- Research Centre for Plant Growth and Development, School of Life Sciences, University of KwaZulu-Natal, Pietermaritzburg, South Africa
The study investigated the antibacterial activity of crude extracts of C. edwardsii, Combretum krausii, and Maytenus nemorosa as well as their interactions with selected antibiotics against drug resistant bacterial strains. Using the rapid p-iodonitrotetrazolium chloride colorimetric assay, minimum inhibitory concentration values of plant extracts and antibiotics were determined. The interactions of plant extracts and antibiotics were studied using a checkerboard method. The MICs of the plant extracts and antibiotics were in the range of 0.037–6.25 and 0.001–2.5 mg/ml, respectively. The plant fractions tested in the present study displayed varying levels of antibacterial activity depending on the bacterial strains. Generally, Staphylococcus aureus was the most susceptible of the three strains of bacteria while the other two beta-lactamase producing Gram-negative bacteria were the most resistant. The hexane leaf extract of M. nemorosa was the most active (MIC = 37 μg/ml) against S. aureus. Ethyl acetate leaf extract of C. krausii was the most active against Klebsiella pneumoniae and ethyl acetate leaf extract of C. edwardsii was the most active against Escherichia coli. Synergistic interactions were detected in 13% of the combinations against E. coli, 27% of the combinations against K. pneumoniae and 80% of the combinations against S. aureus. The few synergistic interactions observed in the present study suggest that the crude extracts of the leaves of M. nemorosa, C. edwardsii, and C. krausii could be potential sources of broad spectrum antibiotic resistance modifying compounds.
Introduction
The emergence and spread of drug-resistant bacteria remains a major challenge to public health in the treatment of bacterial infections. Due to this resistance, the clinical efficacy of current antimicrobial agents is decreasing against many pathogens. Some of the commonly used antibiotics, especially beta-lactam antibiotics are rendered infective through some resistance mechanisms employed by drug-resistant bacteria. One of such resistance mechanisms is the hydrolysis of the active site, beta lactam ring, of beta-lactam antibiotics by beta-lactamases, and thereby rendering the antibiotic ineffective. Beta-lactamases are classified into four different classes (A, B, C, and D) based on structural comparisons or four groups (1–4) based on hydrolytic and inhibitor profiles (Ambler et al., 1991; Bush and Jacoby, 2010). Class A, C, and D beta-lactamases use a serine as a nucleophile to hydrolyze the beta-lactam bond while class B beta-lactamases (carbapenemases) use Zn2+ to deactivate beta-lactams. Beta-lactamases are the most significant and prevalent mechanism of resistance to beta-lactams. Members of the family Enterobacteriaceae commonly express plasmid-encoded beta-lactamases (TEM and SHV) which confer resistance to penicillins but not to expanded-spectrum cephalosporins. TEM-1 (class A) is the most commonly encountered beta-lactamase in Gram-negative bacteria. Up to 90% of ampicillin resistance in E. coli is due to the production of TEM-1 (Cooksey et al., 1990) while the most common class A beta-lactamases found in Klebsiella are the chromosomal and plasmid-borne SHV enzymes and the plasmid-mediated TEM enzymes (Bush, 2001). Combination therapy has over the years become one of the most effective strategies in combating bacterial infections caused by drug resistant pathogens. The rationale is to enhance the activity by achievement of a synergistic effect. According to Rasoanaivo et al. (2011), “synergy” or “potentiation” means that the effect of the combination is greater than the sum of the individual effects. Synergistic effects manifest in different ways: improving bioavailability; decreasing metabolism, and excretion of the active component; reversal of resistance; and modulation of adverse effects (Wagner and Ulrich-Merzenich, 2009; Rasoanaivo et al., 2011). Combination therapy is becoming a theme of infectious diseases and is increasingly being accepted as a reducer of microbial resistance.
Medicinal plants are known to be very rich in phytochemicals with diverse biological activities. Researchers have shown that co-occurring compounds in medicinal plants play a role in enhancing the bioavailability and distribution of various phytochemicals, and reversal of resistance (Butterweck et al., 2003; Rath et al., 2004).
Maytenus nemorosa (Eckl. & Zeyh.) Marais [Syn: Gymnosporia nemorosa (Eckl. & Zeyh.) Szyszyl.], (Celastraceae), is a spiny evergreen shrub or small tree with drooping branches growing up to 5 m tall. It grows on forest edges in Mpumalanga, Swaziland, and KwaZulu-Natal, Eastern, and Western Cape. As far as we know, there is no information on the use of M. nemorosa in traditional medicine. However, some members of the genus, notably Maytenus senegalensis and Maytenus acuminate are used to treat a wide range of ailments such as pneumonia, tuberculosis, venereal diseases, epilepsy, diarrhea, sore throats and stomach ailments (Pooley, 1993).
The genus Combretum, a member of the plant family Combretaceae are found mainly in tropical and subtropical areas of Africa and Asia. Some members of the genus are used in traditional medicine to treat a wide range of ailments that include inflammation, infections, diabetes, malaria, bleeding and diarrhea. Combretum krausii Hochst, commonly known as the forest bush willow, is a medium-sized to large tree found in Eastern South Africa, Swaziland and Southern Mozambique (Van Wyk, 1997; Le Roux and Reynolds, 2003). It is used as local medicine as antiseptic, antidiuretic, tonic and appetite stimulant, to treat wounds, and eye infections (Quattrocchi, 2012). As far as we know there is no record of ethnopharmacological use of Combretum edwardsii Exell. Limited information exists on the biological activities of both C. edwardsii and C. krausii. Antiinflammatory and antioxidant activites were reported for both Combretum species (McGaw et al., 2001; Masoko and Eloff, 2007). A derivative of mollic acid; 1α-hydroxycycloartenoid mollic acid α-l-arabinoside was isolated from C. edwardsii (Rogers, 1989) while uteroactive compounds; combretastatin, allegic acid and their derivatives were reported for C. krausii (Brookes et al., 1999).
