- 1Princess Margaret Cancer Centre and Techna Institute, University Health Network, Toronto, ON, Canada
- 2Institute of Biomaterials and Biomedical Engineering, University of Toronto, Toronto, ON, Canada
- 3Department of Medical Biophysics, University of Toronto, Toronto, ON, Canada
Scavenger receptor class B type I (SR-BI) plays an important role in trafficking cholesteryl esters between the core of high density lipoprotein and the liver. Interestingly, this integral membrane protein receptor is also implicated in the metabolism of cholesterol by cancer cells, whereby overexpression of SR-BI has been observed in a number of tumors and cancer cell lines, including breast and prostate cancers. Consequently, SR-BI has recently gained attention as a cancer biomarker and exciting target for the direct cytosolic delivery of therapeutic agents. This brief review highlights these key developments in SR-BI-targeted cancer therapies and imaging probes. Special attention is given to the exploration of high density lipoprotein nanomimetic platforms that take advantage of upregulated SR-BI expression to facilitate targeted drug-delivery and cancer diagnostics, and promising future directions in the development of these agents.
Introduction
Upregulation of cell-surface receptors in malignant tissue is both a hindrance and an opportunity in cancer therapy. On one hand, receptor upregulation enhances cancer cell response to growth factors, enabling one of their defining characteristics of self-sufficient proliferation (Hanahan and Weinberg, 2000). However, these overexpressed cell-surface receptors can also be opportunistically used to target cancer therapies. This is a highly desirable treatment strategy with the potential to enhance therapeutic indices by confining antineoplastic and cytotoxic effects to tumors. To this end, scavenger receptor class B type I (SR-BI) has recently been pursued as a target to facilitate cancer therapy and imaging.
SR-BI is an integral membrane glycoprotein receptor that plays a crucial role in the metabolism of high-density lipoprotein (HDL; Krieger, 1999). SR-BI binds HDL with high affinity to mediate selective cellular uptake and efflux of cholesteryl esters from the lipoprotein core (Acton et al., 1996; Ji et al., 1997). This uptake is made highly efficient via the formation of a non-aqueous channel that permits the direct cytosolic influx of the lipid core with no corresponding lysosomal degradation (Rodrigueza et al., 1999). In addition to mediating the transfer of cholesterol between HDL and healthy cells (predominantly within the liver and steroidogenic tissue), SR-BI also facilitates the selective uptake of cholesterol by malignant cells. As summarized in Table 1, several patient tumors and cancer cell lines display upregulated expression of SR-BI relative to healthy tissue, rendering SR-BI an interesting cell-surface receptor to pursue for targeted cancer therapy and imaging. In this mini review, we highlight recently explored strategies that take advantage of SR-BI overexpression and its capacity for direct cytosolic delivery of HDL cargo for the development of targeted cancer drug-delivery systems, imaging probes, and biomarkers.
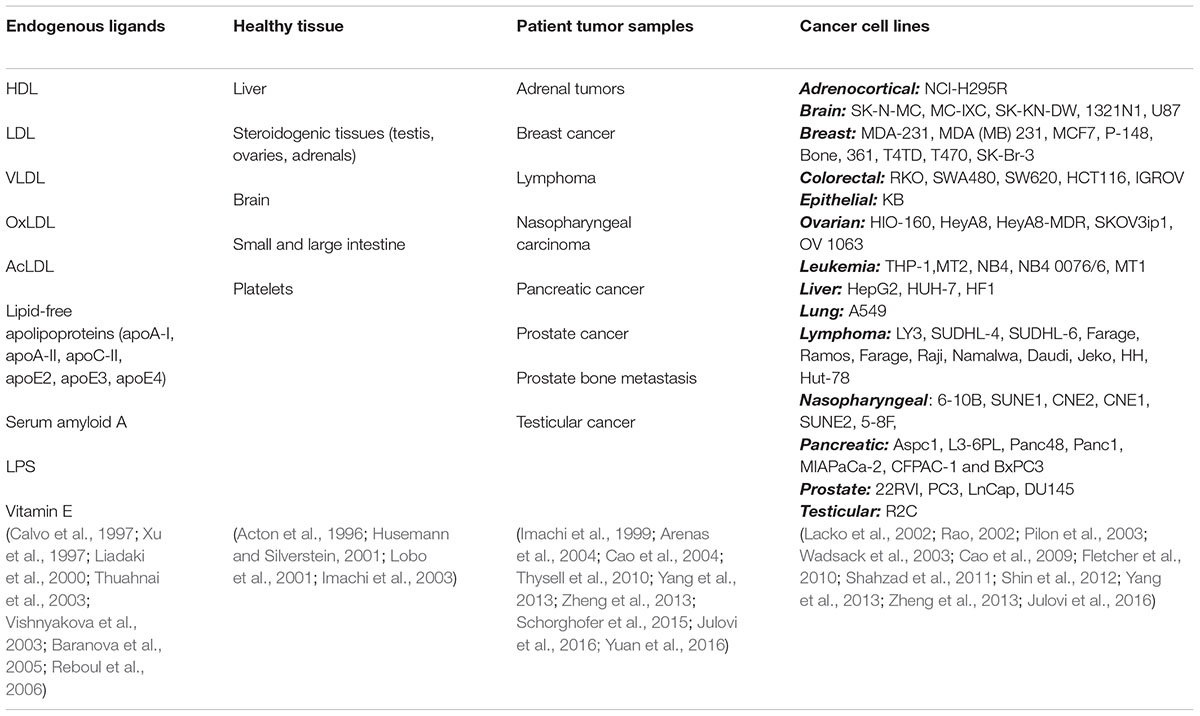
TABLE 1. SR-BI endogenous ligands, expression in healthy tissue, upregulation in patient derived tumor samples and expression by cancer cell lines (OxLDL, AcLDL, and LPS represent oxidized LDL, acetylated LDL and lipopolysaccharides, respectively).
SR-BI Targeting Strategies for Cancer Therapy
The ability of SR-BI to mediate direct cytosolic transport of HDL cholesteryl esters is a particularly lucrative characteristic to exploit for drug-delivery, as it overcomes reliance on endo-lysosomal uptake routes that lead to drug degradation. HDL-nanomimetics, composed of phospholipids and the most abundant HDL apolipoprotein, A-I (apoA-I), have thus been designed to exploit this cytosolic drug-delivery pathway. As reviewed elsewhere (Ng et al., 2011; Thaxton et al., 2016), HDL-nanomimetics possess many traits suited for drug-delivery, including lengthy circulation half-lives, stable core-loading with hydrophobic drugs, surface-functionalization by cholesterol-conjugated agents, and a sub-30 nm size favorable for enhanced nanoparticle permeation through tumor extracellular matrices. Furthermore, reviews of clinical HDL-infusion therapies demonstrate that elevations in plasma HDL levels (up to 30-fold) are well-tolerated following HDL-nanomimetic administration at doses of 0.25–135 mg/kg, whereby the nanoparticles subsequently participate in endogenous HDL receptor binding and metabolic pathways of interest for drug-delivery and atherosclerosis therapy, including reverse cholesterol transport leading to remodeling and equilibration of the administered HDL into spherical nanoparticles (Kingwell and Chapman, 2013; Simonsen, 2016). To this end, HDL-nanomimetics have been explored for SR-BI-mediated delivery of chemotherapeutics, small interfering ribonucleic acid (siRNA), and photosensitizers, as summarized in Table 2. Although lipoprotein-mimetic platforms have been widely explored more generally for cancer theranostics, this review focuses on agents that were studied to specifically target SR-BI.
