- 1State Key Laboratory of Quality Research in Chinese Medicine, Institute of Chinese Medical Sciences, University of Macau, Macao, China
- 2Key Lab for Pharmacology of Ministry of Education, Department of Pharmacology, Zunyi Medical College, Zunyi, China
Dihydrotanshinone I (DHT) is a natural compound extracted from Salvia miltiorrhiza Bunge which has been widely used for treating cardiovascular diseases. However, its role in atherosclerosis remains unclear. In this study, the effect of DHT on atherosclerosis were investigated using apolipoprotein E-deficient (ApoE-/-) mice and endothelial cells. In lipopolysaccharide (LPS)-stimulated human umbilical vein endothelial cells (HUVECs), DHT (10 nM) decreased lectin-like ox-LDL receptor-1 (LOX-1) and NADPH oxidase 4 (NOX4) expression, reactive oxygen species (ROS) production, NF-κB nuclear translocation, ox-LDL endocytosis and monocytes adhesion. Silence NOX4 inhibited LPS-induced LOX-1 expression, NF-κB nuclear translocation, ox-LDL endocytosis and monocytes adhesion. In ApoE-/- mice fed with an atherogenic diet, DHT (10 and 25 mg kg-1) significantly attenuated atherosclerotic plaque formation, altered serum lipid profile, decreased oxidative stress and shrunk necrotic core areas. The enhanced expression of LOX-1, NOX4, and NF-κB in aorta was also dramatically inhibited by DHT. In conclusion, these results suggested that DHT showed anti-atherosclerotic activity through inhibition of LOX-1 mediated by NOX4/NF-κB signaling pathways both in vitro and in vivo. This finding suggested that DHT might be used as a potential vascular protective candidate for the treatment of atherosclerosis.
Introduction
Atherosclerosis is considered to be a chronic inflammatory disease and plays a key role in heart attacks, strokes, and peripheral vascular diseases (Libby, 2002; Frostegard, 2013). Over the last decades, atherosclerotic cardiovascular diseases remain the leading cause of mortality worldwide (Gaziano et al., 2010). One critical event in the initiation of atherosclerosis is the ox-LDL uptake, which contributes to the atherosclerotic foam cell and plaque formation (Steinberg, 1997; Kita et al., 2001). Ox-LDL uptake is principally mediated by a variety of specific receptors including SR-A I/II, CD36, SR-BI, etc. (Li and Glass, 2002; Hu et al., 2010). Recently, LOX-1 has been identified as the main endothelial receptor for ox-LDL (Sawamura et al., 1997; Chen et al., 2002). LOX-1 is a 50-kD transmembrane protein highly expressed on macrophages, vascular smooth muscle cells, and especially endothelial cells, which plays important roles in the pathogenesis of diabetic cardiovascular complications, myocardial ischemia, and atherosclerosis (Lu and Mehta, 2011; Yan et al., 2011; Xu et al., 2013b). Disturbance of LOX-1 mediated signaling pathways has been proposed as a potential strategy for the anti-atherosclerotic drug discovery (Sawamura et al., 1997; Chen et al., 2002, 2007). Although a variety of cells, including monocytes, dendritic cells, lymphocytes, eosinophils, mast cells and smooth muscle cells, contribute to atherogenesis (Moore et al., 2013), endothelial dysfunction is considered to be an early marker and played important roles in the pathophysiology of atherosclerosis (Davignon and Ganz, 2004). ROS, an important second messenger, is linked to many vascular diseases including atherosclerosis (Vara and Pula, 2014). NOX4 is an important source of ROS in endothelial cells (Ray and Shah, 2005). However, the role of NOX4 in atherosclerosis is controversial (Hu et al., 2008; Zhao et al., 2014). Furthermore, the TLRs-NF-κB pathway has been reported to participate in the anti-atherosclerotic effect of several natural products such as quercetin, pycnogenol, and procyanidins (Martinez-Micaelo et al., 2012; Luo et al., 2015; Bhaskar et al., 2016).
Salvia miltiorrhiza Bunge (Danshen) is a famous medical herb with a long history of clinical application for treating cardiovascular diseases, cancer, and osteoporosis (Cao et al., 2012; Chen et al., 2014; Guo et al., 2014). Its ingredients have been widely explored since last century. Two groups of ingredients, the hydrophilic phenolic acids and the lipophilic tanshinones, have been identified as the main bioactive components. Tanshinones are a group of abietane diterpenes composed of four rings. Totally, more than 50 tanshinones have been isolated and identified since 1934 (Fan et al., 2012). However, only a few of them, such as tanshinone IIA (Xu and Liu, 2013a) and cryptotanshinone (Chen et al., 2013), have been widely investigated. DHT (Supplementary Figure S2), a tanshinone isolated from Danshen, is structurally similar to that of tanshinone IIA and cryptotanshinone. For a long time, investigation to its bioactivities was mainly focused on its cytotoxicity to a variety of tumor cells (Liu et al., 2015; Wang et al., 2015). Although increasing evidence suggested that DHT has beneficial potentials for the treatment of cardiovascular diseases (Zhang et al., 2009; Liu et al., 2010), its anti-atherosclerotic effect, either in vivo or in vitro, has not been fully established. Here, this effect was evaluated with experimental atherosclerosis model in ApoE-/- mice and HUVECs. Furthermore, the underlying mechanisms were explored as well.
Materials and Methods
Materials
DHT (purity ≥ 98% by HPLC analysis, a lipophilic diterpenes) was purchased from Chengdu Herbpurify Co. Ltd. (Chendu, China). Hoechst 33342, N-acetyl cysteine (NAC), DPI, Rot, TTFA, AA, All, NDGA, LPS (Escherichia coli serotype 055:B5, LPS), 5-(6)-carboxy-2′,7′-dichlorodihydrofluorescein diacetate (DCFH2-DA), PDTC, 2,2-Diphenyl-1-picrylhydrazyl (DPPH) and lucigenin were purchased from Sigma–Aldrich (St. Louis, MO, USA). Lipofectamine TM2000, DHE and Amplex Red were purchased from Life Technology (Grand Island, NY, USA). Antibodies for NOX4, p22-phox, TLR4, MyD88 were purchased from Santa Cruz Biotechnology (Santa Cruz, CA, USA). Antibodies for NF-κB p65, GAPDH, and Histone H3 were purchased from Cell Signaling Technology (Beverly, MA, USA). Anti-LOX-1 antibody was obtained from R&D (Minneapolis, MN, USA). The BCA protein kits were purchased from Thermo Fisher (Suwanee, GA, USA). Fraction-PREP Cell Fractionation Kit was purchased from BioVision (Milpitas, CA, USA). SiRNA for TLR4, MyD88, and NOX4 were purchased from Gene Pharma Company (Shanghai, China). Primers and other materials for real-time PCR were purchased from Sangon Biotech (Haimen, China) and TaKaRa Bio Group (Shiga, Japan). DiI-ox-LDL was purchased from Yiyuan Biotechnology (Guangzhou, China).
Kits for TC, LDL-C, HDL-C, TG, MDA, GSH, and SOD were obtained from Nanjing Jiancheng Bioengineering Research Institute (Nanjing, China).
Cell Culture
Human umbilical vein endothelial cells were cultured in Vascular Cell Basal Medium with Endothelial Cell Growth Kit-BBE at 37°C in a humidified atmosphere of 5% CO2. Before passaging cells, issue culture flasks, 96-well plates and 6-well plates were pre-coated with 0.1% gelatin. All assays were conducted using low cell passage cells (2–5 passages).
Human monocyte cell line (THP-1) obtained from ATCC (no: TIB-202, Rockville, MA, USA) was cultured in RPMI 1640 medium containing 10% fetal calf serum, 2 mM glutamine, 100 U/mL penicillin, and 100 μg/mL streptomycin. Cells were seeded at 37°C in a humidified atmosphere of 5% CO2 and 95% air.
Animal Experiment
Male ApoE-/- mice (6–8 weeks old) on C57BL/6J background and age-matched wild-type C57BL/6J controls were purchased from Beijing HFK Bioscience Co., Ltd. (Beijing, China). Mice were housed in SPF-grade animal facilities (Certificate no. SCXK 2014-0004) at Zunyi Medical College, with a 12 h light/dark cycle, at 23°C (±2°C). All animal procedures follow the NIH guide for the Care and Use of Laboratory Animals (NIH Publications No. 80-23, revised 1978), and approved by the Institutional Animal Use and Care Committee of Zunyi Medical College. Starting from 6 weeks, the mice were fed with a HCD (54.35% raw grain, 20% lard, 0.15% cholesterol, 15% sucrose, 0.5% Sodium Cholate, 10% yolk powder) for 12 weeks. All ApoE-/- mice were dosed daily via intragastric gavage with 10 and 25 mg kg-1 DHT dissolved in 0.5% CMC-Na or administered 0.5% CMC-Na alone (vehicle control) (n = 8 per group).
Immunofluorescence Assay
Cells (1 × 104 cells/well) were seeded on glass slides in 96-well plates. After LPS treatment (Sigma–Aldrich, St. Louis, MO, USA) (with or without DHT pretreatment), the slides were fixed with 4% PFA for 30 min. Then the slides were permeabilized with PBS-T (containing 0.1% Triton x-100 in PBS solution) and blocked with PBS-B (containing 4% BSA in PBS solution). After incubated with primary antibody (1:1000) and secondary antibody (1:2000), cells were stained with Hoechst 33342 in dark for 5 min. The protein location and expression were observed with IN Cell Analyzer 2000 (GE Healthcare).
