- 1Sections of Human Anatomy and Histology, Department of Biomedical and Biotechnological Sciences, University of Catania, Catania, Italy
- 2San Raffaele Open University of Rome, Rome, Italy
- 3Section of Anatomic Pathology, Department of Medical and Surgical Sciences and Advanced Technologies, G.F. Ingrassia, Azienda Ospedaliero-Universitaria “Policlinico-Vittorio Emanuele”, University of Catania, Catania, Italy
- 4Institute of Neurological Sciences, National Research Council, Catania, Italy
- 5Section of Pharmacology, Department of Biomedical and Biotechnological Sciences, University of Catania, Catania, Italy
Pituitary adenylate cyclase-activating polypeptide (PACAP) and vasoactive intestinal peptide (VIP) through the binding of vasoactive intestinal peptide receptors (VIPRs), perform a wide variety of effects in human cancers, including glioblastoma multiforme (GBM). This tumor is characterized by extensive areas of hypoxia, which triggers the expression of hypoxia-inducible factors (HIFs). HIFs not only mediate angiogenesis but also tumor cell migration and invasion. Furthermore, HIFs activation is linked to epidermal growth factor receptor (EGFR) overexpression. Previous studies have shown that VIP interferes with the invasive nature of gliomas by regulating cell migration. However, the role of VIP family members in GBM infiltration under low oxygen tension has not been clarified yet. Therefore, in the present study we have investigated, for the first time, the molecular mechanisms involved in the anti-invasive effect of PACAP or VIP in U87MG glioblastoma cells exposed to hypoxia induced by treatment with desferrioxamine (DFX). The results suggest that either PACAP or VIP exert an anti-infiltrative effect under low oxygen tension by modulating HIFs and EGFR expression, key elements involved in cell migration and angiogenesis. These peptides act through the inhibition of PI3K/Akt and MAPK/ERK signaling pathways, which are known to have a crucial role in HIFs regulation.
Introduction
Pituitary adenylate cyclase-activating polypeptide (PACAP) and vasoactive intestinal peptide (VIP) belong to a family of polypeptides, comprising also peptide histidine-methionine (PHM), secretin, glucagon, glucagon-like peptide (GLP), glucose-dependent insulinotropic polypeptide (GIP), growth hormone releasing hormone (GHRH), and helodermin (Sherwood et al., 2000). They have sequence homology and explicate their functions by binding to vasoactive intestinal peptide receptors (VIPRs) including PAC1, VPAC1, and VPAC2 receptors.
Pituitary adenylate cyclase-activating polypeptide interacts, with high affinity, to PAC1 receptor, whereas both PACAP and VIP can activate either VPAC1 or VPAC2 receptor (Harmar et al., 2012; Tang et al., 2014). VIPRs consist of seven transmembrane domains, a large N-terminal fragment that includes the binding site for PACAP/VIP and an intracellular C-terminal region coupled to heterodimeric G-proteins associated with various signal transduction pathways (Laburthe et al., 2002). Indeed, their stimulation ultimately results in the activation of Protein Kinase A (PKA) (Couvineau et al., 2003; Dickson et al., 2006; Dickson and Finlayson, 2009; Vaudry et al., 2009), Protein Kinase C (PKC) (Vaudry et al., 2000), MAPKs (mitogen-activated protein kinases) (Barrie et al., 1997; Lelièvre et al., 1998), NF-κB signaling pathways (Delgado and Ganea, 1999).
Pituitary adenylate cyclase-activating polypeptide and VIP perform a regulatory role as neurotransmitters and/or neuromodulators in the peripheral and central nervous system (CNS). They are involved in different biological processes such as neuronal survival, stress response, cell division, neuro-, and glio-protective actions (Cavallaro et al., 1995; Canonico et al., 1996; D’Agata et al., 1996; D’Agata and Cavallaro, 1998; Jaworski, 2000; Castorina et al., 2008, 2010a, 2014; Giunta et al., 2012). Furthermore, they play neuroprotective action in various neurodegenerative diseases, comprising Parkinson’s disease (Brenneman, 2007; Dejda et al., 2008; Reglodi et al., 2011; Seaborn et al., 2011; Waschek, 2013). Different studies have also shown that these peptides, through the binding to VIPRs, play a wide variety of effects in human tumors, including GBM (Robberecht et al., 1993, 1994; Oka et al., 1998; Reubi et al., 2000; Juarranz et al., 2001; Isobe et al., 2003). However, their role seems to be controversial depending on histopathological features of cancer. In fact, in some instances, PACAP and VIP stimulate tumor mass growth whereas in others they show antiproliferative effect (Castorina et al., 2008, 2012; Giunta et al., 2010; D’Amico et al., 2013).
More recently, Cochaud et al. (2015) have suggested that VIP interferes with the infiltrative nature of GBM by regulating cell migration. This tumor is the most common and malignant form of primary brain cancer in adults (Wen and Kesari, 2008). Its poor prognosis is related to the therapeutic failure, mainly due to its highly invasive features leading to local or distant recurrences. Indeed, neoplastic cells can migrate to the surrounding tissue, travel along white matter tracts and blood vessel walls to reach other brain areas (Nakada et al., 2007; Dunn et al., 2012).
Glioblastoma multiforme, like other solid tumors, contains extensive areas of hypoxia associated to tissue necrosis and development of aberrant neovascularization (Kaur et al., 2005). In fact, in these regions, low oxygen tension induces expression of hypoxia-inducible factors (HIFs) which mediate the adaptive response through new blood vessels formation. These factors are heterodimeric complexes, including an oxygen-labile α- and a more stable β-subunit (ARNT), involved either in physiological or pathological angiogenesis (Semenza, 1999a,b; Maynard and Ohh, 2004).
In humans there are three HIF-α genes: HIF-1α, HIF-2α, and HIF-3α. In particular, HIF-1α is the key modulator in cellular response to low oxygen tension. It is overexpressed in GBM thereby contributing to intense angiogenesis (Kaur et al., 2005; Zagzag et al., 2006). HIF-2α is also induced by hypoxia, but it plays a major role under chronic insult (Tian et al., 1997; Koh et al., 2011). Several studies have associated HIF-2α up-regulation to development of an aggressive tumor phenotype (Helczynska et al., 2008; Qing and Simon, 2009; Koh and Powis, 2012). Moreover, overexpression of both HIF-1α and HIF-2α has been related to poor prognosis of different cancer, including GBM (Holmquist-Mengelbier et al., 2006; Franovic et al., 2009).
In this tumor, these factors are not only involved in angiogenesis but they also stimulate tumor cell migration and invasion (Zagzag et al., 2006; Fujiwara et al., 2007). Furthermore, the hypoxic microenvironment and activation of HIF-2α in the core of solid tumors induces overexpression of epidermal growth factor receptor (EGFR). Amplification and/or mutations of EGFR are commonly found in GMB. EGFR overexpression, indeed, has been recognized as a prognostic marker of advanced tumoral stage, resistance to standard therapy and reduced patient’s survival (Blehm et al., 2006; Halatsch et al., 2006; Franovic et al., 2007). Under low oxygen tension, accumulation of elevated EGFR levels, in turn mediated by increased HIF-2α, participates to autonomy in tumor cell growth through an autocrine signaling mechanism (Franovic et al., 2007).
A previous study has suggested the involvment of VIP in tumor invasion, but the role of PACAP and its receptors in this biological context still needs to be elucidated. Considering the relevance of the hypoxic microenvironment in determining tumor aggressivity, in the present study we investigated the effect of these peptides in the modulation of HIFs and EGFR expression, both key elements involved in cell migration and angiogenesis. To this end, we also analyzed the underlying molecular pathways by focusing on phosphoinositide three kinase (PI3K)/Akt and mammalian mitogen activated protein kinase/Erk kinase (MAPK/ERK) signaling cascades, since, as previously demonstrated, they interfere with HIF-1α and HIF-2α expression (Mottet et al., 2002; Lim et al., 2004; Park et al., 2011; Zhang et al., 2011).
Our results suggest that these peptides exert an anti-invasive action under hypoxia by modulating HIFs and EGFR expression. This effect is mediated through the inhibition of PI3K/Akt and MAPK/ERK signaling pathways. These data confirm previous findings suggesting that tumor microenvironment may act as an oncogene promoter triggering the autonomous growth of tumor cells. Therefore, the identification of molecules modulating the hypoxic event might give new insights in the therapeutic approach to GBM.
Materials and Methods
Human Brain Samples and Cell Lines
The study was performed on glioblastoma frozen sections from a sample provided by Anatomic Pathology of “G.F. Ingrassia” Department, after patient’s signed informed consent. Experiments were also carried on human glioblastoma cell line, U87MG (ATCCC number #HTB-14). Cells were cultured in Dulbecco’s modified Eagle’s medium (DMEM) supplemented with 10% of heat-inactivated fetal bovine serum (FBS), 100 U/ml penicillin and 100-μg/ml streptomycin (Sigma–Aldrich, Steinheim, Germany). They were incubated at 37°C in a humidified atmosphere with 5% CO2 as previously described by Maugeri et al. (2016). Cells grown under hypoxia were exposed for 24 h to 100 μM desferrioxamine mesylate salt (DFX) (Sigma–Aldrich), an hypoxia-mimetic agent, which induces hypoxia via inhibition of the HIF prolyl hydroxylases (Epstein et al., 2001; Hirsilä et al., 2005). As compared to the cell incubation method in hypoxic chamber, this offers the advantage to the experimentator to open the culture plate/dish/flask many times without affecting the hypoxic condition.
