- 1The Second School of Clinical Medicine, Zhejiang Chinese Medical University, Hangzhou, China
- 2Department of Nephrology, Zhejiang Provincial People’s Hospital (Affiliated People’s Hospital), Hangzhou Medical College, Hangzhou, China
- 3Jinzhou Medical University, Graduate School of Clinical Medicine, Jinzhou, China
- 4School of Medicine, Hangzhou Normal University, Hangzhou, Zhejiang, China
- 5School of Chinese Medicine, Faculty of Medicine, The Chinese University of Hong Kong, Hong Kong, Hong Kong SAR, China
- 6Department of Nephrology, The First Affiliated Hospital of Zhejiang Chinese Medical University (Zhejiang Provincial Hospital of Traditional Chinese Medicine), Hangzhou, China
Over the past few decades, significant progress has been made in the development of drugs to combat cancer. It is unfortunate that these drugs can also lead to various kidney injuries and imbalances in electrolyte levels. Nephrotoxicity caused by chemotherapy drugs can impact different parts of the kidneys, including the glomeruli, renal tubules, interstitium, or renal microvessels. Despite the existing knowledge, our understanding of the mechanisms underlying the renal damage caused by antitumoral drugs remains incomplete. In this review, we aim to provide a comprehensive overview of the specific types of kidney injury and the mechanisms responsible for the drug-mediated renal damage, and briefly discuss possible prevention and treatment measures. Sensitive blood and urine biomarkers can provide clinicians with more information about kidney injury detection and reference value for subsequent treatment options. In addition, we emphasize that both oncologists and nephrologists have a responsibility to remain vigilant against the potential nephrotoxicity of the drugs. It’s crucial for experts in both fields to collaborate in early detection, monitoring and prevention of kidney damage.
Introduction
Antitumor drugs are mainly divided into alkylating agents, antitumor antibiotics, antitumor hormones, metal platinum, anti-metabolites, antitumor plants, and molecular targeted drugs, etc. Among them, alkylating agents are simple in synthesis with an important value for tumor treatment, while antibiotic drugs exert a good therapeutic effect on malignant tumors, but with the poor selectivity, extensive adverse reactions and easy development of drug resistance. As new anticancer drugs specifically targeting cancer cells are emerging, the prognosis of cancer patients is slowly improving, but the targeting and toxicity of drugs are two major problems. The kidneys are an important component of xenobiotic metabolism, and nephrotoxicity remains an unavoidable complication that may lead to adjustment or discontinuation of an anticancer therapy, thus affecting patient survival. Many drug-related nephrotoxicities do not have a clear mechanism of injury or pathophysiology, which poses a specific challenge to clinicians in selecting their treatment strategies. For instance, traditional chemotherapy drugs, such as cisplatin and alkylating agent ifosfamide, etc., often act on renal tubules, resulting in acute tubular necrosis or injury. In contrast, molecularly targeted drugs may cause glomerular lesions characterized by podocyte disease or thrombotic microangiopathy. This article provides a comprehensive review of the occurrence and underlying mechanisms of renal function insufficiency associated with the commonly used drugs. A key focus is on reducing the frequency and severity of nephrotoxicity resulting from chemotherapy drugs. To achieve this goal, researchers should emphasize the evaluation of early biomarkers for kidney injury, especially ones obtainable through noninvasive techniques. By employing such noninvasive methods, clinicians can promptly detect kidney damage and intervene accordingly. This proactive approach will contribute to minimizing the incidence and severity of drug-induced nephrotoxicity, ultimately improving patient outcomes.
Platinum-derived compounds
Mechanisms and clinical manifestations
Cisplatin is approved for the treatment of many types of cancers (1) and is the most nephrotoxic chemotherapy drug detected early. The most common and important clinical manifestation of cisplatin nephrotoxicity is acute kidney injury(AKI). In the first 24 hours after cisplatin administration, with more than 50% of the drug excreted in the urine, and the concentration of platinum reached in the renal cortex is several times higher than in plasma and other organs. Drugs that accumulate in the urinary system cause renal dysfunction through a variety of mechanisms, including toxicity to tubular epithelial cells, vasoconstriction and proinflammatory effects of renal microvessels (2) (Figure 1). The toxicity to renal tubular cells is mainly reflected in apoptosis and necrosis of the S3 segment of the proximal tubule, distal curved tubule and collecting duct. The expression of proximal tubular organic cation transporter-2 (OCT2) enhances renal uptake, mediating the accumulation of cisplatin in proximal tubular epithelial cells (3). In addition, renal microvasoconstriction is observed after cisplatin injection, resulting in reduced renal blood flow. The renal response to cisplatin also includes the increased expression of proinflammatory cytokines (4, 5). Cisplatin has the ability to decrease the expression and activity of glucose and amino acid transporters that rely on sodium, as well as magnesium and water transporters. Furthermore, it hinders the metabolism of glutathione and cysteine-glycine conjugates, and also suppresses the production of reactive oxygen species. These mechanisms help to explain the manifestation of Fanconi-like syndrome often observed in patients undergoing cisplatin therapy. When cisplatin is used in combination with bleomycin or gemcitabine, thrombotic microangiopathy(TMA) may lead to direct endothelial injury and secondary platelet activation (6–9). Renal impairment is dose-dependent, and we have summarized the incidence of AKI in several studies reported in Table 1 (6, 42, 43). In a recent study of 821 adult cancer patients with multiple tumor types treated with cisplatin, after 6 years of follow-up, 31.5% patients developed AKI, with an estimated initial reduction in median eGFR of 10mL/min/1.73m2. Most patients have a small but permanent decrease in eGFR without progression to end-stage renal disease requiring dialysis (10). Other renal manifestations include hypomagnesemia, hypokalemia, sodium depletion, Fanconi-like syndrome, and anemia (Table 2) (7, 44, 52).
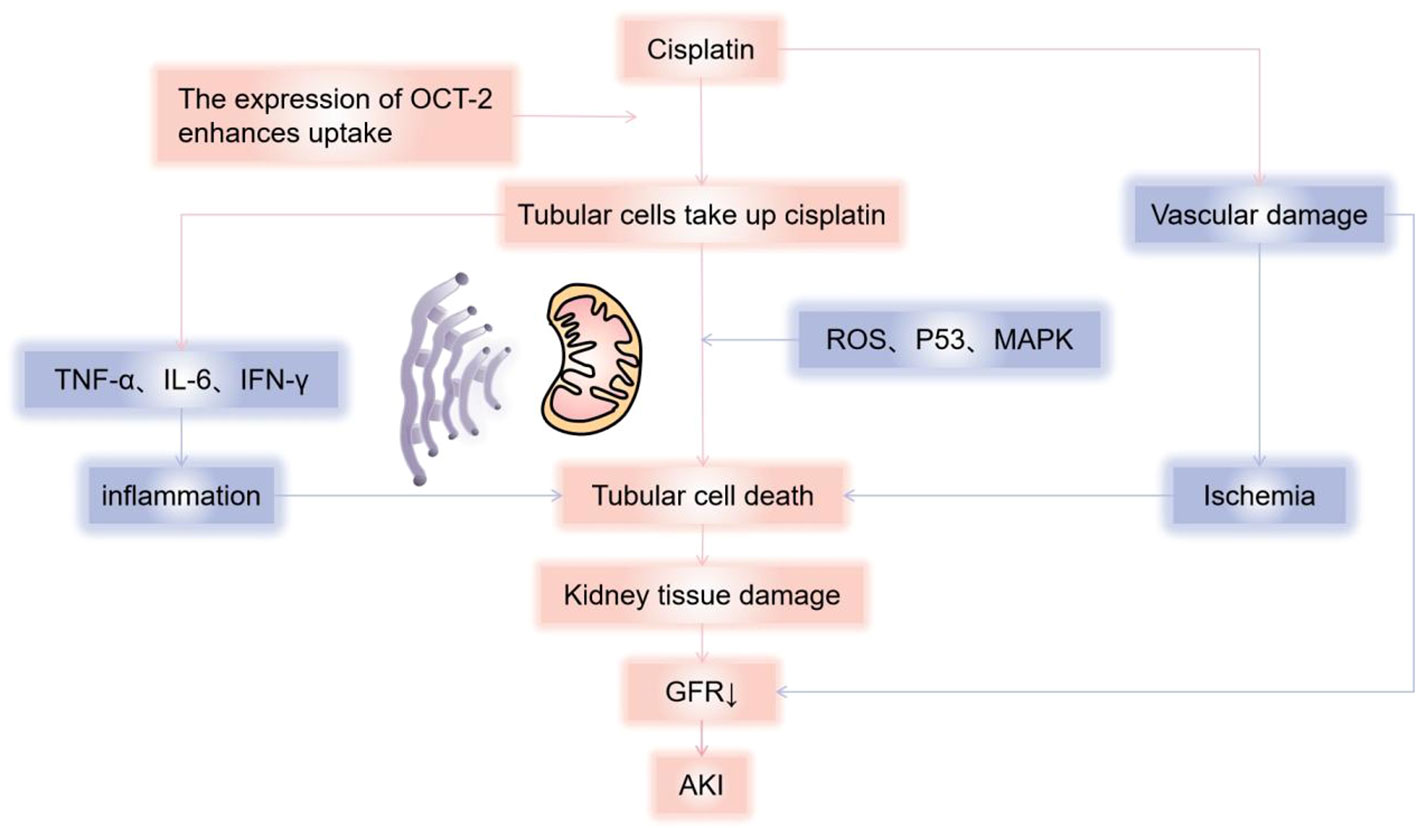
Figure 1 Mechanisms of cisplatin causing kidney damage. Cisplatin causes renal dysfunction through a variety of mechanisms, including toxicity to tubular epithelial cells, vasoconstriction, and pro-inflammatory effects of renal microvessels. Cisplatin enters cells through organic cation transporters (OCTs), which activates signaling pathways (p21) that promote cell death (MAPK, p53, ROS, etc.) or cell protection, and damage the renal tubules, mainly reflected in apoptosis and necrosis of the S3 segment of the proximal tubule, distal curved tubule and collecting duct, resulting in a decrease in glomerular filtration rate. According to current reports, it is mainly mitochondria-triggered intrinsic pathways, extrinsic pathways mediated by TNF (tumor necrosis factor) receptor/ligand, and endoplasmic reticulum stress pathways. Cisplatin can also cause renal vascular damage, resulting in ischemic tubular cell death and decreased glomerular filtration rate (GFR). AKI, Acute kidney injury; GFR, Glomerular Filtration Rate.
