- Department of Gynecological Oncology, Beijing Obstetrics and Gynecology Hospital, Capital Medical University, Beijing Maternal and Child Health Care Hospital, Beijing, China
Cervical cancer is the second most common gynecological malignant tumor endangering the health of women worldwide. Despite advances in the therapeutic strategies available to treat cervical cancer, the long-term prognosis of patients with recurrent and metastatic cervical cancer remains unsatisfactory. In recent years, immune checkpoint inhibitors (ICIs) have shown encouraging efficacy in the treatment of cervical cancer. ICIs have been approved for use in both first- and second-line cervical cancer therapies. This review summarizes the current knowledge of ICIs and the application of ICIs in clinical trials for the treatment of cervical cancer.
1 Introduction
Cervical cancer is the fourth most common malignant tumor among women in the world in terms of morbidity and mortality (1). According to the latest global cancer data released by the World Health Organization’s International Agency for Research on Cancer (IARC), it is estimated that there were 604,000 new cases of cervical cancer associated with 342,000 deaths in 2020 worldwide. They account for 6.5% and 7.7%, respectively, of new cancer cases and deaths among women worldwide (2).
Generally, the prognosis of early-stage cervical cancer is good, with an overall 5-year survival rate of approximately 70%-90% (3). However, the prognosis of locally advanced cervical cancer and recurrent/metastatic cervical cancer is still poor (4). For patients with locally advanced cervical cancer (FIGO 2018 stages IB3-IVA), concomitant cisplatin-based chemoradiation followed by intrauterine brachytherapy is both recommended by the European Society of Gynecological Oncology (ESGO) and the National Comprehensive Cancer Network (NCCN) (5, 6), but the prognosis is relatively poor, with a 5-year survival rate of approximately 65% (7). The main treatments for patients with recurrent or metastatic cervical cancer are surgery, radiotherapy, and chemotherapy. Unfortunately, the 5-year survival rate for women with recurrent or metastatic cervical cancer is only 16.5% (8, 9).
Breakthroughs in advanced cervical cancer treatments have been slow. It becomes more difficult to further prolong the survival time of patients with radiotherapy and chemotherapy. In recent years, targeted therapy and immunotherapy have emerged to treat recurrent and metastatic cervical cancer. In particular, immune checkpoint inhibitors (ICIs) have greatly improved the survival of patients with cervical cancer in several clinical studies, thus providing new hope for patients with metastatic and recurrent cervical cancer. In this narrative review, we searched the PubMed database, abstracts of major scientific meetings, and clinical trials database (ClinicalTrials.gov) up to July 2022 to provide an overview of the use of ICIs in the treatment of advanced cervical cancer, including its mechanism of action and relevant clinical trials.
2 ICIs in cervical cancer
2.1 ICIs and cancer immunotherapy
With the advancement of tumor immunology research in the last two decades, immunotherapy has emerged as the most promising anti-cancer strategy. The anticancer immune response can be described as the “cancer immunity cycle” (Figure 1) (10). The cancer cell death caused by chemotherapy, radiotherapy, and targeted therapy leads to the release of neoantigens created by oncogenesis, which are presented by antigen presenting cells (APC) to effector T cells. Effector T cells are then activated in lymph nodes and travel to infiltrate the tumor, specifically to recognize and kill their target cancer cell. Immunotherapy can target specific steps in the “cancer immunity cycle” and stimulate the immune system to kill cancer cells.
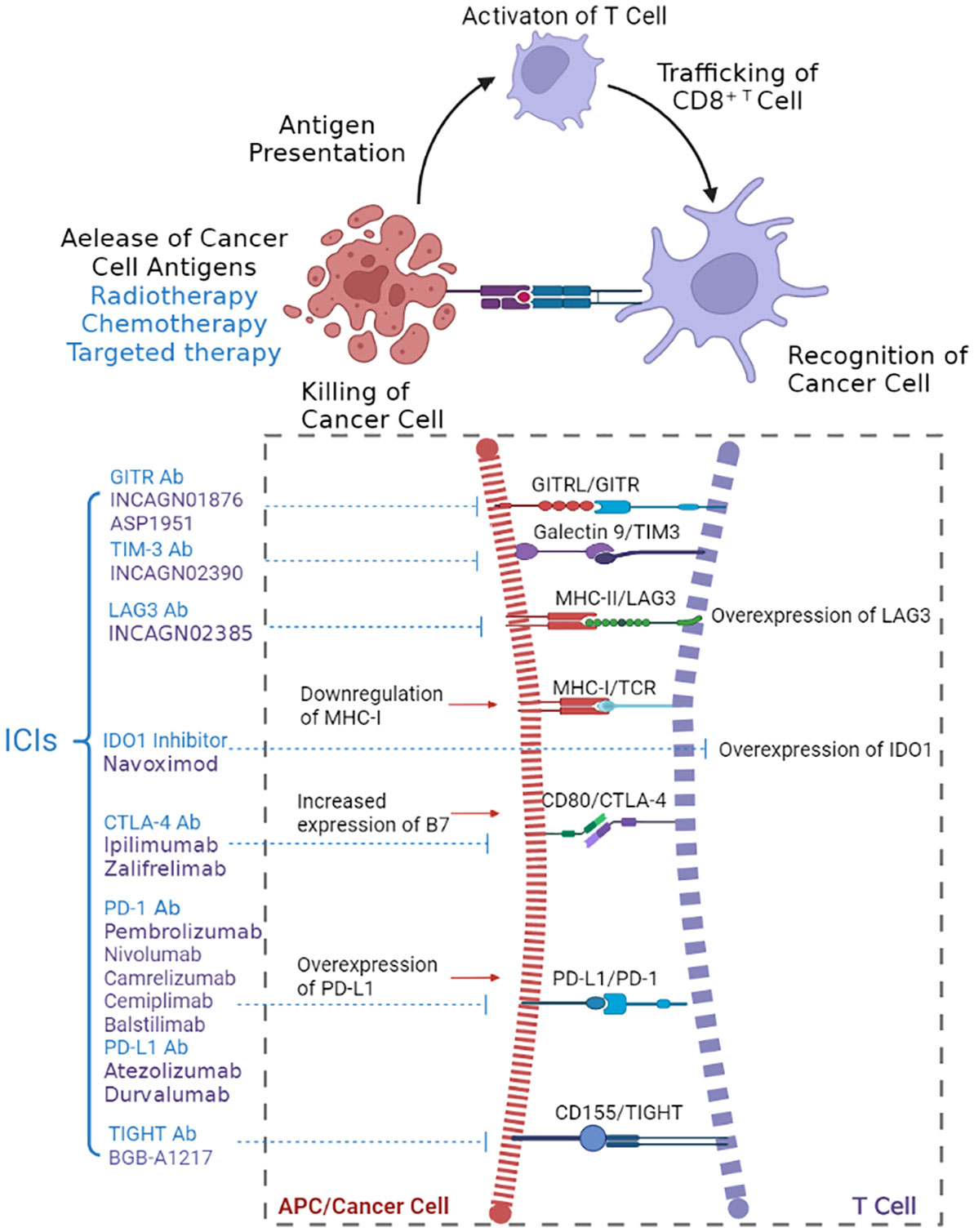
Figure 1 Mechanism of action of ICIs in the anticancer immune response. Created with ©BioRender.