Treatments
Pituitary adenylate cyclase activating polypeptide-38 (PACAP38, 100 nM; cat no. A1439, Sigma–Aldrich), VIP, (100 nM; cat no. V3628, Sigma–Aldrich), PACAP receptor antagonist (PACAP6–38, 10 μM; cat no.3236, Tocris Biosciences, Bristol, UK) and selective VIP receptor antagonist (D-p-Cl-Phe6,Leu17-VIP, 10 μM; cat no. 3054, Tocris Biosciences) were added to U87MG cells for 24 h in normoxic or hypoxic condition.
Inhibition of PI3K/Akt and MAPK/ERK signaling pathways was performed following a pretreatment of 30 min with a PI3K (Wortmannin, 10 μM; cat no.1232/5, Tocris Biosciences) or MEK1 inhibitor (PD98059, 50 μM; cat no. #P215, Sigma–Aldrich,) under normoxia or hypoxia (Aleppo et al., 1992; Castorina et al., 2010b).
Cell Migration Assay
After trypsinization, U87MG cells were resuspended, counted and seeded onto a six-well plate at a density of 5 × 104 cells/well. A scratch was made in cell monolayer with a 200-μL pipette tip. Then, to remove the suspended cells, it was washed twice with PBS and incubated in medium containing 100 nM PACAP38 or 100 nM VIP or 10 μM Wortmannin or 50 μM PD98059, either in normoxic or hypoxic condition. The wounded areas were visualized under a microscope for quantification. The distance that the advancing cells had moved into the cell-free (wound) area was measured after 24 h by staining with crystal violet. Migration was calculated as the average number of cells observed in five random high power wounded fields/per well in duplicate wells.
Western Blot Analysis
Western blot analysis was performed according to the procedures previously described by Magro et al. (2011). Proteins were extracted with buffer containing 20 mM Tris (pH 7.4), 2 mM EDTA, 0.5 mM EGTA; 50 mM mercaptoethanol, 0.32 mM sucrose and a protease inhibitor cocktail (Roche Diagnostics, Monza, Italy) using a Teflon-glass homogenizer and then sonicated twice for 20 s using an ultrasonic probe, followed by centrifugation at 10,000 × g for 10 min at 4°C. Protein concentrations were determined by the Quant-iT Protein Assay Kit (Invitrogen,Carlsbad, CA, USA). About 65 μg from fresh frozen section per sample, and about 30 μg of protein homogenate were diluted in 2X Laemmli buffer (Invitrogen), heated at 70°C for 10 min and then separated on a Biorad Criterion XT 4–15% Bis-tris gel (Invitrogen) by electrophoresis and then transferred to a nitrocellulose membrane (Invitrogen). Blots were blocked using the Odyssey Blocking Buffer (Li-Cor Biosciences, Nebraska, NE, USA). The transfer was monitored by a prestained protein molecular weight marker [BioRad Laboratories, Segrate (MI), Italy]. Immunoblot analysis was performed by using appropriate antibodies: rabbit anti-PACAP (H-76, cat no. sc-25439, Santa Cruz Biotechnology, Texas City, TX, USA 1:200), mouse anti-VIP (H-6, cat no. sc-25347, Santa Cruz Biotechnology; 1:100), (rabbit anti-PAC1 receptor (H-55, cat no.sc-30018, Santa Cruz Biotechnology; 1:300), rabbit anti-VPAC1 (H-130, cat no. sc-30019, Santa Cruz Biotechnology; 1:200), rabbit anti-VPAC2 (H-50, cat no. sc-30020, Santa Cruz Biotechnology; 1:200), mouse anti- HIF-1alpha (cat.n. NB100-105, Novus Biologicals, Littleton, CO, USA 1:500), rabbit anti- HIF-2alpha (cat.n. NB100-122, Novus Biologicals, 1:500), rabbit anti- EGFR (cat.n. sc-03 Santa Cruz Biotechnology), rabbit anti-phospho Akt (Ser473 residue) (D9E, cat no. #4060, Cell Signaling, Danver, MA, USA; 1:1000), rabbit anti-total Akt (C67E7, cat no. #4691, Cell Signaling; 1:1000), mouse anti-phospho Erk-1/2 (Thr202 and Tyr204 residues) (pT202/pY204.22A, cat no. sc-136521, Santa Cruz Biotechnology; 1:200), mouse anti-total Erk-1/2 (MK1, cat no. sc-135900, Santa Cruz Biotechnology; 1:200), and rabbit anti-β-tubulin (cat n.sc-9104, Santa Cruz Biotechnology; 1:500). The secondary antibody goat anti-rabbit IRDye 800CW (cat #926-32211; Li-Cor Biosciences) and goat antimouse IRDye 680CW (cat #926-68020D, Li-Cor Biosciences) were used at 1:20,000 and 1:30,000, respectively. Blots were scanned with an Odissey Infrared Imaging System (Odyssey). Densitometric analyses of Western blot signals were performed at non-saturating exposures and analyzed using the ImageJ software1 (NIH, Bethesda, MD, USA). Values were normalized to β-tubulin, which served as loading control, as previously described by Maugeri et al. (2015).
Immunohistochemical Analysis
Fresh-frozen sections of a surgically resected tumor included in OCT were cut and fixed in 4% paraformaldehyde for 30 min. Then, they were treated with 3% H2O2 in methanol for 10 min to inhibit the endogenous peroxidase activity as previously described by D’Agata et al. (2000). To reduce non-specific staining, sections were treated with 1% bovine serum albumin (BSA) in PBS for 1 h, and incubated overnight at 4°C with appropriate antibody. The sections were rinsed in PBS and incubated with diaminobenzidine (DAB) for 5 min. Hematoxylin was used as nuclear counterstain. The stained sections were dehydrated through graded alcohols, cleared in xylene, and covered with neutral balsam.
Statistical Analysis
Data are represented as mean ± standard error (SEM). One-way analysis of variance (ANOVA) was used to compare differences among groups, and statistical significance was assessed by the Tukey–Kramer post hoc test. The level of significance for all statistical tests was set at p ≤ 0.05.
Results
Expression of PACAP, VIP, VIPRs, HIF-1α, HIF-2α, and EGFR in Human Glioblastoma
As previously demonstrated, here, we found that the precursor proteins of PACAP and VIP, and VIPRs are expressed in GBM; however, precursor peptides levels seemed to be lower as compared to their receptors (Figure 1A). To correlate this finding with tumor malignancy, we have confirmed HIF-1α, HIF-2α, and EGFR expression in sections from the same frozen sample by western blot analysis (Figure 1B). Furthermore, we have determined their tissue localization by immunohistochemical analysis. An heterogeneous tissue staining of HIF-1α, HIF-2α, and EGFR has been observed in this tumor (Figure 1B).
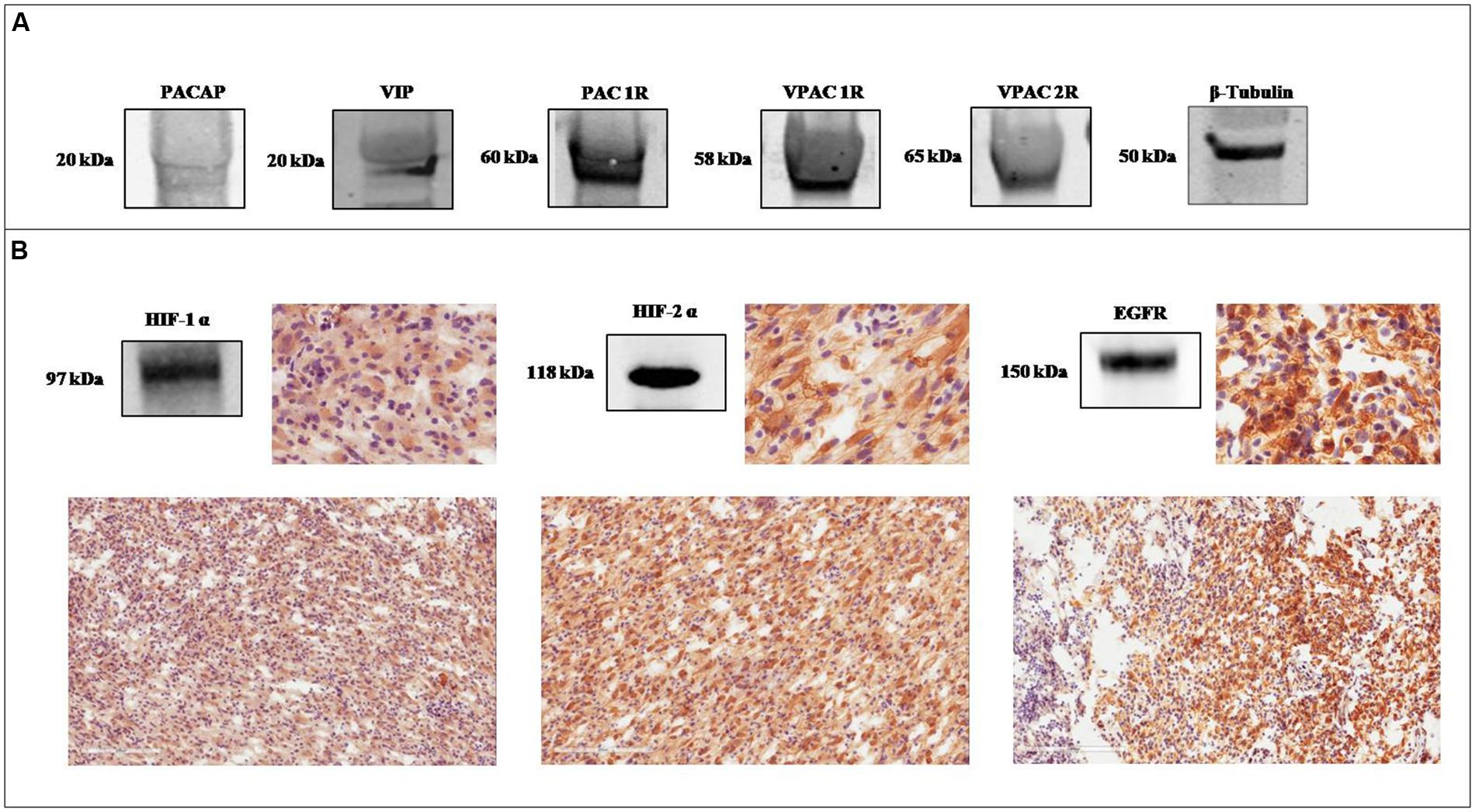
FIGURE 1. Expression of PACAP, VIP, VIPRs, HIF-1α, HIF-2α, and EGFR in glioblastoma multiforme (GBM). (A) Representative immunoblot of PACAP and VIP precursor peptides and PAC1R, VPAC1R, and VPAC2R expression on frozen glioblastoma sample. (B) Representative immunoblot and photomicrographs of signals detected by antibodies direct against HIF-1α, HIF-2α, and EGFR in a frozen glioblastoma sample.
