- 1Department of Medical Sciences, University of Ferrara, Ferrara, Italy
- 2Department of Translational Medicine and for Romagna, University of Ferrara, Ferrara, Italy
- 3Department of Specialized Surgery, Section of Ophthalmology, Sant’Anna University Hospital, Ferrara, Italy
- 4Department of Head and Neck, Section of Ophthalmology, San Paolo Hospital, Savona, Italy
- 5Department of Neuroscience and Rehabilitation, Section of Ophthalmology, University of Ferrara, Ferrara, Italy
Uveal melanoma (UM) is the most frequently found primary intraocular tumor, although it accounts for only 5% of all melanomas. Despite novel systemic therapies, patient survival has remained poor. Indeed, almost half of UM patients develop metastases from micro-metastases which were undetectable at diagnosis. Genetic analysis is crucial for metastatic risk prediction, as well as for patient management and follow-up. Several prognostic parameters have been explored, including tumor location, basal dimension and thickness, histopathologic cell type, vascular mimicry patterns, and infiltrating lymphocytes. Herein, the Authors review the available literature concerning cytogenetic prognostic markers and biochemical pathways correlated to UM metastasis development.
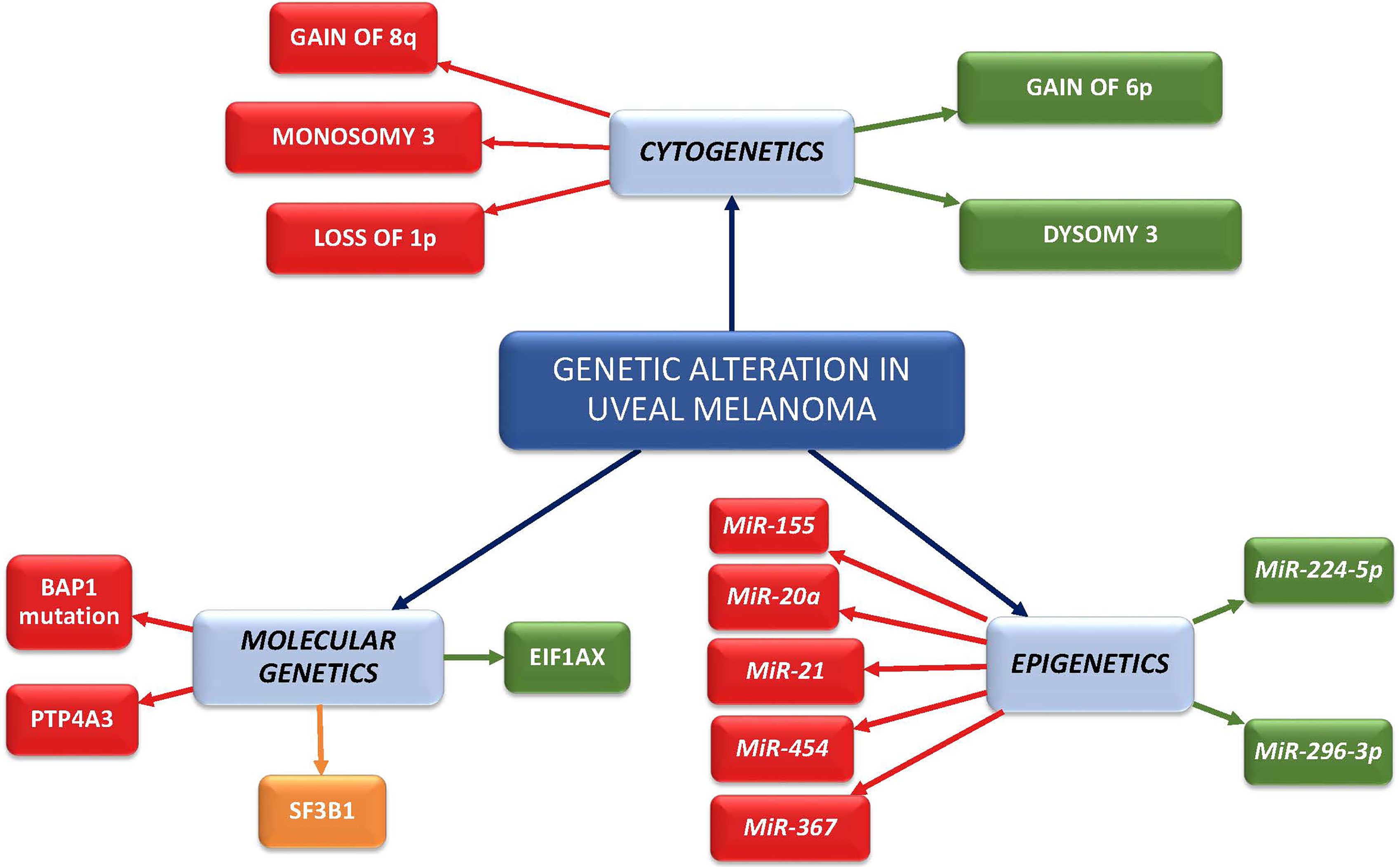
Graphical Abstract Red boxes represent high metastatic risk factors; orange box represents intermediate metastatic risk factor (late onset metastasis); green boxes represent low metastatic risk factors.
1 Introduction
Uveal melanoma (UM), though a relatively rare disease, is the most frequent primary malignant eye tumor in Caucasian adults. While local tumor control is outstanding, metastasis-related mortality remains relatively high. Almost 50% of patients develop metastases within 10 years of diagnosis, commonly in the liver (89%), lung (29%) and bone (17%) (1, 2) regardless of the type of treatment (3).
Several histopathological, clinical, radiological, cytogenetic and gene expression features can be used to estimate patient metastatic risk and prognosis (4) (Figures 1, 2).
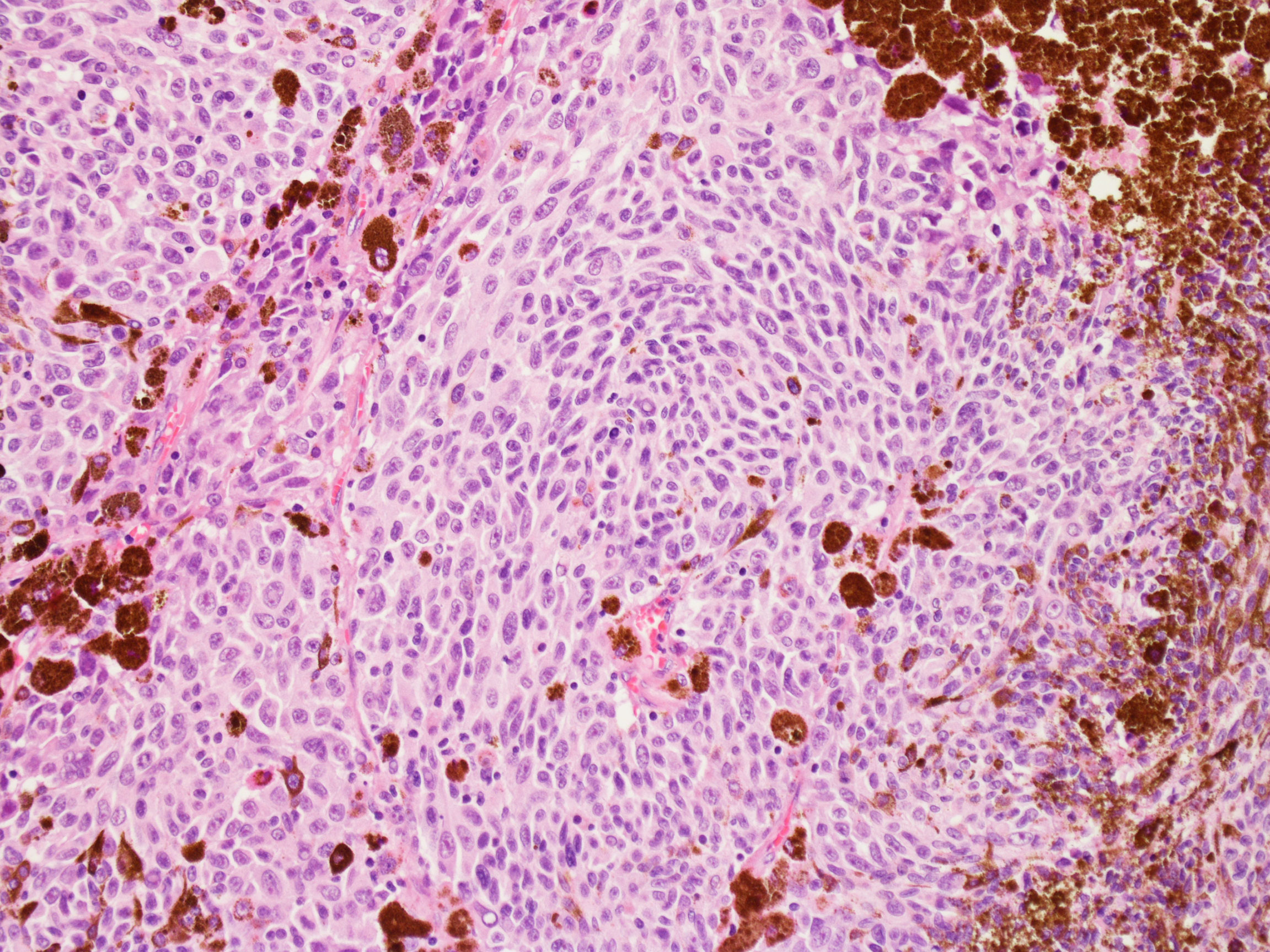
Figure 1 Haematoxylin-eosin histopathological analysis of uveal melanoma. (With permission of Prof. Giovanni Lanza, Institute of Pathological Anatomy, University of Ferrara).
