- Centro E. Grossi Paoletti, Dipartimento di Scienze Farmacologiche e Biomolecolari, Università degli Studi di Milano, Milan, Italy
Several lines of evidence suggest that, besides being a strong independent predictor of the occurrence of primary coronary events, a low plasma high density lipoprotein (HDL) cholesterol level is also associated with short- and long-term unfavorable prognosis in patients, who have recovered from a myocardial infarction, suggesting a direct detrimental effect of low HDL on post-ischemic myocardial function. Experiments performed in ex vivo and in vivo models of myocardial ischemia/reperfusion (I/R) injury have clearly shown that HDL are able to preserve cardiac function when given before ischemia or at reperfusion; the protective effects of HDL against I/R injury have been also confirmed in other tissues and organs, as brain and hind limb. HDL were shown to act on coronary endothelial cells, by limiting the increase of endothelium permeability and promoting vasodilation and neoangiogenesis, on white blood cells, by reducing their infiltration into the ischemic tissue and the release of pro-inflammatory and matrix-degrading molecules, and on cardiomyocytes, by preventing the activation of the apoptotic cascade. Synthetic HDL retains the cardioprotective activity of plasma-derived HDL and may become a useful adjunctive therapy to improve clinical outcomes in patients with acute coronary syndromes or undergoing coronary procedures.
A series of large population studies have revealed the existence of a strong inverse correlation between plasma levels of high density lipoprotein cholesterol (HDL-C) and the incidence of coronary heart disease; interestingly, the inverse relation is observed at all levels of low density lipoprotein cholesterol, including very low concentrations achieved in statin-treated patients (Barter et al., 2007). The same relationship was also found for the incidence of ischemic cerebrovascular events (Koren-Morag et al., 2002; Soyama et al., 2003).
Besides being independent predictors of the incidence of acute ischemic diseases, low plasma levels of HDL-C were shown to be associated to an unfavorable prognosis; indeed, plasma levels of HDL-C at admission are inversely associated with the severity of the ischemic insult, with 1-year mortality, and with the recurrence of subsequent clinical events (Olsson et al., 2005; Ghazzal et al., 2009; Yeh et al., 2013). The relationship between HDL-C and prognosis is not likely due to an accelerated atherogenesis, but may suggest a detrimental effects of low HDL on post-ischemic recovery; low levels of HDL-C are indeed associated with post-ischemic left ventricular dysfunction independent of coronary atherosclerosis severity (Kempen et al., 1987; Wang et al., 1998, 1999). Thus, in recent years several studies have been devoted to assess whether plasma-derived and synthetic HDL (sHDL) could reduce ischemic injury using in vitro, ex vivo, and in vivo models, and to investigate the HDL components and the mechanisms responsible for the protective effects.
HDL and Myocardial I/R Injury
Acute myocardial infarction is the result of the occlusion of an epicardial coronary artery, generally due to the sudden rupture of an atherosclerotic plaque, limiting the perfusion of the cardiac region distal to the occlusion site (the so-called “area at risk”). If a timely reperfusion is not achieved and no collateral circulation is present, cardiomyocytes will be irreversibly damaged and substituted by fibrous tissue with impairment of contractile function. Thus, reperfusion is mandatory to limit infarct size and improving post-ischemic cardiac function; however, reperfusion itself is associated with additional myocardial damage, generating the definition of “ischemia/reperfusion (I/R) injury” (Carden and Granger, 2000).
To evaluate the I/R pathology and possible therapeutic approaches to limit I/R damage, different models can be used, ranging from ex vivo isolated hearts to in vivo ligation of coronary arteries in small to large mammals. Isolated hearts are useful for their easiness of manipulation (i.e., duration and nature of I/R) and allow a complete biochemical, physiological, and morphological evaluation. However, the isolated heart has a limited life-span and does not allow to evaluate the role of hemodynamic and systemic factors, as in vivo models do (i.e., permanent or temporary ligation of the left descending coronary artery, LAD; Hearse and Sutherland, 2000). Small animals as rodents are of great help for their ease of breeding, low cost, and availability of transgenic models, but their translational relevance is limited by the huge anatomical and physiological differences with humans; large animals as pigs, dogs, and primates surely display a much closer phenotype to humans and represent a mandatory step toward clinical trials, but still suffer of differences in the extent of collateral blood flow and resistance to infarct development (Ibanez et al., 2015).
