- 1Smilow Cancer Center, Yale School of Medicine, New Haven, CT, United States
- 2Clinical Science, Clovis Oncology UK, Ltd, Cambridge, United Kingdom
- 3Translational Medicine, Clovis Oncology, Inc., Boulder, CO, United States
Background: The poly(ADP-ribose) polymerase (PARP) inhibitor rucaparib was approved in the United States based on the phase 2 TRITON2 study of patients with BRCA1 or BRCA2 (BRCA)–mutated metastatic castration-resistant prostate cancer (mCRPC). Although genomic screening is recommended as part of a comprehensive assessment of prostate cancer prognosis and treatment options, the best way to select patients with mCRPC for treatment with a PARP inhibitor depends on individual clinical circumstances. For example, assessment of tumor tissue may not always be feasible. Genomic testing of DNA from plasma has become more readily available, providing a minimally invasive option to evaluate DNA from primary and metastatic lesions simultaneously.
Case Presentation: A patient from TRITON2 with BRCA-mutated mCRPC had a response to the PARP inhibitor rucaparib and remained on treatment for 32 weeks, which was >2 times longer than the duration of each of his prior therapies (bicalutamide, docetaxel, abiraterone). The patient enrolled in TRITON2 based on results of local genomic testing of an archival biopsy that indicated the presence of a BRCA1 T1399I (allelic fraction, 19%) mutation. Local testing also identified an ATM G1663C mutation, a TP53 P191del mutation, and a BRAF K601E mutation. Analysis of a plasma sample obtained before the patient started rucaparib detected the same alterations as those in the archival biopsy, but it also revealed the presence of a BRCA2 homozygous loss (whole gene, 26 of 26 exons) and several other alterations of unknown functional impact. We hypothesize the response of the patient’s tumor to rucaparib was likely driven by DNA damage repair deficiency caused by homozygous loss of all BRCA2 exons. Following discontinuation from rucaparib due to clinical disease progression, the patient received carboplatin and cabazitaxel for ≈3 weeks. The patient died due to progression of his disease.
Conclusions: A notable aspect of this case is the differences in alterations detected in the archival tumor sample and a more recent plasma sample. This highlights the advantages of plasma testing compared with tissue testing when selecting targeted therapies for treatment of mCRPC; however, physicians must determine which tool presents the best solution for each individual case.
Introduction
Molecular characterization of prostate cancer has become increasingly relevant with the identification of high-risk hereditary factors (1–4) and development of targeted therapies with genomic biomarkers. Among the targeted therapies approved for patients with metastatic castration-resistant prostate cancer (mCRPC) are the poly(ADP-ribose) polymerase (PARP) inhibitors olaparib and rucaparib (5, 6), which have demonstrated efficacy in patients with DNA damage repair (DDR) defects, particularly BRCA1 or BRCA2 (BRCA) alterations (7, 8). Such mutations are associated with adverse clinical features and poor outcomes in patients (9). Rucaparib was approved in the United States based on the phase 2 TRITON2 study of patients with BRCA-mutated mCRPC (5, 7).
Genomic screening for pathogenic alterations in multiple genes, including BRCA1 and BRCA2, is recommended as part of a comprehensive assessment of prostate cancer prognosis and treatment options (10–13). However, the best way to select patients with mCRPC for treatment with a PARP inhibitor depends on a patient’s individual clinical circumstances. Molecular assessment of tumor tissue, historically the gold standard, may not always be feasible due to lack of tissue samples of sufficient quality or difficulty in obtaining contemporaneous biopsies (14). Genomic testing of cell-free DNA (cfDNA) in plasma has advanced technically and has become more readily available, providing a minimally invasive option to evaluate DNA from primary and metastatic lesions simultaneously (15). Plasma-based assays, such as FoundationOne Liquid CDx (Foundation Medicine, Inc., Cambridge, MA), have been approved as companion diagnostics for the selection of patients for treatment with PARP inhibitors.
