- 1Institute for Translational Medicine, The Affiliated Hospital of Qingdao University, Qingdao Medical College, Qingdao University, Qingdao, China
- 2School of Basic Medical Sciences, Qingdao Medical College, Qingdao University, Qingdao, China
Gastric cancer (GC) is the third leading cause of cancer-related deaths worldwide, with high recurrence and mortality rate. Chemotherapy, including 5-fluorouracil (5-FU), adriamycin (ADR), vincristine (VCR), paclitaxel (PTX), and platinum drugs, remains one of the fundamental methods of GC treatment and has efficiently improved patients’ prognosis. However, most patients eventually develop resistance to chemotherapeutic agents, leading to the failure of clinical treatment and patients’ death. Recent studies suggest that long non-coding RNAs (lncRNAs) are involved in the drug resistance of GC by modulating the expression of drug resistance-related genes via sponging microRNAs (miRNAs). Moreover, lncRNAs also play crucial roles in GC drug resistance via a variety of mechanisms, such as the regulation of the oncogenic signaling pathways, inhibition of apoptosis, induction of autophagy, modulation of cancer stem cells (CSCs), and promotion of the epithelial-to-mesenchymal transition (EMT) process. Some of lncRNAs exhibit great potential as diagnostic and prognostic biomarkers, as well as therapeutic targets for GC patients. Therefore, understanding the role of lncRNAs and their mechanisms in GC drug resistance may provide us with novel insights for developing strategies for individual diagnosis and therapy. In this review, we summarize the recent findings on the mechanisms underlying GC drug resistance regulated by lncRNAs. We also discuss the potential clinical applications of lncRNAs as biomarkers and therapeutic targets in GC.
Introduction
Gastric cancer (GC) is one of the most serious malignant gastrointestinal neoplasms worldwide, representing the third leading cause of cancer-related mortality after lung cancer and liver cancer (1). According to the latest statistics from GLOBOCAN database, about more than one million new GC cases and approximately 769,000 deaths occurred in 2020 (2). Although the overall incidence of GC has shown a downward trend, it is still a terminal disease threatening human health and considerably affecting the quality of human lives. Currently, chemotherapy is still the best option for metastatic GC patients followed by surgical resection, and it can effectively prolong their five-year overall survival (OS) rate and quality of life. The commonly used chemotherapeutic drugs for GC treatment mainly include 5-fluorouracil (5-FU), adriamycin (ADR), vincristine (VCR), paclitaxel (PTX), and platinum drugs (3). At the beginning of chemotherapy, the efficiency of these drugs is remarkable and the prognosis is good. However, poor or even no response to chemotherapy is often observed in GC patients due to the development of drug resistance, which ultimately leads to the failure of clinical treatment and patients’ death (4). The underlying mechanisms of GC drug resistance are very complicated and still not fully understood. Therefore, in-depth research is of great importance for GC patients in the development of individual diagnosis and therapeutic strategy (5, 6).
Long non-coding RNAs (lncRNAs) are a large class of endogenous ncRNAs with a length of more than 200 nucleotides (7). In recent years, with the rapid development of bioinformatics analysis and the application of high-throughput sequencing technologies, a large number of lncRNAs has been found to occur widely and stably in eukaryotes, including those of plant, animal, and human being (8, 9). They are involved in various biological processes, such as proliferation, apoptosis, invasion, and metastasis, by regulating the timing and degree of gene expression via multiple mechanisms, including signal, decoy, scaffold, guide, and SINEUPs (9). In addition, lncRNAs also play crucial roles in the regulation of multiple pathological and physiological processes, such as embryonic development, gene expression regulation, and carcinogenesis, by acting as a competing endogenous RNA (ceRNA) to modulate the expression of specific microRNAs (miRNAs), thereby targeting the downstream genes of these miRNAs (10–12). Therefore, the dysregulation of lncRNAs is closely associated with a lot of diseases, including GC (13–16). In fact, the aberrant expression of lncRNAs has been observed in GC samples and cell lines (17, 18). A growing amount of evidence suggests that lncRNAs are involved in practically all aspects of GC progression, including drug resistance (19–21). Exosomes are a novel extracellular vesicles that play crucial roles in cancer progression by transferring multiple biologically active molecules, such as proteins, lipids, and lncRNAs. It has been shown that exosomes can mediate intracellular communication to promote drug resistance in cancer (22, 23). A large number of lncRNAs are found to be selectively enriched in exosomes and some of them also exhibit great clinical value as biomarkers and/or therapeutic targets for drug-resistant GC patients (6, 24).
In this review, we systematically summarize the characteristics of lncRNAs associated with drug resistance in GC, specifically exploring the underlying mechanisms by which lncRNAs produce the drug resistance. Additionally, we highlight their clinical applications as biomarkers and therapeutic targets for GC patients.
Long Non-Coding RNAs and Chemotherapeutic Drugs in Gastric Cancer
Currently, the first-line chemotherapeutic drugs commonly used in clinic for GC patients include 5-FU, platinum drugs, ADR, PTX, and VCR, all of which have effectively improved the poor outcome of patients. However, the development of resistance to these drugs is still the principal limiting factor in achieving cures in GC patients. Drug resistance can be divided into single drug resistance and multidrug resistance (MDR) (25–27). In the following section, we will describe the lncRNAs involved in GC drug resistance (Table 1).
Long Non-Coding RNAs and 5-Fluorouracil
5-FU is an antimetabolite drug that has been widely applied for the treatment of different types of cancer in clinic, including GC (90). 5-FU is converted intracellularly to several active metabolites, including fluorodeoxyuridine monophosphate, fluorodeoxyuridine triphosphate, and fluorouridine triphosphate (91). These active metabolites can interfere in DNA replication by inhibiting thymidylate synthase (TS) activity and can also induce cytotoxicity by misincorporating its metabolites into RNA and DNA (92). However, its clinical application is still limited due to the resistance development of GC patients to this drug. Multiple lncRNAs have been found to be involved in GC resistance to 5-FU. Several oncogenic lncRNAs, such as metastasis associated in colon cancer 1-antisense 1 (MACC1-AS1), Plasmacytoma variant translocation 1 (PVT1), and HOXD antisense growth-associated long noncoding RNA (HAGLR), can promote the 5-FU resistance of GC cells. For instance, the upregulation of MACC1-AS1, induced by transforming growth factor β1 (TGF-β1), was shown to facilitate the fatty acid oxidation (FAO)-dependent resistance of GC cells to 5-FU by sponging miR-145-5p (93). In another study, Hu et al. revealed that HAGLR was upregulated in both GC tissues and cell lines. The overexpression of HAGLR significantly promoted the 5-FU resistance of GC cells by increasing the lactate dehydrogenase-A (LDHA) expression via sponging miR-338-3p. Consistent with this, the silencing of HAGLR sensitized GC cells to 5-FU (29). In addition, Du et al. showed that PVT1 promoted the drug resistance of GC cells to 5-FU through the activation of B-cell lymphoma-2 (Bcl-2) (28).
In contrast, several tumor suppressor lncRNAs can reverse 5-FU resistance in GC. For instance, Zhang et al. found that lncRNA colorectal neoplasia differentially expressed (CRNDE) was downregulated in 5-FU-resistant GC cells. The overexpression of CRNDE significantly enhanced the chemosensitivity of GC cells to 5-FU by increasing the expression of the long isoform of PICALM (encoding PICALML) by directly targeting serine/arginine-rich splicing factor 6 (SRSF6) (19). Furthermore, Han et al. revealed that lncRNA lower expression in gastric cancer (LEIGC) expressed at low levels in GC tissues. The overexpression of LEIGC significantly promoted the sensitivity of GC cells to 5-FU by inhibiting the epithelial-to-mesenchymal transition (EMT) process, whereas the knockdown of LEIGC showed the opposite effect (38).
Long Non-Coding RNAs and Platinum Drugs
Platinum drugs, such as cisplatin (CDDP), oxaliplatin (OXA), and carboplatin, are widely used in the treatment of human cancers, including GC (94). These drugs represent a class of cell cycle non-specific chemotherapeutic agents that can directly insert platinum into DNA to generate monoaddutcts and DNA crosslinks, which block the unwinding of DNA double helix and inhibit DNA replication, finally leading to the necrosis or apoptosis of tumor cells (3). Moreover, the monoaddutcts formed by platinum drugs and DNA also can interact with several effector proteins to produce a series of abnormal signaling factors, thereby inducing cell death (95). However, the clinical success of platinum drugs is limited due to their severe, dose-limiting side effects and resistance to treatment. Multiple lncRNAs have been reported to be involved in the GC resistance to platinum drugs (3, 96)
Several oncogenic lncRNAs can promote the resistance of GC cells to platinum drugs. For instance, Pei et al. showed that lncRNA small nucleolar RNA host gene 7 (SNHG7) was upregulated in GC tissues and CDDP-resistant cells. The silencing of SNHG7 significantly enhanced the sensitivity of the CDDP-resistant cells. Mechanistically, SNHG7 conferred to resistance of GC cells to CDDP by downregulating the expression of LDHA via sponging miR-34a (49). In another study, Li et al. revealed that lncRNA prostate cancer-associated transcript 1 (PCAT-1) was highly expressed in CDDP-resistant GC tissues and cell lines. Similarly, PCAT-1 knockdown also enhanced the sensitivity of chemoresistant GC cells to CDDP. Mechanistically, PCAT-1 epigenetically silenced phosphatase and tensin homolog deleted on chromosome 10 (PTEN) by upregulating trimethylation of histone H3 lysine 27 (H3K27me3) via targeting enhancer of zeste homolog 2 (EZH2). Consistent with this, the knockdown of PTEN counteracted the PCAT-1 silencing-induced enhancement in the CDDP sensitivity of the chemoresistant GC cells (41). In addition, Luo et al. found that lncRNA EIF3J-DT was overexpressed in OXA-resistant GC tissues and cell lines. EIF3J-DT promoted the drug resistance of GC cells to OXA by increasing autophagy-related gene 14 (ATG14) expression via sponging miR188-3p (32).
In contrast, a number of tumor suppressor lncRNAs can reverse the platinum drugs resistance. For instance, lncRNA a disintegrin-like and metalloprotease with thrombospondin type I motif 9 antisense RNA 2 (ADAMTS9-AS2) was found to be downregulated in GC tissues and cell lines. Specifically, the overexpression of ADAMTS9-AS2 enhanced the sensitivity of chemoresistant GC cells to CDDP by increasing the NACHT, LRR, and PYD domains-containing protein 3 (NLRP3) expression via sponging miR-223-3p (39). Another study revealed that the expression lncRNA cisplatin resistance-associated lncRNA (CRAL) was decreased in CDDP-resistant GC cells. The overexpression of CRAL promoted the enhancement of CDDP sensitivity of GC cells by upregulating the expression of cylindromatosis (CYLD) via sponging miR-505 (69). Additionally, in another study, lncRNA cancer susceptibility candidate 2 (CASC2) overexpression enhanced the CDDP sensitivity of GC cells by sponging miR-19a, whereas CASC2 knockdown reversed the response of GC cells to CDDP. Consistent with this, miR-19a overexpression counteracted the CASC2 overexpression-induced enhancement in the CDDP sensitivity of GC cel (70).
Long Non-Coding RNAs and Adriamycin
ADR, also known as doxorubicin (DOX), is one of the most commonly prescribed and time-tested chemotherapeutic drugs (97). It can produce free radical to induce the impairment of DNA and the cell membrane, thereby resulting in tumor cell death. Moreover, ADR suppresses the biosynthesis of macromolecules by interacting with DNA via intercalation, thereby inhibiting DNA topoisomerase II, and leading to the stagnation of DNA replication (98). Several oncogenic lncRNAs, such as lncR-D63785, urothelial cancer-associated 1 (UCA1), and nuclear paraspeckle assembly transcript 1 (NEAT1), have been reported to promote ADR resistance in GC (81, 83, 84). For instance, in our previous work, we found that lncR-D63785 was highly expressed in both GC tissues and cell lines. The overexpression of lncR-D63785 conferred the ADR resistance of GC cells by increasing myocyte enhancer factor 2D (MEF2D) expression via sponging miR-422 (84). In another study, Shang showed that UCA1 was significantly upregulated in GC tissues and cells. The silencing of UCA1 could reverse the ADR resistance of GC cells and promote apoptosis induced by ADR by increasing cleaved Poly(ADP-ribose) polymerase (PARP) expression and decreasing Bcl-2 expression (81). Similarly, Zhang et al. showed that NEAT1 knockdown in ADR-resistant GC cells could enhance the sensitivity of GC cells to ADR and promote apoptosis induced by AD (83). In addition, another two lncRNAs, regulator of reprogramming (ROR) and MDR-related and upregulated lncRNA (MURL), were also found to promote ADR resistance in GC cells via regulating the expression of various genes (85, 99).
Long Non-Coding RNAs and Paclitaxel
PTX is a tricyclic diterpenoid compound produced from Taxus brevifolia and is considered to be one of the most successful natural chemotherapeutic agents available (100). As an antimitotic agent, PTX facilitates the assembly of tubulin into microtubules and the lengthening of the tubulin polymer, leading to the inhibition of microtubule depolymerization. The enhancement of microtubule stability interferes with microtubules’ polymerization dynamics, leading to G2/M cell cycle arrest of tumor cells (101, 102). Several oncogenic lncRNAs have been shown to be responsible for the development of PTX resistance in GC. For instance, Shang et al. found that lncRNA cancer susceptibility candidate 9 (CASC9) was significantly upregulated in both GC tissues and cells. The silencing of CASC9 in drug-resistant GC cells restored the sensitivity of cells to PTX via downregulating the expression of multidrug resistance protein 1 (MDR1) (79). Moreover, An et al. revealed that lncRNA ZNFX1 antisense RNA 1 (ZFAS1) was highly expressed in GC tissues. The knockdown of ZFAS1 enhanced the sensitivity of GC cells to PTX by decreasing β-catenin expression. Consistent with this, the overexpression of β-catenin reversed the ZFAS1 knockdown-induced resistance of GC cells to PTX (87). Wang et al. showed that lncRNA HOX transcript antisense RNA (HOTAIR) was upregulated in GC tissues. The overexpression of HOTAIR enhanced the PTX and ADR resistance in GC cells by upregulating glypican-5 (GPC5) and protein tyrosine phosphatase non-receptor type 14 (PTPN14) expression via sponging miR-217 (88). In another study, Hu et al. demonstrated that the expression of lncRNA metastasis-associated lung adenocarcinoma transcript 1 (MALAT1) was upregulated in PTX-resistant GC cells and that the silencing of MALAT1 enhanced the sensitivity of GC cells to PTX. Mechanistically, MALAT1 acted as a ceRNA to decrease ATG12 expression by sponging miR-23b-3p, leading to the enhancement of PTX resistance in GC cells (103).