Plasma-derived HDL were first shown to limit I/R injury in an ex vivo model on isolated rat hearts retroperfused through the aorta according to the Langendorff technique (Calabresi et al., 2003b). A moderate I/R injury was induced by reducing the perfusion flow for 20 min and then reperfusing the hearts for additional 30 min; this protocol resulted in an remarkable post-ischemic impairment of contractile function, increase of coronary resistance to perfusion flow and cardiac necrosis. In this model, HDL significantly limited I/R injury, when given immediately before ischemia or during the first minutes of reperfusion; the ability of HDL to limit I/R injury even when administered after ischemia could be clinically relevant and was further confirmed in isolated mice hearts treated with HDL at reperfusion (Frias et al., 2013).
Cardioprotection by HDL against I/R injury was definitely proven in vivo. HDL were given to mice 30 min before the ligation of left descending coronary artery; after 30 min, blood flow was restored for additional 24 h. Mice treated with HDL displayed a large reduction of infarct size with less myocyte necrosis and inflammation compared to vehicle-treated animals (Theilmeier et al., 2006). In agreement with this evidence, mice lacking apoA-I: display increased infarct size after LAD in a gene-dose-dependent way (Dadabayev et al., 2014).
sHDL and Myocardial I/R Injury
The translational potential of HDL as cardioprotective agents against I/R injury is hampered by several difficulties, including their heterogeneity and safety concerns; these limitations can be overcome by the use of sHDL. These particles are usually composed by recombinant proteins as apoA-I, the main protein component of HDL, and phospholipids, generally phosphatidylcholine, and are now undergoing the clinical phase of development as plaque-stabilizers in the context of an acute coronary syndrome (ACS; Krause and Remaley, 2013). By using the same ex vivo experimental protocol described above, we showed that these sHDL are able to limit I/R injury when given before or after the ischemic insult and that the two sHDL components, apoA-I and phospholipids, are not effective when given alone (Rossoni et al., 2004). However, the effect of sHDL is about 50% lower than that of plasma-derived particles, suggesting that the basic sHDL composition should be modified in order to achieve maximal cardioprotective activity. Marchesi et al. (2004) confirmed the sHDL-mediated cardioprotection against I/R injury in vivo by testing the administration of sHDL containing the apoA-I Milano variant to rabbits before temporary LAD; at reperfusion infarct size was reduced in sHDL-treated compared to vehicle-treated animals. Several agents are able to inhibit infarct size in animal models, however, a parallel improvement of cardiac function has not been always observed. HDL were instead shown to limit the decline of contractile function and the increase of coronary resistance to perfusion flow; in addition, sHDL are able to reduce the duration of ventricular tachycardia or fibrillation at reperfusion when given to rats before LAD (Imaizumi et al., 2008). Contrary to what observed ex vivo, where apoA-I had to be associated with phospholipids to exert cardioprotection, infarct size after LAD was significantly reduced in rats infused with unlipidated apoA-I 10 min before artery ligation (Hibert et al., 2013); this discrepancy is likely explained by the rapid interaction of infused apoA-I with circulating lipoproteins and the increase of endogenous HDL pool, which is not possible in blood-free experimental settings.
As stated above, an optimization of sHDL composition is needed to achieve a cardioprotective effect comparable to that of plasma-derived lipoproteins. Changes could involve the protein and/or the lipid components, and the inclusion of proteins that circulate in the bloodstream bound to HDL. For what concerns the protein composition, apoA-I can be substituted by apoA-I mimetic peptides, which surely represents an advantage for the large-scale preparation of sHDL, or apoA-I variants. In the ex vivo model of I/R injury, particles containing 37 pA displayed the same cardioprotective activity of those with apoA-I (Gomaraschi et al., 2008), while sHDL containing the apoA-I Milano variant display a superior protection against I/R injury in terms of reduction of cardiomyocyte damage (Calabresi et al., 2006; Marchesi et al., 2008). Regarding the lipid composition, a key indication comes from the study by Theilmeier et al. (2006), in which the cardioprotective effect of plasma-derived HDL was shown to be mediated by the interaction of the carried sphingolipid sphingosine-1-phosphate (S1P) with its G-protein coupled receptor. The addition of S1P to sHDL containing apoA-I and phosphatidylcholine resulted in a significant increase of sHDL-mediated cardioprotection ex vivo and in vivo (Brulhart-Meynet et al., 2015).