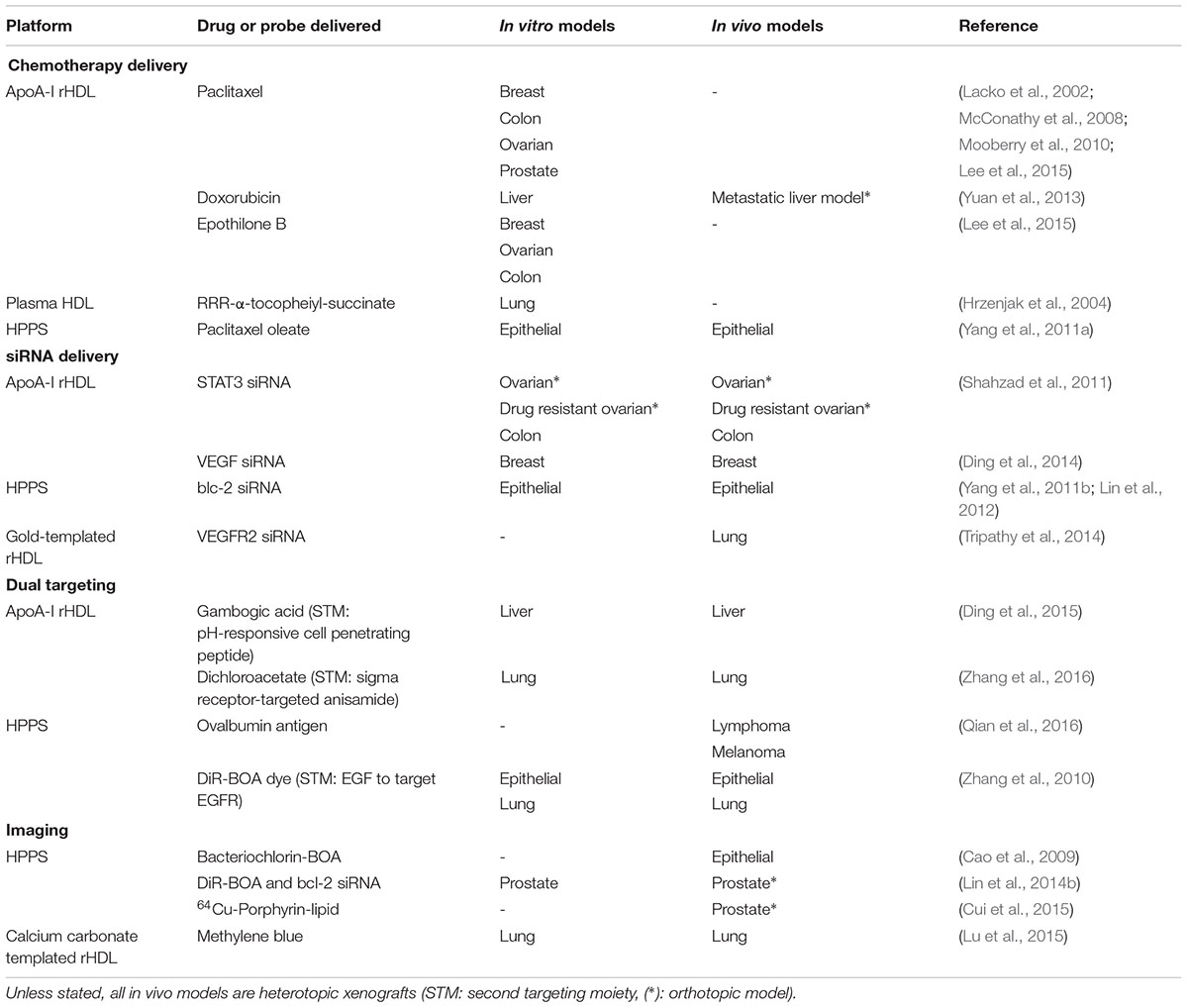
TABLE 2. Examples of SR-BI-targeted drug and imaging probe delivery vehicles applied to in vitro and in vivo cancer models.
Delivery of Chemotherapeutics
The first reports of SR-BI-targeted drug-delivery vehicles for cancer therapy aimed to enhance therapeutic effects and reduce off-target toxicity of chemotherapeutics. In 2002, Lacko et al. generated a paclitaxel-loaded apoA-I-reconstituted HDL (rHDL) vehicle that underwent uptake by various SR-BI-expressing cancer cell lines, including human prostate DU145 and PC3 cells (Lacko et al., 2002). Encapsulation of cargo within apoA-I-rHDL delayed its degradation in serum and facilitated SR-BI-selective cargo delivery (McConathy et al., 2011). In follow-up studies, this uptake resulted in a five-fold enhancement in paclitaxel delivery to OVAR-3 cells and 5- to 20-fold enhancement in cytotoxicity against ovarian, prostate and breast cancer cells versus free paclitaxel (McConathy et al., 2008; Mooberry et al., 2010). This enhanced in vitro therapeutic efficacy was coupled with increased in vivo tolerance of the paclitaxel-rHDL formulation relative to free paclitaxel or the clinically approved Abraxane® albumin-paclitaxel nanoformulation (McConathy et al., 2008). ApoA-I-rHDL nanoconstructs were similarly used by other researchers to facilitate SR-BI-targeted delivery of paclitaxel and other hydrophobic agents such as RR-α-tocopheryl-succinate and epothilone B to adeno, breast and lung cancer cells (Table 2). In each case, drug-rHDL treatment increased SR-BI+ cancer cell cytotoxicity versus free drug with the added benefit, as reported by Ding et al. (2014), of diminishing undesired cytotoxicity against cells with limited SR-BI expression. Interestingly, this SR-BI-targeting strategy was also extended to the encapsulation and delivery of the hydrophilic drug, doxorubicin (Yuan et al., 2013), wherein the efficient (>70%) loading of drug within rHDL halved its IC50 in hepatocellular carcinoma (HCC) cells, yielded sustained drug release, and reduced tumor size in an apoA-I-dependent manner.