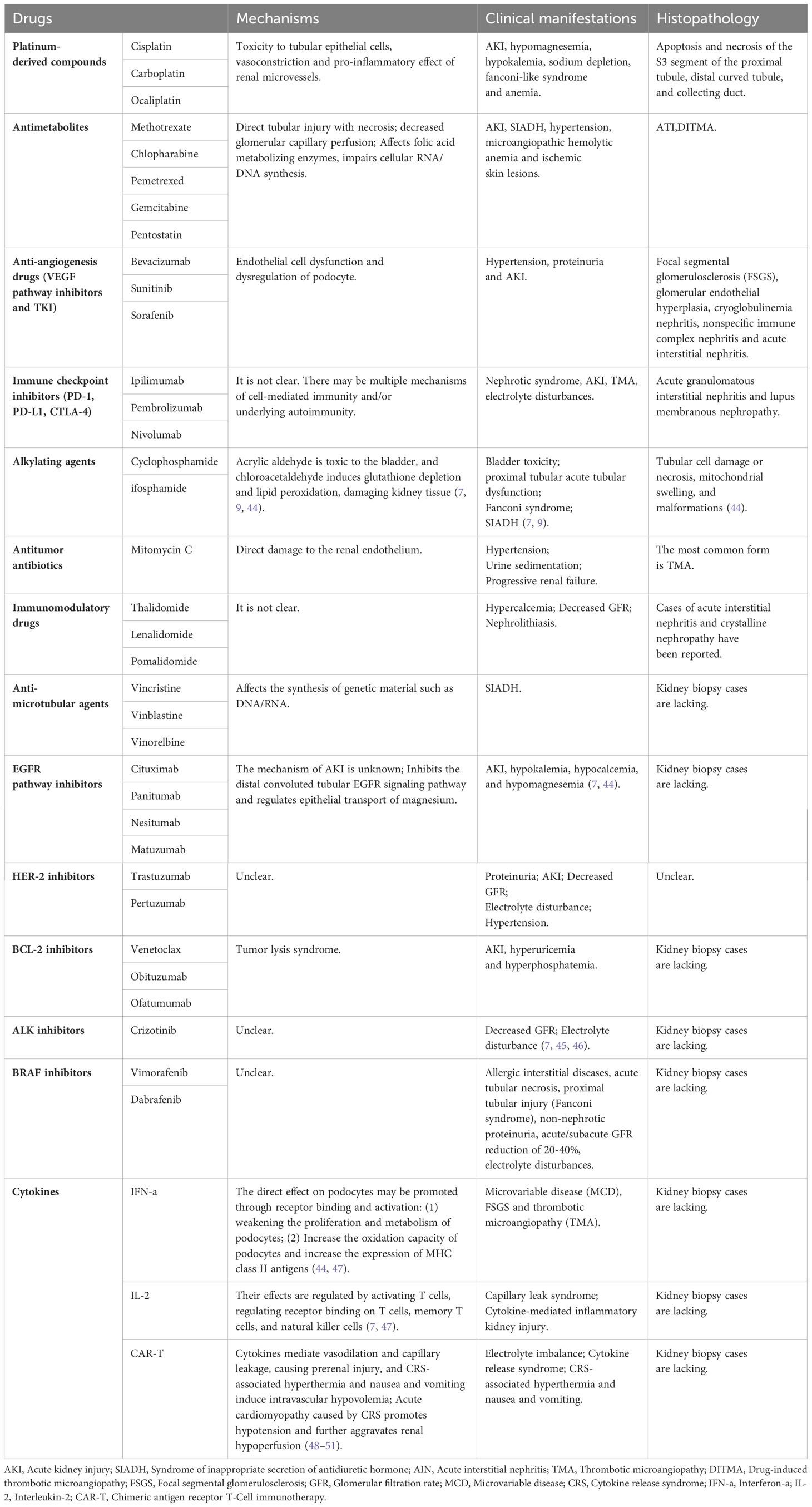
Table 2 Mechanisms, pathological manifestations and clinical manifestations of renal injury caused by different antineoplastic drugs.
Treatments
Appropriate fluid therapy, correction of electrolyte abnormalities, prompt diuresis, and monitoring of renal function are particularly important to prevent platinum-based renal injury (53).There is currently no effective way to reverse AKI or tubular dysfunction, and treatment of renal toxic manifestations is primarily supportive with an aim to relieve symptoms. Advanced AKI exhibits uremia, metabolic derangement, and hypervolemia and requires dialysis, but dialysis is not effective in removing cisplatin. Intravenous saline or oral sodium chloride to correct hypovolemia and symptomatic hypomagnesium due to table salt, and intravenous/oral magnesium are necessary. Fanconi syndrome is notoriously difficult to treat (7, 9, 44).
Antimetabolites
Mechanisms and clinical manifestations
Methotrexate is widely used to treat malignancies such as advanced lymphoma, and its high-dose therapy (1-12 g/m2) is known to have an effect on renal function, with nephrotoxicity occurring during long-term therapy at conventional dose (54, 55). Dihydrofolate reductase inhibitors and their metabolites are filtered by the glomeruli and secreted from the proximal tubule to the cleft, and methotrexate favors crystal precipitation in the tubular lumen in a setting of slow urine flow rate and low urine pH, resulting in AKI due to direct tubular injury and necrosis (56). Methotrexate may also lead to a transient decrease in glomerular filtration rate due to a decrease in pressure because of contraction of glomerular capillary surface area and glomerular capillary perfusion, and afferent arteriolar or mesangial contraction. It can even cause syndrome of inappropriate secretion of antidiuretic hormone(SIADH) (7, 45).
Pemetrexed, a derivative of methotrexate excreted by the kidneys (70 to 90 percent within 24 hours, with a half-life of 3.5 hours), inhibits enzymes involved in purine/pyrimidine metabolism by affecting folate-metabolizing enzymes, impairs cellular RNA/DNA synthesis, and is approved for the treatment of advanced non-small cell lung cancer and pleural mesothelioma (44, 57) (58). Clofabine is also a purine nucleoside analog approved for the treatment of refractory childhood acute lymphoblastic leukemia and adult acute myeloid leukemia. It is possible that the inhibition of DNA synthesis, ribonucleotide reductase, and mitochondrial repair processes by clofarabine leads to ATI, while the ultimate understanding of mechanisms still requires renal biopsy. The nucleoside pyrimidine analogue, gemcitabine, is effective in the treatment of certain malignancies, mainly including lung, pancreatic, bladder, and breast cancers. Unfortunately, as with other chemotherapy drugs, many case reports and case series have documented AKI for gemcitabine, primarily TMA, which leads to drug-induced and dose-dependent thrombotic microangiopathy (DITMA) through immuno- and toxicity-mediated mechanisms.
In addition, hypertension, microangiopathic hemolytic anemia, and ischemic skin lesions may occur. The incidence and cases of renal adverse reactions caused by the antimetabolites above are detailed in Table 1.
Treatments
Treatment involves the addition of folinic acid salvage to methotrexate to reduce nonmalignant cell damage and cleavage of methotrexate into noncytotoxic metabolites using glucosidase. High-throughput hemodialysis well clears plasma methotrexate (76 percent), but plasma concentrations may rebound after dialysis, and it can be used for severe acute kidney injury and may be unnecessary with the spread of glucosidase (44). In the case of immune-mediated DITMA, the best solution is to avoid the substance in question for life (7, 44).
Anti-angiogenic drugs (VEGF pathway inhibitors and TKI)
Mechanisms and clinical manifestations
The premise of this type of targeted drugs is that tumor growth is highly dependent on local pathological angiogenesis induced by vascular endothelial growth factor (VEGF), which promotes tumor development by regulating vascular permeability, endothelial cell migration, proliferation, and survival (44). VEGF is produced by renal visceral epithelial cells and binds to VEGF receptors located on the glomerular endothelium, mesangium, and periductal capillaries, while local VEGF synthesis is responsible for maintaining normal glomerular function and the integrity of the glomerular basement membrane, including damage repair and cell renewal (Figure 2). The most important and dose-dependent renal effects of antiangiogenic therapy are hypertension and kidney-specific impairment, including proteinuria and AKI (57, 59–62). However, the effects of antiangiogenic drugs are predominantly histopathologically manifested as thrombotic microangiopathy (Figure 2). Other lesions described on renal biopsy include focal segmental glomerulosclerosis (FSGS), parathyroid proliferative nephritis, glomerular endothelial hyperplasia, cryoglobulinaemic nephritis, nonspecific immune complex nephritis, and acute interstitial nephritis (63–65). These drugs are a logically targeted therapy that complements other tumor-targeted therapies. Similar renal TMA-like results can be seen due to the anti-VEGF effects ofTyrosine Kinase Inhibitors(TKIs) (66), and sunitinib or sorafenib can also cause acute and chronic interstitial nephritis, as well as chronic interstitial and endothelial damage, leading to CKD. Multitarget TKIs (eg, imani) and novel TKIs (eg, dasatinib and nilotinib) are associated with renal functional insufficiency and subsequent CKD (7, 9, 67). In animal experiments, the histopathology of the kidney of mice injected with a single dose of anti-VEGF antibody has shown glomerular endothelial cell swelling, vacuolization, detachment, and the rupture of the epithelial septum, while immunohistochemistry has also demonstrated that renin is down-regulated, and that the nephron could be partially restored with recombinant VEGF (68). There are also numerous reports of kidney injury associated with these drugs (Table 1).
