- 1Laboratoire de Biologie Tissulaire et Ingénierie Thérapeutique, UMR 5305, CNRS, Institut de Biologie et Chimie des Protéines, SFR BioSciences Gerland-Lyon Sud, Université Lyon 1, Lyon, France
- 2Bases Moléculaires et Structurales des Systèmes Infectieux UMR 5086, CNRS, Institut de Biologie et Chimie des Protéines, SFR BioSciences Gerland-Lyon Sud, Université Lyon 1, Lyon, France
Syndecans are transmembrane receptors with ectodomains that are modified by glycosaminoglycan chains. The ectodomains can interact with a wide variety of molecules, including growth factors, cytokines, proteinases, adhesion receptors, and extracellular matrix (ECM) components. The four syndecans in mammals are expressed in a development-, cell-type-, and tissue-specific manner and can function either as co-receptors with other cell surface receptors or as independent adhesion receptors that mediate cell signaling. They help regulate cell proliferation and migration, angiogenesis, cell/cell and cell/ECM adhesion, and they may participate in several key tumorigenesis processes. In some cancers, syndecan expression regulates tumor cell proliferation, adhesion, motility, and other functions, and may be a prognostic marker for tumor progression and patient survival. The short cytoplasmic tail is likely to be involved in these events through recruitment of signaling partners. In particular, the conserved carboxyl-terminal EFYA tetrapeptide sequence that is present in all syndecans binds to some PDZ domain-containing proteins that may function as scaffold proteins that recruit signaling and cytoskeletal proteins to the plasma membrane. There is growing interest in understanding these interactions at both the structural and biological levels, and recent findings show their high degree of complexity. Parameters that influence the recruitment of PDZ domain proteins by syndecans, such as binding specificity and affinity, are the focus of active investigations and are important for understanding regulatory mechanisms. Recent studies show that binding may be affected by post-translational events that influence regulatory mechanisms, such as phosphorylation within the syndecan cytoplasmic tail.
Introduction
Syndecans are transmembrane proteoglycans that are found on the surface of many types of mammalian cells. The four syndecans in mammals are encoded by four genes, but invertebrates have just one syndecan. Based on chromosomal localization and exon organization studies, all syndecans arise from a single ancestral gene. Syndecans are expressed in a development-, cell-type-, and tissue-specific manner and function either as independent or co-receptors that mediate cell signaling (Bishop et al., 2007; Multhaupt et al., 2009). In these type I transmembrane glycoproteins, the core protein ranges in size from 20 to 45 kDa. Syndecan core proteins include an extracellular domain (ectodomain) that carries either heparan sulfate only or heparan sulfate and chondroitin sulfate a single transmembrane (TM) domain; and a short cytoplasmic domain (Figure 1A). The ectodomain can interact with a wide variety of molecules, including growth factors, cytokines, proteinases, adhesion receptors, and ECM components. Syndecan-1 is mainly expressed in mesenchymal and epithelial cells. Syndecan-2 is highly expressed in endothelial and mesenchymal tissues and in liver, neural, and fibroblast cells. Syndecan-3, the longest of the four syndecans, is expressed in neural tissue and developing musculoskeletal system, but is undetectable in epithelial cells. Finally, syndecan-4, which has the shortest core protein, is widely expressed.
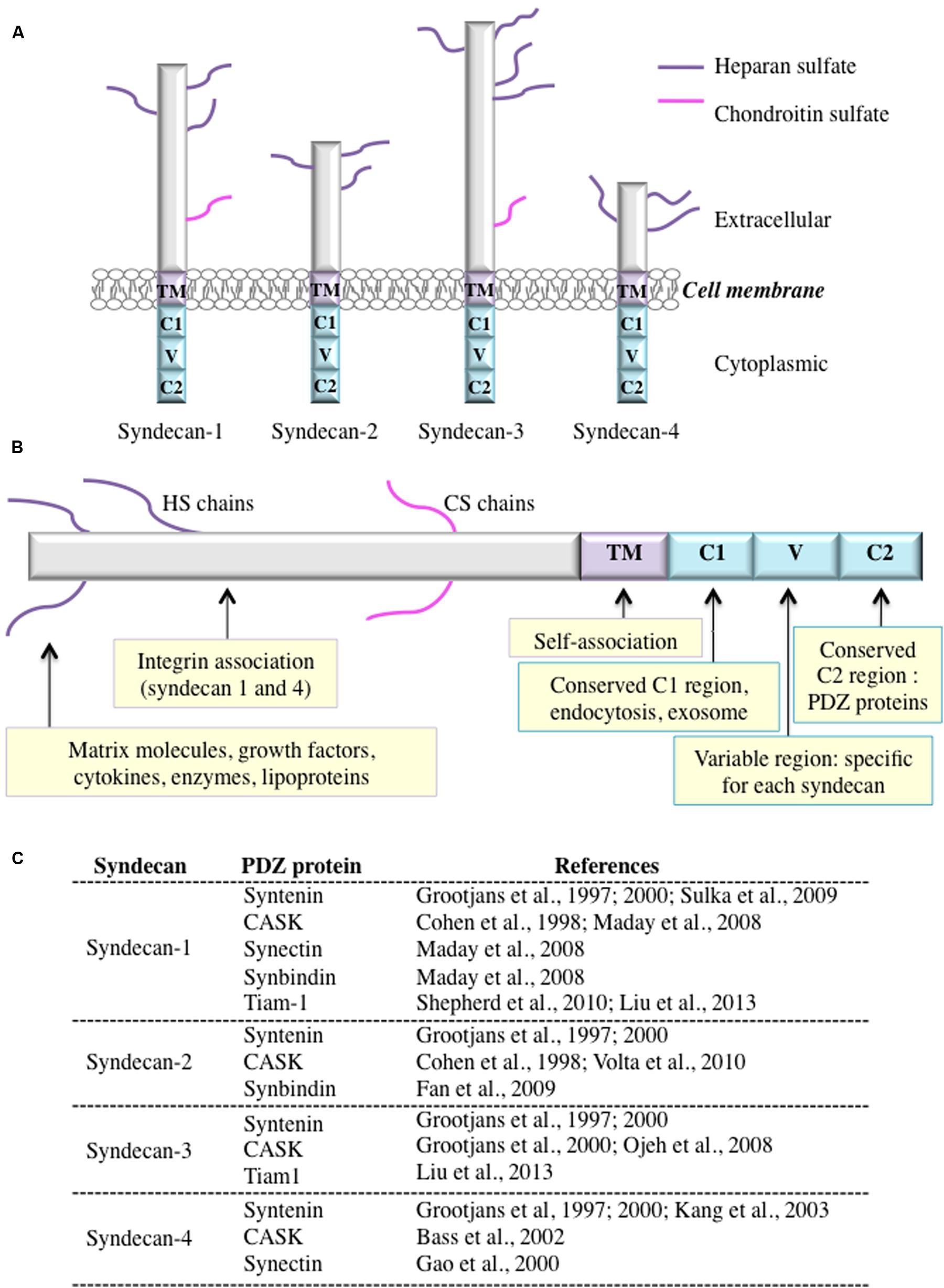
FIGURE 1. (A) The syndecan family of heparan sulfate proteoglycans. Syndecans consist of a transmembrane core protein with a short cytoplasmic tail and an ectodomain. All syndecan ectodomains are modified with either heparan sulfate only or heparan sulfate and chondroitin sulfate sugar chains. (B) A schematic showing the syndecan domains and examples of functions and interacting partners. (C) List of known PDZ-containing proteins that interact with syndecans.