However, cancer cells develop a variety of mechanisms to evade the identification and eradication of immune cells (11). Immune checkpoints play a key role in the immune escape of tumor cells. Immune checkpoints are immunosuppressive molecules that function as negative regulators to regulate the immune response, which are important pathways for the immune system to avoid self-immune response (12, 13). The most studied immune checkpoints in cervical cancer include programmed death 1/programmed death ligand-1 (PD-1/PD-L1) and cytotoxic T lymphocyte antigen (CTLA-4). PD-1 is a trans-membrane protein expressed on a variety of immune cells, including T cells, B cells, monocytes, natural killer cells, and dendritic cells (11, 14–16). PD-1 in T cells binds to overexpressed PD-L1 in tumor cells, leading to the immunosuppressive activity of T cells (17). CTLA-4 protein is a T cell surface receptor constitutively expressed on regulatory T cells (Tregs) and binds to its ligands (CD80 and CD86). CTLA-4 is believed to inhibit T-cell proliferation by blunting the antigen presenting cells thus providing negative feedback in the immune response (12, 18).Other immune checkpoints being studied in cervical cancer include indoleamine 2,3-dioxygenase 1 (IDO1), lymphocyte-activation gene 3 (LAG3), T-cell immunoglobulin and mucin domain-containing protein 3 (TIM3), glucocorticoid-induced TNFR-related protein (GITR), and T cell immunoreceptor with Ig and ITIM domains (TIGHT). IDO1 is a rate-limiting metabolic enzyme overexpressed in T cells of tumor patients. IDO1 can inhibit the function of CD8+ effector T cells and natural killer (NK) cells in the tumor microenvironment, mediating potent immunosuppressive effects in cancer (19, 20). LAG3 is an inhibitory receptor that is overexpressed on T cells in many types of cancer and can negatively regulate T cell function (21). GITR is a transmembrane protein expressed on Treg, CD4+ and CD8+ T cells, and B cells, inducing responder T cell resistance to Treg-mediated suppression (22).TIM3, and TIGHT are two other inhibitory receptors that are expressed in multiple immune cells and can also regulate the immune response through complex mechanisms (23, 24). TIM3 can mediate inhibition of T cell proliferation, reduction in cytokine production via TIM3/Gal9 signaling pathway (25). TIGHT binds to CD155, which expressed on multiple cell types, and induces a tolerogenic phenotype in T cells (24).
Given the role of immune checkpoints in immunosuppression, targeted blockage of immune checkpoint molecules pathway would theoretically enhance the anti-tumor effect of immune cells. ICIs are antibodies targeting immune checkpoint molecules that negatively regulate the anticancer immune response and have been approved as first- or second-line therapies for multiple solid tumors (26, 27). With the advance of clinical research, ICIs have changed the clinical practice of cervical cancer.
2.2 Biomarkers for ICI immunotherapy
PD-L1 has been reported to be a new biomarker of cervical cancer, and its expression has been shown to be related to the response to ICI therapy in multiple solid tumors (28, 29). Studies have shown that PD-L1 is overexpressed in cervical intraepithelial neoplasia and cervical cancer but not in normal cervical tissue (30, 31). The PD-L1 expression rates in cervical squamous cell carcinoma (SCC) and cervical adenocarcinoma (ADC) are 55-85% and 64%, respectively (32). In addition, studies have shown a significant association between HPV positivity and PD-L1 expression (31, 33). Liu et al. (33) reported that there was a positive correlation between HPV E7 oncoprotein and PD-L1 expression in cervical cancer. Additionally, PD-L1 can also be widely observed in tumor-invading lymphocytes (TILs) of cervical cancer (34).
Tumor mutation burden (TMB) is a numeric index used to evaluate the number of mutations in a neoplasm (35). As many studies have shown correlations between TMB and response to ICI therapies in multiple tumors (36, 37), TMB has been used as a biomarker to predict the response to immune checkpoint inhibitor treatment (38). In 2020, TMB was approved as a companion diagnostic for the use of pembrolizumab in the treatment of patients with advanced or metastatic solid cancers (39). Data from the KEYNOTE-158 trial showed that the proportion of TMB-H cases for cervical cancer is approximately 15%, and TMB-H cervical cancer tumors showed a trend toward better response rates (36). In turn, McGrail et al. (40) analyzed the data from The Cancer Genome Atlas, which included 21 cervical cancer patients, and obtained a negative outcome. Therefore, the predictive value of TMB in ICI treatment of cervical cancer needs further study.
The DNA mismatch repair (MMR) system is a critical mechanism for identifying and repairing mismatched nucleotides (41). Microsatellites are short, repetitive elements in genomic DNA. The microsatellite repeat number may be altered due to mismatch repair deficiency (dMMR), which is called microsatellite instability (MSI) (42, 43). Deficiency in DNA repair mechanisms results in a hypermutated genome, making it a surrogate marker of TMB, as well as a predictive biomarker for ICI therapy. Patients with MSI/dMMR showed a lasting response to pablizumab in multiple clinical trials (28, 44–46), but the proportion of MSI mutations in cervical cancer is only approximately 3-%-11.3%, as shown in previous studies (47, 48).
In addition, dMMR may lead to mutation of the DNA polymerase gene ϵ/delta 1 (POLE/POLD1) (49), which is another potential biomarker for ICI therapy. Wang et al. (50) analyzed the data from 47,721 patients with multiple cancer types and found that patients with POLE/POLD1 mutations have a significantly longer median overall survival (mOS) than the wild-type population (34 m vs. 18 m log-rank test, χ2 = 8.4; P = 0.004). However, there is no research on the mutation of POLE/POLD1 in cervical cancer. In addition, Qu’s study identified a novel six-gene (APOC1, GLTP, ISG20, SPP1, SLC24A3 and UPP1) signature using bioinformatics technology to predict the response of patients with cervical cancer to ICI therapy (51).
The phenotype of tumor-infiltrating lymphocytes (TILs) was also associated with the ICI therapy response. In a study on T-cell subsets in cervical cancer, Heeren et al. (52) found that activated regulatory T-cell (aTreg) rates were higher in anti-PD-1 nonresponders, while the levels of CD8FoxP3CD25 T cells were elevated in responders.
3 Clinical research progress of ICIs in cervical cancer
3.1 ICI used in the treatment of cervical cancer
A variety of ICIs have been reported to be used in clinical research of cervical cancer since 2015. These ICIs include anti-PD-1 antibodies [pembrolizumab (53–55), nivolumab (56–58), camrelizumab (59), cemiplimab (60), and balstilimab (61)], anti-PD-L1 antibodies [atezolizumab (62) and durvalumab (63)], anti-CTLA4 antibodies [ipilimumab (64) and zalifrelimab (65)], anti-transforming growth factor-β (TGF-β)/PD-L1 bispecific antibodies [M7824/bintrafusp alfa (66)], IDO1 inhibitors [navoximod (67)] and anti-NKG2A antibodies [monalizumab (68)]. Other novel ICIs that have been registered for clinical research include the anti-PD-1 antibodies tislelizumab (NCT04693234), the anti-GITR antibodies ASP1951 (NCT03799003) and INCAGN01876 (NCT03126110), the anti-TIM-3 antibody INCAGN02390 (NCT03652077), the anti-LAG-3 antibody INCAGN02385 (NCT03538028), and the anti-TIGIT antibody BGB-A1217 (NCT04693234). Among those ICIs, pembrolizumab and nivolumab have been recommended by the NCCN guidelines for the treatment of cervical cancer.
