, identifying antitumor mechanisms, and reducing toxic side effects. A greater number of clinical studies should be carried out under the premise of safe medication in order to ensure the clinical efficacy and absolute safety of ChanSu and its effective active components.
Conclusion
This article reviews the antitumor effects of the five active components of the traditional Chinese medicine ChanSu and their molecular mechanisms. Previous research has revealed that the inhibition of tumor cells by these active components is achieved through multiple pathways, and the primary anticancer mechanisms include inducing cancer cell apoptosis, inducing autophagy, arresting cell cycle, suppressing metastasis, repressing growth, and reversing drug resistance. A substantial amount of evidence indicates that these active components may become potential drugs for clinical treatment of cancers in the future. Before clinical application, further experimental studies are needed to elucidate their exact molecular mechanisms. Overall, ChanSu and its active compounds can antagonize cancer cells through a variety of mechanisms, inhibit cancer cell proliferation, migration, and differentiation, and promote cancer cell apoptosis (17).
The clinical application of many chemotherapeutic agents can cause numerous serious adverse reactions, leading to unsatisfactory clinical outcomes. Therefore, anticancer agents derived from natural resources may become the most promising agents for cancer treatment. As a representative of antitumor drugs in traditional Chinese medicine, ChanSu is broadly utilized in clinical practice. However, the composition of compounds extracted from ChanSu is complex with a lack of detailed clinical trial processes. The toxicity of ChanSu has not been supported thorough experimental investigation, and there are still many gaps to be filled regarding the anticancer aspects.
Gaining a better understanding of tumorigenesis and the pharmacological effects of the natural medicine ChanSu, as well as its active components, will be helpful for the large-scale clinical studies on these drugs and their targets in the future. This article reviews the research on the therapeutic effects of ChanSu active components in the treatment of cancer, in order to provide theoretical support and medication guidance in their clinical treatment.
Author contributions
Conceptualization: JL and DL; data curation: JJ; writing – original draft: JL, JJ, and DL; writing – review and editing: QZ and DL. All authors contributed to the article and approved the submitted version.
Funding
This research was funded by the National Natural Science Foundation of China (82073313 to DL, 31870338 to QZ), Taishan Scholars Construction Engineering of Shandong Province (to DL), the Yantai High-End Talent Introduction Plan “Double Hundred” (to DL).
Conflict of interest
The authors declare that the research was conducted in the absence of any commercial or financial relationships that could be construed as a potential conflict of interest.
Publisher’s note
All claims expressed in this article are solely those of the authors and do not necessarily represent those of their affiliated organizations, or those of the publisher, the editors and the reviewers. Any product that may be evaluated in this article, or claim that may be made by its manufacturer, is not guaranteed or endorsed by the publisher.
References
1. Song G, Cheng L, Chao Y, Yang K, Liu Z. Emerging nanotechnology and advanced materials for cancer radiation therapy. Adv Mater (2017) 29:1700996. doi: 10.1002/adma.201700996
2. Chaisakul J, Hodgson WC, Kuruppu S, Prasongsook N. Effects of animal venoms and toxins on hallmarks of cancer. J Cancer (2016) 7:1571–8. doi: 10.7150/jca.15309
3. Chang Y, He L, Li Z, Zeng L, Song Z, Li P, et al. Designing core-shell gold and selenium nanocomposites for cancer radiochemotherapy. ACS Nano (2017) 11:4848–58. doi: 10.1021/acsnano.7b01346
4. Zhang X, Qiu H, Li C, Cai P, Qi F. The positive role of traditional Chinese medicine as an adjunctive therapy for cancer. Biosci Trends (2021) 15:283–98. doi: 10.5582/bst.2021.01318
5. Liu J, He C, Tang Y, Liu W, Xu Y, Li Z, et al. A review of cremastra appendiculata (D.Don) makino as a traditional herbal medicine and its main components. J Ethnopharmacol (2021) 279:114357. doi: 10.1016/j.jep.2021.114357
6. Li Y, Zhang J, Zhang K, Chen Y, Wang W, Chen H, et al. Scutellaria barbata inhibits hepatocellular carcinoma tumorigenicity by inducing ferroptosis of hepatocellular carcinoma cells. Front Oncol (2022) 12:693395. doi: 10.3389/fonc.2022.693395
7. Liang X, Hu C, Han M, Liu C, Sun X, Yu K, et al. Solasonine inhibits pancreatic cancer progression with involvement of ferroptosis induction. Front Oncol (2022) 12:834729. doi: 10.3389/fonc.2022.834729
8. Ge H, Xu C, Chen H, Liu L, Zhang L, Wu C, et al. Traditional Chinese medicines as effective reversals of epithelial-mesenchymal transition induced-metastasis of colorectal cancer: Molecular targets and mechanisms. Front Pharmacol (2022) 13:842295. doi: 10.3389/fphar.2022.842295
9. Gowda RM, Cohen RA, Khan IA. Toad venom poisoning: resemblance to digoxin toxicity and therapeutic implications. Heart (2003) 89:e14. doi: 10.1136/heart.89.4.e14
10. Shu X, Mcculloch M, Xiao H, Broffman M, Gao J. Chinese Herbal medicine and chemotherapy in the treatment of hepatocellular carcinoma: a meta-analysis of randomized controlled trials. Integr Cancer Ther (2005) 4:219–29. doi: 10.1177/1534735405279927
11. Zhao Z, Liang Z, Ping G. Macroscopic identification of Chinese medicinal materials: traditional experiences and modern understanding. J Ethnopharmacol (2011) 134:556–64. doi: 10.1016/j.jep.2011.01.018
12. Zhan X, Wu H, Wang R, Luo C, Gao B, Chen Z, et al. Metabolites from bufo gargarizans (Canto): A review of traditional uses, pharmacological activity, toxicity and quality control. J Ethnopharmacol (2020) 246:112178. doi: 10.1016/j.jep.2019.112178
13. Yan X, Xie G, Zhou J, Milne GW. Traditional chinese medicines: molecular structures, natural sources and applications. New York: Routledge. (2018).