Detection of Reactive Oxygen Species, Superoxide Anion (), and Hydrogen Peroxide (H2O2)
The effect of DHT on LPS-induced , H2O2, and ROS production were measured by DHE, Amplex Red, and DCFH2-DA, respectively. Briefly, after LPS treatment for 30 min, cells were washed with PBS and incubated with DHE (10 μM), Amplex Red (50 μM), or DCFH2-DA (10 μM) for 30 min in the dark at 37°C. Then, cells were washed with PBS for three times and fluorescence density was measured by Spectra Max M5 Microplate Reader (Molecular Devices, Sunnyvale, CA, USA) or by a flow cytometry using a FACSCantoTM system (BD Biosciences).
DPPH Assay
To evaluate the direct scavenging effect of DHT on free radicals in cell-free system, the DPPH system was adapted. DHT (0.01–1 μM, final concentration) was incubated with ethanol solution of DPPH (5.0 × 10-4 M). After thoroughly mixing, the solutions were kept in the dark for 20 min and the absorbency was measured at 517 nm.
Western Blotting
Treated HUVECs were washed twice with ice-cold PBS and lysed with RIPA buffer supplemented with a protease cocktail and phosphatase inhibitors. The cell lysates were separated using 8–12% SDS-PAGE and transferred onto PVDF membranes. After blocked with 5% non-fat milk in TBST (20 mM Tris-HCl, 500 mM NaCl, and 0.1% Tween 20) at room temperature for 1 h, membranes were incubated with specific primary antibodies and secondary antibodies. The protein-antibody complexes were detected by ECL Advanced Western Blot detection Kit. The intensity of the band was quantitated with Quantity One software (Bio-Rad).
Preparation of Membrane, Cytoplasmic and Nuclear Fractions
The cytoplasmic and membrane proteins were isolated using Fraction-PREP cell fractionation kit (Biovision Inc., Milpitas, CA, USA) according to the manufacturer’s instructions1.
SiRNA Transfection
Gene silencing experiment of TLR4, MyD88, and NOX4 with siRNA was performed according to our previous report (Zhao et al., 2014).
Real-Time RT-PCR
The mRNA expression of LOX-1 was determined with real-time PCR as our previous report (Zhao et al., 2014).
DiI-ox-LDL Uptake
The DiI-ox-LDL uptake assay was performed according to our previous study with minor revisions (Zhang et al., 2006). Cells (1.0 × 104/well) in 96-well plate cultured for overnight were pretreated with DHT, inhibitors, or anti-LOX-1 antibody for 1 h and then co-treated with LPS for another 24 h. After incubated with DiI-ox-LDL (1 μg/mL) for 1 h at 37°C in the dark, the cells were gently washed with PBS for three times to remove the free DiI-ox-LDL and the ox-LDL uptake was measured with IN Cell Analyzer 2000.
Monocyte-endothelial Cell Adhesion Assay
After various treatments, HUVECs were co-incubated with THP-1 cells labeled with Hoechst 33342 for 3 h in the dark at 37°C. The non-adherent THP-1 cells were washed gently with PBS. Images were obtained under random fields of each well using IN Cell Analyzer 2000.
Immunohistochemistry
Immunohistochemical analysis on aortic sinus was performed as described previously in detail (Xu et al., 2011). Sections, 8 μm thick, were used for immunohistochemical staining with LOX-1, NOX4 and phosphorylated NF-κB p65 antibodies. Color reaction was developed with diaminobenzidine.
Aorta Collection and Lesion Size Evaluation
To evaluate plaque extension, frozen sections of the aortic sinus (8 μm) were stained using Oil Red O, hematoxylin and eosin (H&E), respectively. Related experiments were performed following the method of Paigen et al. (1987).
Blood Parameters
Blood samples were collected from the orbit before the mice were killed. Serum TC, LDL-C, HDL-C, and TG were measured using standard enzymatic colorimetric assays. MDA, GSH, and SOD were detected by commercial kits.
Statistical Analysis
Twenty four ApoE-/- mice were randomly allocated to groups and equal group sizes were obtained (n = 8 per group). Data were expressed as the means ± SD. The differences between groups were analyzed using Prism 5.0 (Graph Pad Software Inc, San Diego, CA, USA) and the statistical analysis was performed by analysis of variance (one-way ANOVA) followed by Student Newman–Keuls test. p < 0.05 is considered statistically significant.
Results
DHT Inhibited LPS-Induced LOX-1 Expression
Firstly, the cytotoxic effect of DHT on HUVECs was determined by MTT assay. Results showed that DHT was cytotoxic to HUVECs at 1 μM (Supplementary Figure S1). To minimize the cytotoxic effect of DHT on cell viability, 10 nM DHT was chosen for further study. Compared with untreated HUVECs, 24 h stimulation with LPS induced significant LOX-1 expression at both mRNA (Figure 1A) and protein (Figure 1B) levels, which were dramatically inhibited by DHT pretreatment. Furthermore, immunofluorescence results showed that LPS-induced LOX-1 expression was mainly localized on the membrane, which was significantly suppressed by DHT as well (Figure 1C).
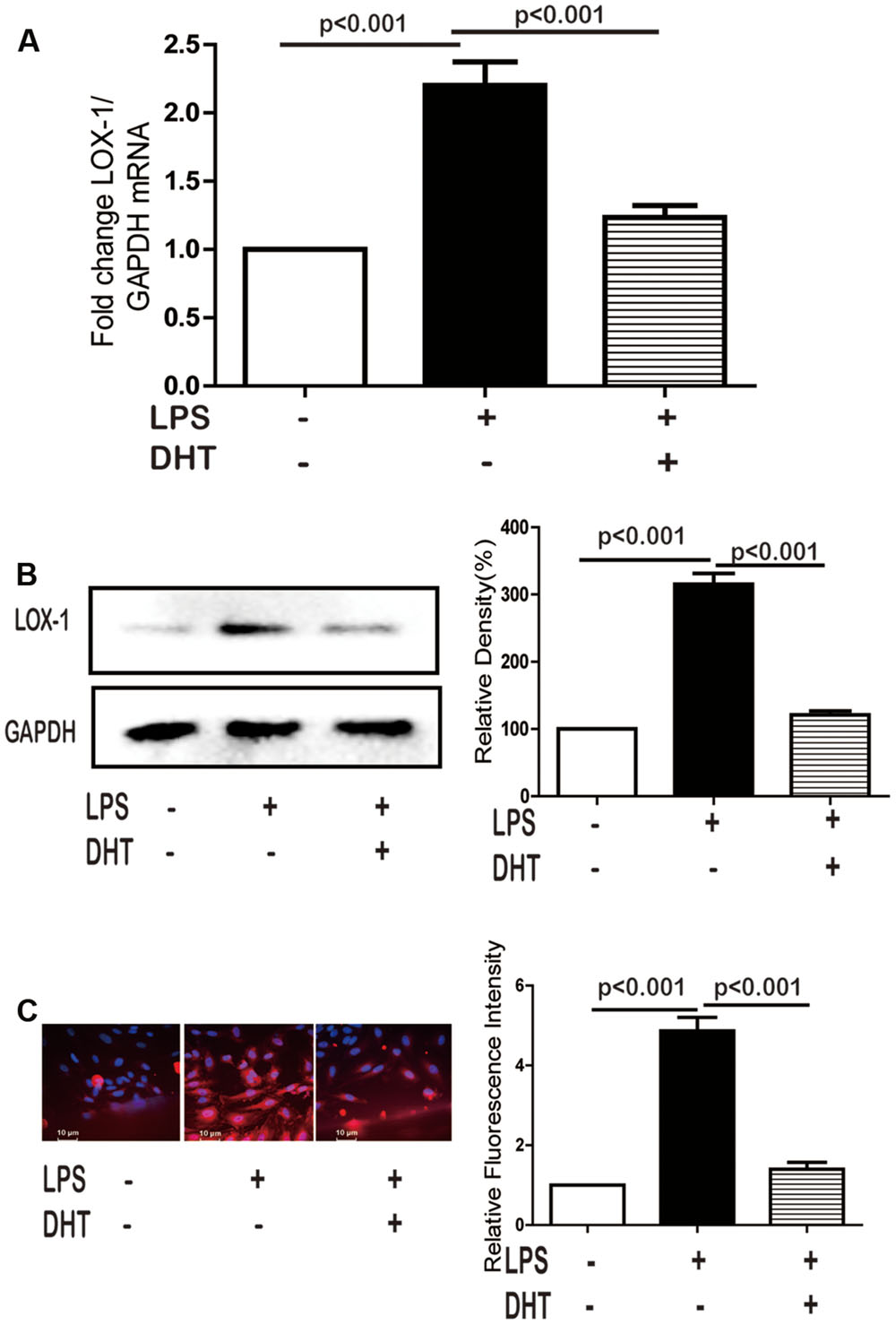
FIGURE 1. DHT inhibited LPS-induced LOX-1 expression. Cells were pretreated with DHT (10 nM) for 1 h and then stimulated with LPS (5 μg/mL) for 24 h, the LOX-1 mRNA (A), protein (B), and localization (C) were detected by real-time PCR, western blotting and immunofluorescence (60×), respectively. DHT, dihydrotanshinone I.
DHT Inhibited LPS-Induced LOX-1 Expression via TLR4/Myd88
Our previous study demonstrated that TLR4/MyD88 signal pathway was involved in LPS-induced LOX-1 expression in endothelial cells (Zhao et al., 2014). Here, LPS-induced expression of TLR4 and MyD88 was obviously inhibited by DHT (Figure 2A). Furthermore, silence of either TLR4 or MyD88 did not affect the expression of LOX-1 in the absence of LPS (Figures 2B–D). In addition, the LPS-induced expression of LOX-1 was significantly decreased in both TLR4 and MyD88 silenced cells (Figure 2E).