Long Non-Coding RNAs and Vincristine
VCR is a vinca alkaloid that is often used in combination with other chemotherapeutic agents to treat a variety of cancers, including GC (104). Similar with PTX, VCR is also an antimitotic agent that blocks tubulin polymerization and disables spindle formation. This inhibition causes the mitosis of tumor cells to arrest at metaphase, particularly during the M and S phases (105). In addition, VCR also can interfere with the synthesis of nucleic acids and proteins by blocking glutamic acid utilization (106). Several lncRNAs have been reported to be involved in the resistance of GC cells to VCR. For instance, the high expression of oncogenic lncRNA ROR was associated with the increased MDR of GC patients. The silencing of ROR promoted the apoptosis of drug-resistant GC cells in response to VCR and ADR treatment by decreasing multidrug resistance-associated protein 1 (MRP1) expression (78). In Wang et al.’s study, lncRNA MRUL was found to be upregulated in the VCR-resistant GC cells. The knockdown of MRUL promoted apoptosis and decreased VCR release in the VCR-resistant GC cells by upregulating MRP1 expression (85).
Mechanisms of Long Non-Coding RNAs in Gastric Cancer Drug Resistance
The underlying mechanisms of GC drug resistance are very complex and still not fully understood. LncRNAs have been shown to play crucial roles in the development of GC drug resistance, indicating their great potential in precisely evaluating the sensitivity of cancer cells to chemotherapy in GC. LncRNAs regulate the development of drug resistance in GC via various mechanisms, such as the regulation of the oncogenic signaling pathways, inhibition of apoptosis, induction of autophagy, modulation of CSCs, and promotion of the EMT process (Figure 1).
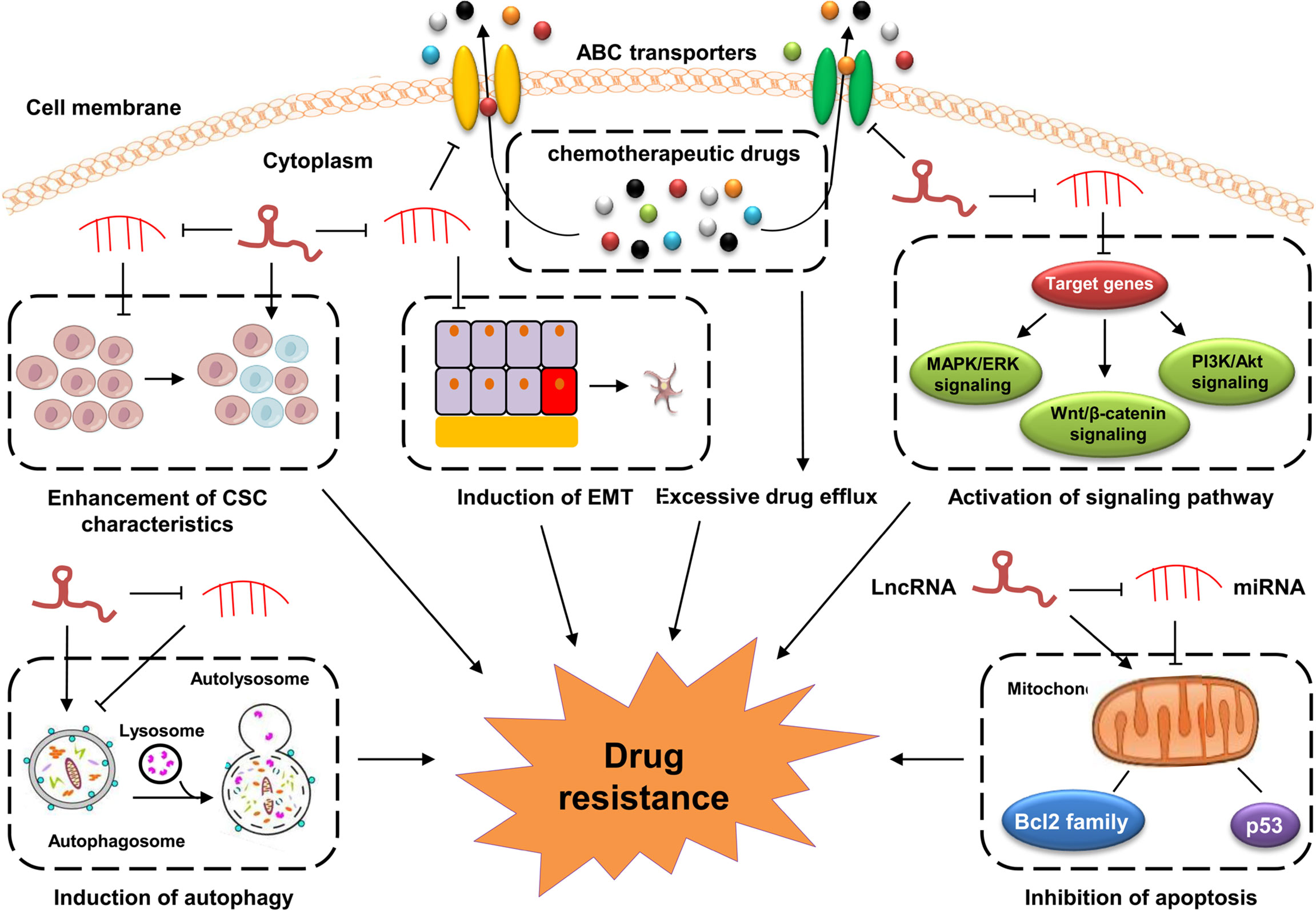
Figure 1 Mechanisms of lncRNAs in GC drug resistance. Dysregulated lncRNAs are involved in GC drug resistance by directly binding to related proteins or sponging miRNAs to altering the expression of downstream target genes involved in cell apoptosis, drug efflux system, EMT, CSCs and drug resistance-related signaling pathways.
Long Non-Coding RNAs Target Signaling Pathways in Gastric Cancer Drug Resistance
An increasing number of studies have shown that lncRNAs regulate GC drug resistance by targeting cancer-related signaling pathways, such as the phosphatidylinositol 3-kinase (PI3K)/AKT, Wnt/β−catenin, and mitogen-activated protein kinase/extracellular signal regulated kinase (MAPK/ERK) signaling pathways (46, 53, 107, 108) (Figure 2). These lncRNAs can change the sensitivity of GC cells to chemotherapy drugs by altering the activity or expression of some key components in these signaling pathways.
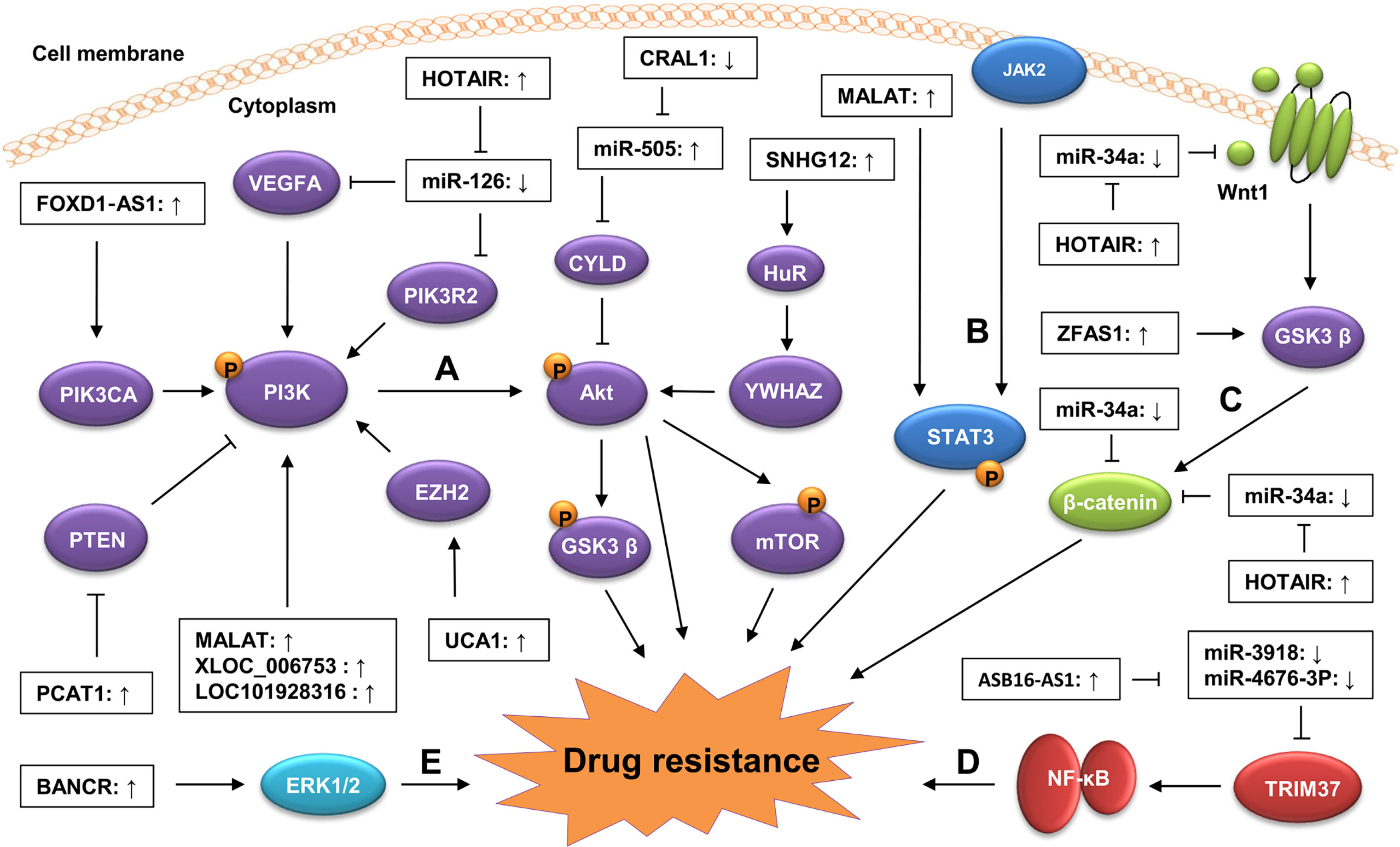
Figure 2 Regulation of lncRNAs on signaling pathways in GC drug resistance. Dysregulated lncRNAs contribute to GC drug resistance by targeting oncogenic signaling pathways, including the PI3K/AKT (A), STAT3 (B), Wnt/β-catenin (C), NF-kB (D), and (E) MAPK/ERK signaling pathways. Generally, the dysregulated lncRNAs can activate oncogenic signaling pathways by modulating the expression of key components in these signaling pathways via sponging miRNAs. They also can influence these signaling pathways by altering the modification of their key components via regulating the activity of upstream regulators.
The PI3K/AKT signaling pathway is a carcinogenic pathway involved in a variety of physiological processes, including proliferation, apoptosis, differentiation, migration, and invasion (109). The aberrant activation of this pathway is closely associated with many aspects of cancer progression, including drug resistance (110, 111). Several lncRNAs have been found to be involved in GC drug resistance by modulating the activity of the PI3K/AKT signaling pathway. For instance, Dai et al. revealed that lncRNA MALAT1 was highly expressed in GC tissues and that its upregulation enhanced the resistance of GC cells to CDDP. Consistent with this, the knockdown of MALAT1 reduced the inhibition of MALAT1 on the apoptosis of the CDDP-resistant GC cells. Mechanistically, MALAT1 activated the PI3K/AKT signaling pathway by upregulating the level of phosphorylated PI3K (p-PI3K) and p-AKT, leading to the enhancement of the CDDP resistance of GC cells (40). Zeng et al. found that lncRNA XLOC_006753 promoted the resistance of GC cells to 5-FU and CDDP by activating the PI3K/AKT signaling pathway. The silencing of XLOC_006753 in MDR GC cells significantly decreased the levels of PI3K, p-AKT, and phosphorylated mechanistic target of rapamycin (p-mTOR) (36). Moreover, Wu et al. demonstrated that the high expression of lncRNA Forkhead Box D1-antisense 1 (FOXD1‐AS1) increased the resistance of GC cells to CDDP by activating the PI3K/AKT signaling pathway via the upregulation of PIK3CA (60). PTEN is a well-known negative regulator of the PI3K/AKT signaling pathway (112, 113). Li et al. showed that PCAT-1 could downregulate PTEN expression by targeting EZH2 in CDDP-resistant GC cells, indicating that PCAT-1 might contribute to the resistance of GC cells to CDDP by activating the PI3K/AKT signaling pathway via downregulating PTEN expression (41).
The Wnt/β−catenin signaling pathway is a conserved cancer-related pathway participating in cancer progression by promoting CSC renewal, proliferation, and differentiation, thereby playing crucial roles in carcinogenesis and therapy response (114, 115). The aberrant activation of the Wnt/β−catenin pathway contributes to the development of drug resistance in a variety of cancers, including GC (116, 117). Several lncRNAs have been reported to be involved in GC drug resistance by regulating the expression of key components in the Wnt/β-catenin signaling pathway. For instance, Xu et al. showed that lncRNA ZFAS1 was highly expressed in GC tissues. The knockdown of ZFAS1 enhanced the sensitivity of GC cells to PTX and cis-platinum by inactivating the Wnt/β-catenin signaling pathway via downregulating β-catenin and phosphorylated glycogen synthase kinase-3β (p-GSK3β) level and increasing naked cuticle homolog 2 (NKD2) expression. Consistent with this, the overexpression of β-catenin reversed the inhibition of the GC drug resistance induced by the ZFAS1 knockdown (87). In another study, Cheng et al. revealed that lncRNA HOTAIR was upregulated in both GC tissues and cell lines. The knockdown of HOTAIR reduced the resistance of GC cells to CDDP. Mechanistically, HOTAIR activated the Wnt/β-catenin signaling pathway by upregulating the level of Wnt1 and β-catenin via sponging miR-34a in GC cells, resulting in the enhancement of CDDP resistance (107). In addition, some lncRNAs, such as small nucleolar RNA hostgene 11 (SNHG11), HOXC cluster antisense RNA 1 (HOXC-AS1), and DLGAP1 antisense RNA 2 (DLGAP1-AS2), have also been found to regulate the expression of the key components in the Wnt/β-catenin signaling pathway during GC progression (20, 118–120), indicating that they may also influence the development of drug resistance in GC by targeting the Wnt/β-catenin pathway.