HDL and I/R Injury in Other Tissues
Some evidences suggest that the protective role of HDL against I/R injury is not limited to the cardiac muscle but could be extended to other organs and tissues. In particular, recent studies demonstrated that HDL can limit I/R injury also in brain, hindlimb, kidney, intestine, liver, and lung. Both plasma-derived and sHDL were tested in stroke models. In the first study, cerebral ischemia was induced in rats by temporary ligation of the middle cerebral artery (MCA) for 30 min and apoA-I containing sHDL were infused before ischemia; a dramatic reduction of brain necrotic area was observed in animals treated with sHDL compared to vehicle-infused ones 24 h after MCA reopening (Paterno et al., 2004). The effect of plasma-derived HDL was tested in a model of focal cerebral ischemia induced by embolization of a preformed clot in the MCA; in this case, HDL were administered immediately after stroke onset and caused marked reductions of mortality rate, infarct volume, blood–brain barrier breakdown, and neurological deficits at 24 h (Lapergue et al., 2010). Interestingly, HDL exerted their protective effects even when infused up to 5 h after stroke onset. Using the same experimental model HDL were also shown to decrease the rate of mortality and hemorrhagic transformation induced by tissue plasminogen activator (Lapergue et al., 2013). The protective effects of sHDL and unlipidated apoA-I were assessed in the context of hindlimb ischemia. Unilateral hindlimb ischemia was induced by resecting the right femoral and saphenous artery and apoA-I containing sHDL were injected twice per week, starting 1 week before surgery; 4 weeks after surgery, animals treated with sHDL displayed superior blood flow recovery and capillary density than vehicle-treated animals (Sumi et al., 2007). Similarly, the infusion of apoA-I every second day after ligation of the femoral artery increased neovessel formation and blood perfusion in mice (Prosser et al., 2014). HDL markedly reduced renal dysfunction and I/R injury when given before arterial and venous clamping: at reperfusion serum, urinary and histological markers confirmed less renal dysfunction and cellular damage in HDL-treated rats (Thiemermann et al., 2003). Intestinal I/R injury was also attenuated by sHDL: when given before splanchnic artery occlusion, sHDL limited histological and clinical signs of ileum injury and the infiltration of inflammatory cells at reperfusion (Cuzzocrea et al., 2004). The overall protective effect of both plasma-derived and sHDL against I/R injury is well recapitulated in a model of multiple organ dysfunction syndrome; this syndrome is a typical consequence of hemorrhagic shock in which I/R injury occurs at several organs due to a reduced blood flow and oxygen supply. A rapid fall of arterial pressure was obtained by blood withdrawn from rat carotid artery and subsequent resuscitation by reinjection of blood and isotonic saline (Cockerill et al., 2001b). The administration of HDL or sHDL immediately before resuscitation reduced I/R damage in the liver, kidneys, pancreas, brain, muscles, lung, and intestine, as demonstrated by serum markers of cell necrosis (i.e., transaminases, creatinine, creatinine kinase, etc.) and histological analyses: organs from HDL-treated rats showed less edema and loss of normal tissue structure, less cellular infiltration and endothelial/epithelial expression of cell adhesion molecules (CAMs; Cockerill et al., 2001b).
Mechanisms of HDL Protection Against I/R Injury
In recent years, several studies have been performed to elucidate the mechanisms underlying plasma-derived and sHDL-mediated protection against I/R injury. The proposed mechanisms can be divided into two groups: the stimulation of endogenous protective responses and the inhibition of damaging processes (Figure 1). Protective responses are aimed at sustaining cell viability and at ensuring the maximal perfusion of ischemic tissues. HDL can promote the activation of different pro-survival pathways, as the survivor activating factor enhancement pathway (SAFE; Frias et al., 2013). In addition, HDL administration causes the release of molecules favoring vasodilation and myocyte relaxation as prostanoids and nitric oxide ex vivo and in vivo (Calabresi et al., 2003b; Theilmeier et al., 2006). The increase of HDL-mediated NO production through the PI3K/Akt pathway is almost blunted in the absence of the S1P3 receptor and is involved in the anti-arrhythmogenic effect of HDL (Theilmeier et al., 2006; Imaizumi et al., 2008).