In line with the ethnobotanical use of C. edwardsii, C. krausii, M. nemorosa, the present work was designed to evaluate the antibacterial activities of C. edwardsii, C. krausii, M. nemorosa as well as their interactions with selected antibiotics against drug-resistant bacterial strains.
Materials and Methods
Plant Collection
The plant materials used in the present study were leaves of M. nemorosa (Eckl. & Zeyh.) Marais, C. edwardsii Exell. and C. krausii Hochst. They were collected in January, 2015 from the Botanical Garden of the University of KwaZulu-Natal, Pietermaritzburg, South Africa. The plants were appropriately identified by the Curator of the garden and voucher specimens (C. edwardsii—Chukwujekwu #8 NU; C. krausii—Chukwujekwu #9 NU; M. nemorosa—Chukwujekwu #10 NU) were deposited in the Herbarium of the University of KwaZulu-Natal, Pietermaritzburg.
Preparation of Plant Extracts
Plant materials were dried at 50°C (in the dark), powdered and stored in paper containers at ambient temperature for < 24 h prior to extraction. The oven-dried powdered leaves (30 g of each plant) were extracted with 80% methanol (300 ml) with sonication for 1 h and then soaked overnight. The extracts were filtered through a Büchner funnel using Whatman No.1 filter paper, and the solvent evaporated under reduced pressure at 30°C. Liquid–liquid partitioning was done by dissolving the crude extracts (M. nemorosa = 954 mg; C. edwardsii = 1070 mg; C. krausii = 983 mg) in aqueous methanol (250 ml, 80% v/v) followed by extraction with hexane three times (3 × 300 ml) in a separating funnel. The hexane layers were combined and dried under reduced pressure, and the remaining aqueous layer was concentrated by evaporation of the methanol (MeOH) under reduced pressure and then diluted with distilled water to a volume of 300 ml. The aqueous residues were extracted three times with dichloromethane (3 × 300ml) in a separating funnel. The dichloromethane layers were combined and dried under reduced pressure. The aqueous layer was concentrated and diluted as described earlier and then extracted with ethyl acetate. Finally, the aqueous fraction was first concentrated under reduced pressure and subsequently freeze dried. The four fractions (hexane, dichloromethane, ethyl acetate, and aqueous) were used in the present study.
Chemicals for Antimicrobial Assays
P-Iodonitrotetrazolium chloride (INT; Sigma-Aldrich) was used as microbial growth indicator and Chloramphenicol (CHL), Ampicillin (AMP), Amoxicillin (AMX), Penicillin (PEN), Cefotaxime (CEF; Sigma-Aldrich) were used as reference antibiotics.
Preparation of Microorganisms
The bacteria used in this study were Klebsiella pneumoniae ATCC 700603, Escherichia coli ATCC 25218, Staphylococcus aureus ATCC 11632. The cultures of bacteria were maintained on Mueller Hinton Agar (MHA) slants at 4°C throughout the study and used as stock cultures.
Determination of Minimum Inhibitory Concentration (MIC)
The MIC values of plant extracts and antibiotics against the bacterial strains were determined using a rapid p-iodonitrotetrazolium chloride (INT) colorimetric assay (Eloff, 1998). Stock solutions of plant extracts (100 mg/ml) and antibiotic (10 mg/ml) were prepared with absolute ethanol and sterilized distilled water, respectively. Prior to the assay, stock solutions (plant extracts) were subsequently diluted with sterilized distilled water to a concentration of 12.5 mg/ml. These (plant extracts and antibiotics) were then added to Mueller Hinton Broth (MHB), and serially diluted 2-fold in a 96-well-microplate to a final concentration range of 3120–24 μg/ml for plant extracts and 2500–0.61 μg/ml for antibiotics. Bacterial strains were cultured overnight at 37°C on MHB and adjusted to a final density of 106 cfu/ml with MHB. These were subsequently used as inocula. One hundred microliters (100 μl) of inoculum was added to each well. The plates were covered with a sterile plate sealer and then incubated at 37°C for 20 h. Wells containing 20% aqueous ethanol, MHB and 100 μl of inoculum served as the negative controls. The total volume in each well was 200 μl. The MICs of samples were observed after 20 h incubation at 37°C, and subsequent 30 min incubation after the addition 40 μl of 0.2 mg/ml INT. Clear wells with INT after incubation indicate inhibition of bacterial growth. Minimum inhibitory concentration (MIC) values were recorded as the lowest concentration of the sample that completely inhibited bacterial growth.
Determination of In vitro Synergistic Activity
Combinations of the plant extracts and antibiotics were tested by the checkerboard method. For each plant extract and antibiotics combination, fifty microliters of MHB was added in each well of a 96-well-microplate. Fifty microliters of each plant extract was added in row A and was 2-fold serially diluted down to row H. Fifty microliters of appropriate dilutions of antibiotics was added in columns with column one having the highest concentration and column eight the lowest concentration of antibiotics. To the 100 μl of different combinations in each of the 96-well-microplates, 100 μl of bacterial inoculum as described earlier, was added. The final concentration of plant extract and antibiotics in combinations ranged from 1/64 times the MIC (1/64 × MIC) to 2 × MIC. Further, dilutions were made where the lowest concentrations of the plant extracts and antibiotics in combination inhibited the growth of the test organism. Plates were incubated for 20 h. Interpretation of the data was achieved by calculating the fractional inhibitory concentration index (FICI) as follows:
FIC A + FIC B.