The MAPK/ERK signaling pathway is a classical oncogenic pathway, and its dysregulation has been shown to be closely associated with the development of drug resistance in GC (121, 122). Particularly, Miao et al. found that the overexpression of lncRNA BRAF-activated non-coding RNA (BANCR) enhanced the resistance of GC cells to CDDP by activating the MAPK/ERK signaling pathway via upregulating the level of p-ERK1/2. Consistent with this, Ly3214996 (an ERK inhibitor) treatment reversed the BANCR overexpression-induced CDDP resistance of GC cells by decreasing the p-ERK1/2 level (53). In addition, lncRNAs can also regulate GC drug resistance by targeting the nuclear factor-kappaB (NF-κB) signaling pathway. Fu et al. revealed that lncRNA ASB16 antisense RNA 1 (ASB16-AS1) promoted the CDDP resistance of GC cells by activating the NF-κB signaling pathway. Mechanistically, the overexpression of ASB16-AS1 upregulated the level of NF-κB pathway-related proteins (p-IKK, p-IκB and Nuclear p65) by increasing tripartite motif-containing 37 (TRIM37) expression via sponging miR-3918 and miR4676-3p, leading to the enhancement of CDDP resistance in GC (63). Collectively, these findings suggest that targeting the oncogenic signaling pathways is a common mechanism for lncRNAs in the development of GC drug resistance. Thus, in-depth studies on the underlying mechanisms of lncRNAs in the regulation of the GC signaling pathway may provide novel insights into the development of therapeutic strategies against GC drug resistance.
Long Non-Coding RNAs Regulate Cell Apoptosis in Gastric Cancer Drug Resistance
An important function of most chemotherapeutic drugs is to promote the effective elimination of cancer cells by inducing apoptosis (123, 124). Therefore, the dysregulation of apoptosis is one of the main factors resulting in the occurrence of drug resistance. An increasing amount of evidence has shown that lncRNAs participate in the development of GC drug resistance by regulating apoptotic proteins or related pathways (65, 74, 125).
Bcl-2 family proteins are key regulators of the mitochondrial apoptotic pathway and are divided into two major groups: anti-apoptotic (e.g., Bcl-2, Mcl-1, and Bcl-xl) and pro-apoptotic (e.g., Bax, Bim, and Bak) (126, 127). Li et al. showed that lncRNA small nucleolar RNA hostgene 5 (SNHG5) was upregulated in CDDP-resistant GC tissues and cell lines. Specifically, SNHG5 overexpression in GC cells reduced the CDDP cytotoxicity and promoted cell apoptosis by upregulating Bax and downregulating Bcl-2 level. Consistent with this, the silencing of SNHG5 promoted the apoptosis of the CDDP-resistant GC cells (45). Qiao et al. revealed that lncRNA HMGA1P4 overexpression inhibited apoptosis of the CDDP-resistant GC cells by decreasing Bcl-2 and caspase3 expression and increasing Bax expression (37). Moreover, Fang et al. found that lncRNA UCA1 was highly expressed in GC tissues. The overexpression of UCA1 increased the MDR of GC cells to ADR, DDP, and 5-FU and decreased the cell apoptosis induced by ADR by upregulating Bcl-2 and downregulating caspase3 via sponging miR-27b. Consistent with this, UCA1 knockdown decreased the MDR of GC cells, and increased the ADR-induced cell apoptosis (31). Zhang et al. demonstrated that lncRNA gastric carcinoma high expressed transcript 1 (GHET1) overexpression significantly enhanced the resistance of GC cells to CDDP and inhibited cell apoptosis by downregulating Bax level and upregulating Bcl-2, MDR1 and MRP1 level. Conversely, the knockdown of GHET1 decreased MDR and promoted apoptosis in CDDP-resistant GC cells (61). Moreover, Zong et al. found that lncRNA LINC00162 overexpression increased the sensitivity of GC cells to 5-aza-2’-deoxycytidine and cell apoptosis by interacting with heterogeneous nuclear ribonucleoprotein H1 (HNRNPH1) and downregulating Bcl-XL level (128).
In addition, Cheng et al. showed that the overexpression of UCA1 could inhibit the apoptosis induced by CDDP in GC cells by upregulating cytochrome P450 1B1 enzyme (CYP1B1) expression via sponging miR-513a-3p, which lead to the enhancement of the CDDP resistance of GC cells (129). In another study, the overexpression of lncRNA differentiation antagonizing non-protein coding RNA (DANCR) was found to increase survival and decrease apoptosis in CDDP-resistant GC cells by upregulating the expression of MDR1 and MRP1 (52). Moreover, Wang et al. revealed that lncRNA CRAL overexpression promoted CDDP-induced apoptosis in GC cells by upregulating CYLD expression via sponging miR-505 (69). Zhang et al. demonstrated that lncRNA FAM84B-AS promoted the resistance of GC cells to platinum drugs by inhibiting the apoptotic pathways. Mechanistically, FAM84B-AS knockdown significantly increased the level of Bax, caspase3, caspase7, and caspase9 and then decreased the level of Bcl-2 and Bcl-xl by upregulating FAM84B expression to further inhibit the GC cell apoptosis (58). Taken together, these findings demonstrate that lncRNAs can act as oncogenes to contribute to drug resistance by suppressing cell apoptosis in GC, at least partially due to their modulation on the expression of apoptotic proteins. However, the exact mechanisms are still not fully elucidated. Therefore, in-depth studies are required to further understand the detailed mechanism of lncRNAs in GC drug resistance, which may provide new insights for the development of lncRNA-based therapeutics strategies for patients against drug resistance.
Long Non-Coding RNAs Alter Drug Efflux In Gastric Cancer Drug Resistance
Excessive drug efflux mediated by multiple cell membrane transporter proteins, such as ATP-binding cassette (ABC) proteins, is one of the main mechanisms of drug resistance in cancer cells (130). It has been reported that the overexpression of ABC proteins could enhance the efflux of anticancer drugs from cancer cells, thereby reducing their efficacy and leading to the resistance of cancer cells to these anticancer drugs (131). Currently, at least three members of ABC family have been shown to be closely associated with MDR, particularly MDR1 (also known as ABCB1 and P-glycoprotein), MRP (also known as ABCC1), and breast cancer resistant protein (BCRP) (also known as ABCG2) (132).
An accumulating number of studies have shown that lncRNAs participate in the regulation of excessive drug efflux in GC cells by targeting ABC proteins (Figure 3). MDR1 was the first-identified ABC protein associated with MDR (133). Several lncRNAs, such as bladder cancer-associated transcript 1 (BLACAT1), MRUL, PVT1, and CASC9, have been reported to regulate the development of MDR in GC by directly or indirectly targeting MDR1. For instance, Xu et al. found that lncRNA BLACAT1 was upregulated in OXA-resistant GC tissues and cell lines and that its overexpression promoted the OXA resistance of these GC cells by upregulating MDR1 expression via sequestering miR-361 (72). Wang et al. revealed that lncRNA MRUL was highly expressed in MDR GC cells with the knockdown of MRUL enhancing the sensitivity of these cells to ADR and VCR by downregulating MDR1 expression (85). Shang et al. showed that lncRNA CASC9 knockdown restored the sensitivity of MDR GC cells to PTX and ADR by decreasing MDR1 expression (79). In addition, Zhang et al. demonstrated that the upregulation of lncRNA GHET1 facilitated the development of MDR in GC cells by upregulating MDR1, Bcl-2, and MRP1 level and downregulating Bax level (61). Taken together, these findings indicate that lncRNAs can participate in the regulation of drug resistance in GC cells by altering the drug efflux. Further investigations on the underlying mechanisms of lncRNAs in drug efflux regulation is of great importance in helping GC patients overcome drug resistance.
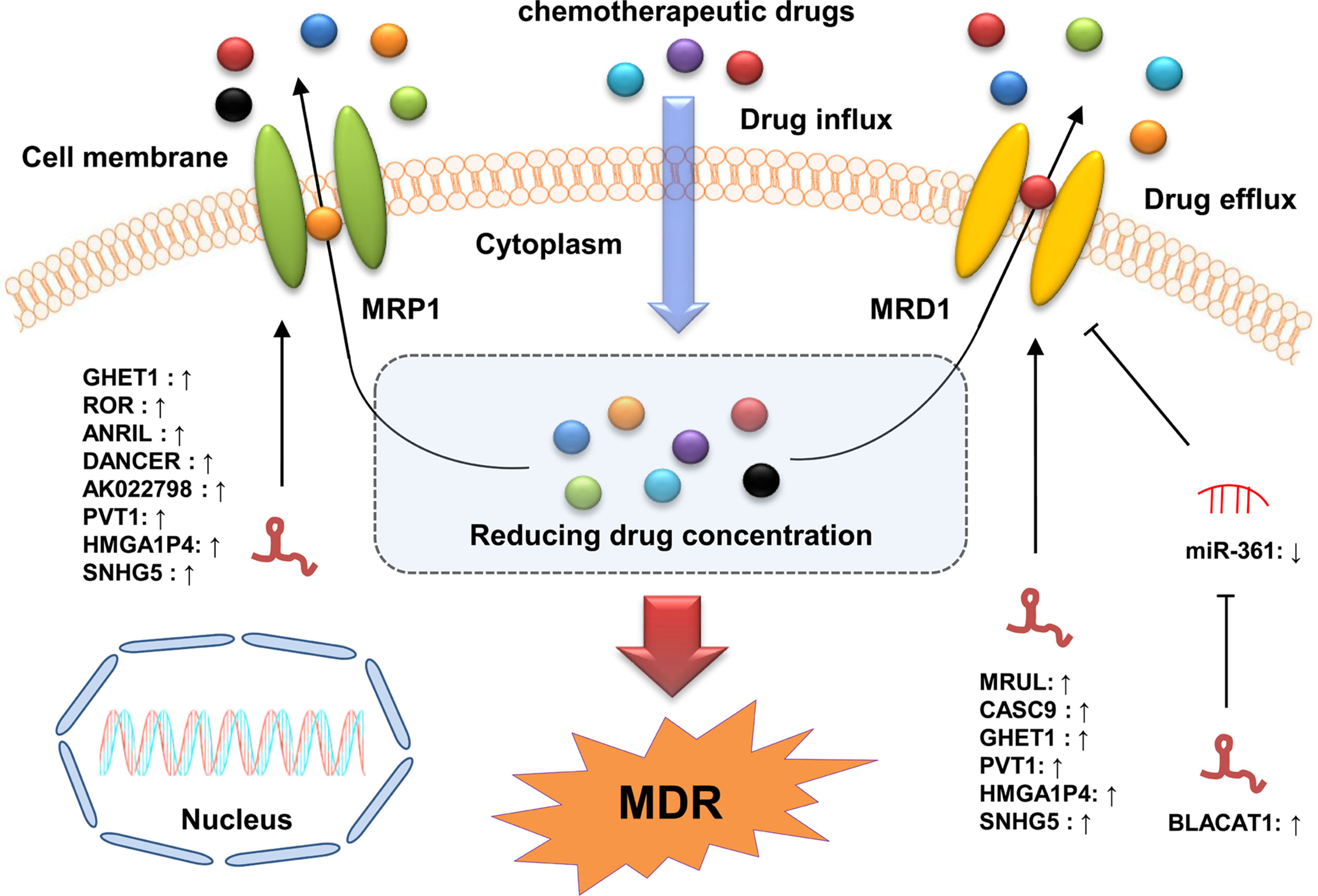
Figure 3 LncRNAs participate in GC drug resistance by regulating MDR-related genes. The ABC transporters export chemotherapeutic drugs out of GC cells, resulting in chemotherapy resistance with decreased concentrations of the drugs intracellularly. LncRNAs can modulate MDR by increasing the expression of MDR-related genes (MDR1 and MRP1) via directly binding to specific miRNAs or proteins.
Long Non-Coding RNAs and Epithelial-To-Mesenchymal Transition in Gastric Cancer Drug Resistance
EMT is a process by which epithelial cells lose their junctions and polarity, and gain migratory and invasive characteristics, producing a fibroblast-like phenotype (134, 135). The aberrant activation of the EMT process is closely associated with GC drug resistance (136). An increasing amount of evidence has shown that lncRNAs are involved in GC drug resistance by modulating the EMT process. For instance, Mao et al. revealed that lncRNA HOXA transcript at the distal tip (HOTTIP) was upregulated in CDDP-resistant GC cells. The knockdown of HOTTIP promoted the EMT progress by upregulating the level of the epithelial markers (E-cadherin and ZO-1) and downregulating the level of the mesenchymal markers (N-cadherin, vimentin, ZEB-1, and Twist), leading to the enhancement of MDR in GC cells (42). In another study, Han et al. found that lncRNA LEIGC was downregulated in GC tissues with its overexpression enhancing the sensitivity of GC cells to 5-FU by suppressing the EMT process (38). Moreover, Jia et al. demonstrated that lncRNA HOTAIR was highly expressed in GC tissues. The overexpression of HOTAIR significantly promoted the EMT process by increasing the level of N‐cadherin and vimentin and decreasing E‐cadherin level in GC cells that were subject to treatment via chemo‐therapies (80). In addition, some lncRNAs, such as metastasis-associated lung adenocarcinoma transcript-1 (MALAT-1), microvascular invasion in hepatocellular carcinoma (MVIH), NEAT1, and gastric cancer associated transcript 3 (GACAT3), have also been found to modulate the expression of EMT markers during GC progression (16, 137–140), indicating that these lncRNAs may also be involved in the development of drug resistance in GC by regulating the EMT process. EMT is a core link of metastatic process, which is closely associated with cancer recurrence and drug resistance. Therefore, dysregulated lncRNAs are likely to play a positive role in the development of GC drug resistance by aberrantly activating the EMT process. These findings support the hypothesis that lncRNAs are key regulators in GC drug resistance, but the molecular mechanisms still need further investigation.
