- Department of Health Sciences, Section of Clinical Pharmacology and Oncology, University of Florence, Florence, Italy
Editorial on the Research Topic
Microbiota and metabolites in cancer immunotherapy
In the last two decades, immunotherapy has transformed cancer treatment, resulting in unprecedented survival improvement in several advanced and metastatic malignancies. Immune checkpoint inhibitors (ICIs) of cytotoxic T-lymphocyte antigen (CTLA-4) and programmed death/ligand-1 (PD-1/PD-L1) are the main immunotherapeutic tools currently approved, alone or in combination, for the treatment of many tumors (1). However, the downsides of these drugs, including the development of primary or secondary resistance mechanisms to immune-related and occasionally fatal side effects, are well known. Attempts by researchers to identify biomarkers capable of predicting the response to ICIs have been extensive, although not yet completely satisfactory. Continually emerging evidence has confirmed the role of gut microbiota (GM) as immune modulators that can influence the efficacy and toxicity of ICIs (2).
The purpose of this Research Topic is to highlight the evidence regarding the relationship between immunotherapy and GM, and examine the possibility of modulating GM to favor beneficial therapeutic outcomes and improve clinical tolerance to immunotherapy (Figure 1).
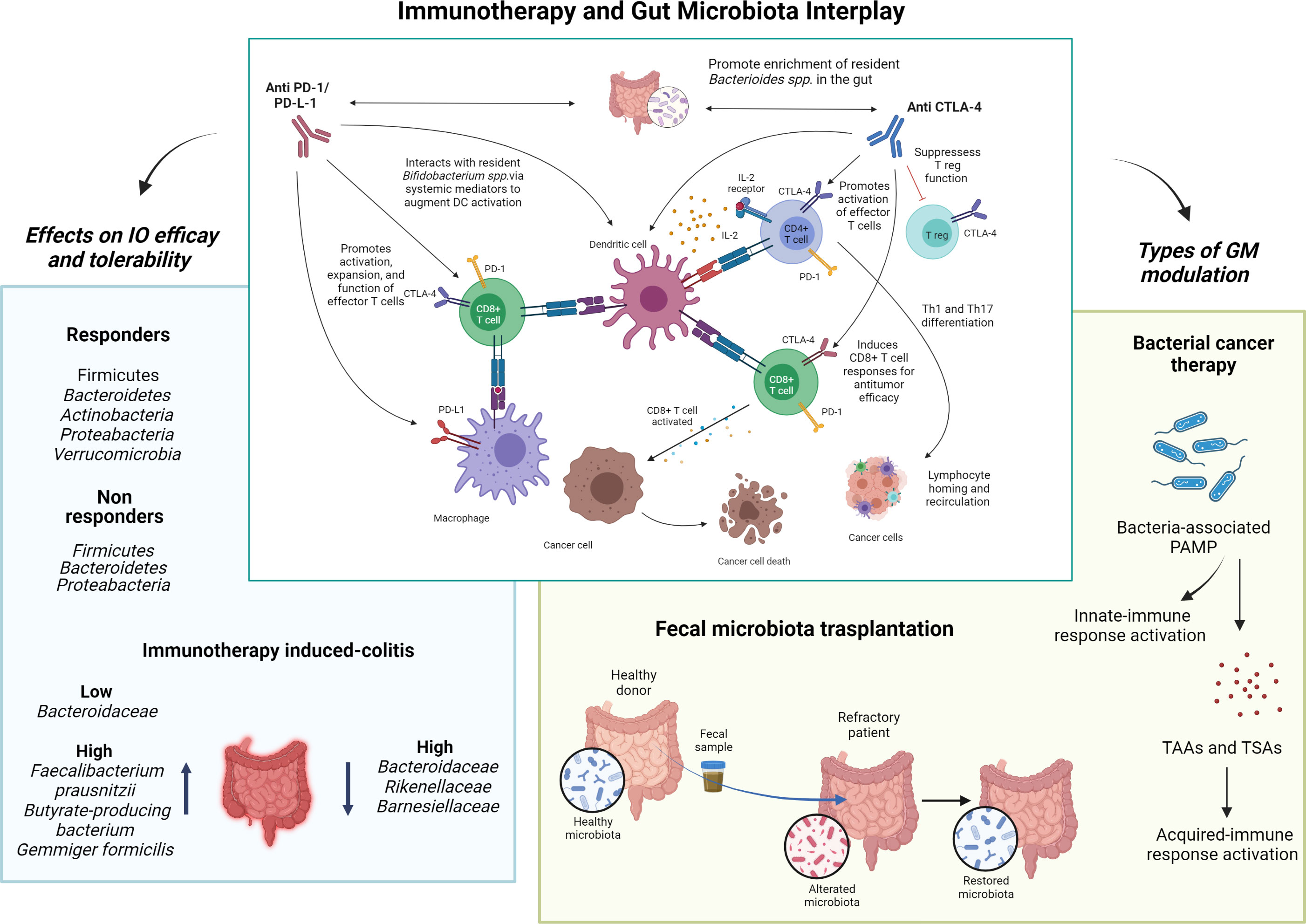
Figure 1 Immunotherapy and gut microbiota interplay. The interaction between gut microbiota and immune checkpoint inhibitors, and the expected consequences on the immune system, is represented in the center. Left, the impact of different microbial species on the efficacy of immune checkpoint inhibitors and immune-related toxicity is shown. Right, the two main gut microbiota modulation strategies discussed in this Research Topic are shown as possible tools for improving the efficacy and toxicity of immunotherapy. I-O, immuno-oncology; GM, gut microbiota; PD-(L)1, programmed death cell-(ligand)1; CTLA-4, cytotoxic T-lymphocytes associated protein-4; PAMP, molecular patterns associated with pathogens; TAAs, tumor-associated antigens; TASs, tumor-specific antigens. Image created using BioRender.com.