14. Runhui L, Heng L, Yongli L, Ming Y, Xike X, Huiliang L, et al. N-containing compounds from the traditional Chinese medicine ChanSu. Chem Natural compounds (2009) 45:599–600. doi: 10.1007/s10600-009-9380-4
15. Wei WL, Hou JJ, Wang X, Yu Y, Li HJ, Li ZW, et al. Venenum bufonis: An overview of its traditional use, natural product chemistry, pharmacology, pharmacokinetics and toxicology. J Ethnopharmacol (2019) 237:215–35. doi: 10.1016/j.jep.2019.03.042
16. Qi J, Zulfiker AHM, Li C, Good D, Wei MQ. The development of toad toxins as potential therapeutic agents. Toxins (Basel) (2018) 10:336. doi: 10.3390/toxins10080336
17. Zhu Z, Li E, Liu Y, Gao Y, Sun H, Wang Y, et al. Bufalin induces the apoptosis of acute promyelocytic leukemia cells via the downregulation of survivin expression. Acta Haematol (2012) 128:144–50. doi: 10.1159/000339424
18. Xie RF, Li ZC, Chen PP, Zhou X. Bufothionine induced the mitochondria-mediated apoptosis in H22 liver tumor and acute liver injury. Chin Med (2015) 10:5. doi: 10.1186/s13020-015-0033-1
19. Wang J, Jin Y, Xu Z, Zheng Z, Wan S. Involvement of caspase-3 activity and survivin downregulation in cinobufocini-induced apoptosis in a 549 cells. Exp Biol Med (Maywood) (2009) 234:566–72. doi: 10.3181/0811-RM-326
20. Yu CH, Kan SF, Pu HF, Jea Chien E, Wang PS. Apoptotic signaling in bufalin- and cinobufagin-treated androgen-dependent and -independent human prostate cancer cells. Cancer Sci (2008) 99:2467–76. doi: 10.1111/j.1349-7006.2008.00966.x
21. Li C, Hashimi SM, Cao S, Mellick AS, Duan W, Good D, et al. The mechanisms of chansu in inducing efficient apoptosis in colon cancer cells. Evid Based Complement Alternat Med (2013) 2013:849054. doi: 10.1155/2013/849054
22. Liu YF, Chen X, Ling H, Liu DC, Xia MY, Wang D. [Cloning, prokaryotic expression and function of the bufo bufo gargarizans tryptophan hydroxylase I gene]. Yao Xue Xue Bao (2016) 51:1158–64. doi: 10.16438/j.0513-4870.2015-1095
23. Kong WS, Shen FX, Xie RF, Zhou G, Feng YM, Zhou X. Bufothionine induces autophagy in H22 hepatoma-bearing mice by inhibiting JAK2/STAT3 pathway, a possible anti-cancer mechanism of cinobufacini. J Ethnopharmacol (2021) 270:113848. doi: 10.1016/j.jep.2021.113848
24. Wang G, Liu G, Ye Y, Fu Y, Zhang X. Bufothionine exerts anti-cancer activities in gastric cancer through Pim3. Life Sci (2019) 232:116615. doi: 10.1016/j.lfs.2019.116615
25. Li C, Hashimi SM, Cao S, Qi J, Good D, Duan W, et al. Chansu inhibits the expression of cortactin in colon cancer cell lines in vitro and in vivo. BMC Complement Altern Med (2015) 15:207. doi: 10.1186/s12906-015-0723-3
26. Maiese K, Chong ZZ, Shang YC, Wang S. Targeting disease through novel pathways of apoptosis and autophagy. Expert Opin Ther Targets (2012) 16:1203–14. doi: 10.1517/14728222.2012.719499
27. Chen T, Wong YS. Selenocystine induces apoptosis of A375 human melanoma cells by activating ROS-mediated mitochondrial pathway and p53 phosphorylation. Cell Mol Life Sci (2008) 65:2763–75. doi: 10.1007/s00018-008-8329-2
28. Chen T, Wong YS. Selenocystine induces caspase-independent apoptosis in MCF-7 human breast carcinoma cells with involvement of p53 phosphorylation and reactive oxygen species generation. Int J Biochem Cell Biol (2009) 41:666–76. doi: 10.1016/j.biocel.2008.07.014
29. Kim S, Jary M, Andre T, Vendrely V, Buecher B, Francois E, et al. Docetaxel, cisplatin, and 5-fluorouracil (DCF) chemotherapy in the treatment of metastatic or unresectable locally recurrent anal squamous cell carcinoma: a phase II study of French interdisciplinary GERCOR and FFCD groups (Epitopes-HPV02 study). BMC Cancer (2017) 17:574. doi: 10.1186/s12885-017-3566-0
30. Gudaityte J, Dvylys D, Simeliunaite I. Anaesthetic challenges in cancer patients: current therapies and pain management. Acta Med Litu (2017) 24:121–7. doi: 10.6001/actamedica.v24i2.3493
