- 1Department of Medical Research, Chi Mei Medical Center, Tainan, Taiwan
- 2Department of Nursing, Min-Hwei Junior College of Health Care Management, Tainan, Taiwan
- 3Department of Obstetrics and Gynecology, National Cheng Kung University Hospital, College of Medicine, National Cheng Kung University, Tainan, Taiwan
The extracellular matrix (ECM) plays an important role in the progression of cancer. Collagen is the most abundant component in ECM, and is involved in the biological formation of cancer. Although type XI collagen is a minor fibrillar collagen, collagen XI alpha 1 chain (COL11A1) expression has been found to be upregulated in a variety of human cancers including colorectal, esophagus, glioma, gastric, head and neck, lung, ovarian, pancreatic, salivary gland, and renal cancers. High levels of COL11A1 usually predict poor prognosis, owing to its association with angiogenesis, invasion, and drug resistance in cancer. However, little is known about the specific mechanism through which COL11A1 regulates tumor progression. Here, we have organized and summarized recent developments regarding the interactions between COL11A1 and intracellular signaling pathways and selected therapeutic agents targeting COL11A1, as these indicate its potential as a target for treatment of cancers, especially epithelial ovarian cancer.
Introduction
Cancer has become the third leading cause of death worldwide, with approximately 1,918,030 new cancer cases and 609,360 cancer deaths projected to occur in the United States in 2022 (1). Although clinical classification, staging, and treatment guidelines for various cancers are constantly being updated, improvements in the long-term prognosis of cancer patients remain difficult and therefore it is of extreme significance to explore effective cancer treatment strategies. Previously, researchers focused on the biological mechanisms in tumor cells, while ignoring the relationship between cancer cells and the surrounding tissues. Recent studies have focused to a greater extent on the tumor microenvironment, proving that it is crucial in the progression of cancer.
The extracellular matrix (ECM) is a scaffold of fibrillar proteins, accessory proteins, and molecules, and provides structural and biochemical support for cells. The ECM consists of a basement membrane (located at the basal aspect of epithelial or endothelial cells in normal tissues) and the interstitial (stromal) ECM. In most tissues, the basement membrane consists largely of collagen IV, together with laminin, fibronectin, and several types of proteoglycans (2). The main role of the basement membrane is to provide a physical barrier between the epithelial cells and the connective tissue (stroma) of the organ, whilst still allowing the diffusion of gases and transport of signaling molecules. The interstitial ECM, mainly produced by mesenchymal cells, consists primarily of collagens I and III, fibronectin, and proteoglycans. It regulates cell adhesion, cell migration, angiogenesis, and tissue repair (3). In cancer, rupture of the basement membrane permits epithelial cells to undergo epithelial–mesenchymal transition (EMT), migrate into the surrounding stroma, and invade through the interstitial ECM. Epithelial cells that have undergone EMT can cause activation of stromal cells to yield pro-tumorigenic stromal cells, which can in turn remodel the ECM to create a tumor-permissive environment (4). The ECM is indispensable for cell proliferation, differentiation, and maintenance of tissue homeostasis (5), and its influence on cancer cell plasticity is modulated by a variety of cell types that reside within the tumor stroma. Under the influence of systemic regulators as well as cancer cells, these stromal cells produce not only tumor ECM, which qualitatively and quantitatively differs from a normal ECM, but also an array of cytokines and other secreted and membrane-bound factors that influence cancer cell plasticity. Cancer cells, cancer-associated fibroblasts (CAFs), immune cells, adipocytes, and other stromal cells act on the ECM and as a result promote angiogenesis, decrease cell adhesion, induce loss of tissue polarity, and increase EMT. This increases the invasion and distant metastasis of tumor cells and their resistance to chemotherapy drugs; these are generally the leading causes of cancer mortality (6–9).
Mechanisms Underlying COL11A1 Involvement in Cancer Progression
Collagen type XI alpha 1 (COL11A1) belongs to the collagen family, a major component of the interstitial ECM. Collagen XI is a right-handed triple helix complex consisting of three different α-chains, namely alpha 1, alpha 2, and alpha 3. Although type XI collagen is a minor fibrillar collagen, it is essential for normal tissue integrity and function. The lack of type XI collagen leads to abnormal thickening of the tissue in cartilage (10, 11); animals have been reported to develop chondrodysplasia where the cartilage was devoid of fibrils in two separate mouse models in which collagen XI expression was abrogated (12). These data demonstrate the significant role of collagen XI in nucleation of assembly of short, small-diameter protofibrils. The collagen alpha chain consists of 662–3152 amino acids. COL11A1 and COL11A2 encode the alpha 1 chain and alpha 2 chain, respectively; COL2A1 encodes the alpha 3 chain (10). COL11A1 therefore requires two other alpha chains (COL11A2, COL2A1) to form collagen fibers. COL11A1 expression is absent in benign pathological conditions involving desmoplasia such as hyperplasia, fibrosis, cirrhosis, pancreatitis and inflammatory bowel disease or premalignant lesions (10–13). In contrast, COL11A1 has been found to be upregulated in a variety of cancers including breast, colorectal, esophagus, glioma, gastric, head and neck, lung, ovarian, pancreatic, salivary gland, and renal cancers, and it can be used as a tumor marker to predict the prognosis of cancer (Table 1) (14–46). In these cancers, the expression of COL11A1 positively correlates with tumor progression and lymph node metastasis.
Mechanisms Underlying COL11A1 Involvement in CAF Activation
COL11A1 expression has been detected in tumor cells as well as in tumor-associated stromal cells. It has been shown that CAFs, which express and secrete COL11A1 into the ECM, promote the proliferation, angiogenesis, invasion, and drug resistance of cancer cells (10, 17, 28, 47–51). COL11A1 overexpression has only been observed in desmoplastic areas of the tumors, which are composed primarily of CAFs in cancers (28, 29, 48–50). However, such overexpression has not been demonstrated in fibroblasts in inflammatory diseases, making COL11A1 a unique marker for CAFs (10, 48). The malignant transformation of colorectal cancer is associated with the differential expression of COL11A1 in the stroma (49). Navab et al. (52) established CAF primary cultures, matching normal fibroblasts from 15 non–small-cell lung cancer samples, and identified COL11A1 as the gene with the most highly elevated expression in CAFs. Other studies have also recorded COL11A1 overexpression in CAFs of pancreatic cancer (52) and suggested that it is a CAF-specific marker (48, 53). However, several studies have found that cancer cells secrete the COL11A1 they produce into the ECM, to regulate their own biological behavior as well as that of the surrounding cancer cells (34, 54–57). Recently, we (36) investigated the role of COL11A1 in inducing CAF activation and its interaction with cancer cells. The results showed that human ovarian fibroblasts acquire CAF markers and functions, such as enhanced cell migration and contraction abilities and ECM deposition, and express COL11A1 when cocultured with high COL11A1-expressing epithelial ovarian cancer (EOC) cell lines or conditioned medium of coculture. In contrast, coculturing human ovarian fibroblasts with low COL11A1-expressing EOC cells or COL11A1 knockdown in EOC cells abrogated the expression levels of COL11A1 and CAF markers in human ovarian fibroblasts. We also demonstrated that COL11A1 regulated transforming growth factor beta 3 (TGF-β3) levels in EOC cells, thereby promoting CAF activation by activating the nuclear factor kappa-light-chain-enhancer of activated B/insulin-like growth factor binding protein-2 (NF-κB/IGFBP2) axis, which was attenuated by COL11A1 knockdown or pharmacological inhibition of TGF-β3. Notably, elevated p-SP1 expression generated by COL11A1-mediated ERK activation induced p65 translocation into the nucleus, thereby enhancing its binding to the IGFBP2 promoter. TGF-β3 induction by COL11A1 reinforced tumor–fibroblast crosstalk via heightened IL-6 production to stimulate the proliferation and invasion of tumor cells. Furthermore, in vivo findings from mouse xenografts indicated the essential role of COL11A1 in tumor progression and CAF activation, and that anti-TGF-β3 therapy could inhibit tumor growth and reverse CAF activation. These in vitro results were confirmed by clinical findings that high TGF-β3 mRNA levels are associated with extra-pelvic spread, disease progression, and poor progression-free survival (PFS) and overall survival (OS) of ovarian cancer patients.