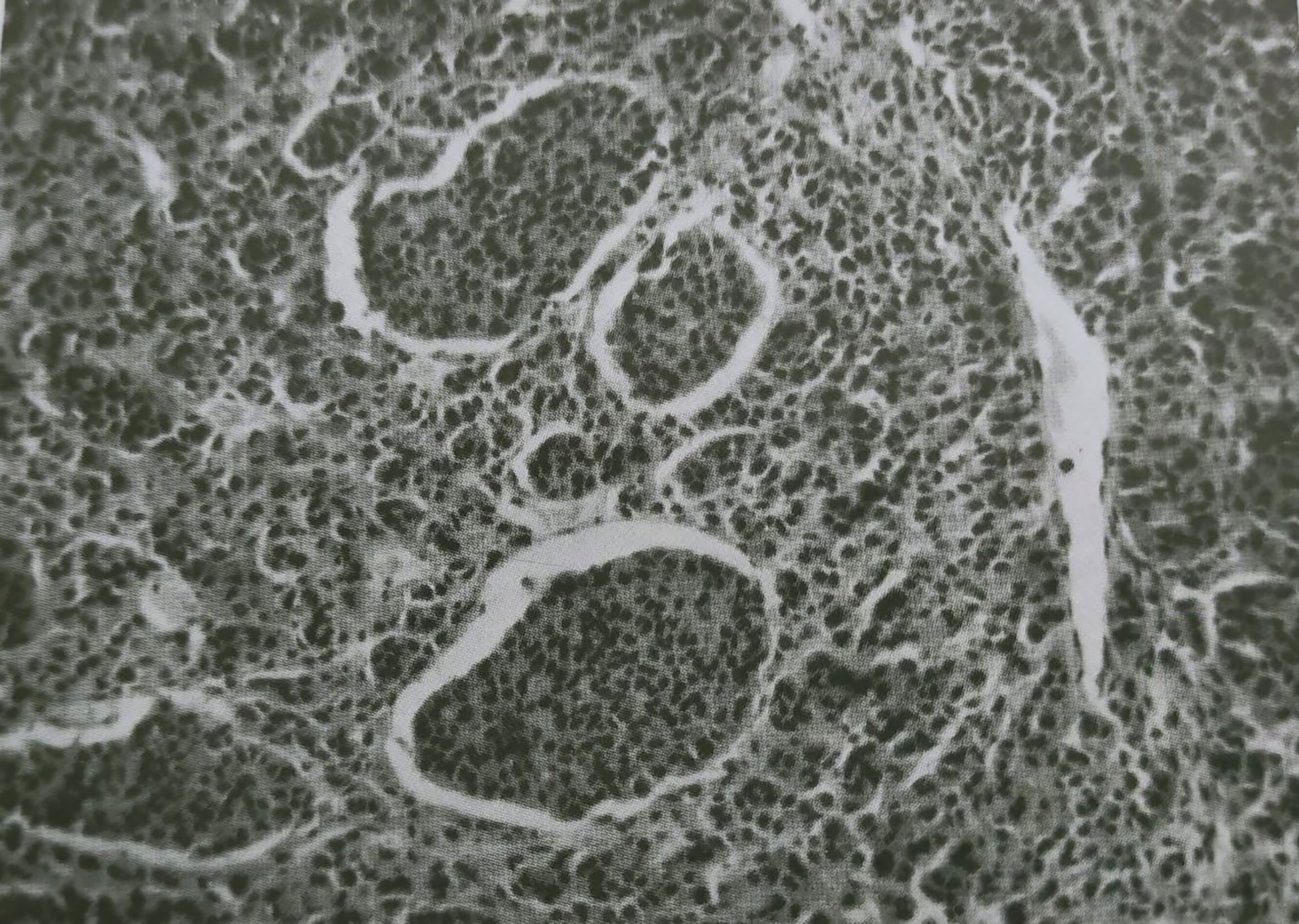
Figure 2 Malignant choroidal melanoma showing masses of neoplastic epithelioid cells invading a tumor vessel lumen. (Reproduced with permission from Prof. Gallenga P.E., Rass. Ital. Ottal.; published by Clinica oculistica dell’Università di Torino, 1961).
For example, cell type, tumor basal diameter, location of tumor epicenter, anterior tumor extension and tumor diameter, are known to correlate with distinct risk classes of melanoma-related survival (5, 6). Other features have also been studied, including Ki-67 proliferation index, inflammatory phenotype, deeper scleral extension, mixed/epithelioid cell type, as well as extracellular matrix patterns and high mitotic figures (7).
Additionally, certain clinical characteristics have been proven to be outcome predictors for UM patients: developing UM before the age of 21 is associated with better prognosis, compared to middle or older-age patients (8), while metastatic risk seems higher for males than females (9, 10).
Cytogenetic studies have highlighted the role of genetic factors, such as chromosomal aberrations and genetic mutations, in predicting patient survival. Indeed, tumors with monosomy 3 or gain of 8q are associated with poor prognosis. Monosomy 3 is also an independent risk factor for metastasis, if corrected for tumor site and diameter, whereas it correlates with significantly reduced disease-free survival (11). On the other hand, gain of 6p correlates with good prognosis, while loss of chromosome 1p and chromosome 8 have significant prognostic value, independently (12, 13). Single Nucleotide Polymorphism (SNP)-array analysis seems to be the most sensitive technique for investigating these chromosomal aberrations (14).
As for prognostic gene mutations, BAP1 loss (BRCA1-associated protein, located on chromosome 3), strictly correlates with metastasis development and poor prognosis. Additionally, BAP1 loss is associated with monosomy 3 (15–17).
Finally, UM patients can be classified based on gene expression profiling through high-resolution, genome-wide Comparative Genomic Hybridization (CGH) or SNP array techniques (18–20), and Multiplex ligation dependent probe amplification (MLPA) (21). Two profiles can be distinguished: class 1 tumors lead to good overall survival and low metastatic risk, whereas class 2 tumors are more likely to metastasize (20, 22). Alongside gene expression profiling, the ability to differentiate these two subgroups also relies on mutational status and micro-RNA expression analysis (23), proving that the deregulation of certain microRNAs has prognostic significance (24).
In this review, the genetic and molecular basis of uveal melanoma is reported on, with a focus on biomarkers that appear to underline prognosis and metastasis.
2 Pathological and Radiological Prognostic Factors
A multidisciplinary approach is mandatory for an accurate diagnostic and therapeutic management of UM.
Classical histologic findings have an impact on patient outcome, such as cell type, greatest thickness and largest basal diameter of the tumor, proliferative activity (both mitotic index and Ki67 proliferation rate) and specific growth pattern. Other useful histopathologic data are the deeper scleral extension, particularly the possible evidence of extraocular extension, with infiltration of the sclera along emissary nerves and vessels. Tumors confined to the choroid have basically a more favorable prognosis than melanomas extending into the ciliary body, so that the exact location of the lesion is important too (25).
The potential presence of prominent tumor-infiltrating lymphocytes and tumor-associated macrophages should be reported, as they seem to have a correlation with a higher risk of metastasis. A strong independent association with tumor-related mortality is linked to closed loops of PAS-positive material encircling tumor cells. Highly invasive tumor cells give rise to these loops through a mechanism of vasculogenic mimicry, allowing conduction of red blood cells and plasma cells (26, 27).
Measurements of UM are routinely acquired by US high frequency probes. Nevertheless, large tumors may have basal diameter too large for the limited field of view of US transducer and 2D US measurements of irregular shapes may be operator dependent. Accurate 3D volumetric data obtained through MRI are useful to evaluate the maximum tumor thickness (including sclera thickness), basal diameters of UM and possible extra-scleral or orbital extension (28, 29).
Some authors argue that a high degree of pigmentation (depending on the melanin content) may be associated with a less favorable prognosis. MRI can provide a quantitative evaluation of whole lesion pigmentation that seems to be more related to histopathologic examination. On the other hand, ophthalmoscopy allows a qualitative assessment of the melanin distribution, evaluating only the ventral portion of the tumor (30).
MRI should be included in the diagnostic management of selected UM. Thus, its role would be expanded from diagnostic to prognostic, with a positive impact on the therapeutic personalized planning (31).
3 Genomic Abnormalities in Uveal Melanoma
3.1 Cytogenetics
Cytogenetic rearrangements in UM have been extensively studied and mainly affect chromosomes 1, 3, 6, and 8. Among those studied, monosomy 3 appears as an early event in 50–60% of tumors and is often associated with isochromosome 8q. Isochromosome 8q is prone to segregate abnormally during mitosis and generates high levels of 8q gain. The correlation between these rearrangements and the risk of metastasis has been studied in the literature (11). Despite its pivotal role in the early identification of chromosome abnormalities in UM, karyotypic analysis has the serious limitation of being applicable only to large tumor samples obtained by enucleation and local resection (11).
Recently, several authors have focused their attention on the genetic evolution of uveal melanoma, which leads from primary tumor to metastatic disease. This is confirmed by the fact that metastasis show different mutations than primary tumors (32).
The evolutionary pathway starts with activation of the G-protein alfa signaling cascade, through a series of different harbor mutations that are mutually exclusive. After, there are further somatic mutations affecting BAP1, SF3B1 or EIFA1AX (see below), while cytogenetic rearrangements occur later, as an intermediate point of the evolutionary cascade and before the metastatic dissemination. It has to be noted that sometimes the latter can precede 8q gain (32).
Below we list the main cytogenetic alterations with the corresponding prognostic value.
3.1.1 Monosomy 3
For almost 25 years, monosomy 3 has been known to strongly correlate with metastasis development (33) as well as clinicopathologic features indicating poor prognosis, for instance, ciliary body involvement, large diameter, high mitotic activity, extra-scleral extension, vascular loops, and epithelioid histology (34). On the other hand, metastases rarely develop in tumors with disomy 3 (13, 35).
Whether this abnormality in monosomy 3 tumors is homogeneously present has been a matter of debate (36). Patients with uveal melanoma carrying complete monosomy 3 determined by Fine Needle Aspiration Biopsy (FNAB) have substantially poorer 3-year prognosis than those with partial monosomy 3 or disomy 3. In particular, the cumulative probability for metastasis at 3 years is 2.6% for disomy 3, 5.3% for partial monosomy 3 (equivocal monosomy 3), and 24.0% for complete monosomy 3. Clinical outcomes for patients with partial monosomy 3 or disomy 3 do not significantly differ (37).
When there is a normal copy number of chromosome 3, tumors can show other chromosome alterations, such as 6p gain and 1p loss (11, 13).
3.1.2 Isochromosome 8q
Chromosome 8 is also commonly altered in UM (38). Gain of the long arm of chromosome 8 (8q), which often results from isochromosome formation, occurs in 37% to 63% of primary UM (10, 11, 17, 39–42) and is associated with poor prognosis.
The frequent coexistence of 8q and monosomy 3 gain is associated with higher metastatic rates than a single aberration (18, 43): 5-year mortality rate is reported to be 66% in cases of concomitant monosomy 3 and 8q gain, 40% in cases of monosomy 3 and 31% in cases with 8q gain (34, 44).
In a recent study, Dogrusoz et al. investigated whether information on chromosome 3 and 8q status could enhance the prognostic value of the American Joint Committee on Cancer (AJCC) staging system. In the study cohort of 470 UMs with known chromosome 3 and 8q status, tumors with monosomy 3 and 8q gain showed an increased risk of metastatic death (40).