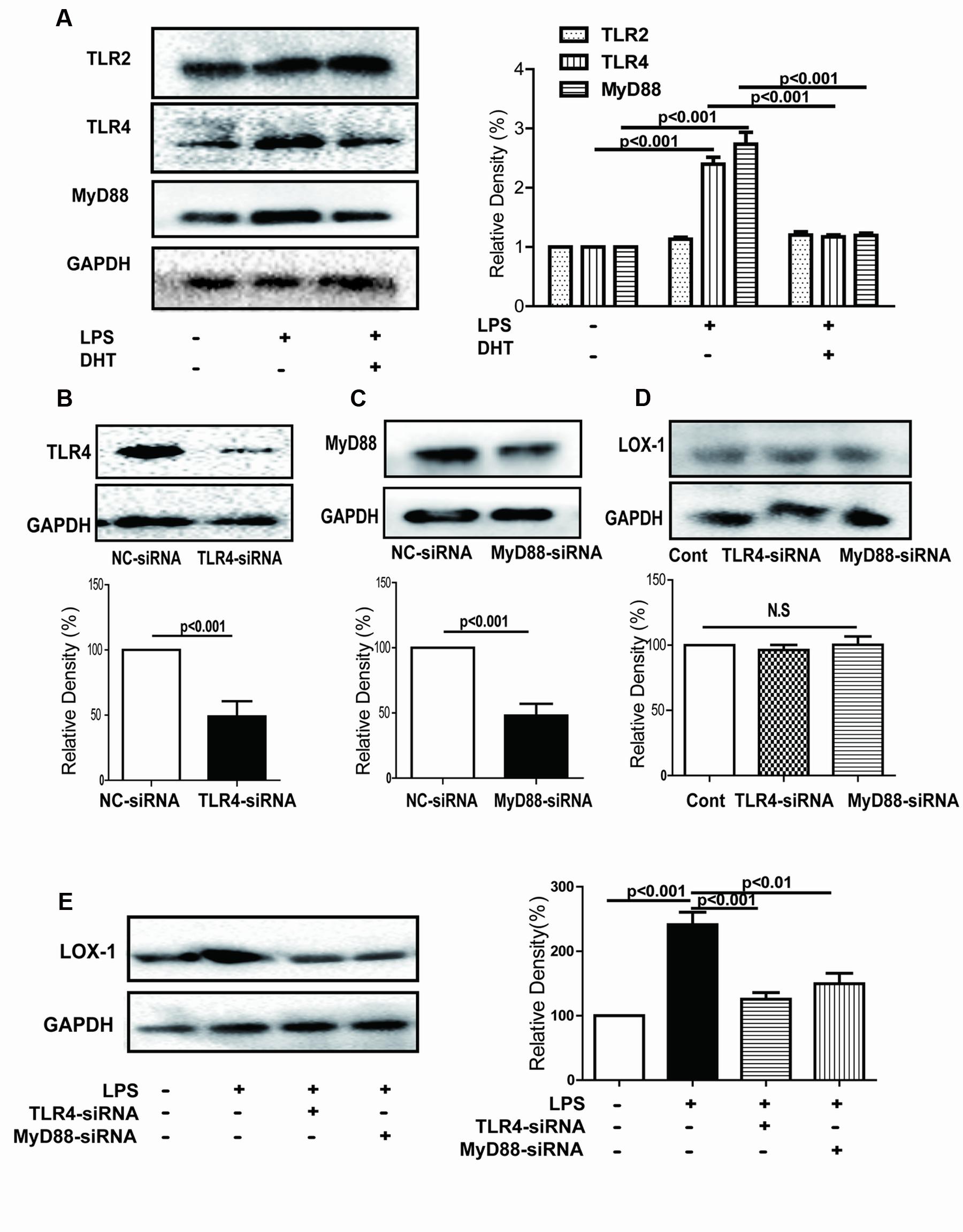
FIGURE 2. DHT inhibited LPS-induced LOX-1 expression via TLR4/MyD88. Cells were pretreated with DHT (10 nM) for 1 h and then stimulated by LPS (5 μg/mL) for 24 h, expressions of TLR2, TLR4 and MyD88 were determined by western blotting (A). Cells were transfected with siRNAs for TLR4 (B) and MyD88 (C) and then stimulated with LPS (5 μg/mL) for 24 h and the LOX-1 expression were determined by western blotting (D,E). Cont, control; NC-siRNA, negative control siRNA. DHT, dihydrotanshinone I; N.S, no significant differences.
DHT Inhibited LPS-Induced ROS Generation
LOX-1 was reported to be upregulated by oxidative stress in vascular smooth muscle cells (Sun and Chen, 2011) and endothelial cells (Zhao et al., 2014). LPS treatment for 4 h induced endothelial ROS generation as detected by DCFH2-DA (Figure 3A). Amplex Red and DHE assay showed that the generation of both H2O2 and was also significantly increased after LPS stimulation. DHT and NAC pretreatment dramatically inhibited these increases (Figures 3B,C). However, no effect of DHT on DPPH was observed (Figure 3D).
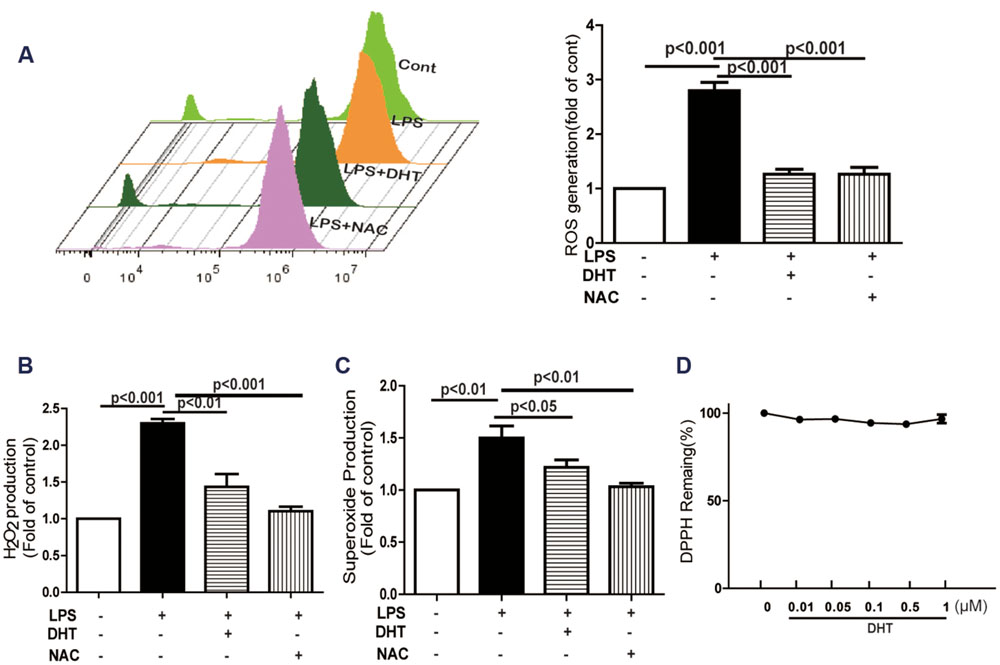
FIGURE 3. DHT inhibited LPS-induced ROS, H2O2, and production. ROS (A), H2O2 (B), and (C) production were determined by DCFH2-DA, Amplex Red and DHE respectively, after LPS (5 μg/mL) treatment for 1 h with or without DHT (10 nM) or NAC (5 mM) pretreatment for 1 h. Effect of DHT on DPPH radical was determined in a cell-free system (D). DHT, dihydrotanshinone I; ROS, reactive oxygen species; NAC, N-acetyl cysteine.
DHT Inhibited LPS-Induced LOX-1 Expression Through NOX4
LPS-induced ROS generation was significantly inhibited by DPI while NDGA, allopurinol (All), Rot, TTFA, and AA showed no obvious effect (Figure 4A). Furthermore, LPS increased NOX4 protein expression (Figure 4B) and membrane aggregation (Figure 4C) which was also inhibited by DHT. LPS-induced p22phox expression was suppressed by DHT (Figure 4D). In addition, silence NOX4 (Figure 4E) dramatically inhibited LPS-induced LOX-1 expression (Figure 4F) (Supplementary Figure S3).