31. Ahn D, Sohn JH, Kim JH, Lee JE, Park SH, Kim JC. Concurrent chemoradiotherapy with capecitabine/cisplatin versus 5-fluorouracil/cisplatin in resectable laryngohypopharyngeal squamous cell carcinoma. Ear Nose Throat J (2016) 95:E34–42. doi: 10.1177/014556131609500210
32. Shen X, Tao Y, Yang Y, Wang R, Fang J, Lian M. Combination of TPF regimen and cinobufotalin inhibits proliferation and induces apoptosis in human hypopharyngeal and laryngeal squamous cell carcinoma cells. Onco Targets Ther (2019) 12:341–8. doi: 10.2147/OTT.S185428
33. Iseli E, Kotake M, Weiss E, Reichstein T. Die sterine und bufadienolide der haut von bufo formosus BOULENGER. Über krötengifte, 31. mitteilung. Helv Chimica Acta (1965) 48:1093–112. doi: 10.1002/hlca.19650480517
34. Li FJ, Hu JH, Ren X, Zhou CM, Liu Q, Zhang YQ. Toad venom: A comprehensive review of chemical constituents, anticancer activities, and mechanisms. Arch Pharm (Weinheim) (2021) 354:e2100060. doi: 10.1002/ardp.202100060
35. Kamano Y, Morita H, Takano R, Kotake A, Nogawa T, Hashima H, et al. Bufobutanoic acid and bufopyramide, two new indole alkaloids from the Chinese traditional drug ch’an su. Heterocycles (1999) 50:499. doi: 10.3987/COM-98-S(H)55
36. Davison EK, Sperry J. Alkaloids from the traditional Chinese medicine ChanSu: synthesis-enabled structural reassignment of bufopyramide to bufoserotonin c. Org Biomol Chem (2015) 13:7911–4. doi: 10.1039/C5OB01051A
37. Dai YH, Shen B, Xia MY, Wang AD, Chen YL, Liu DC, et al. A new indole alkaloid from the toad venom of bufo bufo gargarizans. Molecules (2016) 21:349. doi: 10.3390/molecules21030349
38. Dai YH, Wang AD, Chen YL, Xia MY, Shao XY, Liu DC, et al. A new indole alkaloid from the traditional Chinese medicine chansu. J Asian Nat Prod Res (2018) 20:581–5. doi: 10.1080/10286020.2017.1339697
39. Cao Y, Wu J, Pan H, Wang L. Chemical profile and multicomponent quantitative analysis for the quality evaluation of toad venom from different origins. Molecules (2019) 24:3595. doi: 10.3390/molecules24193595
40. Xie RF, Li ZC, Gao B, Shi ZN, Zhou X. Bufothionine, a possible effective component in cinobufocini injection for hepatocellular carcinoma. J Ethnopharmacol (2012) 141:692–700. doi: 10.1016/j.jep.2011.12.018
41. Halberstadt AL, Nichols DE, Geyer MA. Behavioral effects of alpha,alpha,beta,beta-tetradeutero-5-MeO-DMT in rats: comparison with 5-MeO-DMT administered in combination with a monoamine oxidase inhibitor. Psychopharmacol (Berl) (2012) 221:709–18. doi: 10.1007/s00213-011-2616-6
42. Zulfiker AH, Hashimi SM, Qi J, Grice ID, Wei MQ. Aqueous and ethanol extracts of Australian cane toad skins suppress pro-inflammatory cytokine secretion in U937 cells via NF-kappaB signaling pathway. J Cell Biochem (2016) 117:2769–80. doi: 10.1002/jcb.25577
43. Nogawa T, Kamano Y, Yamashita A, Pettit GR. Isolation and structure of five new cancer cell growth inhibitory bufadienolides from the Chinese traditional drug ch’an su. J Nat Prod (2001) 64:1148–52. doi: 10.1021/np0101088
44. Li FJ, Hu JH, Ren X, Zhou CM, Liu Q, Zhang YQ. Toad venom: A comprehensive review of chemical constituents, anticancer activities, and mechanisms. Archiv der Pharmazie (2021) 354:2100060. doi: 10.1002/ardp.202100060
45. Kamano Y, Yamamoto H, Hatayama K, Tanaka Y, Shinohara M, Komatsu M. The isolation and structure of new bufadienolide, resibufagin and the isolation of marinobufagin. Tetrahedron Lett (1968) 9:5669–5672. doi: 10.1016/S0040-4039(00)70747-7
46. Qiao L, Huang YF, Cao JQ, Zhou YZ, Qi XL, Pei YH. One new bufadienolide from Chinese drug “Chan’Su”. J Asian Nat Prod Res (2008) 10:233–7. doi: 10.1080/10286020701603146
47. Yang Z, Luo H, Wang H, Hou H. Preparative isolation of bufalin and cinobufagin from Chinese traditional medicine ChanSu. J Chromatogr Sci (2008) 46:81–5. doi: 10.1093/chromsci/46.1.81