3.2 ICI monotherapy in cervical cancer
Several studies have explored the role of ICI monotherapy in recurrent or metastatic cervical cancer, as shown in Table 1. However, ICI monotherapy in cervical cancer did not show satisfactory results in most previous studies.
KEYNOTE-158 is a phase II basket study that included 98 patients with advanced cervical cancer. Patients in the study received 3 weekly doses of pembrolizumab 200 mg for up to 2 years. All responses were only seen in patients with PD-L1-positive tumors, for an overall response rate (ORR) of 14.6% (95% CI, 7.8% to 24.2%) (53). In the Checkmate-358 study, nivolumab showed an ORR of 26.3% (95% CI: 9.1%–51.2%) in patients with advanced cervical cancer (58). Another phase II study reported by Kenji Tamura showed that the ORR of nivolumab in advanced cervical cancer patients with PD-L1-positive tumors was 33% (80% CI: 17%–53%) (57).Based on the results of the above studies, the NCCN guidelines recommended pembrolizumab as a second-line regimen indicated for recurrent PD-L1-positive or MSI-H/dMMR cervical cancer in 2020 (71) and added nivolumab as the second-line therapy for PD-L1-positive cervical cancer in 2022 (5).
The latest research (EMPOWER-Cervical 1/GOG-3016/ENGOT-cx9) published in the New England Journal of Medicine is the only and largest phase III clinical trial on ICI monotherapy in cervical cancer (69). A total of 608 patients with advanced cervical cancer were included in this study and received either cemiplimab 350 mg every 3 weeks or chemotherapy (pemetrexed, vinorelbine, gemcitabine, irinotecan or topotecan). The results showed that in the overall trial population, mOS was longer in the cemiplimab group than in the chemotherapy group (12.0 m vs. 8.5 m; hazard ratio for death: 0.69; 95% CI: 0.56–0.84; P<0.001). The ORR of the patients in the cemiplimab group was 16.4% (95% CI, 12.5%– 21.1%), compared with 6.3% (95% CI, 3.8%-9.6%) in the chemotherapy group (69). Another phase II clinical trial evaluated the antitumor activity of balstilimab in patients with recurrent/metastatic cervical cancer. This study included 140 patients, and the ORR was 15% (95% CI, 10.0%-21.8%) (61). These studies showed that cemiplimab may be another promising ICI for the treatment of cervical cancer.
It is worth noting that some monotherapy with bispecific antibodies has shown a better effect in cervical cancer. An analysis of data from two trials (NCT02517398/NCT03427411) provided encouraging outcomes for the use of bintrafusp alfa (anti-TGF-ß/PD-L1 bispecific antibody) in advanced cervical cancer, with 30% (10/33) of patients having a clinical response (66). A larger clinical trial (NCT04246489) of bintrafusp alfa monotherapy in patients with advanced, unresectable cervical cancer is underway. Furthermore, some completed studies (NCT03652077, NCT03538028, NCT03126110) about other novel ICIs may provide more encouraging results.
3.3 Combinations of different ICIs: Are two better than one
The anticancer immune response is a complex physiological process, which involves antigen presentation, activation of T cells, and recognition of cancer cells (10). Various types of immune checkpoints may mediate immunosuppression through different and complementary pathways, which may result in low response rates to ICI monotherapy. Virous ICIs and immunotherapy play a role in the steps of the “cancer immunity cycle” because of their different targets. Therefore, the combined use of multiple ICIs and ICIs combined with other immunotherapies can theoretically further exert the antitumor effect of immunotherapy. A number of clinical trials have been conducted to evaluate the efficacy of two ICI combinations and ICIs combined with other immunotherapies in the treatment of cervical cancer (Table 2).
The C550 study is the largest reported phase II study that evaluated the antitumor activity of the combination of balstilimab (an anti-PD-1 antibody) with zalifrelimab (an anti-CTLA-4 antibody) as a second-line therapy for advanced cervical cancer. A total of 125 patients with recurrent or metastatic cervical cancer were included and were followed up for 21 months. The confirmed ORR was 25.6% (95% CI, 18.8%-33.9%), with an ORR of 32.8% in patients with PD-L1-positive tumors (74). The Checkmate-358 study evaluated the effectiveness of the combination of nivolumab with ipilimumab. A total of 91 patients with recurrent or metastatic cervical cancer were randomized 1:1 to either nivolumab (3 mg/kg/2 weeks) + ipilimumab (1 mg/kg/6 weeks) (nivo3 + ipi1) or nivolumab (1 mg/kg/2 weeks) + ipilimumab (3 mg/kg/3 weeks for 4 doses) followed by nivolumab (240 mg/2 weeks) (nivo1 + ipi3), and obtained an ORR of 34.1% for all populations (73). The above studies suggest that double ICIs may be a promising therapy for advanced cervical cancer. Some studies (NCT04693234, NCT03799003) on the combination of anti-PD-1 and anti-TIGHT antibodies or with anti-GITR antibody are underway. At the same time, some dual ICIs, such as AK-104 (anti-PD-1/CTLA-4 bispecific antibody), have been registered for clinical research on cervical cancer (NCT04380805; NCT05063916; NCT05235516; NCT04982237; NCT04868708).
Other studies have evaluated the antitumor activity of ICIs combined with another immunotherapy in cervical cancer, including therapeutic cancer vaccines and tumor-infiltrating lymphocyte (TIL) therapy. The interim results of a phase II clinical study published in Lancet Oncology revealed the efficacy of ICIs combined with a therapeutic cervical cancer vaccine in advanced cervical cancer. Twenty-six patients in that study received seven doses of the 2 mg GX-188Evaccine (a therapeutic HPV DNA vaccine) in addition to pembrolizumab 200 mg every 3 weeks and were evaluable for interim activity assessment. The results showed that 11 of 26 patients achieved an overall response (ORR=42%; 95% CI 23-63) (72). TIL therapy is an adoptive cell therapy (adoptive cell therapy, ACT), in which tumor-infiltrating lymphocytes are isolated, cultured and expanded and then injected into patients to exert their antitumor activity (76). A phase I study investigated the efficacy of TIL and anti-PD1 combination therapy in patients with advanced cervical cancer. An ORR was observed in 20 (25.0%) out of 80 patients with PD-L1-negative and low MSI expression tumors (75). Further phase II studies are needed to evaluate the efficacy and safety of TIL (LN-145) and pembrolizumab combination therapy in patients with advanced cervical carcinoma. These studies are still in their infancy, but they have provided hope for the application of immunotherapy in cervical cancer. In most studies, combination therapy showed a better anti-tumor activity, but whether the combination therapy will cause more treatment emergent adverse events (TEAEs) remains to be further studied.
3.4 Combinations of ICIs with chemotherapy, antiangiogenic therapy, radiotherapy, and targeted therapy: The future trend
In view of the unsatisfactory response rate of ICI monotherapy, ICIs are usually used in combination with other therapies. The combinations of ICIs with radiotherapy, chemotherapy, antiangiogenic therapy, and targeted therapy have improved the ORR of cervical cancer in a number of clinical trials (Table 3).