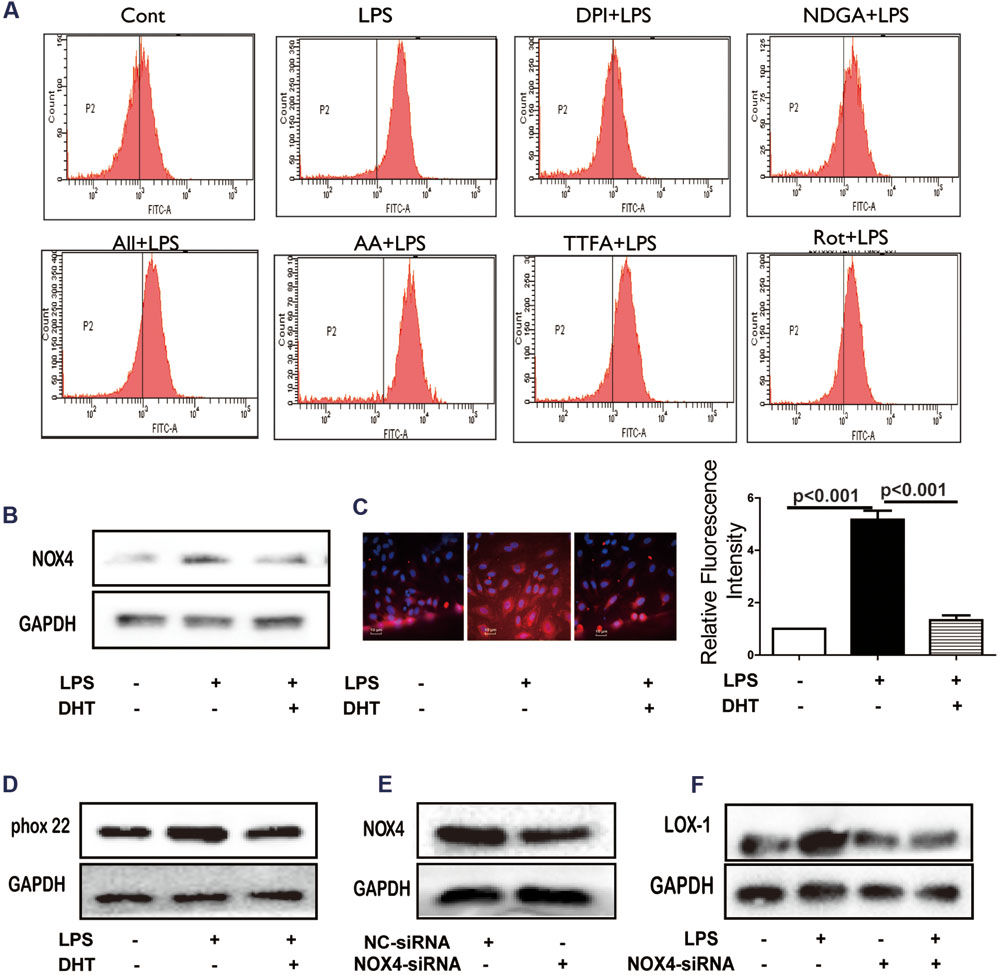
FIGURE 4. DHT inhibited LPS-induced NOX4 dependent ROS generation. Cells were treated with LPS (5 μg/mL) with or without 1 h pretreatment with DPI (1 μM), NDGA (10 μM), All (10 μM), Rot (20 μM), TTFA (10 μM) or AA (5 μM) and the ROS was determined (A). NOX4 expression and localization were determined by western blotting (B) and immunofluorescence (C) (60×). P22phox expression was determined by western blotting (D). Cells were transfected with NOX4 siRNA, NOX4 (E) and LOX-1 expression (F) were determined by western blotting. Cont, control group; DHT, dihydrotanshinone I; NC-siRNA, negative control siRNA; DPI, diphenyleneiodonium chloride; NDGA, nordihydroguaiaretic acid; All, allopurinol; AA, antimycin A; Rot, rotenone; TTFA, 2-thenoyltrifluoroacetone.
DHT Inhibited LPS-Induced LOX-1 Expression by Suppressing NF-κB Activation
NF-κB pathway plays an essential role in LPS-induced LOX-1 expression in HUVECs (Zhao et al., 2014). The NF-κB p65 in the nuclear in response to LPS was significantly increased which was remarkably suppressed by DHT treatment (Figure 5A). Furthermore, LPS-induced LOX-1 expression was inhibited by PDTC, a NF-κB inhibitor (Figure 5B). In addition, LPS-induced p65 nuclear translocation was significantly suppressed by NOX4 siRNA (Figure 5C).
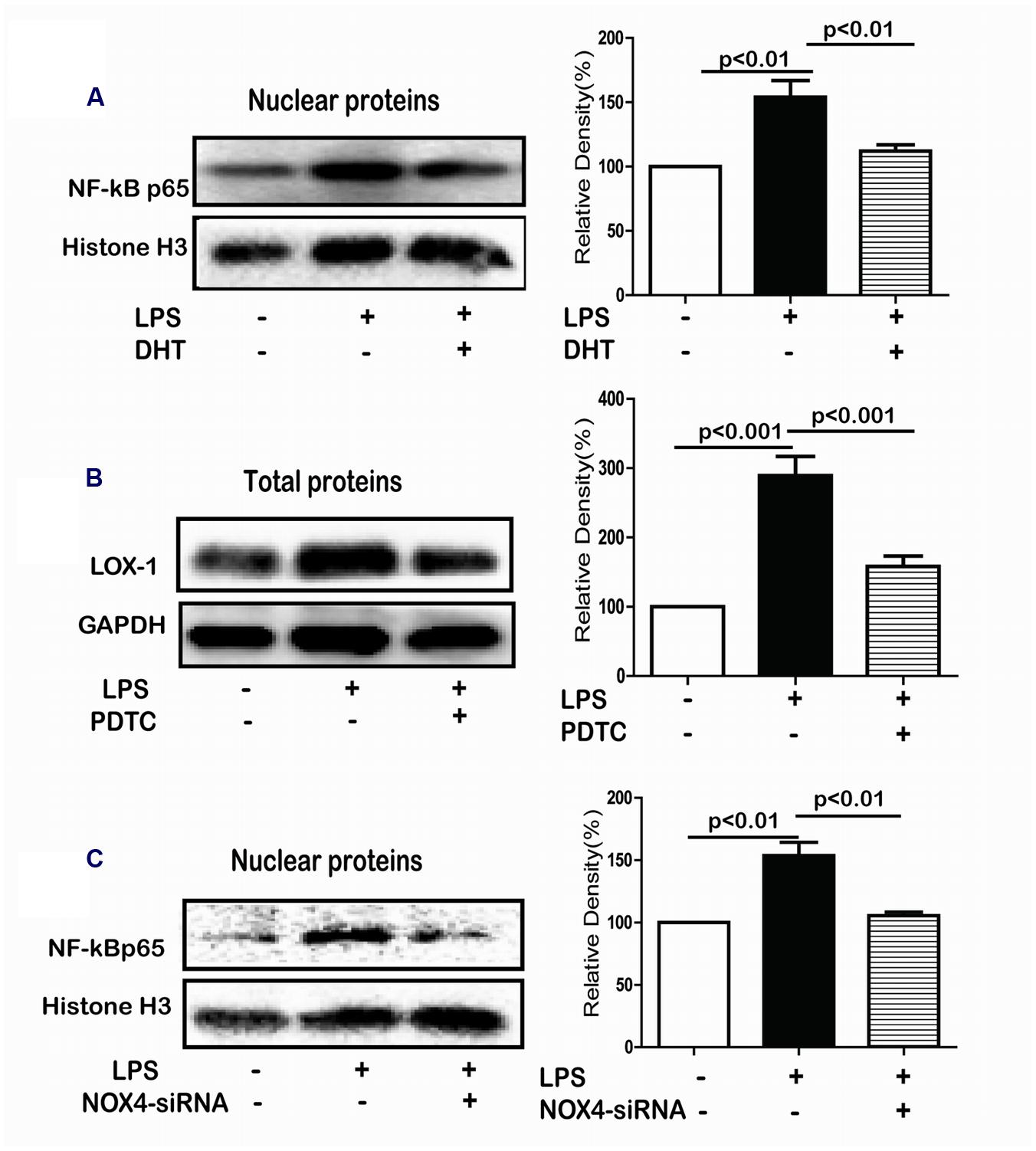
FIGURE 5. DHT inhibited LPS-induced LOX-1 expression via NF-κB. Cells were treated with LPS (5 μg/mL) for 24 h with or without pretreatment with DHT, PDTC, or NOX4 siRNA and the expression of p65 (A), LOX-1 (B), and p65 (C) was detected by western blotting. DHT, dihydrotanshinone I; PDTC, pyrrolidine dithiocarbamic acid.
DHT Inhibited LPS-Induced Ox-LDL Uptake Mediated by LOX-1
In the DiI-ox-LDL uptake assay, compared with untreated cells, LPS stimulation resulted in increased intracellular red fluorescence in stimulated endothelial cells suggesting the increase of DiI-ox-LDL internalization (Figure 6A). This increase was significantly reversed by pretreatment with DHT, NOX4 siRNA, PDTC, and anti-LOX-1 antibody.
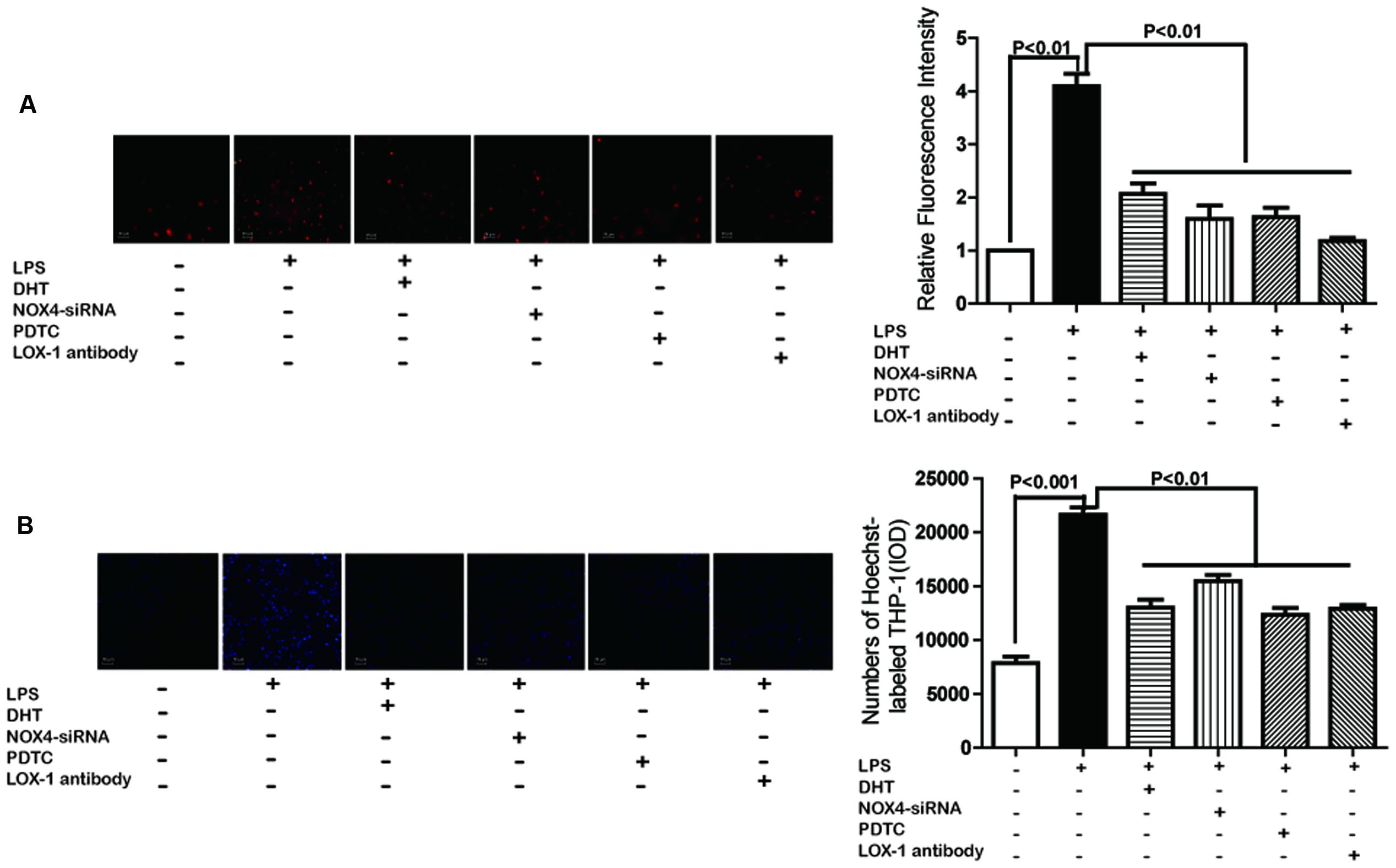
FIGURE 6. DHT inhibited LPS-induced ox-LDL uptake and monocyte adhesion mediated by LOX-1. Cells were pretreated with DHT (10 nM), siNOX4, PDTC (10 μM), or anti-LOX-1 Ab (10 μg/mL) for 1 h before LPS treatment for 24 h. Then cells were incubated with DiI-ox-LDL (5 μg/mL) and the ox-LDL uptake was measured with an inverted fluorescent microscopy (20×) (A). Cells received same treatment and were co-incubated with Hoechst 33342 labeled THP-1 cells. The attached THP-1 cells were visualized by an inverted fluorescent microscopy (20×) (B). DHT, dihydrotanshinone I; NC-siRNA, negative control siRNA; PDTC, pyrrolidine dithiocarbamic acid.