In TRITON2, patients were prospectively selected based on alteration status from central tissue, central plasma, or local test results (blood, tissue, and/or plasma), reflecting the real-world landscape of clinical genomic testing in patients with mCRPC. Here we report a case study of a patient enrolled in TRITON2 based on next-generation sequencing (NGS) of an archival biopsy with subsequent investigation of pretreatment plasma cfDNA that revealed additional alterations of interest which we believe to have contributed to the patient’s clinical response to rucaparib.
Case Presentation
A 52-year-old White man presented with intermittent constipation and back pain, with a history of a decrease in lymphocyte count (documented two months prior). The patient was a never smoker and had no family history of cancer or other cancer risk factors. Initial computed tomography scans revealed stage T4 prostatic adenocarcinoma with invasion into adjacent structures, metastasis to regional lymph nodes (stage N1), and metastases to the liver, bone, and a distant lymph node (stage M1) (16, 17). A retroperitoneal lymph node was biopsied to confirm histology. His prostate-specific antigen (PSA) level was 1291ng/mL.
The patient started the antiandrogen bicalutamide (oral) shortly after confirmed diagnosis (Figure 1A) and a gonadotrophin-releasing hormone agonist, leuprorelin (depot injection), was subsequently initiated to affect androgen deprivation. The patient received treatment until PSA values began to rise ≈15 weeks later and the patient discontinued bicalutamide. Docetaxel (intravenous infusion; 4 cycles) plus prednisone (oral; continuous dosing) was administered as standard of care; prednisone was continued for 1 week after the end of docetaxel treatment for symptom control. The patient ultimately discontinued docetaxel/prednisone due to radiographic disease progression and PSA progression and immediately started on abiraterone as an androgen receptor targeting therapy, which continued for 7 weeks until radiographic disease progression and PSA progression. The patient also received palliative radiation of the right femur and acetabula around the time abiraterone was initiated.
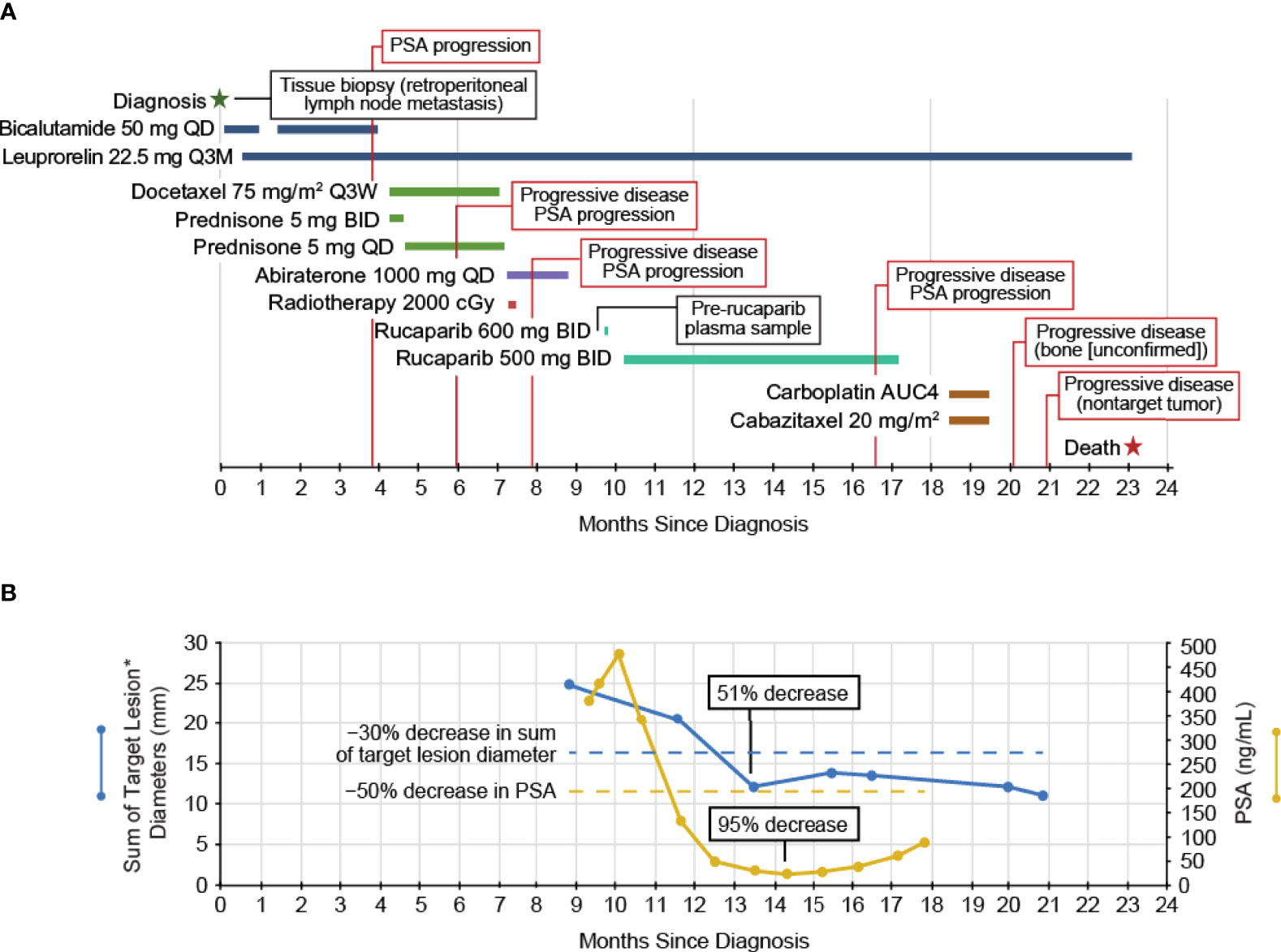
Figure 1 (A) The clinical course of the patient and (B) the PSA and tumor measurements. *Two measurable liver lesions. BID, twice daily; PSA, prostate-specific antigen; Q3M, every 3 months; Q3W, every 3 weeks; QD, once daily.