PACAP and VIP Antagonize Hypoxia-Mediated GBM Cell Migration by Reducing HIFs and EGFR Expression
To characterize the role of these peptides in hypoxic areas of GBM, we have analyzed the expression of PACAP or VIP precursor proteins in U87MG tumor cells, grown for 24 h under normoxia or hypoxia. As shown in Figure 2, both precursor peptides and VIPRs receptors were expressed in these cells under normal oxygen tension, however, their levels were significantly increased following the hypoxic insult (Figure 2B, *p < 0.05 or ***p < 0.001 vs. normoxia). In view of this result, we have investigated the effect of these peptides on proliferation and invasion of gliomas cells during hypoxic process, which represents a distinctive biological feature of malignant cells. Therefore, we have analyzed the effect of 100 nM PACAP and VIP on tumoral cells invasivity. At this concentration, both peptides showed antiproliferative properties, as previously described by D’Amico et al. (2013). The dose used is higher as compared to their tumor tissues level. However, considering that tumoral mass is highly heterogeneous, comprehending various cell types with different mutations and degree of differentiation, in the present study, we decided to omit the characterization of their physiological role in cancer. Then, we have focused on the effect of exogenous peptides in a homogeneous cell culture. As shown in Figure 3, migration rate increased in GBM cells exposed to DFX as compared to normoxic control (Vhl). PACAP or VIP treatment significantly decreased cell invasion both under normal or low oxygen tension. Similarly, treatment with Wortmannin or PD98059, a PI3K and MEK1 inhibitors, respectively, induced significant reduction of cell’s migration confirming that tumor cell invasion and proliferation is mediated via activation of PI3K/AKT and ERK pathway (***p < 0.001 vs. Vhl in normoxia; ###p < 0.001 vs. Vhl in hypoxia).
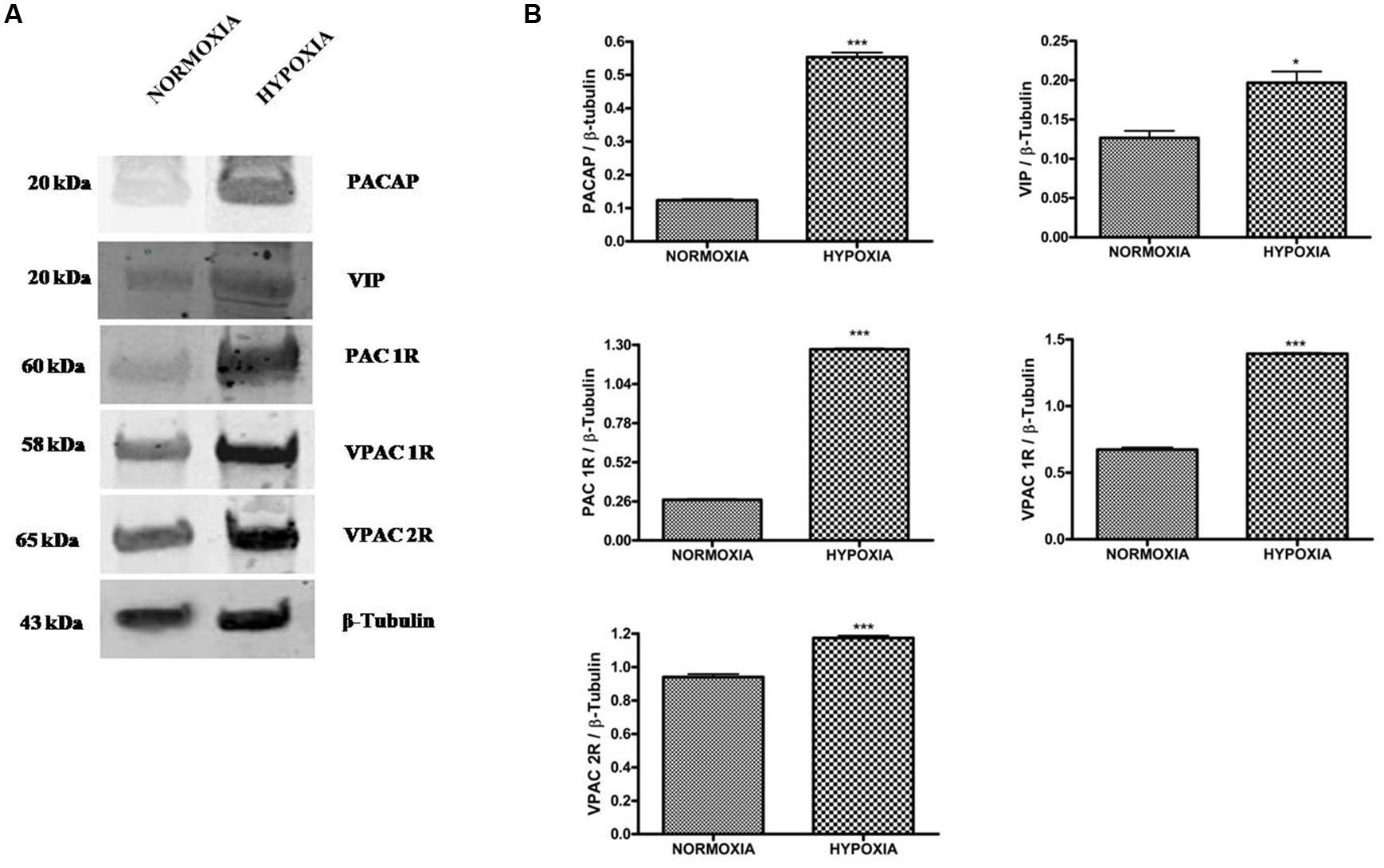
FIGURE 2. Expression of PACAP, VIP, PAC1R, VPAC1R, and VPAC2R in glioblastoma cells under normoxic or hypoxic conditions. (A) Representative immunoblot of PACAP and VIP precursor peptides and PAC1R, VPAC1R, and VPAC2R expression on U87MG cells grown normoxia or exposed to hypoxia. (B) The bar graphs show quantitative analysis of signals obtained on immunoblots resulting from three independent experiments. Relative band densities were quantified by using ImageJ software. Protein levels are expressed as arbitrary units obtained after normalization to β-tubulin which was used as loading control. Data represent means ± SEM (*p < 0.05 or ***p < 0.001 vs. Normoxia as determined by unpaired two-tailed Student t-test).
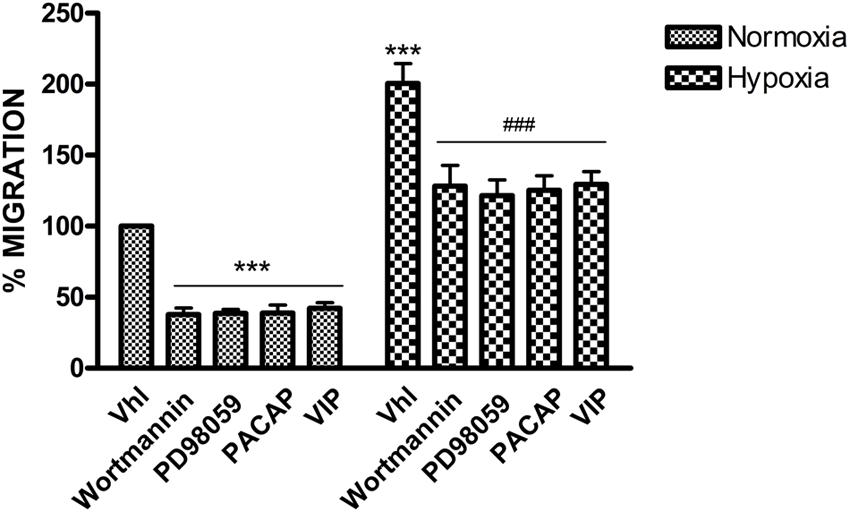
FIGURE 3. Effect of PACAP, VIP, PI3K, and MEK1 inhibitors on U87-MG cells migration. Cells monolayer was scraped by a pipette tip and incubated with PACAP, VIP, Wortmannin or PD98059 for 24 h under normoxia or hypoxia. The wounded areas were visualized under a microscope for quantification. Migration was calculated as the average number of cells observed in five random high power wounded fields/per well in duplicate wells. In the bar graph values are expressed as percentage of control. (***p < 0.001 vs. Vhl under normoxia; ###p < 0.001 vs. Vhl under hypoxia).