Syndecans are implicated in the control of cell–cell, cell–pathogen, and cell–matrix interactions via the recruitment of the actin cytoskeleton, as well as in cellular proliferation, differentiation, and migration. Syndecans can be found in cell protrusions and focal adhesions, where they colocalize with actin (Granés et al., 1999; Berndt et al., 2004). Importantly, they can act as co-receptors of other cell surface receptors like growth factor receptors and integrins (Morgan et al., 2007; Couchman, 2010; Rapraeger, 2013). In this context, syndecans can bind, immobilize, concentrate, and induce conformational changes in growth factors, adhesion molecules, and other signaling molecules via their heparan sulfate chains, thus facilitating their receptor interaction. They can also protect ligands from activation or sequester them away from membrane receptors (Zimmermann and David, 1999; Alexopoulou et al., 2007).
Syndecans undergo regulated physiological shedding of their extracellular domain, a process that may be increased in pathological conditions, thereby allowing them to act as soluble effectors and/or antagonists (Kim et al., 1994; Manon-Jensen et al., 2010). In addition, syntenins/syndecans, in conjunction with the syntenin-binding protein ALIX, are likely to be involved in or to enhance exosome production (Baietti et al., 2012; Friand et al., 2015).
Syndecans and Cancer
Syndecans are involved in cancers, infectious diseases, obesity, wound healing, and angiogenesis. As documented in recent reviews, they are considered key regulators of tumor progression (Barbouri et al., 2014; Couchman et al., 2015; Theocharis et al., 2015). In some cancers, syndecan expression may regulate tumor cell function and serve as a prognostic marker for tumor progression and patient survival. Syndecan-1 expression is dysregulated in a number of cancers, including head and neck, ovarian, breast, and colorectal carcinomas (Teng et al., 2012). Syndecan-1 acts as a tumor suppressor in MDA-MB-231 breast cancer cells (Hassan et al., 2013). Treating these cells with syndecan-1 small interfering RNA not only enhances β1-integrin and focal adhesion kinase activity, leading to increased cellular adhesion and migration, but it also improves cellular resistance to irradiation. A study on pre-invasive breast cancer revealed an inverse correlation between the expression of syndecan-1 and the pro-metastatic microRNA miR-10b, suggesting a potential novel mode of post-transcriptional regulation of syndecan-1 (Hannafon et al., 2011). Studies revealing the negative regulation of syndecan-1 by miR-10b and its pro-invasive consequences in human breast cancer cells, reported syndecan-1 as a new regulatory target of miR-10b (Ibrahim et al., 2012). Other studies revealed that syndecan-1 decreases cell migration in lung epithelium via activation of Rap1, which slows focal adhesion disassembly (Altemeier et al., 2012). Syndecan-1 also plays a role in squamous cell carcinoma collagen-mediated motility and invasion by modulating RhoA and Rac activity, suggesting that decreased syndecan-1 expression during carcinoma progression may enhance tumor cell invasiveness (Ishikawa and Kramer, 2010).
The presence of syndecan-1 is associated with favorable outcomes in both lung cancer and mesothelioma (Kumar-Singh et al., 1998; Anttonen et al., 2001), and the loss of syndecan-1 is a feature of hepatocellular carcinoma with high metastatic potential (Matsumoto et al., 1997). Low syndecan-1 expression correlates with gastric carcinoma invasion and metastasis (Chu et al., 2008). In contrast, studies have reported that high expression levels of syndecan-1 in breast carcinoma are associated with high histological grade, high mitotic count, large tumor size, c-erbB-2 over-expression, and estrogen receptor-negative status. These studies show that high syndecan-1 expression correlates with the most invasive breast carcinomas (Stanley et al., 1999; Barbareschi et al., 2003; Leivonen et al., 2004; Lendorf et al., 2011). Studies using an in vitro breast cancer model also suggest that syndecan-1 participates directly in tumor cell spreading and adhesion (Beauvais and Rapraeger, 2003). In prostate cancer, high syndecan-1 expression is a feature of biologically aggressive progression (Zellweger et al., 2003). As stated in recent comprehensive reviews, stromal expression of syndecan-1 may have negative prognostic value, and elevated serum levels of the shed syndecan ectodomain might also be a prognostic indicator (Gharbaran, 2015; Szatmári et al., 2015). Studies have revealed a mechanism by which syndecan-1 and -4 ectodomains, may capture and induce autophosphorylation of the tyrosine kinase receptors HER2 and EGFR respectively, leading to integrin mediated carcinoma cell migration (Wang et al., 2014, 2015).
Nuclear localization of syndecan-1 has been reported, suggesting that it may function as a transcription factor and therefore impact gene regulation affecting cancer pathogenesis (Brockstedt et al., 2002). In addition, heparanase and syndecan-1 may cooperate to drive growth factor signaling and to regulate cell behavior, thus enhancing tumor growth and dissemination (Ramani et al., 2013; Palaiologou et al., 2014; Roucourt et al., 2015). One study found that syndecan-4 inhibited breast carcinoma cell invasion (Beauvais and Rapraeger, 2003), and its expression in human breast carcinoma was described as being associated with good prognosis (Lendorf et al., 2011). In contrast, another study found that syndecan-4 expression correlated significantly with high histological grade and negative estrogen receptor status (Baba et al., 2006) and was therefore a marker of poorer prognosis. Furthermore, a study of pancreatic cancer showed that syndecan-2 was involved in perineural invasion of pancreatic adenocarcinoma cells (De Oliveira et al., 2012). Silencing syndecan-2 expression in these cells significantly reduced motility and invasiveness. Syndecan-2 is upregulated in breast tumors (Lim et al., 2015) and in colon carcinomas (Park et al., 2002; Ryu et al., 2009; Choi et al., 2010). In highly metastatic colorectal cancer cells, syndecan-2 expression is enhanced by fibronectin secreted by stromal cells (Vicente et al., 2013). In colorectal carcinoma, low epithelial expression of syndecan-1 is associated with a higher histological grade, with more advanced clinical stage of the patients, and with potentially more unfavorable prognosis (Lundin et al., 2005; Hashimoto et al., 2008; Mitselou et al., 2012). Results from a recent meta-analysis of colorectal cancer studies demonstrated that loss of syndecan-1 expression in colorectal cancer correlates with histological grade and tumor stage, but not with lymph node or distant metastasis (Wei et al., 2015). The authors also reported that syndecan-1 expression does not have prognostic value in colorectal carcinoma patients. To date, syndecan-3 has not been implicated in cancer. Although the mechanisms are not yet fully understood, these examples highlight the important role of syndecans in tumor progression and suggest that they are relevant and promising therapeutic targets (Ramani et al., 2013; Barbouri et al., 2014; Theocharis et al., 2015). For instance, the anti-tumoral activity of zoledronic acid on breast cancer cells was reported to correlate with a differential modulation of syndecans (Dedes et al., 2012). Synstatin peptides based on HER2 and EGFR interaction motifs on syndecan-1 and -4 respectively can competitively displace receptor tyrosine kinase interaction and disrupt activation of cell motility (Wang et al., 2015). Similar peptides were designed to block IGF1R binding to syndecan-1/αvβ3 integrin complex and inhibit the integrin activity in endothelial and tumor cells (Rapraeger, 2013).
The Transmembrane Domain-Induced Oligomerization Properties of Syndecans
Syndecans transmembrane domain is composed of 25 hydrophobic amino acid residues responsible for the molecular interaction that causes homo-oligomerization of syndecan core proteins (Asundi and Carey, 1995; Choi et al., 2005), a step essential for their signaling activation. The conserved GXXXG (where X is any amino acid) motif is involved in this process. Recent studies have revealed the potential of syndecan-2 and -4 to form hetero-oligomers, reducing each syndecan activity (Choi et al., 2015). This hetero-oligomerization capacity may offer insight into an underlying modulating mechanism (Kwon et al., 2015).