It has not yet been fully clarified which is the first chromosomal alteration in UM malignant transformation. Some authors have found that monosomy 3 is the first step and that 8q gain occurs subsequently (19), but others highlighted that 8q gain precedes chromosome 3 loss (45). Finally, other authors reported that the gain of the telomeric part of 8q is present in 92% of studied UM, so probably it has a central role in UM tumorigenesis (46).
3.1.3 Loss of the short arm of chromosome 1
Loss of the short arm of chromosome 1 (1p) is frequently associated with monosomy 3 in 19–34% of UM and in 33% of metastasizing tumors (10, 11, 39, 41, 47). The concurrent loss of 1p and monosomy 3 has been reported to be an independent prognostic parameter for disease-free survival (11).
3.1.4 Gain of 6p
Gain of the short arm of chromosome 6 (6p) was the first chromosome aberration to be reported in UM. The prevalence of this mutation is between 18% and 54% in UM and is associated with good prognosis (10, 11, 39, 41, 47).
Monosomy 3 or 6p gain are both very early events in tumorigenesis and they are probably involved in two mutually exclusive evolutionary pathways, as the occurrence of both of them is reported to be only in 4% of UM (18, 19, 48, 49).
3.2 Genome-Wide DNA Copy Number Profiling
3.2.1 Genomic Classification of Tumors
Recently, FNABs from smaller tumors in vivo have been analyzed, using genome and expression profiling on microarrays, enabling precise analysis of combined chromosome imbalances in UM to be carried out (18–20, 50, 51). CGH, single nucleotide polymorphism (SNP) arrays and MLPA technique produce high-density, genome-wide DNA copy number profiles (21).
SNP array analyses have allowed the allelic status of chromosome 3 to be determined, showing that 3-5% of all UM exhibit isodisomy 3 (20, 52), in which one copy of chromosome 3 is lost, whereas the other copy is duplicated, with the same prognostic value as monosomy 3. This abnormality is copy-neutral, and therefore undetectable by CGH array or MLPA (38). Therefore, SNP array appears to be the most sensitive technique for investigating these prognostic correlations, thanks to its ability to detect chromosome 3 isodisomy.
Microsatellite analysis, MLPA, or high-resolution genome-wide techniques have shown the existence of partial deletions of chromosome 3, in either or both arms, but its prognostic implications are still under review (20, 51–55). A minimal region of deletion in 3p26.3 has also been found in liver metastases (20).
Onken et al. classified UMs in 2 groups based on gene expression profile: class 1 tumors (40%), with monosomy 3 and low metastatic risk, and class 2 tumors (60%), with 2 copies of chromosome 3 and high metastatic risk (50). Expression profiling showed that class 1 tumors were mainly characterized by a gain of 6p and 8q distal from band q21, while class 2 tumors revealed a gain of the entire 8q. It is worth noting that a minority of class 1 tumors, probably about 15%, is able to metastasize, as confirmed by class 1 profiles observed in some liver metastases (20).
Sometimes the small sample size obtained with FNABs can lead to wrong genetic categorization related to genetic heterogeneity of the tumor. Bagger et al. highlighted the importance of transvitreal retinochoroidal biopsy (TVRC) in obtaining larger and more representative tissue samples. The authors recommended a combination of Fluorescence in Situ Hybridization (FISH) and MLPA to better identify patients with a higher risk of developing metastasis and founded a genetic heterogeneity of chromosome 3 in a minority of tumors (56).
3.2.2 Candidate Genes
Molecular genetics analyses with DNA sequencing techniques have identified several alterations that seem to play a role in the metastatic UM. The incidence of metastasis in UM is partly determined by random variables, related to mutation rate and type of mutation involved. It has been reported that smaller tumors have a higher mutation rate, while mutation type can affect the timing of onset of metastasis (57).
Van Raamsdonk was the first to describe the GNAQ/GNA11 mutations in UM (58). GNAQ and GNA11 are the driver mutations in 71-93% of UMs (59). They are mutually exclusive in the vast majority of tumors (7, 60–62), but it seems that they are not associated with metastasis and survival in UM (57).
Secondary driver may affect BAP1, SF3B1 and EIF1AX (~45%, ~25%, and ~20% of the primary posterior UM, respectively), which are generally mutually exclusive and have prognostic value (60). In particular, the Rotterdam Ocular Melanoma Study Group highlighted as association among BAP1 mutation and early metastasis with decreased survival rate; SF3B1 mutations with late-onset metastasis; and EIFAX mutations with low metastatic risk and longer disease-free survival (63).
P53 and BRCA pathways may contribute to unfavorable prognosis only in a subset of patients (64).
Additional mutations have been recently described: loss of heterozygosity over the GNAQ locus, loss-of-function mutations affecting CDKN2A, PBRM1, PIK3R2, and PTEN, and gain-of-function mutations affecting EZH2, PIK3CA, and MED12. The majority of them has been found in just one region of the primary tumor or private to their metastases; for this reason, they may be considered as tertiary driver mutations and are supposed to arise later during progression (32).
3.2.2.1 GNAQ and GNA11
GNAQ and GNA11 code for the alpha-subunit of the heterotrimeric GTP-binding protein that couples G-protein-coupled receptor signaling to the RAS-RAF-MEK-ERK (MAPK) pathway. Alterations in this pathway are considered as an early event in cancer development and lead to the activation of multiple cascade pathways involved in cell growth and proliferation (7).
GNAQ mutation can be observed in all stages of malignant progression, suggesting it is an early event in UM, but uncorrelated with disease free survival (65). On the other hand, GNA11 mutations may occur at different stages of UM progression.
Most uveal nevi show either GNAQ or GNA11 mutations, while GNA11-mutated tumors might be more aggressive than GNAQ-mutated forms. This is probably related to the fact that GNAQ, differently from GNA11, requires a second hit to be fully activated (32). Despite these mutations do not seem to have meaningful prognostic value (66–68), recent data highlight the sensitivity of GNAQ and GNA11 mutations to MAP kinase, protein kinase C, AKT and YAP inhibitors. It is therefore not excluded that the analysis of GNAQ/GNA11 mutation could become a routine diagnostic-therapeutic test for UM (69).
3.2.2.2 BAP1
BAP1 is a tumor-suppressor gene located on chromosome 3 (3p21.1), which encodes for a nuclear deubiquitinase involved in cell growth and cancer pathogenesis (17, 70). BAP1 mutation or inexpression are associated with high metastatic risk (71, 72). In up to 84% of metastasizing, class-2 UM, BAP1 inactivation coincides with the onset of metastatic behavior (16, 17). Furthermore, tumors presenting monosomy 3 and BAP1 mutations are characterized by decreased disease-free survival rates (73). No correlation between GNAQ and BAP1 has been reported (70, 74).
A germline mutation of BAP1 can be detected in rare cases. It correlates not only with a higher risk of UM, but also with other tumors. Under these circumstances it may be considered a broader approach, comprising genetic counselling and screening of family members (75, 76).
3.2.2.3 EIF1AX
EIF1AX (located on chromosome 10) encodes for the X-linked Eukaryotic Translation Initiation Factor 1A protein (Eif1A), which regulates the initiation of protein translation. Its mutations lead to mis-selection of start sites, with suppressed translation of canonical transcripts or potential upregulation of oncogenes (77). Nevertheless, the exact biological function of EIF1AX and its contribution to tumorigenesis have not yet been fully clarified (70, 78).
EIF1AX mutations occur in 8% to 19% of all UM [58], in association with disomy-3 (60). Yavuzyigitoglu et al. showed that EIF1AX-mutated tumors have good prognosis and a low risk of metastasis (63, 79–81).
3.2.2.4 SF3B1
SF3B1 (splicing factor 3b subunit 1), located on chromosome 2, encodes for a spliceosome’s component (82). Harbour et al. reported that 18.6% of UM mutations affect the SF3B1 gene (83).
Patients with SF3B1-mutated UM are younger at diagnosis (54.5 years of age) compared to patients with EIF1AX or BAP1 mutations (64 years) (84).
SF3B1-mutated UMs are mainly disomy 3 tumors with an intermediate level of metastatic risk, and metastases seem to occur later than in the presence of BAP1 mutations. Indeed, these patients have an apparent 34% risk of late-onset metastases (mean 11.2 years from diagnosis).
Exceptionally, SF3B1 or EIF1AX mutations occur in combination with monosomy 3, as well as BAP1 mutations in combination with disomy 3. Moreover, despite being defined as mutually exclusive, SF3B1 mutations can coexist with either EIF1AX or BAP1 mutations (85).
3.2.3 Genome-Wide Expression Profiling
GEP classification has greater prognostic accuracy than cytogenetic methods, as it can be carried out on FNAB even when RNA quantity is below detectable limits, with a technical failure rate of only 3% (86). GEP analysis is also very sensitive for capturing overall tumor functional complexity in heterogeneous tumors with its simultaneous evaluation of several genes involved in the tumor microenvironment (87). Onken e al proposed a platform for predicting the risk of UM metastasis based on a 15-gene PCR-based assay (52, 88).
UMs can be classified based on GEP analysis as class 1 (low-risk), which are subsequently subdivided into 1A and 1B with 2% and 21% five-year metastatic risk, respectively, or class 2 (high-risk) tumors with a five-year metastatic risk of 72% (83).
The class 2 gene expression profile is strictly associated with classical factors of bad prognosis, such as larger tumor size, epithelioid cytology, extravascular looping matrix patterns, and monosomy 3 with BAP1 mutations. On the other hand, GEP class 1 tumors are characterized by disomy 3 and EIF1AX or SF3B1 mutations (89–91), but the prognostic accuracy of the class 2 expression profile is higher than many of these factors taken individually or in combination.
Preferentially expressed antigen in melanoma (PRAME) status has been recognized as an independent prognostic biomarker for UM, in fact it identifies increased metastatic risk in patients with Class 1 tumors. For this reason, GEP classification was revised based on PRAME status. It has been reported that when combined with a 12-gene expression panel, PRAME expression predicted a five-year metastatic rate of 0 in class 1/PRAME−, 38% in class 1/PRAME+, and 71% in class 2 tumors, respectively. PRAME expression is also positively correlated with larger tumor diameter and SF3B1 mutations as well as gain of 1q, 6p, 8q, and 9q and loss of 6q and 11q (92).