DHT Inhibited LPS-Induced THP-1 Adhesion Mediated by LOX-1
We previously reported the potential role of LOX-1 in LPS-mediated monocyte-endothelial cells adhesion (Zhao et al., 2014). In present research, LPS treatment for 24 h significantly increased THP-1 cell adhesion to endothelial cells, which was also dramatically inhibited by DHT, NOX4 siRNA, PDTC, and anti-LOX-1 antibody (Figure 6B).
DHT Ameliorated the Serum Lipid Profile in ApoE-/- Mice
As shown in Figures 7A–C, compared with the WT mice, high-fat diet induced dramatically increase in TG, TC, LDL-C, as well as HDL-C in ApoE-/- mice. Low dosage of DHT mildly decreased the lipid levels of TC and LDL-C but showed no effect on TG while at high dosage DHT dramatically inhibited TG, TC, and LDL-C. Both dosages of DHT showed no effect on HDL-C (Figure 7D).
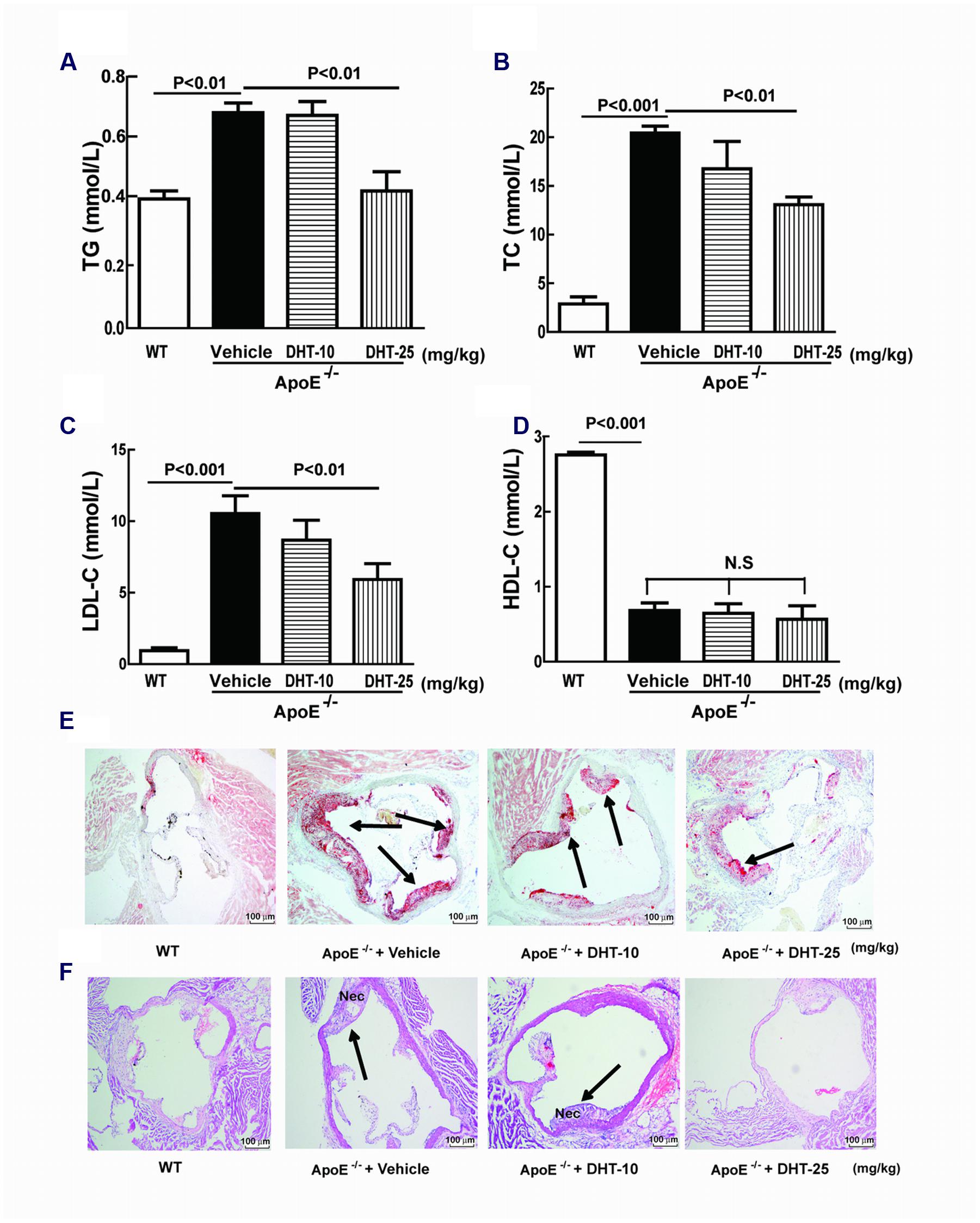
FIGURE 7. DHT ameliorated serum lipid profile and reduced atherosclerosis in ApoE-/- mice. The serum TG, TC, LDL-C, and HDL-C were measured by colorimetric assays (A–D). Aortic sinus sections were stained with Oil Red O and H&E to detect plaque sizes (E) (40×) and necrotic core areas (F) respectively (40×). DHT, dihydrotanshinone I; TC, total cholesterol; TG, triglycerides; LDL-C, LDL cholesterol; HDL-C, HDL- cholesterol; Nec, necrotic core area; WT, wild type; ApoE-/-, apolipoprotein E-deficient; N.S, no significant differences.
DHT Reduced Atherosclerotic Plaque Development in ApoE-/- Mice
Histopathological studies had showed that an atherosclerotic plaques (Oil red O-stained red area) was formed and obvious necrotic core areas (H&E-stained area) were observed in ApoE-/- mice. DHT administration significantly reduced plaque sizes as well as shrank necrotic core areas. High dosage showed more potent inhibitory effect (Figures 7E,F).
DHT Reduced Oxidative Stress in ApoE-/- Mice
As shown in Figures 8C–E, there was a slight decrease of SOD while nearly fivefolds decrease of GSH and twofolds increase of MDA in ApoE-/- mice compared to WT mice. The decreased SOD and GSH and the increased MDA levels in the serum were significantly reversed by DHT administration.