One concern associated with the clinical translation of SR-BI-targeted apoA-I-rHDL delivery platforms is the necessity of apoA-I protein for particle functionalization. Apo-AI is isolated from human plasma or derived from bacterial recombinant protein expression, and consequently may be prone to low collection yields, batch-to-batch variability, and contamination. To this end, our group introduced HDL-mimicking-peptide-phospholipid nanoscaffolds (HPPS). These HDL-nanomimetics are built with an 18 residue apoA-I-mimetic α-helical amphipathic peptide, which similarly to apoA-I, constrains the particle size below 30 nm and directs the selective cytosolic delivery of core-loaded cargo to SR-BI+ cells both in vitro and in vivo (Zhang et al., 2009; Lin et al., 2014a). When core-loaded with paclitaxel oleate (PTXOL), HPPS suppressed tumor growth selectively in SR-BI+ lesions to the same extent as PTXOL, but unlike the free drug, exerted no significant tumoricidal effects in non-target SR-BI- tumors (Yang et al., 2011a). Combined with an absence of incurred acute liver toxicity, these results demonstrate that HDL-nanomimetics such as HPPS are a suitable and therapeutically effective strategy to attenuate off-target toxicity via SR-BI-homing.
Direct Cytosolic Delivery of siRNA
Perhaps one of the most promising therapeutic utilities of SR-BI-mediated cytosolic drug-delivery is stable transfection of cancer cells with siRNA. As widely reviewed (Devi, 2006; Oh and Park, 2009; Petrocca and Lieberman, 2011; Zuckerman and Davis, 2015), RNA interference (RNAi) is an intriguing cancer therapy whereby short double-stranded RNA interacts with complimentary messenger RNA within the cell cytoplasm for sequence-specific post-transcriptional silencing of target oncogenes. Barriers to successful RNAi therapy include rapid siRNA degradation in circulation, off-target accumulation, toxicity, inefficient intracellular delivery of siRNA complexes, and endosomal escape (Whitehead et al., 2009; Pecot et al., 2011). Conversely, SR-BI-facilitated siRNA delivery to cancer cells has several advantages. Cholesterol-conjugated siRNA readily binds to HDL, which mediates its cellular uptake in vivo via SR-BI (Wolfrum et al., 2007). This allows for endosome-independent, direct cytosolic siRNA delivery, significantly enhancing siRNA transfection and reducing in vitro and in vivo target protein expression in SR-BI+ cancer cells relative to free siRNA and on par with liposomal-siRNA complexes, which are the current clinical gold standards for mediating siRNA delivery in vivo (Yang et al., 2011b; Lin et al., 2012; Ding et al., 2014; Tripathy et al., 2014; Zuckerman and Davis, 2015). This SR-BI-mediated siRNA delivery has been conducted via apoA-I-rHDL, HPPS, and gold-templated HDL-nanomimetics to silence oncogenes involved in cell proliferation, differentiation, anti-apoptotic pathways, and angiogenesis in vitro and in vivo models of breast, epidermal, lung, ovarian, and colorectal cancer (Table 2). Encapsulation of siRNA within SR-BI-targeted nanoparticles delayed its degradation and extended its circulation half-life (Lin et al., 2012; Ding et al., 2014; Tripathy et al., 2014). Furthermore, SR-BI-targeting of siRNA yielded potent in vivo effects. In metastatic and taxane-resistant models of ovarian cancer, rHDL-mediated siRNA delivery reduced tumor burden by 60%, and when combined with chemotherapy decreased metastatic lesions by 86% and tumor growth by over 90% relative to controls (Shahzad et al., 2011). Tripathy et al. (2014) demonstrated potent inhibition of neovascularization and tumor growth in a Lewis lung carcinoma model using gold-templated HDL-nanomimetics, while Ding et al. (2014) observed similar effects in a breast cancer model with the use of apoA-I-rHDL. Importantly, in vivo therapeutic effects of bcl-2-siRNA-HPPS demonstrated by Lin et al. (2012) in KB tumor models coincided with an absence of acute off-target toxicity. Though further in vivo investigation of SR-BI-targeting, longitudinal safety, and therapeutic efficacy must be conducted, these preliminary studies showcase the exciting potential of SR-BI-mediated siRNA cancer therapy.
Combining SR-BI-Homing with Alternative Drug-Delivery Strategies
Despite the successes described thus far in targeting SR-BI to enhance and confine cancer therapy to target tumor tissue, associated biodistribution data demonstrated significant off-target accumulation of drugs, particularly in the liver and spleen (Zhang et al., 2009; Yang et al., 2011a; Tripathy et al., 2014); an unsurprising observation given the abundant expression of SR-BI in normal hepatic and steroidogenic tissue (Table 1). Thus, to limit potential drug-induced systemic toxicity and to further amplify and target therapeutic activity to tumor tissue, the following strategies combining SR-BI-homing with additional targeting functionality were recently explored:
(1) Photosensitive porphyrin nanoparticles: Porphyrins are non-toxic, naturally occurring heterocyclic molecules that require activation by near infrared (NIR) light to generate cytotoxic reactive oxygen species for cancer photodynamic therapy. When formulated with porphyrin-lipid, HDL-nanomimetics can thus exhibit multidimensional tumor targeting via apoA-I/SR-BI interactions, site-specific laser irradiation, and the inherent cancer cell affinity displayed by porphyrins (Zheng et al., 2007). This was demonstrated by Ng et al. (2013), who showed that porphyrin-HDL nanodisc-induced cytotoxicity required both cellular SR-BI expression and laser light.
(2) pH-sensitive nanoparticles: The acidic microenvironment of tumors has been employed to enhance drug accumulation at target lesions in combination with SR-BI targeting. By functionalizing the surface of apoA-I-rHDL with pH-sensitive cell-penetrating peptides, Ding et al. (2015) demonstrated enhanced SR-BI-mediated uptake of the hydrophobic apoptosis inducer gambogic acid within the cytosol of HCC cells at a pH of 6.4 versus a normal physiological pH of 7.4.
(3) Dual receptor-targeted agents: The incorporation of a second targeting vector directed toward upregulated cell-surface receptors in malignant cells, such as the sigma receptor or epidermal growth factor receptor, was also explored to enhance the targeting specificity of SR-BI-directed therapies. Compared with singularly targeting SR-BI, this multi-receptor-targeted approach increased drug accumulation in cancer cells in vitro and tumor xenografts in vivo, and was shown to enhance the dichlororacetate/p53-induced suppression of tumor growth in a model of lung adenocarcinoma (Zhang et al., 2010, 2016).