3.2.4 Molecular and Biochemical Pathways Correlated With Metastasis Development
3.2.4.1 Epithelial-to-Mesenchymal Transition
Epithelial-to-mesenchymal transition (EMT) represents an important event in the late stage of UM. With EMT, in relation to adherent-junction breakdown, cells acquire a spindle-shaped, highly motile fibroblastoid phenotype. Several transcription factors can regulate EMT, contributing to carcinogenesis and metastasis in many tumors with different histotypes, but little is known about UM. Early investigations demonstrated that ZEB1, Twist-related protein 1 (Twist1), and Snail Family Transcriptional Repressor 1 (Snail1) downregulation reduces the invasive properties of uveal melanoma cells, whereas elevated mRNA levels of ZEB1 and Twist1 are associated with a more aggressive clinical phenotype in uveal melanoma samples (93). Other authors have highlighted that EMT in UM cells can be initiated by long-term stimulation with proinflammatory cytokine IL-6. IL-6/STAT3 (Signal transducer and activator of transcription 3) signaling induces the transactivation of v-jun avian sarcoma virus 17 oncogene homolog (JunB), resulting in EMT changes. Therefore, the IL-6/STAT3/JunB pathway has a driving effect on the migration and invasion of UM cells (94).
3.2.4.2 Cancer Stem Cells
Cancer stem cells are cells with self-renewal and multidirectional differentiation potential, leading to tumor invasion and metastasis (95). Recent reports indicate that the presence of cells with stem-cell-like features contributes to drug resistance, partially as a result of the EMT process (96, 97). Only a few studies have investigated the role of cancer stem cells in UM. Universally recognized markers of melanoma stem cells are lacking, while their pathways are far from being fully characterized (98). Though class 1 tumors show similarity to more mature neural crest cells and differentiated melanocytes, class 2 tumors are transcriptionally similar to primitive neural and ectodermal stem cells. Interestingly, class 2 tumors show no similarity to undifferentiated embryonic stem cells, suggesting that the class 2 signature does correspond to the emergence of a lineage-specific primitive transcriptional program, rather than a generalized ‘dedifferentiation’ (99).
3.2.4.3 EMT-Associated Genes
As UM continues to genetically develop from primary tumor to metastatic disease, new genes other than those involved in pathogenesis has been discovered for the metastatic progression. Through GEP several authors have identified several genes involved in the EMT and linked to the presence of monosomy 3.
C-C chemokine ligands 18 (CCL18) gene was found to be the highest up-regulated. CCL18 has a chemotactic activity for naive T-cells, CD4+, and CD8+ T-cells, and plays a key role in creating of tumor-infiltrating lymphocytes (TILs). In contrast to other malignancies, the presence of tumor-infiltrating lymphocytes in UM has been associated with poor prognosis (100), but how specifically promote the disease progression has not been yet been established.
On the contrary, a high expression level of PTP4A3/PRL-3 (gene encoding protein tyrosine phosphatase type IV A member 3/protein of regenerating liver-3) is known to be highly predictive of metastasis by increasing UM cells migration in vitro and invasiveness in vivo (101–105). PTP4A3 acts directly and indirectly through the membrane accumulation of matrix metalloproteinase 14 (MMP14), a membrane-anchored metalloprotease with a central role in the extracellular matrix (ECM) remodeling, invasion and turnover needed for migration or invasiveness, representing a key metastatic event involved in oncogenesis (106, 107). Moreover, PTP4A3 correlated with the expression of several proteases like ADAM10 (A Disintegrin and Metalloproteinase 10), which is upregulated in melanoma metastases and related to many adhesion molecules that have a central role in developing malignant melanoma (108).
Using 2D phosphoprotein analysis, it has been discovered collapsing response mediator protein 2 (CRMP2) as a new target for PTP4A3. The presence of CRMP2 is a good prognostic factor, predictive of low risk for metastasis formation, as it slow down the UM cell migration and invasion process. PTP4A3 inhibit CRMP2 expression (109).
Motility and invasion is also promoted by other genes, like S100A4, a member of S100 family proteins, that have been implicated in tumor metastasis formation (110).
Another up-regulated gene is PRRX1 transcription factor, that facilitates the EMT, conferring migratory and invasive properties (111).
3.2.4.4 Epigenetic Alterations
Epigenetics mechanisms have shown to be involved in UM. They include DNA methylation, chromatin remodeling, histone modification and non-coding RNAs (miRNAs).
3.2.4.4.1 Methylation
Methylation in UM may involve tumor suppressor genes; among the others, RAS association domain family 1 isoform A (RASSF1A) and cyclin-dependent kinase inhibitor 2A (p161INK4a) have been deeply studied (112).
As RASSF1A is usually involved in cell-cycle regulation and apoptosis, a loss of function due to its promoter methylation has been associated with UM pathogenesis and metastatic progression (113).
Methylation of p161INK4 leads to a loss of function of the protein, with subsequent increased tumor cell proliferation and bad prognosis (114).
Hypermetilation of Ras and EF-hand domain containing (RASEF), in combination with homozygosity of RASEF, has been related with increased risk of death due to metastasis (115).
Recently, also BAP1 methylation has been recognized as an important prognostic marker of UM metastasis. Robertson et al. identified four different UM subtypes, based on chromosome 3 status and BAP1 methylation: two groups with bad prognosis and with monosomy 3 and two with better prognosis and with disomy 3. In the first group BAP1 showed a different DNA methylation if compared with that observed in the second group (116).
In recent years, several studies have highlighted that DNA methylation can be used to trace the tissue of origin of various tumors (117, 118). Jurmeister at al. investigated the possibility of using DNA methylation profiling to relate melanomas to their respective primary sites. They found out that only uveal melanomas are characterized by a different global DNA methylation profile, with distinct epigenetic signatures. Thus DNA methylation analysis differentiate uveal melanomas from melanomas of other primary sites (119).
Interestingly, it seems that the methylation patterns of primary tumors and metastases are different. Previous authors compared the methylation status of metastatic primary UM and their corresponding metastases and founded that in the latter case methylation events are likely random events or eventually patient specific (120).
3.2.4.4.2 MicroRNAs
MicroRNAs (miRNAs or miRs) are a class of small (19 to 24 nucleotides) non-coding RNAs that are able to modulate target mRNA expression through sequence-specific interaction. Aberrant expression due to epigenetic modifications in miRNA has been linked to carcinogenic processes, altering many cellular activities, such as differentiation, proliferation, apoptosis and migration (24, 121, 122).
Despite numerous studies, our knowledge about miRNA–mRNA interactions remains poor. This is also because it is not a unique relationship: one miRNA can modulate several mRNA and one mRNA can bind to several miRNA (123, 124)
Recent researchers have found that altered expression levels of miRNA are one of the epigenetic mechanisms responsible for UM tumorigenesis (125). In fact, some miRNAs, which are highly accurate biomarkers of metastatic risk, have demonstrated the ability to affect cellular migration and invasions in UM (23, 126) (Table 1).
Previous authors have highlighted how it is possible to split UM into two prognostic clusters based on miRNA expression. Specifically, 6 upregulated miRNAs are differently expressed as having low metastatic risk class 1 and high metastatic risk class 2 UM (127).
MiRNA can act as oncogenes or as tumor suppressors in UM. Phosphatase and tensin homolog (PTEN) is a tumor suppressor gene that can be regulated by several miRNAs, like MiR-454 and miR-367. An oncogenic role is played by regulating PTEN, which promotes UM cell proliferation, colony formation and invasion (128). MiR-296-3p targets matrix metalloproteinase (MMP) 2 and 9, which are closely related to UM angiogenesis and metastasis. Wang et al. (129) demonstrated that miR-296-3p transfection in UM cells could repress cell proliferation, migration and invasion by regulating MMP-2/MMP-9.
MiR-20a and miR-155 also have an oncogenic role in UM (130), by enhancing cell motility, proliferation and invasion.
MiR-21 is more highly expressed in UM cells than in uveal melanocytes (131). MiR-21 promotes cell migration and invasion by regulating p53 and its downstream targets glutathione S transferase pi (GST-Pi) and LIM and SH3 protein 1 (LASP1).
MiR‐224‐5p is down-expressed in UM cells (132). Its target genes are PIK3R3 (phosphatidylinositol 3 kinase) and AKT3 (protein kinase B). Therefore, miR‐224‐5p acts as a tumor suppressor via the miR‐224‐5p/PIK3R3/PI3K/AKT axis, which takes part in malignantly transforming various carcinomas. MiR-23a is involved in the epithelial-mesenchymal transition (EMT) process, which is important for initiating tumor metastasis. Previous research has shown that this begins with the loss of E-cadherin, a component in the adherent junction of epithelial cells (133). After loss of cell adhesion, epithelial cells can transform into mesenchymal cells and gain the ability to migrate and invade. Wang et al. (134) reported that miR-23a can degrade Zinc Finger E-Box Binding Homeobox 1 (ZEB1), by eliminating its suppression over E-cadherin, thus, in turn, miR-23a can upregulate E-cadherin, then reverse the EMT process in UM cells and finally decrease cellular migration capacity.
In order to deeply understand the effect of miRNA in the evolution of UM, Smit et al. studied the expression of their putative downstream mRNA-targets. They identified 4 target genes negatively correlated with miRNA expression and involved in the regulation of the cell cycle (HDAC4, CDK6, E2F8, and CCND2) (135).
Sharma et al. investigated the potential consequences of germline and somatic mutations in the miRNA binding region of the BAP1 gene. Overall, they found 69 target genes associated with these BAP1-associated miRNAs, which were distinct from other miRNAs associated with UM. Among the others, several chromatin-associated genes were founded to be target of these BAP1-associated miRNAs (136).
Van Essen showed a positive correlation between HLA Class I and the quantity if infiltration in UM by macrophages and lymphocytes. In addition, he found out that mRNA expression is associated with immune-histochemical staining for HLA Class I (137).
Therefore Souri et al. compared HLA expression with miRNA levels in the same tumors, using mRNA expression with four different HLA Class I probes. They identified two clusters of miRNAs, one that positively correlates with HLA Class I and infiltrating leukocytes, the other with an opposite relationship. Both miRNAs expression patterns in UM show a relation are to chromosome 3/BAP1 status. This study could be the starting point to consider the miRNAs as regulators of inflammation in UM, regulated by BAP1 (138).
4 Conclusions
Current learning on UM biology and genetics will enable further development of prognostic tests, as well as patient stratification based on long-term prognosis and metastatic risk. Moreover, advances in UM molecular characterization may support the development of therapeutic strategies by targeting relevant signaling pathways.
Despite its debated role, the genetic analysis could identify patients with high risk for metastasis, allowing a better management and follow-up of patients.
As also clinical, pathological, and radiological features have important role in determining UM prognosis, it would be valuable to use a staging system that incorporates both clinical and genetic data.