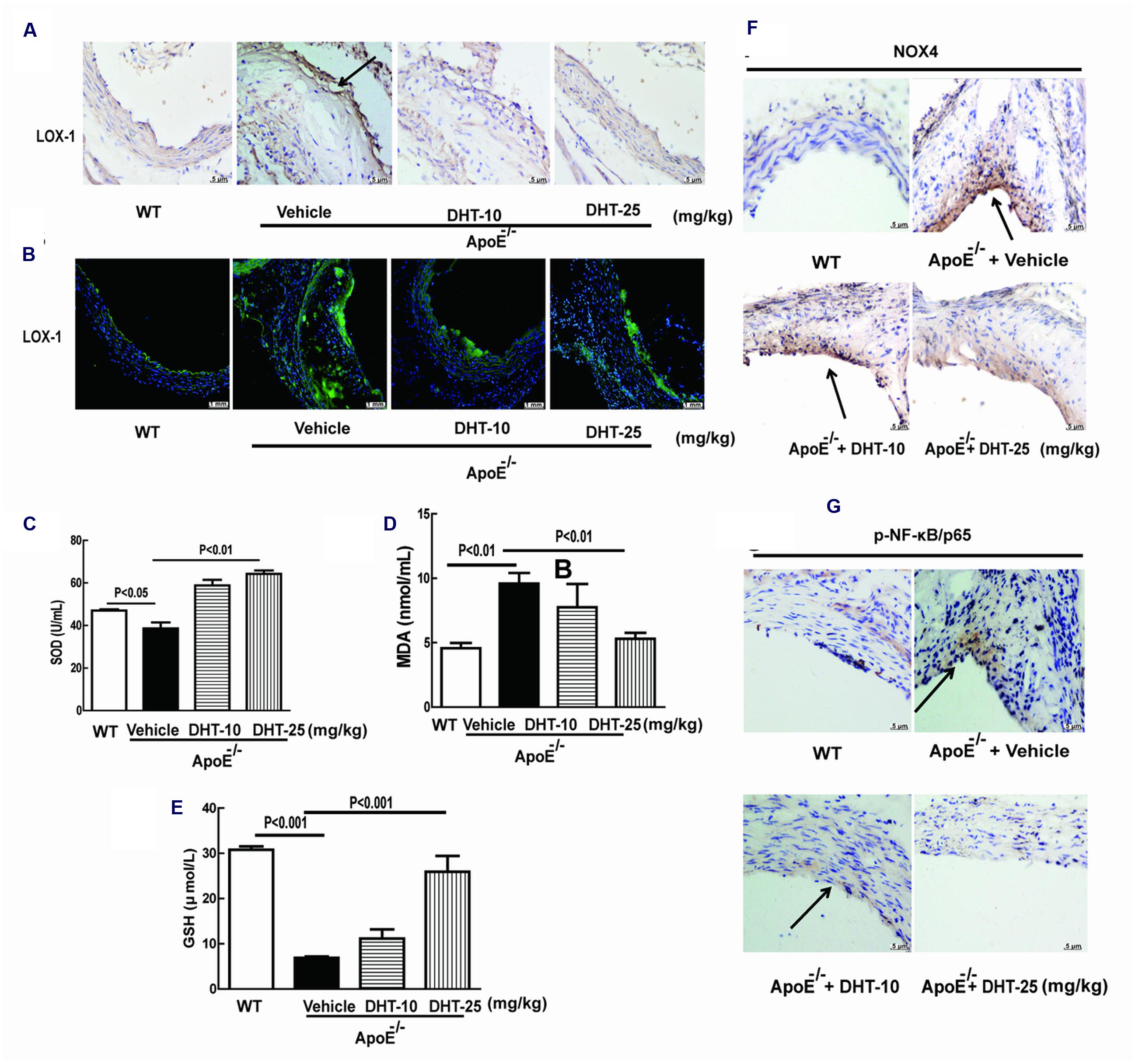
FIGURE 8. DHT reduced oxidative stress, inhibited LOX-1, NF-κB, and LOX-1 expression in ApoE-/- mice. Expression of LOX-1 (A), NOX4 (F), and NF-κB p65 (G) in the aorta was detected by immunohistochemistry. The MDA, GSH and SOD levels were detected by kits (C–E). LOX-1 expression was detected by immunofluorescence (B). DHT, dihydrotanshinone I; MDA, malondialdehyde; GSH, glutathione; SOD, superoxide dismutase; WT, wild type; ApoE-/-, apolipoprotein E-deficient.
DHT Inhibited NOX4, NF-κB and LOX-1 Expression in ApoE-/- Mice
Immunohistochemistry showed that LOX-1 was expressed at low level in aorta of WT mice but significantly increased in ApoE-/- mice, which was dramatically decreased in DHT treated mice (Figure 8A). LOX-1 was mainly expressed on the endothelial cells, which was further confirmed by immunofluorescence (Figure 8B). Furthermore, immunohistochemistry analysis of aortic sinus showed that the expression of NOX4 and phosphorylated NF-κB p65 were remarkably increased in ApoE-/- mice, which were significantly inhibited by DHT as well (Figures 8F,G).
Discussion
Herb extracts and single compounds from Salvia miltiorrhiza, Curcuma longa, Rheum undulatum, and Panax notoginseng, have been reported to regulate multiple targets that involved in the initiation and progress of atherosclerosis (Quiles et al., 2002; Fan et al., 2012; Zeng et al., 2012). Here, we showed that DHT isolated from Salvia miltiorrhiza Bunge significantly attenuated atherosclerosis through regulating LOX-1 expression both in vivo and in vitro. The main findings of this study are: (1). DHT inhibited LPS-induced LOX-1 expression through TLR4/NOX4/NF-κB pathway in endothelial cells. (2). DHT benefited atherosclerosis by inhibiting LOX-1-mediated ox-LDL endocytosis and monocyte-endothelial cells adhesion in endothelial cells. (3). DHT attenuated atherosclerosis in ApoE-/- mice through regulating LOX-1 expression.
Our previous study demonstrated that LPS induced LOX-1 expression in a TLR4/MyD88-dependent manner (Zhao et al., 2014). Here, we found that LPS-induced expression of TLR4 and MyD88 was dramatically inhibited by DHT. However, DHT showed no effect on TLR2 expression. Furthermore, silencing of TLR4 and MyD88 significantly reversed LPS-induced LOX-1 expression. Thus, DHT might inhibit LPS-induced LOX-1 expression in a TLR4/MyD88 manner.
TLR4/MyD88 functions as the upstream of ROS (Zhang et al., 2009). Cryptotanshinone, an analog of DHT, has been reported to inhibit ox-LDL- or LPS- induced endothelial ROS formation (Chen et al., 2008; Zhao et al., 2014). In present study, DHT significantly inhibited LPS-induced ROS production in endothelial cells. Further investigation showed that DHT inhibited both LPS-induced and H2O2 production but has no effect on DPPH free radical. Thus suggested that DHT may not be a ROS scavenger. Since LPS-induced ROS production was potentially inhibited by NDAPH oxidase inhibitor DPI but not by LOX inhibitor NDGA, XO inhibitor ALL, mitochondria respiratory electron-transport chain inhibitors AA, TIFA and Rot, it suggested that NADPH oxidase was the main source of ROS in response to LPS. Among the 7 NADPH oxidase isoforms NOX4 was identified as the main contributor for LPS-induced ROS in HUVECs (Mehta et al., 2006; Park et al., 2006; Zhao et al., 2014). In our study, DHT suppressed LPS-induced NOX4 expression and membrane translocation. Previous studies showed that activated TLR4/MyD88 upregulated NOX4 expression in LPS-activated cells and lead to atherosclerosis (Patel et al., 2006; Petry et al., 2006). Consistent with these findings, here we found that silence TLR4 decreased NOX4 expression in response to LPS in endothelial cells (data not shown). Furthermore, DHT inhibited LPS induced p22phox, an important NOX4 adaptor for NOX4 activation (Geiszt and Leto, 2004), expression. In addition, silence NOX4 inhibited LPS-induced LOX-1 expression. Collectively, these results suggested that DHT inhibited LPS-induced LOX-1 expression mediated by NOX4-dependent ROS.
The transcription factor NF-κB plays an essential role in regulating of various inflammatory cytokines secretion mediated by the intracellular ROS levels (Tak and Firestein, 2001). Increased nuclear translocation of NF-κB p65 by LPS was inhibited by DHT and NOX4 siRNA. Increased LOX-1 expression was suppressed by PDTC, a NF-κB inhibitor. Thus, NOX4 mediated the downregulation of LOX-1 expression by DHT through NF-κB.
Ox-LDL uptake and monocyte-endothelial cells adhesion play important roles in promoting atherosclerosis (Mestas and Ley, 2008; Di Pietro et al., 2016). Binding and endocytosis of ox-LDL is considered the primary function of LOX-1 in endothelial cells (Sawamura et al., 1997) and macrophages (Li et al., 2009). LOX-1 also act as an important adhesion molecule leading monocyte-endothelial cell adhesion (Honjo et al., 2003; Bai et al., 2005). LPS-induced ox-LDL uptake and THP-1 cells adhesion were inhibited by anti-LOX-1 antibody suggesting the key roles of LOX-1. The inhibitory effect of NOX4 siRNA, PDTC, and DHT provide evidence that DHT inhibited ox-LDL uptake and monocyte adhesion by downregulating LOX-1.
To further confirm the anti-atherosclerotic effect of DHT, a high fat diet induced atherosclerosis was established in ApoE-/- mice. Imbalance of lipid profile, formation of atherosclerotic plaque and occurrence of necrotic cores are important characteristics of atherosclerosis (Ku et al., 1985; Shahar et al., 2003; Cai et al., 2005). Oral administration of DHT for 12 weeks dramatically decreased the serum levels of TG, TC, and LDL-C suggesting that DHT might benefit atherosclerosis by decreasing lipid levels. The decreased MDA and increased SOD and GSH by DHT also suggested that it also regulated oxidative stress in vivo. The reduction of atherosclerotic plaque sizes and the shrinkage of necrotic core areas in DHT treated ApoE-/- mice provides the direct evidence for its anti-atherosclerotic effect. Furthermore, consistent with the in vitro results, expression of LOX-1, NOX4, and NF-κB in the aorta was also reduced by DHT. Thus, DHT showed anti-atherosclerotic effect in animal model.
Conclusion
In summary, as depicted in Figure 9, this study showed that natural product DHT inhibited NF-κB mediated LOX-1 expression both in vivo and in vitro through NOX4-derived ROS generation. In view of the key roles of LOX-1 in the pathogenesis of atherosclerosis, inhibition of LOX-1 contributed to the anti-atherosclerotic effect of DHT.