(4) Antigen-mediated immunotherapy: HPPS was formulated with a fusion peptide consisting of α-helical apoA-I-mimetic peptide and an antigen peptide against ovalbumin (expressed by E.G7 lymphoma cells) to generate a lymphoma nanovaccine, which demonstrated the most potent in vivo therapeutic effects of the four multi-homing strategies described herein. The particles targeted SR-BI+ mature dendritic cells, augmenting antigen uptake in vivo by up to 100-fold relative to free antigen delivery. This increased the ability of dendritic cells to augment CD8+ T-cell populations in vivo, allowing for immunization against lymphoma tumor growth, which was completely inhibited in a synergistic fashion when particles were further functionalized with cholesterol-conjugated toll-like receptor agonist CpG2395. This ultimately led to a survival rate of 83 %, whereas free fusion peptide was associated with complete mortality.
The positive findings from these preliminary studies support further evaluation of SR-BI-facilitated multi-targeted nanomedicines for cancer therapy, including complete characterization of particle biodistribution and therapeutic efficacy in orthotopic animal cancer models.
Antagonizing SR-BI Activity
Dysregulation of cholesterol metabolism has been associated with tumor growth since the early 1900’s, whereby the proliferation and migration of cancer cells is thought to require increased cholesterol influx, yielding higher cellular cholesterol levels in malignant tissue (Swyer, 1942; Dessì et al., 1994; Thysell et al., 2010; Cruz et al., 2013; de Gonzalo-Calvo et al., 2015). SR-BI was shown to play a role in this process, such that its inhibition reduced cancer cell proliferation (Pussinen et al., 2000; Cao et al., 2004; Leon et al., 2010; Julovi et al., 2016). Thus, a few recent reports sought to therapeutically mitigate the pro-tumorigenic activity of SR-BI. Twiddy et al. (2012) demonstrated that siRNA knockdown of SR-BI in castration-resistant prostate cancer cells significantly decreased cell viability and secretion of prostate-specific antigen, a biomarker of prostate cancer. Subsequently, Yang et al. (2013) designed 13 nm gold-templated, apoA-I-functionalized HDL-nanomimetics that selectively inhibited SR-BI+ B-cell lymphoma growth by attenuating cholesterol influx into SUDHL-4 lymphoma cells, while displaying no apoptosis induction in human primary hepatocytes and macrophages. HPPS was similarly shown to attenuate tumor growth in a nasopharyngeal carcinoma animal model, which the authors proposed via in vitro studies to be a result of inhibiting SR-BI-regulated cell motility (Zheng et al., 2013). The findings from these studies stand in line with the known protective effects of ApoA-I and HDL on tumor growth (Su et al., 2010; Zamanian-Daryoush and DiDonato, 2015), presenting an alternative and less explored strategy of enforcing SR-BI-directed cancer therapy.
SR-BI-Mediated Cancer Imaging and Diagnostics
The overexpression of SR-BI in patient tumor samples relative to healthy tissue also potentiates its use for cancer imaging and diagnostics. Although many of the SR-BI-targeted nanoparticle drug-delivery systems discussed above were formulated with NIR dyes for biodistribution analyses, limited studies have actively pursued SR-BI-targeting for the generation of cancer imaging probes. For example, in 2009, Cao et al. developed apoA-I-rHDL core-loaded with bis-oleate-functionalized bacteriochlorin (BChl), a fluorescent dye excitable by 750–850 nm NIR light; a range that falls within the optical window optimal for fluorescence imaging in vivo with minimal absorbance interference from hemoglobin and water (Cao et al., 2009). The authors demonstrated SR-BI-selective cellular uptake of the BChl core in vitro, which translated into strong contrast visualized with a small animal fluorescence imaging system in KB tumor tissue in vivo. ApoA-I HDL-mimetics were also core-loaded with methylene blue for apoA-I-mediated in vivo NIR fluorescence imaging of SR-BI+ lung carcinoma xenografts (Lu et al., 2015). Core-loading of HPPS with NIR fluorophores was also conducted to image the biodistribution of co-loaded siRNA for treatment planning purposes (Lin et al., 2014b). By monitoring fluorescence contrast at tumor sites, the authors were able to select a dose regimen that confined particle accumulation and potent apoptotic effects of the siRNA therapy to the target lesion. This selective fluorescence contrast was further proposed by the authors to have utility in image-guided tumor resection. In addition to fluorescence imaging, SR-BI-targeted HPPS was also amenable to generating contrast for positron emission tomography imaging of deep-seated orthotopic prostate tumors when formulated with a 64Cu-porphyrin-lipid shell (Cui et al., 2015). It should be noted that the tumor-homing displayed by these particles is likely contributed to by the enhanced permeability and retention (EPR) effect, hallmarked by the increasing contrast observed at the tumor site over a 24–48 h time period following agent administration. Consequently, further in vivo characterization of selective particle uptake must be conducted to ensure SR-BI-specificity is maintained by the contrast agents for accurate diagnostic and imaging purposes.
The upregulated expression of SR-BI in itself has been proposed to serve as a biomarker for tumor malignancy. The histological analysis of 106 prostate cancer biopsies by Schorghofer et al. (2015) revealed a positive correlation between elevated SR-BI expression and tumor grade, metastasis, and poorer patient outcomes. Similarly, higher SR-BI expression in breast cancer tissue was associated with increased disease aggressiveness and patient mortality (Yuan et al., 2016), while RNA microarrays of samples from patients with chronic myeloid leukemia identified a six gene profile including SCARB1 (the gene encoding for SR-BI) that discriminated early and late-stage disease (Oehler et al., 2009). These promising initial studies thus suggest that SR-BI may be a viable biomarker of cancer prognosis. Nevertheless, due to the ubiquitous expression of SR-BI in malignant and healthy tissue, key consideration of the specificity associated with the use of SR-BI as a biomarker will be imperative in assessing its prognostic value.