After discontinuing abiraterone, the patient was enrolled in the TRITON2 study based on results of local genomic testing of an archival tissue biopsy (retroperitoneal lymph node metastasis, 90% tumor purity) obtained at initial diagnosis. Local testing utilized the Oncomine™ Comprehensive Assay v3 (Thermo Fisher Scientific Inc., Waltham, MA, USA), which can detect single-nucleotide variants, copy-number variations, gene fusions, and insertions/deletions in 161 cancer-related genes. This local test indicated the presence of a BRCA1 T1399I (allelic fraction [AF], 19%) mutation (Table 1), a novel variant of uncertain significance within a coiled-coil domain which bioinformatics analyses predicted to have a deleterious effect on the BRCA1-PALB2 interaction. A deleterious or probably damaging ATM G1663C mutation, a damaging TP53 P191del mutation, and an oncogenic, activating BRAF K601E mutation were also detected; no gene amplifications or gene fusions were detected. TRITON2 patients provided plasma samples for central genomic analysis prior to starting rucaparib. Analysis of the patient’s pre-rucaparib plasma sample was conducted using the FoundationOne Liquid CDx assay, which analyzes 324 cancer-related genes and identifies the same classes of BRCA alterations, including homozygous deletions. The FoundationOne Liquid CDx assay detected the same alterations as the Oncomine analysis of the archival tissue biopsy but also revealed the presence of a BRCA2 homozygous loss (whole gene, 26 of 26 exons) and several other alterations of unknown functional impact in a plasma sample with 28% tumor content (Table 1).
The patient started at the recommended dose of rucaparib, 600 mg twice daily, but the dose was reduced to 500 mg twice daily due to nausea/fatigue, with the patient ultimately receiving rucaparib for 32 weeks (Figure 1A). At enrollment into TRITON2, the patient had >21 bone-associated lesions and multiple liver lesions. Treatment with rucaparib resulted in a confirmed partial response per modified Response Evaluation Criteria In Solid Tumors, version 1.1 (51% decrease in liver metastasis target lesion diameters; Figure 1B) lasting 13 weeks, ongoing as of the last radiographic assessment before subsequent anti-cancer therapy, resulting in a rPFS of 29 weeks, with no confirmed progression in bone. The patient also had a confirmed PSA response (maximum decrease, 95%; Figure 1B) lasting 28 weeks from the first dose of rucaparib. The patient discontinued rucaparib treatment due to clinical disease progression after 32 weeks on study and subsequently received palliative radiotherapy due to painful bone lesions.