Based on these results, we further explored whether these peptides have performed their effect through modulation of HIFs and EGFR expression. PACAP treatment induced a significant reduction of HIF-1α, HIF-2α, and EGFR, either in normoxia or hypoxia, as compared to control. Furthermore, to confirm whether the effect of this peptide was mediated through the selective activation of PAC1 receptor, we also treated cells with a PACAP antagonist, such as PACAP 6–38, under normoxic or hypoxic condition. PACAP 6–38 treatment restored the expression of these proteins to their relative control levels, both in normoxia or hypoxia, confirming the involvement of PAC1 receptor (Figure 4B, *p < 0.05, **p < 0.01 and ***p < 0.001 vs. Vhl; ###p < 0.001 vs. Vhl in hypoxia; $$p < 0.01 $$$p < 0.001 vs. PACAP).
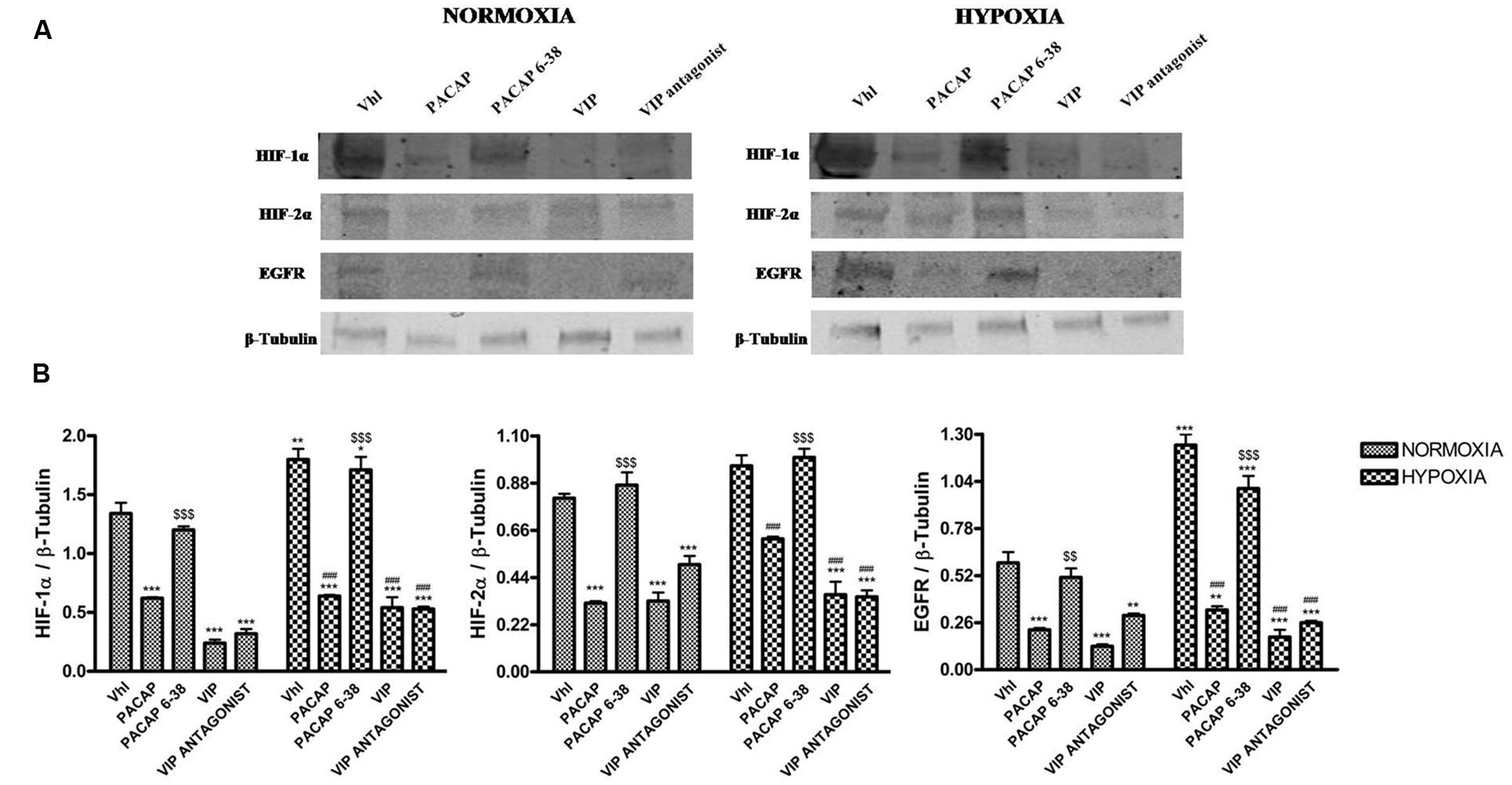
FIGURE 4. Effect of PACAP, PACAP6-38, VIP, and VIP antagonist on expression of HIF-1α, HIF-2α, and EGFR in U87-MG cells under normoxia and hypoxia. (A) Representative immunoblots of HIF-1α, HIF-2α, and EGFR expression on U87MG cells grown normoxia or exposed to hypoxia. (B) The bar graphs show quantitative analysis of signals obtained by immunoblots resulting from three independent experiments. Relative band densities were quantified by using ImageJ software. Protein levels are expressed as arbitrary units obtained after normalization to β-tubulin which was used as loading control. Data represent means ± SEM (*p < 0.05, **p < 0.01, or ***p < 0.001 vs. Vhl under normoxia; ###p < 0.001 vs. Vhl under hypoxia $$p < 0.01 or $$$p < 0.001 vs. PACAP, as determined by one-way ANOVA followed by the Tukey post hoc test).
Similarly to PACAP, also VIP treatment significantly reduces expression levels of HIFs and EGFR as compared to relative controls. However, VIP antagonist treatment was not able to restore HIF-1α, HIF-2α, and EGFR expression to control levels (Figure 4B, *p < 0.05, **p < 0.01, and ***p < 0.001 vs. Vhl; ###p < 0.001 vs. Vhl in hypoxia).
PACAP and VIP Decrease HIFs and EGFR Expression, through Inhibition of PI3K/Akt and MAPK/ERK Pathways
Remarkably, PI3K/Akt and the MAPK/ERK signaling cascades are aberrantly activated in many cancers, including GBM. The stimulation of these pathways leads to increase of HIF-1α and HIF-2α levels, which are involved in the aggressive behavior of tumor and promotion of angiogenesis. Furthermore, HIFs promote EGFR activation. To confirm this crucial link, we have treated cells with Wortmannin or PD98059. As shown in Figure 5, both substances induced a statistically significant decrease of HIF-1α, HIF-2α and EGFR expression as compared to control cells grown either under normoxia or hypoxia (Figure 5, **p < 0.01, ***p < 0.001 vs. Vhl in normoxia; ###p < 0.001 vs. Vhl in hypoxia).
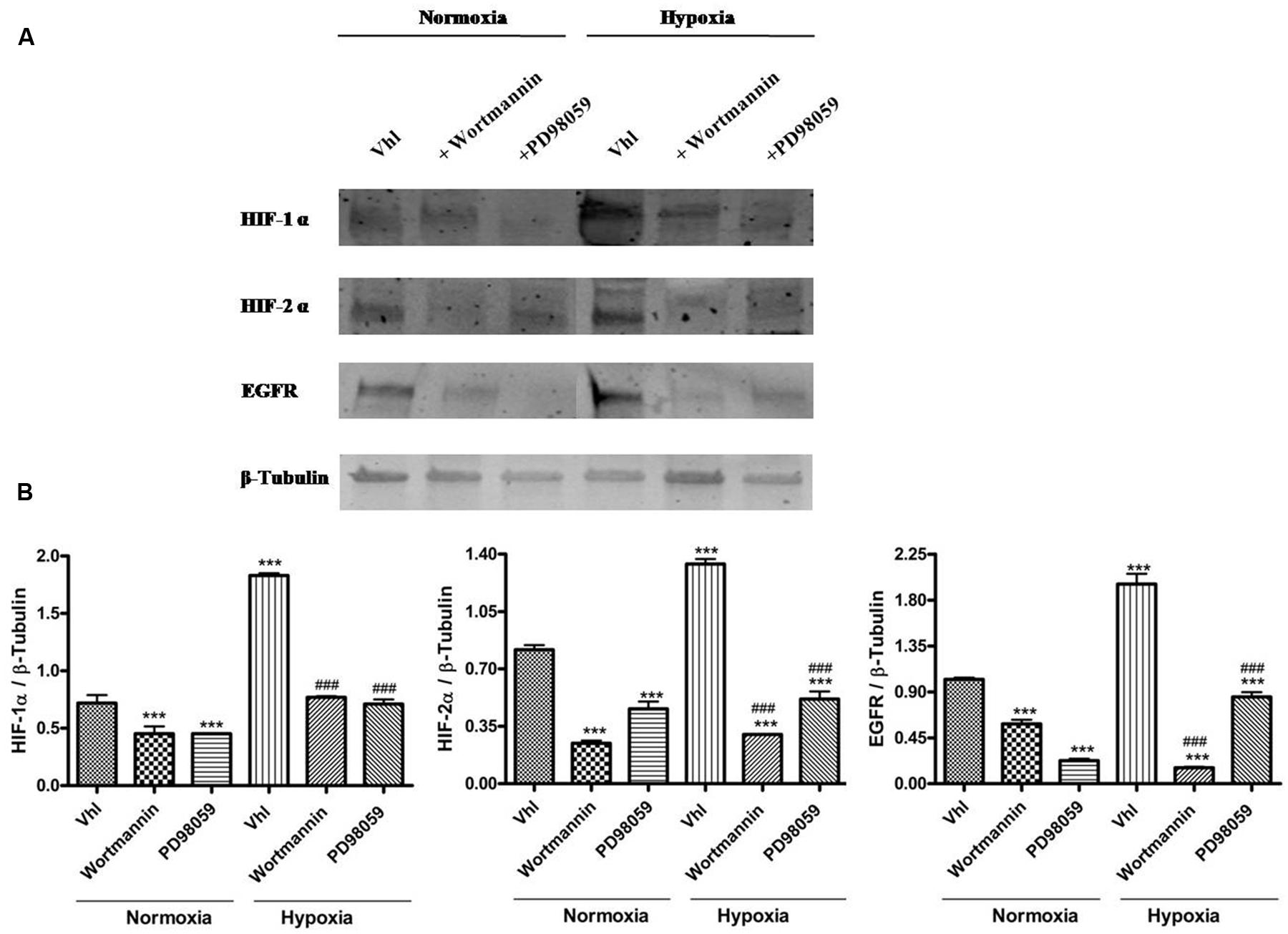
FIGURE 5. Expression of HIF-1α, HIF-2α, and EGFR following inhibition of PI3K/Akt or MAPPK/Erk kinase signaling pathway. (A) Representative immunoblot of HIF-1α, HIF-2α, and EGFR expression on U87MG cells treated with 10 μM Wortmannin or with 50 μM PD98059 and grown normoxia or exposed to hypoxia. (B) Relative density of each band was quantified using ImageJ software. Each signal was normalized on correspondent β-tubulin signal. Data are expressed as mean ± SEM (**p < 0.01 and ***p < 0.001 vs. Vhl under normoxia; ###p < 0.001 vs. Vhl under hypoxia as determined by one-way ANOVA followed by the Tukey post hoc test).