Summary and Perspectives
The overexpression of SR-BI in malignant tissue has been exploited for the development of targeted cancer therapies, imaging probes, and prognostic biomarkers. SR-BI-targeted vehicles can mediate the selective transfer of drugs from HDL-mimetics into the cytosol of malignant cells, which is particularly valuable for enhancing siRNA delivery efficiency for cancer gene therapy. Although promising in vivo therapeutic effects were demonstrated by the SR-BI-directed therapies overviewed herein, a number of key challenges must be addressed to advance the utility of what is still a relatively unexplored targeting strategy. Firstly, the ubiquitous expression of SR-BI in healthy tissue, and particularly its abundant expression in the liver and steroidogenic organs, presents a challenge in assuring the in vivo specificity of SR-BI-targeted agents. As such, careful consideration is due when exclusively targeting SR-BI for the delivery of chemotherapeutics with non-specific mechanisms of action, especially those which may cause liver toxicity. To this end, it is imperative that researchers expand upon the currently limited evaluation of acute and chronic systemic toxicity of SR-BI-targeting agents. Furthermore, quantification of drug-delivery to tumors and major organs and the unequivocal demonstration of SR-BI targeting in vivo (for example via competitive inhibition and comparative studies in SR-BI+ and SR-BI- tumor models) should become mainstays within the field. In order to obtain more clinically relevant information, these evaluations should extend beyond typically used heterotopic tumor models to include orthotopic models. The reliance on inherently disrupted tumor vasculature and the EPR effect for tumor accumulation of the explored HDL-nanomimetics also creates a barrier to efficient SR-BI-targeted cancer therapy. Though widely used as a targeting strategy in nanomedicine development, the clinical relevance of the EPR effect is disputed as it does not accurately represent the vasculature and microenvironment of many human tumors (Gillies et al., 1999; Nichols and Bae, 2012). Strategies that target nanoparticle extravasation to tumor sites without requiring inherently disrupted tumor vasculature to facilitate delivery may thus enhance the delivery efficiency and therapeutic relevance of SR-BI-targeted HDL-mimetics. For example, Mulik et al. (2016) employed microbubble-enhanced focused ultrasound to selectively disrupt the blood-brain barrier in rats to enhance the site-specific delivery of lipoprotein-mimetics to target brain tissue. Additionally, given the multi-ligand status of SR-BI (Table 1), ample opportunity exists for the exploration of alternative targeting ligands to apoA-I, which may access a wider variety of tumors and alter particle distribution profiles. Ultimately, with further exploration of alternative SR-BI-targeting vectors and more rigorous in vivo characterization of existing delivery platforms, a better understanding of the utility of SR-BI-targeting for cancer therapy and imaging will be gained.
Author Contributions
MR and GZ were involved in the conception, drafting, and editing of the manuscript to ensure an accurate review of literature.
Conflict of Interest Statement
The authors declare that the research was conducted in the absence of any commercial or financial relationships that could be construed as a potential conflict of interest.
Acknowledgments
The authors would like to thank their funding sources: Canadian Institutes of Health Research, Natural Sciences and Engineering Research Council of Canada, Princess Margaret Cancer Foundation, Prostate Cancer Canada, and the Vanier Canada Graduate Scholarship.
References
Acton, S., Rigotti, A., Landschulz, K. T., Xu, S., Hobbs, H. H., and Krieger, M. (1996). Identification of scavenger receptor SR-BI as a high density lipoprotein receptor. Science 271, 518–520. doi: 10.1126/science.271.5248.518
Arenas, M. I., Lobo, M. V. T., Caso, E., Huerta, L., Paniagua, R., and Martıìn-Hidalgo, M. A. (2004). Normal and pathological human testes express hormone-sensitive lipase and the lipid receptors CLA-1/SR-BI and CD36. Hum. Pathol. 35, 34–42. doi: 10.1016/j.humpath.2003.08.015
Baranova, I. N., Vishnyakova, T. G., Bocharov, A. V., Kurlander, R., Chen, Z., Kimelman, M. L., et al. (2005). Serum amyloid A binding to CLA-1 (CD36 and LIMPII analogous-1) mediates serum amyloid A protein-induced activation of ERK1/2 and p38 mitogen-activated protein kinases. J. Biol. Chem. 280, 8031–8040. doi: 10.1074/jbc.M405009200
Calvo, D., Gómez-Coronado, D., Lasunción, M. A., and Vega, M. A. (1997). CLA-1 is an 85-kD plasma membrane glycoprotein that acts as a high-affinity receptor for both native (HDL, LDL, and VLDL) and modified (OxLDL and AcLDL) lipoproteins. Arterioscler. Thromb. Vasc. Biol. 17, 2341–2349. doi: 10.1161/01.ATV.17.11.2341
Cao, W., Ng, K. K., Corbin, I., Zhang, Z., Ding, L., Chen, J., et al. (2009). Synthesis and evaluation of a stable bacteriochlorophyll-analog and its incorporation into high-density lipoprotein nanoparticles for tumor imaging. Bioconjug. Chem. 20, 2023–2031. doi: 10.1021/bc900404y
Cao, W. M., Murao, K., Imachi, H., Yu, X., Abe, H., Yamauchi, A., et al. (2004). A mutant high-density lipoprotein receptor inhibits proliferation of human breast cancer cells. Cancer Res. 64, 1515–1520. doi: 10.1158/0008-5472.CAN-03-0675
Cruz, P. M., Mo, H., McConathy, W. J., Sabnis, N., and Lacko, A. G. (2013). The role of cholesterol metabolism and cholesterol transport in carcinogenesis: a review of scientific findings, relevant to future cancer therapeutics. Front. Pharmacol. 4:119. doi: 10.3389/fphar.2013.00119
Cui, L., Lin, Q., Jin, C. S., Jiang, W., Huang, H., Ding, L., et al. (2015). A PEGylation-free biomimetic porphyrin nanoplatform for personalized cancer theranostics. ACS Nano 9, 4484–4495. doi: 10.1021/acsnano.5b01077