Following discontinuation from the rucaparib treatment, the patient received carboplatin and cabazitaxel for 2 cycles (intravenous infusion) until subsequent scans indicated progressive disease in nontarget liver lesions two months later. The patient discontinued carboplatin/cabazitaxel and did not receive any further anticancer therapies. The patient died ≈23 months after initial diagnosis due to progression of his disease.
Discussion and Conclusions
Here we have reported on a patient with BRCA-mutated mCRPC who had a response to treatment with the PARP inhibitor rucaparib, remaining on rucaparib treatment for >2 times longer than each of the other therapies he received. We hypothesize the response of the patient’s tumor to rucaparib was likely driven by DDR deficiency caused by homozygous loss of all BRCA2 exons. Notably, tissue NGS was conducted on an archival tumor sample obtained at the time of initial diagnosis, while plasma NGS was conducted on samples obtained prior to rucaparib treatment (43 weeks after diagnosis). The tissue contained a BRCA1 missense alteration with a computationally inferred deleterious effect, while the patient’s plasma yielded a homozygous whole-gene BRCA2 deletion in addition to the BRCA1 alteration.
This result demonstrates the challenges of selecting the “right” sample and assay type as well as the right time for molecular profiling. Since both Oncomine and FoundationOne Liquid CDx assays are capable of detecting all alteration types (19, 20), a possible explanation for the discordance is temporal and/or spatial heterogeneity between tumor deposits. For example, the retroperitoneal lymph node (which did not meet size criteria for tracking as a malignant lesion, after biopsy) may not have contained the BRCA2 homozygous loss (yet), whereas the metastases, such as those in the liver (which did respond) and bone, may have contained this alteration. This highlights the ability of plasma testing to overcome the limitation of sampling a single lesion because plasma may contain DNA from both primary and metastatic lesions (15), provided that sufficient tumor content is present in the plasma, which may not be the case for patients with low tumor burden or who are responding to a therapy. Alternatively, it is possible that the BRCA2 homozygous loss was acquired subsequent to the initial lymph node biopsy after docetaxel and abiraterone treatment. Somatic BRCA alterations that arise during the course of disease progression and/or treatment have been shown to make up half of all BRCA alterations in mCRPC (21), among them homozygous BRCA loss, which accounts for roughly 20% of all BRCA alterations in mCRPC (22). Therefore, another advantage of plasma testing is its ability to query the current genomic landscape rather than that of an earlier disease stage when tissue biopsies are typically performed.
In a recent analysis, >90% of patients with mCRPC had detectable circulating tumor DNA (ctDNA) (23), and the frequency of alterations detected in ctDNA was similar to that reported in studies of tissue biopsies (21, 24, 25). Further, the high concordance (75–90%) between matched plasma and tissue pairs in analyses from TRITON2 (26) and the phase 3 PROfound study of olaparib (27) highlights the utility of plasma testing in detecting alterations in genes of interest, such as BRCA1 and BRCA2. However, it is interesting to note cases such as the current patient, where discordant results have identified additional actionable gene alterations. Importantly, the objective and PSA response rates following rucaparib treatment were similar in TRITON2 patients with mCRPC who had BRCA alterations detected by tissue or by plasma (26).