5 Dedication
We want to dedicate this review to our Master, Prof. Antonio Rossi (1924 – 2006), Head of University Eye Clinic and Rector Magnificus of Ferrara University, reporting the image of a cluster of metastasizing melanocytic cells within a new-formed tumor vessel, published in 1961 (Rossi A. and Alfieri G., Rass. Ital. Ottal., 30, 81,161, 1961, also published and quoted in Duke-Elder, S. Disease of the Uveal Tract, Vol.IX, in System of 401 Ophthalmology. Henry Kimpton Publishers, London, 1966 (Figure 2).
Author Contributions
Conceptualization: CG, PT, MT, and PP. Validation: MT and PP. Writing—original draft preparation: CG, EF, GA, and SV. Writing—review and editing: CG, EF, and GA. Supervision: PT, MT, and PP. Project administration: PT. All authors have read and agreed to the published version of the manuscript.
Conflict of Interest
The authors declare that the research was conducted in the absence of any commercial or financial relationships that could be construed as a potential conflict of interest.
Publisher’s Note
All claims expressed in this article are solely those of the authors and do not necessarily represent those of their affiliated organizations, or those of the publisher, the editors and the reviewers. Any product that may be evaluated in this article, or claim that may be made by its manufacturer, is not guaranteed or endorsed by the publisher.
Acknowledgments
We would like to thank Prof. Giovanni Lanza, Full Professor of Pathological Anatomy, University of Ferrara for his contribution, for sharing his experience and support.
References
1. Singh AD, Turell ME, Topham AK. Uveal Melanoma: Trends in Incidence, Treatment, and Survival. Ophthalmology (2011) 118:1881–5. doi: 10.1016/j.ophtha.2011.01.040
2. Diener-West M, Reynolds SM, Agugliaro DJ, Caldwell R, Cumming K, Earle JD, et al. Development of Metastatic Disease After Enrollment in the COMS Trials for Treatment of Choroidal Melanoma. Arch Ophthalmol (2005) 123(12):1639–43. doi: 10.1001/archopht.123.12.1639
3. Kujala E, Mäkitie T, Kivelä T. Very Long-Term Prognosis of Patients With Malignant Uveal Melanoma. Investig Ophthalmol Vis Sci (2003) 44:4651–9. doi: 10.1167/iovs.03-0538
4. Rantala ES, Hernberg MM, Piperno-Neumann S, Grossniklaus HE, Kivela T. Metastatic Uveal Melanoma: The Final Frontier. Retin Eye Res (2022) 101041. doi: 10.1016/j.preteyeres
5. Seddon JM, Albert DM, Robinson N, Lavin PT. A Prognostic Factor Study Of Disease-Free Interval and Survival Following Enucleation for Uveal Melanoma. Arch Ophthalmol (1983) 101:1894–9. doi: 10.1001/archopht.1983.01040020896012
6. Kaliki S, Shields CL, Shields JA. Uveal Melanoma: Estimating Prognosis. Indian J Ophthalmol (2015) 63:93–102. doi: 10.4103/0301-4738.154367
7. Staby KM, Gravdal K, Mørk SJ, Heegaard S, Vintermyr OK, Krohn J. Prognostic Impact of Chromosomal Aberrations and GNAQ , GNA11 and BAP1 Mutations in Uveal Melanoma. Acta Ophthalmol (2018) 96(1):31–8. doi: 10.1111/aos.13452
8. Fry MV, Augsburger JJ, Hall J, Corrêa ZM. Posterior Uveal Melanoma in Adolescents and Children: Current Perspectives. Clin Ophthalmol (2018) 12:2205–12. doi: 10.2147/OPTH.S142984
9. Zloto O, Pe’er J, Frenkel S. Gender Differences in Clinical Presentation and Prognosis of Uveal Melanoma. Investig Ophthalmol Vis Sci (2013) 54:652–6. doi: 10.1167/iovs.12-10365
10. Ewens KG, Kanetsky PA, Richards-Yutz J, Al-Dahmash S, de Luca MC, Bianciotto CG, et al. Genomic Profile of 320 Uveal Melanoma Cases: Chromosome 8p-Loss and Metastatic Outcome. Investig Ophthalmol Vis Sci (2013) 54:5721–9. doi: 10.1167/iovs.13-12195
11. Kilic E, Van Gils W, Lodder E, Beverloo HB, Van Til ME, Mooy CM, et al. Clinical and Cytogenetic Analyses in Uveal Melanoma. Investig Ophthalmol Vis Sci (2006) 47:3703–7. doi: 10.1167/iovs.06-0101
12. Harbour JW. Molecular Prognostic Testing and Individualized Patient Care in Uveal Melanoma. Am J Ophthalmol (2009) 148:823–829.e1. doi: 10.1016/j.ajo.2009.07.004
13. Mudhar HS, Parsons MA, Sisley K, Rundle P, Singh A, Rennie IG. A Critical Appraisal of the Prognostic and Predictive Factors for Uveal Malignant Melanoma. Histopathology (2004) 45:1–12. doi: 10.1111/j.1365-2559.2004.01874.x
14. Singh AD, Aronow ME, Sun Y, Bebek G, Saunthararajah Y, Schoenfield LR, et al. Chromosome 3 Status in Uveal Melanoma: A Comparison of Fluorescence In Situ Hybridization and Single-Nucleotide Polymorphism Array. Investig Ophthalmol Vis Sci (2012) 53:3331–9. doi: 10.1167/iovs.11-9027
15. Shah AA, Bourne TD, Murali R. BAP1 Protein Loss by Immunohistochemistry: A Potentially Useful Tool for Prognostic Prediction in Patients With Uveal Melanoma. Pathology (2013) 45:651–6. doi: 10.1097/PAT.0000000000000002
16. Kalirai H, Dodson A, Faqir S, Damato BE, Coupland SE. Lack of BAP1 Protein Expression in Uveal Melanoma Is Associated With Increased Metastatic Risk and has Utility in Routine Prognostic Testing. Br J Cancer (2014) 111:1373–80. doi: 10.1038/bjc.2014.417
17. Koopmans AE, Verdijk RM, Brouwer RWW, Van Den Bosch TPP, Van Den Berg MMP, Vaarwater J, et al. Clinical Significance of Immunohistochemistry for Detection of BAP1 Mutations in Uveal Melanoma. Mod Pathol (2014) 27:1321–30. doi: 10.1038/modpathol.2014.43
18. Hughes S, Damato BE, Giddings I, Hiscott PS, Humphreys J, Houlston RS. Microarray Comparative Genomic Hybridisation Analysis of Intraocular Uveal Melanomas Identifies Distinctive Imbalances Associated With Loss of Chromosome 3. Br J Cancer (2005) 93:1191–6. doi: 10.1038/sj.bjc.6602834
19. Ehlers JP, Worley L, Onken MD, Harbour JW. Integrative Genomic Analysis of Aneuploidy in Uveal Melanoma. Clin Cancer Res (2008) 14:115–22. doi: 10.1158/1078-0432.CCR-07-1825
20. Trolet J, Hupé P, Huon I, Lebigot I, Decraene C, Delattre O, et al. Genomic Profiling and Identification of High-Risk Uveal Melanoma by Array CGH Analysis of Primary Tumors and Liver Metastases. Investig Ophthalmol Vis Sci (2009) 50:2572–80. doi: 10.1167/iovs.08-2296
21. Damato B, Dopierala J, Klaasen A, van Dijk M, Sibbring J, Coupland SE. Multiplex Ligation-Dependent Probe Amplification of Uveal Melanoma: Correlation With Metastatic Death. Investig Ophthalmol Vis Sci (2009) 50:3048–55. doi: 10.1167/iovs.08-3165
22. Worley LA, Onken MD, Person E, Robirds D, Branson J, Char DH, et al. Transcriptomic Versus Chromosomal Prognostic Markers and Clinical Outcome in Uveal Melanoma. Clin Cancer Res (2007) 13:1466–71. doi: 10.1158/1078-0432.CCR-06-2401
23. Worley LA, Long MD, Onken MD, Harbour JW. Micro-RNAs Associated With Metastasis in Uveal Melanoma Identified by Multiplexed Microarray Profiling. Melanoma Res (2008), 184–90. doi: 10.1097/CMR.0b013e3282feeac6
24. Falzone L, Romano GL, Salemi R, Bucolo C, Tomasello B, Lupo G, et al. Prognostic Significance of Deregulated microRNAs in Uveal Melanomas. Mol Med Rep (2019) 19:2599–610. doi: 10.3892/mmr.2019.9949
25. Kivelä T, Kujala E. Prognostication in Eye Cancer: The Latest Tumor, Node, Metastasis Classification and Beyond. Eye (2013) 27:243–52. doi: 10.1038/eye.2012.256
26. Broggi G, Russo A, Reibaldi M, Russo D, Varricchio S, Bonfiglio V, et al. Histopathology and Genetic Biomarkers of Choroidal Melanoma. Appl Sci (2020) 10(22):8081. doi: 10.3390/app10228081
27. Mlecnik B, Bindea G, Pagès F, Galon J. Tumor Immunosurveillance in Human Cancers. Cancer Metastasis Rev (2011) 30(1):5–12. doi: 10.1007/s10555-011-9270-7
28. Jaarsma-Coes MG, Ferreira TA, Luyten GPM, Beenakker JWM. Reaction on "Ocular Ultrasound Versus MRI in the Detection of Extrascleral Extension in a Patient with Choroidal Melanoma". BMC Ophthalmol (2019) 19(1):193. doi: 10.1186/s12886-019-1206-y
29. Jager MJ, Shields CL, Cebulla CM, Abdel-Rahman MH, Grossniklaus HE, Stern MH, et al. Uveal Melanoma. Nat Rev Dis Primers (2020) 6(1):24. doi: 10.1038/s41572-020-0158-0. Erratum in: Nat Rev Dis Primers. 2022 Jan 17;8(1):4.