Long Non-Coding RNAs Influence Gastric Cancer Drug Resistance by Regulating Cancer Stem Cell Characteristics
CSCs are a unique subpopulation of cancer cells within a tumor with high tumorigenic potency and have been recognized as the main cause of the chemotherapeutic resistance, metastasis, and recurrence of cancer (141, 142). An accumulating number of studies have shown that lncRNAs can participate in the regulation of GC drug resistance by regulating the characteristics of CSC. For instance, Sun et al. showed that lncRNA small nucleolar RNA hostgene 3 (SNHG3) upregulation was positively associated with the CDDP resistance and stemness of GC cells, whereas SNHG3 downregulation inhibited the stem cell-like properties of these GC cells. Mechanistically, SNHG3 significantly enhanced the stemness of GC cells by increasing ADP-ribosylation factor-like protein 2 (ARL2) expression via sponging miR-3619-5p, leading to the enhancement of GC CDDP resistance (66). Fu et al. revealed that lncRNA ASB16 antisense RNA 1 (ASB16-AS1) was highly expressed in GC tissues. Specifically, the overexpression of ASB16-AS1 strengthened the stem cell-like features of GC cells by upregulating TRIM37 expression via sponging miR-3918 and miR-4676-3p, resulting in the enhancement of CDDP resistance in GC (63). Wang et al. found that lncRNA ROR was highly expressed in CD133+ gastric CSCs (GCSCs). The overexpression of ROR promoted the pluripotent state of GCSCs by upregulating several key stemness transcriptional factors, such as octamer-binding transcription factor 4 (OCT4), SRY-box transcription factor 2 (SOX2), and NANOG (143). Furthermore, lncRNAs MALAT1 and testis-associated highly-conserved oncogenic long non-coding RNA (THOR) have been shown to increase the stemness of GC cells by enhancing SOX2 and SOX9 mRNA stability, respectively (144–146). In addition, the upregulation of lncRNAs histocompatibility leukocyte antigen complex P5 (HCP5) and MACC1-AS1 induced via mesenchymal stem cell (MSC) co-culturing was found to enhance the stemness and drug-resistance of GC cells by droving FAO (93, 147).
Long Non-Coding RNAs and Autophagy in Gastric Cancer Drug Resistance
Autophagy is a complex, highly conserved self-metabolic process that is essential for maintaining cellular homeostasis during stress conditions, such as hypoxia and nutrient deprivation (148, 149). It has been reported that autophagy plays dual roles in GC drug resistance. On the one hand, it can promote drug resistance by protecting GC cells from the cytotoxicity of chemotherapeutic agents. On the other hand, it can reverse drug resistance through facilitating apoptosis and/or inhibiting EMT (150). Therefore, targeting autophagy may be one of the crucial therapeutic strategies against GC drug resistance. Furthermore, an increasing number of studies have shown that lncRNAs are involved in GC drug resistance by targeting autophagy (68, 103) (Figure 4).
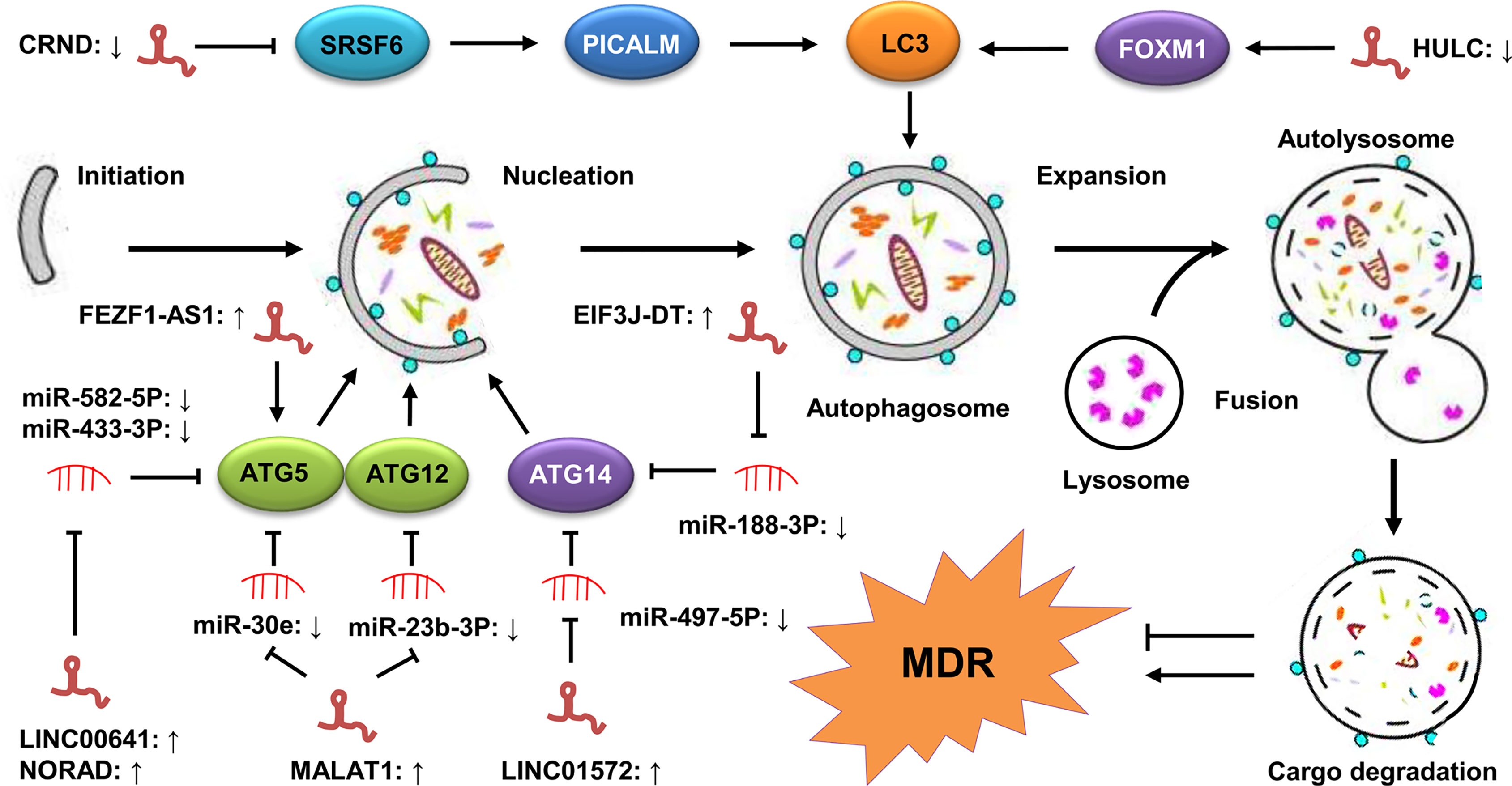
Figure 4 LncRNAs regulate autophagy-mediated GC drug resistance. The process of autophagy contains five steps, including initiation, vesicle nucleation, vesicle expansion, vesicle fusion, and cargo degradation. These steps are tightly regulated by a series of ATGs, including ATG5, ATG12, ATG14 and LC3. LncRNAs are involved in autophagy-mediated MDR of GC cells by modulating the expression of these ATGs via sponging miRNAs or directly targeting them.
Zhang et al. showed that the expression of lncRNA CRNDE was downregulated in CDDP-resistant GC cells. The low level of CRNDE promoted the MDR of GC cells to 5-FU and OXA by upregulating autophagy flux in GC. Mechanistically, CRNDE reduced the alternative splicing of PICALM by decreasing the protein stability of SRSF6, leading to the enhancement of autophagy-mediated drug resistance (19). In another study, Wang et al. found that lncRNA non-coding RNA activated by DNA damage (NORAD) was highly expressed in OXA-resistant GC tissues, with its upregulation in the OXA-resistant GC cells promoting the autophagy flux by stabilizing the autophagy related gene 5 (ATG5)-ATG12 complex via sponging miR-433-3p, leading to the enhancement of CDDP resistance (76). Moreover, Luo et al. revealed that lncRNA EIF3J-DT was upregulated in drug-resistant GC cells. The high expression of EIF3J-DT activated autophagy and induced drug resistance in these GC cells by upregulating ATG14 expression via sequestering miR188-3p (32). In addition, some lncRNAs, such as FEZF1 antisense RNA 1 (FEZF1-AS1), LINC00641, and MALAT1, have also been found to mediate drug resistance in GC by activating autophagy (33, 75, 103). Collectively, these findings suggest that the lncRNA regulation of autophagy is an important mechanism in the development of GC drug resistance. However, how lncRNAs participate in GC drug resistance by modulating autophagy is still not fully understood. Accordingly, in-depth studies are required to elucidate its exact mechanisms.
Clinical Implication of Drug Resistance-Related Long Non-Coding RNAs in Gastric Cancer
Long Non-Coding RNAs as Diagnostic and Prognostic Biomarkers
The recurrence and mortality rates of GC are still high due to the lack of efficient approaches for its early diagnosis and prognostic assessment in clinical practice. Currently, some conventional biomarkers, such as carcinoembryonic antigen (CEA), carbohydrate antigen 19-9 (CA19-9), and cancer antigen 72-4 (CA72-4), have been used in clinic, but the low diagnostic accuracies of these biomarkers limit their further application (151, 152). Thus, the screening and identification of valuable molecular biomarkers with high specificity and sensitivity is very essential for GC patients, particularly those with a poor response to chemotherapy, to obtain timely and effective treatment.
In recent years, a large number of studies have shown the key role of drug resistance-related lncRNAs in the pathogenesis of GC. Additionally, the differential expression of these lncRNAs endows them with great potential as biomarkers for the diagnosis, prognosis and treatment of GC patients (Table 2). For instance, the expression of lncRNA SNHG5 was significantly downregulated in the serum and tissues of GC patients before operation as well as those with poor prognosis, and its downregulation was closely associated with drinking history and Tumor-Node-Metastasis (TNM) stages in these patients, indicating that SNHG5 is a survival predictor of short-term adverse events in GC patients (155). In another study, the overexpression of SNHG5 decreased CDDP cytotoxicity and inhibited apoptosis in GC cells (45). This data suggests that SNHG5 processes a high diagnostic value in prognosis prediction and is a promising biomarker for the early diagnosis of GC. In another study, lncRNA PVT1 was upregulated in both the tissues and cells of CDDP‐resistant patients. This overexpression of PVT1 promoted the apoptosis induced by CDDP in GC cells by upregulating the expression of MDR‐related genes, such as MDR1, mTOR, and MRP (156). Furthermore, the upregulation of PVT1 was closely associated with late tumor stage (III–IV), lymph node metastasis, and OS/disease-free survival (DFS) (153). These findings provide evidence that PVT1 can be used as a candidate biomarker for the diagnosis and personalized therapeutic treatment of GC patients. Besides, lncRNA NORAD was found to be highly expressed in OXA-resistant GC tissues. The overexpression of NORAD enhanced the autophagy flux in OXA-resistant GC cells by stabilizing the ATG12 complex via sponging miR-433-3p. This data suggests that NORAD can serve as a clinically useful biomarker for predicting OXA resistance in GC. In addition, some drug resistance-related lncRNAs, such as UCA1, DDX11 antisense RNA 1 (DDX11-AS1), and XLOC_006753, have also exhibited great potential as prognostic biomarkers for GC patients (36, 73, 81). However, the potential value of lncRNAs as biomarkers for GC patients needed be further confirmed via larger population-based studies.
Long Non-Coding RNAs as Therapeutic Targets
LncRNAs play crucial roles in practically all aspects of GC progression, including drug resistance, suggesting their great clinical value as therapeutic targets or therapeutic agents in GC patients, particularly those exhibiting a poor response to chemotherapy. Targeting these lncRNAs may provide novel insights into the development of therapeutic strategies for reversing GC drug resistance. For instance, Xu et al. showed that lncRNA DANCR was strongly upregulated in CDDP-resistant GC tissues and cell lines, and its knockdown by small interfering RNA (siRNA) was shown to decrease survival and increase apoptosis in CDDP-resistant GC cells (52). Lan et al. revealed that lncRNA antisense non-coding RNA in the INK4 locus (ANRIL) was highly expressed in CDDP and 5-FU-resistant GC tissues and cell lines. The knockdown of ANRIL by siRNA could inhibit the development of the MDR of GC cells to CDDP and 5-FU by upregulating the expression of MDR1 and MRP1 (34). Han et al. found that the high expression of lncRNA X inactive-specific transcript (XIST) contributed to the CDDP resistance of GC cells. The knockdown of XIST by siRNA significantly inhibited cell proliferation and promoted apoptosis via modulating the expression of Aurora kinase B (AURKB) (50). These evidences strongly suggest that lncRNAs possess great potential as effective therapeutic targets reversing GC drug resistance. Thus, the identification and synthesis of novel drugs targeting lncRNA is a promising pathway for the clinical treatment of GC patients, particularly those with drug resistance. Furthermore, lncRNAs can be regulate by several oncogenic signaling pathways, such as Wnt/β−catenin and STAT3 signaling pathways (157, 158), indicating that the screening and synthesis of novel inhibitors targeting the nodes of these pathways is also an attractive strategy to treat GC. In addition, several efficient RNA‐based techniques, such as siRNA, clustered regularly interspaced short palindromic repeats (CRISPR)/CRISPR-associated (Cas) protein 9 (CRISPR/Cas9), epigenetic modifiers, and transcription activator-like effector nuclease, are also used to target lncRNAs in GC treatment (159). However, lncRNA-based therapies have not yet been translated into GC treatment in clinic, and further investigations are still needed to elucidate the mechanisms of lncRNAs in GC drug resistance.
Conclusion and Perspective
GC is the most common malignant diseases in the digestive system, with high recurrence and mortality rate (139). Chemotherapy remains the first-line standard therapeutic method for GC patients and has efficiently improved patients’ prognosis. However, most patients eventually develop resistance to chemotherapeutic agents, leading to the failure of clinical treatment and, ultimately patients’ death (160). In recent years, with the rapid development of high-throughput sequencing techniques and bioinformatics, a large number of aberrantly expressed lncRNAs were found to be involved in GC drug resistance via various mechanisms, including targeting the oncogenic signaling pathways, inhibition of apoptosis, induction of autophagy, modulation of CSCs, and promotion of the EMT process.