de Gonzalo-Calvo, D., Lopez-Vilaro, L., Nasarre, L., Perez-Olabarria, M., Vazquez, T., Escuin, D., et al. (2015). Intratumor cholesteryl ester accumulation is associated with human breast cancer proliferation and aggressive potential: a molecular and clinicopathological study. BMC Cancer 15:460. doi: 10.1186/s12885-015-1469-5
Dessì, S., Batetta, B., Pulisci, D., Spano, O., Anchisi, C., Tessitore, L., et al. (1994). Cholesterol content in tumor tissues is inversely associated with high-density lipoprotein cholesterol in serum in patients with gastrointestinal cancer. Cancer 73, 253–258. doi: 10.1002/1097-0142(19940115)73:2<253::AID-CNCR2820730204>3.0.CO;2-F
Devi, G. R. (2006). siRNA-based approaches in cancer therapy. Cancer Gene Ther. 13, 819–829. doi: 10.1038/sj.cgt.7700931
Ding, Y., Wang, Y., Opoku-Damoah, Y., Wang, C., Shen, L., Yin, L., et al. (2015). Dual-functional bio-derived nanoparticulates for apoptotic antitumor therapy. Biomaterials 72, 90–103. doi: 10.1016/j.biomaterials.2015.08.051
Ding, Y., Wang, Y., Zhou, J., Gu, X., Wang, W., Liu, C., et al. (2014). Direct cytosolic siRNA delivery by reconstituted high density lipoprotein for target-specific therapy of tumor angiogenesis. Biomaterials 35, 7214–7227. doi: 10.1016/j.biomaterials.2014.05.009
Fletcher, N. F., Yang, J. P., Farquhar, M. J., Hu, K., Davis, C., He, Q., et al. (2010). Hepatitis C virus infection of neuroepithelioma cell lines. Gastroenterology 139, 1365–1374. doi: 10.1053/j.gastro.2010.06.008
Gillies, R. J., Schornack, P. A., Secomb, T. W., and Raghunand, N. (1999). Causes and effects of heterogeneous perfusion in tumors. Neoplasia 1, 197–207. doi: 10.1038/sj.neo.7900037
Hanahan, D., and Weinberg, R. A. (2000). The hallmarks of cancer. Cell 100, 57–70. doi: 10.1016/S0092-8674(00)81683-9
Hrzenjak, A., Reicher, H., Wintersperger, A., Steinecker-Frohnwieser, B., Sedlmayr, P., Schmidt, H., et al. (2004). Inhibition of lung carcinoma cell growth by high density lipoprotein-associated alpha-tocopheryl-succinate. Cell. Mol. Life Sci. 61, 1520–1530. doi: 10.1007/s00018-004-4101-4
Husemann, J., and Silverstein, S. C. (2001). Expression of scavenger receptor class B, type I, by astrocytes and vascular smooth muscle cells in normal adult mouse and human brain and in Alzheimer’s disease brain. Am. J. Pathol. 158, 825–832. doi: 10.1016/S0002-9440(10)64030-8
Imachi, H., Murao, K., Cao, W., Tada, S., Taminato, T., Wong, N. C., et al. (2003). Expression of human scavenger receptor B1 on and in human platelets. Arterioscler. Thromb. Vasc. Biol. 23, 898–904. doi: 10.1161/01.ATV.0000067429.46333.7B
Imachi, H., Murao, K., Sayo, Y., Hosokawa, H., Sato, M., Niimi, M., et al. (1999). Evidence for a potential role for HDL as an important source of cholesterol in human adrenocortical tumors via the CLA-1 pathway. Endocr. J. 46, 27–34. doi: 10.1507/endocrj.46.27
Ji, Y., Jian, B., Wang, N., Sun, Y., Moya, M. L., Phillips, M. C., et al. (1997). Scavenger receptor BI promotes high density lipoprotein-mediated cellular cholesterol efflux. J. Biol. Chem. 272:20982. doi: 10.1074/jbc.272.34.20982
Julovi, S. M., Xue, A., Thanh, L. T., Gill, A. J., Bulanadi, J. C., Patel, M., et al. (2016). Apolipoprotein A-II plus lipid emulsion enhance cell growth via SR-B1 and target pancreatic cancer in vitro and in vivo. PLoS ONE 11:e0151475. doi: 10.1371/journal.pone.0151475
Kingwell, B. A., and Chapman, M. J. (2013). Future of high-density lipoprotein infusion therapies: potential for clinical management of vascular disease. Circulation 128, 1112–1121. doi: 10.1161/CIRCULATIONAHA.113.002683
Krieger, M. (1999). Charting the fate of the “good cholesterol”: identification and characterization of the high-density lipoprotein receptor SR-BI. Annu. Rev. Biochem. 68, 523–528. doi: 10.1146/annurev.biochem.68.1.523
Lacko, A. G., Nair, M., Paranjape, S., Johnson, S., and McConathy, W. J. (2002). High density lipoprotein complexes as delivery vehicles for anticancer drugs. Anticancer Res. 22, 2045–2049.
Lee, J.-C., Kong, B.-J., Shin, J. Y., Shin, J., Oh, J.-S., Cho, J. Y., et al. (2015). A pH-responsive high density lipoprotein-like nanoparticle of epothilone B. Biotechnol. Bioprocess. Eng. 20, 249–258. doi: 10.1007/s12257-014-0368-y
Leon, C. G., Locke, J. A., Adomat, H. H., Etinger, S. L., Twiddy, A. L., Neumann, R. D., et al. (2010). Alterations in cholesterol regulation contribute to the production of intratumoral androgens during progression to castration-resistant prostate cancer in a mouse xenograft model. Prostate 70, 390–400. doi: 10.1002/pros.21072
Liadaki, K. N., Liu, T., Xu, S., Ishida, B. Y., Duchateaux, P. N., Krieger, J. P., et al. (2000). Binding of high density lipoprotein (HDL) and discoidal reconstituted HDL to the HDL receptor scavenger receptor class B type I. Effect of lipid association and APOA-I mutations on receptor binding. J. Biol. Chem. 275, 21262–21271. doi: 10.1074/jbc.M002310200
Lin, Q., Chen, J., Jin, H., Ng, K. K., Yang, M., Cao, W., et al. (2012). Efficient systemic delivery of siRNA by using high-density lipoprotein-mimicking peptide lipid nanoparticles. Nanomedicine (Lond) 7, 1813–1828. doi: 10.2217/nnm.12.73
Lin, Q., Chen, J., Ng, K. K., Cao, W., Zhang, Z., and Zheng, G. (2014a). Imaging the cytosolic drug delivery mechanism of HDL-like nanoparticles. Pharm. Res. 31, 1438–1449. doi: 10.1007/s11095-013-1046-z
Lin, Q., Jin, C. S., Huang, H., Ding, L., Zhang, Z., Chen, J., et al. (2014b). Nanoparticle-enabled, image-guided treatment planning of target specific RNAi therapeutics in an orthotopic prostate cancer model. Small 10, 3072–3082. doi: 10.1002/smll.201303842
Lobo, M. V., Huerta, L., Ruiz-Velasco, N., Teixeiro, E., de la Cueva, P., Celdrán, A., et al. (2001). Localization of the lipid receptors CD36 and CLA-1/SR-BI in the human gastrointestinal tract: towards the identification of receptors mediating the intestinal absorption of dietary lipids. J. Histochem. Cytochem. 49, 1253–1260. doi: 10.1177/002215540104901007