48. Li J, Ma X, Li F, Wang J, Chen H, Wang G, et al. Preparative separation and purification of bufadienolides from Chinese traditional medicine of ChanSu using high-speed counter-current chromatography. J Sep Sci (2010) 33:1325–30. doi: 10.1002/jssc.200900782
49. Hu Y, Yu Z, Yang ZJ, Zhu G, Fong W. Comprehensive chemical analysis of venenum bufonis by using liquid chromatography/electrospray ionization tandem mass spectrometry. J Pharm BioMed Anal (2011) 56:210–20. doi: 10.1016/j.jpba.2011.05.014
50. Qi F, Li A, Inagaki Y, Xu H, Wang D, Cui X, et al. Induction of apoptosis by cinobufacini preparation through mitochondria- and fas-mediated caspase-dependent pathways in human hepatocellular carcinoma cells. Food Chem Toxicol (2012) 50:295–302. doi: 10.1016/j.fct.2011.10.040
51. Chen H, Meng YH, Guo DA, Liu X, Liu JH, Hu LH. New cytotoxic 19-norbufadienolide and bufogargarizin isolated from chan su. Fitoterapia (2015) 104:1–6. doi: 10.1016/j.fitote.2015.05.011
52. Gai JQ, Sheng X, Qin JM, Sun K, Zhao W, Ni L. The effect and mechanism of bufalin on regulating hepatocellular carcinoma cell invasion and metastasis via wnt/beta-catenin signaling pathway. Int J Oncol (2016) 48:338–48. doi: 10.3892/ijo.2015.3250
53. Park JS, Shin DY, Lee YW, Cho CK, Kim GY, Kim WJ, et al. Apoptotic and anti-metastatic effects of the whole skin of venenum bufonis in A549 human lung cancer cells. Int J Oncol (2012) 40:1210–9. doi: 10.3892/ijo.2011.1310
54. Yu Z, Guo W, Ma X, Zhang B, Dong P, Huang L, et al. Gamabufotalin, a bufadienolide compound from toad venom, suppresses COX-2 expression through targeting IKKbeta/NF-kappaB signaling pathway in lung cancer cells. Mol Cancer (2014) 13:203. doi: 10.1186/1476-4598-13-203
55. Cao Y, Yu L, Dai G, Zhang S, Zhang Z, Gao T, et al. Cinobufagin induces apoptosis of osteosarcoma cells through inactivation of notch signaling. Eur J Pharmacol (2017) 794:77–84. doi: 10.1016/j.ejphar.2016.11.016
56. Lee CH, Shih YL, Lee MH, Au MK, Chen YL, Lu HF, et al. Bufalin induces apoptosis of human osteosarcoma U-2 OS cells through endoplasmic reticulum stress, caspase- and mitochondria-dependent signaling pathways. Molecules (2017) 22:437. doi: 10.3390/molecules22030437
57. Yuan Z, Shi X, Qiu Y, Jia T, Yuan X, Zou Y, et al. Reversal of p-gp-mediated multidrug resistance in colon cancer by cinobufagin. Oncol Rep (2017) 37:1815–25. doi: 10.3892/or.2017.5410
58. Han Q, Ma Y, Wang H, Dai Y, Chen C, Liu Y, et al. Resibufogenin suppresses colorectal cancer growth and metastasis through RIP3-mediated necroptosis. J Transl Med (2018) 16:201. doi: 10.1186/s12967-018-1580-x
59. Gao Y, Shi L, Cao Z, Zhu X, Li F, Wang R, et al. Telocinobufagin inhibits the epithelial-mesenchymal transition of breast cancer cells through the phosphoinositide 3-kinase/protein kinase b/extracellular signal-regulated kinase/Snail signaling pathway. Oncol Lett (2018) 15:7837–45. doi: 10.3892/ol.2018.8349
60. Chen Q, Yang XF. Research progress in targeted therapy of hepatocellular carcinoma. Chin Med Sci J (2021) 36:57–65. doi: 10.24920/003737
61. Su CL, Lin TY, Lin CN, Won SJ. Involvement of caspases and apoptosis-inducing factor in bufotalin-induced apoptosis of hep 3B cells. J Agric Food Chem (2009) 57:55–61. doi: 10.1021/jf802769g
62. Hetz C. The unfolded protein response: controlling cell fate decisions under ER stress and beyond. Nat Rev Mol Cell Biol (2012) 13:89–102. doi: 10.1038/nrm3270
63. Waiwut P, Inujima A, Inoue H, Saiki I, Sakurai H. Bufotalin sensitizes death receptor-induced apoptosis via bid- and STAT1-dependent pathways. Int J Oncol (2012) 40:203–8. doi: 10.3892/ijo.2011.1182
64. Zhang DM, Liu JS, Tang MK, Yiu A, Cao HH, Jiang L, et al. Bufotalin from venenum bufonis inhibits growth of multidrug resistant HepG2 cells through G2/M cell cycle arrest and apoptosis. Eur J Pharmacol (2012) 692:19–28. doi: 10.1016/j.ejphar.2012.06.045