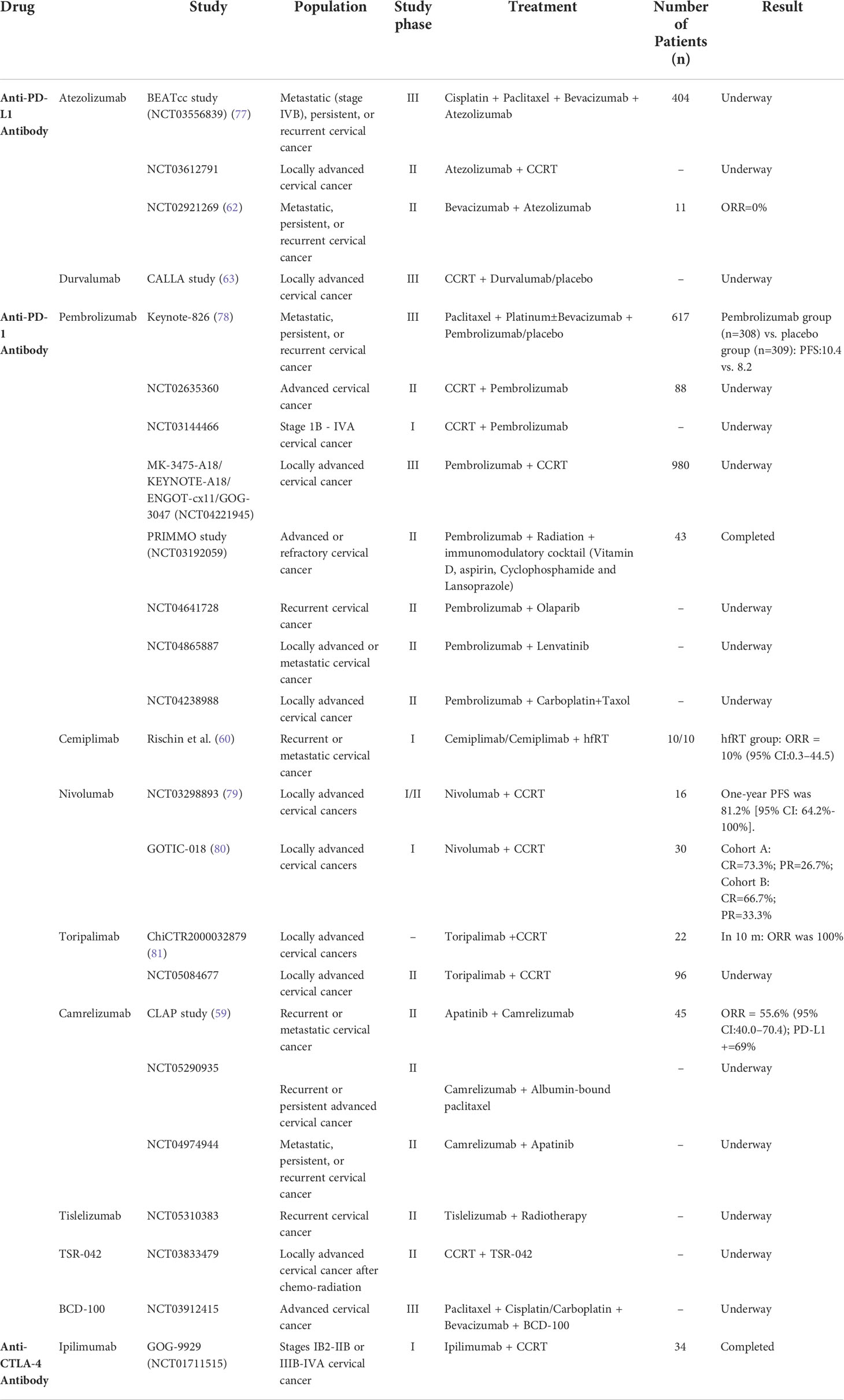
Table 3 Trials combining the use of ICIs with radiotherapy, chemotherapy, antiangiogenic therapy, and targeted therapy.
3.4.1 ICI + chemotherapy
The combinations of ICIs with chemotherapy may enhance the anti-cancer activity of ICIs in two ways. First, chemotherapies may stimulate the immune system by triggering antigen release via cytotoxic cell death activity (82). In addition, chemotherapies may impact the activation status of immunocytes in the tumor microenvironment (83). In published clinical trials, the combination of ICI with chemotherapy has shown better anti-tumor activity than chemotherapy alone.The Keynote-826 study (NCT03635567) is the only published phase III clinical study of ICIs combined with chemotherapy and anti-angiogenesis in cervical cancer. The results were published in the New England Journal of Medicine in 2021. A total of 617 patients with persistent, recurrent, or metastatic cervical cancer were randomly assigned in a 1:1 ratio to receive pembrolizumab (n=308) or placebo (n=309) plus platinum-based chemotherapy and, per investigator discretion, bevacizumab. Among 548 patients with a PD-L1 combined positive score (CPS) ≥1, the OS was significantly longer in the pembrolizumab group than in the placebo group (24-month estimate of patients alive, 53.0% [95% CI, 46.0 to 59.4] vs. 41.7% [95% CI, 34.9 to 48.2]; hazard ratio for death, 0.64; 95% CI, 0.50 to 0.81; P<0.001), and the mPFS was 10.4 months in the pembrolizumab group and 8.2 months in the placebo group (78). Based on the exciting results of this study, the NCCN guideline (2022. V1) recommended pembrolizumab + chemotherapy, with or without bevacizumab as the first-line therapy for patients with PD-L1-positive metastatic, persistent, and recurrent cervical cancer (5). Another large phase III clinical study (BEATcc study) evaluating the efficacy of atezolizumab combined with cisplatin-paclitaxel plus bevacizumab in the treatment of cervical cancer is underway (77). In the second-line treatment of cervical cancer, camrelizumab combined with albumin-bound paclitaxel has been used in a study of recurrent or persistent advanced cervical cancer patients after first-line chemotherapy (NCT05290935).
3.4.2 ICI + radiotherapy
Similar to the effects of chemotherapy, radiotherapy turns the immunologically ‘cold’ tumors ‘hot’ and enhances the anti-tumor response of the immune system by triggering the release of neoantigens and increasing tumor-infiltrating immunostimulatory cells (84, 85). However, further large-scale studies of ICIs combined with radiotherapies for cervical cancer are still needed. Rischin et al. (60) reported phase I clinical trials that evaluated the antitumor activity of cemiplimab as a monotherapy or in combination with hypofractionated radiation therapy (hfRT) in patients with recurrent or metastatic cervical cancer, with an ORR of 10% for 10 patients in the hfRT group. Other than that, no clinical study results have been reported for the combination of ICIs with radiotherapy in advanced cervical cancer. However, there are several registered studies evaluating the efficacy of ICIs + concurrent chemoradiotherapy (CCRT) or ICIs + radiotherapy in advanced cervical cancer (NCT03612791, CALLA study, NCT02635360, NCT03144466, MK-3475-A18/KEYNOTE-A18/ENGOT-cx11/GOG-3047, NCT03298893, NCT05310383, NCT03833479, NCT01711515) (Table 3).
The interim results of several studies have been reported on the 2022 American Society of Clinical Oncology (ASCO) annual meeting to assess the efficacy and safety of the combination of ICIs with concurrent chemoradiation (CCRT) in patients with locally advanced cervical cancer (GOTIC-018, ChiCTR2000032879, NCT03298893) (79–81). In the NiCOL phase I trial, nivolumab+CCRT showed good efficacy in locally advanced cervical cancer, with a one-year PFS of 81.2% (95% CI: 64.2%-100%) (79). A trial carried out in China showed that toripalimab + CCRT has promising antitumor activity in patients with locally advanced cervical cancer, and the ORR was 100% for 22 patients during the ten-month follow-up period (81). Meanwhile, a phase II study of toripalimab combined with CCRT in locally advanced cervical cancer is underway (NCT05084677). With the release of the research results, ICIs combined with CCRT might represent a novel treatment option for patients with locally advanced cervical cancer.