As mentioned previously, COL11A1 encodes one alpha chain out of three alpha chains of type XI collagen; thus, two other alpha chains (COL11A2, COL2A1) are required to form collagen fibers. Interestingly, our results showed that COL2A1 did not activate the CAF phenotype, suggesting that CAF activation may be specific to COL11A1 (36).
Regulation of COL11A1 in Human Cancer
Multiple studies have proven that COL11A1 participates in cancer progression through various signaling pathways (Table 1). COL11A1, as a component of collagen, is mainly present in the extracellular matrix. The mechanism through which it transmits signals through the outside-in pathway is still unclear. Rada et al. (33) demonstrated that integrin α1β1 and discoid domain receptor 2 (DDR2) acted as the receptors for the binding of COL11A1 on cell membranes, activating intracellular signaling pathways, and thus causing cancer progression.
TGF-β1 has been found to regulate COL11A1 in colorectal, pancreatic, and renal cancers, thereby promoting cancer proliferation and invasion (17, 40, 42). We demonstrated that TGF-β1 directly acts on the NF-YA binding site of the promoter of COL11A1 in ovarian cancer cells, activating COL11A1 and thereby inducing migration and invasion (29). Our results were confirmed by Cheon et al. (30), who demonstrated that the knockdown of COL11A1 reduced the migration and invasion of ovarian cancer cells, which was directly regulated by TGF-β1 signaling. Hida et al. (58) also revealed that NF-YA regulates the promoter region of COL11A1 in rat chondrosarcoma (RCS) as well as mouse pre-chondrocyte ATDC5 cells.
In addition to TGF-β1, several factors have been shown to regulate COL11A1 expression. Fibroblast growth factor-14 (FGF-14) is downregulated in lung adenocarcinoma patient samples (27), and its overexpression in the lung adenocarcinoma cell line A549 results in downregulation of COL11A1 expression. In addition, the glycoprotein microfibril-associated protein 5 (MFAP5) has been found to enhance COL11A1 expression in ovarian fibroblasts (35). Oncostatin M (OSM), an inflammatory cytokine, has also been shown to bind to collagen type XI after being deposited by neutrophils in MDA-MB-231 breast cancer cell-derived matrices in vitro (16). Although this interaction is not known to regulate COL11A1 function, binding, or signaling, OSM has been noted to be immobilized to type XI collagen induced signal transducer and activator of transcription (STAT) signaling in the breast cancer cell line T47D.
Other transcription factors known to regulate COL11A1 expression in the context of cancer include SP1, B-myb, and c/EBPβ. Watanabe et al. (59) showed that Sp1 regulates COL11A1 expression in rodent RCS cells. Jin et al. (26) revealed that B-myb is upregulated in 0 (NSCLC) and B-myb overexpression in H1299 cells leads to COL11A1 overexpression. Matsuo et al. (60) showed that the CCAAT-binding factor CBF/NF-Y, and not c/EBP, positively regulates the transcription of COL11A1 in A204 (a human rhadomyosarcoma cell line) by directly binding to the promoter region of COL11A1. Interestingly, our report (31) demonstrated that anticancer drugs, such as cisplatin and paclitaxel, preferentially induce COL11A1 expression in the cisplatin-resistant ovarian cancer cell line A2780CP70, but not in its cisplatin-sensitive counterpart, the A2780 cell line. Furthermore, knockdown of the transcription factor c/EBPβ attenuated the increase in COL11A1 expression in A2780CP70 cells treated with cisplatin or paclitaxel and phosphoinositide-dependent kinase 1 (PDK1)/Akt signaling activation upregulated c/EBPβ/COL11A1 expression in these cells. These findings suggest that COL11A1 transcription is regulated differentially depending on the cell type.
Some microRNA and long non-coding RNA (lncRNA) are involved in the regulation of COL11A1. miR-139-5p expression is low in breast cancer cells and COL11A1 is predicted to be a target gene of miR-139-5p. Overexpression of miR-139-5p or silencing of COL11A1 could inhibit the proliferation of breast cancer cells and promote apoptosis. Simultaneous overexpression of miR-139-5p and COL11A1 could reverse this effect, indicating that COL11A1 is a downstream target of miR-139-5p (14). Another study has found that microRNA let-7b is a tumor suppressor, and the binding of CDX2 to let-7b can promote the transcription of let-7b and inhibit the expression of COL11A1, thereby reducing the proliferation, invasion, and migration of breast cancer cells (15). Studies on a nude mouse subcutaneous tumor model have also shown that the overexpression of CDX2 and let-7b or the knockdown of COL11A1 could effectively reduce the volume and weight of the tumor (15). Yang et al. (61) demonstrated that the overexpression of microRNA-145 (miR-145) downregulates COL11A1 expression and that of other chondrocyte markers in the mouse mesenchymal stem cell line C3H10T1/2, while suppression of this microRNA enhances COL11A1 expression.
There is also evidence that miR-29 downregulates COL11A1 expression during zebrafish development (62). Although no study has directly shown that miR-29 downregulates COL11A1 in mammalian cells, there are at least two studies that predict the role of miR-29 in the downregulation of COL11A1 in a mammalian cell context (63, 64). In addition, protein-protein interaction (PPI) studies have shown that miR-29 affects the progression of colon cancer by targeting COL11A1 (18).
We (37) identified miR-335 as the candidate miRNAs that may regulate COL11A1 expression through an online database search. Further mechanistic experiments revealed that epithelial ovarian cancer (EOC) cell treatment with miR-335 results in phenotypes that mimic those induced by abrogated COL11A1 expression and suppresses cell proliferation and invasion, in addition to increasing the sensitivity of EOC cells to cisplatin. Conversely, treatment with miR-335 inhibitors prompts cell growth/invasiveness and chemoresistance of EOC cells. miR-335 inhibited COL11A1 transcription, thus reducing the invasiveness and chemoresistance of EOC cells via the Ets-1/MMP3 and Akt/c/EBPβ/PDK1 axes, respectively. Furthermore, miR-335 did not directly regulate PDK1 but increased PDK1 ubiquitination and degradation through COL11A1 inhibition. In vivo findings have shown a significant decrease in the miR-335 expression level in EOC samples compared to that in non-cancerous specimens. Furthermore, patients with low miR335 levels were susceptible to advanced-stage cancer, poor response to chemotherapy, and early relapse. These findings highlighted the importance of miR-335 in downregulating COL11A1-mediated ovarian tumor progression, chemoresistance, and poor survival and suggested the potential application of miR-335 as a therapeutic target (37). Kang et al. (65) demonstrated that COL11A1 promotes esophageal squamous cell carcinoma proliferation and metastasis and is inversely regulated by miR-335-5p.
The lncRNA small nucleolar RNA host gene 12 (SNHG12) is overexpressed in a variety of cancers. Xu et al. (43) found that in the renal cancer cells lines A498 and 786O, the apoptosis of cells increased, and the cell survival rate and cell invasion ability decreased when SNHG12 was knocked out. A luciferase reporter assay indicated that SNHG12 binds to and downregulates miR-200c-5p, thereby increasing COL11A1 expression (43).
Mechanisms of COL11A1 Participating in Chemoresistance
COL11A1 is one of the top genes in the gene signature that predicts an outcome to standard chemotherapy in ovarian and other cancers. Our report indicates that ovarian cancer patients who did not respond to standard platinum-based chemotherapies express elevated levels of COL11A1 (31), where COL11A1 was among the top overexpressed genes. Furthermore, high levels of COL11A1 protein secretion have been linked with resistance to platinum-based therapies in ovarian cancer (66). Recently, high levels of circulating COL11A1 have been identified in patients with non-small cell lung cancer (67) and breast cancer (68), which has been correlated with increased aggressiveness of the disease. It is interesting to note that the expression of COL11A1 is elevated post chemotherapy in several cancer types and can mediate resistance to cisplatin chemotherapy (Table 1). In lung cancer specimens it has been observed that COL11A1 expression is increased in recurrent tumors (24). In lung cancer cell lines, elevated COL11A1 expression also mediates resistance of cancer cells to cisplatin. Similarly, in ovarian cancer specimens, the expression of COL11A1 is the highest in recurrent tumors compared to primary and metastatic tumors, suggesting that COL11A1 promotes tumor recurrence post chemotherapy (28–30). These studies have been successful in reporting that in human ovarian cancer cell lines and xenograft mouse models COL11A1 is not only associated with poor response to cisplatin, but also confers cisplatin resistance though multiple mechanisms.