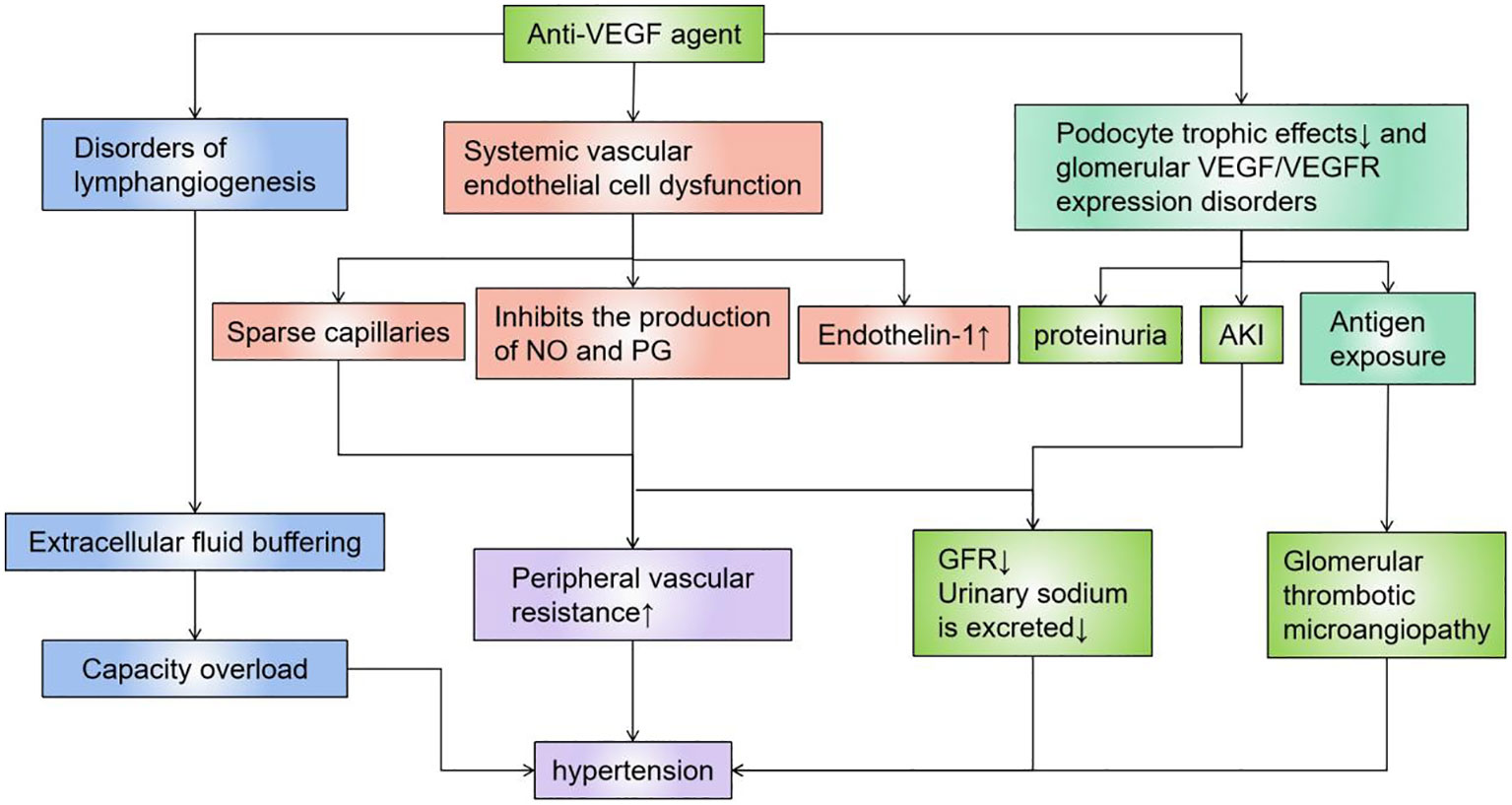
Figure 2 Mechanisms of action of anti-VEGF agents. VEGF is produced by renal visceral epithelial cells and binds to VEGF receptors located on the glomerular endothelium, mesangium, and periductal capillaries, and local VEGF synthesis is responsible for maintaining normal glomerular function and the integrity of the glomerular basement membrane, including damage repair and cell renewal. Anti-VEGF agents directly cause AKI by causing dysfunction such as vascular endothelium/lymphatic vessels, or podocytes, or indirectly cause kidney damage and hypertension by destroying blood vessels of the filtration system. VEGF, Vascular Endothelial Growth Factor.
Treatments
Therefore, exacerbations in hypertensive patients on such drugs often predict a stronger response to antineoplastic therapy (69), which in turn motivates clinicians to continue antiangiogenic therapy while using antihypertensive drugs to control blood pressure rather than discontinuing antiangiogenic therapy.
Immune checkpoint inhibitors (PD-1, PD-L1, CTLA-4)
Mechanisms and clinical manifestations
Immune checkpoint inhibitors (ICIs) are complementary therapies of cancers and are considered to be the most innovative and promising drugs in cancer treatment, including programmed death 1(PD-1), programmed cell death-Ligand 1(PD-L1), and cytotoxic T lymphocyte-associated antigen 4 (CTLA-4) monoclonal antibodies against these suppressor receptors expressed by tumor cells, T cells, and other immune cells. Immune-related Adverse Effects (IrAE) affects all systems, most commonly in the gastrointestinal tracts, endocrine glands, skin, and liver, and less commonly in the central nervous, cardiovascular, pulmonary, musculoskeletal, and hematologic systems (29, 70, 71). Some researchers have reported that ICIs-induced AKI may be caused by a unique mechanism of action involved in immune system reprogramming, leading to loss of tolerance (25). Pembrolizumab has also been reported to cause renal insufficiency (21). Ipilimumab is approved for treating advanced melanoma, and in a retrospective study of rare adverse events caused by ipilimumab, as reported in two cases of acute renal failure in 120 exposed patients, renal biopsy was more useful in examining renal manifestations (72, 73). Nephrotic syndrome, acute tubular injury and other manifestations of acute tubular necrosis in AKI have also been reported. Renal biopsy shows acute granulomatous interstitial nephritis and lupus membranous nephropathy (74). In some reports, steroid therapy has been associated with improved renal function (73, 75), although the mechanisms by which ipilimumab causes kidney disease are not well understood. Dual mechanisms are possible, including cell-mediated immunity (76) and/or underlying autoimmune mechanisms (74) (Figure 3).
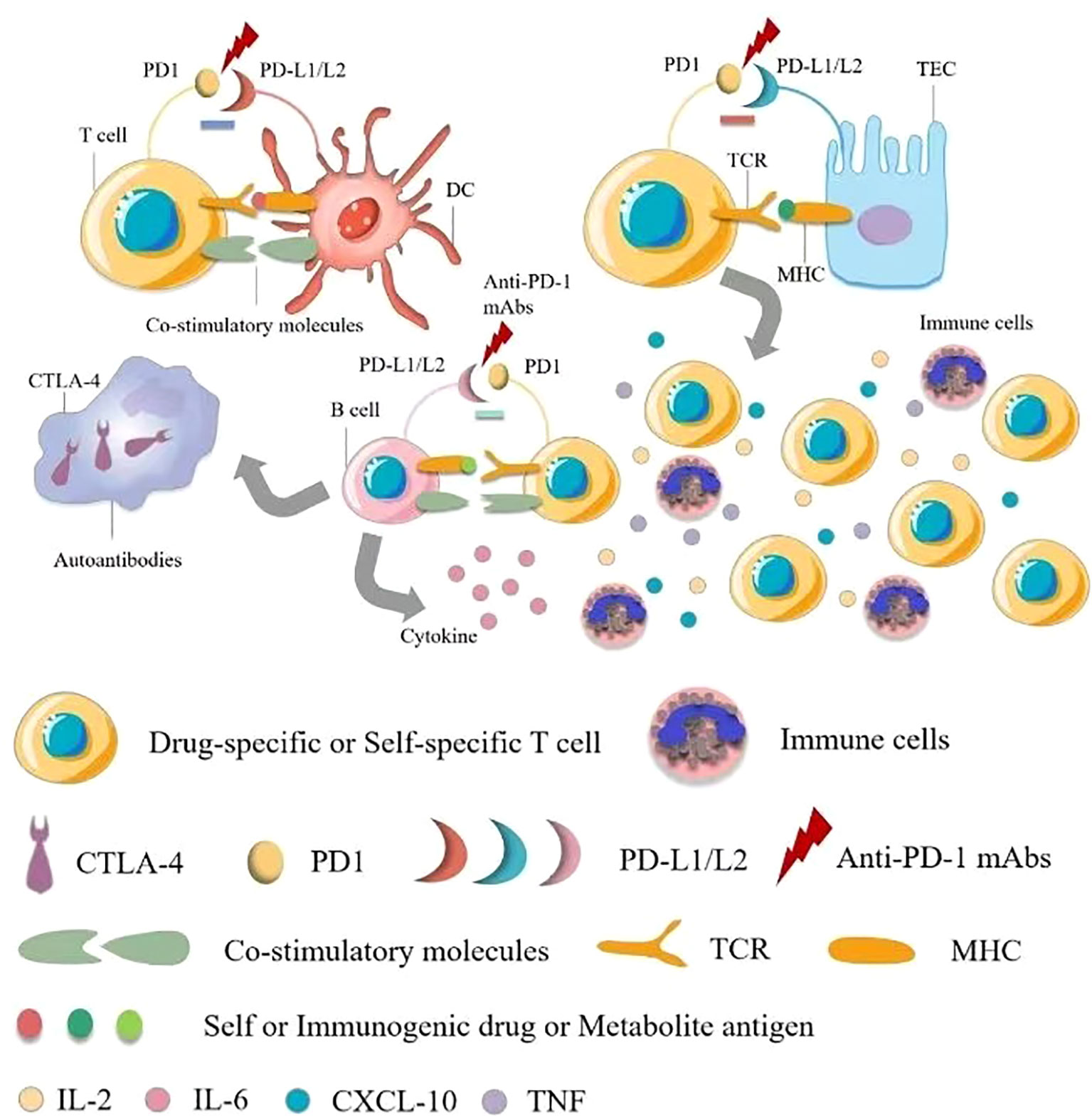
Figure 3 Pathophysiology of immune checkpoint inhibitor-associated acute kidney injury. There are multiple mechanisms of immune checkpoint inhibitor (ICI)-associated development of acute kidney injury (AKI). The first mechanism is loss of tolerance to autoantigens. Normally, effector-specific T cells are silenced by PD-1 signaling, which serves as a checkpoint for inhibiting T cell responses, and after using ICIs, T cells activate and cells are damaged. The second possible mechanism is through reactivation of drug-specific effector T cells. Proton pump inhibitors, antibiotics and other drugs can bind to tubular antigens and directly or indirectly trigger an immune response as haptens, which are trapped in the renal parenchyma, and reactive T cells can be dormant and activated when using ICIs. At the same time, serum cytokines such as CXCL10, TNF, and IL-6 levels are elevated, and administration may also lead to the production of autoantibodies against autoantigens presented by tubular epithelial cells, mesangial cells, or podocytes, resulting in an inflammatory environment. TCR, T cell receptor; TEC, tubular epithelial cells.
