- Department of Radiation Oncology, Weill Cornell Medicine, New York, NY, United States
Background: A major challenge in breast radiotherapy is accurately targeting the surgical cavity volume. Application of the emerging MRI-guided radiotherapy (MRgRT) technique in breast radiotherapy may enable more accurate targeting and potentially reduce side effects associated with treatment.
Purpose: To study the feasibility of delivering MRI-guided partial breast radiotherapy or Precision Prone Irradiation (PPI) to treat DCIS and early stage breast cancer patients.
Materials and methods: Eleven patients with diagnosed DCIS or early stage breast cancer treated with lumpectomy underwent CT-based and MRI-based simulations and treatment planning in the prone position. MRI-guided radiotherapy was utilized to deliver partial breast irradiation. A customized adaptive plan was created for each delivered radiotherapy fraction and the cumulative doses to the target volumes and nearby organs at risk were determined. The CT-based and the MRI-guided radiotherapy plans were compared with respect to target volumes, target volume coverage, and dose to nearby organs.
Results: All patients receiving PPI successfully completed their treatments as planned. Clinical target volume (CTV) and planning target volume (PTV) dose coverage and organs-at-risk (OAR) dose constraints were met in all fractions planned and delivered and the MRI-guided clinical target volumes were smaller when compared to those of the CT-based partial breast radiotherapy plans for these eleven patients.
Conclusions: MRI-guided partial breast radiotherapy as a breast radiotherapy technology is feasible and is a potential high clinical impact application of MRgRT. PPI has the potential to improve the therapeutic index of breast radiotherapy by more accurately delivering radiation dose to the cavity target and decreasing toxicities associated with radiation to the surrounding normal tissues. Prospective clinical data and further technical refinements of this novel technology may broaden its clinical implementation.
Introduction
Patients with ductal carcinoma in situ or early stage breast cancer account for approximately 64% of newly diagnosed breast cancer patients (1). For early stage breast cancer patients, adjuvant radiotherapy after breast conserving surgery is the most commonly utilized treatment approach (49%) (2). Recent advances in breast radiotherapy have enabled shorter treatment courses, smaller radiation fields, prone positioning, and less side effects associated with breast cancer treatment (3–10).
Magnetic Resonance-guided Radiotherapy (MRgRT) for breast cancer treatment is a potential high impact application of an emerging radiotherapy technology (11, 12). Potential advantages of radiotherapy utilizing on-table Magnetic Resonance Imaging (MRI) include enhanced visualization of the surgical cavity after lumpectomy and real time visualization and motion management of the cavity and nearby organs at risk (OARs). Furthermore, adaptive planning with MRgRT enables customized daily treatment planning to account for day-to-day anatomical variation. Finally, prone positioning during breast irradiation leads to decreased lung and heart radiation doses during treatment (3). By combining MRgRT and adaptive planning with prone positioning, a technique we call precision prone irradiation (PPI), we can improve the therapeutic index of breast radiotherapy by more accurately delivering radiation dose to the cavity target and decreasing toxicities associated with radiation to the surrounding normal tissues.
In this study, eleven patients with either early stage breast cancer or ductal carcinoma in situ (DCIS) were treated with PPI utilizing prone breast MRgRT and adaptive planning as their adjuvant breast radiotherapy treatment. To our knowledge, this is the first reported patient experience with this technology to date.
Materials and methods
Eleven patients with diagnosed DCIS or early stage breast cancer who underwent lumpectomy with negative margins underwent CT and MRI-based simulations and treatment planning. The patients consented for and underwent adjuvant breast radiotherapy treatments at New York Presbyterian Hospital – Weill Cornell Medicine with Eastern Cooperative Oncology Group Performance status (13) of 0-1 and histologically confirmed breast cancer between March 2021 and July 2021. This retrospective series included the first eleven consecutive patients who received prone MRI-guided breast radiotherapy and who are undergoing regular oncological follow-up examinations in our clinic. The characteristics of these eleven patients are shown in Table 1.