3.4.3 ICI + antiangiogenic therapy
Although many studies have reported that antiangiogenic drugs can improve the efficacy of ICIs by enhancing antigen presentation, activating cytotoxic CD8 T cells, and promoting lymphocyte infiltration into tumors (86–89).The clinical efficacy of antiangiogenic therapy combined with ICIs in the treatment of cervical cancer remains to be verified. In the reported phase II studies, atezolizumab combined with bevacizumab did not show antitumor activity in advanced cervical cancer (62). However, camrelizumab + apatinib showed satisfactory results in a multicenter, open-label, single-arm, phase II study (CLAP study), with an ORR of 55.6% (95% CI: 40.0–70.4) (59). In some ongoing studies, ICIS plus poly ADP-ribose polymerase (PARP) inhibitors have been used as the second-line treatment of advanced cervical cancer (NCT04641728).
4 Conclusion
In recent years, a large number of clinical studies have been carried out to evaluate the safety and effectiveness of immunotherapy in cervical cancer. There is no doubt that ICIs have made a breakthrough in the treatment of advanced metastatic and recurrent cervical cancer. ICIs such as pablizumab have been used in many clinical studies and have achieved encouraging results. With the publication of these clinical studies, the NCCN guidelines recommended pembrolizumab plus chemotherapy, with or without bevacizumab as the first-line therapy for patients with PD-L1-positive advanced cervical cancer, and nivolumab as the second-line therapy for PD-L1-positive cervical cancer. However, how to improve the response rate of cervical cancer to ICI therapy is still a problem worthy of further exploration. According to the existing research results, the combination of ICIs with radiotherapy, chemotherapy and other targeted drugs seems to be an effective treatment. Furthermore, we still need a more accurate model, including the biomarker status and clinical characteristics of patients, to predict the response of cervical cancer to ICIs.
Author contributions
YX and WK contributed to the conception of the study and wrote the manuscript. WK contributed. All authors contributed to the article and approved the submitted version.
Conflict of interest
The authors declare that the research was conducted in the absence of any commercial or financial relationships that could be construed as a potential conflict of interest.
Publisher's note
All claims expressed in this article are solely those of the authors and do not necessarily represent those of their affiliated organizations, or those of the publisher, the editors and the reviewers. Any product that may be evaluated in this article, or claim that may be made by its manufacturer, is not guaranteed or endorsed by the publisher.
References
1. Bray F, Ferlay J, Soerjomataram I, Siegel RL, Torre LA, Jemal A. Global cancer statistics 2018: GLOBOCAN estimates of incidence and mortality worldwide for 36 cancers in 185 countries. CA Cancer J Clin (2018) 68:394–424. doi: 10.3322/caac.21492
2. Sung H, Ferlay J, Siegel RL, Laversanne M, Soerjomataram I, Jemal A, et al. Global cancer statistics 2020: GLOBOCAN estimates of incidence and mortality worldwide for 36 cancers in 185 countries. CA Cancer J Clin (2021) 71:209–49. doi: 10.3322/caac.21660
3. Guimaraes YM, Godoy LR, Longatto-Filho A, Reis RD. Management of early-stage cervical cancer: A literature review. Cancers (Basel) (2022) 14:575. doi: 10.3390/cancers14030575
4. Wright JD, Matsuo K, Huang Y, Tergas AI, Hou JY, Khoury-Collado F, et al. Prognostic performance of the 2018 international federation of gynecology and obstetrics cervical cancer staging guidelines. Obstet Gynecol (2019) 134:49–57. doi: 10.1097/AOG.0000000000003311
5. NCCN. Clinical practice guidelines in oncology: Cervical cancer version 1.2022. Plymouth meeting. Available at: https://www.nccn.org/professionals/physician_gls/pdf/cervical.pdf.
6. Cibula D, Potter R, Planchamp F, Avall-Lundqvist E, Fischerova D, Haie Meder C, et al. The European society of gynaecological Oncology/European society for radiotherapy and Oncology/European society of pathology guidelines for the management of patients with cervical cancer. Int J Gynecol Cancer (2018) 28:641–55. doi: 10.1097/IGC.0000000000001216
7. Gennigens C, De Cuypere M, Hermesse J, Kridelka F, Jerusalem G. Optimal treatment in locally advanced cervical cancer. Expert Rev Anticancer Ther (2021) 21:657–71. doi: 10.1080/14737140.2021.1879646
8. Ferlay J, Steliarova-Foucher E, Lortet-Tieulent J, Rosso S, Coebergh JW, Comber H, et al. Cancer incidence and mortality patterns in Europe: estimates for 40 countries in 2012. Eur J Cancer (2013) 49:1374–403. doi: 10.1016/j.ejca.2012.12.027
9. Howlader N, Noone A, Krapcho M, Miller D, Brest A, Yu M, et al. SEER cancer statistics review (CSR) 1975-2017. Bethesda MD: Natl Cancer Institute (2019). Available at: https://seer.cancer.gov/csr/1975_2017/
10. Chen DS, Mellman I. Oncology meets immunology: the cancer-immunity cycle. Immunity (2013) 39:1–10. doi: 10.1016/j.immuni.2013.07.012
11. Li B, Chan HL, Chen P. Immune checkpoint inhibitors: Basics and challenges. Curr Med Chem (2019) 26:3009–25. doi: 10.2174/0929867324666170804143706
12. Pirs B, Skof E, Smrkolj V, Smrkolj S. Overview of immune checkpoint inhibitors in gynecological cancer treatment. Cancers (Basel) (2022) 14:631. doi: 10.3390/cancers14030631
13. Pardoll DM. The blockade of immune checkpoints in cancer immunotherapy. Nat Rev Cancer (2012) 12:252–64. doi: 10.1038/nrc3239
14. Thibult ML, Mamessier E, Gertner-Dardenne J, Pastor S, Just-Landi S, Xerri L, et al. PD-1 is a novel regulator of human b-cell activation. Int Immunol (2013) 25:129–37. doi: 10.1093/intimm/dxs098
15. Lim TS, Chew V, Sieow JL, Goh S, Yeong JP, Soon AL, et al. PD-1 expression on dendritic cells suppresses CD8(+) T cell function and antitumor immunity. Oncoimmunology (2016) 5:e1085146. doi: 10.1080/2162402X.2015.1085146
16. Gros A, Robbins PF, Yao X, Li YF, Turcotte S, Tran E, et al. PD-1 identifies the patient-specific CD8(+) tumor-reactive repertoire infiltrating human tumors. J Clin Invest (2014) 124:2246–59. doi: 10.1172/JCI73639
17. Sun C, Mezzadra R, Schumacher TN. Regulation and function of the PD-L1 checkpoint. Immunity (2018) 48:434–52. doi: 10.1016/j.immuni.2018.03.014
18. Liu X, Hogg GD, DeNardo DG. Rethinking immune checkpoint blockade: ‘beyond the T cell’. J Immunother Cancer (2021) 9:e001460. doi: 10.1136/jitc-2020-001460