Early diagnosis and prognostic assessment are crucial for the effective treatment of GC patients (161). Therefore, the screening and identification of valuable biomarkers with a high specificity and sensitivity is of great importance in the development of individual diagnose and therapeutic strategies for GC patients, particularly those with a poor response to chemotherapy. Accumulating studies suggest that lncRNA profiling can provide a large number of valuable information for cancer diagnosis, prognosis and treatment. This means that it is plausible that integrating lncRNA data into clinical trials to confirm their clinical implication. Based on the association observed between lncRNA and GC progression, it is possible to assume that specific lncRNAs will shortly be used as clinical biomarkers for the diagnosis and prognosis of GC patients. In addition, lncRNAs are also recognized as therapeutic targets or therapeutic agents for GC patients (21). Compared with protein-coding genes, lncRNA therapy exhibits several advantages: Firstly, lncRNAs are highly abundantly expressed in humans with about 5,400 to more than 10,000 lncRNA transcripts (162), indicating that lncRNAs could be more accessible targets for cancer therapy. Secondly, there are no drug resistance reports for lncRNA therapy up to now. Thirdly, chemical modifications can significantly enhance the activity and stability of lncRNAs (163). Currently, some molecular techniques based on lncRNAs, such as siRNA, CRISPR/Cas9, epigenetic modifiers, and transcription activator-like effector nuclease, have been applied to the treatment of cancers, including GC (Figure 5). However, some limitations, such as side effects, off-target effects, and a lack of a reliable delivery system, still exist in translating these research findings on lncRNA-mediated GC drug resistance into clinical application. Nevertheless, lncRNA-based therapeutic strategies have exhibited promising potential in helping GC patients overcome drug resistance. However, in-depth investigations are further required to elucidate the exact mechanisms of lncRNAs in GC drug resistance.
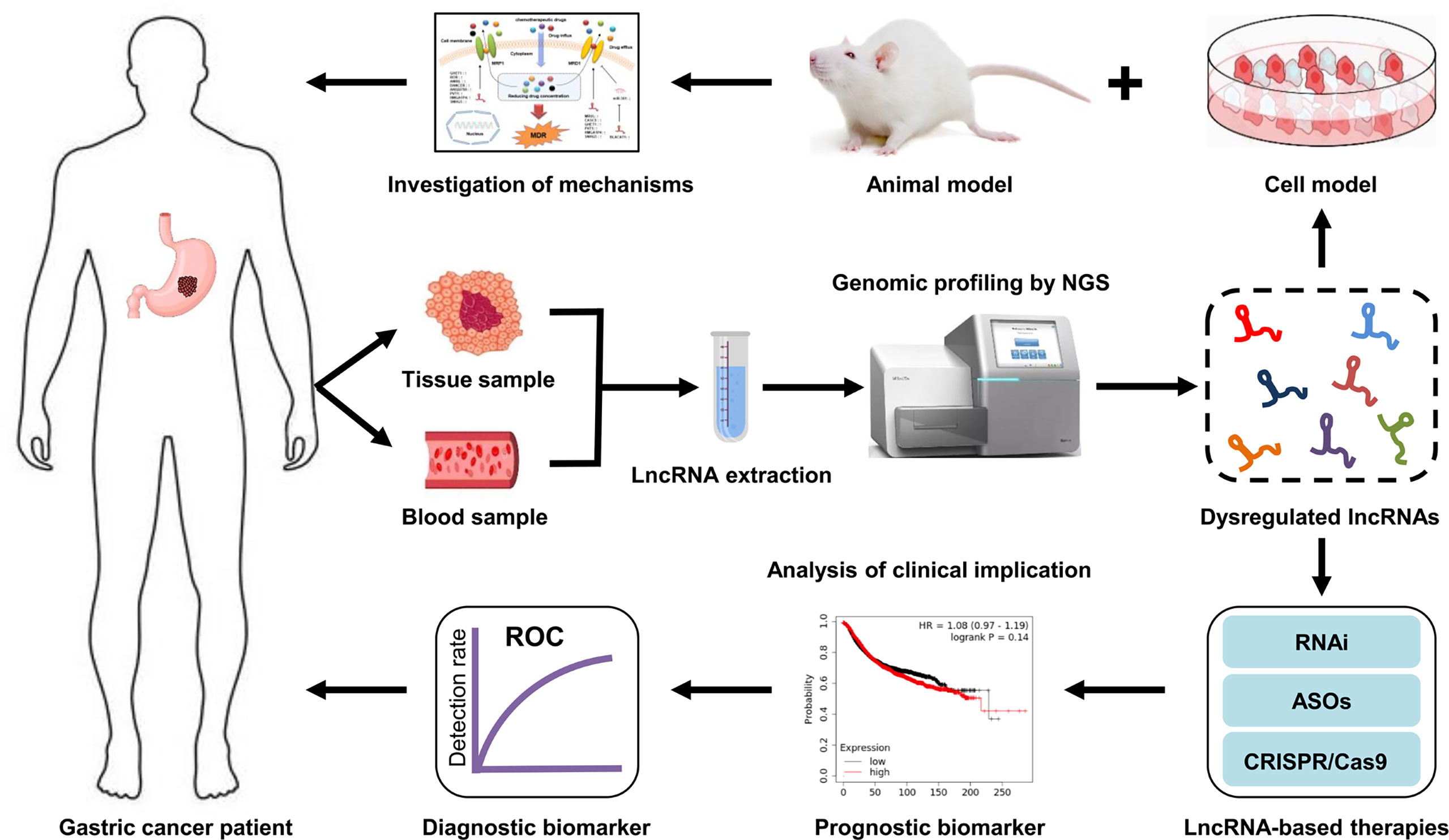
Figure 5 Clinical implications of lncRNAs in GC drug resistance. LncRNAs are extracted from tissue or blood samples of GC patients. Next, the expression profiles of lncRNA are analyzed by high-throughput sequencing technologies. Subsequently, the dysregulated lncRNAs are screened via bioinformatics analysis. The mechanisms of drug resistance-related lncRNAs in GC are investigated using cell and animal models. The aberrantly expressed lncRNAs that can serve as diagnostic or prognostic biomarkers for drug resistant-GC patients are identified. Additionally, targeting specific lncRNAs may be a new therapeutic strategy for GC patients, particularly those with a poor response to chemotherapy.
In summary, research on the association between lncRNAs and GC drug resistance has attracted much attention, with an increasing number of lncRNAs proved to be involved in GC drug resistance. However, our overall understanding of this relationship is still insufficient, and further studies are required to fully elucidate their relevant mechanism, and these may provide novel insights into the development of therapeutic strategies to reverse GC drug resistance.
Author Contributions
YL: Writing—conceptualization, original draft preparation; writing—review and editing, funding acquisition. XA: Data curation, writing—review and editing. YW: Data curation. XL: Data curation. JW: Writing—review and editing. All authors contributed to the article and approved the submitted version.
Funding
All authors are supported by Qingdao Medical College, Qingdao University. This work was funded by the National Natural Science Foundation of China (81802822) and the China Postdoctoral Science Foundation (2018M642607).
Conflict of Interest
The authors declare that the research was conducted in the absence of any commercial or financial relationships that could be construed as a potential conflict of interest.
Publisher’s Note
All claims expressed in this article are solely those of the authors and do not necessarily represent those of their affiliated organizations, or those of the publisher, the editors and the reviewers. Any product that may be evaluated in this article, or claim that may be made by its manufacturer, is not guaranteed or endorsed by the publisher.
References
1. Seidlitz T, Koo BK, Stange DE. Gastric Organoids-an In Vitro Model System for the Study of Gastric Development and Road to Personalized Medicine. Cell Death Differ (2021) 28(1):68–83. doi: 10.1038/s41418-020-00662-2
2. Sung H, Ferlay J, Siegel RL, Laversanne M, Soerjomataram I, Jemal A, et al. Global Cancer Statistics 2020: GLOBOCAN Estimates of Incidence and Mortality Worldwide for 36 Cancers in 185 Countries. CA Cancer J Clin (2021) 71(3):209–49. doi: 10.3322/caac.21660
3. Wei L, Sun J, Zhang N, Zheng Y, Wang X, Lv L, et al. Noncoding RNAs in Gastric Cancer: Implications for Drug Resistance. Mol Cancer (2020) 19(1):62. doi: 10.1186/s12943-020-01185-7
4. He J, Zhu S, Liang X, Zhang Q, Luo X, Liu C, et al. LncRNA as a Multifunctional Regulator in Cancer Multi-Drug Resistance. Mol Biol Rep (2021) 48(8):1–15. doi: 10.1007/s11033-021-06603-7
5. Liu Y, Ao X, Ji G, Zhang Y, Yu W, Wang J. Mechanisms of Action and Clinical Implications of Micrornas in the Drug Resistance of Gastric Cancer. Front Oncol (2021) 11:768918. doi: 10.3389/fonc.2021.768918
6. Yuan L, Xu ZY, Ruan SM, Mo S, Qin JJ, Cheng XD. Long Non-Coding RNAs Towards Precision Medicine in Gastric Cancer: Early Diagnosis, Treatment, and Drug Resistance. Mol Cancer (2020) 19(1):96. doi: 10.1186/s12943-020-01219-0
7. Zhang Y, Wei YJ, Zhang YF, Liu HW, Zhang YF. Emerging Functions and Clinical Applications of Exosomal Ncrnas in Ovarian Cancer. Front Oncol (2021) 11:765458. doi: 10.3389/fonc.2021.765458
8. Zhang YF, Shan C, Wang Y, Qian LL, Jia DD, Zhang YF, et al. Cardiovascular Toxicity and Mechanism of Bisphenol a and Emerging Risk of Bisphenol s. Sci Total Environ (2020) 723:137952. doi: 10.1016/j.scitotenv.2020.137952
9. Liu Y, Ding W, Yu W, Zhang Y, Ao X, Wang J. Long Non-Coding RNAs: Biogenesis, Functions, and Clinical Significance in Gastric Cancer. Mol Ther - Oncolytics (2021) 23:458–76. doi: 10.1016/j.omto.2021.11.005
10. Statello L, Guo CJ, Chen LL, Huarte M. Gene Regulation by Long Non-Coding RNAs and Its Biological Functions. Nat Rev Mol Cell Biol (2021) 22(2):96–118. doi: 10.1038/s41580-020-00315-9
11. Goodall GJ, Wickramasinghe VO. RNA in Cancer. Nat Rev Cancer (2021) 21(1):22–36. doi: 10.1038/s41568-020-00306-0
12. Chen C, Tang X, Liu Y, Zhu J, Liu J. Induction/Reversal of Drug Resistance in Gastric Cancer by Non-Coding RNAs (Review). Int J Oncol (2019) 54(5):1511–24. doi: 10.3892/ijo.2019.4751
13. Guo B, Cheng Y, Yao L, Zhang J, Lu J, Qi H, et al. Lncrna HOTAIR Regulates the Lipid Accumulation in Non-Alcoholic Fatty Liver Disease via miR-130b-3p/ROCK1 axis. Cell Signal (2022) 90:110190. doi: 10.1016/j.cellsig.2021.110190
14. Jayasuriya R, Ganesan K, Xu B, Ramkumar KM. Emerging Role of Long Non-Coding RNAs in Endothelial Dysfunction and Their Molecular Mechanisms. BioMed Pharmacother (2022) 145:112421. doi: 10.1016/j.biopha.2021.112421
15. Li ZY, Xie Y, Deng M, Zhu L, Wu X, Li G, et al. C-Myc-Activated Intronic Mir-210 and Lncrna MIR210HG Synergistically Promote the Metastasis of Gastric Cancer. Cancer Lett (2022) 526:322–34. doi: 10.1016/j.canlet.2021.11.006
16. Shan P, Yang F, Qi H, Hu Y, Zhu S, Sun Z, et al. Alteration of MDM2 by the Small Molecule YF438 Exerts Antitumor Effects in Triple-Negative Breast Cancer. Cancer Res (2021) 81(15):4027–40. doi: 10.1158/0008-5472.CAN-20-0922
17. Zhang H, Ma RR, Zhang G, Dong Y, Duan M, Sun Y, et al. Long Noncoding RNA lnc-LEMGC Combines With DNA-Pkcs to Suppress Gastric Cancer Metastasis. Cancer Lett (2022) 524:82–90. doi: 10.1016/j.canlet.2021.09.042
18. Bossaghzadeh F, Hajjari M, Sheikhi A, Salahshourifar I, Irani S. HOTAIR Induces the Downregulation of Mir-200 Family Members in Gastric Cancer Cell Lines. Iran BioMed J (2022) 26(1):77–84. doi: 10.52547/ibj.26.1.77
19. Zhang F, Wang H, Yu J, Yao X, Yang S, Li W, et al. Lncrna CRNDE Attenuates Chemoresistance in Gastric Cancer via SRSF6-Regulated Alternative Splicing of PICALM. Mol Cancer (2021) 20(1):6. doi: 10.1186/s12943-020-01299-y
20. Wu Q, Ma J, Wei J, Meng W, Wang Y, Shi M. Lncrna SNHG11 Promotes Gastric Cancer Progression by Activating the Wnt/Beta-Catenin Pathway and Oncogenic Autophagy. Mol Ther (2021) 29(3):1258–78. doi: 10.1016/j.ymthe.2020.10.011
21. Chen D, Ping S, Xu Y, Wang M, Jiang X, Xiong L, et al. Non-Coding RNAs in Gastric Cancer: From Malignant Hallmarks to Clinical Applications. Front Cell Dev Biol (2021) 9:732036. doi: 10.3389/fcell.2021.732036
22. Mirzaei S, Zarrabi A, Hashemi F, Zabolian A, Saleki H, Ranjbar A, et al. Regulation of Nuclear Factor-Kappab (NF-Kappab) Signaling Pathway by Non-Coding RNAs in Cancer: Inhibiting or Promoting Carcinogenesis? Cancer Lett (2021) 509:63–80. doi: 10.1016/j.canlet.2021.03.025
23. Ashrafizaveh S, Ashrafizadeh M, Zarrabi A, Husmandi K, Zabolian A, Shahinozzaman M, et al. Long Non-Coding RNAs in the Doxorubicin Resistance of Cancer Cells. Cancer Lett (2021) 508:104–14. doi: 10.1016/j.canlet.2021.03.018