In general, genomic analysis of cfDNA purified from blood can be a practical alternative to tumor tissue testing for patients with mCRPC (28, 29). Patients with mCRPC rarely undergo routine biopsy sampling, and archival biopsy tissue collected from a single site at diagnosis may be less representative of the metastatic disease state (30, 31). As mCRPC largely targets the bones, many patients lack accessible soft tissue lesions for a contemporaneous biopsy (14). However, cfDNA can be obtained from patients through a minimally invasive blood draw to evaluate the contemporaneous genomic tumor landscape. An important concern in plasma testing is potential false-positive results from the cfDNA assay due to technical or biological factors, such as low variant allele frequency and clonal hematopoiesis of indeterminate potential (CHIP); therefore, use of plasma assays may be complemented by sequencing of tissue and/or matched nontumor samples (32–36). Technical and analytical methods have been developed to address difficulties such as low levels of tumor DNA (37) and detecting certain types of genomic changes (eg, fusions, deletions, copy number variations) (38, 39). Simultaneously, missing patients who may be eligible for targeted treatment may be a real concern when sequencing a single sample to obtain a genomic snapshot at one specific timepoint. Testing multiple samples from a patient over the course of his disease to capture the genomic topography as it evolves may be advisable.
In summary, this case is an example highlighting several advantages of plasma testing compared with tissue testing when selecting targeted therapies for treatment of mCRPC. However, in all cases, physicians will have to determine which tool presents the best solution for any given patient’s clinical circumstances.
Data Availability Statement
The original contributions presented in the study are included in the article. Further inquiries can be directed to the corresponding author.
Ethics Statement
The TRITON2 study involved human participants, was reviewed and approved by national or local institutional review boards including Yale IRB (protocol ID 2000020433), and was performed in accordance with the Declaration of Helsinki and Good Clinical Practice guidelines of the International Council for Harmonisation. The patients/ participants provided their written informed consent to participate in this study. Written informed consent was obtained from the individual(s) for the publication of any potentially identifiable images or data included in this article.
Author Contributions
SW and AL were involved in the TRITON2 study design. DP, SW, and AL collected the data, analyzed and interpreted the data, drafted and revised the manuscript, and read and approved the final manuscript. All authors attest to accountability for the accuracy and integrity of the manuscript.
Funding
TRITON2 was funded by Clovis Oncology, Inc.
Conflict of Interest
DP reports consulting fees for Clovis Oncology, Ada Cap (Advanced Accelerator Applications), Amgen, Astellas, AstraZeneca, Bayer, Bicycle Therapeutics, Boehringer Ingelheim, Bristol Myers Squibb, Eli Lilly, Exelixis, Gilead Sciences, Incyte, Ipsen, Janssen, Mirati, Monopteros, Pfizer, Pharmacyclics, Regeneron, Roche, Seattle Genetics, and Urogen, grant support from Clovis Oncology, Ada Cap (Advanced Accelerator Applications), Agensys Inc, Astellas, AstraZeneca, Bayer, BioXcel Therapeutics, Bristol Myers Squibb, Eisai, Eli Lilly, Endocyte, Genentech, Gilead Sciences, Innocrin, MedImmune, Medivation, Merck, Mirati, Novartis, Pfizer, Progenics, Replimune, Roche, Sanofi Aventis, and Seattle Genetics, and prior ownership interest/investment in Bellicum (sold 7/2020), Tyme (sold 10/2019). SW and AL are employees of Clovis Oncology and may own stock or have stock options in that company.
Publisher’s Note
All claims expressed in this article are solely those of the authors and do not necessarily represent those of their affiliated organizations, or those of the publisher, the editors and the reviewers. Any product that may be evaluated in this article, or claim that may be made by its manufacturer, is not guaranteed or endorsed by the publisher.
Acknowledgments
Medical writing and editorial support funded by Clovis Oncology, Inc., were provided by Nathan Yardley and Stephen Bublitz of Ashfield MedComms, an Ashfield Health company.