19. Zhai L, Ladomersky E, Lenzen A, Nguyen B, Patel R, Lauing KL, et al. IDO1 in cancer: A Gemini of immune checkpoints. Cell Mol Immunol (2018) 15:447–57. doi: 10.1038/cmi.2017.143
20. Prendergast GC, Malachowski WJ, Mondal A, Scherle P, Muller AJ. Indoleamine 2,3-dioxygenase and its therapeutic inhibition in cancer. Int Rev Cell Mol Biol (2018) 336:175–203. doi: 10.1016/bs.ircmb.2017.07.004
21. Ruffo E, Wu RC, Bruno TC, Workman CJ, Vignali DAA. Lymphocyte-activation gene 3 (LAG3): The next immune checkpoint receptor. Semin Immunol (2019) 42:101305. doi: 10.1016/j.smim.2019.101305
22. Placke T, Kopp HG, Salih HR. Glucocorticoid-induced TNFR-related (GITR) protein and its ligand in antitumor immunity: functional role and therapeutic modulation. Clin Dev Immunol (2010) 2010:239083. doi: 10.1155/2010/239083
23. Wolf Y, Anderson AC, Kuchroo VK. TIM3 comes of age as an inhibitory receptor. Nat Rev Immunol (2020) 20:173–85. doi: 10.1038/s41577-019-0224-6
24. Annese T, Tamma R, Ribatti D. Update in TIGIT immune-checkpoint role in cancer. Front Oncol (2022) 12:871085. doi: 10.3389/fonc.2022.871085
25. Kandel S, Adhikary P, Li G, Cheng K. The TIM3/Gal9 signaling pathway: An emerging target for cancer immunotherapy. Cancer Lett (2021) 510:67–78. doi: 10.1016/j.canlet.2021.04.011
26. Haanen JB, Robert C. Immune checkpoint inhibitors. Prog Tumor Res (2015) 42:55–66. doi: 10.1159/000437178
27. Morad G, Helmink BA, Sharma P, Wargo JA. Hallmarks of response, resistance, and toxicity to immune checkpoint blockade. Cell (2021) 184:5309–37. doi: 10.1016/j.cell.2021.09.020
28. Bai R, Lv Z, Xu D, Cui J. Predictive biomarkers for cancer immunotherapy with immune checkpoint inhibitors. biomark Res (2020) 8:34. doi: 10.1186/s40364-020-00209-0
29. Wang X, Teng F, Kong L, Yu J. PD-L1 expression in human cancers and its association with clinical outcomes. Onco Targets Ther (2016) 9:5023–39. doi: 10.2147/OTT.S105862
30. Meng Y, Liang H, Hu J, Liu S, Hao X, Wong MSK, et al. PD-L1 expression correlates with tumor infiltrating lymphocytes and response to neoadjuvant chemotherapy in cervical cancer. J Cancer (2018) 9:2938–45. doi: 10.7150/jca.22532
31. Mezache L, Paniccia B, Nyinawabera A, Nuovo GJ. Enhanced expression of PD L1 in cervical intraepithelial neoplasia and cervical cancers. Mod Pathol (2015) 28:1594–602. doi: 10.1038/modpathol.2015.108
32. Heeren AM, Punt S, Bleeker MC, Gaarenstroom KN, van der Velden J, Kenter GG, et al. Prognostic effect of different PD-L1 expression patterns in squamous cell carcinoma and adenocarcinoma of the cervix. Mod Pathol (2016) 29:753–63. doi: 10.1038/modpathol.2016.64
33. Liu C, Lu J, Tian H, Du W, Zhao L, Feng J, et al. Increased expression of PDL1 by the human papillomavirus 16 E7 oncoprotein inhibits anticancer immunity. Mol Med Rep (2017) 15:1063–70. doi: 10.3892/mmr.2017.6102
34. Feng YC, Ji WL, Yue N, Huang YC, Ma XM. The relationship between the PD-1/PD-L1 pathway and DNA mismatch repair in cervical cancer and its clinical significance. Cancer Manag Res (2018) 10:105–13. doi: 10.2147/CMAR.S152232
35. Lawlor RT, Mattiolo P, Mafficini A, Hong SM, Piredda ML, Taormina SV, et al. Tumor mutational burden as a potential biomarker for immunotherapy in pancreatic cancer: systematic review and still-open questions. Cancers (Basel) (2021) 13:3119. doi: 10.3390/cancers13133119
36. Marabelle A, Fakih M, Lopez J, Shah M, Shapira-Frommer R, Nakagawa K, et al. Association of tumour mutational burden with outcomes in patients with advanced solid tumours treated with pembrolizumab: prospective biomarker analysis of the multicohort, open-label, phase 2 KEYNOTE-158 study. Lancet Oncol (2020) 21:1353–65. doi: 10.1016/S1470-2045(20)30445-9
37. Yarchoan M, Hopkins A, Jaffee EM. Tumor mutational burden and response rate to PD-1 inhibition. N Engl J Med (2017) 377:2500–1. doi: 10.1056/NEJMc1713444
38. Chan TA, Yarchoan M, Jaffee E, Swanton C, Quezada SA, Stenzinger A, et al. Development of tumor mutation burden as an immunotherapy biomarker: utility for the oncology clinic. Ann Oncol (2019) 30:44–56. doi: 10.1093/annonc/mdy495
39. FDA Approves Pembrolizumab for Adults FDA. And children with TMB-h solid tumors. Maryland MA: FDA (2020). Available at: https://www.fda.gov/drugs/drug-approvals-and-databases/
40. McGrail DJ, Pilie PG, Rashid NU, Voorwerk L, Slagter M, Kok M, et al. High tumor mutation burden fails to predict immune checkpoint blockade response across all cancer types. Ann Oncol (2021) 32:661–72. doi: 10.1016/j.annonc.2021.02.006
41. Zhao P, Li L, Jiang X, Li Q. Mismatch repair deficiency/microsatellite instability-high as a predictor for anti-PD-1/PD-L1 immunotherapy efficacy. J Hematol Oncol (2019) 12:54. doi: 10.1186/s13045-019-0738-1
42. Shah SN, Hile SE, Eckert KA. Defective mismatch repair, microsatellite mutation bias, and variability in clinical cancer phenotypes. Cancer Res (2010) 70:431–5. doi: 10.1158/0008-5472.CAN-09-3049
43. Baretti M, Le DT. DNA Mismatch repair in cancer. Pharmacol Ther (2018) 189:45–62. doi: 10.1016/j.pharmthera.2018.04.004
44. Overman MJ, McDermott R, Leach JL, Lonardi S, Lenz HJ, Morse MA, et al. Nivolumab in patients with metastatic DNA mismatch repair-deficient or microsatellite instability-high colorectal cancer (CheckMate 142): An open-label, multicentre, phase 2 study. Lancet Oncol (2017) 18:1182–91. doi: 10.1016/S1470-2045(17)30422-9
45. Le DT, Uram JN, Wang H, Bartlett BR, Kemberling H, Eyring AD, et al. PD-1 blockade in tumors with mismatch-repair deficiency. N Engl J Med (2015) 372:2509–20. doi: 10.1056/NEJMoa1500596
46. Marabelle A, Le DT, Ascierto PA, Di Giacomo AM, De Jesus-Acosta A, Delord JP, et al. Efficacy of pembrolizumab in patients with noncolorectal high microsatellite Instability/Mismatch repair-deficient cancer: Results from the phase II KEYNOTE-158 study. J Clin Oncol (2020) 38:1–10. doi: 10.1200/JCO.19.02105
47. Bonneville R, Krook MA, Kautto EA, Miya J, Wing MR, Chen HZ, et al. Landscape of microsatellite instability across 39 cancer types. JCO Precis Oncol (2017) 1:1–15. doi: 10.1200/PO.17.00073
48. Noh JJ, Kim MK, Choi MC, Lee JW, Park H, Jung SG, et al. Frequency of mismatch repair Deficiency/High microsatellite instability and its role as a predictive biomarker of response to immune checkpoint inhibitors in gynecologic cancers. Cancer Res Treat (2021) 54(4):1200–8. doi: 10.4143/crt.2021.828