FIC A = (MIC of sample A in combination with antibiotics/MIC of sample A alone).
FIC B = (MIC of antibiotics A in combination with sample/MIC of antibiotics A alone).
The results were interpreted as follows: FICI ≤ 0.5, synergistic; 0.5 < FICI < 4, no interaction; FICI ≥ 4 antagonistic (Odds, 2003).
Results and Discussion
The MICs of the plant extract fractions and standard antibiotics are shown in Table 1. The MICs of the fractions and standard antibiotics were in the range of 37–6250 and 1–2500 μg/ml, respectively. The plant fractions tested in the present study displayed varying levels of antibacterial activity depending on the bacterial strains (Table 1). Generally, S. aureus was the most susceptible of the three strains of bacteria while the other two beta-lactamase producing Gram-negative bacteria were the most resistant. The antibacterial activity of a plant extract is considered significant when its MIC value is below 100 μg/ml, moderate when ≤ 625 μg/ml and weak when it is above 625 μg/ml (Rios and Recio, 2005; Kuete, 2010). All the fractions of M. nemorosa, except the water fraction, showed the highest activity against S. aureus, the hexane fraction being the most active. The antibacterial activities of hexane, dichloromethane and ethyl acetate fractions of M. nemorosa against S. aureus could be considered significant. No information on the antibacterial activity of M. nemorosa and its phytochemical constituents in the literature. However, many species of Maytenus are known for their antibacterial activities and antibacterial compounds have also been isolated and identified from many of the species (Matu and van Staden, 2003; Lindsey et al., 2006; De León et al., 2010). The antibacterial activities of the two Combretum species also vary depending on the test organism. With respect to the Combretum fractions, the dichloromethane fraction of C. edwardsii displayed the best antibacterial activity (MIC = 0.195 μg/ml) against S. aureus (Table 1) while its ethyl acetate fraction showed the best activity (MIC = 390 μg/ml) against E. coli. All the fractions of C. edwardsii displayed relatively weak activity against K. pneumoniae (Table 1) whereas those of C. Krausii, except the water fraction, showed moderate antibacterial activity against S. aureus and weak activity against E. coli and K. pneumoniae (Table 1). Little or no information exist on the antibacterial activity and phytochemical constituents of C. edwardsii and C. krausii. Nonetheless, members of the genus Combretum have been extensively investigated for their antibacterial activity (Elegami et al., 2002; Fyhrquist et al., 2002; Eloff et al., 2005; Fankam et al., 2015). Antibacterial compounds have been isolated and identified within this genus. Martini et al. (2004) isolated and characterized five antibacterial flavonoids from C. erythrophyllum. Other antibacterial phytochemicals from Combretum species include stilbenoids, and triterpenoids (Angeh et al., 2007; Katerere et al., 2012). This is the first report of antibacterial activity of C. edwardsii. C. krausii has previously been investigated for antibacterial activity (Eldeen et al., 2005), but not against drug-resistant bacteria. The MICs of the standard antibiotics vary. Following the trends of antimicrobial activity pattern displayed by the plant extracts fractions; the standard antibiotics were more active against S. aureus than E. coli and K. pneumoniae. The duo Gram-negative bacteria showed strong resistance to ampicillin, amoxicillin, and penicillin. The results showed the multi-drug resistant status of the two Gram-negative bacteria. They were less resistant to cefotaxime and chloramphenicol. Cefotaxime was the most active of all the standard antibiotics used in the present study. The weak antibacterial activities exhibited by the fractions and some of the antibiotics against the two Gram-negative bacteria could be due to the multidrug-resistant nature of the bacteria. These are Gram-negative drug resistant bacteria that exhibit different mechanisms of resistance to different antibiotics. Unlike Gram-positive bacteria, the peptidoglycan layer of Gram-negative bacteria is surrounded by a second membrane comprised of a bilayer of phospholipids and lipopolysaccharide known as the outer membrane. This provides an extra layer of protection for the cell as compared to Gram-positive bacteria. It plays a vital role in preventing the diffusion of many antibiotics into the cell thereby preventing the drugs from reaching their intercellular targets to confer antibiotic activity (Worthington and Melander, 2013). The Gram-negative bacteria used in the present study are also beta-lactamase producing bacteria. These enzymes are located in the bacterial cell wall and they play a significant role in conferring antibacterial resistance on the bacterial cells by hydrolyzing many classes of antibiotics especially the beta-lactams that target cell wall synthesis (Worthington and Melander, 2013). The penicillin, ampicillin, and amoxicillin used in the present study are all beta-lactam antibiotics and they inhibit the synthesis of bacterial cell wall. The very weak antibacterial activities of penicillin, ampicillin, and amoxicillin observed in the present study confirmed the multi-drug resistant profile of the two Gram-negative bacteria. The Gram-negative bacteria displayed more resistant to beta-lactam antibiotics than the rest of the antibiotics. Cefotaxime is a third generation of Cephalosporins which targets the synthesis of bacterial cell wall while chloramphenicol targets the synthesis of protein within the bacterial cell. They both displayed better antibacterial activity than ampicillin, amoxicillin, and penicillin. This could be attributed to the fact that they are not beta-lactam antibiotics and hence do not get hydrolyzed by beta-lactamases.