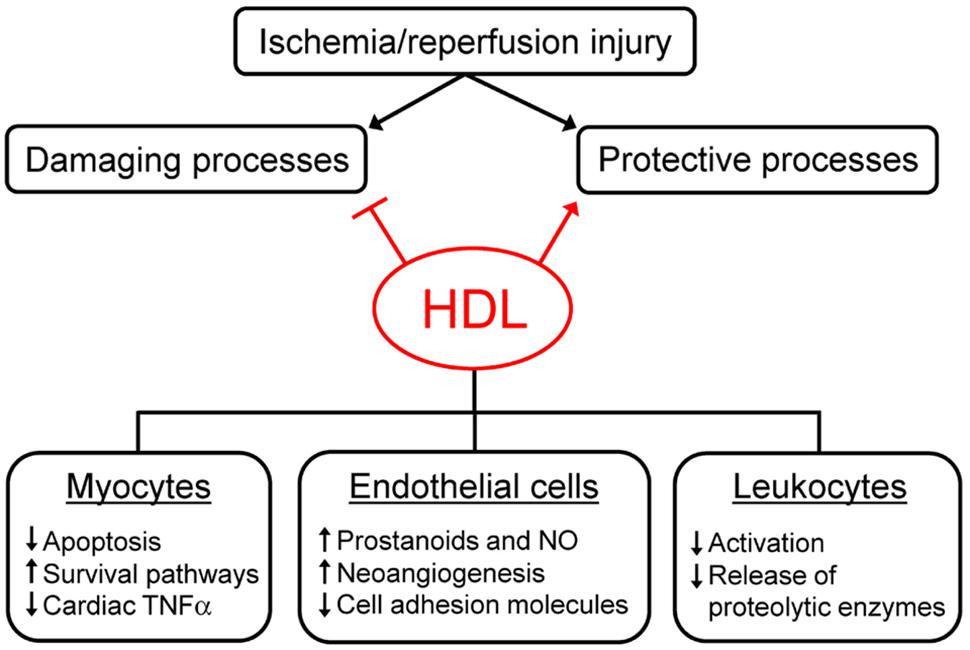
FIGURE 1. Mechanisms of high density lipoprotein (HDL)-mediated protection against ischemia/reperfusion injury. HDL can reduce ischemia/reperfusion (I/R) injury by inhibiting damaging processes and by promoting protective responses elicited by tissues after ischemia/reperfusion. HDL exert their effect on different cell types, as cardiomyocytes, endothelial cells, and leukocytes. See text for details.
Damaging responses are generally consequent to the activation of the inflammatory cascade, the infiltration of circulating leukocytes and the increased oxidative stress. HDL can limit the alterations of endothelial barrier permeability, the extravasation of circulating cells and the release of pro-inflammatory and pro-oxidant molecules like cytokines, chemokines, and reactive oxygen species. In particular, HDL modulate cardiac TNFα content, which seems crucial in the early phase of I/R injury, since it combines the capacity to directly affect myocyte function with the ability to trigger an inflammatory response (Frangogiannis et al., 1998; Meldrum, 1998). Preformed TNFα can be immediately released in the ischemic tissue by the degranulation of resident mast cells and the cleavage of membrane-bound TNFα (Frangogiannis et al., 1998; Kupatt et al., 1999), causing a significant increase of TNFα levels in ischemic hearts (Calabresi et al., 2003b). Both plasma-derived and sHDL act as TNFα scavengers, able to bind TNFα and to remove it from the ischemic tissue, thus preventing its damaging effects (Calabresi et al., 2003b; Rossoni et al., 2004). The mechanisms outlined above suggest that HDL-mediated protection against I/R injury is due to their ability to act on myocytes, but also on endothelial cells and infiltrating leukocytes, as confirmed in cell studies mimicking I/R injury through hypoxia-reoxygenation and glucose deprivation protocols (Figure 1).
Cardiomyocytes
High density lipoprotein limits cardiomyocyte apoptosis in the ischemic area following LAD (Theilmeier et al., 2006). These findings were confirmed in vitro on cardiomyocytes under glucose deprivation or subjected to hypoxia and are linked to the inhibition of the mitochondrial permeability transition pore opening consequent to the activation of the SAFE pathway (Theilmeier et al., 2006; Frias et al., 2013). The preservation of myocyte viability after hypoxia-reoxygenation is mediated by S1P through its receptors S1P1 and S1P3 and the subsequent activation of the PI3K/Akt and the MEK/ERK pathways (Tao et al., 2010). The central role of mitochondria as targets of HDL-mediated myocyte survival after I/R is confirmed by two evidences. First, the administration of sHDL prevents mitochondrial granulation and disorganization in isolated hearts undergoing I/R (Marchesi et al., 2008). Second, apoA-I-deficient mice display impaired mitochondrial function resulting in higher infarct size after LAD compared to wild-type mice. Interestingly, the administration of coenzyme Q to apoA-I null mice normalizes electron transfer rate from complex II to complex III of the electron transfer chain and reduces infarct size (Dadabayev et al., 2014).
Endothelial Cells
The HDL-mediated increase of prostanoids and nitric oxide levels after I/R suggests that HDL-mediated cardioprotection is due at least in part to their role in promoting vasodilation. In addition, it is well known that both HDL and sHDL can inhibit the expression of CAMs, which favor circulating cell adhesion to the endothelial layer (Barter et al., 2002; Calabresi et al., 2003a); indeed, HDL can limit the expression of CAMs, neutrophil binding and transmigration in activated endothelial cells and in several in vivo models of I/R or acute inflammation (Cockerill et al., 2001a,b; Paterno et al., 2004; Theilmeier et al., 2006).