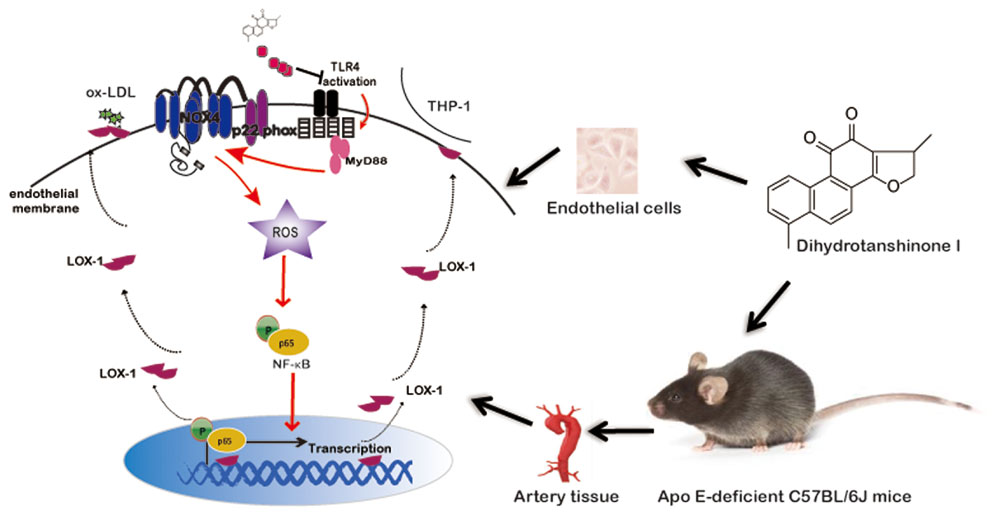
FIGURE 9. Schematic diagram illustrating the molecular mechanisms underlying the anti-atherosclerotic effects of DHT.
Author Contributions
WZ and CL are responsible for the execution of most of the experiments. HG was responsible for parts of the Western blotting. QW and JS provided critical inputs for the experiments. XC was responsible for the conceptualization, experimental design and troubleshooting, preparation of the manuscript and the financial support.
Conflict of Interest Statement
The authors declare that the research was conducted in the absence of any commercial or financial relationships that could be construed as a potential conflict of interest.
Acknowledgments
This study was supported by the Science and Technology Development Fund of Macau Special Administrative Region (039/2014/A1) and the Research Fund of University of Macau (Nos: MYRG118(Y1-L4)-ICMS13-CXP and MYRG2016-00043-ICMS-QRCM).
Supplementary Material
The Supplementary Material for this article can be found online at: http://journal.frontiersin.org/article/10.3389/fphar.2016.00418/full#supplementary-material
Abbreviations
AA, antimycin A; All, allopurinol; ApoE-/- mice, apolipoprotein E-deficient mice; CMC-Na, carboxymethyl cellulose sodium; DCFH2-DA, 5-(6)-carboxy-2′, 7′-dichlor- odihydrofluorescein diacetate; DHE, dihydroethidium; DHT, dihydrotanshinone I; DPI, diphenyleneiodonium chloride; DPPH, 2, 2-diphenyl-1-picrylhydrazyl; GSH, glutathione; HCD, high cholesterol diet; HDL-C, HDL cholesterol; H&E, haematoxylin and eosin; HUVECs, human umbilical vein endothelial cells; ICAM-1, intercellular adhesion molecule-1; LDL-C, LDL cholesterol; LOX, lipoxygenase; LOX-1, lectin-like oxidized low-density lipoprotein receptor-1; LPS, lipopolysaccharide; MDA, malondialdehyde; MTT, 3-(4, 5-dimethyl-2-thiazolyl)-2, 5-diphenyl-2H-tetrazolium bromide; NAC, N-acetyl-L-cysteine; NDGA, nordihydroguaiaretic acid; NOX4, NADPH oxidase 4; N.S, no significant differences; ox-LDL, oxidized low-density lipoprotein; PDTC, pyrrolidine dithiocarbamate; ROS, reactive oxygen species; Rot, rotenone; SOD, superoxide dismutase; TC, total cholesterol; TG, triglyceride; TTFA, 2-thenoyltrifluoroacetone; XO, xanthine oxidase.
Footnotes
References
Bai, X. C., Lu, D., Liu, A. L., Zhang, Z. M., Li, X. M., Zou, Z. P., et al. (2005). Reactive oxygen species stimulates receptor activator of NF-kappa B ligand expression in osteoblast. J. Biol. Chem. 280, 17497–17506. doi: 10.1074/jbc.M409332200
Bhaskar, S., Sudhakaran, P. R., and Helen, A. (2016). Quercetin attenuates atherosclerotic inflammation and adhesion molecule expression by modulating TLR-NF-kappaB signaling pathway. Cell. Immunol. doi: 10.1016/j.cellimm.2016.08.011 [Epub ahead of print].
Cai, J., Hatsukami, T. S., Ferguson, M. S., Kerwin, W. S., Saam, T., Chu, B., et al. (2005). In vivo quantitative measurement of intact fibrous cap and lipid-rich necrotic core size in atherosclerotic carotid plaque: comparison of high-resolution, contrast-enhanced magnetic resonance imaging and histology. Circulation 112, 3437–3444. doi: 10.1161/CIRCULATIONAHA.104.528174
Cao, W., Guo, X. W., Zheng, H. Z., Li, D. P., Jia, G. B., and Wang, J. (2012). Current progress of research on pharmacologic actions of salvianolic acid B. Chin. J. Integr. Med. 18, 316–320. doi: 10.1007/s11655-012-1052-8
Chen, M. J., Masaki, T., and Sawamura, T. (2002). LOX-1, the receptor for oxidized low-density lipoprotein identified from endothelial cells: implications in endothelial dysfunction and atherosclerosis. Pharmacol. Ther. 95, 89–100. doi: 10.1016/S0163-7258(02)00236-X
Chen, W., Lu, Y., Chen, G., and Huang, S. (2013). Molecular evidence of cryptotanshinone for treatment and prevention of human cancer. Anticancer Agents Med. Chem. 13, 979–987. doi: 10.2174/18715206113139990115
Chen, X., Andresen, B. T., Hill, M., Zhang, J., Booth, F., and Zhang, C. (2008). Role of reactive oxygen species in tumor necrosis factor-alpha induced endothelial dysfunction. Curr. Hypertens Rev. 4, 245–255. doi: 10.2174/157340208786241336
Chen, X., Guo, J., Bao, J., Lu, J., and Wang, Y. (2014). The anticancer properties of Salvia miltiorrhiza Bunge (Danshen): a systematic review. Med. Res. Rev. 34, 768–794. doi: 10.1002/med.21304
Chen, X. P., Zhang, T. T., and Du, G. H. (2007). Lectin-like oxidized low-density lipoprotein receptor-1, a new promising target for the therapy of atherosclerosis? Cardiovasc. Drug Rev. 25, 146–161. doi: 10.1111/j.1527-3466.2007.00009.x
Davignon, J., and Ganz, P. (2004). Role of endothelial dysfunction in atherosclerosis. Circulation 109, III27–III32. doi: 10.1161/01.CIR.0000131515.03336.f8
Di Pietro, N., Formoso, G., and Pandolfi, A. (2016). Physiology and pathophysiology of oxLDL uptake by vascular wall cells in atherosclerosis. Vascul. Pharmacol. 84, 1–7. doi: 10.1016/j.vph.2016.05.013
Fan, J. S., Liu, D. N., Huang, G., Xu, Z. Z., Jia, Y., Zhang, H. G., et al. (2012). Panax notoginseng saponins attenuate atherosclerosis via reciprocal regulation of lipid metabolism and inflammation by inducing liver X receptor alpha expression. J. Ethnopharmacol. 142, 732–738. doi: 10.1016/j.jep.2012.05.053
Frostegard, J. (2013). Immunity, atherosclerosis and cardiovascular disease. BMC Med. 11:117. doi: 10.1186/1741-7015-11-117
Gaziano, T. A., Bitton, A., Anand, S., Abrahams-Gessel, S., and Murphy, A. (2010). Growing epidemic of coronary heart disease in low- and middle-income countries. Curr. Probl. Cardiol. 35, 72–115. doi: 10.1016/j.cpcardiol.2009.10.002
Geiszt, M., and Leto, T. L. (2004). The nox family of NAD(P)H oxidases: host defense and beyond. J. Biol. Chem. 279, 51715–51718. doi: 10.1074/jbc.R400024200
Guo, Y. B., Li, Y., Xue, L. M., Severino, R. P., Gao, S. H., Niu, J. Z., et al. (2014). Salvia miltiorrhiza: an ancient Chinese herbal medicine as a source for anti-osteoporotic drugs. J. Ethnopharmacol. 155, 1401–1416. doi: 10.1016/j.jep.2014.07.058
Honjo, M., Nakamura, K., Yamashiro, K., Kiryu, J., Tanihara, H., Mcevoy, L. M., et al. (2003). Lectin-like oxidized LDL receptor-1 is a cell-adhesion molecule involved in endotoxin-induced inflammation. Proc. Natl. Acad. Sci. U.S.A. 100, 1274–1279. doi: 10.1073/pnas.0337528100
Hu, C., Dandapat, A., Sun, L., Chen, J., Marwali, M. R., Romeo, F., et al. (2008). LOX-1 deletion decreases collagen accumulation in atherosclerotic plaque in low-density lipoprotein receptor knockout mice fed a high-cholesterol diet. Cardiovasc. Res. 79, 287–293. doi: 10.1093/cvr/cvn110
Hu, J., Zhang, Z. H., Shen, W. J., and Azhar, S. (2010). Cellular cholesterol delivery, intracellular processing and utilization for biosynthesis of steroid hormones. Nutr. Metab. (Lond). 7:47. doi: 10.1186/1743-7075-7-47
Kita, T., Kume, N., Minami, M., Hayashida, K., Murayama, T., Sano, H., et al. (2001). Role of oxidized LDL in atherosclerosis. Ann. N. Y. Acad. Sc. 947, 199–205. doi: 10.1111/j.1749-6632.2001.tb03941.x
Ku, D. N., Giddens, D. P., Zarins, C. K., and Glagov, S. (1985). Pulsatile flow and atherosclerosis in the human carotid bifurcation. Positive correlation between plaque location and low oscillating shear stress. Arteriosclerosis 5, 293–302.