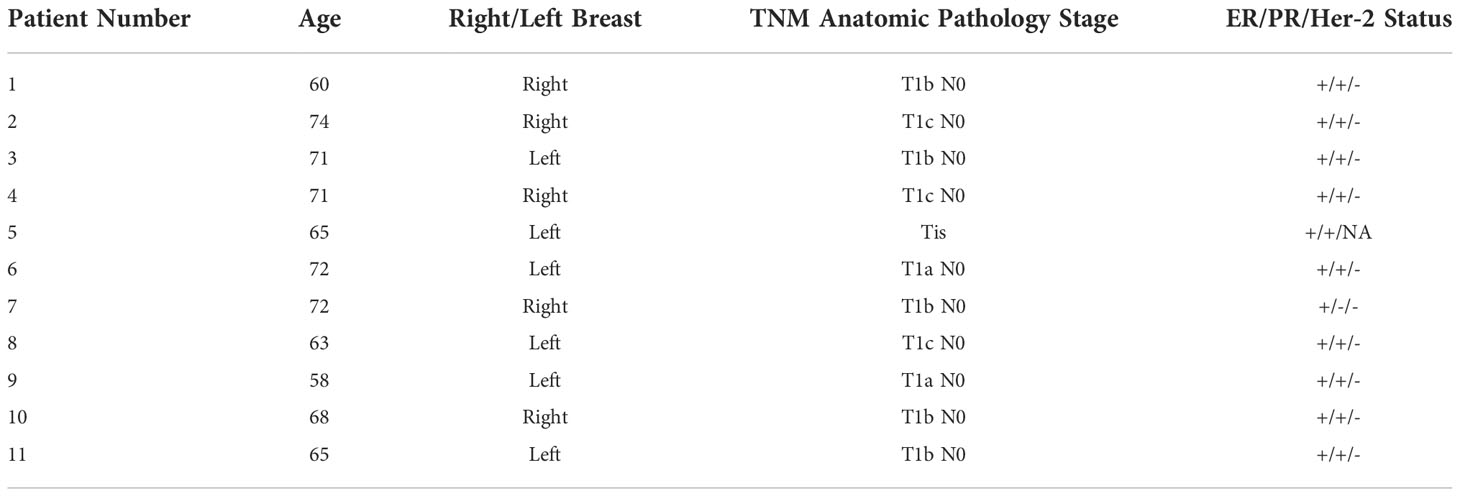
Table 1 Patients Characteristics – (NA: Not applicable, ER: Estrogen Receptor, PR: Progesterone Receptor).
Before simulation, all patients were screened for MRI compatibility. All patients were treated using a 0.35 T hybrid MR Linac system (MRIdian, ViewRay Inc., Mountain View, CA). All patients were planned in the prone breast treatment position within one to three months of surgical resection in accordance with previous published radiotherapy protocols (14, 15). The patients underwent MR simulation on the 0.35 MRIdian system using a balanced steady-state free progression (TrueFISP) imaging sequence. Planning CT using the same patient positioning was performed to acquire the electron density information for treatment planning. The two simulation image sets were fused and target contour delineation was performed on the CT and MRI simulation scans.
On each day of radiation treatment delivery, the surgical bed clinical target volume (CTV) and organs at risk (OAR) (left anterior descending artery, heart, lungs, and breast) were delineated on the MRI simulation scans. The CTV was isotropically expanded by 1.0 to 1.5 cm margins to define the planning target volume (PTV). The dose prescription was 30 Gy in 5 fractions given every other day. The planning and OAR constraints were based on previous published radiotherapy protocols (14, 15). Treatment planning was performed with Varian Eclipse and MRIdian treatment planning software (MC dose calculation algorithm) and treatment delivery consisted of a step and shoot IMRT technique. The linear accelerator radiation delivery utilizes a 6MV flattening free filter.
All patients received partial breast radiotherapy delivered in the prone treatment position with tangential Intensity Modulated Radiation Therapy (IMRT) fields. Each fractional treatment used an on-table adaptive planning workflow, where a new treatment plan was optimized after same day re-contouring of the anatomy from MRI imaging acquired on that day. As each fraction was adaptively planned, there were no imaging shifts used for patient setup. During the radiation delivery, continuous real-time 2D-cine-MRI was used to control for organ and target motion. A target structure was defined in the sagittal view of the volumetric MRI and a surrounding gating boundary contour was created by adding a tracking margin of 3 mm. An example of a surgical cavity target volume delineated from an MRI image acquired on-table on the day of radiation treatment is shown in Figure 1.