Treatments
The basic treatment for IrAEs is steroid medications, prednisone (0.5-1 mg/kg/day) or methylprednisone (1-2mg/kg/day). Of course, hydrocortisone is allowed in exceptional cases. In case reports, treatment with these agents has resulted in partial or complete remission, but relapse has also occurred (53).
Other drugs
Mechanisms and clinical manifestations
Alkylating agents represented by cyclophosphamide and ifosfamide can generate cytotoxicity or nephrotoxicity and give rise to Fanconi syndrome through their metabolites. The antineoplastic antibiotic mitomycin C may cause nephrotoxicity through direct damage to the renal endothelium, which most likely occurs at least six months after treatment, with overall incidence depending on cumulative doses (7, 77). The immunomodulatory drug, thalidomide, and its derivatives are used in the treatment of multiple myeloma and have been reported to cause AKI (7, 45, 78–80). In addition to the programmed cell death protein 1 (PD-1) and its ligand (PD-L1), vascular endothelial growth factor (VEGR), and vascular endothelial growth factor receptor (VEGFR), the most commonly used cancer therapies target proteasomes, epidermal growth factor receptor (EGFR), human epidermal growth factor receptor-2 (HER2), HER2 dimer, v-Raf murine sarcoma virus oncogene homolog B (BRAF), anaplastic lymphoma kinase (ALK), mammalian targets of receptor activators (RANKL) and rapamycin (mTOR) of nuclear factor κβ ligands (7, 81). Monoclonal antibodies, such as cetuximab, have a 10-fold higher affinity for EGFR than natural ligands and can prevent EGF from binding to its receptor, making them an effective targeted therapy for these cancers. But, hypomagnesemia, hypokalemia, and hypocalcaemia may occur (7, 44). Trastuzumab is a recombinant humanized monoclonal antibody against the extracellular domain of HER2, while perstuzumab blocks the dimerization of HER2 required for cell activation and proliferation compared to trastuzumab, which can cause hypertension. Cardiorenal syndrome has been observed when combined with anthracycline antibiotics with cardiotoxicity, while reduced renal function in patients treated with trastuzumab means a higher risk of cardiotoxicity. Nephrotoxic effects also increase when used in combination with cisplatin-based drugs (7, 82). Inhibitors of B-cell lymphoma 2, most commonly used for hematologic tumors, are prone to tumor lysis syndrome with lysis of a large number of tumor cells, resulting in the release of a large amount of nucleic acid, phosphate and potassium into the systemic circulation, formation of nucleic acid metabolite uric acid, hyperuricemia, and uric acid precipitation in the renal tubules. In addition, hyperuricemia can lead to renal vasoconstriction, impaired autoregulation, decreased renal blood flow, and inflammation, resulting in nephrogenic AKI (7). The therapeutic small molecule inhibitor, crizotinib, is mesenchymal lymphoma kinase 1, which is used to treat non-small cell lung cancer. Clazotinib has been associated with a reduced eGFR and an increased risk of renal cyst development and progression (7, 45). Vemurafenib and dabrafenib are potent inhibitors of mutant BRAF kinase domains and are approved for the treatment of patients with advanced melanoma. Wanchoo et al. (83) summarized data of nephrotoxicity for these drugs, in which BRAF is expressed and localized in developing and mature glomerular podocytes in experimental animal models, while in a clinical setting, the putative mechanism of nephrotoxicity is tubular toxicity. Thus, renal biopsy has not yet been performed to elucidate the mechanisms behind the nephrotoxicity of BRAF inhibitors (7, 9, 45). Interferon and high dose IL-2 are the earliest immunotherapies clinically used to treat various malignant tumors, whereas new cancer immunotherapies have emerged in the past decade, such as the above-mentioned immune checkpoint inhibitors that activate quiescent T cells and impair the ability of tumors to turn off activated T cells in the tumor microenvironment. Chimeric antigen receptor (CAR) T cells are also emerging immunotherapies, which can be used to treat various malignant blood diseases and directly bind/destroy cancer cells, thereby overcoming the immune barrier utilized by cancer cells. Of course, nephrotoxicity is inevitable (Figure 4) (47).
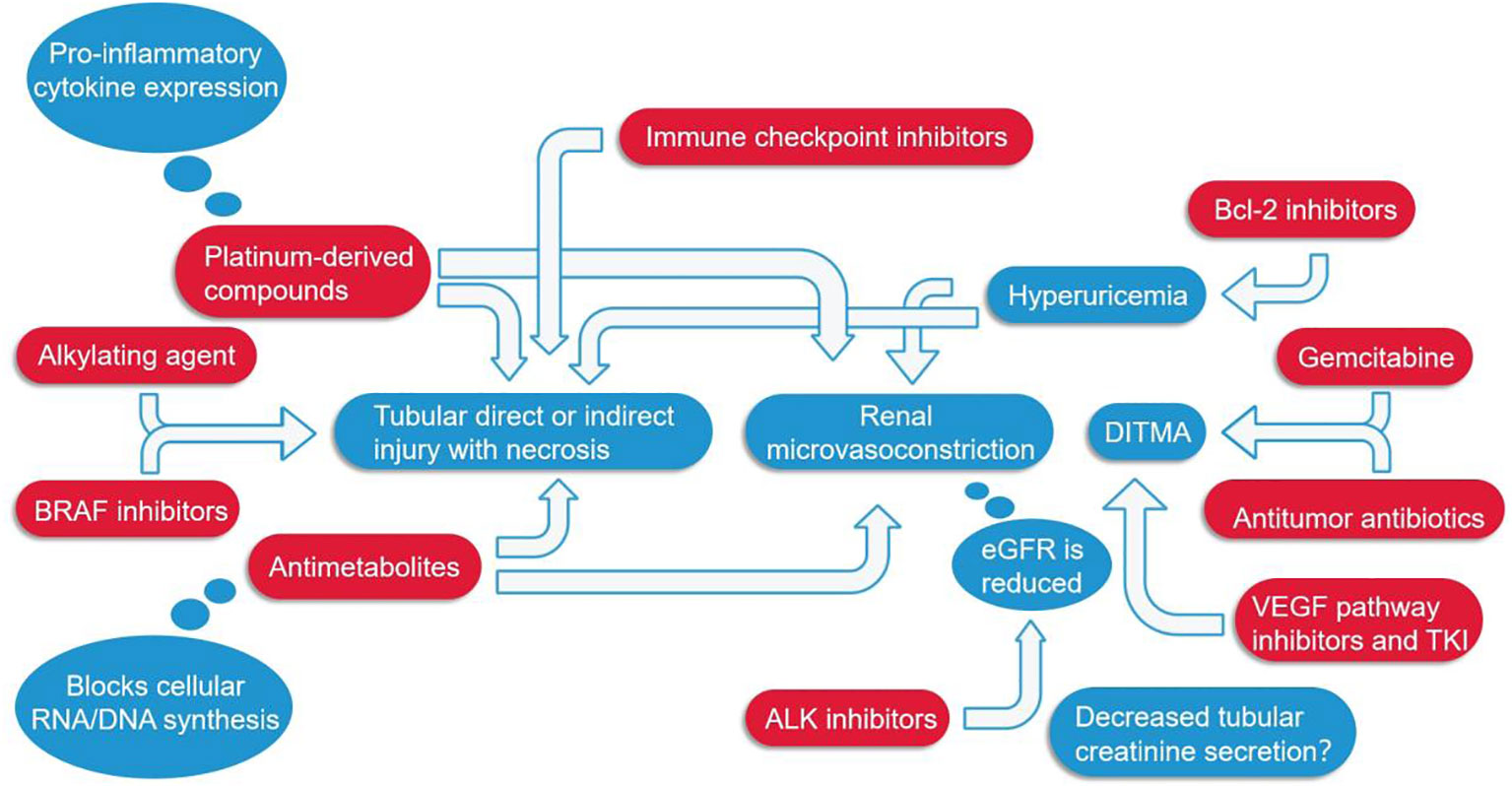
Figure 4 Several major classes of tumor drugs directly or indirectly cause renal function damage. Among them, ICIs can directly lead to renal tubular damage; Platinum-derived compounds cause tubular damage while also increasing the expression of pro-inflammatory cytokines; Antimetabolites mainly affect the synthesis of genetic material and can also cause constriction of renal microvessels; Anti-VEGF agents, antitumor antibiotics, and gemcitabine cause DITMA; Bcl-2 inhibitors increase uric acid, resulting in renal microvasoconstriction; Alkylating agents, BRAF inhibitors, ALK inhibitors, etc. also damage the renal tubules in different ways. DITMA, Drug-induced thrombotic microangiopathy.