Interestingly, HDL increase hypoxia-mediated angiogenesis in coronary artery endothelial cells; HDL promote endothelial cell migration, proliferation, and tubulogenesis by acting on the post-translational regulation of the hypoxia-inducible factor-1/vascular endothelial growth factor axis, with a mechanism requiring HDL interaction with the scavenger receptor SR-BI and the activation of PI3K pathway (Prosser et al., 2014; Tan et al., 2014). The pro-angiogenic effect of HDL can be also mediated by their ability to promote the differentiation of peripheral blood monocytes into endothelial progenitor cells, via activation of the PI3K/Akt/NO pathway (Sumi et al., 2007).
Infiltrating Cells
An HDL-mediated reduction of neutrophil recruitment in the ischemic tissues was demonstrated in vivo in different models of I/R (Cockerill et al., 2001b; Thiemermann et al., 2003; Cuzzocrea et al., 2004; Theilmeier et al., 2006; Lapergue et al., 2010); infiltrating cells may considerably contribute to I/R injury by releasing molecules and enzymes with pro-inflammatory, pro-oxidant, and proteolytic activities. HDL activity on infiltrating cells could be particularly relevant in cerebral ischemia, where the breakdown of the blood brain barrier allows the extravasation of plasma proteins and leukocytes and the development of cerebral edema (Ballabh et al., 2004). Activated leukocytes are thought to take part in this process, since after degranulation they release enzymes responsible for the proteolysis of extracellular matrix (Gidday et al., 2005). A recent elegant study performed on cerebral endothelial cells and leukocytes under oxygen-glucose deprivation conditions showed that the presence of HDL helped in maintaining endothelial layer integrity by inhibiting leukocytes activation (Bao et al., 2013).
Conclusion
Plasma-derived and sHDL have been clearly shown to exert protective effects against I/R injury in animal models. The protective effects are related to HDL ability to interfere with cellular processes known to play a role in myocardial I/R injury. Interestingly, sHDL are cardioprotective even when administered after the ischemic insult, supporting the therapeutic potential of sHDL infusion to reduce I/R injury. sHDL are currently under clinical development for plaque stabilization/regression in the setting of ACS, primarily because of their ability to rapidly remove cholesterol from the arterial wall (Nissen et al., 2003; Tardif et al., 2007). If administered promptly after ACS, sHDL could also promote acute protection against I/R damage. Based on pre-clinical data summarized in the review, this hypothesis warrants clinical investigation, and could be also extended to other organs, since the pathologic mechanisms targeted by HDL in myocardial I/R are likely not specific to the heart.
Author Contributions
MG has drafted the work; MG, LC, and GF have critically revised the work and approved the final version to be published.
Conflict of Interest Statement
The authors declare that the research was conducted in the absence of any commercial or financial relationships that could be construed as a potential conflict of interest.
References
Ballabh, P., Braun, A., and Nedergaard, M. (2004). The blood-brain barrier: an overview: structure, regulation, and clinical implications. Neurobiol. Dis. 16, 1–13. doi: 10.1016/j.nbd.2003.12.016
Bao, D. Q., Lapergue, B., Tran-Dinh, A., Diallo, D., Moreno, J. A., Mazighi, M., et al. (2013). High-density lipoproteins limit neutrophil-induced damage to the blood-brain barrier in vitro. J. Cereb. Blood Flow Metab. 33, 575–582. doi: 10.1038/jcbfm.2012.206
Barter, P. J., Baker, P. W., and Rye, K. A. (2002). Effect of high-density lipoproteins on the expression of adhesion molecules in endothelial cells. Curr. Opin. Lipidol. 13, 285–288. doi: 10.1097/00041433-200206000-00008
Barter, P. J., Gotto, A. M., LaRosa, J. C., Maroni, J., Szarek, M., Grundy, S. M., et al. (2007). HDL cholesterol, very low levels of LDL cholesterol, and cardiovascular events. N. Engl. J. Med. 357, 1301–1310. doi: 10.1056/NEJMoa064278
Brulhart-Meynet, M. C., Braunersreuther, V., Brinck, J., Montecucco, F., Prost, J. C., Thomas, A., et al. (2015). Improving reconstituted HDL composition for efficient post-ischemic reduction of ischemia reperfusion injury. PLoS ONE 10:e0119664. doi: 10.1371/journal.pone.0119664
Calabresi, L., Gomaraschi, M., and Franceschini, G. (2003a). Endothelial protection by high-density lipoproteins: from bench to bedside. Arterioscler. Thromb. Vasc. Biol. 23, 1724–1731. doi: 10.1161/01.ATV.0000094961.74697.54