We found out that c/EBPβ (CCAAT-enhancer-binding protein-beta) has been identified as a transcription factor upregulating COL11A1 expression post chemotherapy (31). COL11A1 promotes the phosphorylation of SP1, which in turn promotes the transcriptional activation of IKKβ, leading to the increased expression of Twist1 induced by NF-κB, thus causing drug resistance in ovarian cancer cells (32). Another study showed that COL11A1 activates Src- phosphoinositide 3-kinase (PI3K)/Akt-NF-kB signaling to induce the expression of three inhibitor apoptosis proteins (IAPs), including XIAP, BIRC2, and BIRC3. Genetic and pharmacological inhibition of XIAP, BIRC2, and BIRC3 is sufficient to restore cisplatin-induced apoptosis in ovarian cancer cells in the presence of COL11A1 in ovarian cancer cells and xenograft mouse models, respectively (33). Nallanthighal et al. (34) identified a novel role of COL11A1 in modulating tumor cell metabolism. COL11A1 signaling in ovarian cancer cell lines makes them more addicted to fatty acid metabolism to drive cisplatin resistance (34). This ability of COL11A1 to alter the metabolic adaptation of cancer cells benefits the cells to survive the harsh environments generated by chemotherapy.
Overexpression and/or activation of the PI3K/Akt survival pathway have been shown to be associated with carcinogenesis in several tissue types (69–73). One of the main downstream effectors of PI3K is the potent oncogenic serine/threonine kinase Akt, also known as protein kinase B (PKB) (74, 75). Akt is activated by phosphorylation of Thr308 by the PDK1 (76, 77) and phosphorylation of Ser473 by the mammalian target of rapamycin complex 2 (mTORC2) (78). Activated Akt functions to regulate several important molecular pathways, including those associated with cell survival, proliferation, and apoptosis. Our report (31) showed that the level of phosphorylated Akt was elevated in chemoresistant ovarian cancer cells and that treatment with an Akt inhibitor (LY294002) enhanced sensitivity to anticancer drugs. These findings are consistent with previous studies showing that Akt is a determinant of cisplatin resistance in chemoresistant ovarian cancer cells (79–85). Another report from us (31) has revealed that COL11A1 could increase phosphorylated Akt in chemoresistant ovarian cancer cells by stabilizing PDK1 protein. Further evidence of these observations is provided by the reduced PDK1 protein expression observed in COL11A1-knockdown cells, the marked increase in PDK1 protein in the presence of the 26S proteasome inhibitor MG132, and the proteasomal degradation of PDK1 observed in the presence of COL11A1 inhibition. We also observed that phosphorylated Akt levels in chemoresistant ovarian cancer cells were increased by COL11A1 via increased binding activity between PDK1 and COL11A1. Collectively, our results demonstrate that the overexpression of COL11A1 leads to chemoresistance, possibly by binding to PDK1-Akt, and subsequently preventing PDK1 degradation. This binding may prevent cisplatin- and paclitaxel-induced PDK1 ubiquitination and degradation in these cells. The results of this study suggest that COL11A1 may be an important determinant of chemoresistance that acts by sequestering PDK1 and preventing its ubiquitination and proteasomal degradation.
Wang et al. (41) demonstrated that COL11A1 phosphorylated AktSer473, promoting proliferation of cancer cells and inhibiting their apoptosis. In pancreatic cancer cells, the COL11A1/Akt axis disrupts the balance between BAX and BCL-2, and inhibits the release of cytochrome C, thereby destroying mitochondrial function and promoting apoptosis escape in turn leading to drug resistance in these cancer cells.
Targeting COL11A1
As COL11A1 tends to accumulate in tumor tissues and promote malignant progression of human cancer, with lower expression levels in normal tissues, targeting COL11A1 has become an attractive strategy for the treatment of various cancers. Although there is no current therapy specifically designed to target COL11A1 in cancer, there are certain drugs that target COL11A1 signal, some of which have been or are currently being tested in clinical trials (44).
Inhibition of TGF-β, a growth factor known to induce COL11A1 expression, has been explored as an anti-cancer therapy in the clinic. Targeting the TGF-β1 signaling pathway can inhibit metastasis and drug resistance in a variety of human cancer models. LY2157299, a small molecule inhibitor of transforming growth factor beta receptor 1 (TβRI), has entered its phase II clinical trial [86]. LY2157299 inhibits the proliferation and invasion of ovarian cancer cells and inhibits tumor growth in nude mouse xenograft models. In addition, LY2157299 has blocked TGF-β1-induced fibroblast activation and inhibited the expression of COL11A1 in tumor stroma (87). This suggests that LY2157299 may preferably target tumor stroma rather than cancer cells when exerting its anticancer effects. The TβRI kinase inhibitor galunisertib has been evaluated in two phase II clinical trials for hepatocellular carcinoma (HCC), and treatment with galunisertib showed improvement in overall survival in both trials (88, 89). Our present study showed that COL11A1 upregulates IGFBP2 transcription by augmenting p65 DNA-binding activity in ovarian cancer cells to constitutively activate the TGF-β3 signaling pathway, thereby promoting CAF activation. These findings signify the intriguing possibility that anti-TGF-β1 therapeutics could be used to target ovarian cancer cells and anti-TGF-β3 therapies could be used to inhibit CAFs in COL11A1-positive tumors (36).
Solanum incanum extract (also known as SR-T100) has been shown to downregulate c/EBPβ and COL11A1 expression, thereby sensitizing melanoma and ovarian cancer cells to cisplatin (90, 91). In addition to targeting COL11A1 transcription, it might also be possible to target COL11A1 post-translation. HSP47 has been shown to be a molecular chaperone required for procollagen folding in the ER (92). Ito et al. (93) screened small-molecule compounds that inhibit Hsp47’s interaction and chaperone activity with collagen and found that a compound AK778 and its cleavage product Col003 disrupted HSP47/collagen binding and also inhibited collagen secretion.
Akt signaling pathway is closely related to cancer progression, metastasis, and drug resistance (94). COL11A1 is involved in the activation of Akt, which in turn promotes the transcription of COL11A1 (95, 96). Therefore, inhibitors of Akt may be candidates for targeting COL11A1. Our report showed that SC66, an Akt inhibitor, was found to inhibit the proliferation of various human ovarian cancer cells in vitro, and the sensitivity of ovarian cancer cells to SC66 was negatively correlated with the content of COL11A1 in cells. SC66 can also increase the sensitivity of ovarian cancer cells to cisplatin and paclitaxel. After SC66 treatment, the invasive ability of ovarian cancer cells was found to have decreased significantly. SC66 has been shown to inhibit the binding of NF-YA and c/ERBβ to COL11A1 promoter, thereby reducing the chemotherapy resistance induced by Twist1 and Mcl-1, and inhibiting the expression of MMP3 and the invasion ability of cancer cells. However, MK-2206 did not regulate the promoter activity changes of COL11A1 (95). After testing three compounds synthesized from AstraZeneca (Cambridge, UK), it was found that only AZD5653 could inhibit the mRNA content and promoter activity of COL11A1, while AZD8835 and AZD8186 inhibited the phosphorylation of Akt, but had no effect on COL11A1. These results indicated that AZD5663 and not the other two inhibitors inhibit COL11A1 transcription by blocking PDK1 activity (96). In conclusion, the selection of appropriate Akt inhibitors is essential for the inhibition of COL11A1.