Lu, H., Zhang, H., Zhang, D., Lu, H., and Ma, D. (2015). A biocompatible reconstituted high-density lipoprotein nano-system as a probe for lung cancer detection. Med. Sci. Monit. 21, 2726–2733. doi: 10.12659/MSM.895255
McConathy, W. J., Nair, M. P., Paranjape, S., Mooberry, L., and Lacko, A. G. (2008). Evaluation of synthetic/reconstituted high-density lipoproteins as delivery vehicles for paclitaxel. Anticancer Drugs 19, 183–188. doi: 10.1097/CAD.0b013e3282f1da86
McConathy, W. J., Paranjape, S., Mooberry, L., Buttreddy, S., Nair, M., and Lacko, A. G. (2011). Validation of the reconstituted high-density lipoprotein (rHDL) drug delivery platform using dilauryl fluorescein (DLF). Drug Deliv. Transl. Res. 1, 113–120. doi: 10.1007/s13346-010-0012-0
Mooberry, L. K., Nair, M., Paranjape, S., McConathy, W. J., and Lacko, A. G. (2010). Receptor mediated uptake of paclitaxel from a synthetic high density lipoprotein nanocarrier. J. Drug Target. 18, 53–58. doi: 10.3109/10611860903156419
Mulik, R. S., Bing, C., Ladouceur-Wodzak, M., Munaweera, I., Chopra, R., and Corbin, I. R. (2016). Localized delivery of low-density lipoprotein docosahexaenoic acid nanoparticles to the rat brain using focused ultrasound. Biomaterials 83, 257–268. doi: 10.1016/j.biomaterials.2016.01.021
Ng, K. K., Lovell, J. F., Vedadi, A., Hajian, T., and Zheng, G. (2013). Self-assembled porphyrin nanodiscs with structure-dependent activation for phototherapy and photodiagnostic applications. ACS Nano 7, 3484–3490. doi: 10.1021/nn400418y
Ng, K. K., Lovell, J. F., and Zheng, G. (2011). Lipoprotein-inspired nanoparticles for cancer theranostics. Acc. Chem. Res. 44, 1105–1113. doi: 10.1021/ar200017e
Nichols, J. W., and Bae, Y. H. (2012). Odyssey of a cancer nanoparticle: from injection site to site of action. Nano Today 7, 606–618. doi: 10.1016/j.nantod.2012.10.010
Oehler, V. G., Yeung, K. Y., Choi, Y. E., Bumgarner, R. E., Raftery, A. E., and Radich, J. P. (2009). The derivation of diagnostic markers of chronic myeloid leukemia progression from microarray data. Blood 114, 3292–3298. doi: 10.1182/blood-2009-03-212969
Oh, Y. K., and Park, T. G. (2009). siRNA delivery systems for cancer treatment. Adv. Drug Deliv. Rev. 61, 850–862. doi: 10.1016/j.addr.2009.04.018
Pecot, C. V., Calin, G. A., Coleman, R. L., Lopez-Berestein, G., and Sood, A. K. (2011). RNA interference in the clinic: challenges and future directions. Nat. Rev. Cancer 11, 59–67. doi: 10.1038/nrc2966
Petrocca, F., and Lieberman, J. (2011). Promise and challenge of RNA interference-based therapy for cancer. J. Clin. Oncol. 29, 747–754. doi: 10.1200/JCO.2009.27.6287
Pilon, A., Martin, G., Bultel-Brienne, S., Junquero, D., Delhon, A., Fruchart, J.-C., et al. (2003). Regulation of the scavenger receptor BI and the LDL receptor by activators of aldosterone production, angiotensin II and PMA, in the human NCI-H295R adrenocortical cell line. Biochim. Biophys. Acta. 1631, 218–228. doi: 10.1016/s1388-1981(03)00020-9
Pussinen, P. J., Karten, B., Wintersperger, A., Reicher, H., McLean, M., Malle, E., et al. (2000). The human breast carcinoma cell line HBL-100 acquires exogenous cholesterol from high-density lipoprotein via CLA-1 (CD-36 and LIMPII analogous 1)-mediated selective cholesteryl ester uptake. Biochem. J. 349, 559–566. doi: 10.1042/bj3490559
Qian, Y., Jin, H., Qiao, S., Dai, Y., Huang, C., Lu, L., et al. (2016). Targeting dendritic cells in lymph node with an antigen peptide-based nanovaccine for cancer immunotherapy. Biomaterials 98, 171–183. doi: 10.1016/j.biomaterials.2016.05.008
Rao, R. M. (2002). Differential regulation of steroid hormone biosynthesis in R2C and MA-10 Leydig tumor cells: role of SR-B1-mediated selective cholesteryl ester transport. Biol. Reprod. 68, 114–121. doi: 10.1095/biolreprod.102.007518
Reboul, E., Klein, A., Bietrix, F., Gleize, B., Malezet-Desmoulins, C., Schneider, M., et al. (2006). Scavenger receptor class B type I (SR-BI) is involved in vitamin E transport across the enterocyte. J. Biol. Chem. 281, 4739–4745. doi: 10.1074/jbc.M509042200
Rodrigueza, W. V., Thuahnai, S. T., Temel, R. E., Lund-Katz, S., Phillips, M. C., and Williams, D. L. (1999). Mechanism of scavenger receptor class B type I-mediated selective uptake of cholesteryl esters from high density lipoprotein to adrenal cells. J. Biol. Chem. 274, 20344–20350. doi: 10.1074/jbc.274.29.20344
Schorghofer, D., Kinslechner, K., Preitschopf, A., Schutz, B., Rohrl, C., Hengstschlager, M., et al. (2015). The HDL receptor SR-BI is associated with human prostate cancer progression and plays a possible role in establishing androgen independence. Reprod. Biol. Endocrinol. 13:88. doi: 10.1186/s12958-015-0087-z
Shahzad, M. M. K., Mangala, L. S., Han, H. D., Lu, C., Bottsford-Miller, J., Nishimura, M., et al. (2011). Targeted delivery of small interfering RNA using reconstituted high-density lipoprotein nanoparticles. Neoplasia 13, 309–319. doi: 10.1593/neo.101372
Shin, J. Y., Yang, Y., Heo, P., Lee, J. C., Kong, B., Cho, J. Y., et al. (2012). pH-responsive high-density lipoprotein-like nanoparticles to release paclitaxel at acidic pH in cancer chemotherapy. Int. J. Nanomedicine 7, 2805–2816. doi: 10.2147/IJN.S29817
Simonsen, J. B. (2016). Evaluation of reconstituted high-density lipoprotein (rHDL) as a drug delivery platform – a detailed survey of rHDL particles ranging from biophysical properties to clinical implications. Nanomedicine doi: 10.1016/j.nano.2016.05.009 [Epub ahead of print].
Su, F., Kozak, K. R., Imaizumi, S., Gao, F., Amneus, M. W., Grijalva, V., et al. (2010). Apolipoprotein A-I (apoA-I) and apoA-I mimetic peptides inhibit tumor development in a mouse model of ovarian cancer. Proc. Natl. Acad. Sci. U.S.A. 107, 19997–20002. doi: 10.1073/pnas.1009010107
Swyer, G. I. M. (1942). The cholesterol content of normal and enlarged prostates. Cancer Res. 2, 372–375.
Thaxton, C. S., Rink, J. S., Naha, P. C., and Cormode, D. P. (2016). Lipoproteins and lipoprotein mimetics for imaging and drug delivery. Adv. Drug Deliv. Rev doi: 10.1016/j.addr.2016.04.020 [Epub ahead of print].