49. Zhu M, Cui H, Zhang L, Zhao K, Jia X, Jin H. Assessment of POLE and POLD1 mutations as prognosis and immunotherapy biomarkers for stomach adenocarcinoma. Transl Cancer Res (2022) 11:193–205. doi: 10.21037/tcr-21-1601
50. Wang F, Zhao Q, Wang YN, Jin Y, He MM, Liu ZX, et al. Evaluation of POLE and POLD1 mutations as biomarkers for immunotherapy outcomes across multiple cancer types. JAMA Oncol (2019) 5:1504–6. doi: 10.1001/jamaoncol.2019.2963
51. Qu X, Shi Z, Guo J, Guo C, Qiu J, Hua K. Identification of a novel six-gene signature with potential prognostic and therapeutic value in cervical cancer. Cancer Med (2021) 10:6881–96. doi: 10.1002/cam4.4054
52. Heeren AM, Rotman J, Stam AGM, Pocorni N, Gassama AA, Samuels S, et al. Efficacy of PD-1 blockade in cervical cancer is related to a CD8+FoxP3+CD25+ T-cell subset with operational effector functions despite high immune checkpoint levels. J Immunother Cancer (2019) 7:43. doi: 10.1186/s40425-019-0526-z
53. Chung HC, Ros W, Delord JP, Perets R, Italiano A, Shapira-Frommer R, et al. Efficacy and safety of pembrolizumab in previously treated advanced cervical cancer: Results from the phase II KEYNOTE-158 study. J Clin Oncol (2019) 37:1470–8. doi: 10.1200/JCO.18.01265
54. Frenel JS, Le Tourneau C, O’Neil B, Ott PA, Piha-Paul SA, Gomez-Roca C, et al. Safety and efficacy of pembrolizumab in advanced, programmed death ligand 1-positive cervical cancer: Results from the phase ib KEYNOTE-028 trial. J Clin Oncol (2017) 35:4035–41. doi: 10.1200/JCO.2017.74.5471
55. Frumovitz M, Westin SN, Salvo G, Zarifa A, Xu M, Yap TA, et al. Phase II study of pembrolizumab efficacy and safety in women with recurrent small cell neuroendocrine carcinoma of the lower genital tract. Gynecol Oncol (2020) 158:570–5. doi: 10.1016/j.ygyno.2020.05.682
56. Santin AD, Deng W, Frumovitz M, Buza N, Bellone S, Huh W, et al. Phase II evaluation of nivolumab in the treatment of persistent or recurrent cervical cancer (NCT02257528/NRG-GY002). Gynecol Oncol (2020) 157:161–6. doi: 10.1016/j.ygyno.2019.12.034
57. Tamura K, Hasegawa K, Katsumata N, Matsumoto K, Mukai H, Takahashi S, et al. Efficacy and safety of nivolumab in Japanese patients with uterine cervical cancer, uterine corpus cancer, or soft tissue sarcoma: Multicenter, open-label phase 2 trial. Cancer Sci (2019) 110:2894–904. doi: 10.1111/cas.14148
58. Naumann RW, Hollebecque A, Meyer T, Devlin MJ, Oaknin A, Kerger J, et al. Safety and efficacy of nivolumab monotherapy in recurrent or metastatic cervical, vaginal, or vulvar carcinoma: Results from the phase I/II CheckMate 358 trial. J Clin Oncol (2019) 37:2825–34. doi: 10.1200/JCO.19.00739
59. Lan C, Shen J, Wang Y, Li J, Liu Z, He M, et al. Camrelizumab plus apatinib in patients with advanced cervical cancer (CLAP): A multicenter, open-label, single-arm, phase II trial. J Clin Oncol (2020) 38:4095–106. doi: 10.1200/JCO.20.01920
60. Rischin D, Gil-Martin M, Gonzalez-Martin A, Brana I, Hou JY, Cho D, et al. PD-1 blockade in recurrent or metastatic cervical cancer: Data from cemiplimab phase I expansion cohorts and characterization of PD-L1 expression in cervical cancer. Gynecol Oncol (2020) 159:322–8. doi: 10.1016/j.ygyno.2020.08.026
61. O’Malley DM, Oaknin A, Monk BJ, Selle F, Rojas C, Gladieff L, et al. Phase II study of the safety and efficacy of the anti-PD-1 antibody balstilimab in patients with recurrent and/or metastatic cervical cancer. Gynecol Oncol (2021) 163:274–80. doi: 10.1016/j.ygyno.2021.08.018
62. Friedman CF, Snyder Charen A, Zhou Q, Carducci MA, Buckley De Meritens A, Corr BR, et al. Phase II study of atezolizumab in combination with bevacizumab in patients with advanced cervical cancer. J Immunother Cancer (2020) 8:e001126. doi: 10.1136/jitc-2020-001126
63. Mayadev J, Nunes AT, Li M, Marcovitz M, Lanasa MC, Monk BJ. CALLA: Efficacy and safety of concurrent and adjuvant durvalumab with chemoradiotherapy versus chemoradiotherapy alone in women with locally advanced cervical cancer: A phase III, randomized, double-blind, multicenter study. Int J Gynecol Cancer (2020) 30:1065–70. doi: 10.1136/ijgc-2019-001135
64. Mayadev JS, Enserro D, Lin YG, Da Silva DM, Lankes HA, Aghajanian C, et al. Sequential ipilimumab after chemoradiotherapy in curative-intent treatment of patients with node-positive cervical cancer. JAMA Oncol (2020) 6:92–9. doi: 10.1001/jamaoncol.2019.3857
65. Bose CK. Balstilimab and other immunotherapy for recurrent and metastatic cervical cancer. Med Oncol (2022) 39:47. doi: 10.1007/s12032-022-01646-7
66. Strauss J, Gatti-Mays ME, Cho BC, Hill A, Salas S, McClay E, et al. Bintrafusp alfa, a bifunctional fusion protein targeting TGF-beta and PD-L1, in patients with human papillomavirus-associated malignancies. J Immunother Cancer (2020) 8:e001395. doi: 10.1136/jitc-2020-001395
67. Jung KH, LoRusso P, Burris H, Gordon M, Bang YJ, Hellmann MD, et al. Phase I study of the indoleamine 2,3-dioxygenase 1 (IDO1) inhibitor navoximod (GDC-0919) administered with PD-L1 inhibitor (Atezolizumab) in advanced solid tumors. Clin Cancer Res (2019) 25:3220–8. doi: 10.1158/1078-0432.CCR-18-2740
68. Tinker AV, Hirte HW, Provencher D, Butler M, Ritter H, Tu D, et al. Dose-ranging and cohort-expansion study of monalizumab (IPH2201) in patients with advanced gynecologic malignancies: A trial of the Canadian cancer trials group (CCTG): IND221. Clin Cancer Res (2019) 25:6052–60. doi: 10.1158/1078-0432.CCR-19-0298
69. Tewari KS, Monk BJ, Vergote I, Miller A, de Melo AC, Kim HS, et al. Survival with cemiplimab in recurrent cervical cancer. N Engl J Med (2022) 386:544–55. doi: 10.1056/NEJMoa2112187
70. Lheureux S, Butler MO, Clarke B, Cristea MC, Martin LP, Tonkin K, et al. Association of ipilimumab with safety and antitumor activity in women with metastatic or recurrent human papillomavirus-related cervical carcinoma. JAMA Oncol (2018) 4:e173776. doi: 10.1001/jamaoncol.2017.3776
71. Yang X, Ren H, Fu J. Combinations of radiotherapy with immunotherapy in cervical cancer. J Cancer (2022) 13:1480–9. doi: 10.7150/jca.65074
72. Youn JW, Hur SY, Woo JW, Kim YM, Lim MC, Park SY, et al. Pembrolizumab plus GX-188E therapeutic DNA vaccine in patients with HPV-16-positive or HPV-18-positive advanced cervical cancer: interim results of a single-arm, phase 2 trial. Lancet Oncol (2020) 21:1653–60. doi: 10.1016/S1470-2045(20)30486-1
73. Oaknin A. Randomized cervical cancer cohorts of CheckMate 358 (NCT02488759) testing 2 combination regimens of nivolumab + ipilimumab for R/M disease. ESMO (Amsterdam: Elsevier) (2019) 12–3.