65. Zhu YR, Xu Y, Fang JF, Zhou F, Deng XW, Zhang YQ. Bufotalin-induced apoptosis in osteoblastoma cells is associated with endoplasmic reticulum stress activation. Biochem Biophys Res Commun (2014) 451:112–8. doi: 10.1016/j.bbrc.2014.07.077
66. Lin S, Lv J, Peng P, Cai C, Deng J, Deng H, et al. Bufadienolides induce p53-mediated apoptosis in esophageal squamous cell carcinoma cells in vitro and in vivo. Oncol Lett (2018) 15:1566–72. doi: 10.3892/ol.2017.7457
67. Pan Z, Qu C, Chen Y, Chen X, Liu X, Hao W, et al. Bufotalin induces cell cycle arrest and cell apoptosis in human malignant melanoma A375 cells. Oncol Rep (2019) 41:2409–17. doi: 10.3892/or.2019.7032
68. Thorburn A, Behbakht K, Ford H. TRAIL receptor-targeted therapeutics: resistance mechanisms and strategies to avoid them. Drug Resist Update (2008) 11:17–24. doi: 10.1016/j.drup.2008.02.001
69. Qi F, Inagaki Y, Gao B, Cui X, Xu H, Kokudo N, et al. Bufalin and cinobufagin induce apoptosis of human hepatocellular carcinoma cells via fas- and mitochondria-mediated pathways. Cancer Sci (2011) 102:951–8. doi: 10.1111/j.1349-7006.2011.01900.x
70. Hong SH, Choi YH. Bufalin induces apoptosis through activation of both the intrinsic and extrinsic pathways in human bladder cancer cells. Oncol Rep (2012) 27:114–20. doi: 10.3892/or.2011.1451
71. Huang WW, Yang JS, Pai SJ, Wu PP, Chang SJ, Chueh FS, et al. Bufalin induces G0/G1 phase arrest through inhibiting the levels of cyclin d, cyclin e, CDK2 and CDK4, and triggers apoptosis via mitochondrial signaling pathway in T24 human bladder cancer cells. Mutat Res (2012) 732:26–33. doi: 10.1016/j.mrfmmm.2011.09.010
72. Hong SH, Kim GY, Chang YC, Moon SK, Kim WJ, Choi YH. Bufalin prevents the migration and invasion of T24 bladder carcinoma cells through the inactivation of matrix metalloproteinases and modulation of tight junctions. Int J Oncol (2013) 42:277–86. doi: 10.3892/ijo.2012.1683
73. Lim B, Allen JE, Prabhu VV, Talekar MK, Finnberg NK, El-Deiry WS. Targeting TRAIL in the treatment of cancer: new developments. Expert Opin Ther Targets (2015) 19:1171–85. doi: 10.1517/14728222.2015.1049838
74. Trivedi R, Mishra DP. Trailing TRAIL resistance: Novel targets for TRAIL sensitization in cancer cells. Front Oncol (2015) 5:69. doi: 10.3389/fonc.2015.00069
75. Liu X, Xiao XY, Shou QY, Yan JF, Chen L, Fu HY, et al. Bufalin inhibits pancreatic cancer by inducing cell cycle arrest via the c-Myc/NF-kappaB pathway. J Ethnopharmacol (2016) 193:538–45. doi: 10.1016/j.jep.2016.09.047
76. Kang KH, Han MH, Jeong JW, Park C, Lee SH, Lee HW, et al. Bufalin sensitizes human bladder carcinoma cells to TRAIL-mediated apoptosis. Oncol Lett (2017) 14:853–9. doi: 10.3892/ol.2017.6223
77. Zhang X, Huang Q, Wang X, Xu Y, Xu R, Han M, et al. Bufalin enhances radiosensitivity of glioblastoma by suppressing mitochondrial function and DNA damage repair. BioMed Pharmacother (2017) 94:627–35. doi: 10.1016/j.biopha.2017.07.136
78. Feng Y, Chen Y, Meng Y, Cao Q, Liu Q, Ling C, et al. Bufalin suppresses migration and invasion of hepatocellular carcinoma cells elicited by poly (I:C) therapy. Oncoimmunology (2018) 7:e1426434. doi: 10.1080/2162402X.2018.1426434
79. Liu J, Zhang Y, Sun S, Zhang G, Jiang K, Sun P, et al. Bufalin induces apoptosis and improves the sensitivity of human glioma stem-like cells to temozolamide. Oncol Res (2019) 27:475–86. doi: 10.3727/096504018X15270916676926
80. Pan L, Nie L, Yao S, Bi A, Ye Y, Wu Y, et al. Bufalin exerts antitumor effects in neuroblastoma via the induction of reactive oxygen speciesmediated apoptosis by targeting the electron transport chain. Int J Mol Med (2020) 46:2137–49. doi: 10.3892/ijmm.2020.4745
81. Kai S, Lu JH, Hui PP, Zhao H. Pre-clinical evaluation of cinobufotalin as a potential anti-lung cancer agent. Biochem Biophys Res Commun (2014) 452:768–74. doi: 10.1016/j.bbrc.2014.08.147