Targeting inhibitor of apoptosis (IAPs) proteins, which have been shown to be upregulated by COL11A1 (33), might be another way to target the COL11A1 signaling. Two phase I clinical trials have demonstrated that IAP inhibitors ASTX660 and APG-1387 were well tolerated by patients (97). Another IAP inhibitor LCL161 (a Smac mimetic) was also well tolerated in a phase II clinical trial for myelofibrosis, a form of leukemia (98). Another clinical trial using birinapant, an XIAP/cIAP1 antagonist, to treat high-grade serous ovarian cancer showed that birinapant was well tolerated and downregulated tumor cIAP1 (99).
Conclusions
In this review, we provide a comprehensive overview of the biological functions of COL11A1 in cancer and discuss how COL11A1 mediates the crosstalk between cancer cells and the tumor microenvironment (TME) to regulate the phenotypes of cancer cell and CAF. Despite our increased understanding of COL11A1 functions in CAF, it remains unclear whether COL11A1 secreted by cancer cells and CAFs has structural and functional similarities. Further evidence is needed to show how COL11A1 regulates the biophysical properties of tumor ECM and how it affects the invasion, migration, and the proliferation of tumor and immune cells. COL11A1 overexpression has been shown to upregulate chemoresistance, and the roles of COL11A1 in cancer stemness, tumor dormancy, inflammation, and recurrence remain unclear.
The PI3K/Akt signaling pathway has been investigated as a critical regulator of cancer cell survival, and a number of Akt pathway inhibitors with different efficacies and specificities have been identified. We speculate that Akt inhibitors may exert their effect on Akt signaling through different mechanisms and the evaluation of PI3K/Akt/mTOR pathway inhibitors is required to confirm the patterns of sensitivity observed in preclinical studies before they can be applied to clinical settings. In our opinion, COL11A1 and Akt play roles as predictive markers in the prognosis of cancer and as drug design targets in epithelial ovarian cancer (Figure 1).
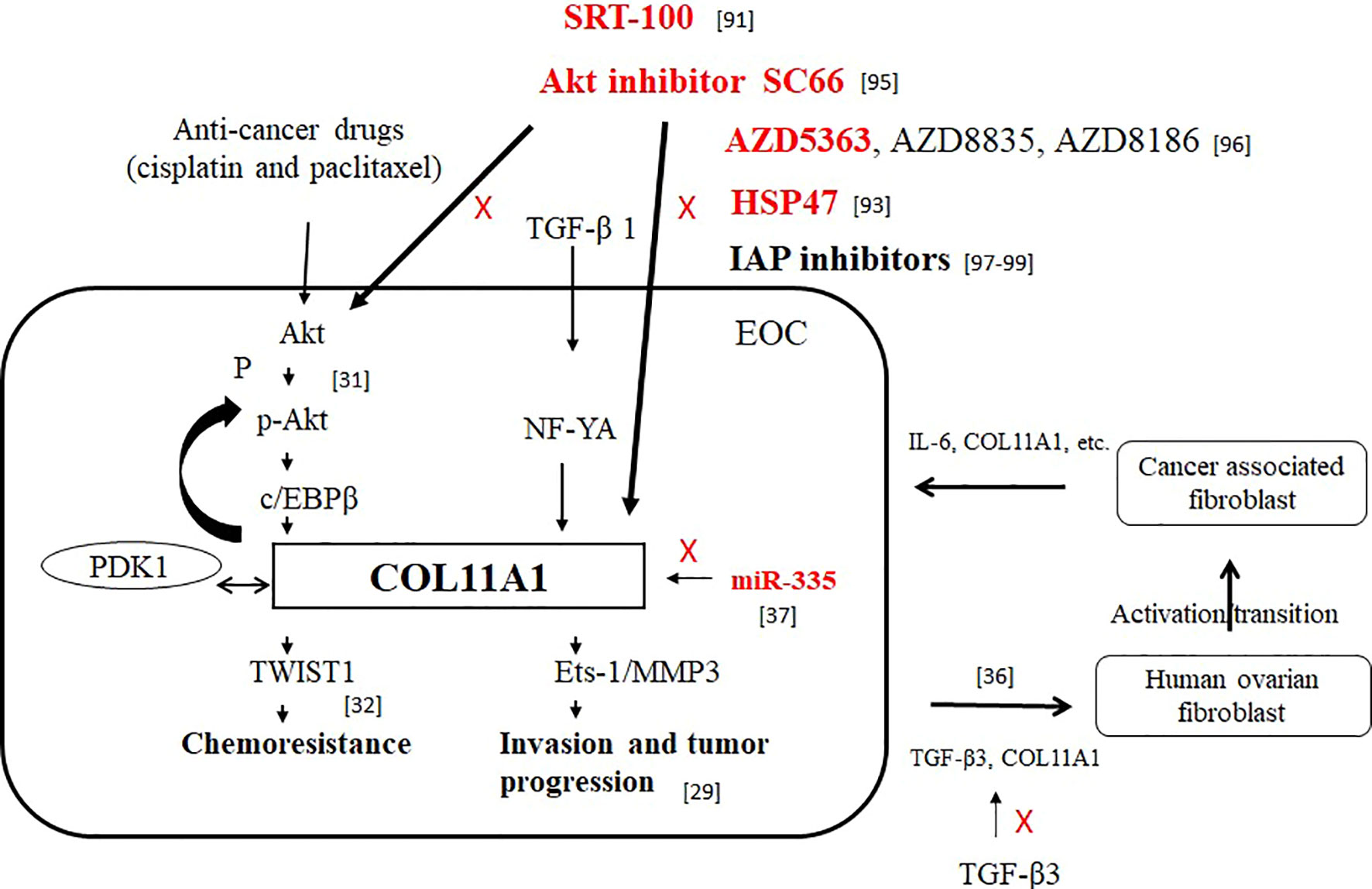
Figure 1 The roles of COL11A1 and Akt as predictive markers in the prognosis of cancer and as drug design targets in EOC. Red font indicates inhibition of COL11A1 expression.
Author Contributions
Y-HW: original draft preparation; C-YC: supervision. All authors have read and agreed to the published version of the manuscript.
Funding
This work was supported by grants from the National Science Council (MOST: No. 108-2314-B-384-011-MY3, 108-2314-B-006-061-MY2 and 110-2314-B-006-036). The study was also supported by grants from the Chi Mei Medical Center, Liouying Campus (CLFHR10822, CLFHR10911, CMLMOST10901, CLFHR10921, CLFHR11015 and CMLMOST11101).
Conflict of Interest
The authors declare that the research was conducted in the absence of any commercial or financial relationships that could be construed as a potential conflict of interest.
Publisher’s Note
All claims expressed in this article are solely those of the authors and do not necessarily represent those of their affiliated organizations, or those of the publisher, the editors and the reviewers. Any product that may be evaluated in this article, or claim that may be made by its manufacturer, is not guaranteed or endorsed by the publisher.
Abbreviations
COL11A1, collagen type XI alpha 1; CAF, cancer associated fibroblast activation; EOC, epithelial ovarian cancer; ECM, extracellular matrix; EMT, epithelial–mesenchymal transition; TGF-β3, transforming growth factor beta 3; NF-κB/IGFBP2, nuclear factor kappa-light-chain-enhancer of activated B/insulin-like growth factor binding protein-2; PFS, progression-free survival; OS, overall survival; DDR2, discoid domain receptor 2; MFAP5, microfibril-associated protein 5; OSM, Oncostatin M; STAT, signal transducer and activator of transcription; NSCLC, non-small cell lung cancer; PDK1, phosphoinositide-dependent kinase 1; lncRNA, long non-coding RNA; PPI, protein-protein interaction; SNHG12, small nucleolar RNA host gene 12; c/EBPβ, CCAAT-enhancer-binding protein-beta; PI3K, phosphoinositide 3-kinase; IAP2, inhibitor apoptosis protein; PKB, protein kinase B; TβRI, transforming growth factor beta receptor 1; HCC, hepatocellular carcinoma.