The cytoplasmic tail has two conserved regions, C1 and C2, that share common characteristics in all syndecans, plus a central variable region (V) that may regulate cell spreading and actin cytoskeleton assembly (Figure 1B; Carey et al., 1996; Chakravarti et al., 2005; Stepp et al., 2015). Each region can support signaling complexes formation (Carey, 1997; Bernfield et al., 1999; Yoneda and Couchman, 2003). The C1 domain is thought to participate in syndecan dimerization (Oh et al., 1997) and in the binding of various intracellular proteins, such as ezrin (Granés et al., 2000). In neuroblastoma, the C1 region of syndecan-3 interacts with a protein complex composed of Src family kinases and the actin-binding proteins cortactin and tubulin (Kinnunen et al., 1998). Likewise, the V region of syndecan-4 interacts with PKCα (protein kinase Cα) as well as with phosphatidylinositol 4,5-bisphosphate (PtdIns-4,5-P2) (Oh et al., 1997, 1998). The C2 carboxyl-terminal tetrapeptide sequence present in all syndecans consists of the highly conserved tetrapeptide sequence Glu-Phe-Tyr-Ala (EFYA) (Bass and Humphries, 2002; Multhaupt et al., 2009; Rousselle and Letourneur, 2009).
The Interactions of Syndecans with Cytoskeleton PDZ Domain Proteins
The EFYA sequence binds to PDZ domain-containing proteins, such as syntenin-1 (Grootjans et al., 1997) and CASK (Cohen et al., 1998), which may function as membrane scaffold proteins that recruit signaling and cytoskeletal proteins to the plasma membrane. The EFYA motif thus belongs to the large family of PDZ-binding motifs (PDZ-BMs). Recent work suggests that PDZ interactions are involved in protein trafficking, possibly routing proteoglycans to the cell surface (Wawrzyniak et al., 2012).
There is growing interest in understanding the binding of syndecans to their PDZ domain-containing counterparts (Figure 1C). Not only are the interactions involved in cytoskeletal rearrangements in response to the signaling activities, but syndecan-PDZ domain-containing protein complexes may also participate in cell-ECM adhesion and migration. For example, synectin binding to syndecan-4 may modulate in vitro cell migration (Gao et al., 2000; Tkachenko et al., 2006). As well, cell adhesion to fibronectin is regulated by the interaction of syndecan-1 with the PDZ domain of the T-cell lymphoma invasion and metastasis gene 1 protein (Tiam1) (Shepherd et al., 2010). A study of hippocampal neurons revealed that syndecan-2 induces spine formation by recruiting intracellular vesicles toward postsynaptic sites through an interaction with synbindin (Ethell et al., 2000).
The name PDZ is an acronym derived from the first three proteins in which these domains were identified: PSD-95 (postsynaptic density PSD-95/SAP90), DLG (Drosophila melanogaster tumor suppressor septate junction protein Disks large-1), and ZO-1 (epithelial tight junction protein Zonula Occludens-1) (Kennedy, 1995; Zimmermann, 2006; Ye and Zhang, 2013). Over 250 non-redundant PDZ domains have been identified in the human proteome (Wang et al., 2010) and are found in proteins involved in diverse cellular functions, such as maintenance of cell polarity, signal transduction in neurons, and cell migration (Harris and Lim, 2001; Sheng and Sala, 2001; Jeleñ et al., 2003).
The number of amino acid residues in a PDZ domain is relatively small (80–100 amino acids) (Hung and Sheng, 2002). Structural analysis of these domains indicates that a canonical PDZ domain consists of five or six β-strands and two or three α-helices (Luck et al., 2012) (Figure 2A). In addition, the domain itself folds into a compact globular shape; this maintains the proximity of the N- and C-termini to each other on opposite sides of the PDZ-BM interaction site (Sheng and Sala, 2001; Jeleñ et al., 2003; Lee and Zheng, 2010). The PDZ-BM fits in the groove between the α2-helix and the β2-strand structure such that the α2-helix is anti-parallel to the β2-strand (Figure 2B). This interaction site is also known as the carboxylate-binding site because of the highly conserved carboxylate-binding loop at the end of the groove that connects the β1- and β2-strand structures: R/K-X-X-X-G-Φ-G-Φ (where Φ is a hydrophobic residue) (Sheng and Sala, 2001; Hung and Sheng, 2002; Lee and Zheng, 2010).
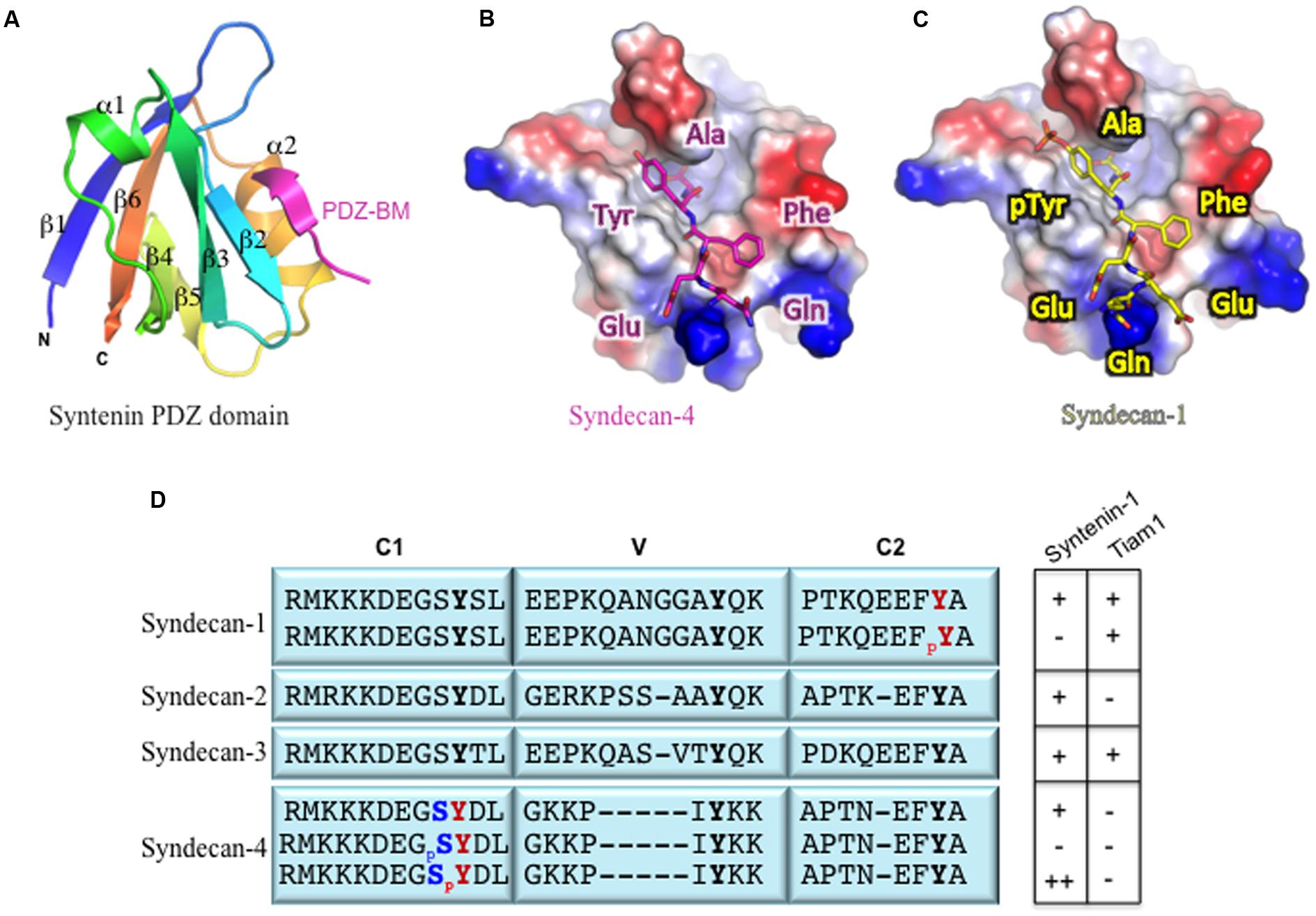
FIGURE 2. (A) Structural definition of syndecan binding to the PDZ domain. Ribbon diagram of the syntenin PDZ2 bound to the syndecan-4 peptide TNEFYA (pdb code 1 OBY, Kang et al., 2003), (B) Surface charge (blue positive and red negative) representation of the PDZ2 domain with syndecan-4 (magenta) peptide displayed as ball-and-stick. (C) Similar representation of PDZ2 with a model of syndecan-1 tyrosine phosphorylated peptide (yellow) showed as ball-and-stick. (D) Phosphorylation of Tyr and Ser residues within syndecan cytoplasmic tails and their effects on PDZ protein binding: (-) no interaction; (+) interaction; (++) enhanced interaction. The conserved (C1 and C2) and variable (V) domain organization is from Couchman et al. (2015).