Treatments
First, adjusting the dose of cyclophosphamide and ifosfamide according to the degree of renal function can reduce nephrotoxicity. Mesna(antidote to acrolein) infusion and appropriate hydration can be used to prevent hemorrhagic cystitis. For complications of mitomycin use, the use of plasmapheresis and steroid therapy may suppress thrombotic microangiopathy without affecting the recovery or improvement of renal function (53).
It is important to emphasize that close monitoring, appropriate hydration, and dose reduction or even suspension of the use of the drug are preferred in these patients.
The patient
The patient’s own baseline characteristics can increase the possibility of drug nephrotoxicity, such as relatively less muscle mass and total body water in women and the elderly, which may lead to drug overdose. These two groups of people have lower serum albumin concentrations, leading to decreased drug binding and thus increased drug free concentrations. In addition to this, other systemic and renal diseases may also increase the risk of nephrotoxicity of drugs. Diseases such as cirrhosis and nephrotic syndrome increase nephrotoxic effects through a variety of mechanisms. Examples include systemic effective volume reduction, renal hypoperfusion, hypoalbuminemia, and renal impairment not exhibiting clinical symptoms. For drugs cleared by the kidney, these complications increase nephrotoxicity through prerenal and renal mechanisms (84).
Preclinical studies of drug-induced renal toxicity
Kidney-on-a-chip technology
In vitro model that mimics kidney physiology and continuous blood flow conditions, can replicate the in vivo environment and predict the nephrotoxicity of certain drugs in the clinical setting (85). Proximal tubular cells cultured in this model show markers of various drug cytotoxicity and have been successfully applied to known nephrotoxins such as cisplatin (86).
Cell- and biomarker-based assays
Biomarkers were added to the preclinical studies. Currently, routine biomarkers, such as serum creatinine and blood urea nitrogen, are the gold standard for assessing renal function and detecting kidney injury, including reversible increases in renal functional insufficiency and absolute increases in structural damage, although these two substances are not early biomarkers with abnormality measured only after nearly 50% GFR loss, limiting their use in clinics. In addition, their levels are affected by age, gender, food, fluid volume, muscle mass, medication, and other factors. Therefore, there is an urgent need for more sensitive biomarkers for early detection of AKI in the clinic. These potential novel biomarkers include neutrophil gelatinase-associated lipids (NGAL, also known as lipid 2), cluster proteins and kidney damage molecule-1 (KIM-1), polyheparin, albumin, total protein, β-2-microglobulin (B2M), cystinstatin C and trilobite 3 (TFF3), metalloproteinase tissue inhibitor 2 (TIMP2), insulin-like growth factor binding protein 7 (IGFBP7), and N-acetyl-d-glucosidase (NAG)(Table 3). In fact, pro-inflammatory interleukins, such as IL-6, IL-8, and IL-18, are also considered potential novel biomarkers for the detection of nephrotoxic kidney injury (7, 8, 45).
Kidney-on-a-chip technology, combined with new biomarkers, may give clinicians a better understanding of whether drugs are nephrotoxic. If so, the location of the injury and the underlying mechanism of kidney damage can be further understood.
Conclusions
Oncological nephrology is a rapidly advancing field that demands close collaboration between oncologists and nephrologists. While the use of conventional and targeted therapies has improved patient outcomes, there have been reported complications due to the nephrotoxic effects of these anti-cancer drugs. Therefore, it is essential to gain a comprehensive understanding of the potential adverse effects associated with these treatments, enabling early detection and prevention of further complications. In this regard, it is crucial to emphasize the importance of integrating “omics” strategies, including the exploration of non-invasive biomarkers, such as urine-based markers, that can accurately reflect the severity of kidney injury and assist in risk stratification and prognostic assessment.
Future studies can appropriately focus on the following aspects: 1) the effects of potential renal dysfunction or complications of anti-tumor drugs in cancer patients. This allows a more comprehensive understanding of the risk of kidney injury in different patient populations. 2) Potential novel anti-tumor drugs that can reduce renal toxicity. 3)Further develop and validate biomarkers with high sensitivity and specificity.
Author contributions
JT: Conceptualization, Data curation, Investigation, Methodology, Validation, Writing – original draft. NY: Conceptualization, Data curation, Investigation, Methodology, Validation, Writing – original draft. SP: Conceptualization, Data curation, Methodology, Supervision, Validation, Writing – original draft. PR: Conceptualization, Data curation, Investigation, Supervision, Writing – original draft. MC: Conceptualization, Data curation, Supervision, Writing – review & editing. JJ: Data curation, Funding acquisition, Supervision, Validation, Writing – review & editing. QH: Funding acquisition, Supervision, Validation, Writing – review & editing. YZ: Conceptualization, Data curation, Funding acquisition, Methodology, Resources, Supervision, Validation, Writing – review & editing.
Funding
The author(s) declare financial support was received for the research, authorship, and/or publication of this article. This work was supported by grants from the National Natural Scientific Foundation of China (82004116), the Zhejiang Provincial Natural Science Foundation of China (LQ20H290006), the Medical Health Science and Technology Project of Zhejiang Provincial Health Commission(2023KY539), the Huadong Medicine Joint Funds of the Zhejiang Provincial Natural Science Foundation of China (No. LHDMZ22H050001), the Construction of Key Projects by Zhejiang Provincial Ministry (No.WKJ-ZJ-2302), The Key Project of Scientific Research Foundation of Chinese Medicine (2022ZZ002), the “Pioneer” and “Leading Goose” R&D Program of Zhejiang (2023C03075), The Key project of Basic Scientific Research Operating Funds of Hangzhou Medical College (KYZD202002), the Medical Science and Technology Project of Zhejiang Province (No.2019320552).
Acknowledgments
The authors gratefully acknowledge the editorial support of YZ in the preparation of this manuscript. The authors are fully responsible for all content and editorial decisions for this manuscript.
Conflict of interest
The authors declare that the research was conducted in the absence of any commercial or financial relationships that could be construed as a potential conflict of interest.
Publisher’s note
All claims expressed in this article are solely those of the authors and do not necessarily represent those of their affiliated organizations, or those of the publisher, the editors and the reviewers. Any product that may be evaluated in this article, or claim that may be made by its manufacturer, is not guaranteed or endorsed by the publisher.