To investigate whether PACAP and VIP regulate HIFs and EGFR levels, through inhibition of these signaling pathways, we assessed their effect on phosphorylation of two signaling proteins. As shown in Figure 6, Akt and ERK1/2 are activated to comparable levels both under normoxia or hypoxia. We have hypothesized that this may be due to lack of the functional gene opposing tumor suppressor lipid phosphatase (PTEN) in the glioma cell line, U87MG, used. This is a tumor suppressor gene acting as a negative regulator of both PI3K/Akt and ERK1/2 signaling pathways (Li et al., 1997; Koul, 2008; Chetram and Hinton, 2012; Song et al., 2012). Instead, the treatment of U87MG cells with these peptides significantly decreased the level of phosphorylated Ser473 AKT and ERK1/2 both in normoxia and hypoxia (Figures 6A,B, ***p < 0.001 vs. Vhl in normoxia; ###p < 0.001 vs. Vhl in hypoxia).
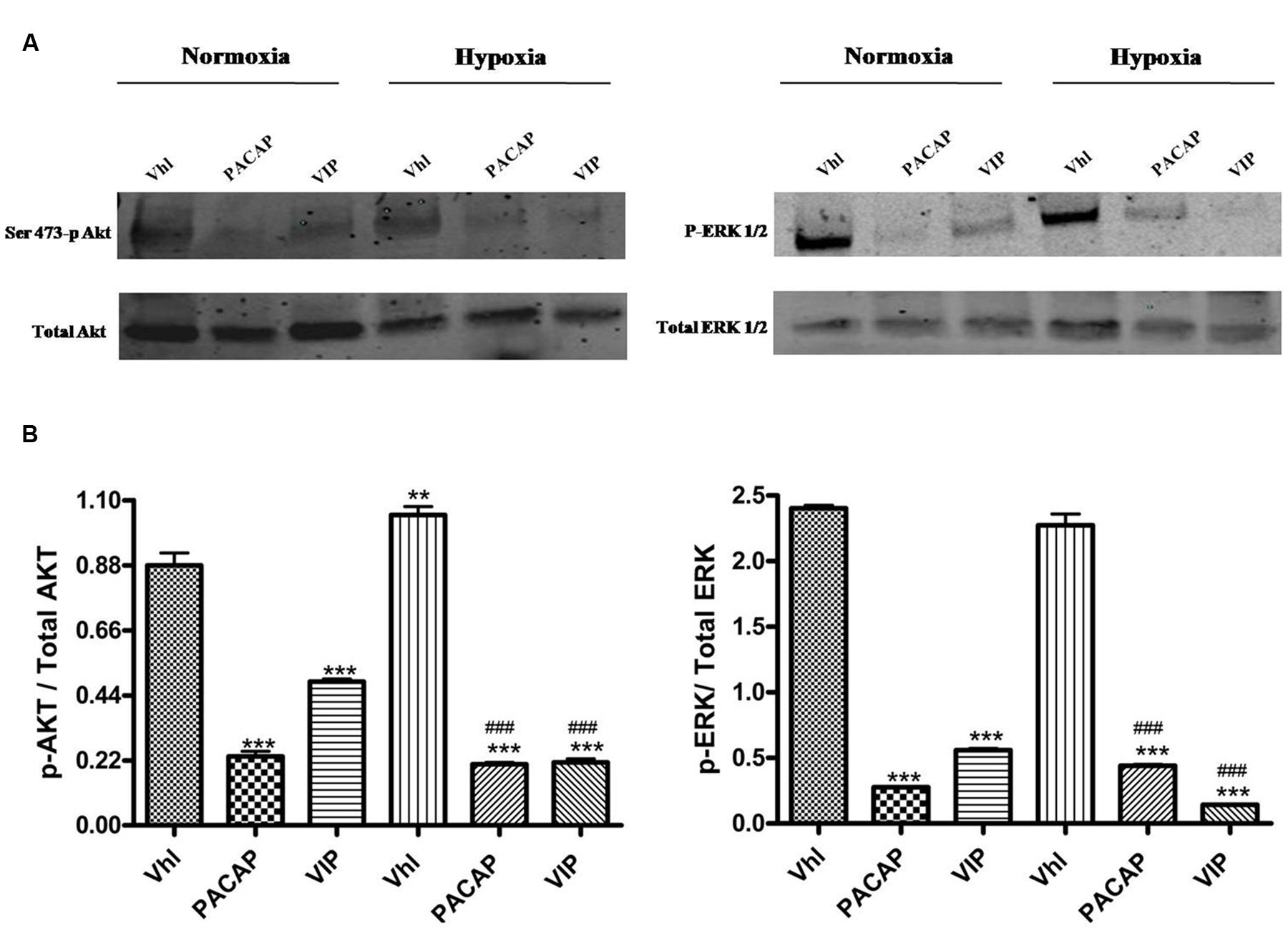
FIGURE 6. Phosphorylation of AKT and ERK1/2 in U87-MG cells under normoxia and hypoxia. (A) Representative immunoblots of Ser473-p Akt or p-Erk1/2 expression on U87MG cells treated with PACAP or VIP under normoxia or hypoxia. (B) The bar graphs show quantitative analysis of signals obtained by immunoblots resulting from three independent experiments. Relative band densities were quantified by using ImageJ software. Each signal of phosphorylated protein was normalized to total protein expression. Data are expressed as mean ± SEM (**p < 0.01, ***p < 0.001 vs. Vhl under normoxia; ###p < 0.001 vs. Vhl under hypoxia as determined by one-way ANOVA followed by the Tukey post hoc test).
Discussion
Pituitary adenylate cyclase-activating polypeptide and VIP are widely expressed in peripheral tissues, CNS and in a wide variety of human tumors, including GBM (Robberecht et al., 1993, 1994; Oka et al., 1998; Reubi et al., 2000; Juarranz et al., 2001; Isobe et al., 2003). They exert various effects by interacting to VIPRs depending on transcript variants express in each cell type. By focusing on cancer, many studies have highlighted their controversial role in the progression of malignancies. In particular, they have shown a prominent growth effect on some common neoplasms, such as lung, gut, prostate and immune system neoplastic diseases (Reubi et al., 2000; Moody et al., 2001; Schulz et al., 2004). However, as we show in this paper, they exert anti-invasive effect, in other tumors, including GBM (Vertongen et al., 1996; Cochaud et al., 2015). During tumorigenesis, hypoxic areas are generated in the neoplastic mass when deregulation in cell proliferation leads to an increase in tissue amount, not supported by an adequate oxygen supply. Hypoxia plays a key role in malignancy not only by stimulating angiogenesis but also by increasing cellular migration. Therefore, in the present study, we evaluated the effect of PACAP and VIP on tumor cell infiltration grown in a hypoxic microenvironment. The present results show that the endogenous expression of PACAP and VIP precursor peptides, and relative VIPRs was increased under hypoxia (Figure 2). Furthermore, these neuropeptides significantly abrogated the hypoxia-enhanced migration of U87MG cells (Figure 3), suggesting that they might play a pivotal role in cellular invasion in GMB hypoxic areas. It is remarkable that HIFs, the main regulators of the transcriptional response to hypoxia, represent one of the distinctive hallmarks in malignancy. Here, we have found that PACAP or VIP treatment, decreases the expression levels of both HIF-1α and HIF-2α. Concomitantly, we have found that their administration have induced a significant reduction in EGFR levels, a biomarker of cell proliferation. The present data, consistently with previous papers, indicate that hypoxia promotes an oncogenic program which results from the translational up-regulation of EGFR, predominantly depending on HIFs levels (Franovic et al., 2007, 2009). This view is also corroborated by the high expression of PACAP, VIP, VIPRs, HIFs, and EGFR in the frozen tumor sample (Figure 1).
Furthermore, we have investigated whether modulation of HIFs and EGFR expression is mediated through VIPRs by treating cells with PAC1 and VPAC1/VPAC2 receptor antagonists, respectively. The results show that PACAP 6–38 treatment highly increased HIFs and EGFR levels, suggesting that hypoxia through activation of endogenous PACAP system may interfere with hypoxia and relative cell proliferation mediated by EGFR (Figure 4). On the other hand, the VIP receptor antagonist was ineffective (Figure 4). This lack of effect might result from a simultaneous block of VPAC1 and VPAC2 receptors, which mediate different functions by activating various pathways, or, more simply, might be due to low specificity of the tested antagonist molecule.