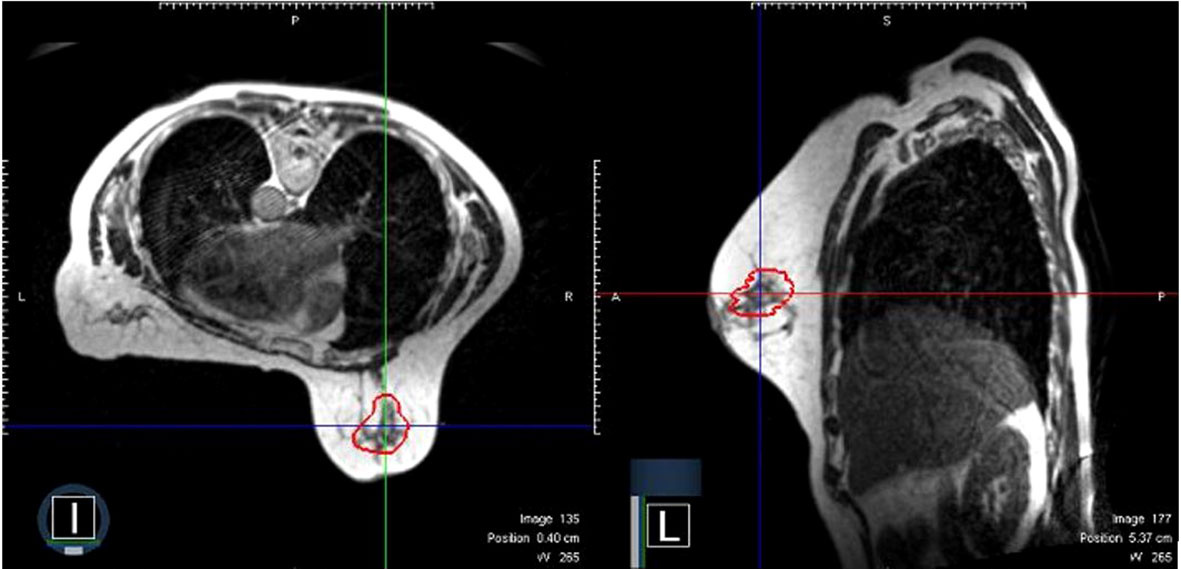
Figure 1 A representative surgical cavity target volume seen from an MRI image acquired on-table on the day of radiation treatment. The MRI scans in both the axial and sagittal planes are shown. The surgical cavity is outlined in red.
All standard radiation treatment plans and dosimetry parameters as described above were stored and analyzed on the Varian Eclipse and MRIdian treatment planning software system. Toxicity was assessed and reported according to Common Terminology Criteria for Adverse Events (CTCAE) v5 and recorded on the hospital’s electronic medical record system.
Results
All patients were able to complete their treatments as planned. Each radiotherapy fraction was adapted to the image acquired on the day of treatment and each adaptive plan successfully met pre-specified dose constraints.
For the delivered treatments based on the daily MRI images, the mean CTV size was 20.2 cc (range: 11.6-33.9 cc) and the mean PTV volume was 78.9 cc (56.8-124.6 cc). For the adapted fractional planning, the mean CTV size was 21.8 cc (3.9-37.4cc). The median LAD maximum dose was 44.5 cGy and the median mean heart dose was 15.5 cGy. The median percentage of the lung getting above 20 Gy dose was 0%. The median % of breast volume getting 100% and 50% of the prescription dose was 15.0% and 34.7% respectively. The average treatment time for an adapted fraction delivery was 38 minutes. Other pertinent dosimetry and treatment characteristics are summarized in Table 2.
Alternative treatment plans were developed based on the conventional CT simulation scans for the eleven patients. In comparison to the MRI plans, the mean CTV size based on alternative CT plan was greater, measuring 73.7 cc (range: 10.2–147.3 cc). The median difference in CTV volumes between CT-based and MRI-based planning was 45.2 cc (range 7.3 cc to 115.2 cc).
The median follow up for all eleven patients treated was 6 months. At time of this report, none of the patients have reported any Grade 2 or higher toxicities. All eleven patients tolerated treatment well with all patients reporting Grade 1 erythema of the skin which had resolved by their first follow up at 4 weeks post-radiotherapy. None of the patients reported any Grade 2 or higher fatigue or any additional adverse side effects.
Discussion
We report on our initial clinical experience of MRI-guided Radiation Therapy (MRgRT) with daily adaptive treatment planning for patients receiving breast radiotherapy in the prone position with a MRI-Linac radiotherapy system. As far as we are aware, this is the first reported clinical experience of breast radiotherapy delivered using the PPI technique.