82. Cheng L, Chen YZ, Peng Y, Yi N, Gu XS, Jin Y, et al. Ceramide production mediates cinobufotalin-induced growth inhibition and apoptosis in cultured hepatocellular carcinoma cells. Tumour Biol (2015) 36:5763–71. doi: 10.1007/s13277-015-3245-1
83. Li Y, Liu X, Lin X, Zhao M, Xiao Y, Liu C, et al. Chemical compound cinobufotalin potently induces FOXO1-stimulated cisplatin sensitivity by antagonizing its binding partner MYH9. Signal Transduct Target Ther (2019) 4:48. doi: 10.1038/s41392-019-0084-3
84. Liu Y, Jiang Q, Liu X, Lin X, Tang Z, Liu C, et al. Cinobufotalin powerfully reversed EBV-miR-BART22-induced cisplatin resistance via stimulating MAP2K4 to antagonize non-muscle myosin heavy chain IIA/glycogen synthase 3beta/beta-catenin signaling pathway. EBioMedicine (2019) 48:386–404. doi: 10.1016/j.ebiom.2019.08.040
85. Zhang X, Liu T, Zhang Y, Liu F, Li H, Fang D, et al. Elucidation of the differences in cinobufotalin’s pharmacokinetics between normal and diethylnitrosamine-injured rats: The role of p-glycoprotein. Front Pharmacol (2019) 10:521. doi: 10.3389/fphar.2019.00521
86. Meng H, Shen M, Li J, Zhang R, Li X, Zhao L, et al. Novel SREBP1 inhibitor cinobufotalin suppresses proliferation of hepatocellular carcinoma by targeting lipogenesis. Eur J Pharmacol (2021) 906:174280. doi: 10.1016/j.ejphar.2021.174280
87. Yin JQ, Wen L, Wu LC, Gao ZH, Huang G, Wang J, et al. The glycogen synthase kinase-3beta/nuclear factor-kappa b pathway is involved in cinobufagin-induced apoptosis in cultured osteosarcoma cells. Toxicol Lett (2013) 218:129–36. doi: 10.1016/j.toxlet.2012.11.006
88. Li X, Chen C, Dai Y, Huang C, Han Q, Jing L, et al. Cinobufagin suppresses colorectal cancer angiogenesis by disrupting the endothelial mammalian target of rapamycin/hypoxia-inducible factor 1alpha axis. Cancer Sci (2019) 110:1724–34. doi: 10.1111/cas.13988
89. Xiong X, Lu B, Tian Q, Zhang H, Wu M, Guo H, et al. Inhibition of autophagy enhances cinobufagininduced apoptosis in gastric cancer. Oncol Rep (2019) 41:492–500. doi: 10.3892/or.2018.6837
90. Zhang C, Ma K, Li WY. Cinobufagin suppresses the characteristics of osteosarcoma cancer cells by inhibiting the IL-6-OPN-STAT3 pathway. Drug Des Devel Ther (2019) 13:4075–90. doi: 10.2147/DDDT.S224312
91. Pan Z, Luo Y, Xia Y, Zhang X, Qin Y, Liu W, et al. Cinobufagin induces cell cycle arrest at the s phase and promotes apoptosis in nasopharyngeal carcinoma cells. BioMed Pharmacother (2020) 122:109763. doi: 10.1016/j.biopha.2019.109763
92. Bai Y, Wang X, Cai M, Ma C, Xiang Y, Hu W, et al. Cinobufagin suppresses colorectal cancer growth via STAT3 pathway inhibition. Am J Cancer Res (2021) 11:200–14. doi: 10.1186/s12935-021-02150-0
93. Cao Y, Song Y, An N, Zeng S, Wang D, Yu L, et al. The effects of telocinobufagin isolated from chan su on the activation and cytokine secretion of immunocytes in vitro. Fundam Clin Pharmacol (2009) 23:457–64. doi: 10.1111/j.1472-8206.2009.00696.x
94. Liang Y, Liu AH, Qin S, Sun JH, Yang M, Li P, et al. Simultaneous determination and pharmacokinetics of five bufadienolides in rat plasma after oral administration of chansu extract by SPE-HPLC method. J Pharm BioMed Anal (2008) 46:442–8. doi: 10.1016/j.jpba.2007.11.001
95. Zhan X, Wu H, Wang R, Li Q. Natural active constituents of bufo bufo gargarizans cantor: A review on pharmacological activity, toxicity and quality control. J ethnopharmacol (2019) 246:112178. doi: 10.1016/j.jep.2019.112178
96. Bailon-Moscoso N, Cevallos-Solorzano G, Romero-Benavides JC, Orellana MI. Natural compounds as modulators of cell cycle arrest: Application for anticancer chemotherapies. Curr Genomics (2017) 18:106–31. doi: 10.2174/1389202917666160808125645
97. Liang ST, Li Y, Li XW, Wang JJ, Tan FX, Han QR, et al. Mechanism of colon cancer cell apoptosis induced by telocinobufagin: role of oxidative stress and apoptosis pathway. Nan Fang Yi Ke Da Xue Xue Bao (2016) 36:921–6. doi: 10.3969/j.issn.1673-4254.2016.07.08