As demonstrated previously, HIFs expression is regulated by PI3K/Akt and MAPK/ERK signaling pathways (Mottet et al., 2002; Lim et al., 2004). In addition, Akt phosphorylation promotes the transformation of anaplastic astrocytoma in GBM, thereby playing a role as oncogenic modulator. In fact, this molecule is involved in cell proliferation by acting on some regulators of cell cycle, apoptosis, and metabolism (Sonoda et al., 2001a,b). MAPK/ERK pathway activation is also involved in tumorigenesis by supporting progression and poor-prognosis of GBM (Kim et al., 2009; Liu et al., 2013).
Our results have confirmed previous data suggesting that the expression of HIFs and consequently EGFR is mediated by activation of both pathways. Indeed, as shown in Figure 5, the pretreatment with a specific PI3K/Akt (wortmannin) or MAPK/ERK (PD98059) pathway inhibitor, strongly decrease HIFs and EGFR levels as compared to own control, in cells grown either in normoxia or exposed to DFX-induced hypoxia. In future, we are planning to deeply investigate the downstream phenotypic effects mediated by EGFR under these experimental conditions.
Thus, we have demonstrated that the anti-invasive effect of PACAP and VIP in GBM cells is mediated through inhibition of these pathways. Indeed, the treatment with these peptides reduces Akt and ERK1/2 phosphorylation which are major targets of PI3K/Akt or MAPK/ERK signaling cascades, respectively (Figure 6).
Apparently, these results are in contrast with other studies showing that PACAP and VIP, through the activation of cAMP/PKA and PI3K signaling pathways, mediated by VIPRs, stimulate a series of transcription factors, which promote proliferation, expression of nuclear oncogenes and growth factors in different cell lines (Whitmarsh and Davis, 1996; Casibang et al., 2001). However, this controversial biological effect might depend by cell or tissue phenotype examined.
In conclusion, these data suggest that, under low oxygen tension, PACAP or VIP reduce cell invasion by acting as negative regulators of HIFs and EGFR through the inhibition of PI3K/Akt and ERK1/2 signaling pathways. Further studies are required to clarify whether PACAP and VIP have the same effects, as observed in the present study, in GBM cells exposed to hypoxia, not chemically induced, but through lowering of oxygen tension (1% O2).
Despite over the last three decades have emerged new treatments of brain tumors, the survival of patients with GBM remains very poor. Therefore, new targeted agents in clinical therapy are needed. Here, we propose that the modulation of PACAP and VIP receptors system in combination with other therapies, might represent a new approach to limit invasion of this devastating tumor.
Conclusion
The modulation of hypoxic event and the anti-invasive effect exerted by some VIP family members might open new insights in the therapeutic approach to GBM.
Author Contributions
VD: Study conception and design; Drafting of manuscript; Critical revision. GM: Study conception and design; Acquisition of data; Analysis and interpretation of data; Drafting of manuscript. AGD: Acquisition of data; Analysis and interpretation of data. RR: Acquisition of data; Analysis and interpretation of data. GM: IHC analysis; Critical revision. SC: Critical revision. SS: Drafting of manuscript; Critical revision.
Conflict of Interest Statement
The authors declare that the research was conducted in the absence of any commercial or financial relationships that could be construed as a potential conflict of interest.
Acknowledgments
These experiments were supported by the international Ph.D. program in Neuroscience, University of Catania, Medical School.
Footnotes
References
Aleppo, G., Pisani, A., Copani, A., Bruno, V., Aronica, E., D’Agata, V., et al. (1992). Metabotropic glutamate receptors and neuronal toxicity. Adv. Exp. Med. Biol. 318, 137–145. doi: 10.1007/978-1-4615-3426-6_12
Barrie, A. P., Clohessy, A. M., Buensuceso, C. S., Rogers, M. V., and Allen, J. M. (1997). Pituitary adenylyl cyclase-activating peptide stimulates extracellular signal-regulated kinase 1 or 2 (ERK1/2) activity in a Ras-independent, mitogen-activated protein Kinase/ERK kinase 1 or 2-dependent manner in PC12 cells. J. Biol. Chem. 272, 19666–19671. doi: 10.1074/jbc.272.32.19666
Blehm, K. N., Spiess, P. E., Bondaruk, J. E., Dujka, M. E., Villares, G. J., Zhao, Y. J., et al. (2006). Mutations within the kinase domain and truncations of the epidermal growth factor receptor are rare events in bladder cancer: implications for therapy. Clin. Cancer Res 12, 4671–4677. doi: 10.1158/1078-0432.CCR-06-0407
Brenneman, D. E. (2007). Neuroprotection: a comparative view of vasoactive intestinal peptide and pituitary adenylate cyclase-activating polypeptide. Peptides 28, 1720–1726. doi: 10.1016/j.peptides.2007.04.002
Canonico, P. L., Copani, A., D’Agata, V., Musco, S., Petralia, S., Travali, S., et al. (1996). Activation of pituitary adenylate cyclase-activating polypeptide receptors prevents apoptotic cell death in cultured cerebellar granule cells. Ann. N. Y. Acad. Sci. 26, 470–472.
Casibang, M., Purdom, S., Jakowlew, S., Neckers, L., Zia, F., Ben-Av, P., et al. (2001). Prostaglandin E2 and vasoactive intestinal peptide increase vascular endothelial cell growth factor mRNAs in lung cancer cells. Lung Cancer 31, 203–212. doi: 10.1016/S0169-5002(00)00168-9
Castorina, A., Giunta, S., Mazzone, V., Cardile, V., and D’Agata, V. (2010a). Effects of PACAP and VIP on hyperglycemia-induced proliferation in murine microvascular endothelial cells. Peptides 31, 2276–2283. doi: 10.1016/j.peptides.2010.08.013
Castorina, A., Giunta, S., Scuderi, S., and D’Agata, V. (2012). Involvement of PACAP/ADNP signaling in the resistance to cell death in malignant peripheral nerve sheath tumor (MPNST) cells. J. Mol. Neurosci. 48, 674–683. doi: 10.1007/s12031-012-9755-z
Castorina, A., Scuderi, S., D’Amico, A. G., Drago, F., and D’Agata, V. (2014). PACAP and VIP increase the expression of myelin-related proteins in rat schwannoma cells: involvement of PAC1/VPAC2 receptor-mediated activation of PI3K/Akt signaling pathways. Exp. Cell Res. 10, 108–121.
Castorina, A., Tiralongo, A., Giunta, S., Carnazza, M. L., Rasi, G., and D’Agata, V. (2008). PACAP and VIP prevent apoptosis in schwannoma cells. Brain Res. 1241, 29–35. doi: 10.1016/j.brainres.2008.09.035
Castorina, A., Tiralongo, A., Giunta, S., Carnazza, M. L., Scapagnini, G., and D’Agata, V. (2010b). Early effects of aluminum chloride on beta-secretase mRNA expression in a neuronal model of beta-amyloid toxicity. Cell Biol. Toxicol. 26, 367–377. doi: 10.1007/s10565-009-9149-3
Cavallaro, S., D’Agata, V., Guardabasso, V., Travali, S., Stivala, F., and Canonico, P. L. (1995). Differentiation induces pituitary adenylate cyclase-activating polypeptide receptor expression in PC-12 cells. Mol. Pharmacol. 48, 56–62.