Thuahnai, S. T., Lund-Katz, S., Anantharamaiah, G. M., Williams, D. L., and Phillips, M. C. (2003). A quantitative analysis of apolipoprotein binding to SR-BI: multiple binding sites for lipid-free and lipid-associated apolipoproteins. J. Lipid Res. 44, 1132–1142. doi: 10.1194/jlr.M200429-JLR200
Thysell, E., Surowiec, I., Hörnberg, E., Crnalic, S., Widmark, A., Johansson, A. I., et al. (2010). Metabolomic characterization of human prostate cancer bone metastases reveals increased levels of cholesterol. PLoS ONE 5:e14175. doi: 10.1371/journal.pone.0014175
Tripathy, S., Vinokour, E., McMahon, K. M., Volpert, O. V., and Thaxton, C. S. (2014). High-density lipoprotein nanoparticles deliver RNAi to endothelial cells to inhibit angiogenesis. Part. Part. Syst. Charact. 31, 1141–1150. doi: 10.1002/ppsc.201400036
Twiddy, A. L., Cox, M. E., and Wasan, K. M. (2012). Knockdown of scavenger receptor class B type I reduces prostate specific antigen secretion and viability of prostate cancer cells. Prostate 72, 955–965. doi: 10.1002/pros.21499
Vishnyakova, T. G., Bocharov, A. V., Baranova, I. N., Chen, Z., Remaley, A. T., Csako, G., et al. (2003). Binding and internalization of lipopolysaccharide by Cla-1, a human orthologue of rodent scavenger receptor B1. J. Biol. Chem. 278, 22771–22780. doi: 10.1074/jbc.M211032200
Wadsack, C., Hirschmugl, B., Hammer, A., Levak-Frank, S., Kozarsky, K. F., Sattler, W., et al. (2003). Scavenger receptor class B, type I on non-malignant and malignant human epithelial cells mediates cholesteryl ester-uptake from high density lipoproteins. Int. J. Biochem. Cell Biol. 35, 441–454. doi: 10.1016/S1357-2725(02)00272-8
Whitehead, K. A., Langer, R., and Anderson, D. G. (2009). Knocking down barriers: advances in siRNA delivery. Nat. Rev. Drug Discov. 8, 129–138. doi: 10.1038/nrd2742
Wolfrum, C., Shi, S., Jayaprakash, K. N., Jayaraman, M., Wang, G., Pandey, R. K., et al. (2007). Mechanisms and optimization of in vivo delivery of lipophilic siRNAs. Nat. Biotechnol. 25, 1149–1157. doi: 10.1038/nbt1339
Xu, S., Laccotripe, M., Huang, X., Rigotti, A., Zannis, V. I., and Krieger, M. (1997). Apolipoproteins of HDL can directly mediate binding to the scavenger receptor SR-BI, an HDL receptor that mediates selective lipid uptake. J. Lipid Res. 38, 1289–1298.
Yang, M., Chen, J., Cao, W., Ding, L., Ng, K. K., Jin, H., et al. (2011a). Attenuation of nontargeted cell-kill using a high-density lipoprotein-mimicking peptide-phospholipid nanoscaffold. Nanomedicine (Lond) 6, 631–641. doi: 10.2217/nnm.11.10
Yang, M., Jin, H., Chen, J., Ding, L., Ng, K. K., Lin, Q., et al. (2011b). Efficient cytosolic delivery of siRNA using HDL-mimicking nanoparticles. Small 7, 568–573. doi: 10.1002/smll.201001589
Yang, S., Damiano, M. G., Zhang, H., Tripathy, S., Luthi, A. J., Rink, J. S., et al. (2013). Biomimetic, synthetic HDL nanostructures for lymphoma. Proc. Natl. Acad. Sci. U.S.A. 110, 2511–2516. doi: 10.1073/pnas.1213657110
Yuan, B., Wu, C., Wang, X., Wang, D., Liu, H., Guo, L., et al. (2016). High scavenger receptor class B type I expression is related to tumor aggressiveness and poor prognosis in breast cancer. Tumour Biol. 37, 3581–3588. doi: 10.1007/s13277-015-4141-4
Yuan, Y., Wang, W., Wang, B., Zhu, H., Zhang, B., and Feng, M. (2013). Delivery of hydrophilic drug doxorubicin hydrochloride-targeted liver using apoAI as carrier. J. Drug Target. 21, 367–374. doi: 10.3109/1061186X.2012.757769
Zamanian-Daryoush, M., and DiDonato, J. A. (2015). Apolipoprotein A-I and Cancer. Front. Pharmacol. 6:265. doi: 10.3389/fphar.2015.00265
Zhang, F., Li, M., Su, Y., Zhou, J., and Wang, W. (2016). A dual-targeting drug co-delivery system for tumor chemo- and gene combined therapy. Mater. Sci. Eng. C Mater. Biol. Appl. 64, 208–218. doi: 10.1016/j.msec.2016.03.083
Zhang, Z., Cao, W., Jin, H., Lovell, J. F., Yang, M., Ding, L., et al. (2009). Biomimetic nanocarrier for direct cytosolic drug delivery. Angew. Chem. Int. Ed. Engl. 48, 9171–9175. doi: 10.1002/anie.200903112
Zhang, Z., Chen, J., Ding, L., Jin, H., Lovell, J. F., Corbin, I. R., et al. (2010). HDL-mimicking peptide-lipid nanoparticles with improved tumor targeting. Small 6, 430–437. doi: 10.1002/smll.200901515
Zheng, G., Chen, J., Stefflova, K., Jarvi, M., Li, H., and Wilson, B. C. (2007). Photodynamic molecular beacon as an activatable photosensitizer based on protease-controlled singlet oxygen quenching and activation. Proc. Natl. Acad. Sci. U.S.A. 104, 8989–8994. doi: 10.1073/pnas.0611142104
Zheng, Y., Liu, Y., Jin, H., Pan, S., Qian, Y., Huang, C., et al. (2013). Scavenger receptor B1 is a potential biomarker of human nasopharyngeal carcinoma and its growth is inhibited by HDL-mimetic nanoparticles. Theranostics 3, 477–486. doi: 10.7150/thno.6617
Keywords: scavenger receptor BI, cancer imaging, cancer therapy, biomarkers, HDL, nanomimetics
Citation: Rajora MA and Zheng G (2016) Targeting SR-BI for Cancer Diagnostics, Imaging and Therapy. Front. Pharmacol. 7:326. doi: 10.3389/fphar.2016.00326
Received: 24 June 2016; Accepted: 06 September 2016;
Published: 27 September 2016.
Edited by:
Colby Shad Thaxton, Northwestern University, USAReviewed by:
Yongmei Song, Peking Union Medical College, ChinaMaria Rosa Ciriolo, University of Rome Tor Vergata, Italy
Copyright © 2016 Rajora and Zheng. This is an open-access article distributed under the terms of the Creative Commons Attribution License (CC BY). The use, distribution or reproduction in other forums is permitted, provided the original author(s) or licensor are credited and that the original publication in this journal is cited, in accordance with accepted academic practice. No use, distribution or reproduction is permitted which does not comply with these terms.
*Correspondence: Gang Zheng, Z2FuZy56aGVuZ0B1aG5yZXMudXRvcm9udG8uY2E=