References
1. Siegel RL, Miller KD, Fuchs HE, Jemal A. Cancer Statistics, 2022. CA Cancer J Clin (2022) 72:7–33. doi: 10.3322/caac.21708
2. Kumar S, Weaver VM. Mechanics, Malignancy, and Metastasis: The Force Journey of a Tumor Cell. Cancer Metastasis Rev (2009) 28:113–27. doi: 10.1007/s10555-008-9173-4
3. Nissen NI, Karsdal M, Willumsen N. Collagens and Cancer Associated Fibroblasts in the Reactive Stroma and its Relation to Cancer Biology. J Exp Clin Cancer Res (2019) 38:115. doi: 10.1186/s13046-019-1110-6
4. Malik R, Lelkes PI, Cukierman E. Biomechanical and Biochemical Remodeling of Stromal Extracellular Matrix in Cancer. Trends Biotechnol (2015) 33:230–6. doi: 10.1016/j.tibtech.2015.01.004
5. Biteau B, Hochmuth CE, Jasper H. Maintaining Tissue Homeostasis: Dynamic Control of Somatic Stem Cell Activity. Cell Stem Cell (2011) 9:402–11. doi: 10.1016/j.stem.2011.10.004
6. Butcher DT, Alliston T, Weaver VM. A Tense Situation: Forcing Tumour Progression. Nat Rev Cancer (2009) 9:108–22. doi: 10.1038/nrc2544
7. Mohan V, Das A, Sagi I. Emerging Roles of ECM Remodeling Processes in Cancer. Semin Cancer Biol (2020) 62:192–200. doi: 10.1016/j.semcancer.2019.09.004
8. Rice AJ, Cortes E, Lachowski D, Cheung BCH, Karim SA, Morton JP, et al. Matrix Stiffness Induces Epithelial-Mesenchymal Transition and Promotes Chemoresistance in Pancreatic Cancer Cells. Oncogenesis (2017) 6:e352. doi: 10.1038/oncsis.2017.54
9. Sun Q, Zhang B, Hu Q, Qin Y, Xu W, Liu W, et al. The Impact of Cancer-Associated Fibroblasts on Major Hallmarks of Pancreatic Cancer. Theranostics (2018) 8:5072–87. doi: 10.7150/thno.26546
10. Vázquez-Villa F, García-Ocaña M, Galván JA, García-Martínez J, García-Pravia C, Menéndez-Rodríguez P, et al. COL11A1/(pro)collagen 11A1 Expression Is a Remarkable Biomarker of Human Invasive Carcinoma-Associated Stromal Cells and Carcinoma Progression. Tumour Biol (2015) 36:2213–22. doi: 10.1007/s13277-015-3295-4
11. Blaschke UK, Eikenberry EF, Hulmes DJ, Galla HJ, Bruckner P. Collagen XI Nucleates Self-Assembly and Limits Lateral Growth of Cartilage Fibrils. J Biol Chem (2000) 275:10370–8. doi: 10.1074/jbc.275.14.10370
12. Sun M, Luo EY, Adams SM, Adams T, Ye Y, Shetye SS, et al. Collagen XI Regulates the Acquisition of Collagen Fibril Structure, Organization and Functional Properties in Tendon. Matrix Biol (2020) 94:77–94. doi: 10.1016/j.matbio.2020.09.001
13. Ricard-Blum S. The Collagen Family. Cold Spring Harb Perspect Biol (2011) 3:a004978. doi: 10.1101/cshperspect.a004978
14. Gu SQ, Luo JH, Yao WX. The Regulation of miR-139-5p on the Biological Characteristics of Breast Cancer Cells by Targeting COL11A1. Math Biosci Eng (2019) 17:1428–41. doi: 10.3934/mbe.2020073
15. Wang H, Ren Y, Qian C, Liu J, Li G, Li Z. Over-Expression of CDX2 Alleviates Breast Cancer by Up-Regulating microRNA Let-7b and Inhibiting COL11A1 Expression. Cancer Cell Int (2020) 20:13. doi: 10.1186/s12935-019-1066-9
16. Ryan RE, Martin B, Mellor L, Jacob RB, Tawara K, McDougal OM, et al. Oncostatin M Binds to Extracellular Matrix in a Bioactive Conformation: Implications for Inflammation and Metastasis. Cytokine (2015) 72:71–85. doi: 10.1016/j.cyto.2014.11.007
17. Galván JA, García-Martínez J, Vázquez-Villa F, García-Ocaña M, García-Pravia C, Menéndez-Rodríguez P, et al. Validation of COL11A1/procollagen 11A1 Expression in TGF-β1-Activated Immortalised Human Mesenchymal Cells and in Stromal Cells of Human Colon Adenocarcinoma. BMC Cancer (2014) 14:867. doi: 10.1186/1471-2407-14-867
18. Wang J, Yu H, Ye L, Jin L, Yu M, Lv Y. Integrated Regulatory Mechanisms of miRNAs and Targeted Genes Involved in Colorectal Cancer. Int J Clin Exp Pathol (2015) 8:517–29.
19. Zhang B, Zhang C, Yang X, Chen Y, Zhang H, Liu J, et al. Cytoplasmic Collagen Xiαi as a Prognostic Biomarker in Esophageal Squamous Cell Carcinoma. Cancer Biol Ther (2018) 19:364–72. doi: 10.1080/15384047.2018.1423915
20. Li A, Li J, Lin J, Zhuo W, Si J. COL11A1 is Overexpressed in Gastric Cancer Tissues and Regulates Proliferation, Migration and Invasion of HGC-27 Gastric Cancer Cells In Vitro. Oncol Rep (2017) 37:333–40. doi: 10.3892/or.2016.5276
21. An JH, Lee SY, Jeon JY, Cho KG, Kim SU, Lee MA. Identification of Gliotropic Factors That Induce Human Stem Cell Migration to Malignant Tumor. J Proteome Res (2009) 8:2873–81. doi: 10.1021/pr900020q
22. Chernov AV, Baranovskaya S, Golubkov VS, Wakeman DR, Snyder EY, Williams R, et al. Microarray-Based Transcriptional and Epigenetic Profiling of Matrix Metalloproteinases, Collagens, and Related Genes in Cancer. J Biol Chem (2010) 285:19647–59. doi: 10.1074/jbc.M109.088153
23. Sok JC, Lee JA, Dasari S, Joyce S, Contrucci SC, Egloff AM, et al. Collagen Type XI α1 Facilitates Head and Neck Squamous Cell Cancer Growth and Invasion. Br J Cancer (2013) 109:3049–56. doi: 10.1038/bjc.2013.624
24. Shen L, Yang M, Lin Q, Zhang Z, Zhu B, Miao C. COL11A1 is Overexpressed in Recurrent non-Small Cell Lung Cancer and Promotes Cell Proliferation, Migration, Invasion and Drug Resistance. Oncol Rep (2016) 36:877–85. doi: 10.3892/or.2016.4869
25. Tu H, Li J, Lin L, Wang L. COL11A1 was Involved in Cell Proliferation, Apoptosis and Migration in non-Small Cell Lung Cancer Cells. J Invest Surg (2021) 34:664–9. doi: 10.1080/08941939.2019.1672839
26. Jin Y, Zhu H, Cai W, Fan X, Wang Y, Niu Y, et al. B-Myb is Up-Regulated and Promotes Cell Growth and Motility in Non-Small Cell Lung Cancer. Int J Mol Sci (2017) 18:860. doi: 10.3390/ijms18060860
27. Turkowski K, Herzberg F, Günther S, Brunn D, Weigert A, Meister M, et al. Fibroblast Growth Factor-14 Acts as Tumor Suppressor in Lung Adenocarcinomas. Cells (2020) 9:1755. doi: 10.3390/cells9081755
28. Jia D, Liu Z, Deng N, Tan TZ, Huang RY-J, Taylor-Harding B, et al. A COL11A1-Correlated Pan-Cancer Gene Signature of Activated Fibroblasts for the Prioritization of Therapeutic Targets. Cancer Lett (2016) 382:203–14. doi: 10.1016/j.canlet.2016.09.001
29. Wu Y-H, Chang T-H, Huang Y-F, Huang H-D, Chou C-Y. COL11A1 Promotes Tumor Progression and Predicts Poor Clinical Outcome in Ovarian Cancer. Oncogene (2014) 33:3432–40. doi: 10.1038/onc.2013.307