Chetram, M. A., and Hinton, C. V. (2012). PTEN regulation of ERK1/2 signaling in cancer. J. Recept. Signal. Transduct. Res. 32, 190–195. doi: 10.3109/10799893.2012.695798
Cochaud, S., Meunier, A. C., Monvoisin, A., Bensalma, S., Muller, J. M., and Chadéneau, C. (2015). Neuropeptides of the VIP family inhibit glioblastoma cell invasion. J. Neurooncol. 122, 63–73. doi: 10.1007/s11060-014-1697-6
Couvineau, A., Lacapere, J. J., Tan, Y. V., Rouyer-Fessard, C., Nicole, P., and Laburthe, M. (2003). Identification of cytoplasmic domains of hVPAC1 receptor required for activation of adenylyl cyclase. Crucial role of two charged amino acids strictly conserved in class II G protein-coupled receptors. J. Biol. Chem. 278, 24759–24766. doi: 10.1074/jbc.M301916200
D’Agata, V., and Cavallaro, S. (1998). Functional and molecular expression of PACAP/VIP receptors in the rat retina. Brain Res. Mol. Brain Res. 54, 161–164. doi: 10.1016/S0169-328X(97)00335-5
D’Agata, V., Cavallaro, S., Stivala, F., and Canonico, P. L. (1996). Tissue-specific and developmental expression of pituitary adenylate cyclase-activating polypeptide (PACAP) receptors in rat brain. Euro. J. Neurosci. 8, 310–318. doi: 10.1111/j.1460-9568.1996.tb01215.x
D’Agata, V., Grimaldi, M., Pascale, A., and Cavallaro, S. (2000). Regional and cellular expression of the parkin gene in the rat cerebral cortex. Euro. J. Neurosci. 12, 3583–3588. doi: 10.1046/j.1460-9568.2000.00244.x
D’Amico, A. G., Scuderi, S., Saccone, S., Castorina, A., Drago, F., and D’Agata, V. (2013). Antiproliferative effects of PACAP and VIP in serum-starved glioma cells. J. Mol. Neurosci. 51, 503–513. doi: 10.1007/s12031-013-0076-7
Dejda, A., Jolivel, V., Bourgault, S., Seaborn, T., Fournier, A., Vaudry, H., et al. (2008). Inhibitory effect of PACAP on caspase activity in neuronal apoptosis: a better understanding towards therapeutic applications in neurodegenerative diseases. J. Mol. Neurosci. 36, 26–37. doi: 10.1007/s12031-008-9087-1
Delgado, M., and Ganea, D. (1999). Vasoactive intestinal peptide and pituitary adenylate cyclase-activating polypeptide inhibit interleukin-12 transcription by regulating nuclear factor kappaB and Ets activation. J. Biol. Chem. 274, 31930–31940. doi: 10.1074/jbc.274.45.31930
Dickson, L., Aramori, I., McCulloch, J., Sharkey, J., and Finlayson, K. (2006). A systematic comparison of intracellular cyclic AMP and calcium signalling highlights complexities in human VPAC/PAC receptor pharmacology. Neuropharmacology 51, 1086–1098. doi: 10.1016/j.neuropharm.2006.07.017
Dickson, L., and Finlayson, K. (2009). VPAC and PAC receptors: from ligands to function. Pharmacol. Ther. 121, 294–316. doi: 10.1016/j.pharmthera.2008.11.006
Dunn, G. P., Rinne, M. L., Wykosky, J., Genovese, G., Quayle, S. N., Dunn, I. F., et al. (2012). Emerging insights into the molecular and cellular basis of glioblastoma. Genes Dev. 26, 756–784. doi: 10.1101/gad.187922.112
Epstein, A. C., Gleadle, J. M., McNeill, L. A., Hewitson, K. S., O’Rourke, J., Mole, D. R., et al. (2001). C. elegans EGL-9 and mammalian homologs define a family of dioxygenases that regulate HIF by prolyl hydroxylation. Cell 107, 43–54. doi: 10.1016/S0092-8674(01)00507-4
Franovic, A., Gunaratnam, L., Smith, K., Robert, I., Patten, D., and Lee, S. (2007). Translational up-regulation of the EGFR by tumor hypoxia provides a nonmutational explanation for its overexpression in human cancer. Proc. Natl. Acad. Sci. U.S.A. 7, 13092–13097. doi: 10.1073/pnas.0702387104
Franovic, A., Holterman, C. E., Payette, J., and Lee, S. (2009). Human cancers converge at the HIF-2alpha oncogenic axis. Proc. Natl. Acad. Sci. U.S.A. 106, 21306–21311. doi: 10.1073/pnas.0906432106
Fujiwara, S., Nakagawa, K., Harada, H., Nagato, S., Furukawa, K., Teraoka, M., et al. (2007). Silencing hypoxia-inducible factor-1alpha inhibits cell migration and invasion under hypoxic environment in malignant gliomas. Int. J. Oncol. 30, 793–802.
Giunta, S., Castorina, A., Adorno, A., Mazzone, V., Carnazza, M. L., and D’Agata, V. (2010). PACAP and VIP affect NF1 expression in rat malignant peripheral nerves heath tumor (MPNST) cells. Neuropeptides 44, 45–51. doi: 10.1016/j.npep.2009.10.003
Giunta, S., Castorina, A., Bucolo, C., Magro, G., Drago, F., and D’Agata, V. (2012). Early changes in pituitary adenylate cyclase-activating peptide, vasoactive intestinal peptide and related receptors expression in retina of streptozotocin-induced diabetic rats. Peptides 37, 32–39. doi: 10.1016/j.peptides.2012.06.004
Halatsch, M. E., Schmidt, U., Behnke-Mursch, J., Unterberg, A., and Wirtz, C. R. (2006). Epidermal growth factor receptor inhibition for the treatment of glioblastoma multiforme and other malignant brain tumours. Cancer Treat. Rev. 32, 74–89. doi: 10.1016/j.ctrv.2006.01.003
Harmar, A. J., Fahrenkrug, J., Gozes, I., Laburthe, M., May, V., Pisegna, J. R., et al. (2012). Pharmacology and functions of receptors for vasoactive intestinal peptide and pituitary adenylate cyclaseactivating polypeptide: IUPHAR review 1. Br. J. Pharmacol. 2166, 4–17. doi: 10.1111/j.1476-5381.2012.01871.x
Helczynska, K., Larsson, A. M., Holmquist Mengelbier, L., Bridges, E., Fredlund, E., Borgquist, S., et al. (2008). Hypoxia-inducible factor-2alpha correlates to distant recurrence and poor outcome in invasive breast cancer. Cancer Res. 68, 9212–9220. doi: 10.1158/0008-5472.CAN-08-1135
Hirsilä, M., Koivunen, P., Xu, L., Seeley, T., Kivirikko, K. I., and Myllyharju, J. (2005). Effect of desferrioxamine and metals on the hydroxylases in the oxygen sensing pathway. FASEB J. 19, 1308–1310.
Holmquist-Mengelbier, L., Fredlund, E., Lofstedt, T., Noguera, R., Navarro, S., Nilsson, H., et al. (2006). Recruitment of HIF-1alpha and HIF-2alpha to common target genes is differentially regulated in neuroblastoma: HIF-2alpha promotes an aggressive phenotype. Cancer Cell 10, 413–423. doi: 10.1016/j.ccr.2006.08.026
Isobe, K., Tatsuno, I., Yashiro, T., Nanmoku, T., Takekoshi, K., Kawakami, Y., et al. (2003). Expression of mRNA for PACAP and its receptors in intra- and extra-adrenal human pheochromocytomas and their relationship to catecholamine synthesis. Regul. Pept. 110, 213–217. doi: 10.1016/S0167-0115(02)00209-4
Jaworski, D. M. (2000). Expression of pituitary adenylate cyclase-activating polypeptide (PACAP) and the PACAP-selective receptor in cultured rat astrocytes, human brain tumors, and in response to acute intracranial injury. Cell Tissue Res. 300, 219–230. doi: 10.1007/s004410000184
Juarranz, M. G., Bolaños, O., Gutiérrez-Cañas, I., Lerner, E. A., Robberecht, P., Carmena, M. J., et al. (2001). Neuroendocrine differentiation of the LNCaP prostate cancer cell line maintains the expression and function of VIP and PACAP receptors. Cell. Signal. 13, 887–894. doi: 10.1016/S0898-6568(01)00199-1
Kaur, B., Khwaja, F. W., Severson, E. A., Matheny, S. L., Brat, D. J., and Van Meir, E. G. (2005). Hypoxia and the hypoxia-inducible-factor pathway in glioma growth and angiogenesis. Neuro Oncol. 7, 134–153. doi: 10.1215/S1152851704001115
Kim, J. Y., Kim, Y. J., Lee, S., and Park, J. H. (2009). The critical role of ERK in death resistance and invasiveness of hypoxia-selected glioblastoma cells. BMC Cancer 9:27. doi: 10.1186/1471-2407-9-27
Koh, M. Y., Lemos, R. Jr., Liu, X., and Powis, G. (2011). The hypoxia-associated factor switches cells from HIF-1a to HIF-2a-dependent signaling promoting stem cell characteristics, aggressive tumor growth and invasion. Cancer Res. 71, 4015–4027. doi: 10.1158/0008-5472.CAN-10-4142
Koh, M. Y., and Powis, G. (2012). Passing the baton: the HIF switch. Trends Biochem. Sci. 37, 364–372. doi: 10.1016/j.tibs.2012.06.004
Koul, D. (2008). PTEN signaling pathways in glioblastoma. Cancer Biol. Ther. 7, 1321–1325. doi: 10.4161/cbt.7.9.6954
Laburthe, M., Couvineau, A., and Marie, J. C. (2002). VPAC receptors for VIP and PACAP. Recept Chann. 8, 137–153. doi: 10.3109/10606820213680
Lelièvre, V., Pineau, N., Du, J., Wen, C. H., Nguyen, T., Janet, T., et al. (1998). Differential effects of peptide histidine isoleucine (PHI) and related peptides on stimulation and suppression of neuroblastoma cell proliferation. A novel VIP-independent action of PHI via MAP kinase. J. Biol. Chem. 273, 19685–19690. doi: 10.1074/jbc.273.31.19685
Li, J., Yen, C., Liaw, D., Podsypanina, K., Bose, S., Wang, S. I., et al. (1997). PTEN, a putative protein tyrosine phosphatase gene mutated in human brain, breast, and prostate cancer. Science 275, 1943–1947. doi: 10.1126/science.275.5308.1943
Lim, J. H., Lee, E. S., You, H. J., Lee, J. W., Park, J. W., and Chun, Y. S. (2004). Ras-dependent induction of HIF-1alpha785 via the Raf/MEK/ERK pathway: a novel mechanism of Ras-mediated tumor promotion. Oncogene 23, 9427–9431. doi: 10.1038/sj.onc.1208003
Liu, T., Ren, D., Zhu, X., Yin, Z., Jin, G., Zhao, Z., et al. (2013). Transcriptional signaling pathways inversely regulated in Alzheimer’s disease and glioblastoma multiform. Sci. Rep. 10:3467. doi: 10.1038/srep03467
Magro, G., Cataldo, I., Amico, P., Torrisi, A., Vecchio, G. M., Parenti, R., et al. (2011). Aberrant expression of TfR1/CD71 in thyroid carcinomas identifies a novel potential diagnostic marker and therapeutic target. Thyroid 21, 267–277. doi: 10.1089/thy.2010.0173
Maugeri, G., D’Amico, A. G., Magro, G., Salvatorelli, L., Barbagallo, G. M., Saccone, S., et al. (2015). Expression profile of parkin isoforms in human gliomas. Int. J. Oncol. 47, 1282–1292. doi: 10.3892/ijo.2015.3105
Maugeri, G., D’Amico, A. G., Reitano, R., Saccone, S., Federico, C., Cavallaro, S., et al. (2016). Parkin modulates expression of HIF-1α and HIF-3α during hypoxia in gliobastoma-derived cell lines in vitro. Cell Tissue Res. doi: 10.1007/s00441-015-2340-3 [Epub ahead of print].