There are no reports of PDZ domains interacting with syndecans through motifs other than the EFYA sequence. Since the EFYA motif is the only PDZ-BM in syndecans, it seems likely that all four syndecans have similar binding affinity for the same PDZ-containing proteins. For example, all syndecans have similar affinity for the PDZ1-PDZ2 tandem domain of syntenin-1 (Grootjans et al., 2000). However, CASK has a higher affinity for syndecan-2 and syndecan-4 than for syndecan-1 and syndecan-3. The molecular mechanism underlying this difference in affinities is unknown. On the other hand, the PDZ domain of Tiam1 binds to a peptide corresponding to the last eight residues of syndecan-1 and -3, but not to those of syndecan-2 and -4 (Liu et al., 2013).
PDZ domain-containing proteins play essential roles in most aspects of cellular homoeostasis and are implicated in diverse aspects of tumor development and metastasis (Subbaiah et al., 2011). A number of studies have established that MDA-9/syntenin has a pivotal role in cancer development and progression, and suggest that it could be a tumor marker (Philley et al., 2016). Recent data indicate that in addition to its involvement in the migration and growth of tumor cells, syntenin appears to be involved in controlling the plasma membrane localization of active β1-integrin (Kashyap et al., 2015). A recent study showed that co-upregulation of CASK and syndecan-2 in colorectal cancer is associated with an unfavorable prognosis (Wei et al., 2014), suggesting that CASK could be a prognostic factor for colorectal cancer metastasis. Synbindin was shown to contribute to the aggressiveness of gastric cancer by activating the ERK signaling pathway (Kong et al., 2013), while synectin was shown to participate in pancreatic cancer growth (Muders et al., 2006). As a guanine exchange factor for Rac1, tiam1 involvement in cancer biology may be linked to its pivotal function in cytoskeletal dynamics (Vigil et al., 2010). One study reported that syndecan-2 regulates colon carcinoma cell migration through Tiam1-dependent Rac activation (Choi et al., 2010).
Regulation of PDZ Binding by Phosphorylation of the Syndecan Cytoplasmic Tail
The phosphorylation of Ser, Thr, or Tyr residues in the syndecan cytoplasmic tail appears to be a key mechanism that regulates its interactions with PDZ domains (Figure 2B). We reported that the formation of membrane protrusions in cells plated on immobilized laminin α3 chain LG45 domain required the dephosphorylation of tyrosine residues in the cytoplasmic tail of syndecan-1 (Sulka et al., 2009; Rousselle and Beck, 2013). Further experiments demonstrated that phosphorylation of the Tyr residue in its EFYA sequence abolished its interaction with syntenin-1 (Figure 2C, Sulka et al., 2009). In contrast, phosphorylation of this Tyr residue did not affect the binding of the PDZ domain of Tiam1 (Figure 2D, Shepherd et al., 2010; Liu et al., 2013). It is not known whether this holds true for phosphorylated syndecan-3 as well. Based on the examination of other syndecan-binding PDZ domains, the PDZ domains of CASK and synectin are predicted to interact with Tyr-phosphorylated syndecan-1 in a manner similar to that seen in the Tiam1 PDZ-phosphorylated syndecan-1 complex. This mechanism may support syndecan signaling specificity (Liu et al., 2013).
Other regulatory mechanisms involving the phosphorylation of a Ser residue in the carboxyl terminus of PDZ-binding proteins may either disrupts or enhances interactions with PDZ domains (Figure 2D, Cohen et al., 1996; Matsuda et al., 1999; Hegedüs et al., 2003). Studies of syndecan-4 revealed that phosphorylation of the Ser residue in the C1 region induces a conformational change in the C2 domain, even though the phosphorylation site is 20 residues away and impedes the PDZ binding ability of syntenin-1 (Horowitz and Simons, 1998; Koo et al., 2006). Furthermore, phosphorylation of the Tyr residue of the syndecan-4 C1 region was shown to enhance syntenin-1 binding and to function as a molecular switch to regulate specific integrin recycling and coordinate focal adhesion dynamics (Morgan et al., 2013).
These findings reinforce the importance of residues upstream of the EFYA motif in the regulation of PDZ domain interactions with syndecans. To date, there are no reports of the phosphorylation of Thr residues in terms of regulation of syndecan binding to PDZ domains. The phosphorylation of Tyr versus Ser residues depends upon which enzymes are involved. For example, Src family kinases and Elk kinases are widely reported to be the enzymes responsible for the phosphorylation of the Tyr residues (Asundi and Carey, 1997; Morgan et al., 2013). In contrast, PKCs are the only enzymes that have been reported to be involved in Ser residue phosphorylation (Prasthofer et al., 1995; Oh et al., 1997; Koo et al., 2006). Moreover, PKC can only recognize the Ser residue in syndecan-2 and syndecan-3, but not those in syndecan-1 and syndecan-4 (Prasthofer et al., 1995). Similarly, endogenously phosphorylated Tyr residues were only found on syndecan-1 and sydecan-4 in B82 fibroblasts, although this cell line also expresses syndecan-2 (Ott and Rapraeger, 1998).
Since phosphorylation is a key mechanism in modulating the interactions of syndecans with cytoplasmic proteins, the process is expected to be tightly regulated and some proportion of syndecans in a cell are expected to be in a phosphorylated state. Indeed, studies have found endogenously phosphorylated syndecans in cultured cells (Asundi and Carey, 1997; Ott and Rapraeger, 1998; Bass and Humphries, 2002; Morgan et al., 2013). These results illustrate the high level of complexity underlying the syndecans “turn on and off” signals.
Conclusion
The study of both the structural and biological aspects of the mechanisms underlying PDZ protein binding to syndecans is an exciting field of research. Due to their high level of complexity, the physiological significance of these interactions is not yet fully clarified; however, ongoing and future work will undoubtedly shed light on these important molecular complexes and their roles in cytoplasmic signaling pathways.
Author Contributions
PR and BC wrote the manuscript. MM prepared Figure 1. LT designed structural models presented in Figure 2.
Funding
Work in the authors’ laboratory was supported by the Agence Nationale de la Recherche (ANR-08-PCVI-0031 and ANR-13-RPIB-0003-01) and by the Ligue Nationale Contre le Cancer. BC received a post-doctoral fellowship (ANR-08-PCVI-0031), and MM received a pre-doctoral fellowship (ANR-13-RPIB-0003-01).
Conflict of Interest Statement
The authors declare that the research was conducted in the absence of any commercial or financial relationships that could be construed as a potential conflict of interest.