30. Cheon D-J, Tong Y, Sim M-S, Dering J, Berel D, Cui X, et al. A Collagen-Remodeling Gene Signature Regulated by TGF-β Signaling Is Associated With Metastasis and Poor Survival in Serous Ovarian Cancer. Clin Cancer Res (2014) 20:711–23. doi: 10.1158/1078-0432.CCR-13-1256
31. Wu Y-H, Chang T-H, Huang Y-F, Chen C-C, Chou C-Y. COL11A1 Confers Chemoresistance on Ovarian Cancer Cells Through the Activation of Akt/C/Ebpβ Pathway and PDK1 Stabilization. Oncotarget (2015) 6:23748–63. doi: 10.18632/oncotarget.4250
32. Wu Y-H, Huang Y-F, Chang T-H, Chou C-Y. Activation of TWIST1 by COL11A1 Promotes Chemoresistance and Inhibits Apoptosis in Ovarian Cancer Cells by Modulating NF-κb-Mediated Ikkβ Expression. Int J Cancer (2017) 141:2305–17. doi: 10.1002/ijc.30932
33. Rada M, Nallanthighal S, Cha J, Ryan K, Sage J, Eldred C, et al. Inhibitor of Apoptosis Proteins (IAPs) Mediate Collagen Type XI Alpha 1-Driven Cisplatin Resistance in Ovarian Cancer. Oncogene (2018) 37:4809–20. doi: 10.1038/s41388-018-0297-x
34. Nallanthighal S, Rada M, Heiserman JP, Cha J, Sage J, Zhou B, et al. Inhibition of Collagen XI Alpha 1-Induced Fatty Acid Oxidation Triggers Apoptotic Cell Death in Cisplatin-Resistant Ovarian Cancer. Cell Death Dis (2020) 11:258. doi: 10.1038/s41419-020-2442-z
35. Yeung T-L, Leung CS, Yip K-P, Sheng J, Vien L, Bover LC, et al. Anticancer Immunotherapy by MFAP5 Blockade Inhibits Fibrosis and Enhances Chemosensitivity in Ovarian and Pancreatic Cancer. Clin Cancer Res (2019) 25:6417–28. doi: 10.1158/1078-0432.CCR-19-0187
36. Wu Y-H, Huang Y-F, Chang T-H, Chen C-C, Wu P-Y, Huang S-C, et al. COL11A1 Activates Cancer-Associated Fibroblasts by Modulating TGF-β3 Through the NF-κb/IGFBP2 Axis in Ovarian Cancer Cells. Oncogene (2021) 40:4503–19. doi: 10.1038/s41388-021-01865-8
37. Wu Y-H, Huang Y-F, Chang T-H, Wu P-Y, Hsieh T-Y, Hsiao S-Y, et al. MiR-335 Restrains the Aggressive Phenotypes of Ovarian Cancer Cells by Inhibiting COL11A1. Cancers (Basel) (2021) 13:6257. doi: 10.3390/cancers13246257
38. Li Y, Liu Z, Sun Y, Ding H, Ren D, Li Y, et al. COL11A1 Acted as a Downstream of Mist1 to Promote the EMT in Pancreatic Cancer (2020). Available at: https://assets.researchsquare.com/files/rs-108113/v1/7ea6f9b7-0a0f-456d-b7d1-e766a5d50cb8.pdf?c=1631861178.
39. Feldmann G, Habbe N, Dhara S, Bisht S, Alvarez H, Fendrich V, et al. Hedgehog Inhibition Prolongs Survival in a Genetically Engineered Mouse Model of Pancreatic Cancer. Gut (2008) 57:1420–30. doi: 10.1136/gut.2007.148189
40. Gaspar NJ, Li L, Kapoun AM, Medicherla S, Reddy M, Li G, et al. Inhibition of Transforming Growth Factor Beta Signaling Reduces Pancreatic Adenocarcinoma Growth and Invasiveness. Mol Pharmacol (2007) 72:152–61. doi: 10.1124/mol.106.029025
41. Wang H, Ren R, Yang Z, Cai J, Du S, Shen X. The COL11A1/Akt/CREB Signaling Axis Enables Mitochondrial-Mediated Apoptotic Evasion to Promote Chemoresistance in Pancreatic Cancer Cells Through Modulating BAX/BCL-2 Function. J Cancer (2021) 12:1406–20. doi: 10.7150/jca.47032
42. Bogusławska J, Rodzik K, Popławski P, Kędzierska H, Rybicka B, Sokół E, et al. TGF-β1 Targets a microRNA Network That Regulates Cellular Adhesion and Migration in Renal Cancer. Cancer Lett (2018) 412:155–69. doi: 10.1016/j.canlet.2017.10.019
43. Xu C, Liang H, Zhou J, Wang Y, Liu S, Wang X, et al. lncRNA Small Nucleolar RNA Host Gene 12 Promotes Renal Cell Carcinoma Progression by Modulating the Mir-200c-5p/Collagen Type XI α1 Chain Pathway. Mol Med Rep (2020) 22:3677–86. doi: 10.3892/mmr.2020.11490
44. Nallanthighal S, Heiserman JP, Cheon D-J. Collagen Type XI Alpha 1 (COL11A1): A Novel Biomarker and a Key Player in Cancer. Cancers (Basel) (2021) 13:935. doi: 10.3390/cancers13050935
45. Zhu M, Ye C, Wang J, Yang G, Ying X. Activation of COL11A1 by PRRX1 Promotes Tumor Progression and Radioresistance in Ovarian Cancer. Int J Radiat Biol (2021) 97:958–67. doi: 10.1080/09553002.2021.1928780
46. Pearce OMT, Delaine-Smith RM, Maniati E, Nichols S, Wang J, Böhm S, et al. Deconstruction of a Metastatic Tumor Microenvironment Reveals a Common Matrix Response in Human Cancers. Cancer Discovery (2018) 8:304–19. doi: 10.1158/2159-8290.cd-17-0284
47. Buckanovich RJ, Sasaroli D, O’Brien-Jenkins A, Botbyl J, Hammond R, Katsaros D, et al. Tumor Vascular Proteins as Biomarkers in Ovarian Cancer. J Clin Oncol (2007) 25:852–61. doi: 10.1200/JCO.2006.08.8583
48. García-Pravia C, Galván JA, Gutiérrez-Corral N, Solar-García L, García-Pérez E, García-Ocaña M, et al. Overexpression of COL11A1 by Cancer-Associated Fibroblasts: Clinical Relevance of a Stromal Marker in Pancreatic Cancer. PLoS One (2013) 8:e78327. doi: 10.1371/journal.pone.0078327
49. Fischer H, Stenling R, Rubio C, Lindblom A. Colorectal Carcinogenesis is Associated With Stromal Expression of COL11A1 and COL5A2. Carcinogenesis (2001) 22:875–78. doi: 10.1093/carcin/22.6.875
50. Fuentes-Martínez N, García-Pravia C, García-Ocaña M, Menéndez-Rodríguez P, Del Amo J, Suárez-Fernández L, et al. Overexpression of Procol11a1 as a Stromal Marker of Breast Cancer. Histol Histopathol (2015) 30:87–93. doi: 10.14670/HH-30.87
51. Navab R, Strumpf D, Bandarchi B, Zhu C-Q, Pintilie M, Ramnarine VR, et al. Prognostic Gene-Expression Signature of Carcinoma-Associated Fibroblasts in non-Small Cell Lung Cancer. Proc Natl Acad Sci U S A (2011) 108:7160–5. doi: 10.1073/pnas.1014506108
52. Erkan M, Weis N, Pan Z, Schwager C, Samkharadze T, Jiang X, et al. Organ-, Inflammation- and Cancer Specific Transcriptional Fingerprints of Pancreatic and Hepatic Stellate Cells. Mol Cancer (2010) 9:88. doi: 10.1186/1476-4598-9-88
53. Park H, Lee Y, Lee H, Kim J-W, Hwang J-H, Kim J, et al. The Prognostic Significance of Cancer-Associated Fibroblasts in Pancreatic Ductal Adenocarcinoma. Tumour Biol (2017) 39:1010428317718403. doi: 10.1177/1010428317718403
54. Raglow Z, Thomas SM. Tumor Matrix Protein Collagen Xiα1 in Cancer. Cancer Lett (2015) 357:448–53. doi: 10.1016/j.canlet.2014.12.011