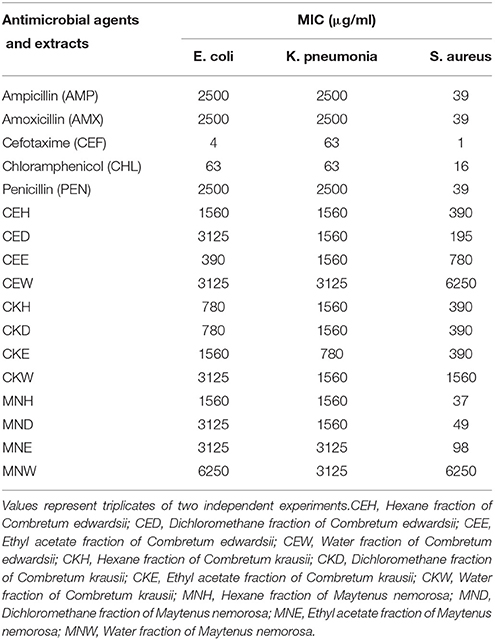
Table 1. MICs of different fractions of Combretum edwardsii (CE), Combretum krausii (CK), and Maytenus nemorosa (MN) and antimicrobial agents against test organisms.
Synergistic activities resulting from the combinations of plant extracts with antibiotics are well known in the literature (Hübsch et al., 2014; Sahu et al., 2014). In the present study, we explored the possible synergistic effects of combinations of fractions of C. krausii, C. edwardsii, and M. nemorosa with selected antibiotics, respectively, against drug resistant pathogenic bacterial strains. The MICs obtained by the combinations of leaf extract fractions of C. edwardsii, C. krausii, and M. nemorosa with ampicillin, amoxicillin, cefotaxime, chloramphenicol, and penicillin, respectively, against E. coli, K. pneumonia, and S. aureus are presented in Tables 2–4. Plant secondary metabolites are known to possess antimicrobial activity. However, when used in combination, they possess the potential to either inhibit the modified target or exhibit a synergy by blocking one or more of the targets in the metabolic pathway thus acting as a modifier of multidrug resistance mechanisms (Hemaiswarya et al., 2008). The E. coli used in the present study is a Tem-1 beta-lactamase-producing strain (Non-ESBL). There were four synergistic (FICI ≤ 0.5) activities detected out of 30 combinations against E. coli (Table 2). All the synergistic activities were combinations with cefotaxime. There were 8–16-fold decreases in the MIC of cefotaxime in all the synergistic activities detected against E. coli. Similarly, the MICs of hexane fraction of C. edwardsii, dichloromethane, and ethyl acetate fractions of C. krausii and hexane fraction of M. nemorosa in combinations with cefotaxime, respectively, that produced synergistic activities, were lowered between 1/130 and 1/260. The best synergistic interaction (FICI = 0.064) was the combination between ethyl acetate fraction of C. krausii leaf extract and cefotaxime, followed by hexane fraction of C. edwardsii and cefotaxime (0.07), dichloromethane fraction of C. krausii and cefotaxime (0.07), and hexane fraction of M. nemorosa and cefotaxime (0.19).
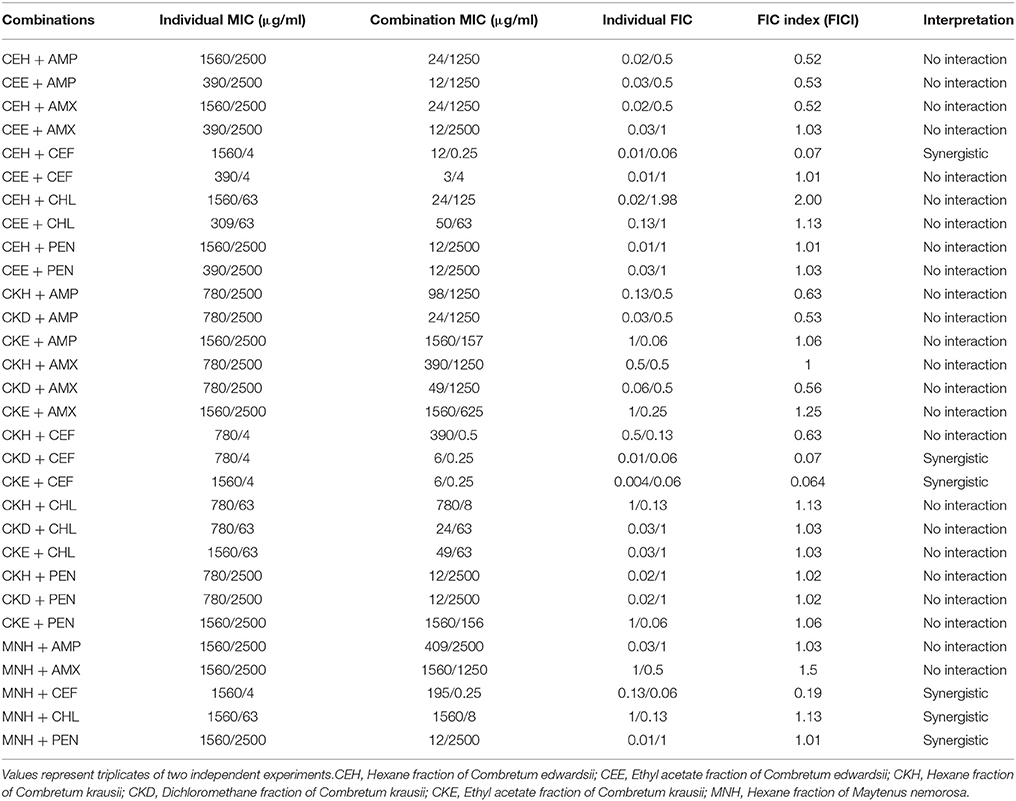
Table 2. Combined effects of different fractions of Combretum edwardsii, Combretum krausii, and Maytenus nemorosa with antibiotics against drug-resistant Escherichia coli.