Abbreviations
ECM, extracellular matrix; GAG, glycosaminoglycan; IGF1R, insulin-like growth factor-1 receptor; PDZ, postsynaptic density-95/disc large protein/zonula occludens-1; PDZ-BM, PDZ binding motif; PKC, protein kinase C.
References
Alexopoulou, A. N., Multhaupt, H. A., and Couchman, J. R. (2007). Syndecans in wound healing, inflammation and vascular biology. Int. J. Biochem. Cell Biol. 39, 505–528. doi: 10.1016/j.biocel.2006.10.014
Altemeier, W. A., Schlesinger, S. Y., Buell, C. A., Parks, W. C., and Chen, P. (2012). Syndecan-1 controls cell migration by activating Rap1 to regulate focal adhesion disassembly. J. Cell Sci. 125, 5188–5195. doi: 10.1242/jcs.109884
Anttonen, A., Heikkilä, P., Kajanti, M., Jalkanen, M., and Joensuu, H. (2001). High syndecan-1 expression is associated with favourable outcome in squamous cell lung carcinoma treated with radical surgery. Lung Cancer 32, 297–305. doi: 10.1016/S0169-5002(00)00230-0
Asundi, V. K., and Carey, D. J. (1995). Self-association of N-syndecan (syndecan-3) core protein is mediated by a novel structural motif in the transmembrane domain and ectodomain flanking region. J. Biol. Chem. 270, 26404–26410. doi: 10.1074/jbc.270.44.26404
Asundi, V. K., and Carey, D. J. (1997). Phosphorylation of recombinant N-Syndecan (Syndecan 3) core protein. Bioch. Biophys. Res. Comm. 240, 502–506. doi: 10.1006/bbrc.1997.7684
Baba, F., Swartz, K., Van Buren, R., Eickhoff, J., Zhang, Y., Wolberg, W., et al. (2006). Syndecan-1 and syndecan-4 are overexpressed in an estrogen receptor-negative, highly proliferative breast carcinoma subtype. Breast Cancer Res. Treat. 98, 91–98. doi: 10.1007/s10549-005-9135-2
Baietti, M. F., Zhang, Z., Mortier, E., Melchior, A., Degeest, G., Geeraerts, A., et al. (2012). Syndecan-syntenin-ALIX regulates the biogenesis of exosomes. Nat. Cell Biol. 14, 677–685. doi: 10.1038/ncb2502
Barbareschi, M., Maisonneuve, P., Aldovini, D., Cangi, M. G., Pecciarini, L., Angelo Mauri, F., et al. (2003). High syndecan-1 expression in breast carcinoma is related to an aggressive phenotype and to poorer prognosis. Cancer 98, 474–483.
Barbouri, D., Afratis, N., Gialeli, C., Vynios, D. H., Theocharis, A. D., and Karamanos, N. K. (2014). Syndecans as modulators and potential pharmacological targets in cancer progression. Front. Oncol. 4:4. doi: 10.3389/fonc.2014.00004
Bass, M. D., and Humphries, M. J. (2002). Cytoplasmic interactions of syndecan-4 orchestrate adhesion receptor and growth factor receptor signalling. Biochem. J. 368, 1–15. doi: 10.1042/bj20021228
Beauvais, D. M., and Rapraeger, A. C. (2003). Syndecan-1-mediated cell spreading requires signaling by αvβ3 integrins in human breast carcinoma cells. Exp. Cell Res. 286, 219–232. doi: 10.1016/S0014-4827(03)00126-5
Berndt, C., Montañez, E., Villena, J., Fabre, M., Vilaró, S., and Reina, M. (2004). Influence of cytoplasmic deletions on the filopodia-inducing effect of syndecan-3. Cell Biol. Int. 28, 829–833. doi: 10.1016/j.cellbi.2004.08.003
Bernfield, M., Götte, M., Park, P. W., Reizes, O., Fitzgerald, M. L., Lincecum, J., et al. (1999). Functions of cell surface heparan sulfate proteoglycans. Annu. Rev. Biochem. 68, 729–777. doi: 10.1146/annurev.biochem.68.1.729
Bishop, J. R., Schuksz, M., and Esko, J. D. (2007). Heparan sulphate proteoglycans fine-tune mammalian physiology. Nature 446, 1030–1037. doi: 10.1038/nature05817
Brockstedt, U., Dobra, K., Nurminen, M., and Hjerpe, A. (2002). Immunoreactivity tocell surface syndecans in cytoplasm and nucleus: tubulin-dependentrearrangements. Exp. Cell Res. 274, 235–245. doi: 10.1006/excr.2002.5477
Carey, D. J. (1997). Syndecans: multifunctional cell-surface co-receptors. Biochem. J. 327, 1–16. doi: 10.1042/bj3270001
Carey, D. J., Bendt, K. M., and Stahl, R. C. (1996). The cytoplasmic domain of syndecan-1 is required for cytoskeleton association but not detergent insolubility. Identification of essential cytoplasmic domain residues. J. Biol. Chem. 271, 15253–15260. doi: 10.1074/jbc.271.25.15253
Chakravarti, R., Sapountzi, V., and Adams, J. C. (2005). Functional role of syndecan-1 cytoplasmic V region in lamellipodial spreading, actin bundling, and cell migration. Mol. Biol. Cell. 16, 3678–3691. doi: 10.1091/mbc.E04-10-0907
Choi, S., Lee, E., Kwon, S., Park, H., Yi, J. Y., Kim, S., et al. (2005). Transmembrane domain-induced oligomerization is crucial for the functions of syndecan-2 and syndecan-4. J. Biol. Chem. 280, 42573–42579. doi: 10.1074/jbc.M509238200
Choi, Y., Kim, H., Chung, H., Hwang, J. S., Shin, J. A., Han, I. O., et al. (2010). Syndecan-2 regulates cell migration in colon cancer cells through Tiam1-mediated Rac activation. Biochem. Biophys. Res. Commun. 391, 921–925. doi: 10.1016/j.bbrc.2009.11.165
Choi, Y., Kwon, M. J., Lim, Y., Yun, J. H., Lee, W., and Oh, E. S. (2015). Trans-regulation of syndecan functions by hetero-oligomerization. J. Biol. Chem. 290, 16943–16953. doi: 10.1074/jbc.M114.611798
Chu, Y. Q., Ye, Z. Y., Tao, H. Q., Wang, Y. Y., and Zhao, Z. S. (2008). Relationship between cell adhesion molecules expression and the biological behavior of gastric carcinoma. World J. Gastroenterol. 14, 1990–1996. doi: 10.3748/wjg.14.1990
Cohen, A. R., Woods, D. F., Marfatia, S. M., Walther, Z., Chishti, A. H., and Anderson, J. M. (1998). Human CASK/LIN-2 binds syndecan-2 and protein 4.1 and localizes to the basolateral membrane of epithelial cells. J. Cell Biol. 142, 129–138. doi: 10.1083/jcb.142.1.129
Cohen, N. A., Brenman, J. E., Snyder, S. H., and Bredt, D. S. (1996). Binding of the inward rectifier K+ channel Kir 2.3 to PSD-95 is regulated by protein kinase A phosphorylation. Neuron 17, 759–767. doi: 10.1016/S0896-6273(00)80207-X
Couchman, J. R. (2010). Transmembrane signaling proteoglycans. Annu. Rev. Cell Dev. Biol. 26, 89–114. doi: 10.1146/annurev-cellbio-100109-104126
Couchman, J. R., Gopal, S., Lim, H. C., Nørgaard, S., and Multhaupt, H. A. (2015). Syndecans: from peripheral coreceptors to mainstream regulators of cell behaviour. Int. J. Exp. Pathol. 96, 1–10. doi: 10.1111/iep.12112
Dedes, P. G., Gialeli, C. H., Tsonis, A. I., Kanakis, I., Theocharis, A. D., Kletsas, D., et al. (2012). Expression of matrix macromolecules and functional properties of breast cancer cells are modulated by the bisphosphonate zoledronic acid. Biochim. Biophys. Acta 1820, 1926–1939. doi: 10.1016/j.bbagen.2012.07.013
De Oliveira, T., Abiatari, I., Raulefs, S., Sauliunaite, D., Erkan, M., Kong, B., et al. (2012). Syndecan-2 promotes perineural invasion and cooperates with K-ras to induce an invasive pancreatic cancer cell phenotype. Mol. Cancer. 11:19. doi: 10.1186/1476-4598-11-19
Ethell, I. M., Hagihara, K., Miura, Y., Irie, F., and Yamaguchi, Y. (2000). Synbindin, a novel syndecan-2 binding protein in neuronal dendritic spines. J. Cell Biol. 151, 53–68. doi: 10.1083/jcb.151.1.53
Friand, V., David, G., and Zimmermann, P. (2015). Syntenin and syndecan in the biogenesis of exosomes. Biol. Cell. 107, 331–341. doi: 10.1111/boc.201500010
Gao, Y., Li, M., Chen, W., and Simons, M. (2000). Synectin, syndecan-4 cytoplasmic domain binding PDZ protein, inhibits cell migration. J. Cell. Physiol. 184, 373–379.