24. Ghafouri-Fard S, Taheri M. Long Non-Coding RNA Signature in Gastric Cancer. Exp Mol Pathol (2020) 113:104365. doi: 10.1016/j.yexmp.2019.104365
25. Vasan N, Baselga J. Hyman DM. A View on Drug Resistance in Cancer. Nature (2019) 575(7782):299–309. doi: 10.1038/s41586-019-1730-1
26. Wang M, Chen W, Chen J, Yuan S, Hu J, Han B, et al. Abnormal Saccharides Affecting Cancer Multi-Drug Resistance (MDR) and the Reversal Strategies. Eur J Med Chem (2021) 220:113487. doi: 10.1016/j.ejmech.2021.113487
27. Wu Q, Yang Z, Nie Y, Shi Y, Fan D. Multi-Drug Resistance in Cancer Chemotherapeutics: Mechanisms and Lab Approaches. Cancer Lett (2014) 347(2):159–66. doi: 10.1016/j.canlet.2014.03.013
28. Du P, Hu C, Qin Y, Zhao J, Patel R, Fu Y, et al. Lncrna PVT1 Mediates Antiapoptosis and 5-Fluorouracil Resistance via Increasing Bcl2 Expression in Gastric Cancer. J Oncol (2019) 2019:9325407. doi: 10.1155/2019/9325407
29. Hu J, Huang L, Ding Q, Lv J, Chen Z. Long Noncoding RNA HAGLR Sponges Mir-338-3p to Promote 5-Fu Resistance in Gastric Cancer Through Targeting the LDHA-Glycolysis Pathway. Cell Biol Int (2021). doi: 10.1002/cbin.11714
30. Gao Y, Xie M, Guo Y, Yang Q, Hu S, Li Z. Long Non-Coding RNA FGD5-AS1 Regulates Cancer Cell Proliferation and Chemoresistance in Gastric Cancer Through Mir-153-3p/CITED2 Axis. Front Genet (2020) 11:715. doi: 10.3389/fgene.2020.00715
31. Fang Q, Chen X, Zhi X. Long Non-Coding RNA (Lncrna) Urothelial Carcinoma Associated 1 (UCA1) Increases Multi-Drug Resistance of Gastric Cancer via Downregulating Mir-27b. Med Sci Monit (2016) 22:3506–13. doi: 10.12659/msm.900688
32. Luo Y, Zheng S, Wu Q, Wu J, Zhou R, Wang C, et al. Long Noncoding RNA (Lncrna) EIF3J-DT Induces Chemoresistance of Gastric Cancer via Autophagy Activation. Autophagy (2021) 17(12):4083–101. doi: 10.1080/15548627.2021.1901204
33. Gui Z, Zhao Z, Sun Q, Shao G, Huang J, Zhao W, et al. Lncrna FEZF1-AS1 Promotes Multi-Drug Resistance of Gastric Cancer Cells via Upregulating ATG5. Front Cell Dev Biol (2021) 9:749129. doi: 10.3389/fcell.2021.749129
34. Lan WG, Xu DH, Xu C, Ding CL, Ning FL, Zhou YL, et al. Silencing of Long Non-Coding RNA ANRIL Inhibits the Development of Multidrug Resistance in Gastric Cancer Cells. Oncol Rep (2016) 36(1):263–70. doi: 10.3892/or.2016.4771
35. Xu Y, Ji T, An N, Wang X, Zhang H, Xu F. LINC00943 Is Correlated With Gastric Cancer and Regulates Cancer Cell Proliferation and Chemosensitivity via Hsa-Mir-101-3p. Int J Clin Oncol (2021) 26(9):1650–60. doi: 10.1007/s10147-021-01945-5
36. Zeng L, Liao Q, Zou Z, Wen Y, Wang J, Liu C, et al. Long Non-Coding RNA XLOC_006753 Promotes the Development of Multidrug Resistance in Gastric Cancer Cells Through the PI3K/AKT/Mtor Signaling Pathway. Cell Physiol Biochem (2018) 51(3):1221–36. doi: 10.1159/000495499
37. Qiao XL, Zhong ZL, Dong Y, Gao F. Lncrna HMGA1P4 Promotes Cisplatin-Resistance in Gastric Cancer. Eur Rev Med Pharmacol Sci (2020) 24(17):8830–6. doi: 10.26355/eurrev_202009_22822
38. Han Y, Ye J, Wu D, Wu P, Chen Z, Chen J, et al. LEIGC Long Non-Coding RNA Acts as a Tumor Suppressor in Gastric Carcinoma by Inhibiting the Epithelial-to-Mesenchymal Transition. BMC Cancer (2014) 14:932. doi: 10.1186/1471-2407-14-932
39. Ren N, Jiang T, Wang C, Xie S, Xing Y, Piao D, et al. Lncrna ADAMTS9-AS2 Inhibits Gastric Cancer (GC) Development and Sensitizes Chemoresistant GC Cells to Cisplatin by Regulating Mir-223-3p/NLRP3 Axis. Aging (Albany NY) (2020) 12(11):11025–41. doi: 10.18632/aging.103314
40. Dai Q, Zhang T, Li C. Lncrna MALAT1 Regulates the Cell Proliferation and Cisplatin Resistance in Gastric Cancer via PI3K/AKT Pathway. Cancer Manag Res (2020) 12:1929–39. doi: 10.2147/CMAR.S243796
41. Li H, Ma X, Yang D, Suo Z, Dai R, Liu C. PCAT-1 Contributes to Cisplatin Resistance in Gastric Cancer Through Epigenetically Silencing PTEN via Recruiting EZH2. J Cell Biochem (2020) 121(2):1353–61. doi: 10.1002/jcb.29370
42. Mao Z, Wu Y, Zhou J, Xing C. Salinomycin Reduces Epithelial-Mesenchymal Transition-Mediated Multidrug Resistance by Modifying Long Noncoding RNA HOTTIP Expression in Gastric Cancer Cells. Anticancer Drugs (2019) 30(9):892–9. doi: 10.1097/CAD.0000000000000786
43. Xin L, Zhou LQ, Liu C, Zeng F, Yuan YW, Zhou Q, et al. Transfer of Lncrna CRNDE in TAM-Derived Exosomes Is Linked With Cisplatin Resistance in Gastric Cancer. EMBO Rep (2021) 22(12):e52124. doi: 10.15252/embr.202052124
44. Sun H, Wu P, Zhang B, Wu X, Chen W. MCM3AP-AS1 Promotes Cisplatin Resistance in Gastric Cancer Cells via the Mir-138/FOXC1 Axis. Oncol Lett (2021) 21(3):211. doi: 10.3892/ol.2021.12472
45. Li M, Zhang YY, Shang J, Xu YD. Lncrna SNHG5 Promotes Cisplatin Resistance in Gastric Cancer via Inhibiting Cell Apoptosis. Eur Rev Med Pharmacol Sci (2019) 23(10):4185–91. doi: 10.26355/eurrev_201905_17921
46. Dai Q, Zhang T, Pan J, Li C. Lncrna UCA1 Promotes Cisplatin Resistance in Gastric Cancer via Recruiting EZH2 and Activating PI3K/AKT Pathway. J Cancer (2020) 11(13):3882–92. doi: 10.7150/jca.43446
47. Song Z, Jia N, Li W, Zhang XY. LINC01572 Regulates Cisplatin Resistance in Gastric Cancer Cells by Mediating Mir-497-5p. Onco Targets Ther (2020) 13:10877–87. doi: 10.2147/OTT.S267915
48. Ye Y, Yang S, Han Y, Sun J, Xv L, Wu L, et al. HOXD-AS1 Confers Cisplatin Resistance in Gastric Cancer Through Epigenetically Silencing PDCD4 via Recruiting EZH2. Open Biol (2019) 9(9):190068. doi: 10.1098/rsob.190068
49. Pei LJ, Sun PJ, Ma K, Guo YY, Wang LY, Liu FD. Lncrna-SNHG7 Interferes With Mir-34a to De-Sensitize Gastric Cancer Cells to Cisplatin. Cancer biomark (2021) 30(1):127–37. doi: 10.3233/CBM-201621
50. Han X, Zhang HB, Li XD, Wang ZA. Long Non-Coding RNA X-Inactive-Specific Transcript Contributes to Cisplatin Resistance in Gastric Cancer by Sponging Mir-Let-7b. Anticancer Drugs (2020) 31(10):1018–25. doi: 10.1097/CAD.0000000000000942
51. Yan J, Dang Y, Liu S, Zhang Y, Zhang G. Lncrna HOTAIR Promotes Cisplatin Resistance in Gastric Cancer by Targeting Mir-126 to Activate the PI3K/AKT/MRP1 Genes. Tumour Biol (2016) 37:16345–55. doi: 10.1007/s13277-016-5448-5
52. Xu YD, Shang J, Li M, Zhang YY. Lncrna DANCR Accelerates the Development of Multidrug Resistance of Gastric Cancer. Eur Rev Med Pharmacol Sci (2019) 23(7):2794–802. doi: 10.26355/eurrev_201904_17554
53. Miao X, Liu Y, Fan Y, Wang G, Zhu H. Lncrna BANCR Attenuates the Killing Capacity of Cisplatin on Gastric Cancer Cell Through the ERK1/2 Pathway. Cancer Manag Res (2021) 13:287–96. doi: 10.2147/CMAR.S269679
54. Wu C, Hu Y, Ning Y, Zhao A, Zhang G, Yan L. Long Noncoding RNA Plasmacytoma Variant Translocation 1 Regulates Cisplatin Resistance via Mir-3619-5p/TBL1XR1 Axis in Gastric Cancer. Cancer Biother Radiopharm (2020) 35(10):741–52. doi: 10.1089/cbr.2019.3342
55. Jin W, Zhang H, Li M, Lin S. Long Noncoding RNA Regulator of Reprogramming Regulates Cell Growth, Metastasis, and Cisplatin Resistance in Gastric Cancer via Mir-519d-3p/HMGA2 Axis. Cancer Biother Radiopharm (2020). doi: 10.1089/cbr.2019.3525
56. Mei J, Liu G, Li R, Xiao P, Yang D, Bai H, et al. Lncrna SNHG6 Knockdown Inhibits Cisplatin Resistance and Progression of Gastric Cancer Through Mir-1297/BCL-2 Axis. Biosci Rep (2021) 41(12):BSR20211885. doi: 10.1042/BSR20211885
57. Ren H, Tang L. HDAC3-Mediated Lncrna-LOC101928316 Contributes to Cisplatin Resistance in Gastric Cancer via Activating the PI3K-Akt-Mtor Pathway. Neoplasma (2021) 68(5):1043–51. doi: 10.4149/neo_2021_210317N356
58. Zhang Y, Li Q, Yu S, Zhu C, Zhang Z, Cao H, et al. Long Non-Coding RNA FAM84B-as Promotes Resistance of Gastric Cancer to Platinum Drugs Through Inhibition of FAM84B Expression. Biochem Biophys Res Commun (2019) 509(3):753–62. doi: 10.1016/j.bbrc.2018.12.177
59. Li Y, Wang L, Xu X, Sun H, Wu L. Lncrna HLA Complex Group 11 Knockdown Alleviates Cisplatin Resistance in Gastric Cancer by Targeting the Mir-144-3p/UBE2D1 Axis. Cancer Manag Res (2021) 13:7543–57. doi: 10.2147/CMAR.S329846
60. Wu Q, Ma J, Wei J, Meng W, Wang Y, Shi M. FOXD1-AS1 Regulates FOXD1 Translation and Promotes Gastric Cancer Progression and Chemoresistance by Activating the PI3K/AKT/Mtor Pathway. Mol Oncol (2021) 15(1):299–316. doi: 10.1002/1878-0261.12728
61. Zhang X, Bo P, Liu L, Zhang X, Li J. Overexpression of Long Non-Coding RNA GHET1 Promotes the Development of Multidrug Resistance in Gastric Cancer Cells. BioMed Pharmacother (2017) 92:580–5. doi: 10.1016/j.biopha.2017.04.111
62. Liang L, Kang H, Jia J. HCP5 Contributes to Cisplatin Resistance in Gastric Cancer Through Mir-128/HMGA2 Axis. Cell Cycle (2021) 20(11):1080–90. doi: 10.1080/15384101.2021.1924948
63. Fu T, Ji K, Jin L, Zhang J, Wu X, Ji X, et al. ASB16-AS1 Up-Regulated and Phosphorylated TRIM37 to Activate NF-Kappab Pathway and Promote Proliferation, Stemness, and Cisplatin Resistance of Gastric Cancer. Gastric Cancer (2021) 24(1):45–59. doi: 10.1007/s10120-020-01096-y
64. Zhang Y, Song X, Wang X, Hu J, Jiang L. Silencing of Lncrna HULC Enhances Chemotherapy Induced Apoptosis in Human Gastric Cancer. J Med Biochem (2016) 35(2):137–43. doi: 10.1515/jomb-2015-0016
65. Liu Y, Cao J, Pu YS, Ma Y, Wu M, Wang JH. RP11-874J12.4, a Novel Lncrna, Confers Chemoresistance in Human Gastric Cancer Cells by Sponging Mir-3972 and Upregulating SSR2 Expression. Am J Transl Res (2021) 13(6):5892–910.