A multidrug resistant strain of K. pneumoniae was used in the present study. It is an extended-spectrum beta-lactamase strain that produces the enzyme SHV-18. Against K. pneumoniae, 12 synergistic effects were detected out of 45 combinations (Table 3). The combinations of plant extracts with cefotaxime produced synergistic effects. The best being the combinations of ethyl acetate fraction of C. edwardsii and cefotaxime (FICI = 0.03), hexane fraction of C. edwardsii and cefotaxime (FICI = 0.06), dichloromethane fraction of C. krausii and cefotaxime (FICI = 0.06), and hexane fraction of C. krausii and cefotaxime (FICI = 0.09). In the combination of ethyl acetate fraction of C. edwardsii and cefotaxime, the MICs of cefotaxime and the plant extract were lowered from 63 to 2 μg/ml (1/32 of MIC) and from 1560 to 3 μg/ml (1/520 of MIC), respectively. Synergistic effects were also observed in the combinations of chloramphenicol with hexane fraction of M. nemorosa (FICI = 0.14), dichloromethane fraction of M. nemorosa (FICI = 0.38), and hexane fraction of C. krausii (FICI = 0.38), respectively.
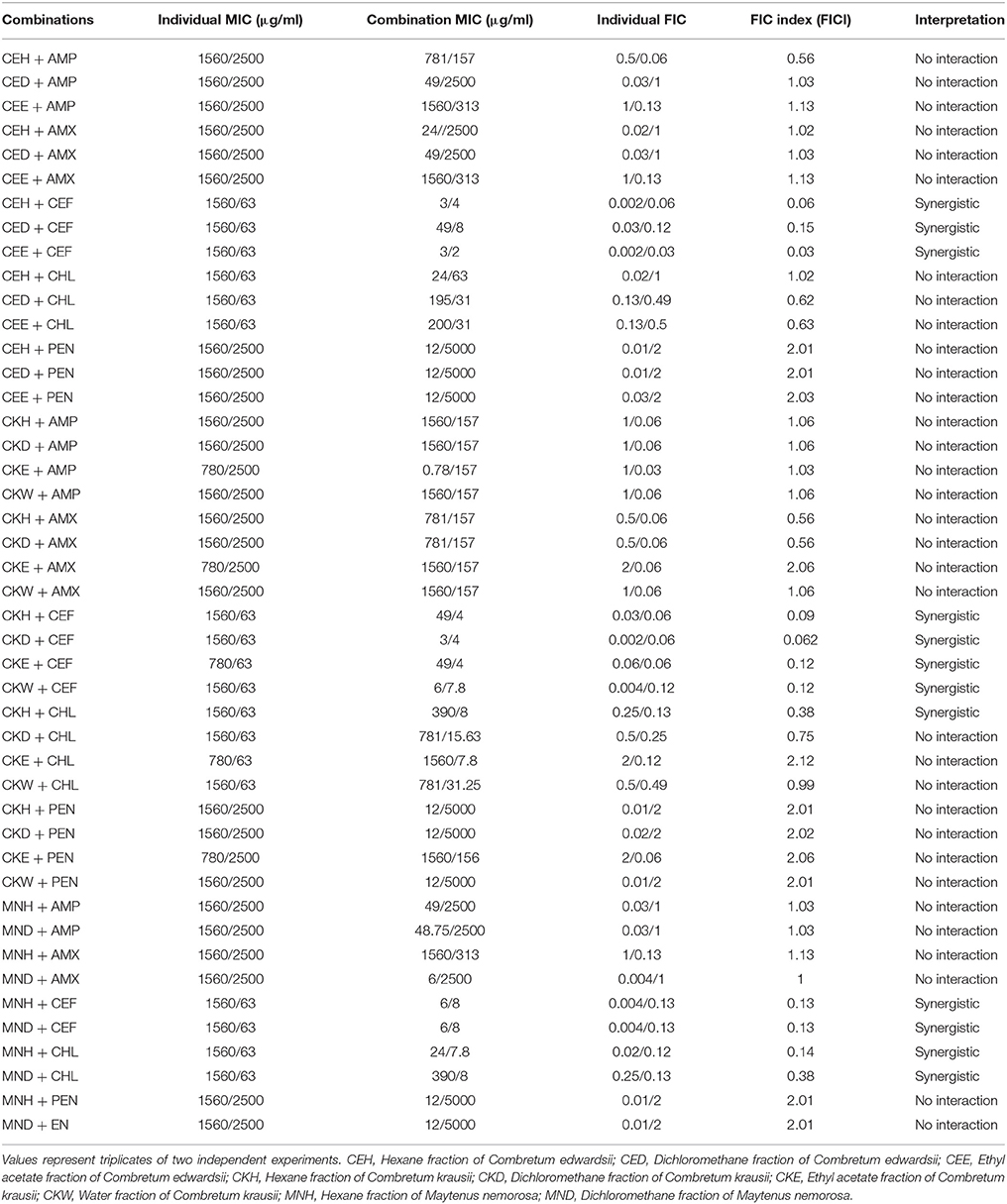
Table 3. Combined effects of different fractions of Combretum edwardsii, Combretum krausii, and Maytenus nemorosa with antibiotics against multidrug resistant Klebsiella pneumonia.