Gharbaran, R. (2015). Advances in the molecular functions of syndecan-1 (SDC1/CD138) in the pathogenesis of malignancies. Crit. Rev. Oncol. Hematol. 94, 1–17. doi: 10.1016/j.critrevonc.2014.12.003
Granés, F., García, R., Casaroli-Marano, R. P., Castel, S., Rocamora, N., Reina, M., et al. (1999). Syndecan-2 induces filopodia by active cdc42Hs. Exp. Cell Res. 248, 439–456. doi: 10.1006/excr.1999.4437
Granés, F., Urena, J. M., Rocamora, N., and Vilaró, S. (2000). Ezrin links syndecan-2 to the cytoskeleton. J. Cell. Sci. 113, 1267–1276.
Grootjans, J. J., Reekmans, G., Ceulemans, H., and David, G. (2000). Syntenin-syndecan binding requires syndecan-synteny and the co-operation of both PDZ domains of syntenin. J. Biol. Chem. 275, 19933–19941. doi: 10.1074/jbc.M002459200
Grootjans, J. J., Zimmermann, P., Reekmans, G., Smets, A., Degeest, G., Durr, J., et al. (1997). Syntenin, a PDZ protein that binds syndecan cytoplasmic domains. Proc. Natl. Acad. Sci. U.S.A. 94, 13683–13688. doi: 10.1073/pnas.94.25.13683
Hannafon, B. N., Sebastiani, P., De Las Morenas, A., Lu, J., and Rosenberg, C. L. (2011). Expression of microRNA and their gene targets are dysregulated in preinvasive breast cancer. Breast Cancer Res. 13:R24. doi: 10.1186/bcr2839
Harris, B. Z., and Lim, W. A. (2001). Mechanism and role of PDZ domains in signaling complex assembly. J. Cell Sci. 114, 3219–3231.
Hashimoto, Y., Skacel, M., and Adams, J. C. (2008). Association of loss of epithelial syndecan-1 with stage and local metastasis of colorectal adenocarcinomas: an immunohistochemical study of clinically annotated tumors. BMC Cancer 8:185. doi: 10.1186/1471-2407-8-185
Hassan, H., Greve, B., Pavao, M. S. G., Kiesel, L., Ibrahim, S. A., and Gotte, M. (2013). Syndecan-1 modulates B-integrin-dependent and interleukin-6-dependent functions in breast cancer cell adhesion, migration, and resistance to irradiation. FEBS J. 280, 2216–2227. doi: 10.1111/febs.12111
Hegedüs, T., Sessler, T., Scott, R., Thelin, W., Bakos,É, Váradi, A., et al. (2003). C-terminal phosphorylation of MRP2 modulates its interaction with PDZ proteins. Biochem. Biophys. Res. Commun. 302, 454–461. doi: 10.1016/S0006-291X(03)00196-7
Horowitz, A., and Simons, M. (1998). Regulation of syndecan-4 phosphorylation in vivo. J. Biol. Chem. 273, 10914–10918. doi: 10.1074/jbc.273.18.10914
Hung, A. Y., and Sheng, M. (2002). PDZ domains: structural modules for protein complex assembly. J. Biol. Chem. 277, 5699–5702. doi: 10.1074/jbc.R100065200
Ibrahim, S. A., Yip, G. W., Stock, C., Pan, J. W., Neubauer, C., Poeter, M., et al. (2012). Targeting of syndecan-1 by microRNA miR-10b promotes breast cancer cell motility and invasiveness via a Rho-GTPase- and E-cadherin-dependent mechanism. Int. J. Cancer. 131, 884–896. doi: 10.1002/ijc.27629
Ishikawa, T., and Kramer, R. H. (2010). Sdc1 negatively modulates carcinoma cell motility and invasion. Exp. Cell Res. 316, 951–965. doi: 10.1016/j.yexcr.2009.12.013
Jeleñ, F., Oleksy, A., Smietana, K., and Otlewski, J. (2003). PDZ domains-common players in the cell signaling. Acta Biochim. Pol. 50, 985–1017.
Kang, B. S., Cooper, D. R., Devedjiev, Y., Derewenda, U., and Derewenda, Z. S. (2003). Molecular roots of degenerate specificity in syntenin’s PDZ2 domain: reassessment of the PDZ recognition paradigm. Structure 11, 845–853. doi: 10.1016/S0969-2126(03)00125-4
Kashyap, R., Roucourt, B., Lembo, F., Fares, J., Carcavilla, A. M., Restouin, A., et al. (2015). Syntenin controls migration, growth, proliferation, and cell cycle progression in cancer cells. Front. Pharmacol. 6:241. doi: 10.3389/fphar.2015.00241
Kennedy, M. B. (1995). Origin of PDZ (DHR, GLGF) domains. Trends Biochem. Sci. 20:350. doi: 10.1016/S0968-0004(00)89074-X
Kim, C. W., Goldberger, O. A., Gallo, R. L., and Bernfield, M. (1994). Members of the syndecan family of heparan sulfate proteoglycans are expressed in distinct cell-tissu, and development-specific patterns. Mol. Biol. Cell. 5, 797–805. doi: 10.1091/mbc.5.7.797
Kinnunen, T., Kaksonen, M., Saarinen, J., Kalkkinen, N., Peng, H. B., and Rauvala, H. (1998). Cortactin-Src kinase signaling pathway is involved in N-syndecan-dependent neurite outgrowth. J. Biol. Chem. 273, 10702–10708. doi: 10.1074/jbc.273.17.10702
Kong, X., Qian, J., Chen, L. S., Wang, Y. C., Wang, J. L., Chen, H., et al. (2013). Synbindin in extracellular signal-regulated protein kinase spatial regulation and gastric cancer aggressiveness. J. Natl. Cancer Inst. 105, 1738–1749. doi: 10.1093/jnci/djt271
Koo, B. K., Jung, Y. S., Shin, J., Han, I., Mortier, E., Zimmermann, P., et al. (2006). Structural basis of syndecan-4 phosphorylation as a molecular switch to regulate signaling. J. Mol. Biol. 355, 651–663. doi: 10.1016/j.jmb.2005.09.087
Kumar-Singh, S., Jacobs, W., Dhaene, K., Weyn, B., Bogers, J., Weyler, J., et al. (1998). Syndecan-1 expression in malignant mesothelioma: correlation with cell differentiation, WT1 expression, and clinical outcome. J. Pathol. 186, 300–305. doi: 10.1002/(SICI)1096-9896(1998110)186:3<300::AID-PATH180>3.3.CO;2-H
Kwon, M. J., Park, J., Jang, S., Eom, C. Y., and Oh, E. S. (2015). The conserved phenylalanine in the transmembrane domain enhances heteromeric interactions of syndecans. J. Biol. Chem. 291, 872–881. doi: 10.1074/jbc.M115.685040
Lee, H. J., and Zheng, J. J. (2010). Review PDZ domains and their binding partners: structure, specificity, and modification. Interactions. PDZ domains and their binding partners: structure, specificity, and modification. Cell Commun. Signal. 8:8. doi: 10.1186/1478-811X-8-8
Leivonen, M., Lundin, J., Nordling, S., Von Boguslawski, K., and Haglund, C. (2004). Prognostic value of syndecan-1 expression in breast cancer. Oncology 67, 11–18. doi: 10.1159/000080280
Lendorf, M. E., Manon-Jensen, T., Kronqvist, P., Multhaupt, H. A., and Couchman, J. R. (2011). Syndecan-1 and syndecan-4 are independent indicators in breast carcinoma. J. Histochem. Cytochem. 59, 615–629. doi: 10.1369/0022155411405057