30. Lemke AJ, Hosten N, Bornfeld N, Bechrakis NE, Schüler A, Richter M, et al. Uveal Melanoma: Correlation of Histopathologic and Radiologic Findings by Using Thin-Section MR Imaging With a Surface Coil. Radiology (1999) 210(3):775–83. doi: 10.1148/radiology.210.3.r99fe39775
31. Foti PV, Travali M, Farina R, Palmucci S, Spatola C, Raffaele L, et al. Diagnostic Methods and Therapeutic Options of Uveal Melanoma With Emphasis on MR Imaging-Part I: MR Imaging With Pathologic Correlation and Technical Considerations. Insights Imaging (2021) 12(1):66. doi: 10.1186/s13244-021-01000-x
32. Shain AH, Bagger MM, Yu R, Chang D, Liu S, Vemula S, et al. The Genetic Evolution of Metastatic Uveal Melanoma. Nat Genet (2019) 51(7):1123–30. doi: 10.1038/s41588-019-0440-9
33. Prescher G, Bornfeld N, Hirche H, Horsthemke B, Jöckel KH, Becher R. Prognostic Implications of Monosomy 3 in Uveal Melanoma. Lancet (1996) 347:1222–5. doi: 10.1016/S0140-6736(96)90736-9
34. Fallico M, Raciti G, Longo A, Reibaldi M, Bonfiglio V, Russo A, et al. Current Molecular and Clinical Insights Into Uveal Melanoma (Review). Int J Oncol (2021) 58:1–22. doi: 10.3892/ijo.2021.5190
35. Kaliki S, Shields CL. Uveal Melanoma: Relatively Rare But Deadly Cancer. Eye (2017) 31:241–57. doi: 10.1038/eye.2016.275
36. Mensink HW, Vaarwater J, Kiliç E, Naus NC, Mooy N, Luyten G, et al. Chromosome 3 Intratumor Heterogeneity in Uveal Melanoma. Investig Ophthalmol Vis Sci (2009) 50:500–4. doi: 10.1167/iovs.08-2279
37. Shields CL, Kaliki S, Cohen MN, Shields PW, Furuta M, Shields JA. Prognosis of Uveal Melanoma Based on Race in 8100 Patients: The 2015 Doyne Lecture. Eye (2015) 29:1027–35. doi: 10.1038/eye.2015.51
38. Dogrusöz M, Jager MJ. Genetic Prognostication in Uveal Melanoma. Acta Ophthalmol (2018) 96:331–47. doi: 10.1111/aos.13580
39. Damato B, Dopierala JA, Coupland SE. Genotypic Profiling of 452 Choroidal Melanomas With Multiplex Ligation-Dependent Probe Amplification. Clin Cancer Res (2010) 16:6083–92. doi: 10.1158/1078-0432.CCR-10-2076
40. Dogrusöz M, Bagger M, Van Duinen SG, Kroes WG, Ruivenkamp CAL, Böhringer S, et al. The Prognostic Value of AJCC Staging in Uveal Melanoma Is Enhanced by Adding Chromosome 3 and 8q Status. Investig Ophthalmol Vis Sci (2017) 58:833–42. doi: 10.1167/iovs.16-20212
41. van den Bosch T, van Beek JGM, Vaarwater J, Verdijk RM, Naus NC, Paridaens D, et al. Higher Percentage of FISH-Determined Monosomy 3 and 8q Amplification in Uveal Melanoma Cells Relate to Poor Patient Prognosis. Investig Ophthalmol Vis Sci (2012) 53:2668–74. doi: 10.1167/iovs.11-8697
42. Versluis M, De Lange MJ, Van Pelt SI, Ruivenkamp CAL, Kroes WGM, Cao J, et al. Digital PCR Validates 8q Dosage as Prognostic Tool in Uveal Melanoma. PloS One (2015) 10:1–14. doi: 10.1371/journal.pone.0116371
43. Cassoux N, Rodrigues MJ, Plancher C, Asselain B, Levy-Gabriel C, Lumbroso-Le Rouic L, et al. Genome-Wide Profiling Is a Clinically Relevant and Affordable Prognostic Test in Posterior Uveal Melanoma. Br J Ophthalmol (2014) 98:769–74. doi: 10.1136/bjophthalmol-2013-303867
44. Scholes AG, Damato BE, Nunn J, Hiscott P, Grierson I, Field JK. Monosomy 3 in Uveal Melanoma: Correlation With Clinical and Histologic Predictors of Survival. Invest Ophthalmol Vis Sci (2003) 44(3):1008–11. doi: 10.1167/iovs.02-0159
45. Lange MJ, van De Pelt SI, Versluis M, Jordanova ES, Van Hall T, Jager MJ, et al. Heterogeneity Revealed by Integrated Genomic Analysis Uncovers a Molecular Switch in Malignant Uveal Melanoma. Oncotarget (2015) 6(35):37824–35. doi: 10.18632/oncotarget.5637
46. Singh N, Singh AD, Hide W. Inferring an Evolutionary Tree of Uveal Melanoma From Genomic Copy Number Aberrations. Invest Ophthalmol Vis Sci (2015) 56(11):6801–9. doi: 10.1167/iovs.15-16822
47. van Beek JGM, Koopmans AE, Vaarwater J, de Rooi JJ, Paridaens D, Naus NC, et al. The Prognostic Value of Extraocular Extension in Relation to Monosomy 3 and Gain of Chromosome 8q in Uveal Melanoma. Investig Ophthalmol Vis Sci (2014) 55:1284–91. doi: 10.1167/iovs.13-13670
48. Young TA, Burgess BL, Rao NP, Gorin MB, Straatsma BR. High-Density Genome Array Is Superior to Fluorescence In-Situ Hybridization Analysis of Monosomy 3 in Choroidal Melanoma Fine Needle Aspiration Biopsy. Mol Vis (2007) 13:2328–33.
49. McCannel TA, Burgess BL, Rao NP, Nelson SF, Straatsma BR. Identification of Candidate Tumor Oncogenes by Integrative Molecular Analysis of Choroidal Melanoma Fine-Needle Aspiration Biopsy Specimens. Arch Ophthalmol (2010) 128:1170–7. doi: 10.1001/archophthalmol.2010.180
50. Onken MD, Worley LA, Ehlers JP, Harbour JW. Gene Expression Profiling in Uveal Melanoma Reveals Two Molecular Classes and Predicts Metastatic Death. Cancer Res (2004) 64:7205–9. doi: 10.1158/0008-5472.CAN-04-1750
51. Parrella P, Sidransky D, Merbs SL. Allelotype of Posterior Uveal Melanoma: Implications for a Bifurcated Tumor Progression Pathway. Cancer Res (1999) 59:3032–7.
52. Onken MD, Worley LA, Person E, Char DH, Bowcock AM, Harbour JW. A Loss of Heterozygosity of Chromosome 3 Detected With Single Nucleotide Polymorphisms Is Superior to Monosomy 3 for Predicting Metastasis in Uveal Melanoma. Clin Cancer Res (2007) 13:2923–7. doi: 10.1158/1078-0432.CCR-06-2383
53. Tschentscher F, Prescher G, Horsman DE, White VA, Rieder H, Anastassiou G, et al. Partial Deletions of the Long and Short Arm of Chromosome 3 Point to Two Tumor Suppressor Genes in Uveal Melanoma. Cancer Res (2001) 61:3439–42.
54. Parrella P, Fazio VM, Gallo AP, Sidransky D, Merbs SL. Fine Mapping of Chromosome 3 in Uveal Melanoma: Identification of a Minimal Region of Deletion on Chromosomal Arm 3p25.1-P25.2. Cancer Res (2003) 63:8507–10.
55. Cross NA, Ganesh A, Parpia M, Murray AK, Rennie IG, Sisley K. Multiple Locations on Chromosome 3 Are the Targets of Specific Deletions in Uveal Melanoma. Eye (2006) 20:476–81. doi: 10.1038/sj.eye.6701906
56. Bagger M, Andersen MT, Heegaard S, Andersen MK, Kiilgaard JF. Transvitreal Retinochoroidal Biopsy Provides a Representative Sample From Choroidal Melanoma for Detection of Chromosome 3 Aberrations. Invest Ophthalmol Vis Sci (2015) 56(10):5917–24. doi: 10.1167/iovs.15-17349.
57. Szalai E, Jiang Y, van Poppelen NM, Jager MJ, de Klein A, Kilic E, et al. Association of Uveal Melanoma Metastatic Rate With Stochastic Mutation Rate and Type of Mutation. JAMA Ophthalmol (2018) 136:1115–20. doi: 10.1001/jamaophthalmol.2018.2986
58. Van Raamsdonk CD, Griewank KG, Crosby MB, Garrido MC, Vemula S, Wiesner T, et al. Mutations in GNA11 in Uveal Melanoma. N Engl J Med (2010) 363:2191–9. doi: 10.1056/NEJMoa1000584
59. van Poppelen NM, de Bruyn DP, Bicer T, Verdijk R, Naus N, Mensink H, et al. Genetics of Ocular Melanoma: Insights into Genetics, Inheritance and Testing. Int J Mol Sci (2020) 22(1):336. doi: 10.3390/ijms22010336.