55. Xu S, Xu H, Wang W, Li S, Li H, Li T, et al. The Role of Collagen in Cancer: From Bench to Bedside. J Transl Med (2019) 17:309. doi: 10.1186/s12967-019-2058-1
56. Arolt C, Meyer M, Hoffmann F, Wagener-Ryczek S, Schwarz D, Nachtsheim L, et al. Expression Profiling of Extracellular Matrix Genes Reveals Global and Entity-Specific Characteristics in Adenoid Cystic, Mucoepidermoid and Salivary Duct Carcinomas. Cancers (Basel) (2020) 12:2466. doi: 10.3390/cancers12092466
57. Costa A, Kieffer Y, Scholer-Dahirel A, Pelon F, Bourachot B, Cardon M, et al. Fibroblast Heterogeneity and Immunosuppressive Environment in Human Breast Cancer. Cancer Cell (2018) 33:463–79.e10. doi: 10.1016/j.ccell.2018.01.011
58. Hida M, Hamanaka R, Okamoto O, Yamashita K, Sasaki T, Yoshioka H, et al. Nuclear Factor Y (NF-Y) Regulates the Proximal Promoter Activity of the Mouse Collagen α1(XI) Gene (Col11a1) in Chondrocytes. In Vitro Cell Dev Biol Anim (2014) 50:358–66. doi: 10.1007/s11626-013-9692-3
59. Watanabe K, Hida M, Sasaki T, Yano H, Kawano K, Yoshioka H, et al. Sp1 Upregulates the Proximal Promoter Activity of the Mouse Collagen α1(XI) Gene (Col11a1) in Chondrocytes. In Vitro Cell Dev Biol Anim (2016) 52:235–42. doi: 10.1007/s11626-015-9959-y
60. Matsuo N, Yu-Hua W, Sumiyoshi H, Sakata-Takatani K, Nagato H, Sakai K, et al. The Transcription Factor CCAAT-Binding Factor CBF/NF-Y Regulates the Proximal Promoter Activity in the Human Alpha 1(XI) Collagen Gene (COL11A1). J Biol Chem (2003) 278:32763–70. doi: 10.1074/jbc.M305599200
61. Yang B, Guo H, Zhang Y, Chen L, Ying D, Dong S. MicroRNA-145 Regulates Chondrogenic Differentiation of Mesenchymal Stem Cells by Targeting Sox9. PLoS One (2011) 6:e21679. doi: 10.1371/journal.pone.0021679
62. Ripa R, Dolfi L, Terrigno M, Pandolfini L, Savino A, Arcucci V, et al. MicroRNA miR-29 Controls a Compensatory Response to Limit Neuronal Iron Accumulation During Adult Life and Aging. BMC Biol (2017) 15:9. doi: 10.1186/s12915-017-0354-x
63. Kamran F, Andrade AC, Nella AA, Clokie SJ, Rezvani G, Nilsson O, et al. Evidence That Up-Regulation of MicroRNA-29 Contributes to Postnatal Body Growth Deceleration. Mol Endocrinol (2015) 29:921–32. doi: 10.1210/me.2015-1047
64. Liao M, Zou S, Weng J, Hou L, Yang L, Zhao Z, et al. A microRNA Profile Comparison Between Thoracic Aortic Dissection and Normal Thoracic Aorta Indicates the Potential Role of microRNAs in Contributing to Thoracic Aortic Dissection Pathogenesis. J Vasc Surg (2011) 53:1341–9.e3. doi: 10.1016/j.jvs.2010.11.113
65. Kang Z, Zhu J, Sun N, Zhang X, Liang G, Kou Y, et al. COL11A1 Promotes Esophageal Squamous Cell Carcinoma Proliferation and Metastasis and Is Inversely Regulated by miR-335-5p. Ann Transl Med (2021) 9:1577. doi: 10.21037/atm-21-4951
66. Teng P-N, Wang G, Hood BL, Conrads KA, Hamilton CA, Maxwell GL, et al. Identification of Candidate Circulating Cisplatin-Resistant Biomarkers From Epithelial Ovarian Carcinoma Cell Secretomes. Br J Cancer (2014) 110:123–32. doi: 10.1038/bjc.2013.687
67. Chong I-W, Chang M-Y, Chang H-C, Yu Y-P, Sheu C-C, Tsai J-R, et al. Great Potential of a Panel of Multiple Hmth1, SPD, ITGA11 and COL11A1 Markers for Diagnosis of Patients With non-Small Cell Lung Cancer. Oncol Rep (2006) 16:981–8. doi: 10.3892/or.16.5.981
68. Giussani M, Landoni E, Merlino G, Turdo F, Veneroni S, Paolini B, et al. Extracellular Matrix Proteins as Diagnostic Markers of Breast Carcinoma. J Cell Physiol (2018) 233:6280–90. doi: 10.1002/jcp.26513
69. Kandel ES, Hay N. The Regulation and Activities of the Multifunctional Serine/Threonine Kinase Akt/PKB. Exp Cell Res (1999) 253:210–29. doi: 10.1006/excr.1999.4690
70. Testa JR, Bellacosa A. AKT Plays a Central Role in Tumorigenesis. Proc Natl Acad Sci U S A (2001) 98:10983–5. doi: 10.1073/pnas.211430998
71. Nicholson KM, Anderson NG. The Protein Kinase B/Akt Signalling Pathway in Human Malignancy. Cell Signal (2002) 14:381–95. doi: 10.1016/s0898-6568(01)00271-6
72. Vivanco I, Sawyers CL. The Phosphatidylinositol 3-Kinase AKT Pathway in Human Cancer. Nat Rev Cancer (2002) 2:489–501. doi: 10.1038/nrc839
73. Brazil DP, Park J, Hemmings BA. PKB Binding Proteins. Getting in on the Akt. Cell (2002) 111:293–303. doi: 10.1016/s0092-8674(02)01083-8
74. Vanhaesebroeck B, Alessi DR. The PI3K-PDK1 Connection: More Than Just a Road to PKB. Biochem J (2000) 346:561–76. doi: 10.1042/bj3460561
75. Jacinto E, Facchinetti V, Liu D, Soto N, Wei S, Jung SY, et al. SIN1/MIP1 Maintains rictor-mTOR Complex Integrity and Regulates Akt Phosphorylation and Substrate Specificity. Cell (2006) 127:125–37. doi: 10.1016/j.cell.2006.08.033
76. Alessi DR, Deak M, Casamayor A, Caudwell FB, Morrice N, Norman DG, et al. 3-Phosphoinositide-Dependent Protein Kinase-1 (PDK1): Structural and Functional Homology With the Drosophila DSTPK61 Kinase. Curr Biol (1997) 7:776–89. doi: 10.1016/s0960-9822(06)00336-8
77. Alessi DR, James SR, Downes CP, Holmes AB, Gaffney PRJ, Reese CB, et al. Characterization of a 3-Phosphoinositide-Dependent Protein Kinase Which Phosphorylates and Activates Protein Kinase Bα. Curr Biol (1997) 7:261–9. doi: 10.1016/s0960-9822(06)00122-9
78. Sarbassov DD, Ali SM, Sengupta S, Sheen J-H, Hsu PP, Bagley AF, et al. Prolonged Rapamycin Treatment Inhibits Mtorc2 Assembly and Akt/PKB. Mol Cell (2006) 22:159–68. doi: 10.1016/j.molcel.2006.03.029
79. Fraser M, Bai T, Tsang BK. Akt Promotes Cisplatin Resistance in Human Ovarian Cancer Cells Through Inhibition of P53 Phosphorylation and Nuclear Function. Int J Cancer (2008) 122:534–46. doi: 10.1002/ijc.23086
80. Abedini MR, Muller EJ, Bergeron R, Gray DA, Tsang BK. Akt Promotes Chemoresistance in Human Ovarian Cancer Cells by Modulating Cisplatin-Induced, P53-Dependent Ubiquitination of FLICE-Like Inhibitory Protein. Oncogene (2010) 29:11–25. doi: 10.1038/onc.2009.300
81. Fraser M, Leung BM, Yan X, Dan HC, Cheng JQ, Tsang BK. P53 Is a Determinant of X-Linked Inhibitor of Apoptosis Protein/Akt-Mediated Chemoresistance in Human Ovarian Cancer Cells. Cancer Res (2003) 63:7081–8.