The most susceptible bacterial strain used in the study was S. aureus. There were 35 synergistic effects out of 44 combinations against this strain (Table 4). Combinations with all antibiotics, except cefotaxime, displayed synergistic activity. The best combinations were water fraction of C. krausii and penicillin (FICI = 0.04); ethyl acetate fraction of C. krausii and penicillin (FICI = 0.05), hexane fraction of C. edwardsii and amoxicillin (FICI = 0.05), hexane fraction of C. edwardsii and penicillin (FICI = 0.06), hexane fraction of C. krausii and amoxicillin (FICI = 0.06), and ethyl acetate of C. krausii and amoxicillin (0.06). In the best combinations, the MICs of penicillin and amoxicillin were reduced from 39 to 1.2 μg/ml and 39 to 2 μg/ml, respectively.
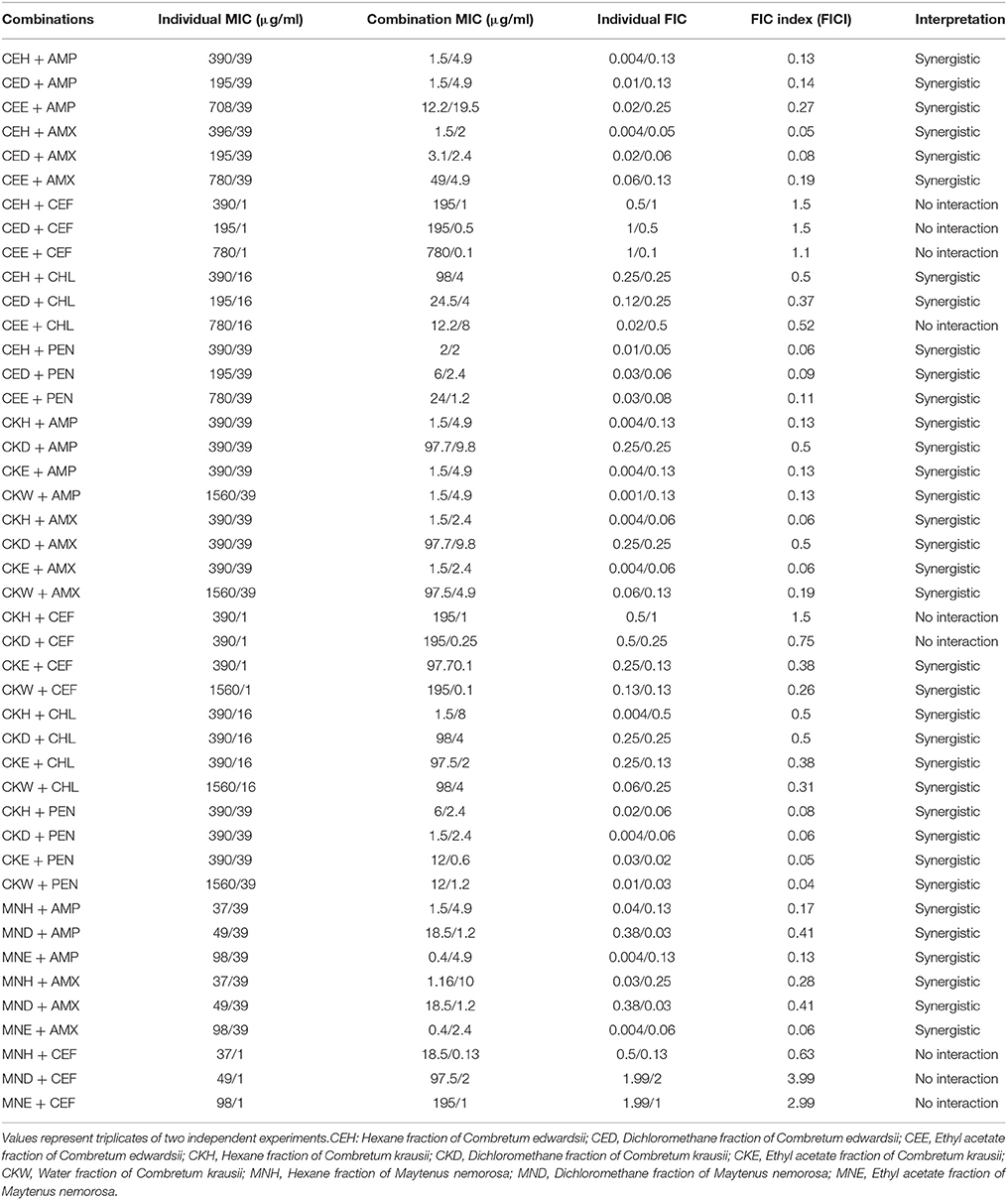
Table 4. Combined effects of different fractions of Combretum edwardsii, Combretum krausii, and Maytenus nemorosa with antibiotics against penicillin resistant S. aureus.
Considering the number and medicinal importance of the members of the genus Combretum, only a few reports exist on their interaction with antibiotics with regards to antibacterial activity. The leaf extract of C. albidum potentiated the antibacterial activity of ceftriaxone against multidrug-resistant Pseudomonas aeruginosa (Sahu et al., 2014). In a separate study, the leaf extract of C. molle also enhanced the antibacterial activities of kanamycin and streptomycin against Gram-negative bacteria including multidrug-resistant strains (Fankam et al., 2015).