Yang et al. conducted a bibliometric analysis of studies evaluating the role of GM in cancer immunotherapy, especially ICIs, and noted that there was a rapid increase in publications in the last 10 years, particularly after 2018. The aim of the study was to understand, through scientometric and visual analysis, the current state of global research regarding interactions between GM and immunotherapy, and anticipate future development directions.
More specifically, Zhou et al. summarized the published research examining the role of microbiota in anti-PD1/PD-L1 therapy, illustrating the underlying mechanisms and providing information on GM manipulation for facilitating PD1/PD-L1 blockade. When considering the literature regarding the synergistic effects of Bacteroides fragilis and CTLA-4 blockers, it has been estimated that GM can equally influence the therapeutic effect of anti-PD1/PD-L1. Evidence suggests that gut flora likely functions in modulating PD1/PD-L1 blockade through the translocation of bacteria or dispatch of bacteria-derived molecules to enhance antigenicity and attempt an antitumor immune response. This has been confirmed by observations that some types of microbiotas are explicitly enriched in ICI-responsive patients, whereas others correspond to PD1/PD-L1 non-responders (3–7). With regard to immune-related adverse events (irAEs), although anti-CTLA-4 enrichment of Bacteroidetes seems to correlate with a lower risk of mediated colitis, the bacterial species related to the reduced toxicity of anti-PD-1/PD-L1 has not yet been identified (8). Based on the available literature, of all bacteria, those belonging to the Ruminococacea family seem to be able to optimize the response to ICI therapy and irAEs (9).