82. Yang X, Fraser M, Moll UM, Basak A, Tsang BK. Akt-Mediated Cisplatin Resistance in Ovarian Cancer: Modulation of P53 Action on Caspase-Dependent Mitochondrial Death Pathway. Cancer Res (2006) 66:3126–36. doi: 10.1158/0008-5472.CAN-05-0425
83. Fraser M, Leung B, Jahani-Asl A, Yan X, Thompson WE, Tsang BK. Chemoresistance in Human Ovarian Cancer: The Role of Apoptotic Regulators. Reprod Biol Endocrinol (2003) 1:66. doi: 10.1186/1477-7827-1-66
84. Yan X, Fraser M, Qiu Q, Tsang BK. Over-Expression of PTEN Sensitizes Human Ovarian Cancer Cells to Cisplatin-Induced Apoptosis in a P53-Dependent Manner. Gynecol Oncol (2006) 102:348–55. doi: 10.1016/j.ygyno.2005.12.033
85. Ali AY, Kim J-Y, Pelletier J-F, Vanderhyden BC, Bachvarov DR, Tsang BK. Akt Confers Cisplatin Chemoresistance in Human Gynecological Carcinoma Cells by Modulating PPM1D Stability. Mol Carcinog (2015) 54:1301–14. doi: 10.1002/mc.22205
86. Brandes AA, Carpentier AF, Kesari S, Sepulveda-Sanchez JM, Wheeler HR, Chinot O, et al. A Phase II Randomized Study of Galunisertib Monotherapy or Galunisertib Plus Lomustine Compared With Lomustine Monotherapy in Patients With Recurrent Glioblastoma. Neuro Oncol (2016) 18:1146–56. doi: 10.1093/neuonc/now009
87. Zhang Q, Hou X, Evans BJ, VanBlaricom JL, Weroha SJ, Cliby WA. LY2157299 Monohydrate, A TGF-βr1 Inhibitor, Suppresses Tumor Growth and Ascites Development in Ovarian Cancer. Cancers (Basel) (2018) 10:260. doi: 10.3390/cancers10080260
88. Kelley RK, Gane E, Assenat E, Siebler J, Galle PR, Merle P, et al. A Phase 2 Study of Galunisertib (TGF-β1 Receptor Type I Inhibitor) and Sorafenib in Patients With Advanced Hepatocellular Carcinoma. Clin Transl Gastroenterol (2019) 10:e00056. doi: 10.14309/ctg.0000000000000056
89. Giannelli G, Santoro A, Kelley RK, Gane E, Paradis V, Cleverly A, et al. Biomarkers and Overall Survival in Patients With Advanced Hepatocellular Carcinoma Treated With TGF-βri Inhibitor Galunisertib. PLoS One (2020) 15:e0222259. doi: 10.1371/journal.pone.0222259
90. Yu S, Sheu H-M, Lee C-H. Solanum Incanum Extract (SR-T100) Induces Melanoma Cell Apoptosis and Inhibits Established Lung Metastasis. Oncotarget (2017) 8:103509–17. doi: 10.18632/oncotarget.21508
91. Wu Y-H, Chiu W-T, Young M-J, Chang T-H, Huang Y-F, Chou C-Y. Solanum Incanum Extract Downregulates Aldehyde Dehydrogenase 1-Mediated Stemness and Inhibits Tumor Formation in Ovarian Cancer Cells. J Cancer (2015) 6:1011–9. doi: 10.7150/jca.12738
92. Nagata K, Saga S, Yamada KM. A Major Collagen-Binding Protein of Chick Embryo Fibroblasts is a Novel Heat Shock Protein. J Cell Biol (1986) 103:223–9. doi: 10.1083/jcb.103.1.223
93. Ito S, Ogawa K, Takeuchi K, Takagi M, Yoshida M, Hirokawa T, et al. A Small-Molecule Compound Inhibits a Collagen-Specific Molecular Chaperone and Could Represent a Potential Remedy for Fibrosis. J Biol Chem (2017) 292:20076–85. doi: 10.1074/jbc.m117.815936
94. Ediriweera MK, Tennekoon KH, Samarakoon SR. Role of the PI3K/AKT/mTOR Signaling Pathway in Ovarian Cancer: Biological and Therapeutic Significance. Semin Cancer Biol (2019) 59:147–60. doi: 10.1016/j.semcancer.2019.05.012
95. Wu Y-H, Huang Y-F, Chen C-C, Chou C-Y. Akt Inhibitor SC66 Promotes Cell Sensitivity to Cisplatin in Chemoresistant Ovarian Cancer Cells Through Inhibition of COL11A1 Expression. Cell Death Dis (2019) 10:322. doi: 10.1038/s41419-019-1555-8
96. Wu Y-H, Huang Y-F, Chen C-C, Huang C-Y, Chou C-Y. Comparing PI3K/Akt Inhibitors Used in Ovarian Cancer Treatment. Front Pharmacol (2020) 11:206. doi: 10.3389/fphar.2020.00206
97. Mita MM, LoRusso PM, Papadopoulos KP, Gordon MS, Mita AC, Ferraldeschi R, et al. A Phase I Study of ASTX660, an Antagonist of Inhibitors of Apoptosis Proteins, in Adults With Advanced Cancers or Lymphoma. Clin Cancer Res (2020) 26:2819–26. doi: 10.1158/1078-0432.CCR-19-1430
98. Xu R, Li Y, Ji J, Qiu M, Zhang Y, Liu W, et al. A Phase I Study of a Novel IAP Inhibitor APG-1387 in Patients With Advanced Solid Tumors. J Clin Oncol (2018) 36:2593. doi: 10.1200/jco.2018.36.15_suppl.2593
99. Noonan AM, Bunch KP, Chen J-Q, Herrmann MA, Lee J-M, Kohn EC, et al. Pharmacodynamic Markers and Clinical Results From the Phase 2 Study of the SMAC Mimetic Birinapant in Women With Relapsed Platinum-Resistant or -Refractory Epithelial Ovarian Cancer. Cancer (2016) 122:588–97. doi: 10.1002/cncr.29783
Keywords: collagen type XI alpha 1, cancer progression, chemoresistance, cancer associated fibroblast activation, epithelial ovarian cancer
Citation: Wu Y-H and Chou C-Y (2022) Collagen XI Alpha 1 Chain, a Novel Therapeutic Target for Cancer Treatment. Front. Oncol. 12:925165. doi: 10.3389/fonc.2022.925165
Received: 21 April 2022; Accepted: 31 May 2022;
Published: 29 June 2022.
Edited by:
Triantafyllos Stylianopoulos, University of Cyprus, CyprusReviewed by:
Dong-Joo (Ellen) Cheon, Albany Medical College, United StatesCopyright © 2022 Wu and Chou. This is an open-access article distributed under the terms of the Creative Commons Attribution License (CC BY). The use, distribution or reproduction in other forums is permitted, provided the original author(s) and the copyright owner(s) are credited and that the original publication in this journal is cited, in accordance with accepted academic practice. No use, distribution or reproduction is permitted which does not comply with these terms.
*Correspondence: Cheng-Yang Chou, Y2hvdWd5bkBtYWlsLm5ja3UuZWR1LnR3