Lim, H., Multhaupt, H., and Couchman, J. R. (2015). Cell surface heparan sulfate proteoglycans control adhesion and invasion of breast carcinoma cells. Mol. Cancer 14:15. doi: 10.1186/s12943-014-0279-8
Liu, X., Shepherd, T. R., Murray, A. M., Xu, Z., and Fuentes, E. (2013). The structure of the Tiam1 PDZ domain/Phospho-Syndecan1 complex reveals a ligand conformation that modulates protein dynamics. Structure 21, 342–354. doi: 10.1016/j.str.2013.01.004
Luck, K., Charbonnier, S., and Travé, G. (2012). The emerging contribution of sequence context to the specificity of protein interactions mediated by PDZ domains. FEBS Lett. 586, 2648–2661. doi: 10.1016/j.febslet.2012.03.056
Lundin, M., Nordling, S., Lundin, J., Isola, J., Wiksten, J. P., and Haglund, C. (2005). Epithelial syndecan-1 expression is associated with stage and grade in colorectal cancer. Oncology 68, 306–313. doi: 10.1159/000086969
Manon-Jensen, T., Itoh, Y., and Couchman, J. R. (2010). Proteoglycans in health and disease: the multiple roles of syndecan shedding. FEBS J. 277, 3876–3889. doi: 10.1111/j.1742-4658.2010.07798.x
Matsuda, S., Mikawa, S., and Hirai, H. (1999). Phosphorylation of serine-880 in GluR2 by protein kinase C prevents its C terminus from binding with glutamate receptor-interacting protein. J. Neurochem. 73, 1765–1768. doi: 10.1046/j.1471-4159.1999.731765.x
Matsumoto, A., Ono, M., Fujimoto, Y., Gallo, R. L., Bernfield, M., and Kohgo, Y. (1997). Reduced expression of syndecan-1 in human hepatocellular carcinoma with highmetastatic potential. Int. J. Cancer 74, 482–491. doi: 10.1002/(SICI)1097-0215(19971021)74:5<482::AID-IJC2>3.0.CO;2-#
Mitselou, A., Skoufi, U., Tsimogiannis, K. E., Briasoulis, E., Vougiouklakis, T., Arvanitis, D., et al. (2012). Association of syndecan-1 with angiogenesis-related markers, extracellular matrix components, and clinicopathological features in colorectal carcinoma. Anticancer Res. 32, 3977–3985.
Morgan, M. R., Hamidi, H., Bass, M. D., Warwood, S., Ballestrem, C., and Humphries, M. J. (2013). Syndecan-4 phosphorylation as a control point for integrin recycling. Dev. Cell. 24, 472–485. doi: 10.1016/j.devcel.2013.01.027
Morgan, M. R., Humphries, M. J., and Bass, M. D. (2007). Synergistic control of cell adhesion by integrins and syndecans. Nat. Rev. Mol. Cell Biol. 8, 957–969. doi: 10.1038/nrm2289
Muders, M. H., Dutta, S. K., Wang, L., Lau, J. S., Bhattacharya, R., Smyrk, T. C., et al. (2006). Expression and regulatory role of GAIP-interacting protein GIPC in pancreatic adenocarcinoma. Cancer Res. 66, 10264–10268. doi: 10.1158/0008-5472.CAN-06-2321
Multhaupt, H. A., Yoneda, A., Whiteford, J. R., Oh, E. S., Lee, W., and Couchman, J. R. (2009). Syndecan signaling: when, where and why. J. Physiol. Pharmacol. 60, 31–38.
Oh, E. S., Woods, A., and Couchman, J. R. (1997). Multimerization of the cytoplasmic domain of syndecan-4 is required for its ability to activate protein kinase C. J. Biol. Chem. 272, 11805–11811. doi: 10.1074/jbc.272.18.11805
Oh, E. S., Woods, A., Lim, S. T., Theibert, A. W., and Couchman, J. R. (1998). Syndecan-4 proteoglycan cytoplasmic domain and phosphatidylinositol 4, 5-bisphosphate coordinately regulate protein kinase C activity. J. Biol. Chem. 273, 10624–10629. doi: 10.1074/jbc.273.17.10624
Ott, V. L., and Rapraeger, A. C. (1998). Tyrosine phosphorylation of syndecan-1 and-4 cytoplasmic domains in adherent B82 fibroblasts. J. Biol. Chem. 273, 35291–35298. doi: 10.1074/jbc.273.52.35291
Palaiologou, M., Delladetsima, I., and Tiniakos, D. (2014). CD138 (syndecan-1) expression in health and disease. Histol. Histopathol. 29, 177–189.
Park, H., Kim, Y., Lim, Y., Han, I., and Oh, E. S. (2002). Syndecan-2 mediates adhesion and proliferation of colon carcinoma cells. J. Biol. Chem. 277, 29730–29736. doi: 10.1074/jbc.M202435200
Philley, J. V., Kannan, A., and Dasgupta, S. (2016). MDA-9/Syntenin Control. J. Cell. Physiol. 231, 545–550. doi: 10.1002/jcp.25136
Prasthofer, T., Ek, B., Ekman, P., Owens, R., Hook, M., and Johansson, S. (1995). Protein kinase C phosphorylates two of the four known syndecan cytoplasmic domains in vitro. Biochem. Mol. Biol. Int. 36, 793–802.
Ramani, V. C., Purushothaman, A., Stewart, M. D., Thompson, C. A., Vlodavsky, I., Au, J. L., et al. (2013). The heparanase/syndecan-1 axis in cancer: mechanisms and therapies. FEBS J. 280, 2294–2306. doi: 10.1111/febs.12168
Rapraeger, A. C. (2013). Synstatin: a selective inhibitor of the syndecan-1-coupled IGF1R- αvβ3 integrin complex in tumorigenesis and angiogenesis. FEBS J. 280, 2207–2215. doi: 10.1111/febs.12160
Roucourt, B., Meeussen, S., Bao, J., Zimmermann, P., and David, G. (2015). Heparanase activates the syndecan-syntenin-ALIX exosome pathway. Cell Res. 25, 412–428. doi: 10.1038/cr.2015.29
Rousselle, P., and Beck, K. (2013). Laminin 332 processing impacts cellular behavior. Cell Adh. Mig. 7, 122–134. doi: 10.4161/cam.23132
Rousselle, P., and Letourneur, F. (2009). Mysterious tasks of tyrosines in syndecan-1 cytoplasmic tail. Sci. World J. 9, 629–632. doi: 10.1100/tsw.2009.87
Ryu, H. Y., Lee, J., Yang, S., Park, H., Choi, S., Jung, K. C., et al. (2009). Syndecan-2 functions as a docking receptor for pro-matrix metalloproteinase-7 in human colon cancer cells. J. Biol. Chem. 284, 35692–35701. doi: 10.1074/jbc.M109.054254
Sheng, M., and Sala, C. (2001). PDZ domains and the organization of supramolecular complexes. Annu. Rev. Neurosci. 24, 1–29. doi: 10.1146/annurev.neuro.24.1.1
Shepherd, T. R., Klaus, S. M., Liu, X., Ramaswamy, S., DeMali, K. A., and Fuentes, E. (2010). The Tiam1 PDZ domain couples to syndecan1 and promotes cell-matrix adhesion. J. Mol. Biol. 398, 730–746. doi: 10.1016/j.jmb.2010.03.047
Stanley, M. J., Stanley, M. W., Sanderson, R. D., and Zera, R. (1999). Syndecan-1 expression is induced in the stroma of infiltrating breast carcinoma. Am. J. Clin. Pathol. 112, 377–383.