Conclusions
It is important to note how some of the extracts in the present study enhanced the activities of some of the clinically ineffective antibiotics against the two multi-drug Gram-negative bacteria. Examples are the reduction of the MICs of ampicillin and amoxicillin by different fractions of C. edwardsii and C. krausii. The enhancement could be through the inhibition of beta-lactamases activity or increase in the permeability of the antibiotics thereby making the antibiotics more effective. In other words, they tend to reverse the antimicrobial resistance. The constituents of these plant extracts therefore, have the potential to enhance and restore the activities of some clinically used antibiotics. Since combinations confirmed that in vitro effects may not be the same in vivo, further studies are still required, especially extensive in vivo studies and research on the toxicity of these combinations. As far as we know, this is the first report on the antibacterial activity of M. nemorosa, individually and in combination with antibiotics. We intend to follow up this study with bioactivity guided isolation of the bioactive compounds.
Author Contributions
JCC undertook the research and wrote the paper. JvS read and approved the paper.
Funding
Funding was provided by UKZN in the form of a Postdoctoral Fellowship.
Conflict of Interest Statement
The authors declare that the research was conducted in the absence of any commercial or financial relationships that could be construed as a potential conflict of interest.
Acknowledgments
The University of KwaZulu-Natal is thanked for financial assistance in the form of a Postdoctoral Fellowship to JCC.
References
Ambler, R. P., Coulson, A. F., Frère, J. M., Ghuysen, J. M., Joris, B., Forsman, M., et al. (1991). A standard numbering scheme for the class A β-lactamases. Biochem. J. 276, 269–270. doi: 10.1042/bj2760269
Angeh, J. E., Huang, X., Sattler, I., Swan, G. E., Dahse, H., Härtl, A., et al. (2007). Antimicrobial and anti-inflammatory activity of four known and one new triterpenoid from Combretum imberbe (Combretaceae). J. Ethnopharmacol. 110, 56–60. doi: 10.1016/j.jep.2006.09.002
Brookes, K. B., Doudoukina, O. V., Katsoulis, L. C., and Veale, D. J. H. (1999). Uteroactive constituents from Combretum kraussii. S. Afr. J. Chem. 52, 127.
Bush, K., and Jacoby, G. A. (2010). Updated functional classification of β-lactamases. Antimicrob. Agents Chemother. 54, 969–976. doi: 10.1128/AAC.01000-09
Bush, K. (2001). New b -lactamases in Gram-negative bacteria: diversity and impact on the selection of antimicrobial therapy. Clin. Infect. Dis. 32, 1085–1089. doi: 10.1128/AAC.01009-09
Butterweck, V., Lieflander-Wulf, U., Winterhoff, H., and Nahrstedt, A. (2003). Plasma levels of hypericin in presence of procyanidin B2 and hyperoside: a pharmacokinetic study in rats. Planta Med. 69, 189–192. doi: 10.1055/s-2003-38495
Cooksey, R., Swenson, J., Clark, N., Gay, E., and Thornsberry, C. (1990). Patterns and mechanisms of beta-lactam resistance among isolates of Escherichia coli from hospitals in the United States. Antimicrob. Agents Chemother. 34, 739–45.
De León, L., López, M. R., and Moujir, L. (2010). Antibacterial properties of zeylasterone, a triterpenoid isolated from Maytenus blepharodes, against Staphylococcus aureus. Microbiol. Res. 165, 617–626. doi: 10.1128/AAC.34.5.739
Eldeen, I. M. S., Elgorashi, E. E., and van Staden, J. (2005). Antibacterial, anti-inflammatory, anti-cholinesterase and mutagenic effects of extracts obtained from some trees used in South African traditional medicine. J. Ethnopharmacol. 102, 457–464. doi: 10.1016/j.jep.2005.08.049
Elegami, A. A., El-Nima, E. I., El Tohami, M. S., and Muddathir, A. K. (2002). Antimicrobial activity of some species of the family Combretaceae. Phytother. Res. 16, 555–561. doi: 10.1002/ptr.995
Eloff, J. N. (1998). A sensitive and quick microplate method to determine the minimal inhibitory concentration of plant extracts for bacteria. Planta Med. 64, 711–713. doi: 10.1055/s-2006-957563
Eloff, J. N., Famakin, J. O., and Katerere, D. R. P. (2005). Combretum woodii (Combretaceae) leaf extracts have high activity against Gram-negative and Gram-positive bacteria. Afr. J. Biotechnol. 4, 1161–1166. doi: 10.4314/ajb.v4i10.71275
Fankam, A. G., Kuiate, J. R., and Kuete, V. (2015). Antibacterial and antibiotic resistance modifying activity of the extracts from Allanblackia gabonensis, Combretum molle and Gladiolus quartinianus against Gram-negative bacteria including multi-drug resistant phenotypes. BMC Complement. Altern. Med. 15:206. doi: 10.1186/s12906-015-0726-0
Fyhrquist, P., Mwasumbi, B. L., Hæggström, C. A., Vuorela, H., Hiltunen, R., and Vuorel, P. (2002). Ethnobotanical and antimicrobial investigation on some species of Terminalia and Combretum (Combretaceae) growing in Tanzania. J. Ethnopharmacol. 79, 169–177. doi: 10.1016/S0378-8741(01)00375-0
Hemaiswarya, S., Kruthieventi, A. K., and Doble, M. (2008). Synergism between natural products and antibiotics against infectious diseases. Phytomedicine 15, 639–652. doi: 10.1016/j.phymed.2008.06.008
Hübsch, Z., Van Zyl, Z. L., Cock, I. E., and Van Vuuren, S. F. (2014). Interactive antimicrobial and toxicity profiles of conventional antimicrobials with Southern African medicinal plants. S. Afr. J. Bot. 93, 185–197. doi: 10.1016/j.sajb.2014.04.005
Katerere, D. R., Gray, A. I., Nash, R. J., and Waigh, R. D. (2012). Phytochemical and antimicrobial investigations of stilbenoids and flavonoids isolated from three species of Combretaceae. Fitoterapia 83, 932–940. doi: 10.1016/j.fitote.2012.04.011
Kuete, V. (2010). Potential of cameroonian plants and derived products against microbial infections: a review. Planta Med. 76, 1479–1491. doi: 10.1055/s-0030-1250027
Le Roux, L., and Reynolds, Y. (2003). Combretum kraussii. Available online at: http://www.plantzafrica.com/plantcd/combretkraus.htm (Accessed September 02, 2015).