In early-stage breast cancer management, there is significant momentum towards de-escalating intensity of treatment. Local recurrence rates in early stage disease have decreased over time and are reported to be less than 5% over 10 years of follow up in recent clinical trials (5, 14, 15). Nevertheless, fear of recurrence after treatment and long-term toxicities associated with treatment remain primary issues in survivorship, and local and distal breast cancer recurrence risk remains a concern even 20 years after treatment for early stage breast cancer patients (16).
De-escalation in breast radiotherapy has emphasized treating smaller target volumes and prioritizing avoiding nearby heart and lungs. A current standard breast radiotherapy modality involves external beam partial breast radiotherapy with CT-based image guidance. Several large Phase 3 trials have demonstrated good efficacy and toxicity of external beam partial radiotherapy when compared with whole breast radiotherapy (5, 15). In this report, we present our initial patient experience of precision prone irradiation (PPI) with MRI-guided prone breast adaptive breast radiotherapy for early stage breast cancer treatment. Our preliminary comparative results indicate that there may be a potential to decrease CTV and PTV volumes based on MRI guided planning.
Image-guided radiotherapy technologies are improving in sophistication and versatility to enhance the ability to target the at-risk regions and avoid healthy tissues during radiation treatments. The capability of on-table MRI guidance, adaptive planning, and delivery with the prone technique has several particular promise advantages to expand the therapeutic index of breast radiotherapy.
First, MRI enables the ability to delineate soft tissue and surgical cavities to a great resolution than CT-based imaging. This superior visualization by MRI guidance can allow more accurate surgical cavity and smaller target volume delineation as suggested from the results of our study. In addition, MRI guidance potentially allows CTV and PTV margins to be reduced from current standards due to better cavity determination and less setup uncertainty. Intra-fractional MR-guided imaging and decreased chest wall excursion with the prone setup furthers accounts for organ motion and can decrease targeting uncertainty (17). With potentially smaller radiation treatment volumes, this technique may decrease skin toxicities associated with breast radiotherapy with current published estimates of grade 2+ NCI CTCAE toxicity to be 15-20% with partial breast radiotherapy and 70-80% with whole breast radiotherapy (18).
The versatility of daily adaptive imaging, contouring, planning, and radiation delivery process can help mitigate the challenges of daily setup uncertainties for breast radiotherapy. The surgical cavity can be difficult to delineate without surgical clip or marker placement within the surgical bed, a practice that varies amongst surgeons. When surgical clips are placed to mark the cavity, they can migrate from their initial position within the breast over time. It is known that the surgical cavity can change through a course of breast radiotherapy (19). Furthermore, the positional setup and the position of the breast tissue can be highly variable during daily setups. Significant changes to the breast surface and topology can occur during the course of radiation treatment (20).
Similar to the breast target, nearby organs at risk can also change in relative position with daily treatments. Cardiac positioning can vary considerably relative to the bony anatomy and other anatomic landmarks used for patient positioning (21). Even with prone positioning, the left anterior descending coronary artery can receive significant radiation dose if daily imaging guidance is not utilized (13). For those patients where positional setup uncertainty is significant, it is recommended that more frequent image-guidance be utilized during breast radiotherapy, such as daily cone-beam CTs (22). PPI enables greater flexibility and potentially more accurate treatment delivery.
Incorporation of PPI and utilizing MR image guidance for breast radiation treatment planning enables minimization or potentially elimination of CT scanning for patients in the simulation and treatment delivery process. CT-based treatment planning often requires daily cone beam CT imaging for setup verification. For patients with high aversion to CT scans or tattoo marking, MRgRT offers the opportunity to potentially bypass the CT simulation, permanent skin tattooing, or regular cone beam CT image verifications which have become standard procedures in the contemporary breast radiotherapy delivery process.
At this stage of the PPI technology development, there are still significant limitations to broad clinical utilization in routine breast radiotherapy. MRI guidance, motion management, and daily adaptive planning are resource intensive and requires the presence of clinicians, dosimetrists, physicists, and therapists at each treatment. The time required for each delivered PPI fraction is longer than that for standard CT-imaged guided breast radiotherapy. The potential promise of smaller radiation treatment volumes through better target delineation and less target uncertainty and decreased toxicities would need to be validated through a prospective clinical trial.