66. Sun B, Han Y, Cai H, Huang H, Xuan Y. Long Non-Coding RNA SNHG3, Induced by IL-6/STAT3 Transactivation, Promotes Stem Cell-Like Properties of Gastric Cancer Cells by Regulating the Mir-3619-5p/ARL2 Axis. Cell Oncol (Dordr) (2021) 44(1):179–92. doi: 10.1007/s13402-020-00560-2
67. Tong KH, Qian J. Long Non-Coding RNA FGD5-AS1 Is an Inducer of Cisplatin Chemoresistance in Gastric Cancer Cells by Sponging Mir-195. J Biol Regul Homeost Agents (2021) 35(2):819–26. doi: 10.23812/20-667-L
68. Xin L, Zhou Q, Yuan YW, Zhou LQ, Liu L, Li SH, et al. Metase/Lncrna HULC/Foxm1 Reduced Cisplatin Resistance in Gastric Cancer by Suppressing Autophagy. J Cancer Res Clin Oncol (2019) 145(10):2507–17. doi: 10.1007/s00432-019-03015-w
69. Wang Z, Wang Q, Xu G, Meng N, Huang X, Jiang Z, et al. The Long Noncoding RNA CRAL Reverses Cisplatin Resistance via the Mir-505/CYLD/AKT Axis in Human Gastric Cancer Cells. RNA Biol (2020) 17(11):1576–89. doi: 10.1080/15476286.2019.1709296
70. Li Y, Lv S, Ning H, Li K, Zhou X, Xv H, et al. Down-Regulation of CASC2 Contributes to Cisplatin Resistance in Gastric Cancer by Sponging Mir-19a. BioMed Pharmacother (2018) 108:1775–82. doi: 10.1016/j.biopha.2018.09.181
71. Zhang Z, Li M, Zhang Z. Lncrna MALAT1 Modulates Oxaliplatin Resistance of Gastric Cancer via Sponging Mir-22-3p. Onco Targets Ther (2020) 13:1343–54. doi: 10.2147/OTT.S196619
72. Wu X, Zheng Y, Han B, Dong X. Long Noncoding RNA BLACAT1 Modulates ABCB1 to Promote Oxaliplatin Resistance of Gastric Cancer via Sponging Mir-361. BioMed Pharmacother (2018) 99:832–8. doi: 10.1016/j.biopha.2018.01.130
73. Song W, Qian Y, Zhang MH, Wang H, Wen X, Yang XZ, et al. The Long Non-Coding RNA DDX11-AS1 Facilitates Cell Progression and Oxaliplatin Resistance via Regulating Mir-326/IRS1 Axis in Gastric Cancer. Eur Rev Med Pharmacol Sci (2020) 24(6):3049–61. doi: 10.26355/eurrev_202003_20669
74. Lin M, Xu M, Xu Z, Weng Z, Lin B, Lan Y, et al. LINC00200 Contributes to the Chemoresistance to Oxaliplatin of Gastric Cancer Cells via Regulating E2F1/RAD51 Axis. Hum Cell (2021) 34(4):1163–73. doi: 10.1007/s13577-021-00523-1
75. Hu Y, Su Y, Lei X, Zhao H, Wang L, Xu T, et al. LINC00641/Mir-582-5p Mediate Oxaliplatin Resistance by Activating Autophagy in Gastric Adenocarcinoma. Sci Rep (2020) 10(1):14981. doi: 10.1038/s41598-020-70913-2
76. Wang J, Sun Y, Zhang X, Cai H, Zhang C, Qu H, et al. Oxidative Stress Activates NORAD Expression by H3k27ac and Promotes Oxaliplatin Resistance in Gastric Cancer by Enhancing Autophagy Flux via Targeting the Mir-433-3p. Cell Death Dis (2021) 12(1):90. doi: 10.1038/s41419-020-03368-y
77. Li Y, Peng C, Fang C, Huang K. Upregulation of Nuclear-Enriched Abundant Transcript 1 Confers Oxaliplatin Resistance to Gastric Cancer. Cell Biol Int (2020) 44(2):446–55. doi: 10.1002/cbin.11245
78. Wang S, Chen W, Yu H, Song Z, Li Q, Shen X, et al. Lncrna ROR Promotes Gastric Cancer Drug Resistance. Cancer Control (2020) 27(1):1073274820904694. doi: 10.1177/1073274820904694
79. Shang C, Sun L, Zhang J, Zhao B, Chen X, Xu H, et al. Silence of Cancer Susceptibility Candidate 9 Inhibits Gastric Cancer and Reverses Chemoresistance. Oncotarget (2017) 8(9):15393–8. doi: 10.18632/oncotarget.14871
80. Jia J, Zhan D, Li J, Li Z, Li H, Qian J. The Contrary Functions of Lncrna HOTAIR/Mir-17-5p/PTEN Axis and Shenqifuzheng Injection on Chemosensitivity of Gastric Cancer Cells. J Cell Mol Med (2019) 23(1):656–69. doi: 10.1111/jcmm.13970
81. Shang C, Guo Y, Zhang J, Huang B. Silence of Long Noncoding RNA UCA1 Inhibits Malignant Proliferation and Chemotherapy Resistance to Adriamycin in Gastric Cancer. Cancer Chemother Pharmacol (2016) 77(5):1061–7. doi: 10.1007/s00280-016-3029-3
82. Li L, Dong Z, Shi P, Tan L, Xu J, Huang P, et al. Bruceine D Inhibits Cell Proliferation Through Downregulating LINC01667/Microrna-138-5p/Cyclin E1 Axis in Gastric Cancer. Front Pharmacol (2020) 11:584960. doi: 10.3389/fphar.2020.584960
83. Zhang J, Zhao B, Chen X, Wang Z, Xu H, Huang B. Silence of Long Noncoding RNA NEAT1 Inhibits Malignant Biological Behaviors and Chemotherapy Resistance in Gastric Cancer. Pathol Oncol Res (2018) 24(1):109–13. doi: 10.1007/s12253-017-0233-3
84. Zhou Z, Lin Z, He Y, Pang X, Wang Y, Ponnusamy M, et al. The Long Noncoding RNA D63785 Regulates Chemotherapy Sensitivity in Human Gastric Cancer by Targeting Mir-422a. Mol Ther Nucleic Acids (2018) 12:405–19. doi: 10.1016/j.omtn.2018.05.024
85. Wang Y, Zhang D, Wu K, Zhao Q, Nie Y, Fan D. Long Noncoding RNA MRUL Promotes ABCB1 Expression in Multidrug-Resistant Gastric Cancer Cell Sublines. Mol Cell Biol (2014) 34(17):3182–93. doi: 10.1128/MCB.01580-13
86. Zhang N, Wang AY, Wang XK, Sun XM, Xue HZ. GAS5 Is Downregulated in Gastric Cancer Cells by Promoter Hypermethylation and Regulates Adriamycin Sensitivity. Eur Rev Med Pharmacol Sci (2016) 20(15):3199–205.
87. Xu W, He L, Li Y, Tan Y, Zhang F, Xu H. Silencing of Lncrna ZFAS1 Inhibits Malignancies by Blocking Wnt/Beta-Catenin Signaling in Gastric Cancer Cells. Biosci Biotechnol Biochem (2018) 82(3):456–65. doi: 10.1080/09168451.2018.1431518
88. Wang H, Qin R, Guan A, Yao Y, Huang Y, Jia H, et al. HOTAIR Enhanced Paclitaxel and Doxorubicin Resistance in Gastric Cancer Cells Partly Through Inhibiting Mir-217 Expression. J Cell Biochem (2018) 119(9):7226–34. doi: 10.1002/jcb.26901
89. Ding J, Li D, Gong M, Wang J, Huang X, Wu T, et al. Expression and Clinical Significance of the Long Non-Coding RNA PVT1 in Human Gastric Cancer. Onco Targets Ther (2014) 7:1625–30. doi: 10.2147/OTT.S68854
90. More LA, Lane S, Asnani A. 5-FU Cardiotoxicity: Vasospasm, Myocarditis, and Sudden Death. Curr Cardiol Rep (2021) 23(3):17. doi: 10.1007/s11886-021-01441-2
91. Longley DB, Harkin DP, Johnston PG. 5-Fluorouracil: Mechanisms of Action and Clinical Strategies. Nat Rev Cancer (2003) 3(5):330–8. doi: 10.1038/nrc1074
92. Sethy C, Kundu CN. 5-Fluorouracil (5-FU) Resistance and the New Strategy to Enhance the Sensitivity Against Cancer: Implication of DNA Repair Inhibition. BioMed Pharmacother (2021) 137:111285. doi: 10.1016/j.biopha.2021.111285
93. He W, Liang B, Wang C, Li S, Zhao Y, Huang Q, et al. MSC-Regulated Lncrna MACC1-AS1 Promotes Stemness and Chemoresistance Through Fatty Acid Oxidation in Gastric Cancer. Oncogene (2019) 38(23):4637–54. doi: 10.1038/s41388-019-0747-0
94. Dilruba S, Kalayda GV. Platinum-Based Drugs: Past, Present and Future. Cancer Chemother Pharmacol (2016) 77(6):1103–24. doi: 10.1007/s00280-016-2976-z
95. Todd RC, Lippard SJ. Inhibition of Transcription by Platinum Antitumor Compounds. Metallomics (2009) 1(4):280–91. doi: 10.1039/b907567d
96. Raei N, Safaralizadeh R, Hesseinpourfeizi M, Yazdanbod A, Pourfarzi F, Latifi-Navid S. Crosstalk Between Lncrnas and Mirnas in Gastrointestinal Cancer Drug Resistance. Life Sci (2021) 284:119933. doi: 10.1016/j.lfs.2021.119933
97. Zhan P, Shu X, Chen M, Sun L, Yu L, Liu J, et al. Mir-98-5p Inhibits Gastric Cancer Cell Stemness and Chemoresistance by Targeting Branched-Chain Aminotransferases 1. Life Sci (2021) 276:119405. doi: 10.1016/j.lfs.2021.119405
98. Rivankar S. An Overview of Doxorubicin Formulations in Cancer Therapy. J Cancer Res Ther (2014) 10(4):853–8. doi: 10.4103/0973-1482.139267
99. Wu Q, Wang H, Liu L, Zhu K, Yu W, Guo J. Hsa_Circ_0001546 Acts as a Mirna-421 Sponge to Inhibit the Chemoresistance of Gastric Cancer Cells via ATM/Chk2/P53-Dependent Pathway. Biochem Biophys Res Commun (2020) 521(2):303–9. doi: 10.1016/j.bbrc.2019.10.117
100. Zhu L, Chen L. Progress in Research on Paclitaxel and Tumor Immunotherapy. Cell Mol Biol Lett (2019) 24:40. doi: 10.1186/s11658-019-0164-y
101. Ojeda-Lopez MA, Needleman DJ, Song C, Ginsburg A, Kohl PA, Li Y, et al. Transformation of Taxol-Stabilized Microtubules Into Inverted Tubulin Tubules Triggered by a Tubulin Conformation Switch. Nat Mater (2014) 13(2):195–203. doi: 10.1038/nmat3858
102. Abu Samaan TM, Samec M, Liskova A, Kubatka P, Busselberg D. Paclitaxel's Mechanistic and Clinical Effects on Breast Cancer. Biomolecules (2019) 9(12):789. doi: 10.3390/biom9120789
103. YiRen H, YingCong Y, Sunwu Y, Keqin L, Xiaochun T, Senrui C, et al. Long Noncoding RNA MALAT1 Regulates Autophagy Associated Chemoresistance via Mir-23b-3p Sequestration in Gastric Cancer. Mol Cancer (2017) 16(1):174. doi: 10.1186/s12943-017-0743-3
104. Said R, Tsimberidou AM. Pharmacokinetic Evaluation of Vincristine for the Treatment of Lymphoid Malignancies. Expert Opin Drug Metab Toxicol (2014) 10(3):483–94. doi: 10.1517/17425255.2014.885016
105. Wang M, Shi J, Jiang H, Xu K, Huang Z. Circ_0014130 Participates in the Proliferation and Apoptosis of Nonsmall Cell Lung Cancer Cells via the Mir-142-5p/IGF-1 Axis. Cancer Biother Radiopharm (2020) 35(3):233–40. doi: 10.1089/cbr.2019.2965
106. Skubnik J, Pavlickova VS, Ruml T, Rimpelova S. Vincristine in Combination Therapy of Cancer: Emerging Trends in Clinics. Biol (Basel) (2021) 10(9):849. doi: 10.3390/biology10090849
107. Cheng C, Qin Y, Zhi Q, Wang J, Qin C. Knockdown of Long Non-Coding RNA HOTAIR Inhibits Cisplatin Resistance of Gastric Cancer Cells Through Inhibiting the PI3K/Akt and Wnt/Beta-Catenin Signaling Pathways by Up-Regulating Mir-34a. Int J Biol Macromol (2018) 107(Pt B):2620–9. doi: 10.1016/j.ijbiomac.2017.10.154
108. Zhang Y, Jia DD, Zhang YF, Cheng MD, Zhu WX, Li PF, et al. The Emerging Function and Clinical Significance of Circrnas in Thyroid Cancer and Autoimmune Thyroid Diseases. Int J Biol Sci (2021) 17(7):1731–41. doi: 10.7150/ijbs.55381
109. Kumar M, Bansal N. Implications of Phosphoinositide 3-Kinase-Akt (PI3K-Akt) Pathway in the Pathogenesis of Alzheimer's Disease. Mol Neurobiol (2021). doi: 10.1007/s12035-021-02611-7
110. Ao X, Ding W, Zhang Y, Ding D, Liu Y. TCF21: A Critical Transcription Factor in Health and Cancer. J Mol Med (Berl) (2020) 98(8):1055–68. doi: 10.1007/s00109-020-01934-7
111. Liu Y, Chen DD, Xing YH, Ge N, Zhang Y, Liu J, et al. A New Oxovanadium Complex Enhances Renal Function by Improving Insulin Signaling Pathway in Diabetic Mice. J Diabetes Complications (2014) 28(3):265–72. doi: 10.1016/j.jdiacomp.2014.02.001
112. Ghafouri-Fard S, Abak A, Shoorei H, Mohaqiq M, Majidpoor J, Sayad A, et al. Regulatory Role of Micrornas on PTEN Signaling. BioMed Pharmacother (2021) 133:110986. doi: 10.1016/j.biopha.2020.110986
113. Wen ZJ, Xin H, Wang YC, Liu HW, Gao YY, Zhang YF. Emerging Roles of Circrnas in the Pathological Process of Myocardial Infarction. Mol Ther Nucleic Acids (2021) 26:828–48. doi: 10.1016/j.omtn.2021.10.002
114. Bugter JM, Fenderico N, Maurice MM. Mutations and Mechanisms of WNT Pathway Tumour Suppressors in Cancer. Nat Rev Cancer (2021) 21(1):5–21. doi: 10.1038/s41568-020-00307-z
115. Liu Y, Ao X, Yu W, Zhang Y, Wang J. Biogenesis, Functions, and Clinical Implications of Circular RNAs in Non-Small Cell Lung Cancer. Mol Ther - Nucleic Acids (2022) 27:50–72. doi: 10.1016/j.omtn.2021.11.013