Lindsey, K. L., Budesinsky, M., Kohout, L., and van Staden, J. (2006). Antibacterial activity of maytenonic acid isolated from the root-bark of Maytenus senegalensis. S. Afr. J. Bot. 72, 473–477. doi: 10.1016/j.sajb.2005.12.011
Martini, N. D., Katerere, D. R. P., and Eloff, J. N. (2004). Biological activity of five antibacterial flavonoids from Combretum erythrophyllum (Combretaceae). J. Ethnopharmacol. 93, 207–212. doi: 10.1016/j.jep.2004.02.030
Masoko, P., and Eloff, J. N. (2007). Screening of twenty-four South African Combretum and six Terminalia species (Combretaceae) for antioxidant activities. Afr. J. Trad. 4, 231–239. doi: 10.4314/ajtcam.v4i2.31213
Matu, E. N., and van Staden, J. (2003). Antibacterial and anti-inflammatory activities of some plants used for medicinal purposes in Kenya. J. Ethnopharmacol. 87, 35–41. doi: 10.1016/S0378-8741(03)00107-7
McGaw, L. J., Rabe, T., Sparg, S. G., Ja¨ger, A. K., Eloff, J. N., and van Staden, J. (2001). An investigation on the biological activity of Combretum species. J. Ethnopharmacol. 75, 45–50. doi: 10.1016/S0378-8741(00)00405-0
Odds, F. C. (2003). Synergy, antagonism, and what the chequerboard puts between them. J. Antimicrob. Chemother. 52, 1. doi: 10.1093/jac/dkg301
Pooley, E. (1993). The Complete Field Guide to Trees of Natal, Zululand and Transkei. Durban: Natal Flora Publications Trust, Natal Herbarium.
Quattrocchi, U. (2012). CRC World Dictionary of Medicinal and Poisonous Plants: Common Names, Scientific Names, Eponyms, Synonyms, and Etymology (5 Volume Set). Boca Raton, FL: Taylor & Francis Group; CRC Press. 1070–1081. doi: 10.1201/b16504
Rasoanaivo, P., Wright, C. W., Willcox, M. L., and Gilbert, B. (2011). Whole plant extracts versus single compounds for the treatment of malaria: synergy and positive interactions. Malar. J. 10:S4. doi: 10.1186/1475-2875-10-S1-S4
Rath, K., Taxis, K., Walz, G., Gleiter, C. H., Li, S.-M., and Heide, L. (2004). Pharmacokinetics study of artemsinin after oral intake of a traditional preparation of Artemisia annua L. (annual wormwood). Am. J. Trop. Med. Hyg. 70, 128–132.
Rios, J. L., and Recio, M. C. (2005). Medicinal plants and antimicrobial activity. J. Ethnopharmacol. 100, 80–84. doi: 10.1016/j.jep.2005.04.025
Rogers, C. B. (1989). Isolation of the 1α-hydroxycycloartenoid mollic acid α-l-arabinoside from Combretum edwardsii leaves. Phytochemistry 28, 279–281. doi: 10.1016/0031-9422(89)85060-5
Sahu, M. C., Patnaik, R., and Padhy, R. N. (2014). In vitro combinational efficacy of ceftriaxone and leaf extract of Combretum albidum G. Don against multidrug-resistant Pseudomonas aeruginosa and host-toxicity testing with lymphocytes from human cord blood. J. Acute Med. 4, 26–37. doi: 10.1016/j.jacme.2014.01.004
Wagner, H., and Ulrich-Merzenich, G. (2009). Synergy research: approaching a new generation of phytopharmaceuticals. Phytomedicine 16, 97–110. doi: 10.1016/j.phymed.2008.12.018
Keywords: antibacterial activity, synergistic effects, Maytenus nemorosa, Combretum edwardsii, Combretum krausii
Citation: Chukwujekwu JC and van Staden J (2016) In vitro Antibacterial Activity of Combretum edwardsii, Combretum krausii, and Maytenus nemorosa and Their Synergistic Effects in Combination with Antibiotics. Front. Pharmacol. 7:208. doi: 10.3389/fphar.2016.00208
Received: 01 February 2016; Accepted: 29 June 2016;
Published: 15 July 2016.
Edited by:
Lyndy Joy McGaw, University of Pretoria, South AfricaReviewed by:
Fang-Rong Chang, Kaohsiung Medical University, TaiwanAshwell Rungano Ndhlala, Agricultural Research Council, South Africa
Copyright © 2016 Chukwujekwu and van Staden. This is an open-access article distributed under the terms of the Creative Commons Attribution License (CC BY). The use, distribution or reproduction in other forums is permitted, provided the original author(s) or licensor are credited and that the original publication in this journal is cited, in accordance with accepted academic practice. No use, distribution or reproduction is permitted which does not comply with these terms.
*Correspondence: Johannes van Staden, cmNwZ2RAdWt6bi5hYy56YQ==