Calabresi, L., Rossoni, G., Gomaraschi, M., Sisto, F., Berti, F., and Franceschini, G. (2003b). High-density lipoproteins protect isolated rat hearts from ischemia-reperfusion injury by reducing cardiac tumor necrosis factor-alpha content and enhancing prostaglandin release. Circ. Res. 92, 330–337. doi: 10.1161/01.RES.0000054201.60308.1A
Calabresi, L., Gomaraschi, M., Rossoni, G., and Franceschini, G. (2006). Synthetic high density lipoproteins for the treatment of myocardial ischemia/reperfusion injury. Pharmacol. Ther. 111, 836–854. doi: 10.1016/j.pharmthera.2006.01.003
Carden, D. L., and Granger, D. N. (2000). Pathophysiology of ischaemia-reperfusion injury. J. Pathol. 190, 255–266. doi: 10.1002/(SICI)1096-9896(200002)190:3<255::AID-PATH526>3.0.CO;2-6
Cockerill, G. W., Huehns, T. Y., Weerasinghe, A., Stocker, C., Lerch, P. G., Miller, N. E., et al. (2001a). Elevation of plasma high-density lipoprotein concentration reduces interleukin-1-induced expression of E-selectin in an in vivo model of acute inflammation. Circulation 103, 108–112. doi: 10.1161/01.CIR.103.1.108
Cockerill, G. W., McDonald, M. C., Mota-Filipe, H., Cuzzocrea, S., Miller, N. E., and Thiemermann, C. (2001b). High density lipoproteins reduce organ injury and organ dysfunction in a rat model of hemorrhagic shock. FASEB J. 15, 1941–1952. doi: 10.1096/fj.01-0075com
Cuzzocrea, S., Dugo, L., Patel, N. S., Di Paola, R., Cockerill, G. W., Genovese, T., et al. (2004). High-density lipoproteins reduce the intestinal damage associated with ischemia/reperfusion and colitis. Shock 21, 342–351. doi: 10.1097/00024382-200404000-00009
Dadabayev, A. R., Yin, G., Latchoumycandane, C., McIntyre, T. M., Lesnefsky, E. J., and Penn, M. S. (2014). Apolipoprotein A1 regulates coenzyme Q10 absorption, mitochondrial function, and infarct size in a mouse model of myocardial infarction. J. Nutr. 144, 1030–1036. doi: 10.3945/jn.113.184291
Frangogiannis, N. G., Lindsey, M. L., Michael, L. H., Youker, K. A., Bressler, R. B., Mendoza, L. H., et al. (1998). Resident cardiac mast cells degranulate and release preformed TNF-alpha, initiating the cytokine cascade in experimental canine myocardial ischemia/reperfusion. Circulation 98, 699–710. doi: 10.1161/01.CIR.98.7.699
Frias, M. A., Pedretti, S., Hacking, D., Somers, S., Lacerda, L., Opie, L. H., et al. (2013). HDL protects against ischemia reperfusion injury by preserving mitochondrial integrity. Atherosclerosis 228, 110–116. doi: 10.1016/j.atherosclerosis.2013.02.003
Ghazzal, Z. B., Dhawan, S. S., Sheikh, A., Douglas, J. S., Veledar, E., Mavromatis, K., et al. (2009). Usefulness of serum high-density lipoprotein cholesterol level as an independent predictor of one-year mortality after percutaneous coronary interventions. Am. J. Cardiol. 103, 902–906. doi: 10.1016/j.amjcard.2008.11.053
Gidday, J. M., Gasche, Y. G., Copin, J. C., Shah, A. R., Perez, R. S., Shapiro, S. D., et al. (2005). Leukocyte-derived matrix metalloproteinase-9 mediates blood-brain barrier breakdown and is proinflammatory after transient focal cerebral ischemia. Am. J. Physiol. Heart Circ. Physiol. 289, H558–H568. doi: 10.1152/ajpheart.01275.2004
Gomaraschi, M., Calabresi, L., Rossoni, G., Iametti, S., Franceschini, G., Stonik, J. A., et al. (2008). Anti-inflammatory and cardioprotective activities of synthetic high-density lipoprotein containing apolipoprotein A-I mimetic peptides. J. Pharmacol. Exp. Ther. 324, 776–783. doi: 10.1124/jpet.107.129411
Hearse, D. J., and Sutherland, F. J. (2000). Experimental models for the study of cardiovascular function and disease. Pharmacol. Res. 41, 597–603. doi: 10.1006/phrs.1999.0651
Hibert, P., Prunier-Mirebeau, D., Beseme, O., Chwastyniak, M., Tamareille, S., Lamon, D., et al. (2013). Apolipoprotein A-I is a potential mediator of remote ischemic preconditioning. PLoS ONE 8:e77211. doi: 10.1371/journal.pone.0077211
Ibanez, B., Heusch, G., Ovize, M., and Van de, W. F. (2015). Evolving therapies for myocardial ischemia/reperfusion injury. J. Am. Coll. Cardiol. 65, 1454–1471. doi: 10.1016/j.jacc.2015.02.032
Imaizumi, S., Miura, S., Nakamura, K., Kiya, Y., Uehara, Y., Zhang, B., et al. (2008). Antiarrhythmogenic effect of reconstituted high-density lipoprotein against ischemia/reperfusion in rats. J. Am. Coll. Cardiol. 51, 1604–1612. doi: 10.1016/j.jacc.2007.12.040
Kempen, H. J., van Gent, C. M., Buytenhek, R., and Buis, B. (1987). Association of cholesterol concentrations in low-density lipoprotein, high-density lipoprotein, and high-density lipoprotein subfractions, and of apolipoproteins AI and AII, with coronary stenosis and left ventricular function. J. Lab. Clin. Med. 109, 19–26.