Maynard, M. A., and Ohh, M. (2004). Von Hippel-Lindau tumor suppressor protein and hypoxia-inducible factor in kidney cancer. Am. J. Nephrol. 24, 1–13. doi: 10.1159/000075346
Moody, T. W., Leyton, J., Chan, D., Brenneman, D. C., Fridkin, M., Gelber, E., et al. (2001). VIP receptor antagonists potentiate the action of chemotherapeutic drugs on breast cancer cells. Breast Cancer Res. Treat. 68, 55–64. doi: 10.1023/A:1017994722130
Mottet, D., Michel, G., Renard, P., Ninane, N., Raes, M., and Michiels, C. (2002). ERK and calcium in activation of HIF-1. Ann. N. Y. Acad. Sci. 973, 448–453. doi: 10.1111/j.1749-6632.2002.tb04681.x
Nakada, M., Nakada, S., Demuth, T., Tran, N. L., Hoelzinger, D. B., and Berens, M. E. (2007). Molecular targets of glioma invasion. Cell Mol Life Sci. 64, 458–478. doi: 10.1007/s00018-007-6342-5
Oka, H., Jin, L., Reubi, J. C., Qian, X., Scheithauer, B. W., Fujii, K., et al. (1998). Pituitary adenylate-cyclase–activating polypeptide (PACAP) binding sites and PACAP/vasoactive intestinal polypeptide receptor expression in human pituitary adenomas. Am. J. Pathol. 153, 1787–1796. doi: 10.1016/S0002-9440(10)65693-3
Park, J. H., Lee, J. Y., Shin, D. H., Jang, K. S., Kim, H. J., and Kong, G. (2011). Loss of Mel-18 induces tumor angiogenesis through enhancing the activity and expression of HIF-1alpha mediated by the PTEN/PI3K/Akt pathway. Oncogene 10, 4578–4589. doi: 10.1038/onc.2011.174
Qing, G., and Simon, M. C. (2009). Hypoxia inducible factor- 2alpha: a critical mediator of aggressive tumor phenotypes. Curr. Opin. Genet. Dev. 19, 60–66. doi: 10.1016/j.gde.2008.12.001
Reglodi, D., Kiss, P., Lubics, A., and Tamas, A. (2011). Review on the protective effects of PACAP in models of neurodegenerative diseases in vitro and in vivo. Curr. Pharm. Des. 17, 962–972. doi: 10.2174/138161211795589355
Reubi, J. C., Läderach, U., Waser, B., Gebbers, J. O., Robberecht, P., and Laissue, J. A. (2000). Vasoactive intestinal peptide/pituitary adenylate cyclase-activating peptide receptor subtypes in human tumors and their tissues of origin. Cancer Res. 60, 3105–3112.
Robberecht, P., Vertongen, P., Velkeniers, B., de Neef, P., Vergani, P., Raftopoulos, C., et al. (1993). Receptors for pituitary adenylate cyclase activating peptides in human pituitary adenomas. J. Clin. Endocrinol. Metab. 77, 1235–1239. doi: 10.1210/jc.77.5.1235
Robberecht, P., Woussen-Colle, M. C., Vertongen, P., De Neef, P., Hou, X., Salmon, I., et al. (1994). Expression of pituitary adenylate cyclase activating polypeptide (PACAP) receptors in human glial cell tumors. Peptides 15, 661–665. doi: 10.1016/0196-9781(94)90092-2
Schulz, S., Röcken, C., Mawrin, C., Weise, W., Höllt, V., and Schulz, S. (2004). Immunocytochemical identification of VPAC1, VPAC2, and PAC1 receptors in normal and neoplastic human tissues with subtype-specific antibodies. Clin. Cancer Res. 10, 8235–8242. doi: 10.1158/1078-0432.CCR-04-0939
Seaborn, T., Masmoudi-Kouli, O., Fournier, A., Vaudry, H., and Vaudry, D. (2011). Protective effects of pituitary adenylate cyclase-activating polypeptide (PACAP) against apoptosis. Curr. Pharm. Des. 17, 204–214. doi: 10.2174/138161211795049679
Semenza, G. (1999a). Perspectives on oxygen sensing. Cell 98, 281–284. doi: 10.1016/S0092-8674(00)81957-1
Semenza, G. (1999b). Regulation of mammalian O2 homeostasis by hypoxia-inducible factor 1. Annu. Rev. Cell Dev. Biol. 15, 551–578. doi: 10.1146/annurev.cellbio.15.1.551
Sherwood, N. M., Krueckl, S. L., and McRory, J. E. (2000). The origin and function of the pituitary adenylate cyclase-activating polypeptide (PACAP)/glucagon superfamily. Endocr. Rev. 21, 619–670. doi: 10.1210/er.21.6.619
Song, M. S., Salmena, L., and Pandolfi, P. P. (2012). The functions and regulation of the PTEN tumour suppressor. Nat. Rev. Mol. Cell Biol. 13, 283–296. doi: 10.1038/nrm3330
Sonoda, Y., Ozawa, T., Aldape, K. D., Deen, D. F., Berger, M. S., and Pieper, R. O. (2001a). Akt pathway activation converts anaplastic astrocytoma to glioblastoma multiforme in a human astrocyte model of glioma. Cancer Res. 61, 6674–6678.
Sonoda, Y., Ozawa, T., Hirose, Y., Aldape, K. D., McMahon, M., Berger, M. S., et al. (2001b). Formation of intracranial tumors by genetically modified human astrocytes defines four pathways critical in the development of human anaplastic astrocytoma. Cancer Res. 61, 4956–4960.
Tang, B., Yong, X., Xie, R., Li, Q. W., and Yang, S. M. (2014). Vasoactive intestinal peptide receptor-based imaging and treatment of tumors. Int. J. Oncol. 44, 1023–1031. doi: 10.3892/ijo.2014.2276
Tian, H., McKnight, S. L., and Russell, D. W. (1997). Endothelial PAS domain protein 1 (EPAS1), a transcription factor selectively expressed in endothelial cells. Genes Dev. 11, 72–82. doi: 10.1101/gad.11.1.72
Vaudry, D., Falluel-Morel, A., Bourgault, S., Basille, M., Burel, D., Wurtz, O., et al. (2009). Pituitary adenylate cyclase-activating polypeptide and its receptors: 20 years after the discovery. Pharmacol. Rev. 61, 283–357. doi: 10.1124/pr.109.001370
Vaudry, D., Gonzalez, B. J., Basille, M., Yon, L., Fournier, A., and Vaudry, H. (2000). Pituitary adenylate cyclase-activating polypeptide and its receptors: from structure to functions. Pharmacol. Rev. 52, 269–324.
Vertongen, P., Camby, I., Darro, F., Kiss, R., and Robberech, P. (1996). VIP and pituitary adenylate cyclase activating polypeptide (PACAP) have an antiproliferative effect on the T98G human glioblastoma cell line through interaction with VIP 2 receptor. Neuropeptides 30, 491–496. doi: 10.1016/S0143-4179(96)90015-3
Waschek, J. A. (2013). VIP and PACAP: neuropeptide modulators of CNS inflammation, injury, and repair. Br. J. Pharmacol. 169, 512–523. doi: 10.1111/bph.12181
Wen, P. Y., and Kesari, S. (2008). Malignant gliomas in adults. N. Engl. J. Med. 359, 492–507. doi: 10.1056/NEJMra0708126
Whitmarsh, A. J., and Davis, R. J. (1996). Transcription factor AP-1 regulation by mitogen-activated protein kinase signal transduction pathways. J. Mol. Med. 74, 589–607. doi: 10.1007/s001090050063
Zagzag, D., Lukyanov, Y., Lan, L., Ali, M. A., Esencay, M., Mendez, O., et al. (2006). Hypoxia-inducible factor 1 and VEGF upregulate CXCR4 in glioblastoma: implications for angiogenesis and glioma cell invasion. Lab. Invest. 86, 1221–1232. doi: 10.1038/labinvest.3700482
Keywords: PACAP, VIP, glioblastoma multiforme invasiveness, hypoxia, HIFs, EGFR
Citation: Maugeri G, D’Amico AG, Reitano R, Magro G, Cavallaro S, Salomone S and D’Agata V (2016) PACAP and VIP Inhibit the Invasiveness of Glioblastoma Cells Exposed to Hypoxia through the Regulation of HIFs and EGFR Expression. Front. Pharmacol. 7:139. doi: 10.3389/fphar.2016.00139
Received: 16 March 2016; Accepted: 12 May 2016;
Published: 31 May 2016.
Edited by:
Cesare Mancuso, Catholic University Medical School, Rome, ItalyReviewed by:
Eileen J. Kennedy, University of Georgia, USAKazuhiro Takahashi, Tohoku University Graduate School of Medicine, Japan
Copyright © 2016 Maugeri, D’Amico, Reitano, Magro, Cavallaro, Salomone and D’Agata. This is an open-access article distributed under the terms of the Creative Commons Attribution License (CC BY). The use, distribution or reproduction in other forums is permitted, provided the original author(s) or licensor are credited and that the original publication in this journal is cited, in accordance with accepted academic practice. No use, distribution or reproduction is permitted which does not comply with these terms.
*Correspondence: Velia D’Agata, vdagata@unict.it