Li, A. C., and Glass, C. K. (2002). The macrophage foam cell as a target for therapeutic intervention. Nat. Med. 8, 1235–1242. doi: 10.1038/nm1102-1235
Li, T., Wang, Y., Liu, C., Hu, Y., Wu, M., Li, J., et al. (2009). MyD88-dependent nuclear factor-kappaB activation is involved in fibrinogen-induced hypertrophic response of cardiomyocytes. J. Hypertens. 27, 1084–1093. doi: 10.1097/HJH.0b013e3283293c93
Liu, Q., Zhang, Y., Lin, Z., Shen, H., Chen, L., Hu, L., et al. (2010). Danshen extract 15,16-dihydrotanshinone I functions as a potential modulator against metabolic syndrome through multi-target pathways. J. Steroid Biochem. Mol. Biol. 120, 155–163. doi: 10.1016/j.jsbmb.2010.03.090
Liu, Z., Xu, S., Huang, X., Wang, J., Gao, S., Li, H., et al. (2015). Cryptotanshinone, an orally bioactive herbal compound from danshen, attenuates atherosclerosis in apolipoprotein E-deficient mice: role of lectin-like oxidized LDL receptor-1 (LOX-1). Br. J. Pharmacol. 172, 5661–5675. doi: 10.1111/bph.13068
Lu, J. J., and Mehta, J. L. (2011). LOX-1: a critical player in the genesis and progression of myocardial ischemia. Cardiovasc. Drugs Ther. 25, 431–440. doi: 10.1007/s10557-011-6329-1
Luo, H., Wang, J., Qiao, C., Ma, N., Liu, D., and Zhang, W. (2015). Pycnogenol attenuates atherosclerosis by regulating lipid metabolism through the TLR4-NF-kappaB pathway. Exp. Mol. Med. 47:e191. doi: 10.1038/emm.2015.74
Martinez-Micaelo, N., Gonzalez-Abuin, N., Terra, X., Richart, C., Ardevol, A., Pinent, M., et al. (2012). Omega-3 docosahexaenoic acid and procyanidins inhibit cyclo-oxygenase activity and attenuate NF-kappaB activation through a p105/p50 regulatory mechanism in macrophage inflammation. Biochem. J. 441, 653–663. doi: 10.1042/BJ20110967
Mehta, J. L., Chen, J., Hermonat, P. L., Romeo, F., and Novelli, G. (2006). Lectin-like, oxidized low-density lipoprotein receptor-1 (LOX-1): a critical player in the development of atherosclerosis and related disorders. Cardiovasc. Res. 69, 36–45. doi: 10.1016/j.cardiores.2005.09.006
Mestas, J., and Ley, K. (2008). Monocyte-endothelial cell interactions in the development of atherosclerosis. Trends Cardiovasc. Med. 18, 228–232. doi: 10.1016/j.tcm.2008.11.004
Moore, K. J., Sheedy, F. J., and Fisher, E. A. (2013). Macrophages in atherosclerosis: a dynamic balance. Nat. Rev. Immunol. 13, 709–721. doi: 10.1038/nri3520
Paigen, B., Holmes, P. A., Mitchell, D., and Albee, D. (1987). Comparison of atherosclerotic lesions and HDL-lipid levels in male, female, and testosterone-treated female mice from strains C57BL/6, BALB/c, and C3H. Atherosclerosis 64, 215–221. doi: 10.1016/0021-9150(87)90249-8
Park, H. S., Chun, J. N., Jung, H. Y., Choi, C., and Bae, Y. S. (2006). Role of NADPH oxidase 4 in lipopolysaccharide-induced proinflammatory responses by human aortic endothelial cells. Cardiovasc. Res. 72, 447–455. doi: 10.1016/j.cardiores.2006.09.012
Patel, D. N., Bailey, S. R., Gresham, J. K., Schuchman, D. B., Shelhamer, J. H., Goldstein, B. J., et al. (2006). TLR4-NOX4-AP-1 signaling mediates lipopolysaccharide-induced CXCR6 expression in human aortic smooth muscle cells. Biochem. Biophys. Res. Commun. 347, 1113–1120. doi: 10.1016/j.bbrc.2006.07.015
Petry, A., Djordjevic, T., Weitnauer, M., Kietzmann, T., Hess, J., and Gorlach, A. (2006). NOX2 and NOX4 mediate proliferative response in endothelial cells. Antioxid. Redox Signal. 8, 1473–1484. doi: 10.1089/ars.2006.8.1473
Quiles, J. L., Mesa, M. D., Ramirez-Tortosa, C. L., Aguilera, C. M., Battino, M., Gil, A., et al. (2002). Curcuma longa extract supplementation reduces oxidative stress and attenuates aortic fatty streak development in rabbits. Arterioscler. Thromb. Vasc. Biol. 22, 1225–1231. doi: 10.1161/01.ATV.0000020676.11586.F2
Ray, R., and Shah, A. M. (2005). NADPH oxidase and endothelial cell function. Clin. Sci. (Lond). 109, 217–226. doi: 10.1042/CS20050067
Sawamura, T., Kume, N., Aoyama, T., Moriwaki, H., Hoshikawa, H., Aiba, Y., et al. (1997). An endothelial receptor for oxidized low-density lipoprotein. Nature 386, 73–77. doi: 10.1038/386073a0
Shahar, E., Chambless, L. E., Rosamond, W. D., Boland, L. L., Ballantyne, C. M., Mcgovern, P. G., et al. (2003). Plasma lipid profile and incident ischemic stroke: the Atherosclerosis Risk in Communities (ARIC) study. Stroke 34, 623–631. doi: 10.1161/01.STR.0000057812.51734.FF
Steinberg, D. (1997). Low density lipoprotein oxidation and its pathobiological significance. J. Biol. Chem. 272, 20963–20966. doi: 10.1074/jbc.272.34.20963
Sun, Y., and Chen, X. (2011). Ox-LDL-induced LOX-1 expression in vascular smooth muscle cells: role of reactive oxygen species. Fundam. Clin. Pharmacol. 25, 572–579. doi: 10.1111/j.1472-8206.2010.00885.x
Tak, P. P., and Firestein, G. S. (2001). NF-kappaB: a key role in inflammatory diseases. J. Clin. Invest. 107, 7–11. doi: 10.1172/JCI11830
Vara, D., and Pula, G. (2014). Reactive oxygen species: physiological roles in the regulation of vascular cells. Curr. Mol. Med. 14, 1103–1125. doi: 10.2174/1566524014666140603114010
Wang, F., Ma, J., Wang, K. S., Mi, C., Lee, J. J., and Jin, X. (2015). Blockade of TNF-alpha-induced NF-kappaB signaling pathway and anti-cancer therapeutic response of dihydrotanshinone I. Int. Immunopharmacol. 28, 764–772. doi: 10.1016/j.intimp.2015.08.003
Xu, S., Little, P. J., Lan, T., Huang, Y., Le, K., Wu, X., et al. (2011). Tanshinone II-A attenuates and stabilizes atherosclerotic plaques in apolipoprotein-E knockout mice fed a high cholesterol diet. Arch. Biochem. Biophys. 515, 72–79. doi: 10.1016/j.abb.2011.08.006
Xu, S., and Liu, P. (2013a). Tanshinone II-A: new perspectives for old remedies. Expert Opin. Ther. Pat. 23, 149–153. doi: 10.1517/13543776.2013.743995
Xu, S., Ogura, S., Chen, J., Little, P. J., Moss, J., and Liu, P. (2013b). LOX-1 in atherosclerosis: biological functions and pharmacological modifiers. Cell. Mol. Life Sci. 70, 2859–2872. doi: 10.1007/s00018-012-1194-z
Yan, M., Mehta, J. L., Zhang, W., and Hu, C. (2011). LOX-1, oxidative stress and inflammation: a novel mechanism for diabetic cardiovascular complications. Cardiovasc. Drugs Ther. 25, 451–459. doi: 10.1007/s10557-011-6342-4
Zeng, Y., Song, J. X., and Shen, X. C. (2012). Herbal remedies supply a novel prospect for the treatment of atherosclerosis: a review of current mechanism studies. Phytother. Res. 26, 159–167. doi: 10.1002/ptr.3587
Zhang, P., Liu, M. C., Cheng, L., Liang, M., Ji, H. L., and Fu, J. (2009). Blockade of LOX-1 prevents endotoxin-induced acute lung inflammation and injury in mice. J. Innate Immun. 1, 358–365. doi: 10.1159/000161070
Zhang, T., Huang, Z. T., Dai, Y., Chen, X. P., Zhu, P., and Du, G. H. (2006). The expression of recombinant human LOX-1 and identifying its mimic ligands by fluorescence polarization-based high throughput screening. J. Biotechnol. 125, 492–502. doi: 10.1016/j.jbiotec.2006.03.040
Keywords: dihydrotanshinone I, lipopolysaccharide, LOX-1, ROS, atherosclerosis
Citation: Zhao W, Li C, Gao H, Wu Q, Shi J and Chen X (2016) Dihydrotanshinone I Attenuates Atherosclerosis in ApoE-Deficient Mice: Role of NOX4/NF-κB Mediated Lectin-Like Oxidized LDL Receptor-1 (LOX-1) of the Endothelium. Front. Pharmacol. 7:418. doi: 10.3389/fphar.2016.00418
Received: 26 July 2016; Accepted: 21 October 2016;
Published: 08 November 2016.
Edited by:
Kalin Yanbo Zhang, University of Hong Kong, Hong KongReviewed by:
Jaya Talreja, Wayne State University School of Medicine, USAKehinde Olugboyega Soetan, University of Ibadan, Nigeria
Junping Kou, China Pharmaceutical University, China
Copyright © 2016 Zhao, Li, Gao, Wu, Shi and Chen. This is an open-access article distributed under the terms of the Creative Commons Attribution License (CC BY). The use, distribution or reproduction in other forums is permitted, provided the original author(s) or licensor are credited and that the original publication in this journal is cited, in accordance with accepted academic practice. No use, distribution or reproduction is permitted which does not comply with these terms.
*Correspondence: Xiuping Chen, eHBjaGVuQHVtYWMubW8=
†Co-first author