References
1. dos Santos NA, Carvalho Rodrigues MA, Martins NM, dos Santos AC. Cisplatin-induced nephrotoxicity and targets of nephroprotection: an update. Arch Toxicol (2012) 86(8):1233–50. doi: 10.1007/s00204-012-0821-7
2. Safirstein R, Miller P, Guttenplan JB. Uptake and metabolism of cisplatin by rat kidney. Kidney Int (1984) 25(5):753–8. doi: 10.1038/ki.1984.86
3. Filipski KK, Loos WJ, Verweij J, Sparreboom A. Interaction of Cisplatin with the human organic cation transporter 2. Clin Cancer Res (2008) 14(12):3875–80. doi: 10.1158/1078-0432.CCR-07-4793
4. Ramesh G, Reeves WB. TNF-α mediates chemokine and cytokine expression and renal injury in cisplatin nephrotoxicity. J Clin Invest (2002) 110(6):835–42. doi: 10.1172/JCI200215606
5. Ramesh G, Reeves WB. p38 MAP kinase inhibition ameliorates cisplatin nephrotoxicity in mice. Am J Physiol Renal Physiol (2005) 289(1):F166–74. doi: 10.1152/ajprenal.00401.2004
6. Baek SH, Kim SH, Kim JW, Kim YJ, Lee KW, Na KY. Effects of a DPP4 inhibitor on cisplatin-induced acute kidney injury: study protocol for a randomized controlled trial. Trials (2015) 16:239. doi: 10.1186/s13063-015-0772-4
7. Malyszko J, Kozlowska K, Kozlowski L, Malyszko J. Nephrotoxicity of anticancer treatment. Nephrol Dial Transpl (2017) 32(6):924–36. doi: 10.1093/ndt/gfw338
8. Tiong HY, Huang P, Xiong S, Li Y, Vathsala A, Zink D. Drug-induced nephrotoxicity: clinical impact and preclinical in vitro models. Mol Pharm (2014) 11(7):1933–48. doi: 10.1021/mp400720w
9. Verzicco I, Regolisti G, Quaini F, Bocchi P, Brusasco I, Ferrari M, et al. Electrolyte disorders induced by antineoplastic drugs. Front Oncol (2020) 10:779. doi: 10.3389/fonc.2020.00779
10. Latcha S, Jaimes EA, Patil S, Glezerman IG, Mehta S, Flombaum CD. Long-term renal outcomes after cisplatin treatment. Clin J Am Soc Nephrol (2016) 11(7):1173–9. doi: 10.2215/CJN.08070715
11. Trevisani F, Di Marco F, Floris M, Pani A, Minnei R, Scartozzi M, et al. Renal function outcomes in metastatic non-small-cell lung carcinoma patients treated with chemotherapy or immune checkpoint inhibitors: an unexpected scenario. Vaccines (Basel) (2022) 10(5):679. doi: 10.3390/vaccines10050679
12. May J, Carson KR, Butler S, Liu W, Bartlett NL, Wagner-Johnston ND. High incidence of methotrexate associated renal toxicity in patients with lymphoma: a retrospective analysis. Leuk Lymphoma (2014) 55(6):1345–9. doi: 10.3109/10428194.2013.840780
13. Wiczer T, Dotson E, Tuten A, Phillips G, Maddocks K. Evaluation of incidence and risk factors for high-dose methotrexate-induced nephrotoxicity. J Oncol Pharm Pract (2016) 22(3):430–6. doi: 10.1177/1078155215594417
14. Latcha S, Gupta M, Lin IH, Jaimes EA. High dose methotrexate-induced acute kidney injury: incidence, risk factors, and recovery. Kidney Int Rep (2023) 8(2):360–4. doi: 10.1016/j.ekir.2022.10.029
15. Abdel-Rahman O, ElHalawani H. Proteinuria in patients with solid tumors treated with ramucirumab: A systematic review and meta-analysis. Chemotherapy (2014) 60(5-6):325–33. doi: 10.1159/000437253
16. Arnold D, Fuchs CS, Tabernero J, Ohtsu A, Zhu AX, Garon EB, et al. Meta-analysis of individual patient safety data from six randomized, placebo-controlled trials with the antiangiogenic VEGFR2-binding monoclonal antibody ramucirumab. Ann Oncol (2017) 28(12):2932–42. doi: 10.1093/annonc/mdx514
17. Lafrenie R, Zhang Z-F, Wang T, Liu L-H, Guo H-Q. Risks of proteinuria associated with vascular endothelial growth factor receptor tyrosine kinase inhibitors in cancer patients: A systematic review and meta-analysis. PLoS One (2014) 9(3):e90135. doi: 10.1371/journal.pone.0090135
18. Sorich MJ, Rowland A, Kichenadasse G, Woodman RJ, Mangoni AA. Risk factors of proteinuria in renal cell carcinoma patients treated with VEGF inhibitors: a secondary analysis of pooled clinical trial data. Br J Cancer (2016) 114(12):1313–7. doi: 10.1038/bjc.2016.147
19. Van Cutsem E, Tabernero J, Lakomy R, Prenen H, Prausova J, Macarulla T, et al. Addition of aflibercept to fluorouracil, leucovorin, and irinotecan improves survival in a phase III randomized trial in patients with metastatic colorectal cancer previously treated with an oxaliplatin-based regimen. J Clin Oncol (2012) 30(28):3499–506. doi: 10.1200/JCO.2012.42.8201
20. Peng D, Cai Y, Chen G, Hou M, Luo X, Dongzhi Z, et al. Efficacy and safety of apatinib versus sorafenib/placebo in first-line treatment for intermediate and advanced primary liver cancer: A systematic review and meta-analysis. Front Pharmacol (2023) 14:1101063. doi: 10.3389/fphar.2023.1101063
21. Hofmann L, Forschner A, Loquai C, Goldinger SM, Zimmer L, Ugurel S, et al. Cutaneous, gastrointestinal, hepatic, endocrine, and renal side-effects of anti-PD-1 therapy. Eur J Cancer (2016) 60:190–209. doi: 10.1016/j.ejca.2016.02.025
22. Wolchok JD, Chiarion-Sileni V, Gonzalez R, Rutkowski P, Grob JJ, Cowey CL, et al. Overall survival with combined nivolumab and ipilimumab in advanced melanoma. New Engl J Med (2017) 377(14):1345–56. doi: 10.1056/NEJMoa1709684
23. Weber JS, Hodi FS, Wolchok JD, Topalian SL, SChadendorf D, Larkin J, et al. Safety profile of nivolumab monotherapy: A pooled analysis of patients with advanced melanoma. J Clin Oncol (2017) 35(7):785–92. doi: 10.1200/JCO.2015.66.1389
24. Xu C, Chen YP, Du XJ, Liu JQ, Huang CL, Chen L, et al. Comparative safety of immune checkpoint inhibitors in cancer: systematic review and network meta-analysis. BMJ (2018) 363:k4226. doi: 10.1136/bmj.k4226
25. Cortazar FB, Marrone KA, Troxell ML, Ralto KM, Hoenig MP, Brahmer JR, et al. Clinicopathological features of acute kidney injury associated with immune checkpoint inhibitors. Kidney Int (2016) 90(3):638–47. doi: 10.1016/j.kint.2016.04.008
26. Shingarev R, Glezerman IG. Kidney complications of immune checkpoint inhibitors: A review. Am J Kidney Diseases (2019) 74(4):529–37. doi: 10.1053/j.ajkd.2019.03.433
27. Seethapathy H, Zhao S, Chute DF, Zubiri L, Oppong Y, Strohbehn I, et al. The incidence, causes, and risk factors of acute kidney injury in patients receiving immune checkpoint inhibitors. Clin J Am Soc Nephrol (2019) 14(12):1692–700. doi: 10.2215/CJN.00990119
28. Manohar S, Kompotiatis P, Thongprayoon C, Cheungpasitporn W, Herrmann J, Herrmann SM. Programmed cell death protein 1 inhibitor treatment is associated with acute kidney injury and hypocalcemia: meta-analysis. Nephrol Dial Transpl (2019) 34(1):108–17. doi: 10.1093/ndt/gfy105
29. Manohar S, Ghamrawi R, Chengappa M, Goksu BNB, KottsChade L, Finnes H, et al. Acute interstitial nephritis and checkpoint inhibitor therapy: single center experience of management and drug rechallenge. Kidney360 (2020) 1(1):16–24. doi: 10.34067/KID.0000152019
30. Seethapathy H, Zhao S, Strohbehn IA, Lee M, Chute DF, Bates H, et al. Incidence and clinical features of immune-related acute kidney injury in patients receiving programmed cell death ligand-1 inhibitors. Kidney Int Rep (2020) 5(10):1700–5. doi: 10.1016/j.ekir.2020.07.011
31. Meraz-Munoz A, Amir E, Ng P, Avila-Casado C, Ragobar C, Chan C, et al. Acute kidney injury associated with immune checkpoint inhibitor therapy: incidence, risk factors and outcomes. J Immunother Cancer (2020) 8(1):e000467. doi: 10.1136/jitc-2019-000467
32. Isik B, Alexander MP, Manohar S, Vaughan L, KottsChade L, Markovic S, et al. Biomarkers, clinical features, and rechallenge for immune checkpoint inhibitor renal immune-related adverse events. Kidney Int Rep (2021) 6(4):1022–31. doi: 10.1016/j.ekir.2021.01.013
33. Sorah JD, Rose TL, Radhakrishna R, Derebail VK, Milowsky MI. Incidence and prediction of immune checkpoint inhibitor-related nephrotoxicity. J Immunother (2021) 44(3):127–31. doi: 10.1097/CJI.0000000000000338
34. Abdelrahim M, Mamlouk O, Lin H, Lin J, Page V, Abdel-Wahab N, et al. Incidence, predictors, and survival impact of acute kidney injury in patients with melanoma treated with immune checkpoint inhibitors: a 10-year single-institution analysis. OncoImmunology (2021) 10(1):1927313. doi: 10.1080/2162402X.2021.1927313
35. Garcia-Carro C, Bolufer M, Bury R, Castaneda Z, Munoz E, Felip E, et al. Acute kidney injury as a risk factor for mortality in oncological patients receiving checkpoint inhibitors. Nephrol Dial Transpl (2022) 37(5):887–94. doi: 10.1093/ndt/gfab034