References
1. Leongamornlert D, Mahmud N, Tymrakiewicz M, Saunders E, Dadaev T, Castro E, et al. Germline BRCA1 Mutations Increase Prostate Cancer Risk. Br J Cancer (2012) 106:1697–701. doi: 10.1038/bjc.2012.146
2. Thompson D, Easton DF. Cancer Incidence in BRCA1 Mutation Carriers. J Natl Cancer Inst (2002) 94:1358–65. doi: 10.1093/jnci/94.18.1358
3. Kote-Jarai Z, Leongamornlert D, Saunders E, Tymrakiewicz M, Castro E, Mahmud N, et al. BRCA2 is a Moderate Penetrance Gene Contributing to Young-Onset Prostate Cancer: Implications for Genetic Testing in Prostate Cancer Patients. Br J Cancer (2011) 105:1230–4. doi: 10.1038/bjc.2011.383
4. van Asperen CJ, Brohet RM, Meijers-Heijboer EJ, Hoogerbrugge N, Verhoef S, Vasen HFA, et al. Cancer Risks in BRCA2 Families: Estimates for Sites Other Than Breast and Ovary. J Med Genet (2005) 42:711–9. doi: 10.1136/jmg.2004.028829
6. Lynparza (Olaparib) Tablets [Prescribing Information]. Wilmington: DE: AstraZeneca Pharmaceuticals (2021).
7. Abida W, Patnaik A, Campbell D, Shapiro J, Bryce AH, McDermott R, et al. Rucaparib in Men With Metastatic Castration-Resistant Prostate Cancer Harboring a BRCA1 or BRCA2 Gene Alteration. J Clin Oncol (2020) 38:3763–72. doi: 10.1200/JCO.20.01035
8. de Bono J, Mateo J, Fizazi K, Saad F, Shore N, Sandhu S, et al. Olaparib for Metastatic Castration-Resistant Prostate Cancer. N Engl J Med (2020) 382:2091–102. doi: 10.1056/NEJMoa1911440
9. Messina C, Cattrini C, Soldato D, Vallome G, Caffo O, Castro E, et al. BRCA Mutations in Prostate Cancer: Prognostic and Predictive Implications. J Oncol (2020) 2020:4986365. doi: 10.1155/2020/4986365
10. NCCN Clinical Practice Guidelines in Oncology. Prostate Cancer (Version 4.2022) National Comprehensive Cancer Network (NCCN) (2022). Available at: https://www.nccn.org/professionals/physician_gls/pdf/prostate.pdf (Accessed May 11, 2022).
11. Gillessen S, Attard G, Beer TM, Beltran H, Bjartell A, Bossi A, et al. Management of Patients Wth Advanced Prostate Cancer: Report of the Advanced Prostate Cancer Consensus Conference 2019. Eur Urol (2020) 77:508–47. doi: 10.1016/j.eururo.2020.01.012
12. Giri VN, Knudsen KE, Kelly WK, Cheng HH, Cooney KA, Cookson MS, et al. Implementation of Germline Testing for Prostate Cancer: Philadelphia Prostate Cancer Consensus Conference 2019. J Clin Oncol (2020) 38:2798–811. doi: 10.1200/JCO.20.00046
13. Pujol P, Barberis M, Beer P, Friedman E, Piulats JM, Capoluongo ED, et al. Clinical Practice Guidelines for BRCA1 and BRCA2 Genetic Testing. Eur J Cancer (2021) 146:30–47. doi: 10.1016/j.ejca.2020.12.023
14. Ross JS, Ali SM, Wang K, Palmer G, Yelensky R, Lipson D, et al. Comprehensive Genomic Profiling of Epithelial Ovarian Cancer by Next Generation Sequencing-Based Diagnostic Assay Reveals New Routes to Targeted Therapies. Gynecol Oncol (2013) 130:554–9. doi: 10.1016/j.ygyno.2013.06.019
15. Oliveira KCS, Ramos IB, Silva JMC, Barra WF, Riggins GJ, Palande V, et al. Current Perspectives on Circulating Tumor DNA, Precision Medicine, and Personalized Clinical Management of Cancer. Mol Cancer Res (2020) 18:517–28. doi: 10.1158/1541-7786.MCR-19-0768
16. Brierley JD, Gospodarowicz MK, Wittekind C. (eds) TNM Classification of Malignant Tumours. Eighth ed. Chichester, West Sussex, UK: John Wiley & Sons, Ltd (2017).
17. Amin MB, Edge SB, Greene FL, Byrd DR, Brookland RK, Washington MK, et al. (eds) AJCC Cancer Staging Manual. 8th ed. New York, NY, USA: Springer (2017).