As the effectiveness and toxicity of ICIs seems to be influenced by GM, preclinical data evaluated GM manipulation as a possible technique for enhancing immunotherapy (3, 10, 11). Several approaches, ranging from target interventions, such as bacteriophages and specific bacterial metabolites, to broader strategies, such as antibiotics, pre or probiotics, dietary changes, and fecal microbiota transplantation (FMT), have been tested for their utility in modulating the gut microbiome (12). Of these, FMT is the most direct approach. FMT involves isolating fecal microbiota from stool donated by healthy people and then transplanting them into the patient’s intestine by perfusion or oral administration (13). Xu et al. recently reported that FMT produces a change in patients’ refractory to ICI, making it a promising approach for cancer treatment. Preclinical mouse models demonstrate that FMT can modulate the sensitivity of patients with malignant tumors to ICIs by altering the tumor microenvironment (TME) and enhancing tumor immunogenicity. In patients with immunotherapy-refractory metastatic melanoma, the combination of FMT and anti-PD-1 has recently been shown to be safe, practical, and potentially effective (14). However, in accordance with the authors’ conclusion, although FMT, by remodeling the GM, shows promise in improving the therapeutic efficacy of immunotherapy by reducing side effects, further studies are needed to validate its safety and define the optimal administration regimen for cancer treatment.
By contrast to FMT, bacteria-mediated cancer therapy (BCT) is a method with much older origins in cancer treatment. Starting with Coley’s Toxin in 1983, several strains of therapeutic bacteria have been identified, showing remarkable therapeutic efficacy in preclinical and clinical models (15).
As shown by Luo et al., therapeutic bacteria are able to penetrate and colonize hypoxic areas in tumors, and are sometimes more effective than traditional cancer therapies (16–18). The combination of BCT and chemotherapy has been shown to inhibit tumor growth and prolong survival in preclinical studies, as well as significantly enhance antitumor activity by modulating tumor angiogenesis and host immune response (19, 20). Additionally, in combination with radiotherapy, BCT has been shown to reduce tumor growth in preclinical studies (21), reducing radiotherapy damage in the surrounding tissues (22). Another advantage of administrating live or inactivated bacteriological preparations involves their substantial influence on immune responses. This influence is caused by the action of the molecular patterns associated with pathogens (PAMP), which initiate prompt innate immune responses, characterized by the accumulation of granulocytes and macrophages, as well as an increase in proinflammatory cytokines and chemokines (23–25). Intense bacterial infection and the subsequent innate immune response results in the lysis of tumor cells and the release of tumor-associated and tumor-specific antigens (26), the presence of which in the antigen presenting cell marks the shift to the acquired immune responses. These results provide hope for a possible clinical translation of BCT; however, the use of live bacteria, although highly attenuated, still poses a serious risk of infection and patients must be carefully selected.
Author contributions
GR had full access to all the data in the study and takes responsibility for the integrity of the data and the accuracy of the data analysis. Study concept and design: GR, MC. Acquisition of data: GR, MC. Analysis and interpretation of data: GR. Drafting of the manuscript: GR. Critical revision of the manuscript for important intellectual content: MC. Statistical analysis: GR. Obtaining funding: None. Administrative, technical, or material support: None Supervision: MC. Financial disclosures: None. Funding/Support and role of the sponsor: None. All authors contributed to the article and approved the submitted version.
Conflict of interest
The authors declare that the research was conducted in the absence of any commercial or financial relationships that could be construed as a potential conflict of interest.
Publisher’s note
All claims expressed in this article are solely those of the authors and do not necessarily represent those of their affiliated organizations, or those of the publisher, the editors and the reviewers. Any product that may be evaluated in this article, or claim that may be made by its manufacturer, is not guaranteed or endorsed by the publisher.
References
1. FDA Approval Timeline of Active Immunotherapies - Cancer Research Institute. Available at: https://www.cancerresearch.org/fda-approval-timeline-of-active-immunotherapies.