98. Yu CL, Hou HM. Plasma pharmacokinetics and tissue distribution of bufotalin in mice following single-bolus injection and constant-rate infusion of bufotalin solution. Eur J Drug Metab Pharmacokinet (2011) 35:115–21. doi: 10.1007/s13318-010-0017-6
99. Ye M, Guo DA. Analysis of bufadienolides in the Chinese drug ChanSu by high-performance liquid chromatography with atmospheric pressure chemical ionization tandem mass spectrometry. Rapid Commun Mass Spectrom (2005) 19:1881–92. doi: 10.1002/rcm.1989
100. Chui-Liang Y, Hui-Min H. Research progress on bufotalin. Natural Product Res Dev (2012) 24:280–3. doi: 10.3969/j.issn.1001-6880.2012.02.030
101. Liu D, Shou-Ying DU, Xiu-Feng HE, Wang AG, Na LI. Studies on simultaneous determination and pharmacokinetics of two bufadienolides in rabbits plasma. Chin J Exp Tradit Med Formulae (2011) 17:135–8. doi: 11-3495/R.20110906.1107.004
102. Liu D, Shou-Ying DU, Xiu-Feng HE, Wang AG, Na LI. Pharmacokinetic study on bufadienolides of bufalin, cinobufagin, and resibufogenin from bufonis venenum in rats in vivo. Chin Tradit Herbal Drugs (2012) 43:734–8. doi: 10.1016/j.jep.2014.07.056
103. Liu D, Xiu-Feng HE, Shou-Ying DU, Wang AG, Dai CH, Xue-Zhu GU, et al. Simultaneous determination and pharmacokinetics of three bufadienolides in dogs. Chin J Exp Tradit Med Formulae (2013) 19:188–2. doi: 10.11653/syfj2013170188
104. Dong L, Shou-Ying D, Xiufeng H, Aiguo WSL, Na L. Pharmacokinetic study on bufadienolides of bufalin, cinobufagin, and resibufogenin from bufonis venenum in rats in vivo. Chin Tradit Herbal Drugs (2012) 43:734–8. doi: 10.1016/j.jpha.2021.02.003
105. Katono K, Sato Y, Jiang SX, Kobayashi M, Nagashio R, Ryuge S, et al. Prognostic significance of MYH9 expression in resected non-small cell lung cancer. PloS One (2015) 10:e0121460. doi: 10.1371/journal.pone.0121460
106. Zhou W, Fan MY, Wei YX, Huang S, Chen JY, Liu P. The expression of MYH9 in osteosarcoma and its effect on the migration and invasion abilities of tumor cell. Asian Pac J Trop Med (2016) 9:597–600. doi: 10.1016/j.apjtm.2016.04.011
107. Li J, Rong MH, Dang YW, He RQ, Lin P, Yang H, et al. Differentially expressed gene profile and relevant pathways of the traditional Chinese medicine cinobufotalin on MCF7 breast cancer cells. Mol Med Rep (2019) 19:4256–70. doi: 10.3969/j.issn.1001-6880.2012.02.03010.3892/mmr.2019.10062
108. Zhang J, Hong Y, Jiang L, Yi X, Chen Y, Liu L, et al. Global metabolomic and lipidomic analysis reveal the synergistic effect of bufalin in combination with cinobufagin against HepG2 cells. J Proteome Res (2020) 19:873–83. doi: 10.1021/acs.jproteome.9b00681
109. Hanahan D, Weinberg RA. Hallmarks of cancer: the next generation. Cell (2011) 144:646–74. doi: 10.1016/j.cell.2011.02.013
110. Zhang L, Li L, Xu J, Pan MH, Sun SC. HT-2 toxin exposure induces mitochondria dysfunction and DNA damage during mouse early embryo development. Reprod Toxicol (2019) 85:104–9. doi: 10.1016/j.reprotox.2019.02.011
111. Mizushima N, Levine B, Cuervo AM, Klionsky DJ. Autophagy fights disease through cellular self-digestion. Nature (2008) 451:1069–75. doi: 10.1038/nature06639
112. Nivon M, Richet E, Codogno P, Arrigo AP, Kretz-Remy C. Autophagy activation by NFkappaB is essential for cell survival after heat shock. Autophagy (2009) 5:766–83. doi: 10.4161/auto.8788
113. Liu Y, Jiang Q, Liu X, Lin X, Tang Z, Liu C, et al. Cinobufotalin powerfully reversed EBV-miR-BART22-induced cisplatin resistance via stimulating MAP2K4 to antagonize non-muscle myosin heavy chain IIA/glycogen synthase 3β/β-catenin signaling pathway. EBioMedicine (2019) 48:386–404. doi: 10.1016/j.ebiom.2019.08.040
114. Su S, Dou H, Wang Z, Zhang Q. Bufalin inhibits ovarian carcinoma via targeting mTOR/HIF-α pathway. Basic Clin Pharmacol Toxicol (2021) 128:224–33. doi: 10.1111/bcpt.13487