Stepp, M. A., Pal-Ghosh, S., Tadvalkar, G., and Pajoohesh-Ganji, A. (2015). Syndecan-1 and its expanding list of contacts. Adv. Wound Care. 4, 235–249. doi: 10.1089/wound.2014.0555
Subbaiah, V. K., Kranjec, C., Thomas, M., and Banks, L. (2011). PDZ domains: the building blocks regulating tumorigenesis. Biochem. J. 439, 195–205. doi: 10.1042/BJ20110903
Sulka, B., Lortat-Jacob, H., Terreux, R., Letourneur, F., and Rousselle, P. (2009). Tyrosine dephosphorylation of the syndecan-1 PDZ binding domain regulates Syntenin-1 recruitment. J. Biol. Chem. 284, 10659–10671. doi: 10.1074/jbc.M807643200
Szatmári, T., Ötvös, R., Hjerpe, A., and Dobra, K. (2015). Syndecan-1 in cancer: implications for cell signaling, differentiation, and prognostication. Dis. Mark. 2015:796052. doi: 10.1155/2015/796052
Teng, Y. H., Aquino, R. S., and Park, P. W. (2012). Molecular functions of syndecan-1 in disease. Matrix Biol. 31, 3–16. doi: 10.1016/j.matbio.2011.10.001
Theocharis, A. D., Skandalis, S. S., Neill, T., Multhaupt, H. A., Hubo, M., Frey, H., et al. (2015). Insights into the key roles of proteoglycans in breast cancer biology and translational medicine. Biochim. Biophys. Acta. 1855, 276–300. doi: 10.1016/j.bbcan.2015.03.006
Tkachenko, E., Elfenbein, A., Tirziu, D., and Simons, M. (2006). Syndecan-4 clustering induces cell migration in a PDZ-dependent manner. Circ. Res. 98, 1398–1404. doi: 10.1161/01.RES.0000225283.71490.5a
Vicente, C. M., Ricci, R., Nader, H. B., and Toma, L. (2013). Syndecan-2 is upregulated in colorectal cancer cells through interactions with extracellular matrix produced by stromal fibroblasts. BMC Cell Biol. 14:25. doi: 10.1186/1471-2121-14-25
Vigil, D., Cherfils, J., Rossman, K. L., and Der, C. J. (2010). Ras superfamily GEFs and GAPs: validated and tractable targets for cancer therapy? Nat. Rev. Cancer 10, 842–857. doi: 10.1038/nrc2960
Wang, C. K., Pan, L., Chen, J., and Zhang, M. (2010). Extensions of PDZ domains as important structural and functional elements. Protein Cell. 1, 737–751. doi: 10.1007/s13238-010-0099-6
Wang, H., Jin, H., Beauvais, D. M., and Rapraeger, A. C. (2014). Cytoplasmic domain interactions of syndecan-1 and syndecan-4 with α6β4 integrin mediate human epidermal growth factor receptor (HER1 and HER2)-dependent motility and survival. J. Biol. Chem. 289, 30318–30332. doi: 10.1074/jbc.M114.586438
Wang, H., Jin, H., and Rapraeger, A. C. (2015). Syndecan-1 and syndecan-4 capture epidermal growth factor receptor family members and the α3β1 integrin via binding sites in their ectodomains: novel synstatins prevent kinase capture and inhibit α6β4-integrin-dependent epithelial cell motility. J. Biol. Chem. 290, 26103–26113. doi: 10.1074/jbc.M115.679084
Wawrzyniak, A. M., Vermeiren, E., Zimmermann, P., and Ivarsson, Y. (2012). Extensions of PDS-95/disc large/ZO-1 (PDZ) domains influence lipid binding and membrane targeting of syntenin-1. FEBS Lett. 586, 1445–1451. doi: 10.1016/j.febslet.2012.04.024
Wei, H. T., Guo, E. N., Dong, B. G., and Chen, L. S. (2015). Prognostic and clinical significance of syndecan-1 in colorectal cancer: a meta-analysis. BMC Gastroenterol. 15:152. doi: 10.1186/s12876-015-0383-2
Wei, J. L., Fu, Z. X., Fang, M., Zhou, Q. Y., Zhao, Q. N., Guo, J. B., et al. (2014). High expression of CASK correlates with progression and poor prognosis of colorectal cancer. Tumour Biol. 35, 9185–9194. doi: 10.1007/s13277-014-2179-3
Ye, F., and Zhang, M. (2013). Structures and target recognition modes of PDZ domains: recurring themes and emerging pictures. Biochem. J. 455, 1–14. doi: 10.1042/BJ20130783
Yoneda, A., and Couchman, J. R. (2003). Regulation of cytoskeletal organization by syndecan transmembrane proteoglycans. Matrix Biol. 22, 25–33. doi: 10.1016/S0945-053X(03)00010-6
Zellweger, T., Ninck, C., Mirlacher, M., Annefeld, M., Glass, A. G., Gasser, T. C., et al. (2003). Tissue microarray analysis reveals prognostic significance of syndecan-1 expression in prostate cancer. Prostate 55, 20–29. doi: 10.1002/pros.10209
Zimmermann, P. (2006). The prevalence and significance of PDZ domain-phosphoinositide interactions. Biochim. Biophys. Acta 1761, 947–956. doi: 10.1016/j.bbalip.2006.04.003
Keywords: syndecan, cancer, PDZ domain, phosphorylation, cytoskeleton, extracellular matrix
Citation: Cheng B, Montmasson M, Terradot L and Rousselle P (2016) Syndecans as Cell Surface Receptors in Cancer Biology. A Focus on their Interaction with PDZ Domain Proteins. Front. Pharmacol. 7:10. doi: 10.3389/fphar.2016.00010
Received: 26 November 2015; Accepted: 12 January 2016;
Published: 02 February 2016.
Edited by:
Hervé Emonard, Centre National de la Recherche Scientifique – Université de Reims Champagne-Ardenne, FranceReviewed by:
Olivier Micheau, Institut National de la Santé et de la Recherche Médicale, FranceStéphane Dedieu, CNRS UMR 7369 MEDYC and Reims University, France
Copyright © 2016 Cheng, Montmasson, Terradot and Rousselle. This is an open-access article distributed under the terms of the Creative Commons Attribution License (CC BY). The use, distribution or reproduction in other forums is permitted, provided the original author(s) or licensor are credited and that the original publication in this journal is cited, in accordance with accepted academic practice. No use, distribution or reproduction is permitted which does not comply with these terms.
*Correspondence: Patricia Rousselle, cGF0cmljaWEucm91c3NlbGxlQGliY3AuZnI=