36. Baker ML, Yamamoto Y, Perazella MA, Dizman N, Shirali AC, Hafez N, et al. Mortality after acute kidney injury and acute interstitial nephritis in patients prescribed immune checkpoint inhibitor therapy. J Immunother Cancer (2022) 10(3):e004421. doi: 10.1136/jitc-2021-004421
37. Kanbay M, Copur S, Siriopol D, Yildiz AB, Berkkan M, Popa R, et al. The association between acute kidney injury and outcomes in cancer patients receiving immune checkpoint inhibitor therapy: a systematic review and meta-analysis. Clin Kidney J (2023) 16(5):817–26. doi: 10.1093/ckj/sfac194
38. Liu F, Wang Z, Li X, Zhang Z, Yang Y, Chen J, et al. Comparative risk of acute kidney injury among cancer patients treated with immune checkpoint inhibitors. Cancer Commun (Lond) (2023) 43(2):214–24. doi: 10.1002/cac2.12396
39. Hájek R, Masszi T, Petrucci MT, Palumbo A, Rosiñol L, Nagler A, et al. A randomized phase III study of carfilzomib vs low-dose corticosteroids with optional cyclophosphamide in relapsed and refractory multiple myeloma (FOCUS). Leukemia (2016) 31(1):107–14. doi: 10.1038/leu.2016.176
40. Richardson PG, Siegel DS, Vij R, Hofmeister CC, Baz R, Jagannath S, et al. Pomalidomide alone or in combination with low-dose dexamethasone in relapsed and refractory multiple myeloma: a randomized phase 2 study. Blood (2014) 123(12):1826–32. doi: 10.1182/blood-2013-11-538835
41. Seethapathy H, Lee MD, Strohbehn IA, Efe O, Rusibamayila N, Chute DF, et al. Clinical features of acute kidney injury in patients receiving dabrafenib and trametinib. Nephrol Dial Transpl (2022) 37(3):507–14. doi: 10.1093/ndt/gfaa372
42. Lebwohl D, Canetta R. Clinical development of platinum complexes in cancer therapy: an historical perspective and an update. Eur J Cancer (1998) 34(10):1522–34. doi: 10.1016/s0959-8049(98)00224-x
43. Wainford RD, Weaver RJ, Hawksworth GM. The immediate early genes, c-fos, c-jun and AP-1, are early markers of platinum analogue toxicity in human proximal tubular cell primary cultures. Toxicol In Vitro (2009) 23(5):780–8. doi: 10.1016/j.tiv.2009.04.004
44. Perazella MA. Onco-nephrology: renal toxicities of chemotherapeutic agents. Clin J Am Soc Nephrol (2012) 7(10):1713–21. doi: 10.2215/CJN.02780312
45. Izzedine H, Perazella MA. Anticancer drug-induced acute kidney injury. Kidney Int Rep (2017) 2(4):504–14. doi: 10.1016/j.ekir.2017.02.008
46. Camidge DR, Brosnan EM, DeSilva C, Koo PJ, Chonchol M. Crizotinib effects on creatinine and non-creatinine-based measures of glomerular filtration rate. J Thorac Oncol (2014) 9(11):1634–7. doi: 10.1097/JTO.0000000000000321
47. Perazella MA, Shirali AC. Nephrotoxicity of cancer immunotherapies: past, present and future. J Am Soc Nephrol (2018) 29(8):2039–52. doi: 10.1681/ASN.2018050488
48. Bonifant CL, Jackson HJ, Brentjens RJ, Curran KJ. Toxicity and management in CAR T-cell therapy. Mol Ther Oncolytics (2016) 3:16011. doi: 10.1038/mto.2016.11
49. Fitzgerald JC, Weiss SL, Maude SL, Barrett DM, Lacey SF, Melenhorst JJ, et al. Cytokine release syndrome after chimeric antigen receptor T cell therapy for acute lymphoblastic leukemia. Crit Care Med (2017) 45(2):e124–e31. doi: 10.1097/CCM.0000000000002053
50. Lee DW, Gardner R, Porter DL, Louis CU, Ahmed N, Jensen M, et al. Current concepts in the diagnosis and management of cytokine release syndrome. Blood (2014) 124(2):188–95. doi: 10.1182/blood-2014-05-552729
51. Frey NV, Porter DL. Cytokine release syndrome with novel therapeutics for acute lymphoblastic leukemia. Hematol Am Soc Hematol Educ Program (2016) 2016(1):567–72. doi: 10.1182/asheducation-2016.1.567
52. Lajer H, Kristensen M, Hansen HH, Nielsen S, Frokiaer J, Ostergaard LF, et al. Magnesium depletion enhances cisplatin-induced nephrotoxicity. Cancer Chemother Pharmacol (2005) 56(5):535–42. doi: 10.1007/s00280-005-1010-7
53. Hałka J, Spaleniak S, Kade G, Antosiewicz S, Sigorski D. The nephrotoxicity of drugs used in causal oncological therapies. Curr Oncol (2022) 29(12):9681–94. doi: 10.3390/curroncol29120760
54. Perazella MA, Moeckel GW. Nephrotoxicity from chemotherapeutic agents: clinical manifestations, pathobiology, and prevention/therapy. Semin Nephrol (2010) 30(6):570–81. doi: 10.1016/j.semnephrol.2010.09.005
55. Sahni V, Choudhury D, Ahmed Z. Chemotherapy-associated renal dysfunction. Nat Rev Nephrol (2009) 5(8):450–62. doi: 10.1038/nrneph.2009.97
56. Widemann BC, Adamson PC. Understanding and managing methotrexate nephrotoxicity. Oncologist (2006) 11(6):694–703. doi: 10.1634/theoncologist.11-6-694
57. Perazella MA, Izzedine H. New drug toxicities in the onco-nephrology world. Kidney Int (2015) 87(5):909–17. doi: 10.1038/ki.2015.30
58. Glezerman IG, Pietanza MC, Miller V, Seshan SV. Kidney tubular toxicity of maintenance pemetrexed therapy. Am J Kidney Dis (2011) 58(5):817–20. doi: 10.1053/j.ajkd.2011.04.030
59. Izzedine H, Ederhy S, Goldwasser F, Soria JC, Milano G, Cohen A, et al. Management of hypertension in angiogenesis inhibitor-treated patients. Ann Oncol (2009) 20(5):807–15. doi: 10.1093/annonc/mdn713
60. Izzedine H, Massard C, Spano JP, Goldwasser F, Khayat D, Soria JC. VEGF signalling inhibition-induced proteinuria: Mechanisms, significance and management. Eur J Cancer (2010) 46(2):439–48. doi: 10.1016/j.ejca.2009.11.001
61. Soria JC, Massard C, Izzedine H. From theoretical synergy to clinical supra-additive toxicity. J Clin Oncol (2009) 27(9):1359–61. doi: 10.1200/JCO.2008.20.8595
62. Vigneau C, Lorcy N, Dolley-Hitze T, Jouan F, Arlot-Bonnemains Y, Laguerre B, et al. All anti-vascular endothelial growth factor drugs can induce 'pre-eclampsia-like syndrome': a RARe study. Nephrol Dial Transpl (2014) 29(2):325–32. doi: 10.1093/ndt/gft465
63. Gurevich F, Perazella MA. Renal effects of anti-angiogenesis therapy: update for the internist. Am J Med (2009) 122(4):322–8. doi: 10.1016/j.amjmed.2008.11.025
64. Izzedine H, Rixe O, Billemont B, Baumelou A, Deray G. Angiogenesis inhibitor therapies: focus on kidney toxicity and hypertension. Am J Kidney Dis (2007) 50(2):203–18. doi: 10.1053/j.ajkd.2007.04.025
65. Eremina V, Jefferson JA, Kowalewska J, Hochster H, Haas M, Weisstuch J, et al. VEGF inhibition and renal thrombotic microangiopathy. New Engl J Med (2008) 358(11):1129–36. doi: 10.1056/NEJMoa0707330
66. Launay-Vacher V, Aapro M, De Castro G Jr, Cohen E, Deray G, Dooley M, et al. Renal effects of molecular targeted therapies in oncology: a review by the Cancer and the Kidney International Network (C-KIN). Ann Oncol (2015) 26(8):1677–84. doi: 10.1093/annonc/mdv136
67. Cosmai L, Gallieni M, Liguigli W, Porta C. Renal toxicity of anticancer agents targeting vascular endothelial growth factor (VEGF) and its receptors (VEGFRs). J Nephrol (2017) 30(2):171–80. doi: 10.1007/s40620-016-0311-8
68. Sugimoto H, Hamano Y, Charytan D, Cosgrove D, Kieran M, Sudhakar A, et al. Neutralization of circulating vascular endothelial growth factor (VEGF) by anti-VEGF antibodies and soluble VEGF receptor 1 (sFlt-1) induces proteinuria. J Biol Chem (2003) 278(15):12605–8. doi: 10.1074/jbc.C300012200
69. De Stefano A, Carlomagno C, Pepe S, Bianco R, De Placido S. Bevacizumab-related arterial hypertension as a predictive marker in metastatic colorectal cancer patients. Cancer Chemother Pharmacol (2011) 68(5):1207–13. doi: 10.1007/s00280-011-1604-1
70. Postow MA, Sidlow R, Hellmann MD. Immune-related adverse events associated with immune checkpoint blockade. New Engl J Med (2018) 378(2):158–68. doi: 10.1056/NEJMra1703481
71. Champiat S, Lambotte O, Barreau E, Belkhir R, Berdelou A, Carbonnel F, et al. Management of immune checkpoint blockade dysimmune toxicities: a collaborative position paper. Ann Oncol (2016) 27(4):559–74. doi: 10.1093/annonc/mdv623
72. Izzedine H, Gueutin V, Gharbi C, Mateus C, Robert C, Routier E, et al. Kidney injuries related to ipilimumab. Invest New Drugs (2014) 32(4):769–73. doi: 10.1007/s10637-014-0092-7
73. Voskens CJ, Goldinger SM, Loquai C, Robert C, Kaehler KC, Berking C, et al. The price of tumor control: an analysis of rare side effects of anti-CTLA-4 therapy in metastatic melanoma from the ipilimumab network. PloS One (2013) 8(1):e53745. doi: 10.1371/journal.pone.0053745
74. Fadel F, El Karoui K, Knebelmann B. Anti-CTLA4 antibody-induced lupus nephritis. New Engl J Med (2009) 361(2):211–2. doi: 10.1056/NEJMc0904283
75. Forde PM, Rock K, Wilson G, O’Byrne KJ. Ipilimumab-induced immune-related renal failure–a case report. Anticancer Res (2012) 32(10):4607–8.