60. Smit KN, Jager MJ, de Klein A, Kiliҫ E. Uveal Melanoma: Towards a Molecular Understanding. Prog Retin Eye Res (2020) 75:100800. doi: 10.1016/j.preteyeres.2019.100800
61. Chua V, Mattei J, Han A, Johnston L, LiPira K, Selig SM, et al. The Latest on Uveal Melanoma Research and Clinical Trials: Updates From the Cure Ocular Melanoma (CURE OM) Science Meeting, (2019). Clin Cancer Res (2021) 27:28–33. doi: 10.1158/1078-0432.CCR-20-2536
62. Chua V, Lapadula D, Randolph C, Benovic JL, Wedegaertner PB, Aplin AE. Dysregulated GPCR Signaling and Therapeutic Options in Uveal Melanoma. (2017) 15(5):501–6. doi: 10.1158/1541-7786.MCR-17-0007
63. Yavuzyigitoglu S, Koopmans AE, Verdijk RM, Vaarwater J, Eussen B, Van Bodegom A, et al. Uveal Melanomas With SF3B1 Mutations: A Distinct Subclass Associated With Late-Onset Metastases. Ophthalmology (2016) 123:1118–28. doi: 10.1016/j.ophtha.2016.01.023
64. Sarubi HC, Pereira NB, Gomes CC, Gomez RS, Carmo ACM, Melo FM, et al. Molecular and Immunohistochemical Analyses of Uveal Melanoma Patient Cohort. Melanoma Res (2019) 29(3):248–. doi: 10.1097/CMR.0000000000000523
65. Bauer J, Kilic E, Vaarwater J, Bastian BC, Garbe C, De Klein A. Oncogenic GNAQ Mutations Are Not Correlated With Disease-Free Survival in Uveal Melanoma. Br J Cancer (2009) 101:813–5. doi: 10.1038/sj.bjc.6605226
66. Vader MJC, Madigan MC, Versluis M, Suleiman HM, Gezgin G, Gruis NA. GNAQ and GNA11 Mutations and Downstream YAP Activation in Choroidal Nevi. Nat Publ Gr (2017) 117:884–7. doi: 10.1038/bjc.2017.259
67. Koopmans AE, Vaarwater J, Paridaens D, Naus NC, Kilic E, De Klein A. Patient Survival in Uveal Melanoma Is Not Affected by Oncogenic Mutations in GNAQ. Br J Cancer (2013), 493–6. doi: 10.1038/bjc.2013.299
68. Griewank KG, Van De Nes J, Schilling B, Moll I, Sucker A, Kakavand H, et al. Genetic and Clinico-Pathologic Analysis of Metastatic Uveal Melanoma. Mod Pathol (2014) 27(2):175–83. doi: 10.1038/modpathol.2013.138
69. Schneider B, Riedel K, Zhivov A, Huehns M, Zettl H, Guthoff RF, et al. Frequent and Yet Unreported GNAQ and GNA11 Mutations Are Found in Uveal Melanomas. Pathol Oncol Res (2019), 1319–25. doi: 10.1007/s12253-017-0371-7
70. Van De Nes JAP, Nelles J, Kreis S, Metz CHD, Hager T, Lohmann DR, et al. Comparing the Prognostic Value of BAP1 Mutation Immunohistochemistry in Uveal Melanoma. Am J Surg Pathol (2016) 40(6):796–805. doi: 10.1097/PAS.0000000000000645
71. Harbour JW, Onken MD, Roberson EDO, Duan S, Cao L, Worley LA, et al. Frequent Mutation of BAP1 in Metastasizing Uveal Melanomas. Sci (80- ) (2010) 330(6009):1410–3. doi: 10.1126/science.1194472
72. Masclef L, Ahmed O, Estavoyer B, Larrivée B, Labrecque N, Nijnik A, et al. Roles and Mechanisms of BAP1 Deubiquitinase in Tumor Suppression. Cell Death Differ (2021) 28(2):606–. doi: 10.1038/s41418-020-00709-4
73. Karlsson J, Nilsson LM, Mitra S, Alsén S, Shelke GV, Sah VR, et al. Molecular Profiling of Driver Events in Metastatic Uveal Melanoma. Nat Commun (2020) 11:1–13. doi: 10.1038/s41467-020-15606-0
74. Patrone S, Maric I, Rutigliani M, Lanza F, Puntoni M, Banelli B, et al. Prognostic Value of Chromosomal Imbalances , Gene Mutations , and BAP1 Expression in Uveal Melanoma. Genes Chromosomes Cancer (2018) 57(8):387–400. doi: 10.1002/gcc.22541
75. Riechardt AI, Kilic E JA. The Genetics of Uveal Melanoma: Overview and Clinical Relevance. Klin Monbl Augenheilkd (2021) 238(7):773–80. doi: 10.1055/a-1513-0789
76. Louie BH, Kurzrock R. BAP1: Not Just a BRCA1-Associated Protein. Cancer Treat Rev (2020) 90:102091. doi: 10.1016/j.ctrv.2020.102091
77. Li Y, Shi J, Yang J, Ge S, Zhang J, Jia R, et al. Uveal Melanoma: Progress in Molecular Biology and Therapeutics. Ther Adv Med Oncol (2020) 12:1758835920965852. doi: 10.1177/1758835920965852
78. Marcel M, Maßhöfer L, Temming P, Rahmann S, Metz C, Bornfeld N, et al. Exome Sequencing Identifies Recurrent Somatic Mutations in EIF1AX and SF3B1 in Uveal Melanoma With Disomy 3. Nat Genet (2013) 45(8):933–6. doi: 10.1038/ng.2674
79. Davies H, Bignell GR, Cox C, Stephens P, Edkins S, Clegg S, et al. Mutations of the BRAF Gene in Human Cancer. Nature (2002) 417:949–54. doi: 10.1038/nature00766
80. Violanti SS, Bononi I, Gallenga CE, Martini F, Tognon M, Perri P. New Insights Into Molecular Oncogenesis and Therapy of Uveal Melanoma. Cancers (Basel) (2019) 11:1–25. doi: 10.3390/cancers11050694
81. Yue H, Qian J, Yuan Y, Zhang R, Bi Y, Meng F, et al. Clinicopathological Characteristics and Prognosis for Survival After Enucleation of Uveal Melanoma in Chinese Patients: Long-Term Follow-Up. Curr Eye Res (2017) 42:759–65. doi: 10.1080/02713683.2016.1245422
82. Seiler M, Peng S, Agrawal AA, Palacino J, Teng T, Zhu P, et al. Somatic Mutational Landscape of Splicing Factor Genes and Their Functional Consequences Across Resource Somatic Mutational Landscape of Splicing Factor Genes and Their Functional Consequences Across 33 Cancer Types. Cell Rep (2018) 23(1):282–96. doi: 10.1016/j.celrep.2018.01.088
83. Field MG, Harbour JW. HHS Public Access (2015). Available at: 10.1097/ICU.0000000000000051.Recent.
84. Yavuzyigitoglu S, Koopmans AE, Verdijk RM, Vaarwater J, Eussen B, van Bodegom A, et al. Rotterdam Ocular Melanoma Study Group. Uveal Melanomas with SF3B1 Mutations: A Distinct Subclass Associated with Late-Onset Metastases. Ophthalmology (2016) 123(5):1118–28. doi: 10.1016/j.ophtha.2016.01.023.
85. Thornton S, Coupland SE, Olohan L, Sibbring JS, Kenny JG, Hertz-fowler C, et al. Targeted Next-Generation Sequencing of 117 Routine Clinical Samples Provides Further Insights Into the Molecular Landscape of Uveal Melanoma. Cancers (Basel) (2020) 12(4):1039. doi: 10.3390/cancers12041039.
86. Mallone F, Sacchetti M, Lambiase A, Moramarco A. Molecular Insights and Emerging Strategies for Treatment of Metastatic Uveal Melanoma. Cancers (Basel) (2020) 12(10):2761. doi: 10.3390/cancers12102761
87. Onken MD, Worley LA, Char DH, Augsburger JJ. Report Number 1 : Prospective Validation of a Multi-Gene Prognostic Assay in Uveal. OPHTHA (2012) 119:1596–603. doi: 10.1016/j.ophtha.2012.02.017
88. Onken MD, Worley LA, Tuscan MD, Harbour JW. An Accurate , Clinically Feasible Multi-Gene Expression Assay for Predicting Metastasis in Uveal Melanoma. J Mol Diagnostics (2010) 12:461–8. doi: 10.2353/jmoldx.2010.090220
89. Xu W, Harrisont SC, Eckt MJ. Three-Dimensional Structure of the Tyrosine Kinase C-Src. Nature (1997) 385(6617):595–602. doi: 10.1038/385595a0
90. Steingrímsson E, Copeland NG, Jenkins NA. Melanocytes and the Microphthalmia Transcription Factor Network. Annu Rev Genet (2004) 38:365–411. doi: 10.1146/annurev.genet.38.072902.092717.
91. Bol KF, Donia M, Heegaard S, Kiilgaard JF, Svane IM. Genetic Biomarkers in Melanoma of the Ocular Region: What the Medical Oncologist Should Know. Int J Mol Sci (2020) 21:1–14. doi: 10.3390/ijms21155231
92. Field MG, Decatur CL, Kurtenbach S, Van Der Velden PA, Jager MJ, Kozak KN, et al. PRAME as an Independent Biomarker for Metastasis in Uveal Melanoma. Clin Cancer Res (2016) 22(5):1234–43. doi: 10.1158/1078-0432.CCR-15-2071
93. Asnaghi L, Gezgin G, Tripathy A, Handa JT, Merbs SL, van der Velden PA, et al. EMT-Associated Factors Promote Invasive Properties of Uveal Melanoma Cells. Mol Vis (2015) 21:919–29.
94. Gong C, Shen J, Fang Z, Qiao L, Feng R, Lin X, et al. Abnormally Expressed Junb Transactivated by Il-6/Stat3 Signaling Promotes Uveal Melanoma Aggressiveness via Epithelial–Mesenchymal Transition. Biosci Rep (2018) 38:1–14. doi: 10.1042/BSR20180532
95. Li F, Li F, Tiede B, Massagué J, Kang Y. Beyond Tumorigenesis : Cancer Stem Cells in Metastasis. Cell Res (2007) 17(1):3–14. doi: 10.1038/sj.cr.7310118
96. Singh A, Settleman J. EMT , Cancer Stem Cells and Drug Resistance : An Emerging Axis of Evil in the War on Cancer. Oncogene (2010), 4741–51. doi: 10.1038/onc.2010.215
97. Thiery JP, Acloque H, Huang RYJ, Nieto MA. Review Epithelial-Mesenchymal Transitions in Development and Disease. Cell (2009) 139(5):871–90. doi: 10.1016/j.cell.2009.11.007
98. Chen YN, Li Y, Bin Wei W. Research Progress of Cancer Stem Cells in Uveal Melanoma. Onco Targets Ther (2020) 13:12243–52. doi: 10.2147/OTT.S284262
99. Chang S, Worley LA, Onken MD, Harbour JW. Prognostic Biomarkers in Uveal Melanoma : Evidence for a Stem Cell-Like Phenotype Associated With Metastasis. Melanoma Res (2008) 18(3):191–200. doi: 10.1097/CMR.0b013e3283005270.