18. Landrum MJ, Lee JM, Benson M, Brown GR, Chao C, Chitipiralla S, et al. ClinVar: Improving Access to Variant Interpretations and Supporting Evidence. Nucleic Acids Res (2018) 46:D1062–D7. doi: 10.1093/nar/gkx1153
19. Dehghani M, Rosenblatt KP, Li L, Rakhade M, Amato RJ. Validation and Clinical Applications of a Comprehensive Next Generation Sequencing System for Molecular Characterization of Solid Cancer Tissues. Front Mol Biosci (2019) 6:82. doi: 10.3389/fmolb.2019.00082
20. Clark TA, Chung JH, Kennedy M, Hughes JD, Chennagiri N, Lieber DS, et al. Analytical Validation of a Hybrid Capture–Based Next-Generation Sequencing Clinical Assay for Genomic Profiling of Cell-Free Circulating Tumor DNA. J Mol Diagn (2018) 20:686–702. doi: 10.1016/j.jmoldx.2018.05.004
21. Pritchard CC, Mateo J, Walsh MF, De Sarkar N, Abida W, Beltran H, et al. Inherited DNA-Repair Gene Mutations in Men With Metastatic Prostate Cancer. N Engl J Med (2016) 375:443–53. doi: 10.1056/NEJMoa1603144
22. Abida W, Campbell D, Patnaik A, Sautois B, Shapiro JD, Vogelzang NJ, et al. Genomic Characteristics Associated With Clinical Activity of Rucaparib in Patients (Pts) With BRCA1 or BRCA2 (BRCA)-Mutated Metastatic Castration-Resistant Prostate Cancer (mCRPC). J Clin Oncol (2020) 38:abst 178. doi: 10.1200/JCO.2020.38.6_suppl.178
23. Tukachinsky H, Madison RW, Chung JH, Gjoerup OV, Severson EA, Dennis L, et al. Genomic Analysis of Circulating Tumor DNA in 3,334 Patients With Advanced Prostate Cancer Identifies Targetable BRCA Alterations and AR Resistance Mechanisms. Clin Cancer Res (2021) 27:3094–105. doi: 10.1158/1078-0432.CCR-20-4805
24. Abida W, Armenia J, Gopalan A, Brennan R, Walsh M, Barron D, et al. Prospective Genomic Profiling of Prostate Cancer Across Disease States Reveals Germline and Somatic Alterations That May Affect Clinical Decision Making. JCO Precis Oncol (2017) 1:1–16. doi: 10.1200/po.17.00029
25. Robinson D, Van Allen Eliezer M, Wu Y-M, Schultz N, Lonigro Robert J, Mosquera J-M, et al. Integrative Clinical Genomics of Advanced Prostate Cancer. Cell (2015) 161:1215–28. doi: 10.1016/j.cell.2015.05.001
26. Abida W, Patnaik A, Campbell D, Shapiro J, Bryce AH, McDermott R, et al. Clinical Activity of Rucaparib in Patients With Metastatic Castration-Resistant Prostate Cancer (mCRPC) and BRCA1 or BRCA2 Mutations Identified by FoundationOne® Liquid CDx (F1L CDx). Prostate Cancer Foundation (2020). doi: 10.26226/morressier.5f69edb69b74b699bf38c657
27. Chi KN, Barnicle A, Sibilla C, Lai Z, Corcoran C, Williams JA, et al. Concordance of BRCA1, BRCA2 (BRCA), and ATM Mutations Identified in Matched Tumor Tissue and Circulating Tumor DNA (ctDNA) in Men With Metastatic Castration-Resistant Prostate Cancer (mCRPC) Screened in the PROfound Study. J Clin Oncol (2021) 39(suppl 6):abst 26. doi: 10.1200/JCO.2021.39.6_suppl.26
28. Hegemann M, Stenzl A, Bedke J, Chi KN, Black PC, Todenhöfer T. Liquid Biopsy: Ready to Guide Therapy in Advanced Prostate Cancer? BJU Int (2016) 118:855–63. doi: 10.1111/bju.13586
29. Frenel JS, Carreira S, Goodall J, Roda D, Perez-Lopez R, Tunariu N, et al. Serial Next-Generation Sequencing of Circulating Cell-Free DNA Evaluating Tumor Clone Response to Molecularly Targeted Drug Administration. Clin Cancer Res (2015) 21:4586–96. doi: 10.1158/1078-0432.CCR-15-0584