2. Roviello G, Iannone LF, Bersanelli M, Mini E, Catalano M. The gut microbiome and efficacy of cancer immunotherapy. Pharmacol Ther (2022) 231:107973. doi: 10.1016/j.pharmthera.2021.107973
3. Sivan A, Corrales L, Hubert N, Williams JB, Aquino-Michaels K, Earley ZM, et al. Commensal bifidobacterium promotes antitumor immunity and facilitates anti-PD-L1 efficacy. Science (2015) 350:1084–9. doi: 10.1126/science.aac4255
4. Gopalakrishnan V, Spencer CN, Nezi L, Reuben A, Andrews MC, Karpinets TV, et al. Gut microbiome modulates response to anti-PD-1 immunotherapy in melanoma patients. Science (2018) 359:97–103. doi: 10.1126/science.aan4236
5. Routy B, Le Chatelier E, Derosa L, Duong CPM, Alou MT, Daillère R, et al. Gut microbiome influences efficacy of PD-1–based immunotherapy against epithelial tumors. Science (2018) 359:91–7. doi: 10.1126/science.aan3706
6. Jin Y, Dong H, Xia L, Yang Y, Zhu Y, Shen Y, et al. The diversity of gut microbiome is associated with favorable responses to anti–programmed death 1 immunotherapy in Chinese patients with NSCLC. J Thorac Oncol (2019) 14:1378–89. doi: 10.1016/j.jtho.2019.04.007
7. Matson V, Fessler J, Bao R, Chongsuwat T, Zha Y, Alegre ML, et al. The commensal microbiome is associated with anti-PD-1 efficacy in metastatic melanoma patients. Science (2018) 359:104–8. doi: 10.1126/science.aao3290
8. Dubin K, Callahan MK, Ren B, Khanin R, Viale A, Ling L, et al. Intestinal microbiome analyses identify melanoma patients at risk for checkpoint-blockade-induced colitis. Nat Commun (2016) 7:10391. doi: 10.1038/ncomms10391
9. Chaput N, Lepage P, Coutzac C, Soularue E, Le Roux K, Monot C, et al. Baseline gut microbiota predicts clinical response and colitis in metastatic melanoma patients treated with ipilimumab. Ann Oncol (2017) 28:1368–79. doi: 10.1093/annonc/mdx108
10. Panebianco C, Andriulli A, Pazienza V. Pharmacomicrobiomics: exploiting the drug-microbiota interactions in anticancer therapies. Microbiome (2018) 6:92. doi: 10.1186/s40168-018-0483-7
11. Vétizou M, Pitt JM, Daillère R, Lepage P, Waldschmitt N, Flament C, et al. Anticancer immunotherapy by CTLA-4 blockade relies on the gut microbiota. Science (2015) 350:1079–84. doi: 10.1126/science.aad1329
12. Fessler J, Matson V, Gajewski TF. Exploring the emerging role of the microbiome in cancer immunotherapy. J ImmunoTher Cancer (2019) 7:1–15. doi: 10.1186/s40425-019-0574-4
13. Zhang J, Wu K, Shi C, Li G. Cancer immunotherapy: Fecal microbiota transplantation brings light. Curr Treat Options Oncol (2022) 24:1–16. doi: 10.1007/s11864-022-01027-2
14. Baruch EN, Youngster I, Ben-Betzalel G, Ortenberg R, Lahat A, Katz L, et al. Fecal microbiota transplant promotes response in immunotherapy-refractory melanoma patients. Science (2021) 371:602–9. doi: 10.1126/science.abb5920
15. Liang S, Wang C, Shao Y, Wang Y, Xing D, Geng Z. Recent advances in bacteria-mediated cancer therapy. Front Bioeng Biotechnol (2022) 10:1026248. doi: 10.3389/fbioe.2022.1026248
16. Wang X, Liu Z, Sui X, Wu Q, Wang J, Xu C. Elemene injection as adjunctive treatment to platinum-based chemotherapy in patients with stage III/IV non-small cell lung cancer: A meta-analysis following the PRISMA guidelines. Phytomedicine (2019) 59:152787. doi: 10.1016/j.phymed.2018.12.010
17. Li Y, Sui X, Su Z, Yu C, Shi X, Johnson NL, et al. Meta-analysis of paclitaxel-based chemotherapy combined with traditional Chinese medicines for gastric cancer treatment. Front Pharmacol (2020) 11:132. doi: 10.3389/fphar.2020.00132