76. Chmiel KD, Suan D, Liddle C, Nankivell B, Ibrahim R, Bautista C, et al. Resolution of severe ipilimumab-induced hepatitis after antithymocyte globulin therapy. J Clin Oncol (2011) 29(9):e237–40. doi: 10.1200/JCO.2010.32.2206
77. Shah R, Beem E, Sautina L, Zharikov SI, Segal MS. Mitomycin- and calcineurin-associated HUS, endothelial dysfunction and endothelial repair: a new paradigm for the puzzle? Nephrol Dial Transplant (2007) 22(2):617–20. doi: 10.1093/ndt/gfl586
78. Batts ED, Sanchorawala V, Hegerfeldt Y, Lazarus HM. Azotemia associated with use of lenalidomide in plasma cell dyscrasias. Leuk Lymphoma (2008) 49(6):1108–15. doi: 10.1080/10428190802023707
79. Lipson EJ, Huff CA, Holanda DG, McDevitt MA, Fine DM. Lenalidomide-induced acute interstitial nephritis. Oncologist (2010) 15(9):961–4. doi: 10.1634/theoncologist.2010-0070
80. Baird P, Leung S, Hoang H, Babalola O, Devoe CE, Wanchoo R, et al. A case of acute kidney injury from crystal nephropathy secondary to pomalidomide and levofloxacin use. J Oncol Pharm Pract (2016) 22(2):357–60. doi: 10.1177/1078155214568581
81. Tonooka A, Ohashi R. Current trends in anti-cancer molecular targeted therapies: renal complications and their histological features. J Nippon Med Sch (2022) 89(2):128–38. doi: 10.1272/jnms.JNMS.2022_89-221
82. Cosmai L, Gallieni M, Porta C. Renal toxicity of anticancer agents targeting HER2 and EGFR. J Nephrol (2015) 28(6):647–57. doi: 10.1007/s40620-015-0226-9
83. Wanchoo R, Jhaveri KD, Deray G, Launay-Vacher V. Renal effects of BRAF inhibitors: a systematic review by the Cancer and the Kidney International Network. Clin Kidney J (2016) 9(2):245–51. doi: 10.1093/ckj/sfv149
84. Perazella MA. Pharmacology behind common drug nephrotoxicities. Clin J Am Soc Nephrol (2018) 13(12):1897–908. doi: 10.2215/CJN.00150118
85. Wilmer MJ, Ng CP, Lanz HL, Vulto P, Suter-Dick L, Masereeuw R. Kidney-on-a-chip technology for drug-induced nephrotoxicity screening. Trends Biotechnol (2016) 34(2):156–70. doi: 10.1016/j.tibtech.2015.11.001
86. Jang K-J, Mehr AP, Hamilton GA, McPartlin LA, Chung S, Suh K-Y, et al. Human kidney proximal tubule-on-a-chip for drug transport and nephrotoxicity assessment. Integr Biol (2013) 5(9):1119–29. doi: 10.1039/c3ib40049b
87. Gaspari F, Cravedi P, Mandala M, Perico N, de Leon FR, Stucchi N, et al. Predicting cisplatin-induced acute kidney injury by urinary neutrophil gelatinase-associated lipocalin excretion: a pilot prospective case-control study. Nephron Clin Pract (2010) 115(2):c154–60. doi: 10.1159/000312879
88. Peterson PA, Evrin PE, Berggård I. Differentiation of glomerular, tubular, and normal proteinuria: determinations of urinary excretion of beta-2-macroglobulin, albumin, and total protein. J Clin Invest (1969) 48(7):1189–98. doi: 10.1172/JCI106083
89. George B, Joy MS, Aleksunes LM. Urinary protein biomarkers of kidney injury in patients receiving cisplatin chemotherapy. Exp Biol Med (Maywood) (2018) 243(3):272–82. doi: 10.1177/1535370217745302
90. Bökenkamp A, van Wijk JA, Lentze MJ, Stoffel-Wagner B. Effect of corticosteroid therapy on serum cystatin C and beta2-microglobulin concentrations. Clin Chem (2002) 48(7):1123–6.
91. Manetti L, Pardini E, Genovesi M, Campomori A, Grasso L, Morselli LL, et al. Thyroid function differently affects serum cystatin C and creatinine concentrations. J Endocrinol Invest (2005) 28(4):346–9. doi: 10.1007/BF03347201
92. Risch L, Huber AR. Glucocorticoids and increased serum cystatin C concentrations. Clin Chim Acta (2002) 320(1-2):133–4. doi: 10.1016/s0009-8981(02)00044-x
93. Takashi M, Zhu Y, Miyake K, Kato K. Urinary 28-kD calbindin-D as a new marker for damage to distal renal tubules caused by cisplatin-based chemotherapy. Urol Int (1996) 56(3):174–9. doi: 10.1159/000282835
94. Aregger F, Uehlinger DE, Witowski J, Brunisholz RA, Hunziker P, Frey FJ, et al. Identification of IGFBP-7 by urinary proteomics as a novel prognostic marker in early acute kidney injury. Kidney Int (2014) 85(4):909–19. doi: 10.1038/ki.2013.363
95. Bigg HF, Morrison CJ, Butler GS, Bogoyevitch MA, Wang Z, Soloway PD, et al. Tissue inhibitor of metalloproteinases-4 inhibits but does not support the activation of gelatinase A via efficient inhibition of membrane type 1-matrix metalloproteinase. Cancer Res (2001) 61(9):3610–8.
96. Pianta TJ, Pickering JW, Succar L, Chin M, Davidson T, Buckley NA, et al. Dexamethasone modifies cystatin C-based diagnosis of acute kidney injury during cisplatin-based chemotherapy. Kidney Blood Press Res (2017) 42(1):62–75. doi: 10.1159/000469715
97. George B, Wen X, Mercke N, Gomez M, O'Bryant C, Bowles DW, et al. Profiling of kidney injury biomarkers in patients receiving cisplatin: time-dependent changes in the absence of clinical nephrotoxicity. Clin Pharmacol Ther (2017) 101(4):510–8. doi: 10.1002/cpt.606
98. Prodjosudjadi W, Daha MR, Gerritsma JS, Florijn KW, Barendregt JN, Bruijn JA, et al. Increased urinary excretion of monocyte chemoattractant protein-1 during acute renal allograft rejection. Nephrol Dial Transpl (1996) 11(6):1096–103.
99. Harrison DJ, Kharbanda R, Cunningham DS, McLellan LI, Hayes JD. Distribution of glutathione S-transferase isoenzymes in human kidney: basis for possible markers of renal injury. J Clin Pathol (1989) 42(6):624–8. doi: 10.1136/jcp.42.6.624
100. Maatman RG, Van Kuppevelt TH, Veerkamp JH. Two types of fatty acid-binding protein in human kidney. Isolation, characterization and localization. Biochem J (1991) 273(Pt 3):759–66. doi: 10.1042/bj2730759
101. Yan J, Gong Y, She YM, Wang G, Roberts MS, Burczynski FJ. Molecular mechanism of recombinant liver fatty acid binding protein's antioxidant activity. J Lipid Res (2009) 50(12):2445–54. doi: 10.1194/jlr.M900177-JLR200
102. Fuchs TC, Frick K, Emde B, Czasch S, von Landenberg F, Hewitt P. Evaluation of novel acute urinary rat kidney toxicity biomarker for subacute toxicity studies in preclinical trials. Toxicol Pathol (2012) 40(7):1031–48. doi: 10.1177/0192623312444618
103. Pianta TJ, Succar L, Davidson T, Buckley NA, Endre ZH. Monitoring treatment of acute kidney injury with damage biomarkers. Toxicol Lett (2017) 268:63–70. doi: 10.1016/j.toxlet.2017.01.001
104. Pinches MD, Betts CJ, Bickerton SJ, Beattie L, Burdett LD, Thomas HT, et al. Evaluation of novel urinary renal biomarkers: biological variation and reference change values. Toxicologic Pathol (2012) 40(3):541–9. doi: 10.1177/0192623311432436
105. Vinken P, Starckx S, Barale-Thomas E, Looszova A, Sonee M, Goeminne N, et al. Tissue Kim-1 and urinary clusterin as early indicators of cisplatin-induced acute kidney injury in rats. Toxicol Pathol (2012) 40(7):1049–62. doi: 10.1177/0192623312444765
106. Wadey RM, Pinches MG, Jones HB, Riccardi D, Price SA. Tissue expression and correlation of a panel of urinary biomarkers following cisplatin-induced kidney injury. Toxicol Pathol (2014) 42(3):591–602. doi: 10.1177/0192623313492044
107. Sprangers B, Leaf DE, Porta C, Soler MJ, Perazella MA. Diagnosis and management of immune checkpoint inhibitor-associated acute kidney injury. Nat Rev Nephrol (2022) 18(12):794–805. doi: 10.1038/s41581-022-00630-8
108. Moledina DG, Wilson FP, Pober JS, Perazella MA, Singh N, Luciano RL, et al. Urine TNF-alpha and IL-9 for clinical diagnosis of acute interstitial nephritis. JCI Insight (2019) 4(10):e127456. doi: 10.1172/jci.insight.127456
Keywords: antineoplastic drugs, nephrotoxicity, renal damage, mechanisms, early markers
Citation: Tang J, Yang N, Pan S, Ren P, Chen M, Jin J, He Q and Zeng Y (2023) The renal damage and mechanisms relevant to antitumoral drugs. Front. Oncol. 13:1331671. doi: 10.3389/fonc.2023.1331671
Received: 01 November 2023; Accepted: 22 November 2023;
Published: 12 December 2023.
Edited by:
Qingbin Cui, University of Toledo College of Medicine and Life Sciences, United StatesReviewed by:
Yang Tian, University of Arkansas, United StatesXiaolin Qian, Southern Research Institute, United States
Huang Yangyu, Sun Yat-sen University Cancer Center (SYSUCC), China
Copyright © 2023 Tang, Yang, Pan, Ren, Chen, Jin, He and Zeng. This is an open-access article distributed under the terms of the Creative Commons Attribution License (CC BY). The use, distribution or reproduction in other forums is permitted, provided the original author(s) and the copyright owner(s) are credited and that the original publication in this journal is cited, in accordance with accepted academic practice. No use, distribution or reproduction is permitted which does not comply with these terms.
*Correspondence: Yuqun Zeng, zengyuqun401@163.com; Juan Jin, lang_018@163.com; Qiang He, qianghe1973@126.com
†These authors have contributed equally to this work