30. Hovelson DH, Tomlins SA. The Role of Next-Generation Sequencing in Castration Resistant Prostate Cancer Treatment. Cancer J (2016) 22:357–61. doi: 10.1097/PPO.0000000000000217
31. Beltran H, Rubin MA. New Strategies in Prostate Cancer: Translating Genomics Into the Clinic. Clin Cancer Res (2013) 19:517–23. doi: 10.1158/1078-0432.ccr-12-1452
32. Bowman RL, Busque L, Levine RL. Clonal Hematopoiesis and Evolution to Hematopoietic Malignancies. Cell Stem Cell (2018) 22:157–70. doi: 10.1016/j.stem.2018.01.011
33. Stetson D, Ahmed A, Xu X, Nuttall BRB, Lubinski TJ, Johnson JH, et al. Orthogonal Comparison of Four Plasma NGS Tests With Tumor Suggests Technical Factors are a Major Source of Assay Discordance. JCO Precis Oncol (2019) 3:1–9. doi: 10.1200/po.18.00191
34. Jensen K, Konnick EQ, Schweizer MT, Sokolova AO, Grivas P, Cheng HH, et al. Association of Clonal Hematopoiesis in DNA Repair Genes With Prostate Cancer Plasma Cell-Free DNA Testing Interference. JAMA Oncol (2021) 7:107–10. doi: 10.1001/jamaoncol.2020.5161%JJAMAOncology
35. Beware Liquid Biopsies to Guide PARP Blockade. Cancer Discovery (2021) 11:6. doi: 10.1158/2159-8290.Cd-nb2020-105
36. Reichert ZR, Jones MA, Alumkal JJ. A CHIP in the Armor of Cell-Free DNA–based Predictive Biomarkers for Prostate Cancer. JAMA Oncol (2021) 7:111–2. doi: 10.1001/jamaoncol.2020.5140%JJAMAOncology
37. Lu YT, Delijani K, Mecum A, Goldkorn A. Current Status of Liquid Biopsies for the Detection and Management of Prostate Cancer. Cancer Manag Res (2019) 11:5271–91. doi: 10.2147/CMAR.S170380
38. Huang C-C, Du M, Wang L. Bioinformatics Analysis for Circulating Cell-Free DNA in Cancer. Cancers (2019) 11:805. doi: 10.3390/cancers11060805
Keywords: plasma, BRCA, prostate cancer, PARP inhibitor, case report, poly(ADP-ribose) polymerase
Citation: Petrylak DP, Watkins SP and Loehr A (2022) What Plasma Can Tell Us When Tissue Cannot: A Case Report of Genomic Testing in mCRPC and Clinical Response to Treatment With the PARP Inhibitor Rucaparib . Front. Oncol. 12:951348. doi: 10.3389/fonc.2022.951348
Received: 23 May 2022; Accepted: 20 June 2022;
Published: 01 August 2022.
Edited by:
Marijo Bilusic, University of Miami Health System, United StatesReviewed by:
Melissa A. Reimers, Washington University in St. Louis, United StatesDawid Sigorski, University of Warmia and Mazury in Olsztyn, Poland
Nobuyuki Takahashi, National Cancer Center Hospital East, Japan
Adam Kase, Mayo Clinic Florida, United States
Copyright © 2022 Petrylak, Watkins and Loehr. This is an open-access article distributed under the terms of the Creative Commons Attribution License (CC BY). The use, distribution or reproduction in other forums is permitted, provided the original author(s) and the copyright owner(s) are credited and that the original publication in this journal is cited, in accordance with accepted academic practice. No use, distribution or reproduction is permitted which does not comply with these terms.
*Correspondence: Andrea Loehr, YWxvZWhyQGNsb3Zpc29uY29sb2d5LmNvbQ==