115. Pan Z, Zhang X, Yu P, Chen X, Lu P, Li M, et al. Cinobufagin induces cell cycle arrest at the G2/M phase and promotes apoptosis in malignant melanoma cells. Front Oncol (2019) 9:853. doi: 10.3389/fonc.2019.00853
116. Li R, Wu H, Wang M, Zhou A, Song S, Li Q. An integrated strategy to delineate the chemical and dynamic metabolic profile of huachansu tablets in rat plasma based on uplc-Esi-Qtof/Mse. SSRN Electron J (2022) 218:114866. doi: 10.2139/ssrn.4015223
117. Li M, Qin Y, Li Z, Lan J, Zhang T, Ding Y. Comparative pharmacokinetics of cinobufacini capsule and injection by UPLC-MS/MS. Front Pharmacol (2022) 13:944041. doi: 10.3389/fphar.2022.944041
118. Qin TJ, Zhao XH, Yun J, Zhang LX, Ruan ZP, Pan BR. Efficacy and safety of gemcitabine-oxaliplatin combined with huachansu in patients with advanced gallbladder carcinoma. World J Gastroenterol (2008) 14:5210–6. doi: 10.3748/wjg.14.5210
119. Sun T, Zhang Y, Shen Y, Hu K, Zuo M. A case of advanced lung cancer with malignant pericardial effusion treated by intrapericardial cinobufacini injection instillation. Biosci Trends (2014) 8:235–9. doi: 10.5582/bst.2014.01073
120. Wu T, Sun R, Wang Z, Yang W, Shen S, Zhao Z. A meta-analysis of cinobufacini combined with transcatheterarterial chemoembolization in the treatment of advanced hepatocellular carcinoma. J Cancer Res Ther (2014) 10 Suppl 1:60–4. doi: 10.4103/0973-1482.139763
121. Zhang X, Yuan Y, Xi Y, Xu X, Guo Q, Zheng H, et al. Cinobufacini injection improves the efficacy of chemotherapy on advanced stage gastric cancer: A systemic review and meta-analysis. Evid Based Complement Alternat Med (2018) 2018:7362340. doi: 10.1155/2018/7362340
122. Bi QR, Hou JJ, Qi P, Ma CH, Feng RH, Yan BP, et al. TXNIP/TRX/NF-κB and MAPK/NF-κB pathways involved in the cardiotoxicity induced by venenum bufonis in rats. Sci Rep (2016) 6:22759. doi: 10.1038/srep22759
123. Xu L, Wang S, Shen H, Feng Q, Zhang X, Ni H, et al. Analgesic and toxic effects of venenum bufonis and its constituent compound cinobufagin: A comparative study. Neurotoxicol Teratol (2019) 73:49–53. doi: 10.1016/j.ntt.2019.03.004
124. Hsu SS, Lin YS, Liang WZ. Investigation of cytotoxic effect of the bufanolide steroid compound cinobufagin and its related underlying mechanism in brain cell models. J Biochem Mol Toxicol (2021) 35:e22862. doi: 10.1002/jbt.22862
125. Bick RJ, Poindexter BJ, Sweney RR, Dasgupta A. Effects of chan su, a traditional Chinese medicine, on the calcium transients of isolated cardiomyocytes: cardiotoxicity due to more than Na, K-ATPase blocking. Life Sci (2002) 72:699–709. doi: 10.1016/S0024-3205(02)02302-0
Keywords: ChanSu, telocinobufagin, bufotalin, bufalin, cinobufotalin, cinobufagin, antitumor
Citation: Jia J, Li J, Zheng Q and Li D (2022) A research update on the antitumor effects of active components of Chinese medicine ChanSu. Front. Oncol. 12:1014637. doi: 10.3389/fonc.2022.1014637
Received: 08 August 2022; Accepted: 07 September 2022;
Published: 27 September 2022.
Edited by:
Mohammed Abu El-Magd, Kafrelsheikh University, EgyptReviewed by:
ElSayed Fatouh, Kafrelsheikh University, EgyptFaisal Alzahrani, King Abdulaziz University, Saudi Arabia
Maha Mohamed Saber-Ayad, University of Sharjah, United Arab Emirates
Copyright © 2022 Jia, Li, Zheng and Li. This is an open-access article distributed under the terms of the Creative Commons Attribution License (CC BY). The use, distribution or reproduction in other forums is permitted, provided the original author(s) and the copyright owner(s) are credited and that the original publication in this journal is cited, in accordance with accepted academic practice. No use, distribution or reproduction is permitted which does not comply with these terms.
*Correspondence: Defang Li, bGlkZWZhbmdAMTYzLmNvbQ==
†There authors have contributed equally to this work