With our reported experience, we hope to demonstrate potential advantages of PPI over conventional CT-based breast radiotherapy. The significance of these advantages will have to be established through prospective clinical trials. As further advances occur in this rapidly emerging field, we expect these clinical advantages and more therapeutic benefits to be realized from this versatile technique.
Data availability statement
The original contributions presented in the study are included in the article. Further inquiries can be directed to the corresponding author.
Ethics statement
The studies involving human participants were reviewed and approved by Weill Cornell Medicine Institutional Review Board. Written informed consent for participation was not required for this study in accordance with the national legislation and the institutional requirements.
Author contributions
All authors listed have made a substantial, direct, and intellectual contribution to the work and approved it for publication.
Conflict of interest
The authors declare that the research was conducted in the absence of any commercial or financial relationships that could be construed as a potential conflict of interest.
Publisher’s note
All claims expressed in this article are solely those of the authors and do not necessarily represent those of their affiliated organizations, or those of the publisher, the editors and the reviewers. Any product that may be evaluated in this article, or claim that may be made by its manufacturer, is not guaranteed or endorsed by the publisher.
Abbreviations
CTV, Clinical Target Volume; DCIS, Ductal Carcinoma in Situ; IMRT, Intensity Modulated Radiation Therapy; MRgRT, Magnetic Resonance guided Radiation Therapy; OAR, Organs at Risk; PBI, Partial Breast Irradiation; PPI, Precision Prone Irradiation; PTV, Planning Target Volume.
References
1. American Cancer Society. Breast cancer facts & figures. (2022-2024). Atlanta: American Cancer Society, Inc. 2022.
2. Miller KD, Nogueira L, Mariotto AB, Rowland JH, Yabroff KR, Alfano CM, et al. Cancer treatment and survivorship statistics, 2019. CA Cancer J Clin (2019) 69(5):363–85. doi: 10.3322/caac.21565
3. Formenti SC, DeWyngaert JK, Jozsef G, Goldberg JD. Prone vs supine positioning for breast cancer radiotherapy. JAMA (2012) 308(9):861–3. doi: 10.1001/2012.jama.10759
4. Whelan TJ, Pignol JP, Levine MN, Julian JA, MacKenzie R, Parpia S, et al. Long-term results of hypofractionated radiation therapy for breast cancer. N Engl J Med (2010) 362:513–20. doi: 10.1056/NEJMoa0906260
5. Vicini FA, Cecchini RS, White JR, Arthur DW, Julian TB, Rabinovitch RA, et al. Long-term primary results of accelerated partial breast irradiation after breast-conserving surgery for early-stage breast cancer: a randomised, phase 3, equivalence trial. Lancet (2019) 394(10215):2155–64. doi: 10.1016/S0140-6736(19)32514-0
6. Huntzinger C, Munro P, Johnson S, Miettinen M, Zankowski C, Ahlstrom G, et al. Dynamic targeting image-guided radiotherapy. Med Dosim (2006) 31(2):113–25. doi: 10.1016/j.meddos.2005.12.014
7. Shah C, Obi E, Tom MC, Manyam BV, Obi B, Al-Hilli Z, et al. Initial outcomes with image-guided partial breast irradiation delivered with intensity-modulated radiation therapy. Breast J (2020) 26(2):227–30. doi: 10.1111/tbj.13544
8. Formenti SC, Truong MT, Goldberg JD, Mukhi V, Rosenstein B, Roses D, et al. Prone accelerated partial breast irradiation after breast-conserving surgery: preliminary clinical results and dose-volume histogram analysis. Int J Radiat Oncol Biol Phys (2004) 60(2):493–504. doi: 10.1016/j.ijrobp.2004.04.036
9. Huppert N, Jozsef G, Dewyngaert K, Formenti SC. The role of a prone setup in breast radiation therapy. Front Oncol (2011) 1:31. doi: 10.3389/fonc.2011.00031
10. Merchant TE, McCormick B. Prone position breast irradiation. Int J Radiat Oncol Biol Phys (1994) 30(1):197–203. doi: 10.1016/0360-3016(94)90535-5