18. Cheng H, Ge X, Zhuo S, Gao Y, Zhu B, Zhang J, et al. β-elemene synergizes with gefitinib to inhibit stem-like phenotypes and progression of lung cancer via down-regulating EZH2. Front Pharmacol (2018) 9:1413. doi: 10.3389/fphar.2018.01413
19. Chen J, Qiao Y, Tang B, Chen G, Liu X, Yang B, et al. Modulation of salmonella tumor-colonization and intratumoral anti-angiogenesis by triptolide and its mechanism. Theranostics (2017) 7:2250–60. doi: 10.7150/thno.18816
20. Din MO, Danino T, Prindle A, Skalak M, Selimkhanov J, Allen K, et al. Synchronized cycles of bacterial lysis for in vivo delivery. Nature (2016) 536:81–5. doi: 10.1038/nature18930
21. Liu X, Jiang S, Piao L, Yuan F. Radiotherapy combined with an engineered salmonella typhimurium inhibits tumor growth in a mouse model of colon cancer. Exp Anim (2016) 65:413–8. doi: 10.1538/expanim.16-0033
22. Bettegowda C, Dang LH, Abrams R, Huso DL, Dillehay L, Cheong I, et al. Overcoming the hypoxic barrier to radiation therapy with anaerobic bacteria. Proc Natl Acad Sci United States America (2003) 100:15083–8. doi: 10.1073/pnas.2036598100
23. Avogadri F, Martinoli C, Petrovska L, Chiodoni C, Transidico P, Bronte V, et al. Cancer immunotherapy based on killing of salmonella-infected tumor cells. Cancer Res (2005) 65:3920–7. doi: 10.1158/0008-5472.CAN-04-3002
24. Gu W, Wang L, Wu Y, Liu JP. Undo the brake of tumour immune tolerance with antibodies, peptide mimetics and small molecule compounds targeting PD-1/PD-L1 checkpoint at different locations for acceleration of cytotoxic immunity to cancer cells. Clin Exp Pharmacol Physiol (2019) 46:105–15. doi: 10.1111/1440-1681.13056
25. Xie Q, Li F, Fang L, Liu W, Gu C. The antitumor efficacy of β -elemene by changing tumor inflammatory environment and tumor microenvironment. BioMed Res Int (2020) 2020. doi: 10.1155/2020/6892961
26. Chen Q, Bai H, Wu W, Huang G, Li Y, Wu M, et al. Bioengineering bacterial vesicle-coated polymeric nanomedicine for enhanced cancer immunotherapy and metastasis prevention. Nano Lett (2020) 20(1):11–21. doi: 10.1021/acs.nanolett.9b02182
27. Yi M, Jiao D, Qin S, Chu Q, Li A, Wu K. Manipulating gut microbiota composition to enhance the therapeutic effect of cancer immunotherapy. Integr Cancer Ther (2019) 18. doi: 10.1177/1534735419876351
Keywords: immunotherapy, cytotoxic T-lymphocyte antigen (CTLA-4), gut microbiota, programmed death/ligand-1, immune checkpoint inhibitors
Citation: Roviello G and Catalano M (2022) Editorial: Microbiota and metabolites in cancer immunotherapy. Front. Oncol. 12:1093941. doi: 10.3389/fonc.2022.1093941
Received: 09 November 2022; Accepted: 11 November 2022;
Published: 06 December 2022.
Edited and Reviewed by:
Katy Rezvani, University of Texas MD Anderson Cancer Center, United StatesCopyright © 2022 Roviello and Catalano. This is an open-access article distributed under the terms of the Creative Commons Attribution License (CC BY). The use, distribution or reproduction in other forums is permitted, provided the original author(s) and the copyright owner(s) are credited and that the original publication in this journal is cited, in accordance with accepted academic practice. No use, distribution or reproduction is permitted which does not comply with these terms.
*Correspondence: Giandomenico Roviello, giandomenicoroviello@hotmail.it