74. O’Malley DM, Neffa M, Monk BJ, Melkadze T, Huang M, Kryzhanivska A, et al. Dual PD-1 and CTLA-4 checkpoint blockade using balstilimab and zalifrelimab combination as second-line treatment for advanced cervical cancer: An open-label phase II study. J Clin Oncol (2022) 40:762–71. doi: 10.1200/JCO.21.02067
75. Yin H, Guo W, Sun X, Li R, Feng C, Tan Y. TILs and anti-PD1 therapy: An alternative combination therapy for PDL1 negative metastatic cervical cancer. J Immunol Res (2020) 2020:8345235. doi: 10.1155/2020/8345235
76. Met O, Jensen KM, Chamberlain CA, Donia M, Svane IM. Principles of adoptive T cell therapy in cancer. Semin Immunopathol (2019) 41:49–58. doi: 10.1007/s00281-018-0703-z
77. Grau JF, Farinas-Madrid L, Oaknin A. A randomized phase III trial of platinum chemotherapy plus paclitaxel with bevacizumab and atezolizumab versus platinum chemotherapy plus paclitaxel and bevacizumab in metastatic (stage IVB), persistent, or recurrent carcinoma of the cervix: the BEATcc study (ENGOT-Cx10/GEICO 68-C/JGOG1084/GOG-3030). Int J Gynecol Cancer (2020) 30:139–43. doi: 10.1136/ijgc-2019-000880
78. Colombo N, Dubot C, Lorusso D, Caceres MV, Hasegawa K, Shapira-Frommer R, et al. Pembrolizumab for persistent, recurrent, or metastatic cervical cancer. N Engl J Med (2021) 385:1856–67. doi: 10.1056/NEJMoa2112435
79. Rodrigues M, Loap P, Dubot C, Durdux C, Bazire L, Minsat M, et al. Combination of nivolumab with chemoradiotherapy for locally advanced cervical cancer: NiCOL phase I trial. J Clin Oncol (2022) 40:5534. doi: 10.1200/JCO.2022.40.16_suppl.5534
80. Yabuno A, Nakamura K, Satoh T, Fujiwara H, Kurosaki A, Yamashita S, et al. GOTIC-018: phase I, open-label, multicenter study to assess the safety of pre- and co-administration of ONO-4538 (nivolumab) with concurrent chemoradiation (CCRT) in patients (pts) with locally advanced cervical carcinoma (LACvCa). J Clin Oncol (2022) 40:5529. doi: 10.1200/JCO.2022.40.16_suppl.5529
81. Ou D, Xu H. Toripalimab combined with chemoradiotherapy for patients with locally advanced cervical squamous cell carcinoma. J Clin Oncol (2022) 40:5538. doi: 10.1200/JCO.2022.40.16_suppl.5538
82. Grasselly C, Denis M, Bourguignon A, Talhi N, Mathe D, Tourette A, et al. The antitumor activity of combinations of cytotoxic chemotherapy and immune checkpoint inhibitors is model-dependent. Front Immunol (2018) 9:2100. doi: 10.3389/fimmu.2018.02100
83. Wu T, Dai Y. Tumor microenvironment and therapeutic response. Cancer Lett (2017) 387:61–8. doi: 10.1016/j.canlet.2016.01.043
84. Formenti SC, Rudqvist NP, Golden E, Cooper B, Wennerberg E, Lhuillier C, et al. Radiotherapy induces responses of lung cancer to CTLA-4 blockade. Nat Med (2018) 24:1845–51. doi: 10.1038/s41591-018-0232-2
85. McLaughlin M, Patin EC, Pedersen M, Wilkins A, Dillon MT, Melcher AA, et al. Inflammatory microenvironment remodelling by tumour cells after radiotherapy. Nat Rev Cancer (2020) 20:203–17. doi: 10.1038/s41568-020-0246-1
86. Georganaki M, van Hooren L, Dimberg A. Vascular targeting to increase the efficiency of immune checkpoint blockade in cancer. Front Immunol (2018) 9:3081. doi: 10.3389/fimmu.2018.03081
87. Song Y, Fu Y, Xie Q, Zhu B, Wang J, Zhang B. Anti-angiogenic agents in combination with immune checkpoint inhibitors: A promising strategy for cancer treatment. Front Immunol (2020) 11:1956. doi: 10.3389/fimmu.2020.01956
88. Malo CS, Khadka RH, Ayasoufi K, Jin F, AbouChehade JE, Hansen MJ, et al. Immunomodulation mediated by anti-angiogenic therapy improves CD8 T cell immunity against experimental glioma. Front Oncol (2018) 8:320. doi: 10.3389/fonc.2018.00320
Keywords: immune checkpoint inhibitors, cervical cancer, PD- 1/L1, immunotherapy, clinical trials
Citation: Xie Y, Kong W, Zhao X, Zhang H, Luo D and Chen S (2022) Immune checkpoint inhibitors in cervical cancer: Current status and research progress. Front. Oncol. 12:984896. doi: 10.3389/fonc.2022.984896
Received: 02 July 2022; Accepted: 17 October 2022;
Published: 27 October 2022.
Edited by:
Annamaria Ferrero, Mauriziano Hospital, ItalyReviewed by:
Rita Trozzi, Unit of Cancer Gynaecology (IRCCS), ItalyRahul Manchanda, Pushpawati Singhania Research Institute Hospital, India
Maria Lee, Seoul National University Hospital, South Korea
Copyright © 2022 Xie, Kong, Zhao, Zhang, Luo and Chen. This is an open-access article distributed under the terms of the Creative Commons Attribution License (CC BY). The use, distribution or reproduction in other forums is permitted, provided the original author(s) and the copyright owner(s) are credited and that the original publication in this journal is cited, in accordance with accepted academic practice. No use, distribution or reproduction is permitted which does not comply with these terms.
*Correspondence: Weimin Kong, a3dtMTk2N0BjY211LmVkdS5jbg==