Koren-Morag, N., Tanne, D., Graff, E., and Goldbourt, U. (2002). Low– and high-density lipoprotein cholesterol and ischemic cerebrovascular disease: the bezafibrate infarction prevention registry. Arch. Intern. Med. 162, 993–999. doi: 10.1001/archinte.162.9.993
Krause, B. R., and Remaley, A. T. (2013). Reconstituted HDL for the acute treatment of acute coronary syndrome. Curr. Opin. Lipidol. 24, 480–486. doi: 10.1097/MOL.0000000000000020
Kupatt, C., Habazettl, H., Goedecke, A., Wolf, D. A., Zahler, S., Boekstegers, P., et al. (1999). Tumor necrosis factor-alpha contributes to ischemia– and reperfusion-induced endothelial activation in isolated hearts. Circ. Res. 84, 392–400. doi: 10.1161/01.RES.84.4.392
Lapergue, B., Dang, B. Q., Desilles, J. P., Ortiz-Munoz, G., Delbosc, S., Loyau, S., et al. (2013). High-density lipoprotein-based therapy reduces the hemorrhagic complications associated with tissue plasminogen activator treatment in experimental stroke. Stroke 44, 699–707. doi: 10.1161/STROKEAHA.112.667832
Lapergue, B., Moreno, J. A., Dang, B. Q., Coutard, M., Delbosc, S., Raphaeli, G., et al. (2010). Protective effect of high-density lipoprotein-based therapy in a model of embolic stroke. Stroke 41, 1536–1542. doi: 10.1161/STROKEAHA.110.581512
Marchesi, M., Booth, E. A., Davis, T., Bisgaier, C. L., and Lucchesi, B. R. (2004). Apolipoprotein A-IMilano and 1-palmitoyl-2-oleoyl phosphatidylcholine complex (ETC-216) protects the in vivo rabbit heart from regional ischemia-reperfusion injury. J. Pharmacol. Exp. Ther. 311, 1023–1031. doi: 10.1124/jpet.104.070789
Marchesi, M., Booth, E. A., Rossoni, G., Garcia, R. A., Hill, K. R., Sirtori, C. R., et al. (2008). Apolipoprotein A-IMilano/POPC complex attenuates post-ischemic ventricular dysfunction in the isolated rabbit heart. Atherosclerosis 197, 572–578. doi: 10.1016/j.atherosclerosis.2007.08.028
Nissen, S. E., Tsunoda, T., Tuzcu, E. M., Schoenhagen, P., Cooper, C. J., Yasin, M., et al. (2003). Effect of recombinant ApoA-I Milano on coronary atherosclerosis in patients with acute coronary syndromes: a randomized controlled trial. JAMA 290, 2292–2300. doi: 10.1001/jama.290.17.2292
Olsson, A. G., Schwartz, G. G., Szarek, M., Sasiela, W. J., Ezekowitz, M. D., Ganz, P., et al. (2005). High-density lipoprotein, but not low-density lipoprotein cholesterol levels influence short-term prognosis after acute coronary syndrome: results from the MIRACL trial. Eur. Heart J. 26, 890–896. doi: 10.1093/eurheartj/ehi186
Paterno, R., Ruocco, A., Postiglione, A., Hubsch, A., Andresen, I., and Lang, M. G. (2004). Reconstituted high-density lipoprotein exhibits neuroprotection in two rat models of stroke. Cerebrovasc. Dis. 17, 204–211. doi: 10.1159/000075792
Prosser, H. C., Tan, J. T., Dunn, L. L., Patel, S., Vanags, L. Z., Bao, S., et al. (2014). Multifunctional regulation of angiogenesis by high-density lipoproteins. Cardiovasc. Res. 101, 145–154. doi: 10.1093/cvr/cvt234
Rossoni, G., Gomaraschi, M., Berti, F., Sirtori, C. R., Franceschini, G., and Calabresi, L. (2004). Synthetic high-density lipoproteins exert cardioprotective effects in myocardial ischemia/reperfusion injury. J. Pharmacol. Exp. Ther. 308, 79–84. doi: 10.1124/jpet.103.057141
Soyama, Y., Miura, K., Morikawa, Y., Nishijo, M., Nakanishi, Y., Naruse, Y., et al. (2003). High-density lipoprotein cholesterol and risk of stroke in Japanese men and women: the Oyabe Study. Stroke 34, 863–868. doi: 10.1161/01.STR.0000060869.34009.38