100. Triozzi PL, Schoen L, Plesec T, Saunthararajah Y, Tubbs RR, Singh AD. Molecular Profiling of Primary Uveal Melanomas With Tumor Infiltrating Lymphocytes. Oncoimmunology (2014) 8(10):e947169. doi: 10.4161/21624011.2014.947169
101. Wu X, Zeng H, Zhang X. Phosphatase of Regenerating Liver-3 Promotes Motility and Metastasis of Mouse Melanoma Cells. Am J Pathol (2004) 164(6):2039–54. doi: 10.1016/S0002-9440(10)63763-7
102. Wang H, Quah SY, Dong JM, Manser E. PRL-3 Down-Regulates PTEN Expression and Signals Through PI3K to Promote Epithelial-Mesenchymal Transition. Cancer Res (2007) 67(7):2922–6. doi: 10.1158/0008-5472.CAN-06-3598
103. Liang F, Liang J, Wang W, Sun J, Udho E, Zhang Z. PRL3 Promotes Cell Invasion and Proliferation by Down-Regulation of Csk Leading to Src Activation *. J Biol Chem (2007) 282:5413–9. doi: 10.1074/jbc.M608940200
104. Saha S, Bardelli A, Buckhaults P, Velculescu VE, Rago C, Croix BS, et al. A Phosphatase Associated With Metastasis of Colorectal Cancer A Phosphatase Associated With Metastasis of Colorectal Cancer. Science (2001) 294(5545):1343–6. doi: 10.1126/science.1065817
105. Anderiani U, Shuho M, Hirotaka S, Hiroshi K. Expression of PRL-3 Phosphatase in Human Gastric Carcinomas : Close Correlation With Invasion and Metastasis. Pathobiology (2004) 71(4):176–84. doi: 10.1159/000078671
106. Foy M, Anézo O, Saule S, Planque N. PRL-3/PTP4A3 Phosphatase Regulates Integrin β1 in Adhesion Structures During Migration of Human Ocular Melanoma Cells. Exp Cell Res (2017) 353:88–99. doi: 10.1016/j.yexcr.2017.03.012
107. Sastre-garau X. High PTP4A3 Phosphatase Expression Correlates With Metastatic Risk in Uveal Melanoma Patients. Cancer Res (2011) 71(3):666–74. doi: 10.1158/0008-5472.CAN-10-0605
108. Lee SB, Schramme A, Doberstein K, Dummer R, Abdel-bakky MS, Keller S, et al. ADAM10 Is Upregulated in Melanoma Metastasis Compared With Primary Melanoma. J Invest Dermatol (2010) 130(3):763–73. doi: 10.1038/jid.2009.335
109. Duciel L, Anezo O, Mandal K, Laurent C, Planque N, Coquelle FM, et al. Protein Tyrosine Phosphatase 4A3 (PTP4A3/PRL-3) Promotes the Aggressiveness of Human Uveal Melanoma Through Dephosphorylation of CRMP2. Sci Rep (2019) 9:1–13. doi: 10.1038/s41598-019-39643-y
110. Onken MD, Ehlers JP, Worley LA, Makita J, Yokota Y, Harbour JW. Functional Gene Expression Analysis Uncovers Phenotypic Switch in Aggressive Uveal Melanomas. Cancer Res (2006) 66(9):4602–10. doi: 10.1158/0008-5472.CAN-05-4196
111. Fazilaty H, Rago L, Kass Youssef K, Ocaña OH, Garcia-Asencio F, Arcas A, et al. A Gene Regulatory Network to Control EMT Programs in Development and Disease. Nat Commun (2019) 10:5115. doi: 10.1038/s41467-019-13091-8
112. Li Y, Jia R, Ge S. Role of Epigenetics in Uveal Melanoma. Int J Biol Sci (2017) 13(4):426–33. doi: 10.7150/ijbs.18331
113. Maat W, van der Velden PA, Out-Luiting C, Plug M, Dirks-Mulder A, Jager MJ, et al. Epigenetic Inactivation of RASSF1a in Uveal Melanoma. Invest Ophthalmol Vis Sci (2007) 48:486–90. doi: 10.1167/iovs.06-0781
114. van der Velden PA, Metzelaar-Blok JA, Bergman W, Monique H, Hurks H, Frants RR, et al. Promoter Hypermethylation: A Common Cause of Reduced P16(INK4a) Expression in Uveal Melanoma. Cancer Res (2001) 61(13):5303–6.
115. Maat W, Beiboer SH, Jager MJ, Luyten GP, Gruis NA, van der Velden PA, et al. Epigenetic Regulation Identifies RASEF as a Tumor-Suppressor Gene in Uveal Melanoma. Invest Ophthalmol Vis Sci (2008) 49:1291–8. doi: 10.1167/iovs.07-1135
116. Robertson AG, Shih J, Yau C, Gibb EA, Oba J, Mungall KL, et al. Integrative Analysis Identifies Four Molecular and Clinical Subsets in Uveal Melanoma. Cancer Cell (2017) 32(2):204. doi: 10.1016/j.ccell.2017.07.003
117. Capper D, Stichel D, Sahm F, Jones D, Schrimpf D, Sill M, et al. Practical Implementation of DNA Methylation and Copy-Number-Based CNS Tumor Diagnostics: The Heidelberg Experience. Acta Neuropathol (2018) 136(2):181–210. doi: 10.1007/s00401-018-1879-y
118. Roh MR, Gupta S, Park KH, Chung KY, Lauss M, Flaherty KT, et al. Promoter Methylation of PTEN Is a Significant Prognostic Factor in Melanoma Survival. J Invest Dermatol (2016) 136(5):1002–11. doi: 10.1016/j.jid.2016.01.024
119. Jurmeister P, Wrede N, Hoffmann I, Vollbrecht C, Heim D, Hummel M, et al. Mucosal Melanomas of Different Anatomic Sites Share a Common Global DNA Methylation Profile With Cutaneous Melanoma But Show Location-Dependent Patterns of Genetic and Epigenetic Alterations. J Pathol (2022) 256(1):61–70. doi: 10.1002/path.5808
120. Smit KN, Boers R, Vaarwater J, Boers J, Brands T, Mensink H, et al. Genome-Wide Aberrant Methylation in Primary Metastatic UM and Their Matched Metastases. Sci Rep (2022) 12(1):42. doi: 10.1038/s41598-021-03964-8
121. MacFarlane L-A, Murphy PR. MicroRNA: Biogenesis, Function and Role in Cancer. Curr Genomics (2010) 11:537–61. doi: 10.2174/138920210793175895
122. Tan W, Liu B, Qu S, Liang G, Luo W, Gong C. MicroRNAs and Cancer: Key Paradigms in Molecular Therapy (Review). Oncol Lett (2018) 15:2735–42. doi: 10.3892/ol.2017.7638
123. Selbach M, Schwanhäusser B, Thierfelder N, Fang Z, Khanin R, Rajewsky N. Widespread Changes in Protein Synthesis Induced by microRNAs. Nature (2008) 455(7209):58–63. doi: 10.1038/nature07228
124. Uhlmann S, Mannsperger H, Zhang JD, Horvat EÁ., Schmidt C, Küblbeck M, et al. Global microRNA Level Regulation of EGFR-Driven Cell-Cycle Protein Network in Breast Cancer. Mol Syst Biol (2012) 8:570. doi: 10.1038/msb.2011.100
125. Yang C, Wang Y HP. Emerging Roles of microRNAs and Their Implications in Uveal Melanoma. Cell Mol Life Sci (2021) 78(2):545–59. doi: 10.1007/s00018-020-03612
126. Li YF, Dong L, Li Y, Bin Wei W. A Review of Microrna in Uveal Melanoma. Onco Targets Ther (2020) 13:6351–9. doi: 10.2147/OTT.S253946
127. Höglund M, Gisselsson D, Hansen GB, White VA, Säll T, Mitelman F, et al. Dissecting Karyotypic Patterns in Malignant Melanomas: Temporal Clustering of Losses and Gains in Melanoma Karyotypic Evolution. Int J Cancer (2004) 108:57–65. doi: 10.1002/ijc.11558
128. Sun L, Wang Q, Gao X, Shi D, Mi S, Han Q. MicroRNA-454 Functions as an Oncogene by Regulating PTEN in Uveal Melanoma. FEBS Lett (2015) 589:2791–6. doi: 10.1016/j.febslet.2015.08.007
129. Wang X, Hu Y, Cui J, Zhou Y, Chen L. Coordinated Targeting of MMP-2/MMP-9 by miR-296-3p/FOXCUT Exerts Tumor-Suppressing Effects in Choroidal Malignant Melanoma. Mol Cell Biochem (2018) 445:25–33. doi: 10.1007/s11010-017-3248-x
130. Zhou J, Jiang J, Wang S, Xia X. Oncogenic Role of microRNA-20a in Human Uveal Melanoma. Mol Med Rep (2016) 14:1560–6. doi: 10.3892/mmr.2016.5433
131. Wang YC, Yang X, Wei W, Xu XL. Role of microRNA-21 in Uveal Melanoma Cell Invasion and Metastasis by Regulating P53 and its Downstream Protein. Int J Ophthalmol (2018) 11:1258–68. doi: 10.18240/ijo.2018.08.03
132. Li J, Liu X, Li C, Wang W. miR-224-5p Inhibits Proliferation, Migration, and Invasion by Targeting PIK3R3/AKT3 in Uveal Melanoma. J Cell Biochem (2019) 120:12412–21. doi: 10.1002/jcb.28507
133. Loh CY, Chai JY, Tang TF, Wong WF, Sethi G, Shanmugam MK, et al. The E-Cadherin and N-Cadherin Switch in Epithelial-To-Mesenchymal Transition: Signaling, Therapeutic Implications, and Challenges, Cells. Cells (2019) 8(10):1118. doi: 10.3390/cells8101118
134. Wang Y, Luo Y, Guan W, Zhao H. Role of Mir-23a/Zeb1 Negative Feedback Loop in Regulating Epithelial-Mesenchymal Transition and Tumorigenicity of Intraocular Tumors. Oncol Lett (2018) 16:2462–70. doi: 10.3892/ol.2018.8940
135. Smit KN, Chang J, Derks K, Vaarwater J, Brands T, Verdijk RM, et al. Aberrant MicroRNA Expression and Its Implications for Uveal Melanoma Metastasis. Cancers (Basel) (2019) 11(6):815. doi: 10.3390/cancers11060815
136. Sharma A, Biswas A, Liu H, Sen S, Paruchuri A, Katsonis P, et al. Mutational Landscape of the BAP1 Locus Reveals an Intrinsic Control to Regulate the miRNA Network and the Binding of Protein Complexes in Uveal Melanoma. Cancers (Basel) (2019) 11(10):1600. doi: 10.3390/cancers11101600
137. van Essen TH, van Pelt SI, Bronkhorst IH, Versluis M, Némati F, Laurent C, et al. Upregulation of HLA Expression in Primary Uveal Melanoma by Infiltrating Leukocytes. PloS One (2016) 11(10):e0164292. doi: 10.1371/journal.pone.0164292
Keywords: uveal melanoma (UM), metastasis, molecular mechanism, prognostic markers, genetic analyses
Citation: Gallenga CE, Franco E, Adamo GG, Violanti SS, Tassinari P, Tognon M and Perri P (2022) Genetic Basis and Molecular Mechanisms of Uveal Melanoma Metastasis: A Focus on Prognosis. Front. Oncol. 12:828112. doi: 10.3389/fonc.2022.828112
Received: 03 December 2021; Accepted: 25 February 2022;
Published: 11 April 2022.
Edited by:
Erik Wiemer, Erasmus University Medical Center, NetherlandsReviewed by:
Emine Kilic, Erasmus University Medical Center, NetherlandsCarla Loreto, University of Catania, Italy
Copyright © 2022 Gallenga, Franco, Adamo, Violanti, Tassinari, Tognon and Perri. This is an open-access article distributed under the terms of the Creative Commons Attribution License (CC BY). The use, distribution or reproduction in other forums is permitted, provided the original author(s) and the copyright owner(s) are credited and that the original publication in this journal is cited, in accordance with accepted academic practice. No use, distribution or reproduction is permitted which does not comply with these terms.
*Correspondence: Carla Enrica Gallenga, Z2xsY2xuQHVuaWZlLml0
†These authors have contributed equally to this work