11. Mutic S, Dempsey JF. The ViewRay system: magnetic resonance-guided and controlled radiotherapy. Semin Radiat Oncol (2014) 24(3):196–9. doi: 10.1016/j.semradonc.2014.02.008
12. Klüter S. Technical design and concept of a 0.35 T MR-linac. Clin Transl Radiat Oncol (2019) 18:98–101. doi: 10.1016/j.ctro.2019.04.007
13. Yao S, Zhang Y, Nie K, Liu B, Haffty BG, Ohri N, et al. Setup uncertainties and the optimal imaging schedule in the prone position whole breast radiotherapy. Radiat Oncol (2019) 14(1):76. doi: 10.1186/s13014-019-1282-4
14. Formenti SC, Hsu H, Fenton-Kerimian M, Roses D, Guth A, Jozsef G, et al. Prone accelerated partial breast irradiation after breast-conserving surgery: five-year results of 100 patients. Int J Radiat Oncol Biol Phys (2012) 84(3):606–11. doi: 10.1016/j.ijrobp.2012.01.039
15. Meattini I, Marrazzo L, Saieva C, Desideri I, Scotti V, Simontacchi G, et al. Accelerated partial-breast irradiation compared with whole-breast irradiation for early breast cancer: Long-term results of the randomized phase III APBI-IMRT-Florence trial. J Clin Oncol (2020) 38(35):4175–83. doi: 10.1200/JCO.20.00650
16. Pan H, Gray R, Braybrooke J, Davies C, Taylor C, McGale P, et al. EBCTCG. 20-year risks of breast-cancer recurrence after stopping endocrine therapy at 5 years. N Engl J Med (2017) 377(19):1836–46. doi: 10.1056/NEJMoa1701830
17. Merfeld EC, Blitzer GC, Kuczmarska-Haas A, Witt JS, Wojcieszynski AP, Mittauer KM, et al. Considering lumpectomy cavity PTV expansions: Characterization of intrafraction lumpectomy cavity motion. Pract Radiat Oncol (2022) 9:S1879–8500(22)00266-1. doi: 10.1016/j.prro.2022.08.011
18. Reddy JP, Lei X, Bloom ES, Reed VK, Schlembach PJ, Arzu I, et al. Optimizing preventive adjuvant linac-based (OPAL) radiation: A phase II tial of daily partial breast irradiation. Int J Radiat Oncol Biol Phys (2022) 7:S0360–3016(22)03419-8. doi: 10.1016/j.ijrobp.2022.09.083
19. Lee G, Parmar H, Li W, Shessel A. The effect of lumpectomy cavity changes on planning dose in breast radiotherapy boost. J Med Imaging Radiat Sci (2019) 50(2):317–22. doi: 10.1016/j.jmir.2019.02.002
20. Seppälä J, Vuolukka K, Virén T, Heikkilä J, Honkanen JTJ, Pandey A, et al. Breast deformation during the course of radiotherapy: The need for an additional outer margin. Phys Med (2019) 65::1–5. doi: 10.1016/j.ejmp.2019.07.021
21. Topolnjak R, Borst GR, Nijkamp J, Sonke JJ. Image-guided radiotherapy for left-sided breast cancer patients: geometrical uncertainty of the heart. Int J Radiat Oncol Biol Phys (2012) 82(4):e647-55. doi: 10.1016/j.ijrobp.2011.08.024
Keywords: breast radiation therapy, partial breast irradiation, magnetic resonance image guided radiation therapy, precision radiation oncology, image guided adaptive radiotherapy
Citation: Ng J, Pennell R and Formenti SC (2022) The initial experience of MRI-guided precision prone breast irradiation with daily adaptive planning in treating early stage breast cancer patients. Front. Oncol. 12:1048512. doi: 10.3389/fonc.2022.1048512
Received: 19 September 2022; Accepted: 01 November 2022;
Published: 23 November 2022.
Edited by:
Ricardo Otazo, Memorial Sloan Kettering Cancer Center, United StatesReviewed by:
Heloisa De Andrade Carvalho, Clinical Hospital, Faculty of Medicine, University of São Paulo, BrazilIvica Ratosa, Institute of Oncology Ljubljana, Slovenia
Copyright © 2022 Ng, Pennell and Formenti. This is an open-access article distributed under the terms of the Creative Commons Attribution License (CC BY). The use, distribution or reproduction in other forums is permitted, provided the original author(s) and the copyright owner(s) are credited and that the original publication in this journal is cited, in accordance with accepted academic practice. No use, distribution or reproduction is permitted which does not comply with these terms.
*Correspondence: John Ng, am9uOTAyNEBtZWQuY29ybmVsbC5lZHU=