Sumi, M., Sata, M., Miura, S., Rye, K. A., Toya, N., Kanaoka, Y., et al. (2007). Reconstituted high-density lipoprotein stimulates differentiation of endothelial progenitor cells and enhances ischemia-induced angiogenesis. Arterioscler. Thromb. Vasc. Biol. 27, 813–818. doi: 10.1161/01.ATV.0000259299.38843.64
Tan, J. T., Prosser, H. C., Vanags, L. Z., Monger, S. A., Ng, M. K., and Bursill, C. A. (2014). High-density lipoproteins augment hypoxia-induced angiogenesis via regulation of post-translational modulation of hypoxia-inducible factor 1alpha. FASEB J. 28, 206–217. doi: 10.1096/fj.13-233874
Tao, R., Hoover, H. E., Honbo, N., Kalinowski, M., Alano, C. C., Karliner, J. S., et al. (2010). High-density lipoprotein determines adult mouse cardiomyocyte fate after hypoxia-reoxygenation through lipoprotein-associated sphingosine 1-phosphate. Am. J. Physiol. Heart Circ. Physiol. 298, H1022–H1028. doi: 10.1152/ajpheart.00902.2009
Tardif, J. C., Gregoire, J., L’Allier, P. L., Ibrahim, R., Lesperance, J., Heinonen, T. M., et al. (2007). Effects of reconstituted high-density lipoprotein infusions on coronary atherosclerosis: a randomized controlled trial. JAMA 297, 1675–1682. doi: 10.1001/jama.297.15.jpc70004
Theilmeier, G., Schmidt, C., Herrmann, J., Keul, P., Schafers, M., Herrgott, I., et al. (2006). High-density lipoproteins and their constituent, sphingosine-1-phosphate, directly protect the heart against ischemia/reperfusion injury in vivo via the s1p3 lysophospholipid receptor. Circulation 114, 1403–1409. doi: 10.1161/CIRCULATIONAHA.105.607135
Thiemermann, C., Patel, N. S., Kvale, E. O., Cockerill, G. W., Brown, P. A., Stewart, K. N., et al. (2003). High density lipoprotein (HDL) reduces renal ischemia/reperfusion injury. J. Am. Soc. Nephrol. 14, 1833–1843. doi: 10.1097/01.ASN.0000075552.97794.8C
Wang, T. D., Lee, C. M., Wu, C. C., Lee, T. M., Chen, W. J., Chen, M. F., et al. (1999). The effects of dyslipidemia on left ventricular systolic function in patients with stable angina pectoris. Atherosclerosis 146, 117–124. doi: 10.1016/S0021-9150(99)00108-2
Wang, T. D., Wu, C. C., Chen, W. J., Lee, C. M., Chen, M. F., Liau, C. S., et al. (1998). Dyslipidemias have a detrimental effect on left ventricular systolic function in patients with a first acute myocardial infarction. Am. J. Cardiol. 81, 531–537. doi: 10.1016/S0002-9149(97)00974-0
Keywords: high density lipoproteins, HDL cholesterol, synthetic HDL, ischemia–reperfusion injury, acute coronary syndrome
Citation: Gomaraschi M, Calabresi L and Franceschini G (2016) Protective Effects of HDL Against Ischemia/Reperfusion Injury. Front. Pharmacol. 7:2. doi: 10.3389/fphar.2016.00002
Received: 21 September 2015; Accepted: 08 January 2016;
Published: 25 January 2016.
Edited by:
Norman Eric Miller, University of Oxford, UKReviewed by:
Alexandre Chigaev, University of New Mexico, USAHaroon Khan, Abdul Wali Khan University Mardan, Pakistan
Antonio Colantuoni, University of Naples Federico II, Italy
Copyright © 2016 Gomaraschi, Calabresi and Franceschini. This is an open-access article distributed under the terms of the Creative Commons Attribution License (CC BY). The use, distribution or reproduction in other forums is permitted, provided the original author(s) or licensor are credited and that the original publication in this journal is cited, in accordance with accepted academic practice. No use, distribution or reproduction is permitted which does not comply with these terms.
*Correspondence: Guido Franceschini, Z3VpZG8uZnJhbmNlc2NoaW5pQHVuaW1pLml0