116. Ghandadi M, Valadan R, Mohammadi H, Akhtari J, Khodashenas S, Ashari S. Wnt-Beta-Catenin Signaling Pathway, the Achilles' Heels of Cancer Multidrug Resistance. Curr Pharm Des (2019) 25(39):4192–207. doi: 10.2174/1381612825666191112142943
117. Chi HC, Tsai CY, Wang CS, Yang HY, Lo CH, Wang WJ, et al. DOCK6 Promotes Chemo- and Radioresistance of Gastric Cancer by Modulating WNT/Beta-Catenin Signaling and Cancer Stem Cell Traits. Oncogene (2020) 39(37):5933–49. doi: 10.1038/s41388-020-01390-0
118. Zhang S, Wang L, Gao Y, Fan Y, Zhang G, Zhang Y. Molecular Mechanism of 73HOXC-AS1-Activated Wntbeta-Catenin Signaling and Eif4aiii in Promoting Progression of Gastric Cancer. BioMed Res Int (2021) 2021:8814843. doi: 10.1155/2021/8814843
119. Lu J, Xu Y, Xie W, Tang Y, Zhang H, Wang B, et al. Long Noncoding RNA DLGAP1-AS2 Facilitates Wnt1 Transcription Through Physically Interacting With Six3 and Drives the Malignancy of Gastric Cancer. Cell Death Discovery (2021) 7(1):255. doi: 10.1038/s41420-021-00649-z
120. Liu Y, Ao X, Jia Z, Bai XY, Xu Z, Hu G, et al. FOXK2 Transcription Factor Suppresses Eralpha-Positive Breast Cancer Cell Growth Through Down-Regulating the Stability of Eralpha via Mechanism Involving BRCA1/BARD1. Sci Rep (2015) 5:8796. doi: 10.1038/srep08796
121. Magnelli L, Schiavone N, Staderini F, Biagioni A, Papucci L. MAP Kinases Pathways in Gastric Cancer. Int J Mol Sci (2020) 21(8):2893. doi: 10.3390/ijms21082893
122. Liu Y, Ao X, Ding W, Ponnusamy M, Wu W, Hao X, et al. Critical Role of FOXO3a in Carcinogenesis. Mol Cancer (2018) 17(1):104. doi: 10.1186/s12943-018-0856-3
123. Carneiro BA, El-Deiry WS. Targeting Apoptosis in Cancer Therapy. Nat Rev Clin Oncol (2020) 17(7):395–417. doi: 10.1038/s41571-020-0341-y
124. Liu X, Wang Q, Song S, Feng M, Wang X, Li L, et al. Epithelial Splicing Regulatory Protein 1 Is Overexpressed in Breast Cancer and Predicts Poor Prognosis for Breast Cancer Patients. Med Sci Monit (2021) 27:e931102. doi: 10.12659/MSM.931102
125. Li Z, Lu M, Zhou Y, Xu L, Jiang Y, Liu Y, et al. Role of Long Non-Coding RNAs in the Chemoresistance of Gastric Cancer: A Systematic Review. Onco Targets Ther (2021) 14:503–18. doi: 10.2147/OTT.S294378
126. Hafezi S, Rahmani M. Targeting BCL-2 in Cancer: Advances, Challenges, and Perspectives. Cancers (Basel) (2021) 13(6):1292. doi: 10.3390/cancers13061292
127. Marin W, Marin D, Ao X, Liu Y. Mitochondria as a Therapeutic Target for Cardiac Ischemiareperfusion Injury (Review). Int J Mol Med (2021) 47(2):485–99. doi: 10.3892/ijmm.2020.4823
128. Zong L, Hattori N, Yasukawa Y, Kimura K, Mori A, Seto Y, et al. LINC00162 Confers Sensitivity to 5-Aza-2'-Deoxycytidine via Modulation of an RNA Splicing Protein, HNRNPH1. Oncogene (2019) 38(26):5281–93. doi: 10.1038/s41388-019-0792-8
129. Cheng H, Sharen G, Wang Z, Zhou J. Lncrna UCA1 Enhances Cisplatin Resistance by Regulating CYP1B1-Mediated Apoptosis via Mir-513a-3p in Human Gastric Cancer. Cancer Manag Res (2021) 13:367–77. doi: 10.2147/CMAR.S277399
130. Du B, Shim JS. Targeting Epithelial-Mesenchymal Transition (EMT) to Overcome Drug Resistance in Cancer. Molecules (2016) 21(7):965. doi: 10.3390/molecules21070965
131. Liu K, Gao L, Ma X, Huang JJ, Chen J, Zeng L, et al. Long Non-Coding RNAs Regulate Drug Resistance in Cancer. Mol Cancer (2020) 19(1):54. doi: 10.1186/s12943-020-01162-0
132. Rendic SP. Metabolism and Interactions of Ivermectin With Human Cytochrome P450 Enzymes and Drug Transporters, Possible Adverse and Toxic Effects. Arch Toxicol (2021) 95(5):1535–46. doi: 10.1007/s00204-021-03025-z
133. Robey RW, Pluchino KM, Hall MD, Fojo AT, Bates SE, Gottesman MM. Revisiting the Role of ABC Transporters in Multidrug-Resistant Cancer. Nat Rev Cancer (2018) 18(7):452–64. doi: 10.1038/s41568-018-0005-8
134. Liu Y, Ding W, Ge H, Ponnusamy M, Wang Q, Hao X, et al. FOXK Transcription Factors: Regulation and Critical Role in Cancer. Cancer Lett (2019) 458:1–12. doi: 10.1016/j.canlet.2019.05.030
135. Liu X, Liu Y, Wang Q, Song S, Feng L, Shi C. The Alterations and Potential Roles of Mcms in Breast Cancer. J Oncol (2021) 2021:7928937. doi: 10.1155/2021/7928937
136. Marin JJG, Perez-Silva L, Macias RIR, Asensio M, Peleteiro-Vigil A, Sanchez-Martin A, et al. Molecular Bases of Mechanisms Accounting for Drug Resistance in Gastric Adenocarcinoma. Cancers (Basel) (2020) 12(8):2116. doi: 10.3390/cancers12082116
137. Chen D, Liu L, Wang K, Yu H, Wang Y, Liu J, et al. The Role of MALAT-1 in the Invasion and Metastasis of Gastric Cancer. Scand J Gastroenterol (2017) 52(6-7):790–6. doi: 10.1080/00365521.2017.1280531
138. Xu HF, Shi DM, Zhu XQ. A Study of Effect of Lncrna MVIH on Sensitivity of Gastric Cancer Cells to Gemcitabine. Eur Rev Med Pharmacol Sci (2019) 23(21):9651–9. doi: 10.26355/eurrev_201911_19459
139. Zhang Y, Chang L, Wu Q, Zuo F. Long non-Coding RNA NEAT1 Increases the Aggressiveness of Gastric Cancer by Regulating the Microrna-142-5p/JAG1 Axis. Exp Ther Med (2021) 22(2):862. doi: 10.3892/etm.2021.10294
140. Zhang Z, Chen J, Zhu Z, Zhu Z, Liao X, Wu J, et al. CRISPR-Cas13-Mediated Knockdown of Lncrna-GACAT3 Inhibited Cell Proliferation and Motility, and Induced Apoptosis by Increasing P21, Bax, and E-Cadherin Expression in Bladder Cancer. Front Mol Biosci (2020) 7:627774. doi: 10.3389/fmolb.2020.627774
141. Huang T, Song X, Xu D, Tiek D, Goenka A, Wu B, et al. Stem Cell Programs in Cancer Initiation, Progression, and Therapy Resistance. Theranostics (2020) 10(19):8721–43. doi: 10.7150/thno.41648
142. Liu Y. Targeting the Non-Canonical AKT-FOXO3a Axis: A Potential Therapeutic Strategy for Oral Squamous Cell Carcinoma. EBioMedicine (2019) 49:6–8. doi: 10.1016/j.ebiom.2019.10.020
143. Wang S, Liu F, Deng J, Cai X, Han J, Liu Q. Long Noncoding RNA ROR Regulates Proliferation, Invasion, and Stemness of Gastric Cancer Stem Cell. Cell Reprogram (2016) 18(5):319–26. doi: 10.1089/cell.2016.0001
144. Xiao Y, Pan J, Geng Q, Wang G. Lncrna MALAT1 Increases the Stemness of Gastric Cancer Cells via Enhancing SOX2 Mrna Stability. FEBS Open Bio (2019) 9(7):1212–22. doi: 10.1002/2211-5463.12649
145. Song H, Xu Y, Shi L, Xu T, Fan R, Cao M, et al. Lncrna THOR Increases the Stemness of Gastric Cancer Cells via Enhancing SOX9 Mrna Stability. BioMed Pharmacother (2018) 108:338–46. doi: 10.1016/j.biopha.2018.09.057
146. Gao XQ, Liu CY, Zhang YH, Wang YH, Zhou LY, Li XM, et al. The Circrna CNEACR Regulates Necroptosis of Cardiomyocytes Through Foxa2 Suppression. Cell Death Differ (2021). doi: 10.1038/s41418-021-00872-2
147. Wu H, Liu B, Chen Z, Li G, Zhang Z. MSC-Induced Lncrna HCP5 Drove Fatty Acid Oxidation Through Mir-3619-5p/AMPK/PGC1alpha/CEBPB Axis to Promote Stemness and Chemo-Resistance of Gastric Cancer. Cell Death Dis (2020) 11(4):233. doi: 10.1038/s41419-020-2426-z
148. Yamazaki T, Bravo-San Pedro JM, Galluzzi L, Kroemer G, Pietrocola F. Autophagy in the Cancer-Immunity Dialogue. Adv Drug Deliv Rev (2021) 169:40–50. doi: 10.1016/j.addr.2020.12.003
149. Lu H, Shi C, Liu X, Liang C, Yang C, Wan X, et al. Identification of ZG16B as a Prognostic Biomarker in Breast Cancer. Open Med (Wars) (2021) 16(1):1–13. doi: 10.1515/med-2021-0004
150. Onorati AV, Dyczynski M, Ojha R, Amaravadi RK. Targeting Autophagy in Cancer. Cancer (2018) 124(16):3307–18. doi: 10.1002/cncr.31335
151. Thanh Huong P, Gurshaney S, Thanh Binh N, Gia Pham A, Hoang Nguyen H, Thanh Nguyen X, et al. Emerging Role of Circulating Tumor Cells in Gastric Cancer. Cancers (Basel) (2020) 12(3):695. doi: 10.3390/cancers12030695
152. Ao X, Ding W, Ge H, Zhang Y, Ding D, Liu Y. PBX1 Is a Valuable Prognostic Biomarker for Patients With Breast Cancer. Exp Ther Med (2020) 20(1):385–94. doi: 10.3892/etm.2020.8705
153. Martinez-Barriocanal A, Arango D, Dopeso H. PVT1 Long Non-Coding RNA in Gastrointestinal Cancer. Front Oncol (2020) 10:38. doi: 10.3389/fonc.2020.00038
154. Zhou DD, Liu XF, Lu CW, Pant OP, Liu XD. Long Non-Coding RNA PVT1: Emerging Biomarker in Digestive System Cancer. Cell Prolif (2017) 50(6):e12398. doi: 10.1111/cpr.12398
155. Li X, Du Y, Wang Y. The Value of Lncrna SNHG5 as a Marker for the Diagnosis and Prognosis of Gastric Cancer. Am J Transl Res (2021) 13(5):5420–7.
156. Zhang XW, Bu P, Liu L, Zhang XZ, Li J. Overexpression of Long Non-Coding RNA PVT1 in Gastric Cancer Cells Promotes the Development of Multidrug Resistance. Biochem Biophys Res Commun (2015) 462(3):227–32. doi: 10.1016/j.bbrc.2015.04.121
157. Yue B, Liu C, Sun H, Liu M, Song C, Cui R, et al. A Positive Feed-Forward Loop Between Lncrna-CYTOR and Wnt/Beta-Catenin Signaling Promotes Metastasis of Colon Cancer. Mol Ther (2018) 26(5):1287–98. doi: 10.1016/j.ymthe.2018.02.024
158. Chen JF, Wu P, Xia R, Yang J, Huo XY, Gu DY, et al. STAT3-Induced Lncrna HAGLROS Overexpression Contributes to the Malignant Progression of Gastric Cancer Cells via Mtor Signal-Mediated Inhibition of Autophagy. Mol Cancer (2018) 17(1):6. doi: 10.1186/s12943-017-0756-y
159. Fattahi S, Kosari-Monfared M, Golpour M, Emami Z, Ghasemiyan M, Nouri M, et al. Lncrnas as Potential Diagnostic and Prognostic Biomarkers in Gastric Cancer: A Novel Approach to Personalized Medicine. J Cell Physiol (2020) 235(4):3189–206. doi: 10.1002/jcp.29260
160. Blay JY, Kang YK, Nishida T, von Mehren M. Gastrointestinal Stromal Tumours. Nat Rev Dis Primers (2021) 7(1):22. doi: 10.1038/s41572-021-00254-5
161. Xu Y, Zhang P, Zhang K, Huang C. The Application of CA72-4 in the Diagnosis, Prognosis, and Treatment of Gastric Cancer. Biochim Biophys Acta Rev Cancer (2021) 1876(2):188634. doi: 10.1016/j.bbcan.2021.188634
162. Liu H, Wan J, Chu J. Long Non-Coding RNAs and Endometrial Cancer. BioMed Pharmacother (2019) 119:109396. doi: 10.1016/j.biopha.2019.109396
Keywords: long non-coding RNA, gastric cancer, drug resistance, biomarker, therapeutic target
Citation: Liu Y, Ao X, Wang Y, Li X and Wang J (2022) Long Non-Coding RNA in Gastric Cancer: Mechanisms and Clinical Implications for Drug Resistance. Front. Oncol. 12:841411. doi: 10.3389/fonc.2022.841411
Received: 22 December 2021; Accepted: 10 January 2022;
Published: 28 January 2022.
Edited by:
Mohamed Hassan, Institut National de la Santé et de la Recherche Médicale (INSERM), FranceReviewed by:
Milad Ashrafizadeh, Sabancı University, TurkeyHaleh Akhavan-Niaki, Babol University of Medical Sciences, Iran
Copyright © 2022 Liu, Ao, Wang, Li and Wang. This is an open-access article distributed under the terms of the Creative Commons Attribution License (CC BY). The use, distribution or reproduction in other forums is permitted, provided the original author(s) and the copyright owner(s) are credited and that the original publication in this journal is cited, in accordance with accepted academic practice. No use, distribution or reproduction is permitted which does not comply with these terms.
*Correspondence: Ying Liu, bGl1eWluZ19oZXJvQDE2My5jb20=